- 1Department of Medical Microbiology, Post Graduate Institute of Medical Education and Research (PGIMER), Chandigarh, India
- 2Origin LIFE Healthcare Solutions and Research Center, Chandigarh, India
Introduction: Endometrial cancer is a hormone-dependent malignancy, and sex steroid hormones play a crucial role in its pathogenesis. Recent studies have demonstrated that microRNAs (miRNAs) can regulate the expression of sex steroid hormone receptors and modulate hormone signaling pathways. Our aim is to provide an overview of the current understanding of the role of miRNAs in endometrial cancer regulated by sex steroid hormone pathways.
Methods: A thorough literature search was carried out in the PubMed database. The articles published from 2018 to the present were included. Keywords related to miRNAs, endometrial cancer, and sex steroid hormones were used in the search.
Results: Dysregulation of miRNAs has been linked to abnormal sex steroid hormone signaling and the development of endometrial cancer. Various miRNAs have been identified as modulators of estrogen and progesterone receptor expression, and the miRNA expression profile has been shown to be a predictor of response to hormone therapy. Additionally, specific miRNAs have been implicated in the regulation of genes involved in hormone-related signaling pathways, such as the PI3K/Akt/mTOR and MAPK/ERK pathways.
Conclusion: The regulation of sex steroid hormones by miRNAs is a promising area of research in endometrial cancer. Future studies should focus on elucidating the functional roles of specific miRNAs in sex steroid hormone signaling and identifying novel miRNA targets for hormone therapy in endometrial cancer management.
Introduction
Endometrial cancer (EC), a malignancy in the inner lining of the uterus, is a major contributor to deaths among gynecological malignancies. EC is characterized by abnormal cell growth, uncontrolled cell proliferation, excessive activation of signaling pathways, and microRNA (miRNA) activity (1). The progression and malignant transformation of EC are linked to central cellular processes such as epithelial-to-mesenchymal transition (EMT) and invasion (2). However, the pathogenesis of EC is complex and multi-factorial, and not fully understood. Studies have explored the influence of various factors in EC, such as estrogen receptor alpha (ER-α) activation of the MAPK signaling pathway (3) and the molecular responses in the endometrium to hormonal stimuli (4). Despite the growing use of molecular techniques in the study of gynecologic malignancies, the underlying molecular mechanisms and pathology remain elusive (5). There are concerns regarding the current guidelines pertaining to the diagnosis, prognosis, and treatment of EC, which are deemed inadequate (6). This highlights the need to explore innovative therapeutic strategies for this common female malignancy.
The role of non-coding RNAs (ncRNAs), such as miRNAs and long non-coding RNAs (lncRNA), in cancer diagnosis, prognosis, and therapy selection is gaining recognition in the scientific community. These ncRNAs play a key role in regulating cellular metabolism and transforming cells into cancer cells (7). Recent studies have identified miRNAs as potential therapeutic targets for EC (8). This review focuses on the role of miRNAs in diagnosing and treating EC, a type of cancer that primarily affects younger and post-menopausal women and is a major health concern. To highlight the most recent advances and gaps in this research area, articles since 2018 are included. This review examines various targets and pathways that may contribute to the development of EC by regulating miRNAs.
Endometrial cancer
EC is the most generally diagnosed form of gynecological cancer in developed nations, making up approximately 5% of all cancers in women (9). EC originates in the endometrium, the inner lining of the uterus. It is most commonly found in post-menopausal women. EC has witnessed a marked surge in recent times, however, the underlying reason behind this trend remains elusive. EC is ranked as the fourth most common cancer in high-income countries and the most prevalent gynecological cancer globally, with 382,069 new cases reported in 2018 (10). In 2020, there were around 417,000 new cases globally and 97,300 deaths from EC, as per statistics (11).
Although, the exact causes of EC are unknown; lifestyle changes, obesity, infertility, hormone replacement therapy, and diabetes are believed to play a role. Advanced disease increases the likelihood of recurrence, and established risk factors include obesity and high estrogen exposure (12). EC is divided into type I (endometrioid) and type II (non-endometrioid). Type-I ECs are estrogen-dependent and have a good prognosis, while type II cancers are nonestrogen-dependent and have a more aggressive clinical course with a poor prognosis (13). However, recent methodologies have challenged this dualistic classification of EC (14). Further, it is found that Type I tumors have downregulation or mutations of the PTEN gene, while type II tumors have high expression of non-functional tumor proteins (7).
A confluence of several factors is believed to increase the likelihood of EC. Risk factors, such as obesity, diabetes, a family history of cancer, and Hormone Replacement Therapy (HRT), play a significant role in EC (15–17). Lifestyle factors like diet, physical activity, and smoking may also contribute to the development of EC (15, 16, 18–20). Additionally, certain medical conditions, such as polycystic ovary syndrome (PCOS), diabetes, and Lynch syndrome, may increase the risk of EC (21). EC is associated with abnormal production of hormones, inflammation, and hyperinsulinemia (22). The use of oral contraceptives reduces EC risk by 30-40% (23, 24). Coffee consumption, use of antidepressants, and male-origin microchimerism are linked to a reduced risk of EC (25–27), while the correlation between aspirin use and EC is inconclusive (28, 29). Smoking is inversely related to endometrial cancer, but the evidence does not support this relationship (18). The prognosis for early EC is generally good, but 7% of cases recur, and the 3-year survival rate is relatively low (30). This emphasizes the importance of conducting additional research into the molecular mechanisms of EC (such as miRNAs) for better prognosis and cancer prevention.
MicroRNA
MicroRNAs or miRNAs or miRs are non-coding RNA molecules that play a crucial role in gene regulation, impacting cellular functions and associated with various pathological conditions, including EC. Their altered expression levels have been linked to prognostic factors and significantly impact tumorigenesis and progression in EC (31, 32). miRNAs have the potential to serve as non-invasive biomarkers for EC diagnosis by distinguishing between different conditions, such as endometrial hyperplasia (33, 34). They can regulate tumor suppressor genes and predict future EC development based on the patient’s immune microenvironment and insulin status (35, 36). miRNAs impact cell proliferation, differentiation, and apoptosis, and a higher accuracy miRNA signature can be obtained using miRNA clusters, serum type, and larger sample sizes (4, 37–39).
miRNAs can also act as oncogenes or tumor suppressors (40). The specific molecular characterization of miRNAs, involved in the regulation of the MAPK signaling pathway, has been shown to discriminate endometrioid endometrial carcinoma (EEC) from serous endometrial carcinoma (SEC) (41). An analysis of miRNA arrays revealed 20 miRNAs that differed significantly between patients with endometrial hyperplasia (EH) and those with simple hyperplasia/complex hyperplasia-non-atypical (SH/CH-nonA) (42). These findings highlight the important role of miRNAs in the regulation of gene expression and the pathophysiology of EC.
Biogenesis of miRNA
miRNAs are small RNA molecules that play important roles in development, cell differentiation, and homeostasis. miRNAs are often present in tandem with multiple miRNAs in intergenic and intragenic genomic regions and are transcribed by RNA polymerase II as a single transcript (Figure 1). The RNase III enzyme Dicer processes them in the cytoplasm to produce mature double-stranded miRNAs. The processing of miRNAs can result in multiple sequence variants known as isomiRs. These isomiRs can form stem-loop structures and are cleaved by the RNase III enzymes Drosha and Dicer to generate precursor miRs and mature miRs. The primary precursor miRNA (primiRNA) is then transported from the nucleus to the cytoplasm by Exportin 5 and processed by Dicer to produce mature double-stranded miRNAs (43). These mature miRNAs are incorporated into the RNA-induced silencing complex (RISC) and target complementary sequences in the 3′ untranslated region (UTR) of mRNAs. By destabilizing target mRNAs, miRNAs reduce protein output and change gene expression (Figure 1) (44).
Role of sex steroid hormones in endometrial cancer
Ovarian hormones, such as progesterone and estrogen, play a crucial role in regulating the endometrium and its monthly cycle. These hormones are responsible for the endometrium’s monthly proliferation, differentiation, and shedding and are the source of EC (45). The presence of Estrogen Receptor (ER) and Progesterone Receptor (PR) is linked to clinicopathological variables and can affect the prognosis of patients with EC. Steroid receptors ER and PR, activated by estrogen and progesterone, respectively, are critical in developing endometrioid-type EC (46, 47). These hormones are a key factor in EC occurrence and development, with 75-90% of EC incidents being dependent on estrogen (11). Estrogen exposure can result in endometrial hyperplasia and EC, while prolonged use of estrogens is associated with type 1 endometrioid malignancies (48). On the other hand, progesterone acts as an antagonist, inhibiting cell division, downregulating ER, and promoting cell differentiation (49). Overexposure to estrogen is a high-risk factor for EC, particularly for women undergoing estrogen-only hormonal therapy, using tamoxifen as an adjunct therapy, dealing with obesity, or suffering from PCOS. On the other hand, the progesterone receptor is a favorable prognostic marker for multiple solid tumors, but its expression is reduced in malignant tumors (50). The relationship between unopposed estrogen and EC is unclear, and progesterone can be used as a targeted therapy (51). Progesterone is used for treating young endometrial cancer patients, and the long non-coding RNA HOTAIR is a potential predictor for progesterone response. The expression of HOTAIR is regulated by LSD1, and inhibiting LSD1 can induce apoptosis (52). The use of oral contraceptives (OCs) and progestin-only contraceptives (POCs) has been linked to an increased risk of cancer (53, 54).
The loss of estrogen and progesterone receptors is associated with a higher rate of an advanced stage of endometrial cancer and increased expression of certain genes (55, 56). An imbalanced hormone state can arise from variations in the manifestation of genes and enzymes involved in the signaling of steroid hormones. Ovarian hormones regulate gene transcription by binding to DNA and initiating signaling pathways. It has been found that estrogen and progesterone are significant predictors of EC and that ER and PR are involved in genomic processes in cancer cells (57). Altered expression of hormone-regulated genes has been linked to a role for PAX2 in fine-tuning the interplay between ER alpha and PR (57, 58). Inhibiting ERRα/TGF-β can suppress in vitro invasion of EC cells, and ER signaling increases the expression of the oncogene PIWIL1 in ER-positive endometrial cancer cells (59, 60). Tumor suppressor genes such as CACNA2D3 are associated with EC and can exert a tumor-suppressive effect in vitro (3, 61). A novel signature of five genes has been developed to improve risk assessment and provide potential targets for EC therapies (11). Work is also being done to develop biomarkers for endometriosis, including the examination of PDCD4 and its regulation by progesterone (62). ER-positive endometrioid endometrial cancer (EEC) exhibits the highest rate of alterations in PTEN, while ARID1A is a common mutation in ER-negative EEC (63, 64). DNA methylation and hydroxymethylation also regulate gene expression and are associated with steroid receptors, enzymes involved in estrogen synthesis, and TET proteins that mediate hydroxymethylation (65).
The role of steroid hormones in EC mediated by insulin have also been investigated and findings showed the increased expression and phosphorylation of Akt, MAPK, and ERK in EC cells (66). Other factors include the regulation of transcription factors by cell adhesion, biomarkers in blood for hormone quantification, and intra-tumoral heterogeneity (67, 68). The immune system component NLRP3 inflammasome and the gene BCL11A have also been linked to EC progression, ERR, USP14, and estrogen (69–71). Additionally, the expression of the hormone receptors can also be used to predict lymph node involvement. A study found that high expression of ER has been associated with poor prognosis in endometrial tumors, and patients with ER/PR loss have deeper myometrial infiltration and higher rates of pelvic lymph node metastasis (72). One study found that decreased expression of combined ER/PR (estrogen receptor/progesterone receptor) was associated with a poorer outcome in endometrial cancer patients, but hormone receptor status alone did not significantly improve mortality prediction (73).
Variation in Hormone replacement therapy (HRT) type and the BMI status of the women is associated with an increased risk of EC (74). Obesity is a well-known risk factor for EC, but the mechanisms of obesity-related carcinogenesis are not well-defined and may vary based on the presence of Lynch syndrome (75). Hormonal therapy is considered best for low-grade disease and hormone receptor positivity, and it is better tolerated than chemotherapy (76). Another study found that oral estradiol plus vaginal progesterone therapy affected endometrial thickness, biopsy pathology, and cancer incidence in post-menopausal women (77). A study on the relationship of menopausal hormone therapy and EC found that patients with hormone therapy had lower BMIs, less diabetes, and fewer recurrences, but age and tumor stage had a bigger impact on overall survival (78). Prognostic factors such as the expression of ER, PR, and HER2 are also being assessed to improve patient outcomes (79). The cutoff values for the positivity of ER/PR in endometrial cancer are still being debated, and the expression of these receptors and other proteins are evaluated to determine their impact on EC prognosis (80).
The recurrence of EC is one of the major problem in its diagnosis and treatment. Researchers explored the role of sex hormones and insulin/insulin-like growth factor axis signaling in endometrial cancer recurrence. It was found that circulating estradiol and tumor tissue phosphorylated IGR1R/IR were associated with a higher risk of recurrence (12). A recurrence prediction model was created to anticipate the likelihood of endometrial cancer reoccurrence in stage I-II patients after surgical treatment. The final prediction model incorporated factors such as age, adjuvant treatment, histologic type, and expression levels of Ki67, ER, PR, and WFDC2 and showed adequate discrimination power (81).
miRNA and EC
Multiple cancers can be identified and differentiated through miRNAs, serving as single biomarkers to diagnose and treat various gynecological cancers such as endometrial, breast, and ovarian cancer (82). miRNAs play important roles in pathological processes, indirectly regulating cell proliferation, differentiation, and apoptosis (35, 38, 39). The expression pattern of miRNAs may vary in EC (Table 1). Abnormal expression of miRNAs plays a critical role in tumorigenesis and progression in EC via significantly impacting PTEN levels (99). miRNAs can distinguish between different subtypes of EC, including endometrial hyperplasia, and have been shown to discriminate EEC from SEC (41).
DNA methylation is a common phenomenon in cancer and suppresses the expression of miRNAs (60). Hypermethylation of miRNAs has been found in EC, and treatment with epigenetic inhibitors has been found to reduce cancer cell proliferation (178). The M6A RNA methylation modification is a pan-prognostic regulator of uterine cancer, with IGF2BP1 being the most important regulator (179). miRNAs influence the expression of tumor suppressor genes, and studies have shown that miRNAs related to the patient’s immune microenvironment and insulin status can predict the future development of EC (36). miRNAs have the potential to serve as novel, non-invasive biomarkers for EC diagnosis, with a miRNA signature that provides higher accuracy obtained by using miRNA clusters, serum type, and large sample sizes (4, 33, 37).
Multiple studies have found that miRNAs present in cluster significantly predict the prognosis and overall survival of EC patients. The miR-503 cluster, composed of six miRNAs, has been linked to endometrial endometrioid adenocarcinoma (EEA) (180). A nomogram based on age, clinical stage, and risk score calculated using a 4-miRNA signature showed high accuracy in predicting the overall survival of EC patients (181). A panel of 11 miRNAs effectively differentiated between ovarian and uterine serous carcinoma with 91.5% accuracy (182), and a 6-microRNA expression signature was established as a predictor for EC patient survival (183). A study found 4 miRNAs to be predictive biomarkers of overall survival among 561 differentially expressed miRNAs (184). A 26-miRNA signature was established as a crucial biomarker in predicting immunotherapy response (185), and a role was found for 21 miRNAs in predicting tumor mutation burden levels and regulating immune checkpoint expression (36).
Multiple miRNAs have also been evaluated as potential biomarkers for EC diagnosis in various biological samples such as urine, plasma/serum, exosomes, and endometrium. Urinary miRNAs, such as miR-205, -223, -182, -183, and -200a, have been up-regulated in EC patients, and their expression levels have the potential as a diagnostic test (93, 186). A six-miRNA signature in plasma has also shown superior performance in diagnosing EC (96). Exosomes derived from various sources, such as cancer-associated fibroblasts, tumor-associated macrophages, and tumor-infiltrating CD8+ T cells, hold the potential for EC diagnosis and treatment. Exosomes have unique miRNA expression profiles, with certain miRNAs, such as miR-148b and miR-320a, playing a role in cancer progression and others, such as miR-15a-5p and miR-192-5p, having the ability to distinguish EC patients from healthy subjects (104, 127, 165, 187).
The role of miRNA in stem cell growth has been demonstrated through in vitro analysis of human endometrial carcinoma stem cells (HuECSCs). It is shown that overexpression of miR-326 in HuECSCs significantly suppressed cell proliferation and progression through the cell cycle (98). miRNAs have been found to regulate genes associated with sensitivity to various anticancer drugs in EC. For example, MiR-98 and NEAT1 reduce drug resistance caused by MRP-7, an ATP-binding cassette transporter (102). MiR-135a is a potential predictor for EC chemotherapy response and prognosis (188). miRNA-29b has demonstrated the ability to inhibit cell proliferation and increase apoptosis in endometriosis cells (31). Overexpression of miR-365 has been found to enhance chemosensitivity to paclitaxel and mitigate cell migration and invasion (159). miRNA regulation in combination with anticancer drugs is a proposed treatment option to reduce EC expression (172). Therefore, miRNAs can be potential targets for future chemotherapy agents or cancer-specific biomarkers, as they are commonly involved in the development of various cancers, including breast, cervical, endometrial, ovarian, and vulvar cancer (189).
Most miRNA analysis in endometrial cancer is performed using qRT-PCR (96). Recently, TWAS has been proposed for identifying important miRNAs in disease (190). EC was also best predicted when PTEN loss and miR-200a were combined (42). Additionally, it has been demonstrated that a miRNA expression-based risk score is a standalone factor for forecasting the overall survival of EC patients (191). miRNA also exhibits differences in expression levels between different ethnic groups for EC. For example, miR-145 and miR-143 were found to be overexpressed in the Uygur group and downregulated in the Han group (192). Meanwhile, the upregulation of miR-223 was found to be a risk factor for death among African American and Caucasian patients with serous uterine carcinoma (94). Additionally, there were gender-specific differences in the interplay between cancer cells and the tumor microenvironment (193).
Recent research highlights the crucial role of the regulatory network between lncRNA-miRNA-mRNA (ceRNAs) in understanding the development of EC. This network identifies 169 prognosis-associated RNAs, including 92 lncRNAs, 16 miRNAs, and 61 mRNAs (194). Several genes, such as ADRA1A, ANGPTL1, FBXO32, KCNMA1, DCN, LEFTY1, LIN28A, LHX3, ST8SIA3, and CEP55, are also regulated by the lncRNA-miRNA-mRNA network (195). Moreover, miRNA-mRNA regulatory networks are linked to the biological process of negative regulation of transcription from the RNA polymerase II promoter and positive regulation from the RNAD promoter (166). Additionally, these networks have prognostic value, as low expression of some miRNA targets, such as LEF1 and NKD1, predicts advanced clinical stages and poor prognosis in EC patients (196). Furthermore, specific ceRNAs have been identified as modulators of the PI3K/AKT signaling pathway, which may suppress the growth and proliferation of tumor cells and could be potential targets for future treatments (170). Identification of 26 miRNAs and 66 target genes involved in various signaling pathways in endometrial tissue biopsies, providing potential targets for molecularly targeted EC therapies (83, 197). Changes in mRNAs and miRNAs also influenced the histaminergic system in endometrioid tissue, where miRNA 27a showed promise as a diagnostic biomarker for EC (100, 197). A review of 106 miRNAs found that miR-145 was the best predictor of EC, and it is widely recognized that miRNAs play important roles in pathological processes by functioning as either oncogenes or tumor suppressors (40, 198). Studies have explored the role of miRNAs in regulating the expression of tumor-related genes and showed that miRNAs could act as oncogenic or tumor suppressors (97, 113, 172, 199).
EC and tumor suppressor miRNA
Several miRNAs have been identified to be involved in the development and progression of EC, and it is established that miRNAs function as either oncogenes or tumor suppressors (Figure 2). The expression of miR-34a, miR-142, and miR-125b has been found to significantly decrease in endometrioid carcinomas with high proliferation, while miR-327, miR-202, and miR-214-3p have been shown to act as tumor suppressors in EC cells (124–126, 134). Downregulation of miR-23b, miR-125b-5p, miR-199a-3p, miR-221-3p, and miR-451a has been associated with EC (138). On the other hand, miR-652, miR-495, miR-1271, miR-381, and miR-29b have been identified as oncogenic miRNAs in EC (105, 154–156, 160). Furthermore, miR-15a-5p and miR-589-5p were tumor suppressors in EC cells (161, 164).
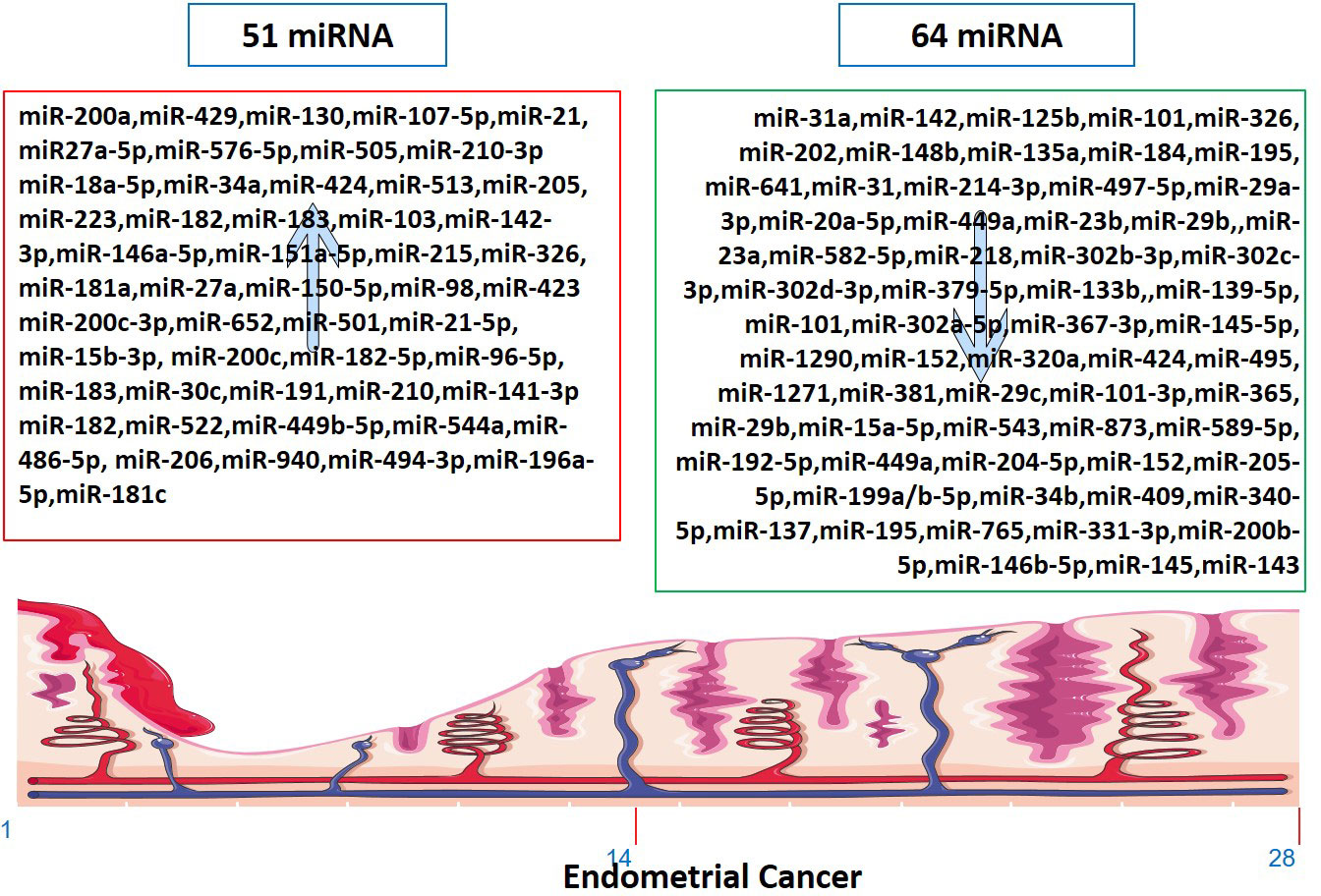
Figure 2 Up-regulated and down-regulated miRNAs in Endometrial Cancer. The markings 1, 14 and 28 in the bottom of endometrium, depicts the days of menstrual cycle.
In cell lines studies, overexpression of miR-641, miR-184, miR-302a-5p/367-3p, miR-152, and knockdown of miR1290 and miR-873 had inhibitory effects on EC cell viability and proliferation (132, 146, 148, 149, 163). MiR-135a downregulation and miR-320a/miR-424 expression decreased EC cell proliferation and migration (129, 153). MiR-29a-3p inhibited EC cell proliferation, migration, and invasion by targeting VEGFA/CDC42/PAK1 signaling (135). MMSET played a pro-metastatic role in EC cell lines and tissues by repressing miR-34a, miR-424, and miR-513 (92). Downregulation of miRs-29-a, -b, and -c inhibited MCL1 apoptosis regulator BCL2 family member, MDM2 proto-oncogene, serum/glucocorticoid regulated kinase 1, sirtuin 1, and vascular endothelial growth factor A in HEC1A cells (157). MTA1 promoted cell proliferation, migration, and invasion abilities of EC cell lines and reversed the negative effect of miR-30c on EC cells (112). These findings provide insights into the potential roles of miRNAs as therapeutic targets in EC.
EC and tumor-causing miRNA
As tumor-causing factors, several miRNAs have been identified as potential biomarkers and regulators of EC (Figure 2). miRNAs such as miR-130, miR-200a, miR-429, miR-107 5p, miR-21, miR-125b, miR-101, miR-27a-5p, miR-576-5p, miR-210-3p, miR-1903p, miR-18a-5p, miR-103, miR 215, miR-27a, miR-34a-5p, miR-146-5p, miR-423, and miR-302 have been shown to play different roles in promoting or suppressing EC progression by targeting various genes and signaling pathways (83–87, 90, 91, 95, 97, 101, 103, 124, 138, 200). These findings provide potential targets for diagnosis, prognosis, and therapeutic interventions in EC. miRNAs have been determined to impact the initiation and advancement of EC substantially. The expression of KLF2 in endometrial cancer cells is promoted by miR-15b-3p, while miR-210 negatively regulates NIFX expression. MiR-940 and miR-494-3p are overexpressed in EC tissues and cell lines, with MRVI1 and PTEN being identified as potential targets, respectively (114, 121, 122, 161). These miRNAs promote the proliferation, migration, and invasion of EC cells.
miRNAs also play a crucial role in regulating EC associated with lymph node metastasis. Downregulation of miRNA-184 is linked to lymph node involvement in low-risk EC patients (201). Overexpression of miR-501 can increase the activation of the AKT/mTOR pathway, leading to higher pelvic lymph node metastasis and reduced overall survival (106). Profiling the miRNA expression in EEC metastatic loci from lymph nodes could aid in identifying novel diagnostic markers and therapeutic targets (202). miR-204-5p is a tumor-suppressor miRNA associated with lymph node metastasis in EC, highlighting the importance of the lymph node status in determining adjuvant treatment (168). Finally, the expression of miR-15a-5p in EC is also correlated with lymph node metastasis, TNM stage, and mortality, and it was significantly reduced in EC patients who experienced recurrence or metastasis (203).
miRNA and sex steroid hormone
Estrogen interacts with ER and GPER1 to control gene transcription and support cancer cell pathways. miRNAs negatively regulate gene expression and interact with estrogen in a complex way (34). MiR-205, -146a, and -1260b discriminate more than 90% of endometrial hyperplasia with atypia and endometrial intraepithelial neoplasia that progress to type I cancer (34). A worse prognosis of type II EC can be predicted by mRNAs linked to Wnt-signaling (196). MiR-22 and miR-206 target ER to prevent the progression of EC (198). miR-195 prevents EC invasion, migration, and EMT in vitro by targeting GPER1. On the other hand, miR-107-5p, miR-222-3p, and miR-205 promote tumor growth and migration in vivo as oncomiRNAs (198). Estrogen-regulated miRNA expression is specific to the cell and tissue and cannot be generalized to other species or tissues (4).
Sex steroid hormones regulated miRNA
In vitro exposure of human endometrial stromal cells to estradiol increased the expression of miR-181b and let-7e while decreasing the expression of miR-27b (4). In EEC cell lines, estrogen treatment improved miR-200c and promoted miR-196a-5p expression, which targeted FOXO1 and increased cell viability (8, 204). On the other hand, progesterone inhibited cell-cycle and cell-viability in EC by regulating NEAT1/miR-146b-5p axis via Wnt/β-catenin signaling. It also induces miR-145/miR-143 to inhibit EC by targeting cyclin D2 and promoting miR-133a to increase endometrial cell proliferation (176, 177). It is also found that endometrial expression of miR-30b, miR-125b, miR-424, and miR-451 was lower in women with high blood progesterone levels compared to those with low blood progesterone levels (4). Moreover, miR-195 functioned as a tumor suppressor that targeted GPER1 to inhibit EC through PI3K/AKT signaling (131).
miRNA regulation of sex steroid hormones
A number of miRNAs have been discovered to control the production of estrogen and progesterone receptors in endometrial cells (Figure 3). For example, miR-107-5p, which promotes tumor proliferation and invasion by targeting ERα, and miR-194-3p and miR-196a, which regulate the expression of PR-A and PR-B proteins (4, 85). Additionally, miR-181c affects estrogen-dependent EC cell growth by targeting PTEN, and miR-92a increases stromal endometrial cell proliferation and progesterone resistance (4, 123). However, the exact mechanisms by which miRNAs regulate ER in the endometrium are not yet fully understood (4). Although, in Figure 4, the possible mechanism by which sex steroid hormones can cause/suppress EC is depicted.
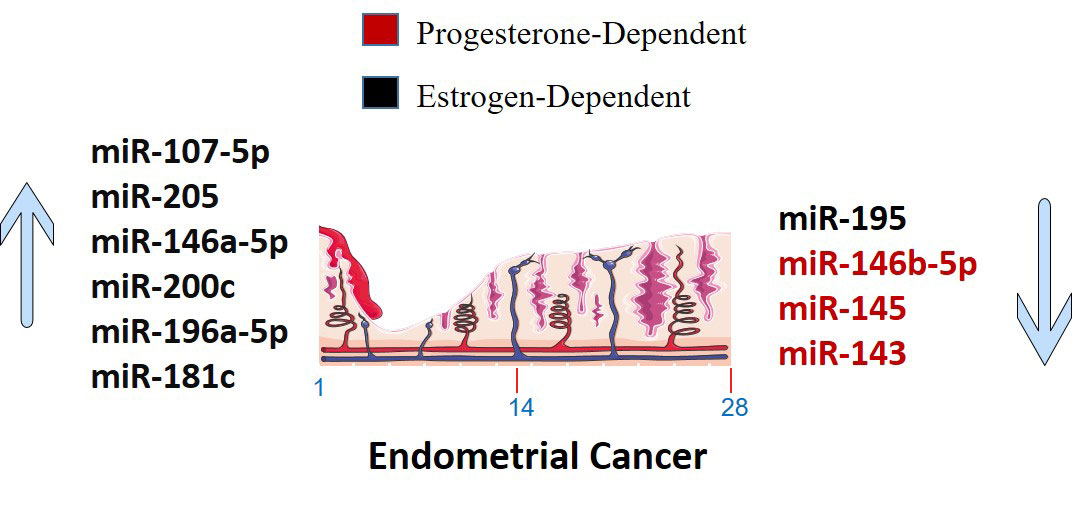
Figure 3 Sex steroid hormones dependent up-regulated and down-regulated miRNAs in Endometrial Cancer. The markings 1, 14 and 28 in the bottom of endometrium, depicts the days of menstrual cycle.
Future directions
The regulation of sex steroid hormones by miRNAs or vice versa holds great promise for the future of EC research. The study of these interactions has the potential to uncover new mechanisms involved in the development and progression of EC, as well as provide new targets for therapeutic intervention. One area of future research could be to understand further the specific miRNAs involved in regulating sex steroid hormones in EC. This could be accomplished through large-scale sequencing studies or microarray analysis to identify miRNAs differentially expressed in EC compared to normal tissue. Additionally, functional studies could assess the effects of inhibiting or overexpressing specific miRNAs on hormone levels and EC cell proliferation. Another area of focus could be investigating the effects of miRNA-mediated regulation of hormones in EC under various conditions, such as in response to different treatments or stages of the disease. This information would be valuable in understanding the changing nature of miRNA control over hormones in EC and its potential as a therapeutic target. In conclusion, regulating sex steroid hormones by miRNAs is a highly promising area of research with numerous potential avenues for future exploration. As the field advances, we can expect a better understanding of the underlying mechanisms of EC and the development of new, more effective treatments for EC patients.
Conclusion
miRNAs are tiny, non-coding RNA molecules that play crucial roles in regulating gene expression, including the regulation of hormones such as estrogen and progesterone. In endometrial cancer, imbalanced sex hormone levels contribute to tumor development and growth, making the study of miRNA-mediated regulation of these hormones a valuable avenue for research. Understanding the dual effect of miRNAs, i.e., the oncogenic and tumor suppressor effects, is warranted in EC. This knowledge can potentially lead to improved diagnosis and treatment options for EC patients.
Author contributions
LT was responsible for conducting the literature review, collecting and analyzing data, and writing the first draft of the manuscript. ST contributed to the literature search, conceptualization and design of the study, provided critical feedback on the manuscript, and assisted with the editing and finalization of the manuscript. All authors contributed to the article and approved the submitted version.
Acknowledgments
The authors express their gratitude to Origin LIFE healthcare solutions and research center’s team for their support in conducting this study.
Conflict of interest
The authors declare that the research was conducted in the absence of any commercial or financial relationships that could be construed as a potential conflict of interest.
Publisher’s note
All claims expressed in this article are solely those of the authors and do not necessarily represent those of their affiliated organizations, or those of the publisher, the editors and the reviewers. Any product that may be evaluated in this article, or claim that may be made by its manufacturer, is not guaranteed or endorsed by the publisher.
References
1. Hermyt E, Zmarzły N, Grabarek B, Kruszniewska-Rajs C, Gola J, Jęda-Golonka A, et al. Interplay between miRNAs and genes associated with cell proliferation in endometrial cancer. Int J Mol Sci (2019) 20(23):6011. doi: 10.3390/ijms20236011
2. Huang Y, Yang N. MicroRNA-20a-5p inhibits epithelial to mesenchymal transition and invasion of endometrial cancer cells by targeting STAT3. Int J Clin Exp Pathol (2018) 1112:5715–24.
3. Liu A, Zhang D, Yang X, Song Y. Estrogen receptor alpha activates MAPK signaling pathway to promote the development of endometrial cancer. J Cell Biochem (2019) 12010:17593–601. doi: 10.1002/jcb.29027
4. Kolanska K, Bendifallah S, Canlorbe G, Mekinian A, Touboul C, Aractingi S, et al. Role of miRNAs in normal endometrium and in endometrial disorders: comprehensive review. J Clin Med (2021) 10(16):3457. doi: 10.3390/jcm10163457
5. Dwivedi SKD, Rao G, Dey A, Mukherjee P, Wren JD, Bhattacharya R. Small non-Coding-RNA in gynecological malignancies. Cancers (Basel) (2021) 13(5):1085. doi: 10.3390/cancers13051085
6. Fridrichova I, Kalinkova L, Karhanek M, Smolkova B, Machalekova K, Wachsmannova L, et al. miR-497-5p decreased expression associated with high-risk endometrial cancer. Int J Mol Sci (2020) 22(1):127. doi: 10.3390/ijms22010127
7. Vallone C, Rigon G, Gulia C, Baffa A, Votino R, Morosetti G, et al. Non-coding RNAs and endometrial cancer. Genes (Basel) (2018) 9(4):187. doi: 10.3390/genes9040187
8. Zhu Y, Tang Y, Fan Y, Wu D. MiR-196a-5p facilitates progression of estrogen-dependent endometrial cancer by regulating FOXO1. Histol Histopathol (2022), 18572. doi: 10.14670/hh-18-572
9. Contreras N-A, Sabadell J, Verdaguer P, Julià C, Fernández-Montolí M-E. Fertility-sparing approaches in atypical endometrial hyperplasia and endometrial cancer patients: current evidence and future directions. Int J Mol Sci (2022) 235:2531. doi: 10.3390/ijms23052531
10. Bray F, Ferlay J, Soerjomataram I, Siegel RL, Torre LA, Jemal A. Global cancer statistics 2018: GLOBOCAN estimates of incidence and mortality worldwide for 36 cancers in 185 countries. CA: Cancer J Clin (2018) 686:394–424. doi: 10.3322/caac.21492
11. Yu J, Yao H-W, Liu T-T, Wang D, Shi J-H, Yuan G-W, et al. Comprehensive analysis and experimental validation of a novel Estrogen/Progesterone-related prognostic signature for endometrial cancer. J Personalized Med (2022) 126:914. doi: 10.3390/jpm12060914
12. Merritt MA, Strickler HD, Hutson AD, Einstein MH, Rohan TE, Xue X, et al. Sex hormones, insulin, and insulin-like growth factors in recurrence of high-stage endometrial cancer. Cancer Epidemiol Biomarkers Prev (2021) 304:719–26. doi: 10.1158/1055-9965.Epi-20-1613
13. O’Mara TA, Glubb DM, Kho PF, Thompson DJ, Spurdle AB. Genome-wide association studies of endometrial cancer: latest developments and future directions. Cancer Epidemiol Biomarkers Prev (2019) 287:1095–102. doi: 10.1158/1055-9965.EPI-18-1031
14. Masood M, Singh N. Endometrial carcinoma: changes to classification (WHO 2020). Diagn Histopatholo (2021) 2712:493–9. doi: 10.1016/j.mpdhp.2021.09.003
15. Hartman TJ, McCullough ML, Hodge JM, Gaudet MM, Wang Y, Gapstur SM. Dietary energy density, glycemic load, glycemic index, and risk for endometrial cancer in the CPS-II nutrition cohort. Cancer Epidemiol Biomarkers Prev (2018) 271:113–5. doi: 10.1158/1055-9965.EPI-17-0964
16. Saint-Maurice PF, Sampson JN, Michels KA, Moore SC, Loftfield E, McClain K, et al. Physical activity from adolescence through midlife and associations with obesity and endometrial cancer risk. Cancer Epidemiol Biomarkers Prev (2021) 304:807. doi: 10.1158/1055-9965.EPI-21-0216
17. Kliemann N, Ammar RO, Biessy C, Gicquiau A, Katzke V, Kaaks R, et al. Metabolically defined body size phenotypes and risk of endometrial cancer in the European prospective investigation into cancer and nutrition (EPIC). Cancer Epidemiol Biomarkers Prev (2022) 31(7):1359–67. doi: 10.1158/1055-9965.EPI-22-0160
18. Dimou N, Omiyale W, Biessy C, Viallon V, Kaaks R, O’Mara TA, et al. Cigarette smoking and endometrial cancer risk: observational and mendelian randomization analyses. Cancer Epidemiol Biomarkers Prev (2022) 31(9):1839–48. doi: 10.1158/1055-9965.EPI-21-1176
19. Lu J, Trabert B, Liao LM, Pfeiffer RM, Michels KA. Dietary intake of nutrients involved in folate-mediated one-carbon metabolism and risk for endometrial cancer. Int J Epidemiol (2019) 482:474–88. doi: 10.1093/ije/dyy270
20. Miyata H, Shirai K, Muraki I, Iso H, Tamakoshi A. Associations of body mass index, weight change, physical activity, and sedentary behavior with endometrial cancer risk among Japanese women: the Japan collaborative cohort study. J Epidemiol (2021) 3112:621–7. doi: 10.2188/jea.JE20200145
21. Sun P, Shen Y, Wang T, He Y, Zhang Y, Tian W, et al. Distinct clinical and genetic mutation characteristics in sporadic and lynch syndrome-associated endometrial cancer in a Chinese population. Cancer Epidemiol (2021) 73:101934. doi: 10.1016/j.canep.2021.101934
22. Dashti SG, English DR, Simpson JA, Karahalios A, Moreno-Betancur M, Biessy C, et al. Adiposity and endometrial cancer risk in postmenopausal women: a sequential causal mediation analysis. Cancer Epidemiol Biomarkers Prev (2021) 301:104–13. doi: 10.1158/1055-9965.EPI-20-0965
23. Burchardt NA, Shafrir AL, Kaaks R, Tworoger SS, Fortner RT. Oral contraceptive use by formulation and endometrial cancer risk among women born in 1947–1964: the nurses’ health study II, a prospective cohort study. Eur J Epidemiol (2021) 368:827–39. doi: 10.1007/s10654-020-00705-5
24. Sponholtz TR, Palmer JR, Rosenberg LA, Hatch EE, Adams-Campbell LL, Wise LA. Exogenous hormone use and endometrial cancer in U.S. black women. Cancer Epidemiol Biomarkers Prev (2018) 275:558–65. doi: 10.1158/1055-9965.EPI-17-0722
25. Crous-Bou M, Du M, Gunter MJ, Setiawan VW, Schouten LJ, Shu X-O, et al. Coffee consumption and risk of endometrial cancer: a pooled analysis of individual participant data in the epidemiology of endometrial cancer consortium (E2C2). Am J Clin Nutr (2022) 1165:1219–28. doi: 10.1093/ajcn/nqac229
26. Sperling CD, Aalborg GL, Dehlendorff C, Friis S, Mørch LS, Kjaer SK. Use of antidepressants and endometrial-cancer risk: a nationwide nested case-control study. Int J Epidemiol (2022) 513:799–806. doi: 10.1093/ije/dyab200
27. Hallum S, Petersen GL, Jakobsen MA, Pinborg A, Kuhlmann C, Tjønneland A, et al. Male-Origin microchimerism and endometrial cancer: a prospective case-cohort study. Cancer Epidemiol (2022) 79:102169. doi: 10.1016/j.canep.2022.102169
28. Sperling CD, Verdoodt F, Aalborg GL, Dehlendorff C, Friis S, Kjaer SK. Low-dose aspirin use and endometrial cancer mortality–a Danish nationwide cohort study. Int J Epidemiol (2020) 491:330–7. doi: 10.1093/ije/dyz253
29. Webb PM, Na R, Weiderpass E, Adami HO, Anderson KE, Bertrand KA, et al. Use of aspirin, other nonsteroidal anti-inflammatory drugs and acetaminophen and risk of endometrial cancer: the epidemiology of endometrial cancer consortium. Ann Oncol (2019) 302:310–6. doi: 10.1093/annonc/mdy541
30. Zhang W, Chen JH, Shan T, Aguilera-Barrantes I, Wang LS, Huang TH, et al. miR-137 is a tumor suppressor in endometrial cancer and is repressed by DNA hypermethylation. Lab Invest (2018) 9811:1397–407. doi: 10.1038/s41374-018-0092-x
31. Kong J, He X, Wang Y, Li J. Effect of microRNA-29b on proliferation, migration, and invasion of endometrial cancer cells. J Int Med Res (2019) 478:3803–17. doi: 10.1177/0300060519844403
32. Delangle R, De Foucher T, Larsen AK, Sabbah M, Azaïs H, Bendifallah S, et al. The use of microRNAs in the management of endometrial cancer: a meta-analysis. Cancers (Basel) (2019) 11(6):832. doi: 10.3390/cancers11060832
33. Gao J, Fan YZ, Gao SS, Zhang WT. Circulating microRNAs as potential biomarkers for the diagnosis of endometrial cancer: a meta-analysis. Reprod Sci (2022) 30(2):464–72. doi: 10.1007/s43032-022-01019-5
34. Giglio S, Annibali V, Cirombella R, Faruq O, Volinia S, De Vitis C, et al. miRNAs as candidate biomarker for the accurate detection of atypical endometrial Hyperplasia/Endometrial intraepithelial neoplasia. Front Oncol (2019) 9:526. doi: 10.3389/fonc.2019.00526
35. Fan X, Cao M, Liu C, Zhang C, Li C, Cheng W, et al. Three plasma-based microRNAs as potent diagnostic biomarkers for endometrial cancer. Cancer Biomark (2021) 312:127–38. doi: 10.3233/cbm-200972
36. Lu N, Liu J, Ji C, Wang Y, Wu Z, Yuan S, et al. MiRNA based tumor mutation burden diagnostic and prognostic prediction models for endometrial cancer. Bioengineered (2021) 121:3603–20. doi: 10.1080/21655979.2021.1947940
37. Sidorkiewicz I, Jóźwik M, Niemira M, Krętowski A. Insulin resistance and endometrial cancer: emerging role for microRNA. Cancers (Basel) (2020) 12(9):2559. doi: 10.3390/cancers12092559
38. Witek Ł, Janikowski T, Gabriel I, Bodzek P, Olejek A. Analysis of microRNA regulating cell cycle-related tumor suppressor genes in endometrial cancer patients. Hum Cell (2021) 342:564–9. doi: 10.1007/s13577-020-00451-6
39. Zheng X, Xu K, Zhu L, Mao M, Zhang F, Cui L. MiR-486-5p act as a biomarker in endometrial carcinoma: promotes cell proliferation, migration, invasion by targeting MARK1. Onco Targets Ther (2020) 13:4843–53. doi: 10.2147/ott.S246841
40. Yu F, Li B, Sun J, Qi J, De Wilde RL, Torres-de la Roche LA, et al. PSRR: a web server for predicting the regulation of miRNAs expression by small molecules. Front Mol Biosci (2022) 9:817294. doi: 10.3389/fmolb.2022.817294
41. Kalinkova L, Kajo K, Karhanek M, Wachsmannova L, Suran P, Zmetakova I, et al. Discriminating miRNA profiles between endometrioid well- and poorly-differentiated tumours and endometrioid and serous subtypes of endometrial cancers. Int J Mol Sci (2020) 21(17):6071. doi: 10.3390/ijms21176071
42. Lin CY, Wu RC, Yang LY, Jung SM, Ueng SH, Tang YH, et al. MicroRNAs as predictors of future uterine malignancy in endometrial hyperplasia without atypia. J Pers Med (2022) 12(2):311. doi: 10.3390/jpm12020311
43. Naydenov M, Nikolova M, Apostolov A, Glogovitis I, Salumets A, Baev V, et al. The dynamics of miR-449a/c expression during uterine cycles are associated with endometrial development. Biol (Basel) (2022) 12(1):55. doi: 10.3390/biology12010055
44. Tamaru S, Kajihara T, Mizuno Y, Mizuno Y, Tochigi H, Ishihara O. Endometrial microRNAs and their aberrant expression patterns. Med Mol Morphol (2020) 533:131–40. doi: 10.1007/s00795-020-00252-8
45. Wilson MR, Reske JJ, Koeman J, Adams M, Joshi NR, Fazleabas AT, et al. SWI/SNF antagonism of PRC2 mediates estrogen-induced progesterone receptor expression. Cells (2022) 11(6):1000. doi: 10.3390/cells11061000
46. Weelden W, Putten L, Inda MA, Brussel Av, Snijders M, Schriever LMM, et al. Oestrogen receptor pathway activity is associated with outcome in endometrial cancer. Br J Cancer (2020) 1235:785–92. doi: 10.1038/s41416-020-0925-4
47. Budak E, Solakoglu Kahraman D, Budak A, Yanarateş A, Inan AH, Kanmaz AG, et al. The prognostic significance of serum CA125 levels with ER, PR, P53 and ki-67 expression in endometrial carcinomas. Ginekol Pol (2019) 9012:675–83. doi: 10.5603/gp.2019.0116
48. Yang B, Chen R, Liang X, Shi J, Wu X, Zhang Z, et al. Estrogen enhances endometrial cancer cells proliferation by upregulation of prohibitin. J Cancer (2019) 107:1616–21. doi: 10.7150/jca.28218
49. Wang C, Tran DA, Fu MZ, Chen W, Fu SW, Li X. Estrogen receptor, progesterone receptor, and HER2 receptor markers in endometrial cancer. J Cancer (2020) 117:1693–701. doi: 10.7150/jca.41943
50. Sato W, Ikeda K, Urano T, Abe Y, Nakasato N, Horie-Inoue K, et al. Efp promotes in vitro and in vivo growth of endometrial cancer cells along with the activation of nuclear factor-[kappa]B signaling.(Research article)(estrogen-responsive RING finger protein). PloS One (2018) 1312:e0208351. doi: 10.1371/journal.pone.0208351
51. Goad J, Ko YA, Kumar M, Jamaluddin MFB, Tanwar PS. Oestrogen fuels the growth of endometrial hyperplastic lesions initiated by overactive wnt/β-catenin signalling. Carcinogenesis (2018) 399:1105–16. doi: 10.1093/carcin/bgy079
52. Chi S, Liu Y, Zhou X, Feng D, Xiao X, Li W, et al. Knockdown of long non-coding HOTAIR enhances the sensitivity to progesterone in endometrial cancer by epigenetic regulation of progesterone receptor isoform b. Cancer Chemother Pharmacol (2019) 832:277–87. doi: 10.1007/s00280-018-3727-0
53. Mahajan V, Gujral P, Jain L, Ponnampalam AP. Differential expression of steroid hormone receptors and ten eleven translocation proteins in endometrial cancer cells. Front Oncol (2022) 12:763464. doi: 10.3389/fonc.2022.763464
54. Williams WV, Mitchell LA, Carlson SK, Raviele KM. Association of combined estrogen-progestogen and progestogen-only contraceptives with the development of cancer. Linacre Q (2018) 854:412–52. doi: 10.1177/0024363918811637
55. Di Donato V, Iacobelli V, Schiavi MC, Colagiovanni V, Pecorella I, Palaia I, et al. Impact of hormone receptor status and ki-67 expression on disease-free survival in patients affected by high-risk endometrial cancer. Int J Gynecol Cancer (2018) 283:505–13. doi: 10.1097/igc.0000000000001191
56. Yaguchi T, Onishi T. Estrogen induces cell proliferation by promoting ABCG2-mediated efflux in endometrial cancer cells. Biochem biophysics Rep (2018) 16:74–8. doi: 10.1016/j.bbrep.2018.10.005
57. Alejandro La G, Bellora N, Francois Le D, Jara R, Javier Quilez O, Villanueva JL, et al. Higher-order chromatin organization defines progesterone receptor and PAX2 binding to regulate estradiol-primed endometrial cancer gene expression. Cold Spring Harbor: Cold Spring Harbor Laboratory Press (2020).
58. La Greca A, Bellora N, Le Dily F, Jara R, Nacht AS, Quilez Oliete J, et al. Chromatin topology defines estradiol-primed progesterone receptor and PAX2 binding in endometrial cancer cells. Elife (2022) 11:e66034. doi: 10.7554/eLife.66034
59. Huang X, Wang X, Shang J, Zhaang Z, Cui B, Lin Y, et al. Estrogen related receptor alpha triggers the migration and invasion of endometrial cancer cells via up regulation of TGFB1. Cell Adh Migr (2018) 126:538–47. doi: 10.1080/19336918.2018.1477901
60. Chen Z, Yang H-J, Lin Q, Zhu M-J, Yu Y-Y, He X-Y, et al. Estrogen-ERα signaling and DNA hypomethylation co-regulate expression of stem cell protein PIWIL1 in ERα-positive endometrial cancer cells. Cell Commun Signal (2020) 181:84–13. doi: 10.1186/s12964-020-00563-4
61. Kong X, Li M, Shao K, Yang Y, Wang Q, Cai M. Progesterone induces cell apoptosis via the CACNA2D3/Ca2+/p38 MAPK pathway in endometrial cancer. Oncol Rep (2020) 431:121–32. doi: 10.3892/or.2019.7396
62. Wang X, Li Y, Wan L, Liu Y, Sun Y, Liu Y, et al. Downregulation of PDCD4 induced by progesterone is mediated by the PI3K/AKT signaling pathway in human endometrial cancer cells. Oncol Rep (2019) 422:849–56. doi: 10.3892/or.2019.7202
63. Liang Y, Lin B, Ye Z, Chen S, Yu H, Chen C, et al. Triple-high expression of phosphatase and tensin homolog (PTEN), estrogen receptor (ER) and progesterone receptor (PR) may predict favorable prognosis for patients with type I endometrial carcinoma. J Cancer (2020) 116:1436–45. doi: 10.7150/jca.33720
64. Suhaimi SS, Ab Mutalib N-S, Khor SS, Zain RRM, Syafruddin SE, Abu N, et al. Targeted next-generation sequencing identifies actionable targets in estrogen receptor positive and estrogen receptor negative endometriod endometrial cancer. Front Pharmacol (2018) 9:750. doi: 10.3389/fphar.2018.00750
65. Mahajan V, Farquhar C, Ponnampalam AP. Could DNA hydroxymethylation be crucial in influencing steroid hormone signaling in endometrial biology and endometriosis? Mol Reprod Dev (2020) 871:7–16. doi: 10.1002/mrd.23299
66. Tian W, Teng F, Gao J, Gao C, Liu G, Zhang Y, et al. Estrogen and insulin synergistically promote endometrial cancer progression via crosstalk between their receptor signaling pathways. Cancer Biol Med (2019) 161:55–65. doi: 10.20892/j.issn.2095-3941.2018.0157
67. Forsse D, Tangen IL, Fasmer KE, Halle MK, Viste K, Almås B, et al. Blood steroid levels predict survival in endometrial cancer and reflect tumor estrogen signaling. Gynecol Oncol (2020) 1562:400–6. doi: 10.1016/j.ygyno.2019.11.123
68. Silva C, Pires-Luís AS, Rocha E, Bartosch C, Lopes JM. Phenotypic intratumoral heterogeneity of endometrial carcinomas. Int J Gynecol Pathol (2018) 372:154–66. doi: 10.1097/pgp.0000000000000400
69. Liu S-G, Wu X-X, Hua T, Xin X-Y, Feng D-L, Chi S-Q, et al. NLRP3 inflammasome activation by estrogen promotes the progression of human endometrial cancer. OncoTargets Ther (2019) 12:6927–36. doi: 10.2147/OTT.S218240
70. Yoriki K, Mori T, Kokabu T, Matsushima H, Umemura S, Tarumi Y, et al. Estrogen-related receptor alpha induces epithelial-mesenchymal transition through cancer-stromal interactions in endometrial cancer. Sci Rep (2019) 91:6697. doi: 10.1038/s41598-019-43261-z
71. You D, Lin L, Wang Q, Yi T, Zhao L, Li M, et al. Clinical significance of BCL11A expression in ER-negative and PR-negative endometrial carcinoma. Int J Clin Exp Pathol (2018) 116:3068–75.
72. Guan J, Xie L, Luo X, Yang B, Zhang H, Zhu Q, et al. The prognostic significance of estrogen and progesterone receptors in grade I and II endometrioid endometrial adenocarcinoma: hormone receptors in risk stratification. J Gynecol Oncol (2019) 301:e13. doi: 10.3802/jgo.2019.30.e13
73. Smith D, Stewart CJR, Clarke EM, Lose F, Davies C, Armes J, et al. ER and PR expression and survival after endometrial cancer. Gynecol Oncol (2018) 1482:258–66. doi: 10.1016/j.ygyno.2017.11.027
74. Liang Y, Jiao H, Qu L, Liu H. Association between hormone replacement therapy and development of endometrial cancer: results from a prospective US cohort study. Front Med (Lausanne) (2021) 8:802959. doi: 10.3389/fmed.2021.802959
75. Dottino JA, Zhang Q, Loose DS, Fellman B, Melendez BD, Borthwick MS, et al. Endometrial biomarkers in premenopausal women with obesity: an at-risk cohort. Am J Obstet Gynecol (2021) 2243:278.e1–.e14. doi: 10.1016/j.ajog.2020.08.053
76. Kailasam A, Langstraat C. Contemporary use of hormonal therapy in endometrial cancer: a literature review. Curr Treat Options Oncol (2022) 2312:1818–28. doi: 10.1007/s11864-022-01031-6
77. Sriprasert I, Mert M, Mack WJ, Hodis HN, Shoupe D. Use of oral estradiol plus vaginal progesterone in healthy postmenopausal women. Maturitas (2021) 154:13–9. doi: 10.1016/j.maturitas.2021.09.002
78. Hein A, Schneider MO, Renner SK, Fasching PA, Fiessler C, Titz S, et al. Risk of postmenopausal hormone therapy and patient history factors for the survival rate in women with endometrial carcinoma. Arch Gynecol Obstet (2020) 3011:289–94. doi: 10.1007/s00404-019-05414-3
79. Pandey J, Yonder S. Premalignant lesions of the endometrium. In: StatPearls. Treasure Island (FL: StatPearls Publishing (2022).
80. van Weelden WJ, Reijnen C, Küsters-Vandevelde HVN, Bulten J, Bult P, Leung S, et al. The cutoff for estrogen and progesterone receptor expression in endometrial cancer revisited: a European network for individualized treatment of endometrial cancer collaboration study. Hum Pathol (2021) 109:80–91. doi: 10.1016/j.humpath.2020.12.003
81. Song W, Zhao Y. A prediction model based on clinical and histological features for predicting recurrence in patients with stage I-II endometrial cancer after surgical treatment. Ann Diagn Pathol (2022) 56:151861. doi: 10.1016/j.anndiagpath.2021.151861
82. Hirschfeld M, Ge I, Rücker G, Waldschmidt J, Mayer S, Jäger M, et al. Mutually distinguishing microRNA signatures of breast, ovarian and endometrial cancers in vitro. Mol Med Rep (2020) 225:4048–60. doi: 10.3892/mmr.2020.11466
83. Ajabnoor G, Alsubhi F, Shinawi T, Habhab W, Albaqami WF, Alqahtani HS, et al. Computational approaches for discovering significant microRNAs, microRNA-mRNA regulatory pathways, and therapeutic protein targets in endometrial cancer. Front Genet (2023) 13:1105173. doi: 10.3389/fgene.2022.1105173
84. Asanoma K, Hori E, Yoshida S, Yagi H, Onoyama I, Kodama K, et al. Mutual suppression between BHLHE40/BHLHE41 and the MIR301B-MIR130B cluster is involved in epithelial-to-mesenchymal transition of endometrial cancer cells. Oncotarget (2019) 1045:4640–54. doi: 10.18632/oncotarget.27061
85. Bao W, Zhang Y, Li S, Fan Q, Qiu M, Wang Y, et al. miR−107−5p promotes tumor proliferation and invasion by targeting estrogen receptor−α in endometrial carcinoma. Oncol Rep (2019) 413:1575–85. doi: 10.3892/or.2018.6936
86. Bouziyane A, Lamsisi M, Benaguida H, Benhessou M, El Kerroumi M, Ennaji MM. Diagnostic value of MicroRNA 21 in endometrial cancer and benign lesions and its differential expression with clinicopathological parameters. Microrna (2021) 102:146–52. doi: 10.2174/2211536610666210604122816
87. Che X, Jian F, Chen C, Liu C, Liu G, Feng W. PCOS serum-derived exosomal miR-27a-5p stimulates endometrial cancer cells migration and invasion. J Mol Endocrinol (2020) 641:1–12. doi: 10.1530/jme-19-0159
88. Chen C, Zhang Q, Kong B. miRNA-576-5p promotes endometrial cancer cell growth and metastasis by targeting ZBTB4. Clin Transl Oncol (2022) 25(3):706–20. doi: 10.1007/s12094-022-02976-8
89. Chen Z, Sun Y, Dong H, Zhang F, Xu H, Zhang J. circRNA circRIMS downregulates miR-505 through methylation to suppress cell proliferation in endometrial cancer. Crit Rev Eukaryot Gene Expr (2022) 324:41–7. doi: 10.1615/CritRevEukaryotGeneExpr.2022039647
90. Dai Z, Luo H, Chen J, Li L. MiR-210-3p accelerates tumor-relevant cell functions of endometrial carcinoma by repressing RUNX1T1. Mutat Res (2022) 825:111793. doi: 10.1016/j.mrfmmm.2022.111793
91. Dong H, Li Y, Zhou J, Song J. MiR-18a-5p promotes proliferation, migration, and invasion of endometrial cancer cells by targeting THBD. Crit Rev Eukaryot Gene Expr (2021) 312:63–73. doi: 10.1615/CritRevEukaryotGeneExpr.2021037776
92. Dong P, Xiong Y, Yue J, Hanley SJB, Watari H. miR-34a, miR-424 and miR-513 inhibit MMSET expression to repress endometrial cancer cell invasion and sphere formation. Oncotarget (2018) 933:23253–63. doi: 10.18632/oncotarget.25298
93. Donkers H, Bekkers R, Galaal K. Diagnostic value of microRNA panel in endometrial cancer: a systematic review. Oncotarget (2020) 1121:2010–23. doi: 10.18632/oncotarget.27601
94. Lee L, Howitt B, Cheng T, King M, Stawiski K, Fendler W, et al. MicroRNA profiling in a case-control study of African American women with uterine serous carcinoma. Gynecol Oncol (2021) 1633:453–8. doi: 10.1016/j.ygyno.2021.09.015
95. Du J, Zhang F, Zhang L, Jia Y, Chen H. MicroRNA-103 regulates the progression in endometrial carcinoma through ZO-1. Int J Immunopathol Pharmacol (2019) 33:2058738419872621. doi: 10.1177/2058738419872621
96. Fan X, Zou X, Liu C, Cheng W, Zhang S, Geng X, et al. MicroRNA expression profile in serum reveals novel diagnostic biomarkers for endometrial cancer. Biosci Rep (2021) 41(6):BSR20210111. doi: 10.1042/BSR20210111
97. Gao X, Cai Y, An R. miR−215 promotes epithelial to mesenchymal transition and proliferation by regulating LEFTY2 in endometrial cancer. Int J Mol Med (2018) 423:1229–36. doi: 10.3892/ijmm.2018.3703
98. Gao Y, Qian H, Tang X, Du X, Wang G, Zhang H, et al. Superparamagnetic iron oxide nanoparticle-mediated expression of miR-326 inhibits human endometrial carcinoma stem cell growth. Int J Nanomed (2019) 14:2719–31. doi: 10.2147/ijn.S200480
99. Geletina NS, Kobelev VS, Babayants EV, Feng L, Pustylnyak VO, Gulyaeva LF. PTEN negative correlates with miR-181a in tumour tissues of non-obese endometrial cancer patients. Gene (2018) 655:20–4. doi: 10.1016/j.gene.2018.02.051
100. Ghazala RA, El-Attar EA, Abouzeid ZS. Circulating miRNA 27a and miRNA150-5p; a noninvasive approach to endometrial carcinoma. Mol Biol Rep (2021) 485:4351–60. doi: 10.1007/s11033-021-06450-6
101. Hao L, Wang J. miR-27a acts as an oncogene to regulate endometrial cancer progression by targeting USP46. Int J Clin Exp Pathol (2021) 146:720–5.
102. Huang W, Zhang J, Dong B, Chen H, Shao L, Li X. A novel miR-98 negatively regulates the resistance of endometrial cancer cells to paclitaxel by suppressing ABCC10/MRP-7. Front Oncol (2021) 11:809410. doi: 10.3389/fonc.2021.809410
103. Li J, Sun H, Liu T, Kong J. MicroRNA-423 promotes proliferation, migration and invasion and induces chemoresistance of endometrial cancer cells. Exp Ther Med (2018) 165:4213–24. doi: 10.3892/etm.2018.6710
104. Srivastava A, Moxley K, Ruskin R, Dhanasekaran DN, Zhao YD, Ramesh R. A non-invasive liquid biopsy screening of urine-derived exosomes for miRNAs as biomarkers in endometrial cancer patients. AAPS J (2018) 205:82. doi: 10.1208/s12248-018-0220-y
105. Sun X, Dongol S, Qiu C, Xu Y, Sun C, Zhang Z, et al. miR-652 promotes tumor proliferation and metastasis by targeting RORA in endometrial cancer. Mol Cancer Res (2018) 1612:1927–39. doi: 10.1158/1541-7786.Mcr-18-0267
106. Sun X, Hou L, Qiu C, Kong B. MiR-501 promotes tumor proliferation and metastasis by targeting HOXD10 in endometrial cancer. Cell Mol Biol Lett (2021) 261:20. doi: 10.1186/s11658-021-00268-7
107. Wang C, Li Q, He Y. MicroRNA−21−5p promotes epithelial to mesenchymal transition by targeting SRY−box 17 in endometrial cancer. Oncol Rep (2020) 436:1897–905. doi: 10.3892/or.2020.7556
108. Wang P, Zhao X, Wu X, Tang G, Yuan L. miR-15b-3p promotes the malignant progression of endometrial cancer cells through targeting KLF2. Cell Cycle (2021) 2014:1431–40. doi: 10.1080/15384101.2021.1941611
109. Wilczynski M, Danielska J, Domanska-Senderowska D, Dzieniecka M, Szymanska B, Malinowski A. Association of microRNA-200c expression levels with clinicopathological factors and prognosis in endometrioid endometrial cancer. Acta Obstet Gynecol Scand (2018) 975:560–9. doi: 10.1111/aogs.13306
110. Xiao Y, Huang W, Huang H, Wang L, Wang M, Zhang T, et al. miR-182-5p and miR-96-5p target PIAS1 and mediate the negative feedback regulatory loop between PIAS1 and STAT3 in endometrial cancer. DNA Cell Biol (2021) 404:618–28. doi: 10.1089/dna.2020.6379
111. Xiong H, Chen R, Liu S, Lin Q, Chen H, Jiang Q. MicroRNA-183 induces epithelial-mesenchymal transition and promotes endometrial cancer cell migration and invasion in by targeting CPEB1. J Cell Biochem (2018) 11910:8123–37. doi: 10.1002/jcb.26763
112. Xu X, Kong X, Liu T, Zhou L, Wu J, Fu J, et al. Metastasis-associated protein 1, modulated by miR-30c, promotes endometrial cancer progression through AKT/mTOR/4E-BP1 pathway. Gynecol Oncol (2019) 1541:207–17. doi: 10.1016/j.ygyno.2019.04.005
113. Yang C, Ota-Kurogi N, Ikeda K, Okumura T, Horie-Inoue K, Takeda S, et al. MicroRNA-191 regulates endometrial cancer cell growth via TET1-mediated epigenetic modulation of APC. J Biochem (2020) 1681:7–14. doi: 10.1093/jb/mvaa014
114. Yang L, Yang Z, Yao R, Li Y, Liu Z, Chen X, et al. miR-210 promotes progression of endometrial carcinoma by regulating the expression of NFIX. Int J Clin Exp Pathol (2018) 1111:5213–22.
115. Yang LJ, Gao L, Guo YN, Liang ZQ, Li DM, Tang YL, et al. Upregulation of microRNA miR-141-3p and its prospective targets in endometrial carcinoma: a comprehensive study. Bioengineered (2021) 121:2941–56. doi: 10.1080/21655979.2021.1943111
116. Yao H, Kong F, Zhou Y. MiR-182 promotes cell proliferation, migration and invasion by targeting FoxF2 in endometrial carcinoma cells. Int J Clin Exp Pathol (2019) 124:1248–59.
117. Zhang HC, Han YY, Zhang XM, Xiao N, Jiang T, Zhu S, et al. miR-522 facilitates the prosperities of endometrial carcinoma cells by directly binding to monoamine oxidase b. Kaohsiung J Med Sci (2019) 3510:598–606. doi: 10.1002/kjm2.12107
118. Zhao J, Dong X, Tao SJ, Liu XL, Li Z, Liu JM, et al. MDM4 is targeted by miR-449b-5p to promote the proliferation of endometrial carcinoma. Eur Rev Med Pharmacol Sci (2020) 2422:11528–35. doi: 10.26355/eurrev_202011_23794
119. Zheng WP, Meng FL, Wang LY. miR-544a stimulates endometrial carcinoma growth via targeted inhibition of reversion-inducing cysteine-rich protein with kazal motifs. Mol Cell Probes (2020) 53:101572. doi: 10.1016/j.mcp.2020.101572
120. Zheng Y, Yang X, Wang C, Zhang S, Wang Z, Li M, et al. HDAC6, modulated by miR-206, promotes endometrial cancer progression through the PTEN/AKT/mTOR pathway. Sci Rep (2020) 101:3576. doi: 10.1038/s41598-020-60271-4
121. Zhou Z, Xu YP, Wang LJ, Kong Y. miR-940 potentially promotes proliferation and metastasis of endometrial carcinoma through regulation of MRVI1. Biosci Rep (2019) 39(6):BSR20190077. doi: 10.1042/bsr20190077
122. Zhu L, Wang X, Wang T, Zhu W, Zhou X. miR−494−3p promotes the progression of endometrial cancer by regulating the PTEN/PI3K/AKT pathway. Mol Med Rep (2019) 191:581–8. doi: 10.3892/mmr.2018.9649
123. Zhuang L, Qu H, Cong J, Dai H, Liu X. MiR-181c affects estrogen-dependent endometrial carcinoma cell growth by targeting PTEN. Endocr J (2019) 666:523–33. doi: 10.1507/endocrj.EJ18-0538
124. Buchynska LG, Borykun TV, Iurchenko NP, Nespryadko SV, Nesina IP. Expression of microRNA in tumor cells of endmetrioid carcinoma of endometrium. Exp Oncol (2020) 424:289–94. doi: 10.32471/exp-oncology.2312-8852.vol-42-no-4.15522
125. Cai L, Chen JJ, Deng FM, Wang L, Chen Y. MiR-326 regulates the proliferation and apoptosis of endometrial cancer by targeting bcl-2. J Obstet Gynaecol Res (2021) 472:621–30. doi: 10.1111/jog.14572
126. Chen P, Xing T, Wang Q, Liu A, Liu H, Hu Y, et al. MicroRNA-202 inhibits cell migration and invasion through targeting FGF2 and inactivating wnt/β-catenin signaling in endometrial carcinoma. Biosci Rep (2019) 3910. doi: 10.1042/bsr20190680
127. Li BL, Lu W, Qu JJ, Ye L, Du GQ, Wan XP. Loss of exosomal miR-148b from cancer-associated fibroblasts promotes endometrial cancer cell invasion and cancer metastasis. J Cell Physiol (2019) 2343:2943–53. doi: 10.1002/jcp.27111
128. Chen R, Ma X, Zhang L. MicorRNA-148b inhibits cell proliferation and facilitates cell apoptosis by regulating DNA methyltransferase 1 in endometrial cancer. Transl Cancer Res (2020) 92:1100–12. doi: 10.21037/tcr.2019.12.79
129. Chen X, Jin P, Tang H, Zhang L. miR-135a acts as a tumor suppressor by targeting ASPH in endometrial cancer. Int J Clin Exp Pathol (2019) 129:3384–9.
130. Chen Z, Zhu Y, Fan X, Liu Y, Feng Q. Decreased expression of miR-184 restrains the growth and invasion of endometrial carcinoma cells through CDC25A-dependent notch signaling pathway. Am J Transl Res (2019) 112:755–64.
131. Deng J, Wang W, Yu G, Ma X. MicroRNA−195 inhibits epithelial−mesenchymal transition by targeting G protein−coupled estrogen receptor 1 in endometrial carcinoma. Mol Med Rep (2019) 205:4023–32. doi: 10.3892/mmr.2019.10652
132. Dong Y, Yang H, Hua H. MicroRNA-641 inhibits endometrial cancer progression via targeting AP1G1. Evid Based Complement Alternat Med (2022) 2022:7918596. doi: 10.1155/2022/7918596
133. Fang X, Wang J, Chen L, Zhang X. circRNA circ_POLA2 increases microRNA-31 methylation to promote endometrial cancer cell proliferation. Oncol Lett (2021) 225:762. doi: 10.3892/ol.2021.13023
134. Fang YY, Tan MR, Zhou J, Liang L, Liu XY, Zhao K, et al. miR-214-3p inhibits epithelial-to-mesenchymal transition and metastasis of endometrial cancer cells by targeting TWIST1. Onco Targets Ther (2019) 12:9449–58. doi: 10.2147/ott.S181037
135. Geng A, Luo L, Ren F, Zhang L, Zhou H, Gao X. miR-29a-3p inhibits endometrial cancer cell proliferation, migration and invasion by targeting VEGFA/CD C42/PAK1. BMC Cancer. (2021) 211:843. doi: 10.1186/s12885-021-08506-z
136. He Y, Ma H, Wang J, Kang Y, Xue Q. miR-20a-5p inhibits endometrial cancer progression by targeting janus kinase 1. Oncol Lett (2021) 215:427. doi: 10.3892/ol.2021.12688
137. Hu Y, Wu AY, Xu C, Song KQ, Wang WJ, Yin X, et al. MicroRNA-449a inhibits tumor metastasis through AKT/ERK1/2 inactivation by targeting steroid receptor coactivator (SRC) in endometrial cancer. J Cancer (2019) 102:547–55. doi: 10.7150/jca.27748
138. Klicka K, Grzywa TM, Klinke A, Mielniczuk A, Wejman J, Ostrowska J, et al. Decreased expression of miR-23b is associated with poor survival of endometrial cancer patients. Sci Rep (2022) 121:18824. doi: 10.1038/s41598-022-22306-w
139. Li HL, Sun JJ, Ma H, Liu SJ, Li N, Guo SJ, et al. MicroRNA-23a inhibits endometrial cancer cell development by targeting SIX1. Oncol Lett (2019) 184:3792–802. doi: 10.3892/ol.2019.10694
140. Li L, Ma L. Upregulation of miR-582-5p regulates cell proliferation and apoptosis by targeting AKT3 in human endometrial carcinoma. Saudi J Biol Sci (2018) 255:965–70. doi: 10.1016/j.sjbs.2018.03.007
141. Li X, Liu LL, Yao JL, Wang K, Ai H. Human umbilical cord mesenchymal stem cell-derived extracellular vesicles inhibit endometrial cancer cell proliferation and migration through delivery of exogenous miR-302a. Stem Cells Int (2019) 2019:8108576. doi: 10.1155/2019/8108576
142. Li Y, Huo J, Pan X, Wang C, Ma X. MicroRNA 302b-3p/302c-3p/302d-3p inhibits epithelial-mesenchymal transition and promotes apoptosis in human endometrial carcinoma cells. Onco Targets Ther (2018) 11:1275–84. doi: 10.2147/ott.S154517
143. Liang M, Chen H, Min J. miR-379-5p inhibits proliferation and invasion of the endometrial cancer cells by inhibiting expression of ROR1. Acta Biochim Pol (2021) 684:659–65. doi: 10.18388/abp.2020_5538
144. Liao L, Chen Y, Zhou J, Ye J. MicroRNA-133b inhibits nTumor cell proliferation, migration and invasion by targeting SUMO1 in endometrial carcinoma. Technol Cancer Res Treat (2021) 20:15330338211065241. doi: 10.1177/15330338211065241
145. Liu J, Li C, Jiang Y, Wan Y, Zhou S, Cheng W. Tumor-suppressor role of miR-139-5p in endometrial cancer. Cancer Cell Int (2018) 18:51. doi: 10.1186/s12935-018-0545-8
146. Ma J, Li D, Kong FF, Yang D, Yang H, Ma XX. miR-302a-5p/367-3p-HMGA2 axis regulates malignant processes during endometrial cancer development. J Exp Clin Cancer Res (2018) 371:19. doi: 10.1186/s13046-018-0686-6
147. Men Y, Zhang L, Ai H. [MicroRNA-145-5p over-expression suppresses proliferation, migration and invasion and promotes apoptosis of human endometrial cancer cells by targeting dual specific phosphatase 6]. Nan Fang Yi Ke Da Xue Xue Bao. (2020) 401:61–6. doi: 10.12122/j.issn.1673-4254.2020.01.10
148. Shen XC, Sun YQ, Yan L, Zhao XX. [Effect of miR1290 on the growth of endometrial cancer and the related mechanism]. Zhonghua Zhong Liu Za Zhi. (2022) 442:130–8. doi: 10.3760/cma.j.cn112152-20210208-00121
149. Shigeta S, Watanabe Y, Suzuki F, Nagase S, Shibuya Y, Ishibashi M, et al. MicroRNA-152 regulates endometrial serous carcinoma cell motility by suppressing matrix metalloproteinase 10 expression. Tohoku J Exp Med (2022) 2563:249–58. doi: 10.1620/tjem.256.249
150. Shu S, Liu X, Xu M, Gao X, Chen S, Zhang L, et al. MicroRNA-320a acts as a tumor suppressor in endometrial carcinoma by targeting IGF-1R. Int J Mol Med (2019) 433:1505–12. doi: 10.3892/ijmm.2019.4051
151. Zhang HH, Li R, Li YJ, Yu XX, Sun QN, Li AY, et al. eIF4E−related miR−320a and miR−340−5p inhibit endometrial carcinoma cell metastatic capability by preventing TGF−β1−induced epithelial−mesenchymal transition. Oncol Rep (2020) 432:447–60. doi: 10.3892/or.2019.7437
152. Zhang N, Wang Y, Liu H, Shen W. Extracellular vesicle encapsulated microRNA-320a inhibits endometrial cancer by suppression of the HIF1α/VEGFA axis. Exp Cell Res (2020) 3942:112113. doi: 10.1016/j.yexcr.2020.112113
153. Shu S, Liu X, Xu M, Gao X, Fan J, Liu H, et al. MicroRNA-424 regulates epithelial-mesenchymal transition of endometrial carcinoma by directly targeting insulin-like growth factor 1 receptor. J Cell Biochem (2019) 1202:2171–9. doi: 10.1002/jcb.27528
154. Tan A, Luo R, Ruan P. miR-495 promotes apoptosis and inhibits proliferation in endometrial cells via targeting PIK3R1. Pathol Res Pract (2019) 2153:594–9. doi: 10.1016/j.prp.2019.01.020
155. Tian Y, Chen YY, Han AL. MiR-1271 inhibits cell proliferation and metastasis by targeting LDHA in endometrial cancer. Eur Rev Med Pharmacol Sci (2019) 2313:5648–56. doi: 10.26355/eurrev_201907_18300
156. Tu C, Wang F, Wan J. MicroRNA-381 inhibits cell proliferation and invasion in endometrial carcinoma by targeting the IGF-1R. Mol Med Rep (2018) 173:4090–8. doi: 10.3892/mmr.2017.8288
157. Van Sinderen M, Griffiths M, Menkhorst E, Niven K, Dimitriadis E. Restoration of microRNA-29c in type I endometrioid cancer reduced endometrial cancer cell growth. Oncol Lett (2019) 183:2684–93. doi: 10.3892/ol.2019.10588
158. Wang C, Liu B. miR-101-3p induces autophagy in endometrial carcinoma cells by targeting EZH2. Arch Gynecol Obstet (2018) 2976:1539–48. doi: 10.1007/s00404-018-4768-7
159. Wang C, Su K, Zhang Y, Zhang W, Chu D, Zhao Q, et al. MicroRNA-365 targets multiple oncogenes to inhibit proliferation, invasion, and self-renewal of aggressive endometrial cancer cells. Cancer Manag Res (2018) 10:5171–85. doi: 10.2147/cmar.S174889
160. Wang H, Wang TT, Lv XP. Expression and prognostic value of miRNA-29b in peripheral blood for endometrial cancer. Future Oncol (2018) 1414:1365–76. doi: 10.2217/fon-2017-0594
161. Wang H, Yang Q, Li J, Chen W, Jin X, Wang Y. MicroRNA-15a-5p inhibits endometrial carcinoma proliferation, invasion and migration via downregulation of VEGFA and inhibition of the wnt/β-catenin signaling pathway. Oncol Lett (2021) 214:310. doi: 10.3892/ol.2021.12570
162. Wang L, Liu D, Wei J, Yuan L, Zhao S, Huang Y, et al. MiR-543 inhibits the migration and epithelial-To-Mesenchymal transition of TGF-β-Treated endometrial stromal cells via the MAPK and wnt/β-catenin signaling pathways. Pathol Oncol Res (2021) 27:1609761. doi: 10.3389/pore.2021.1609761
163. Wang Q, Zhu W. MicroRNA-873 inhibits the proliferation and invasion of endometrial cancer cells by directly targeting hepatoma-derived growth factor. Exp Ther Med (2019) 182:1291–8. doi: 10.3892/etm.2019.7713
164. Wang Y, Dong L, Liu Y. Targeting thyroid receptor interacting protein 6 by MicroRNA-589-5p inhibits cell proliferation, migration, and invasion in endometrial carcinoma. Cancer Biother Radiopharm (2019) 348:529–36. doi: 10.1089/cbr.2018.2766
165. Wang Y, Ma H, Li Y, Su R. MiR-192-5p-Modified tumor-associated macrophages-derived exosome suppressed endometrial cancer progression through targeting IRAK1/NF-κB signaling. Reprod Sci (2022) 292:436–47. doi: 10.1007/s43032-021-00789-8
166. Wu X, Han Y, Liu F, Ruan L. Downregulations of miR-449a and miR-145-5p act as prognostic biomarkers for endometrial cancer. J Comput Biol (2020) 275:834–44. doi: 10.1089/cmb.2019.0215
167. Wu AY, Hu Y, Cang W, Li D, Wang WJ, Tian Q, et al. Suppressive effect of microRNA-449a on the NDRG1/PTEN/AKT axis regulates endometrial cancer growth and metastasis. Exp Cell Res (2019) 3822:111468. doi: 10.1016/j.yexcr.2019.06.013
168. Wu C, Zhou X, Li J, Xiao R, Xin H, Dai L, et al. Serum miRNA−204−5p as a potential non−invasive biomarker for the diagnosis of endometrial cancer with sentinel lymph node mapping. Oncol Lett (2022) 242:1. doi: 10.3892/ol.2022.13368
169. Xie D, Liang Y, Su Y, An Y, Qu P. miR-152 inhibits proliferation of human endometrial cancer cells via inducing G2/M phase arrest by suppressing CDC25B expression. BioMed Pharmacother (2018) 99:299–305. doi: 10.1016/j.biopha.2018.01.046
170. Xin W, Zhao S, Han X, Zhao P, Yu H, Gao X, et al. lncRNA LA16c−313D11.11 modulates the development of endometrial cancer by binding to and inhibiting microRNA−205−5p function and indirectly increasing PTEN activity. Int J Oncol (2020) 571:355–63. doi: 10.3892/ijo.2020.5046
171. Xiong H, Wang N, Chen H, Zhang M, Lin Q. MicroRNA−199a/b−5p inhibits endometrial cancer cell metastasis and invasion by targeting FAM83B in the epithelial−to−mesenchymal transition signaling pathway. Mol Med Rep (2021) 23(5):304. doi: 10.3892/mmr.2021.11943
172. Yanokura M, Banno K, Aoki D. MicroRNA−34b expression enhances chemosensitivity of endometrial cancer cells to paclitaxel. Int J Oncol (2020) 575:1145–56. doi: 10.3892/ijo.2020.5127
173. Zhang C, Wang B, Wu L. MicroRNA−409 may function as a tumor suppressor in endometrial carcinoma cells by targeting Smad2. Mol Med Rep (2019) 191:622–8. doi: 10.3892/mmr.2018.9642
174. Zhao X, Dai L, Yue Q, Wang H, Wang XU, Li Y, et al. MiR-195 inhibits migration, invasion and epithelial-mesenchymal transition (EMT) of endometrial carcinoma cells by targeting SOX4. J Biosci (2019) 44(6):146. doi: 10.1007/s12038-019-9966-3
175. Zmarzły N, Hermyt E, Kruszniewska-Rajs C, Gola J, Witek A, Mazurek U, et al. Expression profile of EMT-related genes and miRNAs involved in signal transduction via the wnt pathway and cadherins in endometrial cancer. Curr Pharm Biotechnol (2021) 2212:1663–71. doi: 10.2174/1389201021666201218125900
176. Huang X, Zhong R, He X, Deng Q, Peng X, Li J, et al. Investigations on the mechanism of progesterone in inhibiting endometrial cancer cell cycle and viability via regulation of long noncoding RNA NEAT1/microRNA-146b-5p mediated wnt/β-catenin signaling. IUBMB Life (2019) 712:223–34. doi: 10.1002/iub.1959
177. Yuan DZ, Lei Y, Zhao D, Pan JL, Zhao YB, Nie L, et al. Progesterone-induced miR-145/miR-143 inhibits the proliferation of endometrial epithelial cells. Reprod Sci (2019) 262:233–43. doi: 10.1177/1933719118768687
178. Zhang G, Cheng Y, Zhang Q, Li X, Zhou J, Wang J, et al. ATX−LPA axis facilitates estrogen−induced endometrial cancer cell proliferation via MAPK/ERK signaling pathway. Mol Med Rep (2018) 173:4245–52. doi: 10.3892/mmr.2018.8392
179. Zou Z, Zhou S, Liang G, Tang Z, Li K, Tan S, et al. The pan-cancer analysis of the two types of uterine cancer uncovered clinical and prognostic associations with m6A RNA methylation regulators. Mol Omics (2021) 173:438–53. doi: 10.1039/d0mo00113a
180. Devor EJ, Cha E, Warrier A, Miller MD, Gonzalez-Bosquet J, Leslie KK. The miR-503 cluster is coordinately under-expressed in endometrial endometrioid adenocarcinoma and targets many oncogenes, cell cycle genes, DNA repair genes and chemotherapy response genes. Onco Targets Ther (2018) 11:7205–11. doi: 10.2147/ott.S180921
181. Huang J, Du F, Wang N. Development of a 4-miRNA prognostic signature for endometrial cancer. Med (Baltimore) (2022) 10141:e30974. doi: 10.1097/md.0000000000030974
182. Hui P, Gysler SM, Uduman M, Togun TA, Prado DE, Brambs CE, et al. MicroRNA signatures discriminate between uterine and ovarian serous carcinomas. Hum Pathol (2018) 76:133–40. doi: 10.1016/j.humpath.2018.02.019
183. Wang Y, Xu M, Yang Q. A six-microRNA signature predicts survival of patients with uterine corpus endometrial carcinoma. Curr Probl Cancer (2019) 432:167–76. doi: 10.1016/j.currproblcancer.2018.02.002
184. Wu YS, Lin H, Chen D, Yi Z, Zeng B, Jiang Y, et al. A four-miRNA signature as a novel biomarker for predicting survival in endometrial cancer. Gene (2019) 697:86–93. doi: 10.1016/j.gene.2019.01.046
185. Zhou H, Chen L, Qin M, Lei Y, Li T, Li H, et al. An miRNA signature associated with tumor mutation burden in endometrial cancer. Biosci Rep (2020) 40(11):BSR20203398. doi: 10.1042/bsr20203398
186. Donkers H, Hirschfeld M, Weiß D, Erbes T, Jaeger M, Pijnenborg JMA, et al. Usefulness of microRNA detection in the diagnostics of endometrial cancer. Acta Obstet Gynecol Scand (2021) 1006:1148–54. doi: 10.1111/aogs.14141
187. Zhou L, Wang W, Wang F, Yang S, Hu J, Lu B, et al. Plasma-derived exosomal miR-15a-5p as a promising diagnostic biomarker for early detection of endometrial carcinoma. Mol Cancer (2021) 201:57. doi: 10.1186/s12943-021-01352-4
188. Wang J, Zhang L, Jiang W, Zhang R, Zhang B, Silayiding A, et al. MicroRNA-135a promotes proliferation, migration, invasion and induces chemoresistance of endometrial cancer cells. Eur J Obstet Gynecol Reprod Biol X (2020) 5:100103. doi: 10.1016/j.eurox.2019.100103
189. Liolios T, Kastora SL, Colombo G. MicroRNAs in female malignancies. Cancer Inform. (2019) 18:1176935119828746. doi: 10.1177/1176935119828746
190. Jayarathna DK, Rentería ME, Malik A, Sauret E, Batra J, Gandhi NS. Integrative transcriptome-wide analyses uncover novel risk-associated MicroRNAs in hormone-dependent cancers. Front Genet (2021) 12:716236. doi: 10.3389/fgene.2021.716236
191. Song H, Li T, Sheng J, Li D, Liu X, Xiao H, et al. Necroptosis-related miRNA biomarkers for predicting overall survival outcomes for endometrial cancer. Front Genet (2022) 13:828456. doi: 10.3389/fgene.2022.828456
192. Wang X, He D, Li WT, Adila S, Han R, Dong Y. [Characteristic and clinical significance of microRNA expression between 144 uygur and han women with endometrial carcinoma]. Beijing Da Xue Xue Bao Yi Xue Ban. (2020) 523:570–7. doi: 10.19723/j.issn.1671-167X.2020.03.026
193. Matarrese P, Mattia G, Pagano MT, Pontecorvi G, Ortona E, Malorni W, et al. The sex-related interplay between TME and cancer: on the critical role of estrogen, MicroRNAs and autophagy. Cancers (2021) 1313:3287. doi: 10.3390/cancers13133287
194. Zheng MJ, Gou R, Zhang WC, Nie X, Wang J, Gao LL, et al. Screening of prognostic biomarkers for endometrial carcinoma based on a ceRNA network. PeerJ (2018) 6:e6091. doi: 10.7717/peerj.6091
195. Song Y, Chu P, Li P, Li F. Construction of endometrial carcinoma ceRNA network and screening of key genes based on TCGA database. Comput Math Methods Med (2022) 2022:1418232. doi: 10.1155/2022/1418232
196. Ye Y, Li H, Bian J, Wang L, Wang Y, Huang H. Exploring prognosis-associated biomarkers of estrogen-independent uterine corpus endometrial carcinoma by bioinformatics analysis. Int J Gen Med (2021) 14:9067–81. doi: 10.2147/ijgm.S341345
197. Czerwiński M, Bednarska-Czerwińska A, Zmarzły N, Boroń D, Oplawski M, Grabarek BO. miRNAs in the expression regulation of dopamine-related genes and proteins in endometrial cancer. J Clin Med (2021) 1021:4939. doi: 10.3390/jcm10214939
198. Klicka K, Grzywa TM, Klinke A, Mielniczuk A, Włodarski PK. The role of miRNAs in the regulation of endometrial cancer invasiveness and metastasis-a systematic review. Cancers (Basel) (2021) 13(14):3393. doi: 10.3390/cancers13143393
199. Favier A, Rocher G, Larsen AK, Delangle R, Uzan C, Sabbah M, et al. MicroRNA as epigenetic modifiers in endometrial cancer: a systematic review. Cancers (Basel) (2021) 13(5):1137. doi: 10.3390/cancers13051137
200. Chen R, Sun K, Hou Y, Shen J, Chen J, Dong F, et al. Identification of m7G methylation-related miRNA signature associated with survival and immune microenvironment regulation in uterine corpus endometrial carcinoma. BioMed Res Int (2022) 2022:8776678. doi: 10.1155/2022/8776678
201. de Foucher T, Sbeih M, Uzan J, Bendifallah S, Lefevre M, Chabbert-Buffet N, et al. Identification of micro-RNA expression profile related to recurrence in women with ESMO low-risk endometrial cancer. J Transl Med (2018) 161:131. doi: 10.1186/s12967-018-1515-6
202. Wilczynski M, Senderowska D, Krawczyk T, Szymanska B, Malinowski A. MiRNAs in endometrioid endometrial cancer metastatic loci derived from positive lymph nodes. Acta Obstet Gynecol Scand (2020) 998:1085–91. doi: 10.1111/aogs.13833
203. Zhang J, Zhang D, Yan X, Jiang F. The expression level and prognostic value of microRNA-15a-5p in endometrial carcinoma. Transl Cancer Res (2021) 1011:4838–44. doi: 10.21037/tcr-21-2079
Keywords: microRNA, endometrial cancer, sex steroid hormones, estrogen, progesterone, hormone therapy
Citation: Thakur L and Thakur S (2023) The interplay of sex steroid hormones and microRNAs in endometrial cancer: current understanding and future directions. Front. Endocrinol. 14:1166948. doi: 10.3389/fendo.2023.1166948
Received: 15 February 2023; Accepted: 07 April 2023;
Published: 21 April 2023.
Edited by:
Prem P. Kushwaha, Case Western Reserve University, United StatesReviewed by:
Jayadev Joshi, Cleveland Clinic, United StatesAnkit Kushwaha, Stanford University, United States
Copyright © 2023 Thakur and Thakur. This is an open-access article distributed under the terms of the Creative Commons Attribution License (CC BY). The use, distribution or reproduction in other forums is permitted, provided the original author(s) and the copyright owner(s) are credited and that the original publication in this journal is cited, in accordance with accepted academic practice. No use, distribution or reproduction is permitted which does not comply with these terms.
*Correspondence: Sunil Thakur, thakursunil177@gmail.com