Abstract
Aim:
The purpose of this study is to conduct a comprehensive investigation into the modulatory effects of Stryphnodendron adstringens (Mart.; S. adstringens), a Brazilian wound-healing plant, on the expression of inflammatory cytokines. This will be achieved using an in vitro protocol with the commercial macrophage cell line RAW 264.7.
Methods:
The macrophage inflammatory response was induced by the natural antigen phytohemagglutinin (PHA), with and without supplementation of different concentrations of S. adstringens extract. The effects on cell proliferation rate and the concentration and production of transcripts of pro-inflammatory cytokines interleukin 1β (IL-1β), IL-6, tumor necrosis factor-alpha (TNF-α), and interferon-gamma (IFN-γ), as well as the anti-inflammatory cytokine IL-10, were assessed using spectrophotometric, immunoassay, and quantitative reverse transcription-polymerase chain reaction (qRT-PCR) techniques.
Results:
S. adstringens extract at all concentrations tested here reduced the cellular proliferation rate of activated macrophages. Extracts at concentrations of 0.49 mg/mL and 0.99 mg/mL decreased the protein and gene expression of pro-inflammatory cytokines, exhibiting the opposite effect concerning IL-10.
Conclusions:
The findings suggest that the wound-healing action of S. adstringens may encompass differential modulation of inflammation associated with tissue injury.
Keywords
Immunomodulation, phytotherapics, cytokines, pharmacogenomicsIntroduction
Brazil presents a great number of natural plants with medicinal use from pre-Colombian to contemporary traditional communities. One of the native trees commonly used is known as “barbatimão”—Stryphnodendron adstringens (Mart.; S. adstringens) Coville, from which stem barks are prepared as decoctions or infusions been characterized by folk medicine as a cicatrizing, astringent, anti-inflammatory, and antimicrobial plant [1–5].
The biological S. adstringens properties have been associated with its chemical matrix that richest in several bioactive molecules that could influence its wound-healing activity, including gallic acid and catechin molecules, such as epigallocatechin, epigallocatechin 3-O-gallate, 4’-O-methyl-epigallocatechin, epigallocatechin-O-methyl gallate, epigallocatechin-epigallocatechin, 4’-O-methyl-epigallocatechin-4’-O-methyl-epigallocatechin, and epigallocatechin-epigallocatechin 3-O-gallate [6]. S. adstringens extracts also identified present other important bioactive polyphenol molecules, such as quercetin, rutin, and kaempferol [7].
Scientific reports corroborate the strong wound-healing activity of S. adstringens [7–11] and experimental findings suggest its therapeutic effect on some chronic inflammatory or infectious conditions [12–18]. These results suggested that S. adstringens had a relevant anti-inflammatory role, involving its ability to modulate bone marrow macrophage polarization showing a reduction in interleukin 6 (IL-6) cytokines.
However, the extent to which S. adstringens may differentially modulate the oxidative metabolism of macrophages and their effect on some chronic inflammatory responses to antigenic exposure is a question that requires further elucidation. Therefore, this study investigated the in vitro effect of different concentrations of the hydroalcoholic extract of S. adstringens on the commercial murine macrophage cell line RAW 264.7, assessing the modulation of pro-oxidant and antioxidant markers, as well as the modulation of the expression of protein and gene pro-inflammatory and anti-inflammatory cytokines in activated macrophages.
Materials and methods
Chemical and equipment
All chemicals were of analytical grade. Acetonitrile (34851), formic acid (F0507), gallic acid (G7384), and caffeic acid (C0625) were purchased from Merck (Darmstadt, Germany). Quercetin (Q4951), catechin (43412), rutin (78095), and kaempferol (60010) were acquired from Sigma Chemical Co. (St. Louis, MO, USA). High-performance liquid chromatography (HPLC)-diode-array detection (DAD) was performed with a Shimadzu Prominence autosampler (SIL-20A) HPLC system (Shimadzu, Kyoto, Japan), equipped with Shimadzu LC-20AT reciprocating pumps connected to a DGU-20A5 degasser with a CBM-20A integrator, SPD-M20A diode array detector and LC solution 1.22 SP1 software.
S. adstringens’s extract characterization
Here, a hydroalcoholic S. adstringens’s extract previously obtained and chemically characterized by Pellenz et al. [7] was used. Briefly, barbatimão’s dried barks were macerated in a 70% ethanol solution and maintained at 4°–8°C temperature for 48 h. Further, the extract was filtered, and the solvent was removed using a rotary evaporator at reduced pressure, 45°C at 115 rpm. From this procedure, a dry extract was obtained by lyophilization and stored at –20°C in a sterile flask and chemically characterized by HPLC-DAD analysis. Briefly, barbatimão extracts at a concentration of 12 mg/mL were injected employing a model SIL-20A Shimadzu autosampler. Separations were carried out using Phenomenex C18 column (4.6 mm × 250 mm × 5 μm particle size). The mobile phase was water with 1% formic acid (v/v; solvent A) and HPLC grade acetonitrile (solvent B) at a flow rate of 0.6 mL/min and injection volume of 40 μL. The sample and mobile phase were filtered through a 0.45 μmol/L membrane filter (Millipore) and then degassed by ultrasonic bath before use. Stock solutions of standards references were prepared in the acetonitrile at a concentration range of 0.030–0.500 mg/mL. The chromatography peaks were confirmed by comparing their retention time with those of reference standards and by DAD spectra (200 nm to 700 nm). All chromatography operations were carried out at room temperature and in triplicate. S. adstringens extract presented follows the main bioactive molecules (mg/g) described in Table 1. Since this study aimed to investigate the modulatory effect of pro- and anti-inflammatory cytokines by S. adstringens on macrophages, a search was also conducted for scientific reviews indicative of the anti-inflammatory activity of each of the main bioactive molecules identified in the extract.
Bioactive molecules present in the S. adstringens hydroalcoholic extract previously characterized by Pellenz et al. [7] and its potential immunomodulatory action on transmissible and/or non-transmissible conditions
Bioactive molecules | Concentration (mg/g) | Scientific evidence |
---|---|---|
Gallic acid | 12.48 ± 0.05 | Attenuation of NF-κβ and MAPK signaling pathways and several pro-inflammatory cytokines, such as TNF-α, IL-1β, and IL-6 [19–21]. Potential cosmetic and dermatological effects involving immunomodulation [22]. |
Caffeic acid | 8.06 ± 0.02 | Potential anti-inflammatory effect on cancer, neurological diseases, and diabetes complications involving attenuation of NF-κβ and TNF-α levels [23, 24]. |
Quercetin | 8.16 ± 0.04 | Potential anti-inflammatory molecule [25]. Reduction in the expression of proinflammatory cytokines in osteoarthritis [26]. Regulation of the TGF-β, α-SMA, ROS, and p-AMPK pathways associated with the inflammatory status of liver fibrosis [27]. Genomic effects on expression of genes and activity of signaling pathways associated with inflammatory status [28]. Therapeutic role in the management of diabetic wounds [29]. |
Rutin | 4.71 ± 0.01 | Antidiabetic effect involving attenuation of proinflammatory cytokines [30]. Rutin reduces the levels of pro-inflammatory markers such as IL-1β, IL-6, TNF-α, and IL-6 blocking NF-κβ and activating MAPK pathways that improve metabolic function [31]. |
Kampferol | 1.37 ± 0.01 | Potential anti-inflammatory molecule [24]. Action on inflammatory status associated with neurodegenerative diseases [32]. |
CatechinEGCG | 5.93 ± 0.013.27 ± 0.02 | Potential anti-inflammatory molecule [24]. Attenuation of cognitive decline and neurodegenerative diseases involving anti-inflammatory action. Beneficial effect on wound healing involving anti-inflammatory action [32, 33]. |
NF-κβ; nuclear factor-kappa beta; MAPK; mitogen-activated protein kinase; TNF-α: tumor necrosis factor-alpha; ROS: reactive oxygen species; TGF-β: transforming growth factor-beta; α-SMA: alpha-smooth muscle actin; p-AMPK: 5’-AMP-activated protein kinase; EGCG: (–)-epigallocatechin-3-gallate
Experimental design and cell culture
In this study, the commercial murine macrophage cell line (RAW 264.7) was employed, obtained from the American Type Culture Collection (ATCC® TIB-71™). These cells were cultured Dulbecco’s modified eagle medium (DMEM) growth medium supplemented with 10% fetal bovine serum (FBS) and 1% antibiotics (penicillin/streptomycin at 100 U/mL) and amphotericin B. Cell cultures were maintained at 37°C, 5% CO2, and 95% humidity, and all experiments were conducted with an initial cell concentration of 1 × 105 cells/mL and were performed in triplicate. Since this study involved in vitro experiments with commercially available cell lines, it did not require prior approval from an ethical research board.
Two complementary protocols were conducted in this study. The first protocol assessed whether different concentrations of S. adstringens could induce an increase in cell proliferation rates, which would indicate potential antigenic effects. In this protocol, we also evaluated the extract’s impact on the modulation of oxidative markers (Figure 1A). Based on the results obtained in the first protocol, and as previously published in a study conducted by Pellenz et al. [7], we further investigated the effect of S. adstringens on cell proliferation and the expression of extracellular proteins and cytokine genes involved in the inflammatory cascade of macrophages activated by exposure to phytohemagglutinin (PHA) (Figure 1B).
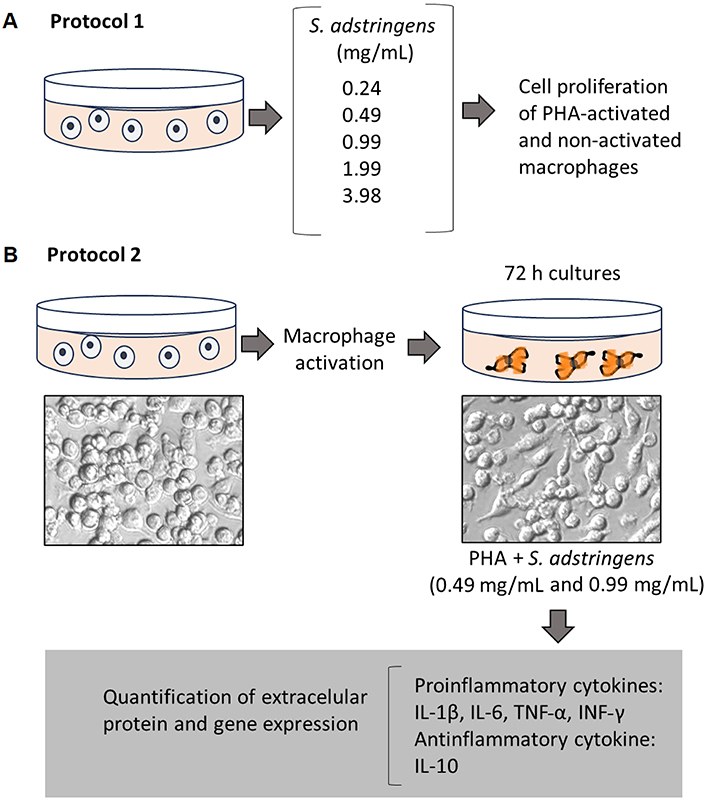
General experimental design of an in vitro study evaluating the potential S. adstringens effect on RAW 264.7 activated macrophages
Cell proliferation assay
Cell culture proliferation was assessed using the 3-(4,5-dimethylthiazol-2-yl)-2,5-diphenyltetrazolic bromide (MTT) spectrophotometric assay, following the method described by Barbisan et al. [34]. In this assay, the cultures were centrifuged, the supernatants from the treatments were aspirated, and the cells were resuspended in phosphate-buffered saline (PBS, 0.01 mol/L; pH 7.4). MTT was dissolved in PBS at a concentration of 5 mg/mL and added (20 µL/well) to a 96-well plate containing the treated samples. The plate was then incubated for 1 h at 37°C, and subsequently, the supernatant was aspirated, and the cells were resuspended in 200 μL of dimethyl sulfoxide (DMSO) per well to dissolve the purple formazan crystals produced during incubation. After centrifugation, the supernatant was transferred to another 96-well plate in triplicate, and absorbance was measured at 560 nm using a SpectraMax M2/M2e multi-mode plate reader (Molecular Devices Corporation, Sunnyvale, CA, USA). The results were expressed as a percentage relative to the untreated control group.
Cytokines immunoassays
Levels of the inflammatory cytokines IL-1β, IL-6, TNF-α, interferon-gamma (IFN-γ), and the anti-inflammatory cytokine IL-10 were quantified in cell culture supernatants via immunoassay using Quantikine Human Kits (R&D Systems, Minneapolis, USA), following the manufacturer’s instructions. The protocol employed closely resembled that outlined by da Cruz Jung et al. [35]. Before the assay, all reagents and working standards were prepared, and any excess microplate strips were removed. Further, 50 µL of RD1W (assay diluent) was added to each well. Following this, 100 µL of standard control or the samples was introduced into the wells, which were then sealed with adhesive strips and incubated for 1.5 h at 25°C. Following incubation, the contents of each well were aspirated, and the wells were washed twice. Next, antiserum specific to each target molecule was added to its respective well.
These wells were resealed with fresh adhesive strips and incubated for 30 min at room temperature. The aspiration and washing steps were repeated once more. Subsequently, 100 µL of the conjugate was added to each well, and the plate was incubated for an additional 30 min at room temperature. Again, aspiration and washing steps were repeated. Then, 100 µL of substrate solution was introduced into each well, and the plate was allowed to incubate at room temperature for 20 min. The assay was terminated by adding 50 µL of stop solution to each well, and the optical density was measured within 30 min using a microplate reader set at 450 nm.
Gene expression assay
Gene expression analysis of inflammatory cytokines including IL-1β, IL-6, TNF-α, IFN-γ, and the anti-inflammatory cytokine IL-10 was conducted using quantitative reverse transcription-polymerase chain reaction (qRT-PCR), following the methodology outlined by Barbisan et al. [34].
Total RNA extraction was performed using TRIzol reagent according to the manufacturer’s instructions (Ludwing Biotec, Brazil). The concentration of extracted RNA was determined using a Thermo Scientific NanoDrop™ 1000 spectrophotometer. For reverse transcription, RNA (1 μg/mL) underwent treatment with 0.2 μL of DNAase (Invitrogen Life Technologies, Carlsbad, CA, USA) for 5 min at 37°C, followed by a 10-min heat treatment at 65°C. Complementary DNA (cDNA) was synthesized using 1 μL of iScript cDNA and 4 μL of Mix iScript (Bio-Rad Laboratories, Hercules, CA, USA). The subsequent steps of the reaction included a 10 min incubation at 5°C, 5 min at 25°C, 5 min at 85°C, and a final 60 min incubation at 5°C.
The qRT-PCR was conducted using the Rotor-Gene Q 5plex high-resolution melting (HRM) technology system (QIAGEN Biotechnology, Germany) with 2× QuantiFast SYBR® Green polymerase chain reaction (PCR) Master Mix (QIAGEN Biotechnology, Germany). The thermal cycling protocol commenced with an initial 3-min step at 95°C, followed by 40 cycles of 10 s at 95°C, 30 s at 60°C, and a melt curve analysis from 60°C to 90°C in 0.5°C increments over 5 s. Each sample underwent triplicate reactions, utilizing 1 μmol/L of each primer, RNase-free water, and 2× QuantiFast SYBR® Green PCR Master Mix (QIAGEN Biotechnology, Germany), resulting in a final volume of 20 μL. The beta-actin gene was employed as the housekeeping gene. Relative expression was quantified using comparative threshold cycle (Ct) analysis and expressed as a fold change relative to control cells. Genes encoding cytokines were amplified using the primers described in Table 2.
Identifier (ID) numbers and amplified fragment lengths of the PCR primers of Mus musculus analyzed
Gene | Gene ID | Primer’s sequence |
---|---|---|
Il-1β | 16176 | F-5’GCGGCATCCAGCTACGAAT3’R-5’ACCAGCATCTTCCTCAGCTTGT3’ |
Il-6 | 16193 | F-5’TACCCCCAGGAGAAGATTCCA3’R-5’CCGTCGAGGATGTACCGAATT3’ |
Tnf-α | 21926 | F-5’CAACGGCATGGATCTCAAAGAC3’R-5’TATGGGCTCATACCAGGGTTTG3’ |
Inf-γ | 15978 | F-5’ACACTGCATCTTGGCTTTGC3’R-5’TGTTGCTGATGGCCTGATTG3’ |
Il-10 | 16153 | F-5’GTGATGCCCCAAGCTGAGA3’R-5’TGCTCTTGTTTTCACAGGGAAGA3’ |
Statistical analysis
The data were statistically analyzed using GraphPad Prism software, version 6.0 (GraphPad Prism Software Company, 2015, San Diego, CA, USA). The comparison between different treatments was performed using a one-way ANOVA followed by the Tukey post-hoc test. These statistical tests were employed because a preliminary analysis using the Kolmogorov-Smirnov method revealed a normal distribution of the data. The results were presented as mean ± standard deviation. Data with P < 0.05 were considered statistically significant and identified in the graphs by letters (Figures 2 and 3).
Results
Initially, the impact of the tested concentrations of S. adstringens on the proliferation of 72-h cultures of non-activated macrophages was assessed. Additionally, in this analysis, cultures activated by exposure to the PHA antigen were included as a positive control, which significantly elevated the cell proliferation rate compared to the negative control. As depicted in Figure 2A, the lower concentrations of extracts (0.24 mg/mL to 0.99 mg/mL) did not alter the pattern of cell proliferation relative to the negative control. However, higher S. adstringens concentrations (1.99 mg/mL and 3.98 mg/mL) decreased the cell proliferation rate, indicating an immunosuppressive or cytotoxic effect (Figure 2A).
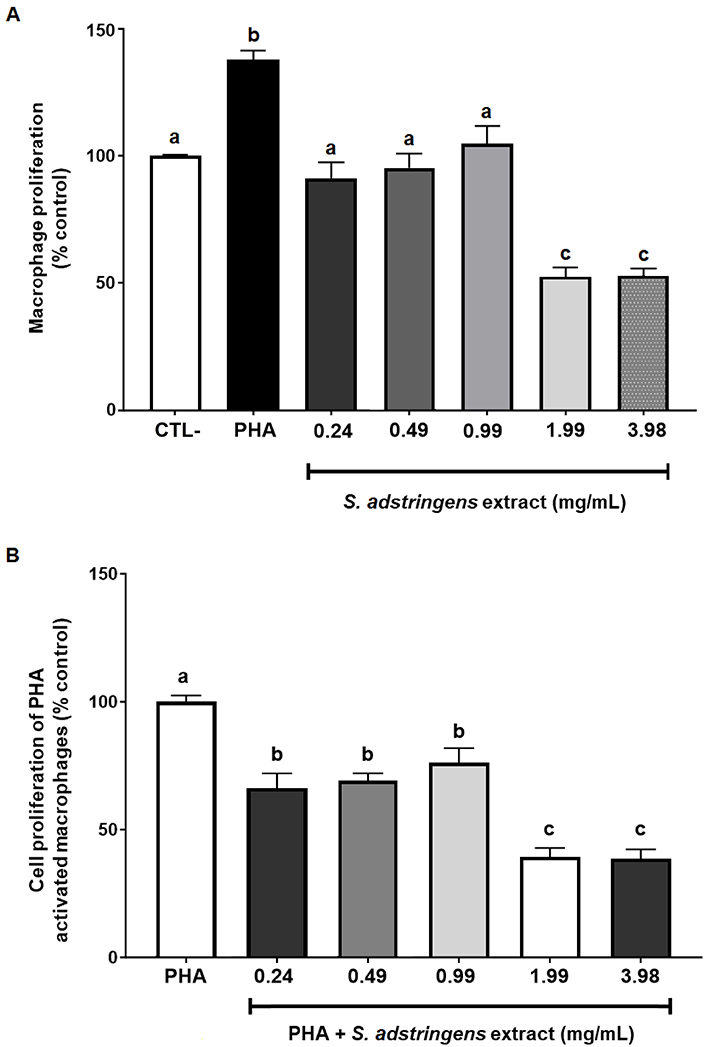
Comparison of cell proliferation rates in 72-h cultures of macrophages exposed to different concentrations of the hydroalcoholic extract of S. adstringens. (A) Influence of the extract on the cell proliferation rate of non-activated macrophages; (B) influence of the extract on the cell proliferation rate in macrophages activated by exposure to the natural antigen (PHA). Data were statistically compared using analysis of variance followed by Bonferroni post-hoc testing. Statistically significant differences from the post-hoc test, with P ≤ 0.05. Different letters represent statistically significant differences between treatments at P < 0.05
Subsequently, macrophages were activated by exposure to PHA, both with and without supplementation of the cultures with S. adstringens. Under these conditions, all tested concentrations significantly reduced the cell proliferation rate. However, this effect was more pronounced in cultures exposed to higher concentrations of S. adstringens (Figure 2B).
Next, we assessed to what extent the hydroalcoholic extract of S. adstringens could modulate the expression of extracellular proteins and the genes associated with pro-inflammatory and anti-inflammatory cytokines in macrophages activated by antigenic exposure. This analysis was conducted at concentrations of 0.49 mg/mL and 0.99 mg/mL, which had demonstrated anti-inflammatory effects as observed in the cell proliferation rate analysis.
In Figure 3, as expected, exposure to PHA increased all pro-inflammatory markers, both protein and gene expression, as following IL-1β (Figure 3A and B), IL-6 (Figure 3C and D), TNF-α (Figure 3E and F), and IFN-γ (Figure 3G and H), while decreased the anti-inflammatory marker IL-10 (Figure 3I and J). On the contrary, exposure to both concentrations of S. adstringens reduced the levels of all pro-inflammatory cytokines assessed in this study, along with their gene expression, increasing the expression of IL-10. However, the effect of the extract concentration on the cytokine levels varied. The levels of IL-1β (Figure 3A), TNF-α (Figure 3E), and IFN-γ (Figure 3G), both in extracellular protein and gene expression, were concentration-dependent concerning S. adstringens. In other words, they decreased more significantly when exposed to higher concentrations of the extract. Conversely, while the reduction in protein levels of IL-6 (Figure 3C) was concentration-dependent, the decrease in the gene expression of this pro-inflammatory cytokine was not influenced by the extract concentration (Figure 3D). On the other hand, exposure to S. adstringens induced a significant increase in the levels and gene overexpression of the anti-inflammatory cytokine IL-10, which occurred independently of its concentration (Figure 3I and J).
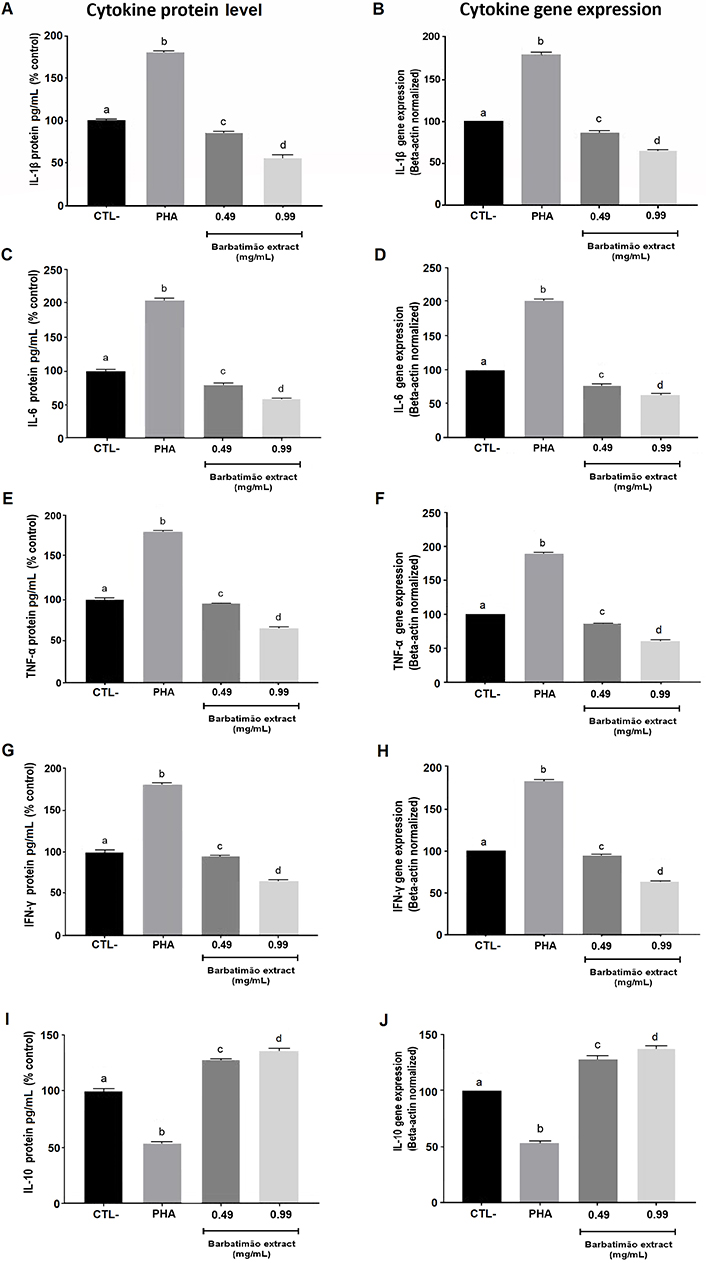
Comparison of extracellular protein concentrations and gene expression of pro-inflammatory cytokines (IL-1β, IL-6, TNF-α, and IFN-γ) and the anti-inflammatory cytokine IL-10 in PHA-activated RAW 264.7 macrophages exposed to two different concentrations of the hydroalcoholic extract of S. adstringens. All gene expression analyses were normalized by the beta-actin housekeeping gene. Gene expression of the negative control sample was used as a reference (100%) in evaluating the gene expression of the samples exposed to PHA and the extracts. The gene expression of the treatments was performed in triplicate, which enabled the calculation of the mean and standard deviation for them and statistical analysis. Statistical comparisons were conducted through analysis of variance followed by Bonferroni post-hoc testing. In each graph different letters represent statistically significant differences between treatments at P < 0.05. CTL-: no treatment
The IL-10 evaluation was the only one concerning anti-inflammatory effects due to its central role in controlling inflammatory processes and preventing tissue impairment. Also, IL-10 is a cytokine that may suppress the M1 phenotype in macrophages, an inflammatory polarization.
Discussion
In this study, the effect of the hydroalcoholic extract of S. adstringens on the modulation of cytokines in macrophages activated by exposure to PHA, considered a natural antigen, was evaluated. A synthesis of the main results found here is presented in Figure 4. The results demonstrated that the extract was capable of reducing the cell proliferation rate, which is a key marker of the inflammatory response, as well as decreasing the protein levels of pro-inflammatory cytokines. Data align with the previous investigation that described the modulatory effect of S. adstringens in the M1/M2 macrophage phenotypes [36].
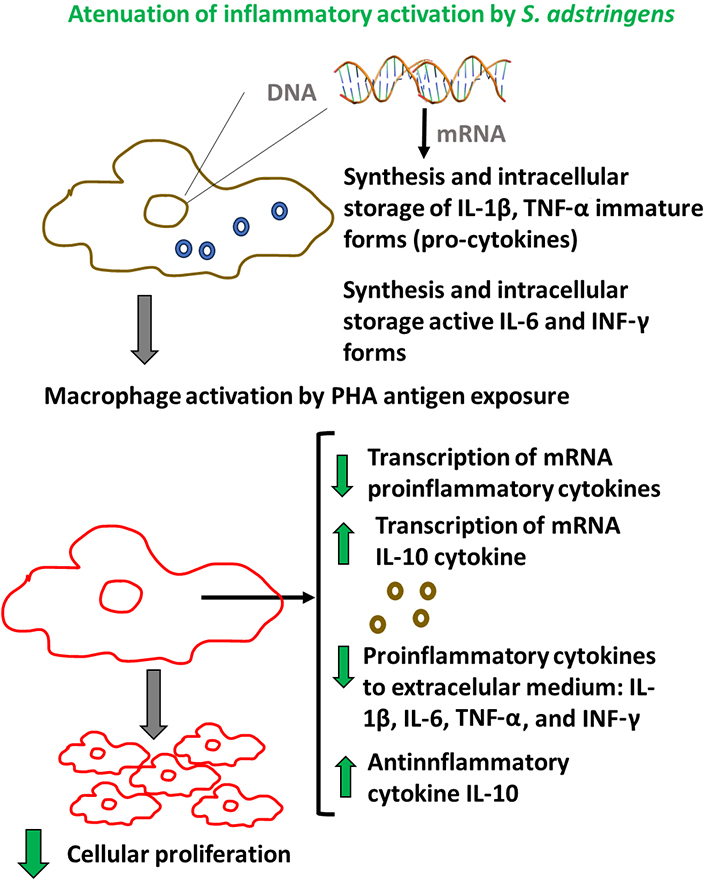
Scheme of main results associated with immunomodulatory in vitro effect of S. adstringens extract on RAW 264.7 activated-macrophages
Conversely, S. adstringens also increased the levels of IL-10, a well-recognized anti-inflammatory molecule. Furthermore, it was observed that the effects of S. adstringens extended beyond the production of mature cytokines released into the extracellular environment, indicating a phyto-genomic effect through the modulation of the gene expression of these cytokines. Notably, the genomic effect of S. adstringens on immune cells such as macrophages had not been previously documented in the literature.
Before discussing the effects of the S. adstringens extract on macrophage activation, it is important to comment on the use of PHA as an activator of the inflammatory response of these immune cells. PHA is a plant-derived agent classified as an exogenous pyrogen able to interact with cells, especially in the immune metabolism, inducing the release of pro-inflammatory cytokines. PHA is a type of lectin, which is a protein that binds to specific carbohydrate structures. It is extracted from plants, most notably from red kidney beans (Phaseolus vulgaris), as well as other legumes. PHA is widely used in cell culture, especially in immunological investigations. It is a potent stimulator of lymphocyte proliferation. When added to cultures of lymphocytes, PHA triggers these cells to enter the cell division cycle, leading to their proliferation. Complementary investigations also showed that PHA could bind in macrophages inducing inflammatory activation [36]. Furthermore, other previous studies have used PHA to verify the effects of plant extracts of bioactive molecules to modulate in vitro macrophage activation [37–39].
Subsequently, pertinent aspects of the data presented here are discussed in the context of the phytotherapeutic action of S. adstringens. In Brazil, extracts of S. adstringens are traditionally and commercially employed due to their cost-effectiveness and significant phytotherapeutic properties. This usage primarily pertains to the healing of acute or chronic wounds. Consequently, the interest in elucidating their mechanisms of action, including their immunomodulatory effects, has increased in recent years.
In this context, Giffoni de Carvalho et al. [40] previously investigated the effect of S. adstringens extract on immune cells from bone marrow cultures, observing a decrease in nitric oxide levels, as well as the pro-inflammatory cytokine IL-6 and other membrane markers associated with macrophage polarization. The results presented here corroborate the findings of these authors who described a significant anti-inflammatory action of S. adstringens. Additionally, our results demonstrated that even at higher concentrations than those tested by Giffoni de Carvalho et al. [40], this extract does not appear to have antigenic effects leading to macrophage activation. These findings are particularly relevant because many commercial phytotherapeutic products containing S. adstringens are formulated as creams with high concentrations of this substance applied to open wounds such as tested here [5].
As established, the inflammatory response triggered by pathogenic or non-pathogenic antigens (especially tissue injuries) initially involves an increase in cytokine levels such as IL-1β and IFN-γ, which drive the inflammatory cascade by elevating the levels of other pro-inflammatory cytokines, particularly IL-6 and TNF-α. In turn, both IL-1β, IL-6, and TNF-α will stimulate relevant physiological changes, such as the dilation of blood vessels and the recruitment of immune cells from the bloodstream to the injured tissue. This initial process amplifies the inflammatory response to eliminate pathogens or reduce debris and cellular remnants from damaged tissues. Subsequently, the levels of pro-inflammatory cytokines begin to decrease as the concentrations of so-called anti-inflammatory cytokines, such as IL-10, increase and promote tissue regeneration [41, 42].
It is important to highlight that cytokines produced by macrophages may be stored intracellularly in the form of immature molecules (pro-cytokines), as is the case with IL-1 and TNF, while there are also cytokines that are already in their mature form, such as IL-6 and TNF-α. It is believed that in non-activated macrophages, there is a relatively constant maintenance in the transcriptional rate, synthesis, and storage of these molecules. Therefore, a specific phytotherapeutics or drug may act in two ways in the macrophage’s inflammatory pathway: by attenuating the release of pro-inflammatory cytokines into the extracellular milieu and by directly affecting the gene expression of these cytokines, which will subsequently be synthesized and stored [43].
In the study conducted here, it became evident that the extract of S. adstringens has an attenuating effect on the release of pro-inflammatory cytokines. However, beyond this effect, the extract also exhibited significant genomic action as it reduced the gene transcription of these cytokines. Therefore, it is possible that the inflammatory response in the presence of S. adstringens, although still present, is attenuated, reducing the likelihood of harmful effects associated with it. This pattern of bioactivity was the opposite in the case of the anti-inflammatory cytokine IL-10, which concurrently increased its concentration in the extracellular medium and gene expression. It is important to emphasize that the decision to analyze a single cytokine as a marker of anti-inflammatory activity was based on the existence of a robust body of evidence demonstrating the strategic role of IL-10 in M1/M2 macrophage polarization [44]. In fact, macrophages are versatile cells of the immune system that play a crucial role in host defense, inflammation, and tissue repair. They exhibit remarkable plasticity, which allows them to adapt to different tissue environments and functional requirements. This adaptability is demonstrated in their ability to polarize into two broad functional categories: M1 and M2 macrophages. M1 macrophages, also known as classically activated macrophages, are typically induced by pro-inflammatory signals like IFN-γ and lipopolysaccharide (LPS). They are associated with the production of pro-inflammatory cytokines (e.g., IL-1β, TNF-α), reactive nitrogen species and ROS, and they play a crucial role in the defense against pathogens and tumor cells. M1 macrophages involved in wound healing, tissue repair, and resolution of inflammation. Secrete anti-inflammatory cytokines (e.g., IL-10) and growth factors, and they help in extracellular matrix remodeling [40, 44–46]. Therefore, IL-10 is considered anti-inflammatory and pro-reparative been modulated by S. adstringens extract.
This dual effect of S. adstringens may explain its significant impact on wound healing, where a rapid inflammatory response is needed to prevent infections while also promoting tissue regeneration. These effects of S. adstringens are possibly associated with its chemical matrix rich in bioactive components described in Table 1, which have anti-inflammatory and cytokine-modulating action previously described in the literature [22–33].
Based on the results described here, despite the methodological limitations associated with in vitro studies, we confirm the immunomodulatory effect of S. adstringens on macrophage activation, observing that this plant extract also has a significant genomic effect. These two actions contribute to the understanding of the causal mechanism of S. adstringens related to its therapeutic action in clinical conditions, such as wound healing. Furthermore, the results described here can serve as support for complementary studies that seek to identify bioactive molecules or chemical matrices of S. adstringens responsible for the potential anti-inflammatory pharmacological action described here.
Abbreviations
DAD: | diode-array detection |
HPLC: | high-performance liquid chromatography |
IL-6: | interleukin 6 |
IFN-γ: | interferon-gamma |
PCR: | polymerase chain reaction |
PHA: | phytohemagglutinin |
S. adstringens: | Stryphnodendron adstringens |
TNF-α: | tumor necrosis factor-alpha |
Declarations
Acknowledgments
The authors thank Dr. Marta Maria Medeiros Frescura Duarte and Bruna Chitolina for their assistance with laboratory analyses.
Author contributions
IBMdC and CFT equally contributed to: Conceptualization, Data curation, Writing—original draft, Funding acquisition. NLP: Resources, Investigation. MHM: Investigation, Writing—review & editing. VFA and FB: Supervision, Writing—review & editing. EER: Resources, Writing—review & editing, Funding acquisition.
Conflicts of interest
Ivana Beatrice Mânica da Cruz who is an Editorial Board Member of Exploration of Drug Science had no involvement in the decision-making or the review process of this manuscript. The other authors declare that they have no conflicts of interest.
Ethical approval
Not applicable.
Consent to participate
Not applicable.
Consent to publication
Not applicable.
Availability of data and materials
The main datasets generated for this study can be found in the OSF storage (https://osf.io/9g8tb/files/onedrive).
Funding
This study is financially supported by Conselho Nacional de Desenvolvimento Científico (CNPq) [301546/2019-3]. The funders had no role in the study design, data collection and analysis, decision to publish, or preparation of the manuscript.
Copyright
© The Author(s) 2024.