Impact of irrigation on vulnerability of winter wheat under extreme climate change scenario: a case study of North China Plain
- 1Key Laboratory of Environmental Change and Natural Disaster, Ministry of Education, Faculty of Geographical Science, Beijing Normal University, Beijing, China
- 2Department of Biological Systems Engineering, University of Nebraska-Lincoln, Lincoln, NE, United States
An inadequate understanding of the impacts of adaptation countermeasures tends to exaggerate the adverse effects of climate change on agricultural systems. Motivated by proposing reasonable climate change adaptation countermeasures, the present study applied the EPIC model to quantify the impacts of climate change and irrigation changes with future socioeconomic development on agricultural production. Winter wheat yield losses using dynamic irrigation parameters in the North China Plain (NCP) from 2010 to 2099 under a scenario coupling climate change and future socioeconomic development (RCP8.5-SSP3), and those under an extreme climate change scenario (RCP8.5), were simulated. Results show that EPIC model demonstrates superior performance in simulating winter wheat yields in NCP (RMSE = 12.79 kg/ha), with the distribution of simulated and observed yields is relatively consistent. The winter wheat yield loss in the NCP was high in the south and low in the north. The yield loss rate of winter wheat was 0.21 under the RCP8.5-SSP3 scenario, compared with 0.35 under the RCP8.5 scenario, indicating a superior climatic adaptation of irrigation. However, under the RCP8.5-SSP3 scenario, the yield loss rate increased from 0.17 in the near term to 0.26 in the long term, implying the benefits of irrigation will be diminished with long-term climate change. It is noteworthy that yield improvement was facilitated by irrigation in part of the NCP (accounting for 14.6% area), suggesting that irrigation may lead to an increase in winter wheat yields in some regions even under extreme climate change conditions. This study highlights the significance of quantitatively revealing the benefits and limitations of adaptive countermeasures which could assist in enhancing climate change adaptation while preserving a sustainable agricultural system.
1 Introduction
Climate change poses a great threat to global food security (Howden et al., 2007; Ault et al., 2014; Trenberth et al., 2014; Lesk et al., 2016; Hasegawa et al., 2018; Nelson et al., 2018; Dasgupta and Robinson, 2022), and significant reductions in global wheat production with increasing temperature were widely reported (Asseng et al., 2015; Chavez et al., 2015; Sendhil et al., 2018; Ray et al., 2019; Verschuur et al., 2021). However, the adverse effects of climate change could be substantially offset by applying adaptation activities (Piao et al., 2010; Chavez et al., 2015; Zhang et al., 2015; Etwire et al., 2022). While previous studies have tended to underestimate the contribution of adaptation activities, and therefore may overestimate the adverse effects of climate change on grain production (Howden et al., 2007; Liu et al., 2010; Ding Y. et al., 2021). In sight of this, quantitatively integrating the effects of adaptation activities in the evaluation of climate change impacts on grain production has not yet been well explored (Tubiello et al., 2007; Sloat et al., 2020).
Empirical statistics based on historical data and mechanistic crop models have been widely acknowledged among various studies evaluating the impact of climate change on grain production (Asseng et al., 2015). The former assesses the impacts of climate change on grain production using statistical analysis approaches based on historical observations (You et al., 2009; Lobell et al., 2011; Attiaoui and Boufateh, 2019; Mahmood et al., 2019; Ray et al., 2019; Chandio et al., 2021). For example, using this approach, it was reported that historical climate trends have led to global declines in maize and wheat yields of 3.8% and 5.5%, respectively (Lobell et al., 2011). However, this empirical statistical approach is limited by the quality of historical observations, making it difficult to apply to regions with insufficient and low-quality historical data. More importantly, this approach lacks the ability to capture the effects of adaptation activities on grain yield under climate change.
Crop models have also been widely applied to quantitatively simulate the impact of future climate change on grain production (White et al., 2011; Rosenzweig et al., 2014; Gammans et al., 2016; Lobell and Asseng, 2017). However, previous studies have tended to underestimate the positive effects of adaptation activities when applying crop models to assess the effects of climate change on grain production (Piao et al., 2010; Janssens et al., 2020; Hasegawa et al., 2021). This problem arises from the neglect or simplification of field management practices in the parameter settings of crop models (Deryng et al., 2011; Balkovič et al., 2014; Asseng et al., 2015; Yin et al., 2015; Yue et al., 2015; Liu et al., 2016; Wang et al., 2018; Ahmad et al., 2020). Previous studies tended to directly employ the default parameters of field management practices (Yin et al., 2015; Yue et al., 2015), or directly disregard water stress (Deryng et al., 2011; Balkovič et al., 2014; Liu et al., 2016), or utilize current field management practices parameters and apply to future scenarios (Asseng et al., 2015; Ahmad et al., 2020), or utilize model automatic parameter settings (Ahmad et al., 2019), or set field practices (irrigation volume) parameters using scenario assumptions (Zhu et al., 2021). For example, Ahmad et al. (2019) use an automated irrigation schedule generated by the Aquacrop irrigation module to estimate the future net irrigation water requirement in the case of no water stress. And Zhu et al. (2021) employed irrigation scenario assumptions, which set up two scenarios, i.e., full irrigation and rain-fed, and full irrigation means that irrigation was immediately triggered once a water shortage occurred. The simplified processing of the field management parameters can lead to an overestimation of the impact of climate change on grain production (Piao et al., 2010; Ding Y. et al., 2021), without properly addressing the role of adaptation activities in coping with climate change (Yue et al., 2018).
Among various stressors, water appears to be the primary one affecting grain production (Lobell, 2014; Zipper et al., 2016; Lehner et al., 2017). Irrigation parameters also usually be simplified, although irrigation is usually a key involved in crop models to simulate grain yield responses to climate change. For example, default irrigation parameters are used (Leng et al., 2015), or different irrigation levels are set directly, or determined irrigation is based on scenarios assumption (Zhang et al., 2022), or predicted irrigation is based on historical irrigation-crop yield relationships (Leng et al., 2016). The aforementioned improper settings for future irrigation in crop model simulation research will lead to significant uncertainty in assessing the impact of climate change on grain production. In practice, irrigation varies continuously. The above parameters setting failed to capture the variation in irrigation under climate change and socioeconomic development.
In summary, few studies have considered the role of dynamically adapting irrigation under socioeconomic development when using crop models to assess the impact of climate change on crop production. Therefore, it is important to accurately assess the capacity of adaptation activities to minimize uncertainty in grain yield simulations under climate change. For this purpose, and to provide important scientific support for the promotion of rational climate change adaptation strategies, this study utilized the EPIC model to quantitatively reveal the effects of irrigation on winter wheat yields in the NCP through the coupling of extreme climatic and socioeconomic scenarios.
The specific objectives are as follows: (1) to identify irrigation evolution with future socioeconomic development scenarios. (2) to reveal the ability of irrigation to potentially adapt to extreme climate change for winter wheat in the NCP. (3) to find out the benefits and limitations of irrigation as a potential strategy for adaptation to climate change.
2 Methodology
2.1 Basic idea
The NCP is one of the most densely irrigated regions globally (Zhang et al., 2022), with relatively low annual precipitation (Qu et al., 2019; Yue et al., 2022). Water scarcity in irrigation (Sun H. et al., 2019) and significant over-exploitation of groundwater (Yan et al., 2021) have posed a great threat to agricultural production and the ecological environment in NCP. To address these challenges, the Chinese government has implemented a series of policies in the NCP (Kumar Jha et al., 2019; Sun H. et al., 2019; Sun Z. et al., 2019; Koch et al., 2020; Yan et al., 2021; Shi et al., 2022). For example, groundwater extraction permits (Li et al., 2019; Si et al., 2020; Feng et al., 2021), and water-saving irrigation technologies (e.g., drip irrigation, sprinkler irrigation, and underground irrigation systems) were promoted.
Timely and appropriate irrigation often shows a positive correlation with grain production (Mwaura and Muwanika, 2018; Bjornlund et al., 2020; Foster et al., 2020; Gómez-Limón et al., 2021; Kafle et al., 2022). To reflect the effect of irrigation on crop yield as a response to climate change, dynamic irrigation parameters should be used in crop growth simulations. However, dynamic irrigation parameters are related to a number of factors. Among these, agricultural production usually keeps pace with socioeconomic development (Fischer et al., 2005; Ali et al., 2017; Li et al., 2021). The effectiveness of national policies and water-saving irrigation technologies applied in the NCP are often manifested through financial investments (Kassie and Alemu, 2021), which are closely intertwined with regional economic conditions. Specifically, we argue that irrigation mainly depends on the irrigation payment ability which is often closely related to Gross Domestic Product (GDP), under the conditions of water resource availability and national policies. In light of this, we regard GDP as a proxy of socioeconomic development, and future irrigation under various socioeconomic development scenarios could be projected referring to the relationship of irrigation and GDP in the historical period.
Based on the above, the research objectives of this study were achieved through the following steps: (1) the NCP was selected as study area (Section 2.2), (2) Irrigation was predicted by the relationships between irrigation guarantee rate and GDP (Section 2.4.1), (3) Winter wheat yield was simulated by well-calibrated and verified EPIC model (Section 2.4.2), (4) Impact of irrigation on winter wheat to extreme climate change scenario was revealed by comparing the yield losses of winter wheat under the RCP8.5-SSP3 scenario to RCP8.5 scenario (Section 2.4.3).
2.2 Study area
As the most important grain production area in China, the North China Plain (32°00'−40°24'N, 112°48'−122°45'E) includes southern Beijing, Tianjin, Shandong, eastern Henan, south-central Hebei, northern Anhui, and northern Jiangsu, with a total area of 4.54 × 105 km2 (Figure 1).
Most of the NCP is below an elevation of 50 m (Li et al., 2015). There is high thermal variability in the NCP; the sunlight duration is approximately 2300–2500 h per year, with an average annual temperature of 8–15°C. Precipitation decreases continuously from east to west, with an average annual precipitation of 600 mm to −800 mm (Wang et al., 2018; Yue et al., 2022; Yu et al., 2022).
2.3 Data sources
Data applied in the present study are shown in Table 1.
Meteorological data including historical observations (1960–2011) and meteorological projections of RCP scenarios (2010–2099). The historical observations download from the National Weather Science Data Center (http://data.cma.cn/), which was processed with careful quality control and error checks. The projected meteorological data at a spatial resolution of 0.5 × 0.5o from 1971 to 2099, was driven by the GFDL-ESM2M model. This dataset was bias-corrected and there were no missing data has been applied in our prior studies (Yue et al., 2018, 2019, 2022; Jiang et al., 2019). Among the RCP scenarios, the RCP8.5 scenario was selected in this study, representing an extreme climate scenario of a future development path without significant emission reduction measures (Schwalm et al., 2020).
Socioeconomic development data including historical statistics data (2000–2009) and the Shared Socioeconomic Pathways (SSPs) scenarios data (2005–2099). The socioeconomic historical statistics data were obtained from the socioeconomic statistics from prefecture-level statistical yearbooks (http://tongji.cnki.net/kns55/Navi/NaviDefault.aspx). The Shared Socioeconomic Pathways (SSPs) scenarios data quantified with Integrated Assessment Models (IAMs) (https://tntcat.iiasa.ac.at/SspDb/dsd?Action=htmlpage&page=about), were selected to represent socioeconomic development (Riahi et al., 2017; Rogelj et al., 2018). These scenarios provide qualitative and quantitative descriptions of alternative socioeconomic developments from 2005 to 2099. The quantitative elements provide data accompanying the scenarios on national population, educational attainment, urbanization and gross domestic product (GDP) per capita. Specifically, the GDP of the SSP3 scenario was applied as one of the socioeconomic development indicators.
The data of winter wheat yield (1999–2011) and field management (including sowing date, harvest date, effective irrigation area) (2000–2009) in the NCP was download from the online database of the China Meteorological Data Service Center of China Meteorological Administration (http://data.cma.cn/), and China National Knowledge Infrastructure (CNKI) (https://data.cnki.net/). These dataset were processed with careful quality control and error checks.
The dataset of Global Harvested Area Fractional for Wheat obtained from Sustainability and the Global Environment (SAGE) provides the winter wheat harvested area ratio data in NCP of 2010, with a resolution of 5′×5′ (http://nelson.wisc.edu/sage/data-and-models/crop-calendar-dataset/index.php). And the Global Agro-Ecological Zones dataset was adopted as a classification reference for agricultural zones.
All data were preprocessed at a spatial resolution of 0.5 × 0.5o, and the geographic coordinate system was WGS1984.
2.4 Methods
2.4.1 Irrigation prejection
In the present study, the irrigation guarantee rate (IGR) is selected as the indicator to reflect the irrigation capacity. We further assume that the future IGR can be predicted following the relationship between historical IGR and GDP. The future IGR is quantified by Equation 1.
Where IGRi is the predicted IGR in the future year i, and GDPi is the GDP in the future year i, N is a constant of the IGR and GDP growth ratio during the historical time period.
N is expressed by Equation 2.
Where GDPh is the growth rate of GDP and IGRh is the growth rate of IGR in historical period, respectively.
GDPh and IGRh can be calculated by Equations 3 and 4.
Where GDPt1 and GDPt2 represent the GDP in the start year t1 and end year t2 during a historical time period, respectively. And, IGRt1 and IGRt2 represent the IGR in the start year t1 and end year t2, correspondingly.
In this study, the Representative Concentration Pathway (RCP) 8.5 scenario represented an extreme climate change scenario, and the Shared-Socioeconomic Pathway (SSP) 3 scenario was selected as a socioeconomic development scenario corresponding to RCP8.5. The historical period of 2000–2009 was defined as the baseline, and the future period is 2010–2099.
It cannot be denied that future irrigation will not necessarily follow the 1991–2009 rules, though we predicted future IGR according to the relationship between historical IGR and GDP. Furthermore, Irrigation is not the only adaptation countermeasure to climate change on winter wheat yield production, and its effects should be comprehensively understood.
2.4.2 Winter wheat yield simulation
2.4.2.1 EPIC model
The EPIC model was adopted to simulate winter wheat yield under climate change.
The EPIC model is capable of simulating crop growth at different spatial scales, including station scale, field scale, and regional scale. Besides, EPIC simulates approximately eighty crops with one crop growth model using unique parameter values for each crop (Williams et al., 1984, 1989; Steduto et al., 1995; Williams, 1997). Together with its multi-scale suitability, high regional simulation accuracy can be obtained by the well-calibrated EPIC model using the observed parameters on the station.
Furthermore, EPIC is capable of predicting the effects of field management parameters, and their combined impact on crop yields for areas (Ko et al., 2009; Chavez et al., 2018; Yang et al., 2019; Feng et al., 2021). For example, simulating the variance of soil water and its effects on crop production. Therefore, EPIC is one of the predominant crop models in the world as an effective decision-support tool in irrigation allocation and scheduling.
Based on the above merits, the EPIC model was adopted in the present and our previous studies to reveal the effects of water utilization on crop yield and disaster risk under different climate change scenarios (Yue et al., 2015, 2018, 2022).
2.4.2.2 Model calibration and validation
Model calibration assists in validating the wheat varieties identified by genetic parameters within the EPIC model. This study used the Shuffled Complex Evolution method developed at the University of Arizona (SCE-UA) (Duan et al., 1993; Zhang et al., 2009) to calibrate the winter wheat sensitivity parameters, to obtain the optimal parameter combinations for each agricultural region in the NCP.
The calibration principle is to minimize the total error between the simulated yield and the actual yield in the same year for a typical site. The NCP was divided into four agricultural regions for parameter calibration, and the calibrated parameters of each agricultural region please refer to Table 2 in our prior study (Yue et al., 2022).
In the present study, the relative root mean square error (RMSE) of the model simulations was adopted to assess the EPIC model performance. The specific evaluation variable is as follows:
Where Xi indicates the observed data, Yi indicates the simulated data, and n denotes the number of samples.
2.4.2.3 Model application
Using the carefully calibrated and validated EPIC model, and taking historical (baseline period: 2000–2009) meteorological data, extreme climate scenario (RCP8.5) data, extreme climate scenario (RCP8.5) and the irrigation data driven by future socio-economic scenario (SSP3) as inputs, the yields of winter wheat under the historical period, RCP8.5 scenario, and RCP8.5-SSP3 combination scenario were obtained respectively. Furthermore, the yields of winter wheat under the RCP8.5 scenario, i.e., yield under extreme climate change, and that under the RCP8.5-SSP3 combined scenario, i.e., yield considering irrigation adaptive measures with economic development, were used to evaluate the benefits and limitations of irrigation on adaptation to climate change of winter wheat production in the NCP.
2.4.3 Winter wheat yield loss assessment
The impacts of irrigation on winter wheat production under future climate change were quantitatively assessed by comparing simulated winter wheat yield losses with/without considering dynamic irrigation parameters. Two kinds of winter wheat yield loss were defined, i.e., physical and comprehensive yield losses. The former is defined as the winter wheat yield loss caused by climate change (Uzielli et al., 2008; Papathoma-Köhle et al., 2011; Quan Luna et al., 2011). The latter is defined as winter wheat yield loss under an irrigation adaptive climate change scenario.
Specifically, physical winter wheat yield loss represents the impact of the unmitigated/extreme climate change scenario, i.e., the RCP8.5 scenario without considering irrigation. While, the comprehensive yield loss of winter wheat under the RCP8.5-SSP3 scenario, which was simulated using dynamic irrigation parameters, i.e., predicted IGR under the SSP3 scenario according to Equations 1-4, represent the impact of adaptive irrigation under socioeconomic development.
Taking the 95% maximum annual wheat yield (Sloat et al., 2020) from 2000 to 2009 as the baseline, the physical winter wheat yield loss (Ylossp) caused by climate change is calculated as:
Where Ybase is the annual baseline yield and YRCP8.5 is the annual winter wheat yield under RCP8.5.
Accordingly, the comprehensive winter wheat yield loss (Ylossc) is calculated as:
where YRCP8.5−SSP3 is annual winter wheat yield under the RCP8.5-SSP3 scenario considering irrigation.
The winter wheat yield losses were grouped into four classes, i.e., very slight (Yloss < 0.3), slight (0.3 ≤ Yloss < 0.5), moderate (0.5 ≤ Yloss < 0.7), and severe (Yloss ≥ 0.7), according to the principle of minimum differences within groups and maximum differences between groups. Besides, the projection period was divided into three periods, i.e., near-term (2010–2039), medium-term (2040–2069), and long-term (2070–2099) term (Carew et al., 2018; Xiao et al., 2018), which will help us to specifically understand the trends over time.
3 Results
3.1 EPIC model calibration and validation
The simulation validation results of winter wheat yield shown in Figure 2 and Table 2. The validation results indicate that our model has good applicability in NCP, with the average RMSE is 12.79 kg/ha (Table 2). Comparing the observed and simulated winter wheat yield in NCP (Figure 2A), the results indicate that the distribution of simulated and observed yields is relatively concentrated, uniformly distributed around the 1:1 line. Further exploration of the data distribution and variability of observed and simulated yields was conducted (Figure 2B). The results indicate that the data distribution ranges of the two yields exhibit a similar normal distribution, with low variability in simulated yields. The above results imply that the EPIC model demonstrates superior performance in simulating winter wheat yields in NCP.
3.2 Physical winter wheat yield loss
Compared to baseline, the Ylossp of winter wheat in the NCP under the RCP8.5 scenario averaged 0.35 (calculated by Equation 6) during 2010–2099. Among which, winter wheat yield loss reaches moderate and severe levels (Ylossp ≥ 0.5) account for 21.1% (Figure 3). This implies that the winter wheat production in the NCP is expected to suffer a significant reduction under the RCP8.5 scenario.
The Ylossp of winter wheat in the NCP varied significantly in the near-, medium-, and long-periods (Figure 3). The average values of Ylossp were 0.32, 0.32, and 0.42 in the corresponding time periods. In particular, the areas with a Ylossp reaching severe level (Ylossp ≥ 0.7) are 11.4%, 11.3%, and 19.2%, respectively. This finding increased by 68.4% in the long-term than that in the near-term. The proportions of Ylossp below the very slight level (Ylossp ≤ 0.3) are 59.9%, 58.3%, and 29.6% in the near-, medium-, and long-term, respectively, and decreased by 50.6% in the long-term compared to the near-term.
These results indicate that winter wheat yield loss in the NCP tend to increase severely over time under the RCP8.5. Specifically, yield loss increased significantly over the long-term, with 27.0% of the areas experiencing a yield loss of over moderate degree (Ylossp ≥ 0.5). This finding implies that climate change may cause a catastrophic strike in food security in China, without taking reasonable adaptation countermeasures.
Spatially, the Ylossp of winter wheat in the NCP is higher in the south and lower in the north, and its spatial distribution is significantly diverse. Areas with Ylossp exceeding severe degree (Ylossp ≥ 0.7) account for 13.1% of the winter wheat-planting areas and are primarily distributed in southern of the NCP, i.e., northern Anhui and Jiangsu, and southeastern Henan. In contrast, the areas with Ylossp below very slight level (Ylossp ≤ 0.3) account for 51.9% and are primarily distributed in the center and northern regions, specifically eastern Shandong and central Hebei (Figure 4A). The Ylossp increased over time from the south to north (Figures 4B–D), with the majority of areas with Ylossp exceeding severe degree (Ylossp≥ 0.7) concentrated in the south.
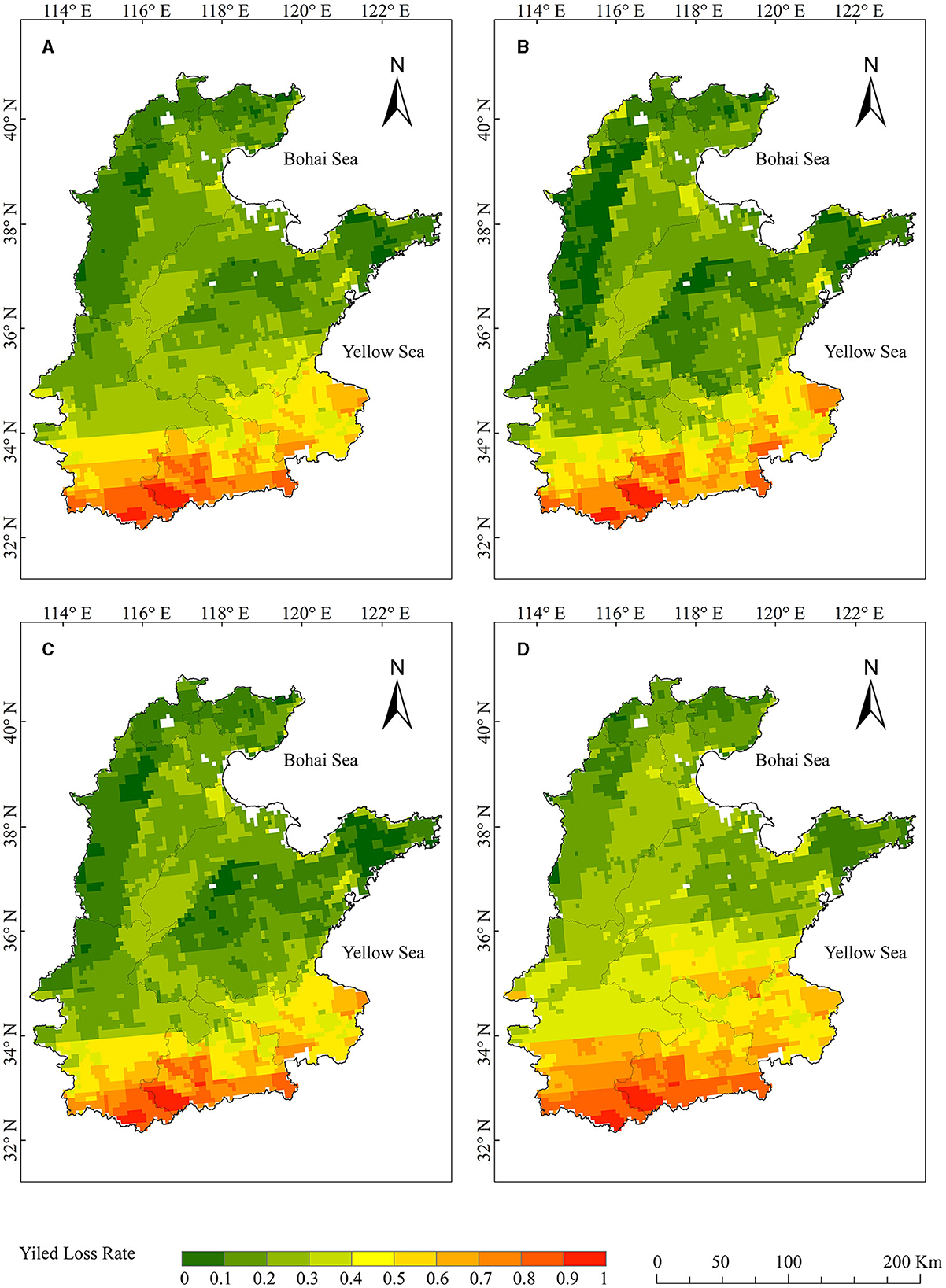
Figure 4. Spatial distribution of wheat physical yield loss rate for 2010–2099 under RCP8.5 scenario [(A) average, (B) near-term, (C) medium-term and (D) long-term].
The above results indicate that under the RCP8.5 scenario, regions that are initially less susceptible to winter wheat are prone to yield loss. In particular, winter wheat south of the NCP may suffer significant yield loss by the end of this century.
3.3 Comprehensive winter wheat yield loss
Integrated climate change (RCP8.5) and socioeconomic development (SSP3) (i.e., the RCP8.5-SSP3 scenario), the Ylossc of winter wheat average approached 0.21 (calculated by Equation 7) from 2010 to 2099 compared to baseline. Among them, the Ylossc of exceeding moderate degree (Ylossc ≥ 0.5) occupied 11.4%, which decreased by 46% to that of Ylossp (Figure 5). This implies that irrigation adjusts to socioeconomic can significantly mitigate the negative impacts of climate change on the yield loss of winter wheat in the NCP.
The Ylossc of winter wheat under irrigation increased incrementally over time (Figure 5). The Ylossc of winter wheat in the NCP in the near-, medium-, and long-term were 0.17, 0.19, and 0.26, respectively. Specifically, proportions of Ylossc exceeding moderate level (Ylossc ≥ 0.5) increase from 1.9 in the near-term to 11.4% in the long-term, indicating that irrigation cannot entirely offset winter wheat yield loss, despite the fact that irrigation effectively mitigates the negative effects of climate change in the majority of winter wheat-planting areas.
Compared to Ylossp, the average Ylossc under irrigation significantly decreased by 40% from to 2010–2099. Of these, Ylossc in the near-term, medium-term, and long-term decreased by 15%, 13%, and 16%, respectively. Moreover, the areas with a winter wheat yield loss above moderate degree (Ylossc ≥ 0.5) decreased from 21.1% under RCP8.5 to 6.1% under RCP8.5-SSP3, i.e., decreased by 85.3%. Our findings indicate that irrigation, as an adaptive activity to climate change, can significantly mitigate the yield loss of winter wheat even under extremely climate change scenarios, that is, adaptive activities play a key role in ensuring agricultural production.
Under the RCP8.5-SSP3 scenario, the Ylossc of winter wheat in the NCP under irrigation were also higher in the south and lower in the north. The proportions of Ylossc exceeding severe degree tended to decrease from 90.8 to 84.6 and 79.0% in the near-, medium-, and long-term, respectively.
The proportions that encountered Ylossc in the NCP account for 85.4% on average. However, the proportions with Ylossc exceeding severe degree only account for 1.5%, which tends to increase from 1.4%, 1.6%, to 6.0% in the near-, medium-, and long-term, respectively. In contrast, the proportions with Ylossc below very slight level account for 78.3%, which tended to decrease from 85.6%, 80.5% to 64.7% in the near-, medium-, and long-term, respectively. Compared with that of the near-term, the proportions with Ylossc below very slight degree decreased by 24.4%, and the proportions with Ylossc exceeding severe degree increased triples in the long-term.
At the same time, winter wheat yields increase were observed in 14.6% of the NCP areas from 2010 to 2099 on average. It is mainly distributed in the north-central and eastern regions of NCP, i.e., the areas with Ylossc are negative (Figure 6). Compared with baseline, the proportions with increased winter wheat yields accounted for 9.2%, 15.4% to 21.0% in the near-, medium- and long-term, respectively. These findings imply that irrigation may potentially be capable of offset the negative impacts of climate change. However, the proportions that encountered winter wheat yield loss in the NCP was still significantly higher than those of the yield increased. In addition, yield loss tends to be exacerbated over time, specifically, the decrease in the low and the increase in the high yield loss areas.
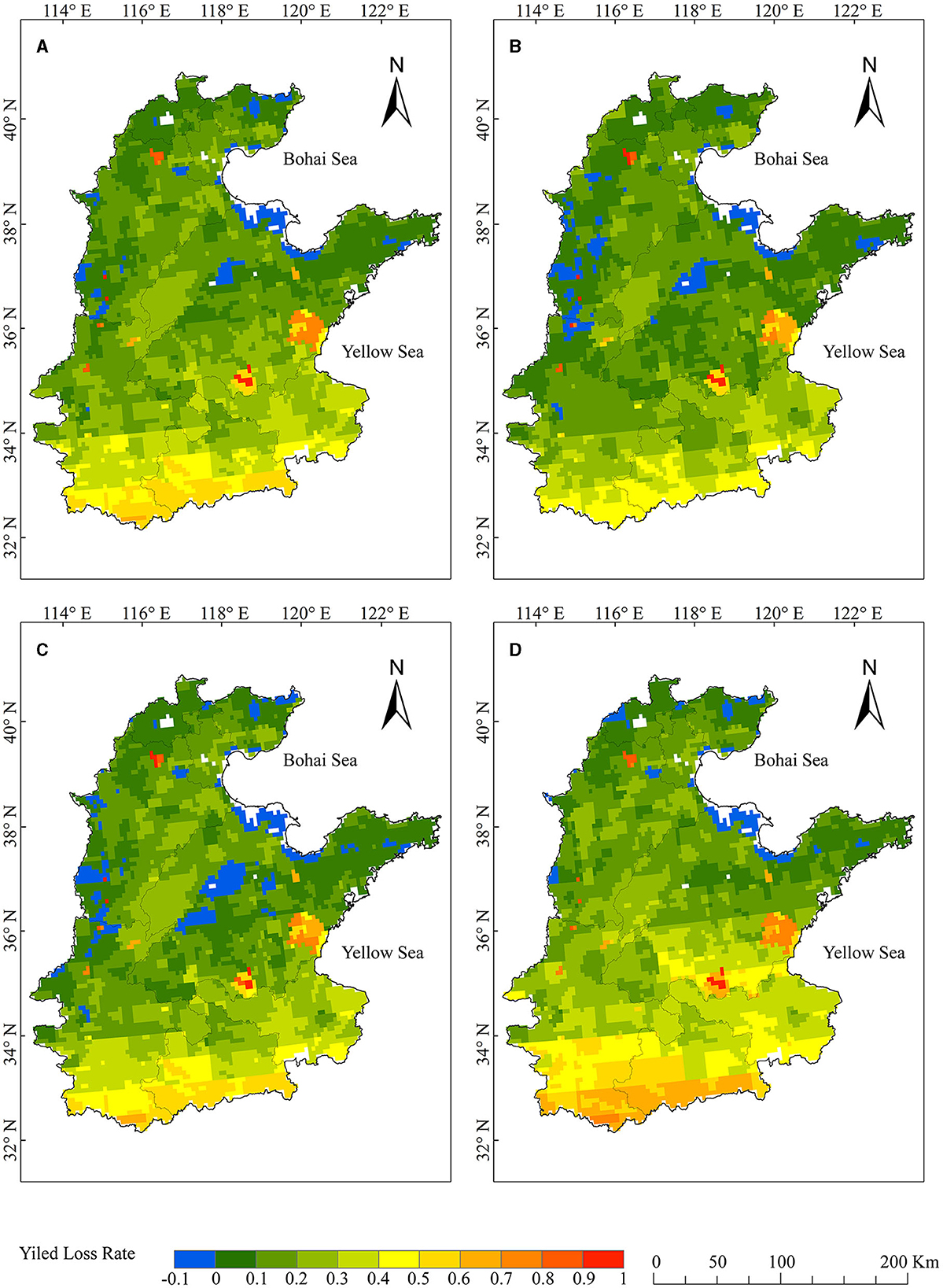
Figure 6. Spatial distribution of wheat physical yield loss rate for 2010–2099 under RCP8.5-SSP3 scenario [(A) average, (B) near-, (C) medium- and (D) long-term].
Compared to the Ylossp, the Ylossc of winter wheat areas significantly shrunk after irrigation. Under the RCP8.5-SSP3 scenario, winter wheat yields growth in the NCP increased by 4.9% from 2010 to 2099. However, the areas with a Ylossc below very slight degree under irrigation increased by 25.6% and Ylossc exceeding severe level under irrigation decreased by 11.6% compared to the Ylossp. The proportions with a Ylossc below very slight degree are primarily distributed in the center and northern of NCP. In contrast, the areas with a Ylossc exceeding severe degree are primarily distributed in the east-central region of NCP.
In summary, our results strongly support these conclusions. First, the continuous strengthening of irrigation with improvements in socioeconomic development can effectively offset the negative impact of extreme climate change on winter wheat production in the NCP. Additionally, it must be recognized that as an effective adaptation activity to climate change, the benefits of irrigation on winter wheat production are limited. Undoubtedly, extreme climate change will have a severe and catastrophic impact on winter wheat production in the NCP in the long run. Based on the above results, we argue that comprehensive adaptation activities, including irrigation, must be considered, rather than relying solely on irrigation for better climate change mitigation. Specifically, in the context of water scarcity in the NCP, adaptation activities other than irrigation, such as crop variety improvement, must be highly valued. We discuss these issues in Section 4.
4 Discussion
Under RCP8.5, an average winter wheat Ylossp of 0.35 was observed during 2010–2099 compared to the baseline period (Figure 4A). This is much higher than that of 0.17 (Liu et al., 2021), and close to but a little bit higher than those of 0.1–0.3 (Yin et al., 2015) reported in prior studies. Among which, areas with Ylossp exceeding moderate and severe degrees will account for 21.1% and 19.2%, respectively (Figure 3). We further found that the Ylossp under the RCP8.5 scenario increases by 13.5% and 50% compared to those of RCP2.6 and RCP4.5 scenarios in the long-term (Figure 7), respectively. Our results highlight that winter wheat losses in the NCP under the RCP8.5 scenario is much higher than those of the global average, i.e., 5.5% (Lobell et al., 2011) and 13.4% (Ray et al., 2019) decline with climate change, and 6% decrease for every 1°C increase (Asseng et al., 2015). These findings suggest that climate change will significantly reduce winter wheat yields in the NCP without any adaptation countermeasures. And, the adverse effects of climate change on winter wheat production may vary significantly among different regions of the world.
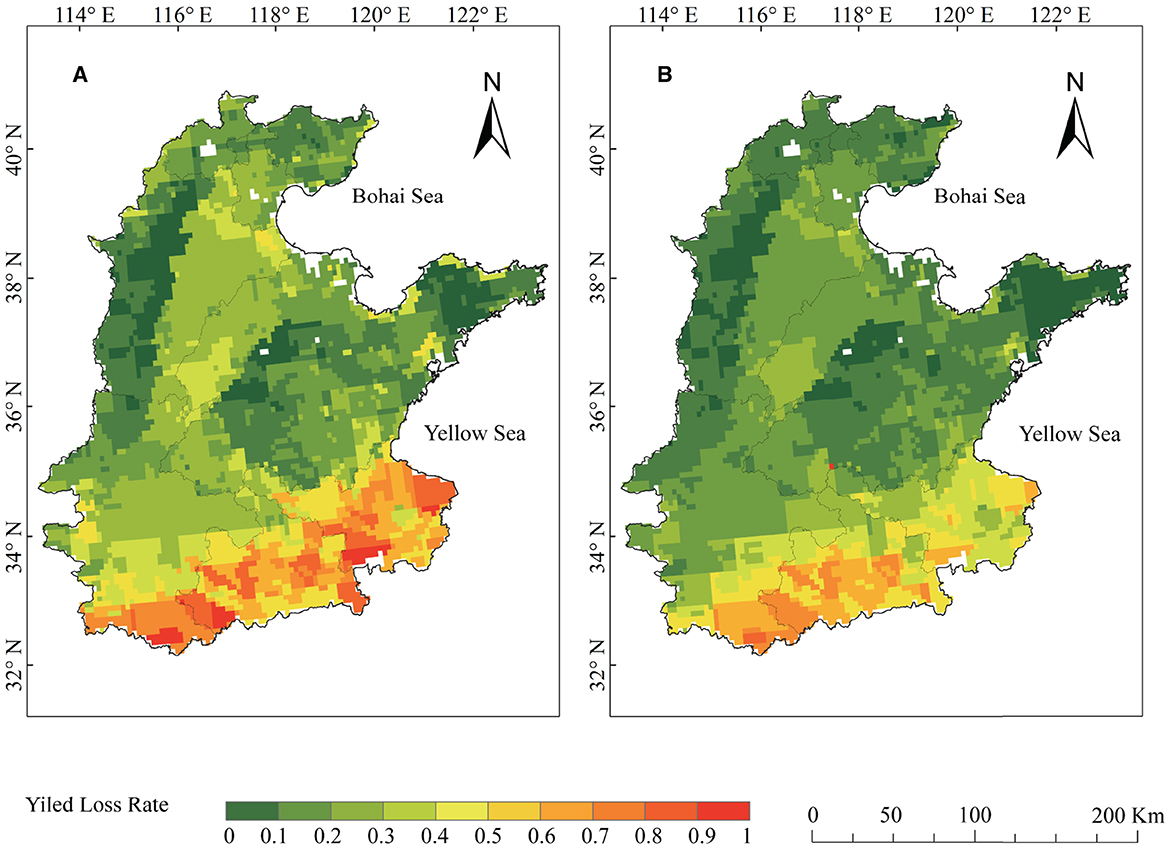
Figure 7. Spatial distribution of wheat physical yield loss rate for 2010–2099 under RCP2.6 (A) and RCP4.5 (B) scenarios.
In light of this, irrigation plays a critical role in coping with the negative impacts of climate change in the NCP. Irrigation was widely acknowledged as an effective adaptation measure to ensure global food security under climate change, especially with aggravating drought trend (Tari, 2016; Zhou et al., 2018; Francaviglia and Di Bene, 2019; Kumar Jha et al., 2019; Jia et al., 2021; Wei et al., 2022). It reported that with every 1% increase in irrigated areas, winter wheat areas have increased by 1.24% in China (Fan et al., 2020). Nevertheless, irrigation is constrained by various factors such as socioeconomic development, irrigation techniques, irrigation equipment, water resource availability (Chen et al., 2015; Bai et al., 2020), and crop varieties (Belaqziz et al., 2021). Based on this, we argue that water-saving irrigation techniques and efficient irrigation schemes are crucial for sustainable agricultural development in the NCP, where precipitation is scarce and irrigation water resources are limited during the winter wheat growing period.
Moreover, inappropriate irrigation causes many negative impacts such as a decrease in runoff and underground water resources (Dalin et al., 2017), accelerated desertification (Fiebig and Dodd, 2016), and secondary soil salinization (Pulido-Velazquez et al., 2018). Notably, the NCP is one of the four regions worldwide with severe underground water overdrafts (Wada et al., 2010). This feature seriously threatens regional environmental safety (Zhao et al., 2020) and food security. Accordingly, over-reliance on irrigation to cope with climate change may entail significant water resources, and environmental and economic costs. Therefore, there is an urgent need to update technologies for regional irrigation to increase grain production (Tari, 2016) and further secure the sustainable development of regional agriculture.
The findings indicates that irrigation can substantially reduce winter wheat yield loss caused by climate change (Figure 6). However, even with the irrigation changes with socioeconomic development, climate change is projected to decrease wheat production potential (Figure 6D). Similarly, model projections indicate that even under the most optimistic assumptions about freshwater distribution and transportation, the beneficial effects of irrigation will be exhausted by the detrimental effects of climate change on crop yields by 2070 at the latest (Elliott et al., 2014). Moreover, adaptation activities include irrigation (Zang et al., 2022), co-application of fertilization (Yu et al., 2022), modification of sowing dates (Chen et al., 2020), alteration of crop distribution (Yue et al., 2019), and crop variety improvement (Zhao et al., 2022). Among these, earlier sowing dates were found to be beneficial for wheat yield, but early sowing opportunities under the dry scenario were extremely limited (Chen et al., 2020). Conversely, delayed sowing date negatively affects the wheat yield (Newport et al., 2020; Sayed et al., 2021). This finding was also found in the NCP, i.e., the yield losses were more severe in areas with late planting dates for winter wheat (Figures 5, 6, 8B).
Meanwhile, many studies claim that the integrated application of multi-adaptation activities can compensate for the limitations of an individual countermeasure. For example, enhancing fertilizer application, such as manure (Ding Z. et al., 2021), and water-use efficiency can significantly increase regional grain yields (Bai and Gao, 2021) while saving irrigation water (Belaqziz et al., 2021). However, we argue that the application of multi-adaptive activities (especially climate-smart agricultural techniques) presupposes a clear understanding of the functions, benefits, and limitations of individual activities. Among the various stressors of agricultural production, water is one of the basic elements of plant growth and a constraint on grain production (Zeng et al., 2021). Therefore, irrigation is a basic and indispensable adaptation activity significantly affected by climate change and influences the effectiveness of the application of other activities (e.g., fertilizer application, cropping system, and sowing dates). Though the other adaptation countermeasures may also be affected by climate change, they seldom should be directly constrained by climate change as irrigation. Therefore, we argue that a comprehensive understanding of the role, benefits, and limitations of irrigation on agricultural production is essential and imperative first and foremost. Our study provides an interesting perspective by quantifying the impacts of climate change and irrigation on winter wheat yield in the NCP. Though this study considers only one climate smart variable, i.e., irrigation, our results could be interesting to people interested in understanding the impact of irrigation as a climate mitigation and adaptation tool.
The yield loss of winter wheat in the NCP was found higher in the south and lower in the north, either under the RCP8.5 scenario or the RCP8.5-SSP3 scenario (Figures 4, 6). This implied that the adverse effects of climate change on winter wheat have gradually intensified from higher to lower latitudes. Many previous studies have observed that grain production in regions with lower latitudes is more vulnerable to climate change (Leng et al., 2015; Liu et al., 2016; Yue et al., 2019). For example, in China, a significant migration of rice planting in Heilongjiang Province has shifted northward by 4° (Piao et al., 2010); climate change may increase land suitability for wheat cultivation in high-latitude regions (Yue et al., 2019; Ahmad et al., 2020). Globally, the future land suitability of wheat cultivation varies significantly, but will benefit wheat production in Europe, Russia, the United States, Canada, China, and Pakistan with changing climate (Yue et al., 2019). Therefore, in the foreseeable long-term, we argue that adequately regarding the planting suitability of regional cultivation (Tahmasebi et al., 2020) and adjusting land use patterns (Lei et al., 2014; Yegbemey, 2021) will have a positive effect on agricultural production under climate change.
Agricultural development stages, field practices, and land-use patterns differed by region around the world (Tahmasebi et al., 2020; Tian and Zhang, 2020; Belaqziz et al., 2021). Increased grain production may incur enormous economic and environmental costs in order to ensure food security in the face of population growth and climate change (Lange et al., 2020; Hong et al., 2022; Yang et al., 2022). Our findings show considerable potential from irrigation to avoid some of the damages from climate change. The remaining losses, however, indicate that irrigation is not a panacea and that more adaptation practices are needed to help farmers and meet future food demands. This study sheds light on the significance of reasonable adaptive countermeasures that can precisely respond to climate change to ensure food security while maintaining a sustainable agricultural system without additional costs to resources, the environment, and the economy.
This study may be limited in the sense it considers only one climate smart variable, i.e., irrigation. Limited to the goals of exploring the benefits and limitations of irrigation on crop yield. The other important variables such as fertilization, crop variety improvement, sowing date, or other adaptation activities that perform a critical role in increasing and stabilizing agricultural production (Cordell et al., 2009; Xiong et al., 2021), were not considered. However, of all variables, irrigation is the most important countermeasure in maintaining/ promoting agricultural production under climate change, especially when facing climate change-related natural hazards, such as drought. In addition, different climate-smart variables have different impact mechanisms on the adaptation of agricultural systems to climate change. If many variables are considered at the same time, it may be impossible to distinguish the benefits and limitations of different variables. We argue that it may be an effective way to evaluate its role in coping with climate change from a single variable.
Besides, the marginal benefits of GDP growth on irrigation were not considered, which theoretically must exist and need to be explored further. Moreover, we only evaluated irrigation from the perspective of adaptive capacity without considering future regional water supply and availability. Furthermore, the discrepancies in climate change on crop yield at different growing stages are ignored. Climatic change-related hazards, such as drought and heat waves, affect crop yield and vary at different growth stages (Zhang et al., 2018; Jiang et al., 2019). Therefore, those limitations could be considered more comprehensively in future evaluation research.
5 Conclusion
Although it is well known that irrigation can promote agricultural yields, the extent to which irrigation as an adaptation measure can withstand the effects of climate change is still not clear. On the contrary, in the assessment of the impact of climate change on grain production, neglect of adaptation countermeasures tends to exaggerate the adverse effects of climate change, thus restricting the proposal of reasonable adaptation strategies to climate change. From this perspective, this study evaluated winter wheat yield gains and losses in the NCP under an extremely unmitigated climate change scenario (RCP8.5) and those considering both climate change and irrigation driven by future socioeconomic development scenarios (RCP8.5-SSP3) from 2010 to 2099.
Our findings confirmed that irrigation can largely mitigate climate-induced reductions in winter wheat yields in the NCP. Under the RCP8.5 scenario, an average yield loss rate of 0.35 was observed. Areas of high-yield loss of winter wheat are primarily distributed in the southern NCP, that is southern Henan, northern Anhui, and Jiangsu. In comparison, the average yield loss rate was 0.21 under the RCP8.5-SSP3 scenario, implying that irrigation significantly eliminates the impact of climate change on the yield loss of winter wheat. However, winter wheat yield loss tends to intensify with changing climate over time even irrigation cannot offset the adverse effects of climate change. Therefore, we argue that irrigation can hardly compensate for losses in winter wheat yield in the long term; that is, irrigation has limited mitigation benefits in adapting to climate change.
Although this study merely explored the effects of irrigation on winter wheat yields under extremely unmitigated climate conditions, the long-term sustainability of irrigation expansion is clearly revealed considering the existing stress on water supplies. The significance lies not only in the capacity to offer a thorough insight into how climate change affects agricultural production but also in similar studies at different scales, such as considering fertilization, adjustment of sowing dates, and variety improvement, which can potentially identify optimal global and regional climate change adaptation countermeasures. We emphasize that to formulate scientifically viable adaptation countermeasures in the context of global climate change, it is vital to consistently focus on the process of adaptive activities on agricultural production and to strengthen the evaluation of the benefits and limitations of various adaptation countermeasures.
Data availability statement
The original contributions presented in the study are included in the article/supplementary material, further inquiries can be directed to the corresponding author.
Author contributions
YG: Writing—review & editing, Data curation, Formal analysis, Investigation, Methodology, Software, Validation, Visualization, Writing—original draft. LW: Data curation, Formal analysis, Investigation, Methodology, Software, Validation, Visualization, Writing—original draft. YY: Investigation, Writing—original draft, Conceptualization, Funding acquisition, Project administration, Supervision, Writing—review & editing.
Funding
The author(s) declare that financial support was received for the research, authorship, and/or publication of this article. This research was financially supported by the National Natural Science Foundation (nos. 41877521 and 41271515) and the National Key Research and Development Program (no. 2016YFA0602402) of China.
Conflict of interest
The authors declare that the research was conducted in the absence of any commercial or financial relationships that could be construed as a potential conflict of interest.
Publisher's note
All claims expressed in this article are solely those of the authors and do not necessarily represent those of their affiliated organizations, or those of the publisher, the editors and the reviewers. Any product that may be evaluated in this article, or claim that may be made by its manufacturer, is not guaranteed or endorsed by the publisher.
References
Ahmad, M. J., Choi, K. S., Cho, G. H., and Kim, S. H. (2019). Future wheat yield variabilities and water footprints based on the yield sensitivity to past climate conditions. Agronomy 9, 744. doi: 10.3390/agronomy9110744
Ahmad, M. J., Iqbal, M. A., and Choi, K. S. (2020). Climate-driven constraints in sustaining future wheat yield and water productivity. Agric. Water Manage. 231, 105991. doi: 10.1016/j.agwat.2019.105991
Ali, S., Liu, Y., Ishaq, M., Shah, T., Abdullah, I. A., and Din, I. U. (2017). Climate change and its impact on the yield of major food crops: evidence from Pakistan. Foods 6, 39. doi: 10.3390/foods6060039
Asseng, S., Ewert, F., Martre, P., Rötter, R. P., Lobell, D. B., Cammarano, D., et al. (2015). Rising temperatures reduce global wheat production. Nat. Clim. Change 5, 143–147. doi: 10.1038/nclimate2470
Attiaoui, I., and Boufateh, T. (2019). Impacts of climate change on cereal farming in Tunisia: a panel ARDL–PMG approach. Environ. Sci. Pollut. Res. 26, 13334–13345. doi: 10.1007/s11356-019-04867-y
Ault, T. R., Cole, J. E., Overpeck, J. T., Pederson, G. T., and Meko, D. M. (2014). Assessing the risk of persistent drought using climate model simulations and paleoclimate data. J. Clim. 20, 7529–7549. doi: 10.1175/JCLI-D-12-00282.1
Bai, S., Kang, Y., and Wan, S. (2020). Correction to: winter wheat growth and water use under different drip irrigation regimes in the North China plain. Irrigation Sci. 38, 479. doi: 10.1007/s00271-020-00677-0
Bai, Y., and Gao, J. (2021). Optimization of the nitrogen fertilizer schedule of maize under drip irrigation in Jilin, China, based on DSSAT and GA. Agric. Water Manage. 244, 106555. doi: 10.1016/j.agwat.2020.106555
Balkovič, J., van der Velde, M., Skalsk,ý, R., Xiong, W., Folberth, C., Khabarov, N., et al. (2014). Global wheat production potentials and management flexibility under the representative concentration pathways. Global Planetar. Change 122, 107–121. doi: 10.1016/j.gloplacha.2014.08.010
Belaqziz, S., Khabba, S., Kharrou, M. H., Bouras, E. H., Er-Raki, S., Chehbouni, A., et al. (2021). Optimizing the sowing date to improve water management and wheat yield in a large irrigation scheme, through a remote sensing and an evolution strategy-based approach. Remote Sens. 13, 3789. doi: 10.3390/rs13183789
Bjornlund, V., Bjornlund, H., and van Rooyen, A. F. (2020). Exploring the factors causing the poor performance of most irrigation schemes in post-independence sub-Saharan Africa. Int. J. Water Resour. Dev. 36, S54–S101. doi: 10.1080/07900627.2020.1808448
Carew, R., Meng, T., Florkowski, W. J., Smith, R., and Blair, D. (2018). Climate change impacts on hard red spring wheat yield and production risk: evidence from Manitoba, Canada. Can. J. Plant Sci. 98, 135. doi: 10.1139/cjps-2017-0135
Chandio, A. A., Jiang, Y., Akram, W., Adeel, S., Irfan, M., Jan, I., et al. (2021). Addressing the effect of climate change in the framework of financial and technological development on cereal production in Pakistan. J. Clean. Prod. 288, 125637. doi: 10.1016/j.jclepro.2020.125637
Chavez, E., Conway, G., Ghil, M., and Sadler, M. (2015). An end-to-end assessment of extreme weather impacts on food security. Nat. Clim. Change 5, 997–1001. doi: 10.1038/nclimate2747
Chavez, J. C., Enciso, J., Meki, M. N., Jeong, J., and Singh, V. P. (2018). Simulation of energy sorghum under limited irrigation levels using the EPIC model. Trans. ASABE 61, 121–131. doi: 10.13031/trans.12470
Chen, C., Wang, B., Feng, P., Xing, H., Fletcher, A. L., Lawes, R. A., et al. (2020). The shifting influence of future water and temperature stress on the optimal flowering period for wheat in Western Australia. Sci. Total Environ. 737, 139707. doi: 10.1016/j.scitotenv.2020.139707
Chen, R., Cheng, W., Cui, J., Liao, J., Fan, H., Zheng, Z., et al. (2015). Lateral spacing in drip-irrigated wheat: The effects on soil moisture, yield, and water use efficiency. Field Crops Res. 179, 52–62. doi: 10.1016/j.fcr.2015.03.021
Cordell, D., Drangert, J., and White, S. (2009). The story of phosphorus: GLOBAL food security and food for thought. Global Environ. Change 19, 292–305. doi: 10.1016/j.gloenvcha.2008.10.009
Dalin, C., Wada, Y., Kastner, T., and Puma, M. J. (2017). Groundwater depletion embedded in international food trade. Nature 553, 366. doi: 10.1038/nature24664
Dasgupta, S., and Robinson, E. J. Z. (2022). Attributing changes in food insecurity to a changing climate. Sci. Rep. 12, 4709. doi: 10.1038/s41598-022-08696-x
Deryng, D., Sacks, W. J., Barford, C. C., and Ramankutty, N. (2011). Simulating the effects of climate and agricultural management practices on global crop yield. Glob. Biogeochem. Cycles 25, GB2006. doi: 10.1029/2009GB003765
Ding, Y., Li, C., Wang, X., Wang, Y., Wang, S., Chang, Y., et al. (2021). An overview of climate change impacts on the society in China. Adv. Clim. Change Res. 12, 210–223. doi: 10.1016/j.accre.2021.03.002
Ding, Z., Ali, E. F., Elmahdy, A. M., Ragab, K. E., Seleiman, M. F., Kheir, A. M. S., et al. (2021). Modeling the combined impacts of deficit irrigation, rising temperature and compost application on wheat yield and water productivity. Agric. Water Manage. 244, 106626. doi: 10.1016/j.agwat.2020.106626
Duan, Q. Y., Gupta, V. K., and Sorooshian, S. (1993). Shuffled complex evolution approach for effective and efficient global minimization. J. Optim. Theor. Appl. 76, 501–521. doi: 10.1007/BF00939380
Elliott, J., Deryng, D., Muller, C., Frieler, K., Konzmann, M., Gerten, D., et al. (2014). Constraints and potentials of future irrigation water availability on agricultural production under climate change. Proc. Nat. Acad. Sci. 111, 3239–3244. doi: 10.1073/pnas.1222474110
Etwire, P. M., Koomson, I., and Martey, E. (2022). Impact of climate change adaptation on farm productivity and household welfare. Clim. Change 170, 11. doi: 10.1007/s10584-022-03308-z
Fan, L., Chen, S., Liang, S., Sun, X., Chen, H., You, L., et al. (2020). Assessing long-term spatial movement of wheat area across China. Agric. Syst. 185, 102933. doi: 10.1016/j.agsy.2020.102933
Feng, G., Zhu, C., Wu, Q., Wang, C., Zhang, Z., Mwiya, R. M., et al. (2021). Evaluating the impacts of saline water irrigation on soil water-salt and summer maize yield in subsurface drainage condition using coupled HYDRUS and EPIC model. Agric. Water Manage. 258, 107175. doi: 10.1016/j.agwat.2021.107175
Fiebig, A., and Dodd, I. C. (2016). Inhibition of tomato shoot growth by over-irrigation is linked to nitrogen deficiency and ethylene. Physiol. Plantar. 156, 70–83. doi: 10.1111/ppl.12343
Fischer, G., Shah, M., Tubiellol, F. N., and Velhuizen, H. V. (2005). socioeconomic and climate change impacts on agriculture: an integrated assessment, 1990-2080. Philos. Trans. Royal Soc. Biol. Sci. 360, 2067–2083. doi: 10.1098/rstb.2005.1744
Foster, T., Mieno, T., and Brozović, N. (2020). Satellite-based monitoring of irrigation water use: assessing measurement errors and their implications for agricultural water management policy. Water Resour. Res. 56, e2020WR.028378. doi: 10.1029/2020WR028378
Francaviglia, R., and Di Bene, C. (2019). Deficit drip irrigation in processing tomato production in the mediterranean basin: a data analysis for Italy. Agriculture 9, 79. doi: 10.3390/agriculture9040079
Gammans, M., Mérel, P., and Ortiz-Bobea, A. (2016). Negative impacts of climate change on cereal yields: statistical evidence from France. Environ. Res. Lett. 12, 054007. doi: 10.1088/1748-9326/aa6b0c
Gómez-Limón, J. A., Gutiérrez-Martín, C., and Montilla-López, N. M. (2021). Priority water rights. Are they useful for improving water-use efficiency at the irrigation district level? Agric. Water Manage. 257, 107145. doi: 10.1016/j.agwat.2021.107145
Hasegawa, T., Fujimori, S., Havlík, P., Valin, H., Bodirsky, B. L., Doelman, J. C., et al. (2018). Risk of increased food insecurity under stringent global climate change mitigation policy. Nat. Clim. Chang. 8, 699–703. doi: 10.1038/s41558-018-0230-x
Hasegawa, T., Sakurai, G., Fujimori, S., Takahashi, S., Hijioka, Y., Masui, T., et al. (2021). Extreme climate events increase risk of global food insecurity and adaptation needs. Nat Food 2, 587–595. doi: 10.1038/s43016-021-00335-4
Hong, C., Zhao, H., Qin, Y., Burney, J. A., Pongratz, J., Hartung, K., et al. (2022). Land-use emissions embodied in international trade. Science 6593, 597–603. doi: 10.1126/science.abj1572
Howden, S. M., Soussana, J. F., Tubiello, F. N., Chhetri, N., Dunlop, M., Meinke, H., et al. (2007). Adapting agriculture to climate change. Proc. Nat. Acad. Sci. 50, 19691–19696. doi: 10.1073/pnas.0701890104
IIASA/FAO (2012). Global Agro-ecological Zones (GAEZ v3.0). Laxenburg; Rome: IIASA; FAO. Available online at: https://www.gaez.iiasa.ac.at/
Janssens, C., Havlík, P., Krisztin, T., Frank, S., Hasegawa, T., Leclère, D., et al. (2020). Global hunger and climate change adaptation through international trade. Nat. Clim. Chang. 10, 829–835. doi: 10.1038/s41558-020-0847-4
Jia, D., Dai, X., Xie, Y., and He, M. (2021). Alternate furrow irrigation improves grain yield and nitrogen use efficiency in winter wheat. Agric. Water Manage. 244, 106606. doi: 10.1016/j.agwat.2020.106606
Jiang, Q., Yue, Y., and Gao, L. (2019). The spatial-temporal patterns of heatwave hazard impacts on wheat in northern China under extreme climate scenarios. Geomatic. Nat. Hazards Risk 1, 2346–2367. doi: 10.1080/19475705.2019.1693435
Kafle, K., Omotilewa, O., Leh, M., and Schmitter, P. (2022). Who is likely to benefit from public and private sector investments in farmer-led irrigation development? Evidence from Ethiopia. J. Dev. Stud. 1, 55–75. doi: 10.1080/00220388.2021.1939866
Kassie, K. E., and Alemu, B. A. (2021). Does irrigation improve household's food security? The case of Koga irrigation development project in northern Ethiopia. Food Secur. 13, 291–307 doi: 10.1007/s12571-020-01129-5
Ko, J., Piccinni, G., and Steglich, E. (2009). Using EPIC model to manage irrigated cotton and maize. Agric. Water Manage. 96, 1323–1331. doi: 10.1016/j.agwat.2009.03.021
Koch, J., Zhang, W., Martinsen, G., He, X., and Stisen, S. (2020). Estimating net irrigation across the North China Plain through dual modelling of evapotranspiration. Water Resour. Res. 56, 2020WR027413. doi: 10.1029/2020WR027413
Kumar Jha, S., Ramatshaba, T. S., Wang, G., Liang, Y., Liu, H., Gao, Y., et al. (2019). Response of growth, yield and water use efficiency of winter wheat to different irrigation methods and scheduling in North China Plain. Agric. Water Manage. 217, 292–302. doi: 10.1016/j.agwat.2019.03.011
Lange, S., Volkholz, J., Geiger, T., Zhao, F., Vega, I., Veldkamp, T., et al. (2020). Projecting exposure to extreme climate impact events across six event categories and three spatial scales. Earth's Fut. 8, e2020EF.001616. doi: 10.1029/2020EF001616
Lehner, F., Coats, S., Stocker, T. F., Pendergrass, A. G., Sanderson, B. M., Raible, C. C., et al. (2017). Projected drought risk in 1.5°C and 2°C warmer climates. Geophys. Res. Lett. 14, 7419–7428. doi: 10.1002/2017GL074117
Lei, Y., Wang, J. A., Yue, Y., Yin, Y., and Sheng, Z. (2014). How adjustments in land use patterns contribute to drought risk adaptation in a changing climate—A case study in China. Land Use Policy 36, 577–584. doi: 10.1016/j.landusepol.2013.10.004
Leng, G., Tang, Q., and Rayburg, S. (2015). Climate change impacts on meteorological, agricultural and hydrological droughts in China. Glob. Planet. Change 126, 23–34. doi: 10.1016/j.gloplacha.2015.01.003
Leng, G., Zhang, X., Huang, M., Yang, Q., Rafique, R., Asrar, G. R., et al. (2016). Simulating county-level crop yields in the conterminous United States using the community land model: the effects of optimizing irrigation and fertilization. J. Adv. Model. Earth Syst. 8, 1912–1931. doi: 10.1002/2016MS000645
Lesk, C., Rowhani, P., and Ramankutty, N. (2016). Influence of extreme weather disasters on global crop production. Nature 529, 84–87. doi: 10.1038/nature16467
Li, J., Zhang, Z., Liu, Y., Yao, C. S., Song, W. Y., Xu, X. X., et al. (2019). Effects of micro-sprinkling with different irrigation amount on grain yield and water use efficiency of winter wheat in the North China Plain. Agric. Water Manage. 224:105736. doi: 10.1016/j.agwat.2019.105736
Li, M., Sun, H., Liu, D., Singh, V. P., and Fu, Q. (2021). Multi-scale modeling for irrigation water and cropland resources allocation considering uncertainties in water supply and demand. Agric. Water Manage. 246, 106687. doi: 10.1016/j.agwat.2020.106687
Li, Y., Huang, H., Ju, H., Lin, E., Xiong, W., Han, X., et al. (2015). Assessing vulnerability and adaptive capacity to potential drought for winter-wheat under the RCP 8.5 scenario in the Huang-Huai-Hai Plain. Agric. Ecosyst. Environ. 209, 125–131. doi: 10.1016/j.agee.2015.03.033
Liu, B., Asseng, S., Müller, C., Ewert, F., Elliott, J., Lobell, D. B., et al. (2016). Similar estimates of temperature impacts on global wheat yield by three independent methods. Nat. Clim. Chang. 6, 1130–1136. doi: 10.1038/nclimate3115
Liu, W., Ye, T., and Shi, P. (2021). Decreasing wheat yield stability on the North China Plain: relative contributions from climate change in mean and variability. Int. J. Climatol. 41, E2820–E2833. doi: 10.1002/joc.6882
Liu, Y., Wang, E., Yang, X., and Wang, J. (2010). Contributions of climatic and crop varietal changes to crop production in the North China Plain, since 1980s. Glob. Chang. Biol. 16, 2287–2299. doi: 10.1111/j.1365-2486.2009.02077.x
Lobell, D. B. (2014). Climate change adaptation in crop production: beware of illusions. Glob. Food Secur. Agric. Policy Econ. Environ. 3, 72–76. doi: 10.1016/j.gfs.2014.05.002
Lobell, D. B., and Asseng, S. (2017). Comparing estimates of climate change impacts from process-based and statistical crop models. Environ. Res. Lett. 12, 15001. doi: 10.1088/1748-9326/aa518a
Lobell, D. B., Schlenker, W., and Costa-Roberts, J. (2011). Climate trends and global crop production since 1980. Science 333, 616–620. doi: 10.1126/science.1204531
Mahmood, N., Arshad, M., Kächele, H., Ma, H., Ullah, A., Müller, K., et al. (2019). Wheat yield response to input and socioeconomic factors under changing climate: evidence from rainfed environments of Pakistan. Sci. Total Environ. 688, 1275–1285. doi: 10.1016/j.scitotenv.2019.06.266
Mwaura, F. M., and Muwanika, F. R. (2018). Providing irrigation water as a public utility to enhance agricultural productivity in Uganda. Utilities Policy 55, 99–109. doi: 10.1016/j.jup.2018.09.003
Nelson, G., Bogard, J., Lividini, K., Arsenault, J., Riley, M., Sulser, T. B., et al. (2018). Income growth and climate change effects on global nutrition security to mid-century. Nat. Sust. 1, 773–781. doi: 10.1038/s41893-018-0192-z
Newport, D., Lobell, D. B., Balwinder-Singh, A. K., Rao, P., Umashaanker, M., Malik, R. K., et al. (2020). Factors constraining timely sowing of wheat as an adaptation to climate change in eastern India. Weather Clim. Soc. 12, 515–528. doi: 10.1175/WCAS-D-19-0122.1
Papathoma-Köhle, M., Kappes, M., Keiler, M., and Glade, T. (2011). Physical vulnerability assessment for alpine hazards: state of the art and future needs. Nat. Hazards 58, 645–680. doi: 10.1007/s11069-010-9632-4
Piao, S., Ciais, P., Huang, Y., Shen, Z., Peng, S., Li, J., et al. (2010). The impacts of climate change on water resources and agriculture in China. Nature 467, 43–51. doi: 10.1038/nature09364
Pulido-Velazquez, D., Renau-Prunonosa, A., Llopis-Albert, C., Morell, I., Collados-Lara, A. J., Senent-Aparicio, J., et al. (2018). Integrated assessment of future potential global change scenarios and their hydrological impacts in coastal aquifers - a new tool to analyse management alternatives in the Plana Oropesa-Torreblanca aquifer. Hydrol. Earth Syst. Sci. 22, 3053–3074. doi: 10.5194/hess-22-3053-2018
Qu, C.H., Li, X.X., Ju, H., and L, Q. (2019). The impacts of climate change on wheat yield in the Huang-Huai-Hai Plain of China using DSSAT-CERES-wheat model under different climate scenarios. J. Integr. Agr. 18, 1379–1391. doi: 10.1016/S2095-3119(19)62585-2
Quan Luna, B., Blahut, J., van Westen, C. J., Sterlacchini, S., van Asch, T. W. J., Akbas, S. O., et al. (2011). The application of numerical debris flow modelling for the generation of physical vulnerability curves. Nat. Hazards Earth Syst. Sci. 11, 2047–2060. doi: 10.5194/nhess-11-2047-2011
Ray, D. K., West, P. C., Clark, M., Gerber, J. S., Prishchepov, A. V., Chatterjee, S., et al. (2019). Climate change has likely already affected global food production. PLoS ONE 14, e0217148. doi: 10.1371/journal.pone.0217148
Riahi, K., Van Vuuren, D. P., Kriegler, E., Edmonds, J., O'Neill, B. C., and Fujimori, N. (2017). The shared socioeconomic pathways and their energy, land use, and greenhouse gas emissions implications: an overview. Global Environ. Change 42, 153–168. doi: 10.1016/j.gloenvcha.2016.05.009
Rogelj, J., Popp, A., Calvin, K. V., Luderer, G., Emmerling, J., Gernaat, D., et al. (2018). 2018, Scenarios towards limiting global mean temperature increase below 1.5°C. Nat. Clim. Change 8, 325–332. doi: 10.1038/s41558-018-0091-3
Rosenzweig, C., Elliott, J., Deryng, D., Ruane, A. C., Müller, C., Arneth, A., et al. (2014). Assessing agricultural risks of climate change in the 21st century in a global gridded crop model intercomparison. Proc. Nat. Acad. Sci. 111, 3268–3273. doi: 10.1073/pnas.1222463110
Sayed, M. A., Said, M. T., and El-Rawy, M. A. (2021). Evaluation of local bread wheat cultivars for grain yield and its attributes at different sowing dates under assiut conditions. Egyptian J. Agron. 43, 189–206. doi: 10.21608/agro.2021.74735.1257
Schwalm, C. R., Glendon, S., and Duffy, P. B. (2020). RCP8 5 tracks cumulative CO2 emissions. Proc. Nat. Acad. Sci. 202007117. doi: 10.1073/pnas.2007117117
Sendhil, R., Jha, A., Kumar, A., and Singh, S. (2018). Extent of vulnerability in wheat producing agro-ecologies of India: tracking from indicators of cross-section and multi-dimension data. Ecol. Indic. 89, 771–780. doi: 10.1016/j.ecolind.2018.02.053
Shi, X., Shi, W., Dai, N., and Wang, M. (2022). Optimal irrigation under the constraint of water resources for winter wheat in the north China plain. Agriculture 12, 2057. doi: 10.3390/agriculture12122057
Si, Z., Zain, M., Mehmood, F., Wang, G., Gao, Y., Duan, A., et al. (2020). Effects of nitrogen application rate and irrigation regime on growth, yield, and water-nitrogen use efficiency of drip-irrigated winter wheat in the North China Plain. Agric. Water Manage. 231, 106002. doi: 10.1016/j.agwat.2020.106002
Sloat, L. L., Davis, S. J., Gerber, J. S., Moore, F. C., Ray, D. K., West, P. C., et al. (2020). Climate adaptation by crop migration. Nat. Commun. 11, 1243. doi: 10.1038/s41467-020-15076-4
Steduto, P., Pocuca, V., Caliandro, A., and Debaeke, P. (1995). An evaluation of the cropgrowth simulation sub model of EPIC for wheat grown in a Mediterranean climate with variable soil-water regimes. Eur. J. Agron. 3, 335–345. doi: 10.1016/S1161-0301(14)80034-8
Sun, H., Zhang, X., Liu, X., Liu, X., Shao, L., Chen, S., et al. (2019). Impact of different cropping systems and irrigation schedules on evapotranspiration, grain yield and groundwater level in the North China Plain. Agric. Water Manage. 211, 202–209. doi: 10.1016/j.agwat.2018.09.046
Sun, Z., Zhang, Y., Zhang, Z., Gao, Y., Yang, Y., Han, M., et al. (2019). Significance of disposable presowing irrigation in wheat in increasing water use efficiency and maintaining high yield under winter wheat-summer maize rotation in the North China Plain. Agric. Water Manage. 225, 105766. doi: 10.1016/j.agwat.2019.105766
Tahmasebi, T., Karami, E., and Keshavarz, M. (2020). Agricultural land use change under climate variability and change: drivers and impacts. J. Arid Environ. 180, 104202. doi: 10.1016/j.jaridenv.2020.104202
Tari, A. F. (2016). The effects of different deficit irrigation strategies on yield, quality, and water-use efficiencies of wheat under semi-arid conditions. Agric. Water Manage. 167, 1–10. doi: 10.1016/j.agwat.2015.12.023
Tian, J., and Zhang, Y. (2020). Detecting changes in irrigation water requirement in Central Asia under CO2 fertilization and land use changes. J. Hydrol. 583, 124315. doi: 10.1016/j.jhydrol.2019.124315
Trenberth, K. E., Dai, A., van der Schrier, G., Jones, P. D., Barichivich, J., Briffa, K. R., et al. (2014). Global warming and changes in drought. Nat. Clim. Chang. 4, 17–22. doi: 10.1038/nclimate2067
Tubiello, F. N., Soussana, J. F., and Howden, S. M. (2007). Crop and pasture response to climate change. Proc. Nat. Acad. Sci. 104, 19686–19690. doi: 10.1073/pnas.0701728104
Uzielli, M., Nadim, F., Lacasse, S., and Kaynia, A. M. (2008). A conceptual framework for quantitative estimation of physical vulnerability to landslides. Eng. Geol. 102, 251–256. doi: 10.1016/j.enggeo.2008.03.011
Verschuur, J., Li, S., Wolski, P., and Otto, F. E. L. (2021). Climate change as a driver of food insecurity in the 2007 Lesotho-South Africa drought. Sci. Rep. 11, 3852. doi: 10.1038/s41598-021-83375-x
Wada, Y., van Beek, L., van Kempen, C. M., Reckman, J., Vasak, S., Bierkens, M., et al. (2010). Global depletion of groundwater resources. Geophys. Res. Lett. 37, L20402. doi: 10.1029/2010GL044571
Wang, Z., Chen, J., Tong, W., Xu, C., and Chen, F. (2018). Impacts of climate change and varietal replacement on winter wheat phenology in the north China plain. Int. J. Plant Prod. 12, 251–263. doi: 10.1007/s42106-018-0024-0
Wei, Y., Jin, J., Cui, Y., Ishidaira, H., Li, H., Jiang, S., et al. (2022). Agricultural drought risk assessment based on crop simulation, risk curves, and risk maps in Huaibei Plain of Anhui Province, China. Stochastic Environ. Res. Risk Assessm. 36, 3335–3353. doi: 10.1007/s00477-022-02197-z
White, J. W., Hoogenboom, G., Kimball, B. A., and Wall, G. W. (2011). Methodologies for simulating impacts of climate change on crop production. Field Crops Res. 124, 357–368. doi: 10.1016/j.fcr.2011.07.001
Williams, J. R. (1997). The EPIC Model. Temple, TX: USDA-ARS, Grassland, Soil, and Water Research Laboratory.
Williams, J. R., Jones, C. A., and Dyke, P. T. (1984). A modeling approach to determining the relationship between erosion and soil productivity. Trans. ASAE 27, 129–144. doi: 10.13031/2013.32748
Williams, J. R., Jones, C. A., Kiniry, J. R., and Spanel, D. A. (1989). The EPIC crop growth-model. Trans. ASABE 32, 497–511. doi: 10.13031/2013.31032
Xiao, D., Bai, H., and Liu, D. (2018). Impact of future climate change on wheat production: a simulated case for China's wheat system. Sustainability 10, 1277. doi: 10.3390/su10041277
Xiong, W., Reynolds, M. P., Crossa, J., Schulthess, U., Sonder, K., Montes, C., et al. (2021). Increased ranking change in wheat breeding under climate change. Nat. Plants 7, 1207–1212. doi: 10.1038/s41477-021-00988-w
Yan, F., Zhang, F., Fan, X., Fan, J., Wang, Y., Zou, H., et al. (2021). Determining irrigation amount and fertilization rate to simultaneously optimize grain yield, grain nitrogen accumulation and economic benefit of drip-fertigated spring maize in northwest China. Agric. Water Manage. 243, 106440. doi: 10.1016/j.agwat.2020.106440
Yang, H., Du, T., Mao, X., Ding, R., and Shukla, M. K. (2019). A comprehensive method of evaluating the impact of drought and salt stress on tomato growth and fruit quality based on EPIC growth model. Agric. Water Manage. 213, 116–127. doi: 10.1016/j.agwat.2018.10.010
Yang, Z., Yang, B., Liu, P., Zhang, Y., Hou, L., Yuan, X., et al. (2022). Exposure to extreme climate decreases self-rated health score: large-scale survey evidence from China. Glob. Environ. Change 74, 102514. doi: 10.1016/j.gloenvcha.2022.102514
Yegbemey, R. N. (2021). Farm-level land use responses to climate change among smallholder farmers in northern Benin, West Africa. Clim. Dev. 13, 593–602. doi: 10.1080/17565529.2020.1844129
Yin, Y., Tang, Q., and Liu, X. (2015). A multi-model analysis of change in potential yield of major crops in China under climate change. Earth Syst. Dyn. 6, 45–59. doi: 10.5194/esd-6-45-2015
You, L., Rosegrant, M. W., Wood, S., and Sun, D. (2009). Impact of growing season temperature on wheat productivity in China. Agric. Forest Meteorol. 149, 1009–1014. doi: 10.1016/j.agrformet.2008.12.004
Yu, W., Yue, Y., and Wang, F. (2022). The spatial-temporal coupling pattern of grain yield and fertilization in the North China plain. Agric. Syst. 196, 103330. doi: 10.1016/j.agsy.2021.103330
Yue, Y., Li, J., Ye, X., Wang, Z., Zhu, A., Wang, J., et al. (2015). An EPIC model-based vulnerability assessment of wheat subject to drought. Nat. Hazards 78, 1629–1652. doi: 10.1007/s11069-015-1793-8
Yue, Y., Wang, L., Li, J., and Zhu, A. (2018). An EPIC model-based wheat drought risk assessment using new climate scenarios in China. Clim. Change 147, 539–553. doi: 10.1007/s10584-018-2150-1
Yue, Y., Yang, W., and Wang, L. (2022). Assessment of drought risk for winter wheat on the Huanghuaihai Plain under climate change using an EPIC model-based approach. Int. J. Dig. Earth 15, 690–711. doi: 10.1080/17538947.2022.2055174
Yue, Y., Zhang, P., and Shang, Y. (2019). The potential global distribution and dynamics of wheat under multiple climate change scenarios. Sci. Total Environ. 688, 1308–1318. doi: 10.1016/j.scitotenv.2019.06.153
Zang, Y., Yao, H., Ran, L., Zhang, R., Duan, Y., Yu, X., et al. (2022). Physicochemical properties of wheat starch under different sowing dates. Starch 74, 2100290. doi: 10.1002/star.202100290
Zeng, R., Yao, F., Zhang, S., Yang, S., Bai, Y., Zhang, J., et al. (2021). Assessing the effects of precipitation and irrigation on winter wheat yield and water productivity in North China Plain. Agric. Water Manage. 256:107063. doi: 10.1016/j.agwat.2021.107063
Zhang, J., Zhang, S., Cheng, M., Jiang, H., Zhang, X., Peng, C., et al. (2018). Effect of drought on agronomic traits of rice and wheat: a meta-analysis. Int. J. Environ. Res. Pub. Health 15, 839. doi: 10.3390/ijerph15050839
Zhang, T., Lin, X., and Sassenrath, G. F. (2015). Current irrigation practices in the central United States reduce drought and extreme heat impacts for maize and soybean, but not for wheat. Sci. Environ. 508, 331–342. doi: 10.1016/j.scitotenv.2014.12.004
Zhang, X., Guo, P., Guo, S., Zhang, F., and Zhang, C. (2022). An integrated model to optimize irrigation amount and time in shallow groundwater area under drought conditions. J. Contam. Hydrol. 246, 103956. doi: 10.1016/j.jconhyd.2022.103956
Zhang, X., Srinivasan, R., Zhao, K., and Liew, M. V. (2009). Evaluation of global optimization algorithms for parameter calibration of a computationally intensive hydrologic model. Hydrol. Proc. Int. J. 23, 430–441. doi: 10.1002/hyp.7152
Zhao, Y., Wang, L., Li, H., Zhu, Y., Wang, Q., Jiang, S., et al. (2020). Evaluation of groundwater overdraft governance measures in Hengshui City, China. Sustainability 12, 3564. doi: 10.3390/su12093564
Zhao, Z., Wang, E., Kirkegaard, J. A., and Rebetzke, G. J. (2022). Novel wheat varieties facilitate deep sowing to beat the heat of changing climates. Nat. Clim. Chang. 12, 291–296. doi: 10.1038/s41558-022-01305-9
Zhou, L., Liao, S., Wang, Z., Wang, P., Zhang, Y., Yan, H., et al. (2018). A simulation of winter wheat crop responses to irrigation management using CERES-Wheat model in the North China Plain. J. Integr. Agric. 17, 1181–1193. doi: 10.1016/S2095-3119(17)61818-5
Zhu, X., Xu, K., Liu, Y., Guo, R., and Chen, L. (2021). Assessing the vulnerability and risk of maize to drought in China based on the AquaCrop model. Agric. Syst. 189, 103040. doi: 10.1016/j.agsy.2020.103040
Keywords: climate change, winter wheat, irrigation, the Representative Concentration Pathways, the Shared Socioeconomic Pathway, the North China plain
Citation: Gao Y, Wang L and Yue Y (2024) Impact of irrigation on vulnerability of winter wheat under extreme climate change scenario: a case study of North China Plain. Front. Sustain. Food Syst. 7:1291866. doi: 10.3389/fsufs.2023.1291866
Received: 10 September 2023; Accepted: 11 December 2023;
Published: 05 January 2024.
Edited by:
Emmanuel Donkor, Humboldt University of Berlin, GermanyReviewed by:
Dengpan Xiao, Hebei Normal University, ChinaYong Sebastian Nyam, University of the Free State, South Africa
Copyright © 2024 Gao, Wang and Yue. This is an open-access article distributed under the terms of the Creative Commons Attribution License (CC BY). The use, distribution or reproduction in other forums is permitted, provided the original author(s) and the copyright owner(s) are credited and that the original publication in this journal is cited, in accordance with accepted academic practice. No use, distribution or reproduction is permitted which does not comply with these terms.
*Correspondence: Yaojie Yue, yjyue@bnu.edu.cn
†ORCID: Yaojie Yue orcid.org/0000-0001-5198-1281