Biomimetic 3D bioprinting approaches to engineer the tumor microenvironment
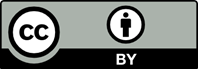
With the increasing incidence and mortality rates, cancer remains a major health challenge in the world. Despite advances in therapies and clinical programs, the efficacy of anti-cancer drugs often fails to translate from pre-clinical models to patient clinical trials. To date, pre-clinical cancer models, including two-dimensional cell cultures and animal models, have limited versatility and accuracy in recapitulating the complexity of human cancer. To address these limitations, a growing focus has fostered the development of three-dimensional (3D) tumor models that closely resemble the in vivo tumor microenvironment and heterogeneity. Recent efforts have leveraged bioengineering technologies, such as biofabrication, to engineer new platforms that mimic healthy and diseased organs, aiming to overcome the shortcomings of conventional models, such as for musculoskeletal tissues. Notably, 3D bioprinting has emerged as a powerful tool in cancer research, offering precise control over cell and biomaterial deposition to fabricate architecturally complex and reproducible functional models. The following review underscores the urgent need for more accurate and relevant 3D tumor models, highlighting the advantages of the use of biofabrication approaches to engineer new biomimetics platforms. We provide an updated discussion on the role of bioengineering technologies in cancer research and modeling with particular focus on 3D bioprinting platforms, as well as a close view on biomaterial inks and 3D bioprinting technologies employed in cancer modeling. Further insights into the 3D bioprinting tissue-specific modeling panorama are presented in this paper, offering a comprehensive overview of the new possibilities for cancer study and drug discovery.
- Fitzmaurice C, Abate D, Abbasi N, et al., 2019, Global, regional, and national cancer incidence, mortality, years of life lost, years lived with disability, and disability-Adjusted life-years for 29 cancer groups, 1990 to 2017: A systematic analysis for the global burden of disease study. JAMA Oncol, 5(12): 1749–1768. https://doi.org/10.1001/jamaoncol.2019.2996
- Siegel RL, Miller KD, Wagle NS, et al., 2023, Cancer statistics, 2023. CA Cancer J Clin, 73(1): 17–48. https://doi.org/10.3322/caac.21763
- Bray F, Ferlay J, Soerjomataram I, et al., 2018, Global cancer statistics 2018: GLOBOCAN estimates of incidence and mortality worldwide for 36 cancers in 185 countries. CA Cancer J Clin, 68(6): 394–424. https://doi.org/10.3322/caac.21492
- Kamb A, 2005, What’s wrong with our cancer models? Nat Rev Drug Discov, 4(2): 161–165. https://doi.org/10.1038/nrd1635
- Pape J, Emberton M, Cheema U, 2021, 3D cancer models: The need for a complex stroma, compartmentalization and stiffness. Front Bioeng Biotechnol, 9, 1–8.
- Sajjad H, Imtiaz S, Noor T, et al., 2021, Cancer models in preclinical research: A chronicle review of advancement in effective cancer research. Anim Model Exp Med, 4(2): 87–103. https://doi.org/10.1002/ame2.12165
- Li M, Song X, Jin S, et al., 2021, 3D tumor model biofabrication. Bio-Design Manuf, 4(3): 526–540. https://doi.org/10.1007/s42242-021-00134-7
- Celikkin N, Presutti D, Maiullari F, et al., 2021, Tackling current biomedical challenges with frontier biofabrication and organ-on-a-chip technologies. Front Bioeng Biotechnol, 9: 732130.
- Murphy SV, Atala A, 2014, 3D bioprinting of tissues and organs. Nat Biotechnol, 32(8): 773–785. https://doi.org/10.1038/nbt.2958
- Kakimi K, Karasaki T, Matsushita H, et al., 2017, Advances in personalized cancer immunotherapy. Breast Cancer, 24(1): 16–24. https://doi.org/10.1007/s12282-016-0688-1
- Balkwill FR, Capasso M, Hagemann T, 2012, The tumor microenvironment at a glance. J Cell Sci, 125(23): 5591–5596. https://doi.org/10.1242/jcs.116392
- Hanahan D, Weinberg RA, 2000, The hallmarks of cancer. Cell, 100(1): 57–70.
- Hanahan D, Robert AW, 2017, Biological hallmarks of cancer. Holland-Frei Cancer Med, 01(April): 1–10. https://doi.org/10.1002/9781119000822.hfcm002
- Hanahan D, Coussens LM, 2012, Accessories to the crime: Functions of cells recruited to the tumor microenvironment. Cancer Cell, 21(3): 309–322. https://doi.org/10.1016/j.ccr.2012.02.022
- Guo S, Deng CX, 2018, Effect of stromal cells in tumor microenvironment on metastasis initiation. Int J Biol Sci, 14(14): 2083–2093. https://doi.org/10.7150/ijbs.25720
- Bussard KM, Mutkus L, Stumpf K, et al., 2016, Tumor-associated stromal cells as key contributors to the tumor microenvironment. Breast Cancer Res, 18(1): 1–11. https://doi.org/10.1186/s13058-016-0740-2
- Wang W, Dai J, Huang Y, et al., 2023, Extracellular matrix mimicking dynamic interpenetrating network hydrogel for skin tissue engineering. Chem Eng J, 457(November 2022): 141362. https://doi.org/10.1016/j.cej.2023.141362
- Meacham CE, DeVilbiss AW, Morrison SJ, 2022, Metabolic regulation of somatic stem cells in vivo. Nat Rev Mol Cell Biol, 23(6): 428–443. https://doi.org/10.1038/s41580-022-00462-1
- Ju F, Atyah MM, Horstmann N, et al., 2022, Characteristics of the cancer stem cell niche and therapeutic strategies. Stem Cell Res Ther, 13(1): 1–17. https://doi.org/10.1186/s13287-022-02904-1
- Papaccio F, Paino F, Regad T, et al., 2017, Concise review: Cancer cells, cancer stem cells, and mesenchymal stem cells: Influence in cancer development. Stem Cells Transl Med, 6(12): 2115–2125. https://doi.org/10.1002/sctm.17-0138
- Winkler J, Abisoye-Ogunniyan A, Metcalf KJ, et al., 2020, Concepts of extracellular matrix remodelling in tumour progression and metastasis. Nat Commun, 11(1): 5120. https://doi.org/10.1038/s41467-020-18794-x
- Lee HM, Lee HJ, Chang JE, 2022, Inflammatory cytokine: An attractive target for cancer treatment. Biomedicines, 10(9): 2116. https://doi.org/10.3390/biomedicines10092116
- Kartikasari AER, Huertas CS, Mitchell A, et al., 2021, Tumor-induced inflammatory cytokines and the emerging diagnostic devices for cancer detection and prognosis. Front Oncol, 11: 692142.
- Tie Y, Tang F, Wei Y, et al., 2022, Immunosuppressive cells in cancer: Mechanisms and potential therapeutic targets. J Hematol Oncol, 15(1): 61. https://doi.org/10.1186/s13045-022-01282-8
- Labani-Motlagh A, Ashja-Mahdavi M, Loskog A, 2020, The tumor microenvironment: A milieu hindering and obstructing antitumor immune responses. Front Immunol, 11: 940.
- Saleh R, Elkord E, 2020, Acquired resistance to cancer immunotherapy: Role of tumor-mediated immunosuppression. Semin Cancer Biol, 65: 13–27. https://doi.org/10.1016/j.semcancer.2019.07.017
- Zhou Y, Sun S, Ling T, et al., 2023, The role of fibroblast growth factor 18 in cancers: Functions and signaling pathways. Front Oncol, 13(May): 1–17. https://doi.org/10.3389/fonc.2023.1124520
- Wang S, Du P, Cao Y, et al., 2022, Cancer associated fibroblasts secreted exosomal miR-1290 contributes to prostate cancer cell growth and metastasis via targeting GSK3β. Cell Death Discov, 8(1): 371. https://doi.org/10.1038/s41420-022-01163-6
- Liu T, Han C, Wang S, et al., 2019, Cancer-associated fibroblasts: An emerging target of anti-cancer immunotherapy. J Hematol Oncol, 12(1): 1–15. https://doi.org/10.1186/s13045-019-0770-1
- Shomar A, Barak O, Brenner N, 2022, Cancer progression as a learning process. iScience, 25(3): 103924. https://doi.org/10.1016/j.isci.2022.103924
- Hoarau-Véchot J, Rafii A, Touboul C, et al., 2018, Halfway between 2D and animal models: Are 3D cultures the ideal tool to study cancer-microenvironment interactions? Int J Mol Sci, 19(1): 181. https://doi.org/10.3390/ijms19010181
- Yada E, Wada S, Yoshida S, et al., 2018, Use of patient-derived xenograft mouse models in cancer research and treatment. Futur Sci OA, 4(3): FSO271. https://doi.org/10.4155/fsoa-2017-0136
- Wu MC, Yu HW, Chen YQ, et al., 2022, Early committed polarization of intracellular tension in response to cell shape determines the osteogenic differentiation of mesenchymal stromal cells. Acta Biomater, 163: 287–301. https://doi.org/10.1016/j.actbio.2022.10.052
- Marcotulli M, Tirelli MC, Volpi M, et al., 2022, Microfluidic 3D printing of emulsion ink for engineering porous functionally graded materials. Adv Mater Technol, 2201244: 1–12. https://doi.org/10.1002/admt.202201244
- Iafrate L, Benedetti MC, Donsante S, et al., 2022, Modelling skeletal pain harnessing tissue engineering. Vitr Model, 1(4– 5): 289–307. https://doi.org/10.1007/s44164-022-00028-7
- Bhimani J, Ball K, Stebbing J, 2020, Patient-derived xenograft models—the future of personalised cancer treatment. Br J Cancer, 122(5): 601–602. https://doi.org/10.1038/s41416-019-0678-0
- Liu Y, Wu W, Cai C, et al., 2023, Patient-derived xenograft models in cancer therapy: Technologies and applications. Signal Transduct Target Ther, 8(1): 160. https://doi.org/10.1038/s41392-023-01419-2
- Singh J, 2012, The national centre for the replacement, refinement, and reduction of animals in research. J Pharm Pharmacol 3(1): 87–9. PMID: 22368436; PMCID: PMC3284057.
- Prina-Mello A, Jain N, Liu B, et al., 2018, Culturing substrates influence the morphological, mechanical and biochemical features of lung adenocarcinoma cells cultured in 2D or 3D. Tissue Cell, 50(December 2017): 15–30. https://doi.org/10.1016/j.tice.2017.11.003
- Cui X, Hartanto Y, Zhang H, 2017, Advances in multicellular spheroids formation. J R Soc Interface, 14(127): 20160877. https://doi.org/10.1098/rsif.2016.0877
- Däster S, Amatruda N, Calabrese D, et al., 2017, Induction of hypoxia and necrosis in multicellular tumor spheroids is associated with resistance to chemotherapy treatment. Oncotarget, 8(1): 1725–1736.https://doi.org/10.18632/oncotarget.13857
- Sontheimer-Phelps A, Hassell BA, Ingber DE, 2019, Modelling cancer in microfluidic human organs-on-chips. Nat Rev Cancer, 19(2): 65–81. https://doi.org/10.1038/s41568-018-0104-6
- Fang L, Liu Y, Qiu J, et al., 2022, Bioprinting and its use in tumor-on-a-chip technology for cancer drug screening: A review. Int J Bioprinting, 8(4): 46–64. https://doi.org/10.18063/ijb.v8i4.603
- Monteiro MV, Zhang YS, Gaspar VM, et al., 2022, 3D-bioprinted cancer-on-a-chip: Level-up organotypic in vitro models. Trends Biotechnol, 40(4): 432–447. https://doi.org/10.1016/J.TIBTECH.2021.08.007
- Cidonio G, Glinka M, Kim Y-H, et al., 2021, Nanocomposite clay-based bioinks for skeletal tissue engineering. In Rainer A, Moroni L, eds. Computer-Aided Tissue Engineering: Methods and Protocols, Springer US, New York, NY, 63–72. https://doi.org/10.1007/978-1-0716-0611-7_6
- Kačarević ŽP, Rider PM, Alkildani S, et al., 2018, An introduction to 3D bioprinting: Possibilities, challenges and future aspects. Materials (Basel), 11(11): 2199. https://doi.org/10.3390/ma11112199
- Menezes R, Vincent R, Osorno L, et al., 2022, Biomaterials and tissue engineering approaches using glycosaminoglycans for tissue repair: Lessons learned from the native extracellular matrix. Acta Biomater, 163: 210–227. https://doi.org/10.1016/j.actbio.2022.09.064
- Groll J, Burdick JA, Cho DW, et al., 2019, A definition of bioinks and their distinction from biomaterial inks. Biofabrication, 11(1): 013001. https://doi.org/10.1088/1758-5090/aaec52
- Gungor-Ozkerim PS, Inci I, Zhang YS, et al., 2018, Bioinks for 3D bioprinting: An overview. Biomater Sci 6(5): 915– 9946. https://doi.org/10.1039/c7bm00765e
- Cidonio G, Glinka M, Dawson JI, et al., 2019, The cell in the ink: Improving biofabrication by printing stem cells for skeletal regenerative medicine. Biomaterials, 209(March): 10–24. https://doi.org/10.1016/j.biomaterials.2019.04.009
- Ji S, Guvendiren M, 2017, Recent advances in bioink design for 3D bioprinting of tissues and organs. Front Bioeng Biotechnol, 5(APR): 1–8. https://doi.org/10.3389/fbioe.2017.00023
- Catoira MC, Fusaro L, Di Francesco D, et al., 2019, Overview of natural hydrogels for regenerative medicine applications. J Mater Sci Mater Med, 30(10): 115. https://doi.org/10.1007/s10856-019-6318-7
- Revete A, Aparicio A, Cisterna BA, et al., 2022, Advancements in the use of hydrogels for regenerative medicine: Properties and biomedical applications. Int J Biomater, 2022: 3606765. https://doi.org/10.1155/2022/3606765
- Suamte L, Tirkey A, Barman J, et al., 2023, Various manufacturing methods and ideal properties of scaffolds for tissue engineering applications. Smart Mater Manuf, 1(November 2022): 100011. https://doi.org/10.1016/j.smmf.2022.100011
- Jung MS, Skhinas JN, Du EY, et al., 2022, A high-throughput 3D bioprinted cancer cell migration and invasion model with versatile and broad biological applicability. Biomater Sci 10(20): 5876–5887. https://doi.org/10.1039/d2bm00651k
- Li J, Chen M, Fan X, et al., 2016, Recent advances in bioprinting techniques: Approaches, applications and future prospects. J Transl Med, 14(1): 1–15. https://doi.org/10.1186/s12967-016-1028-0
- Liu J, Shahriar M, Xu H, et al., 2022, Cell-laden bioink circulation-assisted inkjet-based bioprinting to mitigate cell sedimentation and aggregation. Biofabrication, 14(4). https://doi.org/10.1088/1758-5090/ac8fb7
- Abu Owida H, 2022, Developments and clinical applications of biomimetic tissue regeneration using 3D bioprinting technique. Appl Bionics Biomech, 2022: 2260216. https://doi.org/10.1155/2022/2260216
- Wu Y, Su H, Li M, et al., 2023, Digital light processing-based multi-material bioprinting: Processes, applications, and perspectives. J Biomed Mater Res - Part A, 111(4): 527–542. https://doi.org/10.1002/jbm.a.37473
- Hakobyan D, Médina C, Dusserre N, et al., 2020, Laser-assisted 3D bioprinting of exocrine pancreas spheroid models for cancer initiation study. Biofabrication, 12(3): 035001. https://doi.org/10.1088/1758-5090/ab7cb8
- Vrana NE, Gupta S, Mitra K, et al., 2022, From 3D printing to 3D bioprinting: the material properties of polymeric material and its derived bioink for achieving tissue specific architectures. Cell Tissue Bank, 23(3): 417–440. https://doi.org/10.1007/s10561-021-09975-z
- Chand R, Muhire BS, Vijayavenkataraman S, 2022, Computational fluid dynamics assessment of the effect of bioprinting parameters in extrusion bioprinting. Int J Bioprinting, 8(2): 45–60. https://doi.org/10.18063/ijb.v8i2.545
- van der Heide D, Cidonio G, Stoddart MJ, et al., 2022, 3D printing of inorganic-biopolymer composites for bone regeneration. Biofabrication, 14(4): 042003. https://doi.org/10.1088/1758-5090/ac8cb2
- Cidonio G, Costantini M, Pierini F, et al., 2021, 3D printing of biphasic inks: Beyond single-scale architectural control. J Mater Chem C, 9(37): 12489–12508. https://doi.org/10.1039/D1TC02117F
- Mi S, Yang S, Liu T, et al., 2019, A novel controllable cell array printing technique on microfluidic chips. IEEE Trans Biomed Eng, 66(9): 2512–2520. https://doi.org/10.1109/TBME.2019.2891016
- Kolesky DB, Homan KA, Skylar-Scott MA, et al., 2016, Three-dimensional bioprinting of thick vascularized tissues. Proc Natl Acad Sci U S A, 113(12): 3179–3184. https://doi.org/10.1073/pnas.1521342113
- Hong S, Song JM, 2022, 3D bioprinted drug-resistant breast cancer spheroids for quantitative in situ evaluation of drug resistance. Acta Biomater, 138: 228–239. https://doi.org/10.1016/j.actbio.2021.10.031
- Ling K, Huang G, Liu J, et al., 2015, Bioprinting-based high-throughput fabrication of three-dimensional MCF-7 human breast cancer cellular spheroids. Engineering, 1(2): 269–274. https://doi.org/10.15302/J-ENG-2015062
- Zhou X, Zhu W, Nowicki M, et al., 2016, 3D bioprinting a cell-laden bone matrix for breast cancer metastasis study. ACS Appl Mater Interfaces, 8(44): 30017–30026. https://doi.org/10.1021/acsami.6b10673
- Jiang T, Munguia-Lopez JG, Flores-Torres S, et al., 2017, Directing the self-assembly of tumour spheroids by bioprinting cellular heterogeneous models within alginate/ gelatin hydrogels. Sci Rep, 7(1): 1–9. https://doi.org/10.1038/s41598-017-04691-9
- Grolman JM, Zhang D, Smith AM, et al., 2015, Rapid 3D extrusion of synthetic tumor microenvironments. Adv Mater, 27(37): 5512–5517. https://doi.org/10.1002/adma.201501729
- Reid JA, Palmer XL, Mollica PA, et al., 2019, A 3D bioprinter platform for mechanistic analysis of tumoroids and chimeric mammary organoids. Sci Rep, 9(1): 1–10. https://doi.org/10.1038/s41598-019-43922-z
- Oronsky B, Reid TR, Oronsky A, et al., 2021, A review of newly diagnosed glioblastoma. Front Oncol, 10(February): 1–10. https://doi.org/10.3389/fonc.2020.574012
- Campos DFD, Marquez AB, O’seanain C, et al., 2019, Exploring cancer cell behavior in vitro in three-dimensional multicellular bioprintable collagen-based hydrogels. Cancers (Basel), 11(2): 1–17. https://doi.org/10.3390/cancers11020180
- Dai X, Ma C, Lan Q, et al., 2016, 3D bioprinted glioma stem cells for brain tumor model and applications of drug susceptibility. Biofabrication, 8(4): 1–11. https://doi.org/10.1088/1758-5090/8/4/045005
- Heinrich MA, Bansal R, Lammers T, et al., 2019, 3D-bioprinted mini-brain: A glioblastoma model to study cellular interactions and therapeutics. Adv Mater, 31(14): 1–9. https://doi.org/10.1002/adma.201806590
- Monferrer E, Martín-Vañó S, Carretero A, et al., 2020, A three-dimensional bioprinted model to evaluate the effect of stiffness on neuroblastoma cell cluster dynamics and behavior. Sci Rep, 10(1): 1–12. https://doi.org/10.1038/s41598-020-62986-w
- Yi HG, Jeong YH, Kim Y, et al., 2019, A bioprinted human-glioblastoma-on-a-chip for the identification of patient-specific responses to chemoradiotherapy. Nat Biomed Eng, 3(7): 509–519. https://doi.org/10.1038/s41551-019-0363-x
- Wang X, Zhang X, Dai X, et al., 2018, Tumor-like lung cancer model based on 3D bioprinting. 3 Biotech, 8(12): 1–9. https://doi.org/10.1007/s13205-018-1519-1
- Han S, Kim S, Chen Z, et al., 2020, 3D bioprinted vascularized tumour for drug testing. Int J Mol Sci, 21(8): 1–14. https://doi.org/10.3390/ijms21082993
- Mondal A, Gebeyehu A, Miranda M, et al., 2019, Characterization and printability of sodium alginate - Gelatin hydrogel for bioprinting NSCLC co-culture. Sci Rep, 9(1): 19914. https://doi.org/10.1038/s41598-019-55034-9
- Dong Q, Su X, Li X, et al., 2023, In vitro construction of lung cancer organoids by 3D bioprinting for drug evaluation. Colloids Surf A Physicochem Eng Asp, 666: 131288. https://doi.org/10.1016/j.colsurfa.2023.131288
- Mei Y, Wu D, Berg J, et al., 2023, Generation of a perfusable 3D lung cancer model by digital light processing. Int J Mol Sci, 24(7): 6071. https://doi.org/10.3390/ijms24076071
- Anderson WJ, Doyle LA, 2021, Updates from the 2020 World Health Organization Classification of soft tissue and bone tumours. Histopathology, 78(5): 644–657. https://doi.org/10.1111/his.14265
- Molina ER, Chim LK, Barrios S, et al., 2020, Modeling the tumor microenvironment and pathogenic signaling in bone sarcoma. Tissue Eng Part B Rev, 26(3): 249–271 https://doi.org/10.1089/ten.teb.2019.0302
- Hameed M, Dorfman H, 2011, Primary malignant bone tumors-recent developments. Semin Diagn Pathol, 28(1): 86–101. https://doi.org/10.1053/j.semdp.2011.02.002
- Liu X, Duan Z, Fang S, et al., 2023, Imaging assessment of the efficacy of chemotherapy in primary malignant bone tumors: Recent advances in qualitative and quantitative magnetic resonance imaging and radiomics. J Magn Reson Imaging, 8. https://doi.org/10.1002/jmri.28760
- Palmerini E, Righi A, Staals EL, 2020, Rare primary malignant bone sarcomas. Cancers (Basel), 12(11): 1–14. https://doi.org/10.3390/cancers12113092
- Misaghi A, Goldin A, Awad M, et al., 2018, Osteosarcoma: A comprehensive review. SICOT-J, 4: 12. https://doi.org/10.1051/sicotj/2017028
- Yao M, Zou Q, Zou W, et al., 2021, Bifunctional scaffolds of hydroxyapatite/poly(dopamine)/carboxymethyl chitosan with osteogenesis and anti-osteosarcoma effect. Biomater Sci, 9(9): 3319–3333. https://doi.org/10.1039/D0BM01785J
- Lahr CA, Landgraf M, Sanchez-Herrero A, et al., 2020, A 3D-printed biomaterials-based platform to advance established therapy avenues against primary bone cancers. Acta Biomater, 118: 69–82. https://doi.org/10.1016/j.actbio.2020.10.006
- Chow T, Wutami I, Lucarelli E, et al., 2021, Creating in vitro three-dimensional tumor models: A guide for the biofabrication of a primary osteosarcoma model. Tissue Eng - Part B Rev, 27(5): 514–529. https://doi.org/10.1089/ten.teb.2020.0254
- Delgrosso E, Scocozza F, Cansolino L, et al., 2023, 3D bioprinted osteosarcoma model for experimental boron neutron capture therapy (BNCT) applications: Preliminary assessment. J Biomed Mater Res - Part B Appl Biomater, 111(8): 1571–1580. https://doi.org/10.1002/jbm.b.35255
- Loi G, Stucchi G, Scocozza F, et al., 2023, Characterization of a bioink combining extracellular matrix-like hydrogel with osteosarcoma cells: Preliminary results. Gels, 9(2): 129. https://doi.org/10.3390/GELS9020129
- Sa M-W, Nguyen B-NB, Moriarty RA, et al., 2018, Fabrication and evaluation of 3D printed BCP scaffolds reinforced with ZrO2 for bone tissue applications. Biotechnol Bioeng, 115(4): 989–999. https://doi.org/10.1002/bit.26514
- Zhang W, Zhao W, Li Q, et al., 2021, 3D-printing magnesium–polycaprolactone loaded with melatonin inhibits the development of osteosarcoma by regulating cell-in-cell structures. J Nanobiotechnol, 19(1): 263. https://doi.org/10.1186/s12951-021-01012-1
- Neufurth M, Wang X, Schröder HC, et al., 2014, Engineering a morphogenetically active hydrogel for bioprinting of bioartificial tissue derived from human osteoblast-like SaOS-2 cells. Biomaterials, 35(31): 8810–8819. https://doi.org/10.1016/j.biomaterials.2014.07.002
- Wang X, Tolba E, Schröder HC, et al., 2014, Effect of bioglass on growth and biomineralization of SaOS-2 cells in hydrogel after 3D cell bioprinting. PLoS One, 9(11): e112497. https://doi.org/10.1371/journal.pone.0112497
- Farris AL, Lambrechts D, Zhou Y, et al., 2022, 3D-printed oxygen-releasing scaffolds improve bone regeneration in mice. Biomaterials, 280(June 2021): 121318. https://doi.org/10.1016/j.biomaterials.2021.121318
- Hwang DG, Choi YM, Jang J, 2021, 3D bioprinting-based vascularized tissue models mimicking tissue-specific architecture and pathophysiology for in vitro studies. Front Bioeng Biotechnol, 9(May): 1–16. https://doi.org/10.3389/fbioe.2021.685507
- Kim BS, Cho W-W, Gao G, et al., 2021, Construction of tissue-level cancer-vascular model with high-precision position control via in situ 3D cell printing. Small Methods, 5(7): 2100072. https://doi.org/10.1002/SMTD.202100072
- Franca CM, Athirasala A, Subbiah R, et al., 2023, High-throughput bioprinting of geometrically-controlled pre-vascularized injectable microgels for accelerated tissue regeneration. Adv Healthc Mater, 2202840: 1–11. https://doi.org/10.1002/adhm.202202840
- Swaminathan S, Hamid Q, Sun W, et al., 2019, Bioprinting of 3D breast epithelial spheroids for human cancer models. Biofabrication, 11(2): 025003. https://doi.org/10.1088/1758-5090/aafc49
- Faihs L, Firouz B, Slezak P, et al., 2022, A novel artificial intelligence-based approach for quantitative assessment of angiogenesis in the ex ovo CAM model. Cancers (Basel), 14(17): 4273 6071. https://doi.org/10.3390/cancers14174273
- Parker AL, Benguigui M, Fornetti J, et al., 2022, Current challenges in metastasis research and future innovation for clinical translation. Clin Exp Metastasis, 39(2): 263–277. https://doi.org/10.1007/s10585-021-10144-5
- Ye X, Weinberg RA, 2015, Epithelial-mesenchymal plasticity: A central regulator of cancer progression. Trends Cell Biol, 25(11): 675–686. https://doi.org/10.1016/j.tcb.2015.07.012
- Sökeland G, Schumacher U, 2019, The functional role of integrins during intra- and extravasation within the metastatic cascade. Mol Cancer, 18(1): 1–19. https://doi.org/10.1186/s12943-018-0937-3
- Arvanitis CD, Ferraro GB, Jain RK, 2020, The blood–brain barrier and blood–tumour barrier in brain tumours and metastases. Nat Rev Cancer, 20(1): 26–41. https://doi.org/10.1038/s41568-019-0205-x
- Peinado H, Zhang H, Matei IR, et al., 2017, Pre-metastatic niches: Organ-specific homes for metastases. Nat Rev Cancer, 17(5): 302–317. https://doi.org/10.1038/nrc.2017.6
- Doglioni G, Parik S, Fendt SM, 2019, Interactions in the (pre)metastatic niche support metastasis formation. Front Oncol, 9(MAR): 1–7. https://doi.org/10.3389/fonc.2019.00219
- Khan SU, Fatima K, Malik F, 2022, Understanding the cell survival mechanism of anoikis-resistant cancer cells during different steps of metastasis. Clin Exp Metastasis, 39(5): 715–726. https://doi.org/10.1007/s10585-022-10172-9
- Lugano R, Ramachandran M, Dimberg A, 2020, Tumor angiogenesis: Causes, consequences, challenges and opportunities. Cell Mol Life Sci, 77(9): 1745–1770. https://doi.org/10.1007/s00018-019-03351-7
- Makvandi P, Baghbantaraghdari Z, Zhou W, et al., 2021, Gum polysaccharide/nanometal hybrid biocomposites in cancer diagnosis and therapy. Biotechnol Adv, 48: 107711. https://doi.org/10.1016/j.biotechadv.2021.107711
- Emami Nejad A, Najafgholian S, Rostami A, et al., 2021, The role of hypoxia in the tumor microenvironment and development of cancer stem cell: A novel approach to developing treatment. Cancer Cell Int, 21(1): 62. https://doi.org/10.1186/s12935-020-01719-5
- Lee C, Abelseth E, de la Vega L, et al., 2019, Bioprinting a novel glioblastoma tumor model using a fibrin-based bioink for drug screening. Mater Today Chem, 12: 78–84. https://doi.org/10.1016/j.mtchem.2018.12.005
- Huang J-F, Shen J, Li X, et al., 2020, Incidence of patients with bone metastases at diagnosis of solid tumors in adults: A large population-based study. Ann Transl Med, 8(7): 482–482. https://doi.org/10.21037/ATM.2020.03.55
- Hiraga T, 2019, Bone metastasis: Interaction between cancer cells and bone microenvironment. J Oral Biosci, 61(2): 95–98. https://doi.org/10.1016/j.job.2019.02.002
- Macedo F, Ladeira K, Pinho F, et al., 2017, Bone metastases: An overview. Oncol Rev, 11(1): 321. https://doi.org/10.4081/oncol.2017.321
- Meng F, Meyer CM, Joung D, et al., 2019, 3D bioprinted in vitro metastatic models via reconstruction of tumor microenvironments. Adv Mater, 31(10): 1806899. https://doi.org/10.1002/adma.201806899
- Kingsley DM, Roberge CL, Rudkouskaya A, et al., 2019, Laser-based 3D bioprinting for spatial and size control of tumor spheroids and embryoid bodies. Acta Biomater, 95: 357–370. https://doi.org/10.1016/j.actbio.2019.02.014
- Zhu W, Holmes B, Glazer RI, et al., 2016, 3D printed nanocomposite matrix for the study of breast cancer bone metastasis. Nanomed Nanotechnol Biol Med, 12(1): 69–79. https://doi.org/10.1016/j.nano.2015.09.010
- Dai X, Liu L, Ouyang J, et al., 2017, Coaxial 3D bioprinting of self-assembled multicellular heterogeneous tumor fibers. Sci Rep, 7(1): 1–12. https://doi.org/10.1038/s41598-017-01581-y
- Wang X, Li X, Dai X, et al., 2018, Coaxial extrusion bioprinted shell-core hydrogel microfibers mimic glioma microenvironment and enhance the drug resistance of cancer cells. Colloids Surf B Biointerfaces, 171(April): 291–299. https://doi.org/10.1016/j.colsurfb.2018.07.042
- Hermida MA, Kumar JD, Schwarz D, et al., 2020, Three dimensional in vitro models of cancer: Bioprinting multilineage glioblastoma models. Adv Biol Regul, 75: 100658. https://doi.org/10.1016/j.jbior.2019.100658