Perspective on Clinically-Relevant Antimicrobial Resistant Enterobacterales in Food: Closing the Gaps Using Genomics
- 1Facultad de Ciencias de la Vida, Universidad Andres Bello, Santiago, Chile
- 2Millennium Initiative for Collaborative Research on Bacterial Resistance (MICROB-R), Santiago, Chile
- 3Genomics and Resistant Microbes Group, Facultad de Medicina Clínica Alemana, Universidad del Desarrollo, Santiago, Chile
- 4Departamento de Salud Pública, Escuela de Medicina, Advanced Center for Chronic Diseases (ACCDiS), Pontificia Universidad Católica de Chile, Santiago, Chile
- 5Centro de Desarrollo Urbano Sustentable, Pontificia Universidad Católica de Chile, Santiago, Chile
- 6Escuela de Medicina Veterinaria, Pontificia Universidad Católica de Chile, Santiago, Chile
Antimicrobial resistance is one of the most important public health concerns—it causes 700,000 deaths annually according to the World Health Organization (WHO). Enterobacterales such as E. coli and Klebsiella pneumoniae, have become resistant to many relevant antimicrobials including carbapenems and extended spectrum cephalosporins. These clinically relevant resistant Enterobacterales (CRRE) members are now globally distributed in the environment including different food types (meats, produce, dairy). Unlike known foodborne pathogens, CRRE are not usually part of most food surveillance systems. However, numerous reports of CRRE highlight the importance of these bacteria in food and have been shown to contribute to the overall crisis of antimicrobial resistance. This is especially important in the context of carriage of these pathogens by immuno-compromised individuals. CRRE infections upon consumption of contaminated food could colonize the human gastrointestinal tract and eventually be a source of systemic infections such as urinary tract infections or septicemia. While different aspects need to be considered to elucidate this, whole genome sequencing along with metadata could be used to understand genomic relationships of CRRE obtained from foods and humans, including isolates from clinical infections. Once robust scientific data is available on the role of CRRE in food, countries could move forward to better survey and control CRRE in food.
Introduction
Antimicrobial resistance (AMR) occurs when microorganisms evolve to resist the action of antimicrobials, making infections harder to treat, especially in critical patients leading to premature death (Dadgostar, 2019). Unfortunately, bacteria have progressively acquired resistance to numerous antibiotics exhausting therapeutic alternatives and generating a serious public health problem and a worldwide alert (World Health Organization, 2020). Importantly, AMR can jeopardize many of the advances of modern medicine, and threatens to turn usually treatable conditions into deadly infections that could carry a high death toll around the globe (Menichetti and Tagliaferri, 2012; Chioro et al., 2015). Currently, AMR is estimated to cause 700,000 deaths per year, this number is projected to reach 10 million deaths annually by 2050 (Hoffman et al., 2015).
The dynamics of AMR are complex and poorly understood. However, the connection between humans, animals, food, and the environment is increasingly recognized as a major player in the dissemination of AMR (Ludden et al., 2020). Therefore, the threat of AMR needs an integrated approach such as the collaborative effort of key players from different disciplines at the WHO, Food and Agriculture Organization (FAO), and World Organization for Animal Health (OIE) tripartite (World Health Organization, 2021). To tackle AMR, it is important to reduce transmission of resistant bacteria that are circulating among humans and animals. To accomplish this a better understanding of the role of these organisms in food production is needed. This knowledge will augment the responsible use of antibiotics in humans and animals (Pokharel et al., 2020).
Food products are well-known carriers of foodborne antibiotic-resistant pathogens such as Salmonella spp. or Campylobacter spp. (Nordstrom et al., 2013; Allard et al., 2018). These organisms represent an important public health concern and are, therefore, subject to active surveillance in several countries (Hoffmann et al., 2017). Furthermore, food may also get contaminated with commensal organisms harboring antimicrobial resistance genes, most of these are not part of routine surveillance strategies (Hölzel et al., 2018). Importantly, several studies have already reported the presence of resistant bacteria in foods but only a handful have established relationships between resistant bacteria in food and in humans at the same time. This risk is not comprehensively understood much less controlled (Kim et al., 2015; Liu et al., 2018a; Liu and Song, 2019).
Clinically-Relevant Antimicrobial Resistant Bacteria
In 2017, the WHO published a priority list of antimicrobial resistant pathogens, highlighting the threat posed by multidrug-resistant bacteria (Carmeli et al., 2017). Common characteristics among these priority pathogens are their wide dissemination and their ability to horizontally acquire and transfer resistance traits (Kelly et al., 2017; Logan and Weinstein, 2017; van Duin and Doi, 2017). Importantly, all organisms regarded as “critical” in WHO's priority list are Gram-negatives and include carbapenem-resistant bacteria of different taxa such as Acinetobacter baumannii, Pseudomonas aeruginosa, and Enterobacterales. The list also includes Enterobacterales that produce Extended Spectrum Beta-Lactamases (ESBL)—enzymes that can confer resistance to third-generation cephalosporins (Ghafourian et al., 2015).
Enterobacterales are particularly relevant because they can be found in numerous environments including food and cause significant human infections (Van Loon et al., 2018). For instance, infections caused by antibiotic-resistant Klebsiella spp. and Escherichia coli are associated with mortality rates of around 50% (Logan and Weinstein, 2017; Lipari et al., 2020). The high mortality caused by these pathogens is probably associated with the absence of effective treatments (Temkin et al., 2014). Globally, Klebsiella pneumoniae has been repeatedly identified over the past 30 years, as the most common Enterobacterales linked to the spread of ESBL genes in healthcare settings (Nordmann et al., 2011). Importantly, patients infected with carbapenem-resistant K. pneumoniae have a 4-fold increased risk of death vs. patients infected with antibiotic-susceptible K. pneumoniae (Ben-David et al., 2012). Therefore, carbapenem-resistant Enterobacterales along with those that produce ESBLs are considered clinically relevant-resistant bacteria, these are not only found in human but are also widely reported in the environment and food. However, the fate of clinically relevant resistant bacteria in food and their role in public health are not yet fully understood.
Food Reports and Subtyping of Clinically-Relevant Resistant Enterobacterales
Different food types have been found to contain clinically-relevant resistant Enterobacterales (CRRE)—specifically Enterobacterales producers of ESBL and/or carbapenemases (enzymes that confer resistance to carbapenems) (Liu et al., 2018a; Sugawara et al., 2019; Zhang et al., 2019). Reports of CRRE in food are seen from many different countries; meats, produce, and dairy have all been found to harbor CRRE (Table 1). Most studies have focused on the study of CRRE in different meats such as chicken, beef, and pork; more incipient is the study of CRRE in produce and dairy (Table 1). Overall, the data suggest that CRRE appears to be widely and globally distributed in food.
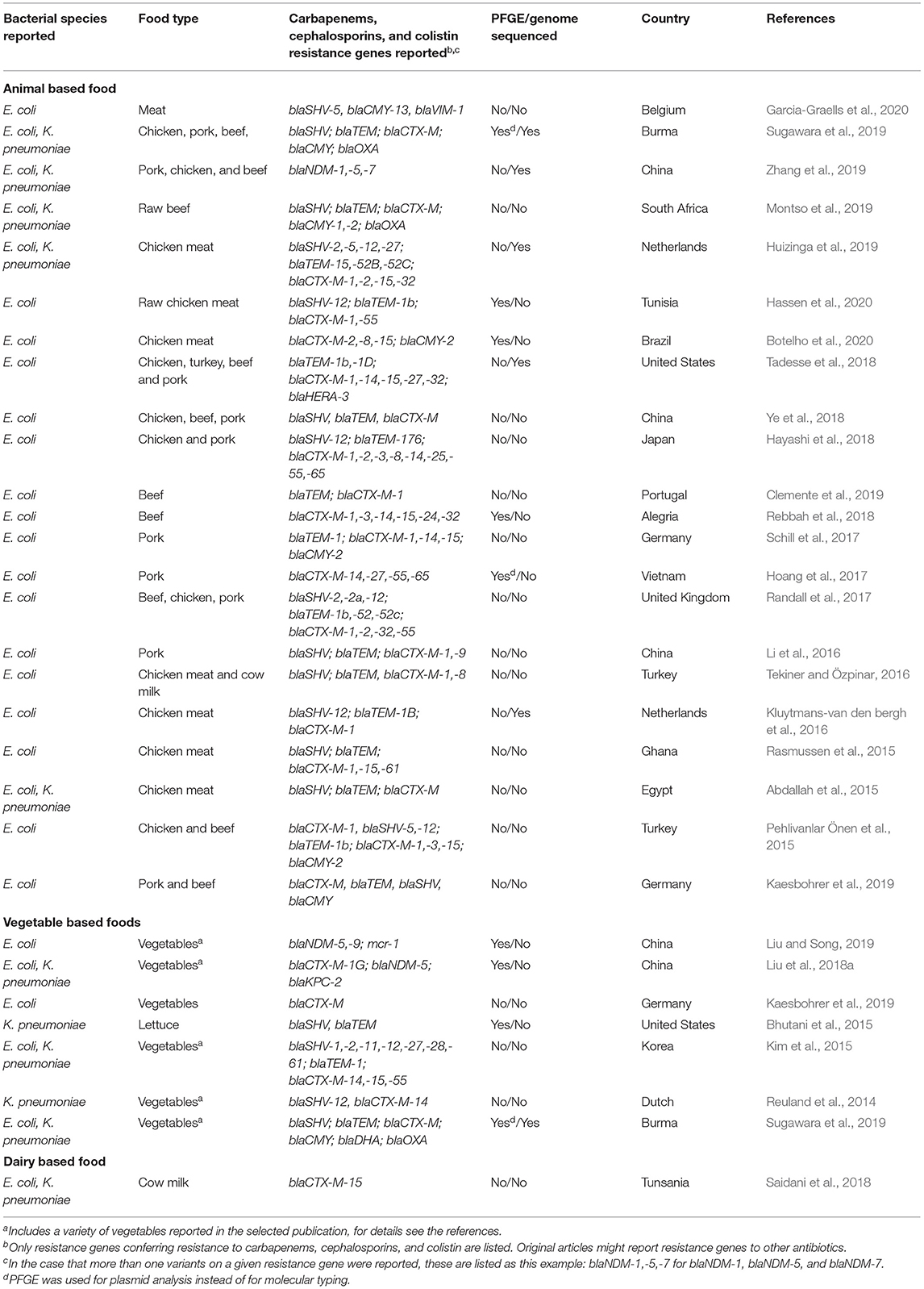
Table 1. Selected reports of clinically relevant antimicrobial resistant bacteria in different foods.
E. coli and K. pneumoniae are the most commonly CRRE reported in food. These organisms are found to harbor genes coding for carbapenemases (e.g., blaOXA−48 and blaNDM). These CRRE containing these genes have been found in meat and produce. CRRE carrying genes coding for the ESBL enzymes blaSHV, blaCTX-M, and blaTEM, have been extensively reported in meat and produce (Table 1). To date, reports of CRRE in food have not necessarily facilitated our understanding of the public health role of the presence of CRRE in food and the risk for consumers of food-mediated acquisition of CRRE.
For decades the gold standard technique to understand the genetic relationships between bacteria has been pulsed-field gel electrophoresis (PFGE) (Salipante et al., 2015). Of the studies selected in Table 1, eight of them used PFGE to understand the relationships of CRRE isolates in food. These previous studies have shown the importance of subtyping isolates; the importance of obtaining greater resolution and summarizing genetic data can improved the study of CRRE in food. For instance, one study used PFGE to type CRRE isolates obtained from vegetables and found two isolates co-carrying relevant resistant genes (i.e., the colistin resistant gene mcr-1 and the ß-lactamase blaNDM). These isolates were PFGE typed along with isolates of avian origin (Liu et al., 2017). However, the PFGE typing showed different patterns between avian and vegetable isolates, suggesting that vegetable isolates were not directly derived from the avian feces previously studied (Liu and Song, 2019).
PFGE has a lower capacity to correctly identify strains in clusters than whole genome sequencing (WGS), which shows better resolution (Gona et al., 2020). To advance this knowledge, some studies have sequenced the genomes of CRRE obtained from food (Table 1); for instance, in Table 1 only 5 of the 28 studies included used genomic information. However, genomic relationships of CRRE isolated from food and CRRE obtained from human clinical cases or human colonization could improve our understanding of the public health risk of food-mediated acquisition of CRRE.
Whole Genome Sequencing as a Tool to Identify the Public Health Risk of CRRE in Food
Whole genome sequencing is a useful tool in food safety and public health facilitating our understanding of transmission and persistence of foodborne pathogens (Kovac et al., 2017). WGS has been crucial for studying foodborne outbreak investigations for almost a decade in the US and Europe (Brown et al., 2019; European Centre for Disease Prevention and Control (ECDC), 2021). Numerous outbreaks have been rapidly resolved using WGS along with epidemiological data. For instance, in 2018 an outbreak of salmonellosis in the European Union related to eggs was resolved using WGS to confirm a source in Poland as the origin of the outbreak. This highlighted the public health value of international sharing of epidemiological data along with rapidly obtained WGS (Pijnacker et al., 2019). In addition, WGS have also been used to track contamination routes of Listeria monocytogenes in food production establishments (Nastasijevic et al., 2017). Therefore, WGS is now widely use in all sectors involved in food safety including governments, academia, and the food industry (Wang et al., 2016).
The use WGS is crucial to improve our understanding of the public health impact on CRRE in food. One study compared the genomes from E. coli obtained from humans, poultry, wild animals, and water collected in the past decade in Australia. Using WGS the study evaluated sequence types, mobile genetic elements, phylogroups, etc. to improve understanding of epidemiological clones and the presence of pervasive horizontal gene transfer across the species in all these sources (Touchon et al., 2020). In this study, the authors compared around 1,300 strains to represent the genetic diversity of E. coli in Australia and looked for associations between the different phylogroups and the environment. Their results showed that the acquisition of mobile genetic elements (MGE) plays an important role in the first stages of genetic divergence between isolates, increasing the genome size of E. coli. The source of isolation would be determined by the number of MGEs acquired, reinforcing the idea that there is an association of certain genetic backgrounds with specific environments. Therefore, understanding the genetic context of each source of isolation is be a key step in understanding the movement of genes by including resistance and virulence genes at a specific location (Touchon et al., 2020). Consequently, it is very important to study the genomic relationships and MGEs of CRRE isolates found in food and to compare them with isolates obtained from human clinical cases or human colonization in healthy people.
To use WGS to infer genomic relationships of CRRE is not an easy task; mostly because the plausible effect of consumption of CRRE in food is not expected to represent an acute disease, but rather gastrointestinal colonization is proposed (Hölzel et al., 2018). Furthermore, the term foodborne urinary tract infection (FUTI) was also proposed as a new paradigm widening the perspective on the public health effects of CRRE in food (Nordstrom et al., 2013; Liu et al., 2018b). WGS was used to study the genomic relationships of CRRE from food to humans using a One Health approach. A 1-year study in Arizona compared the genomes of E. coli isolates from meat products and isolates from human urinary and blood sources (Liu et al., 2018b). The study found one sublineage of E. coli ST131 found in poultry meat and human clinical cases raising the hypothesis of foodborne transmission and remarking the role of antimicrobial resistant E. coli in poultry meat (Liu et al., 2018b). Importantly, the ability to cause UTI of E. coli isolates obtained from different meats was proven in murine models (Jakobsen et al., 2012).
Here, WGS has been an important tool to study the potential public health role of CRRE in food, and the whole transmission route is not completely understood. The variety of food types in which CRRE have been reported include food that is not further processed at home (e.g., fruits and vegetables). To better understand this, CRRE obtained from different food types need to be studied along with human gastrointestinal colonization and clinical cases (Figure 1). WGS can provide information about virulence potential, adaptation and survival such as resistance to biocides, metals, and antimicrobial drugs along with the plasticity of genomes of food isolates. For example, in antibiotic-resistant Salmonella obtained from retail meats and clinical isolates, WGS identified the resistance genes and the genomic environment of these genes (Keefer et al., 2019) providing unique and valuable information for the study of the spread of antimicrobial resistance. Similarly, virulence-associated genes of ESBL-producing K. pneumoniae from different sources including vegetables has been identified by using WGS (Chi et al., 2019). Both examples provide an enormous advance and facilitate the detection of potential risks to public health.
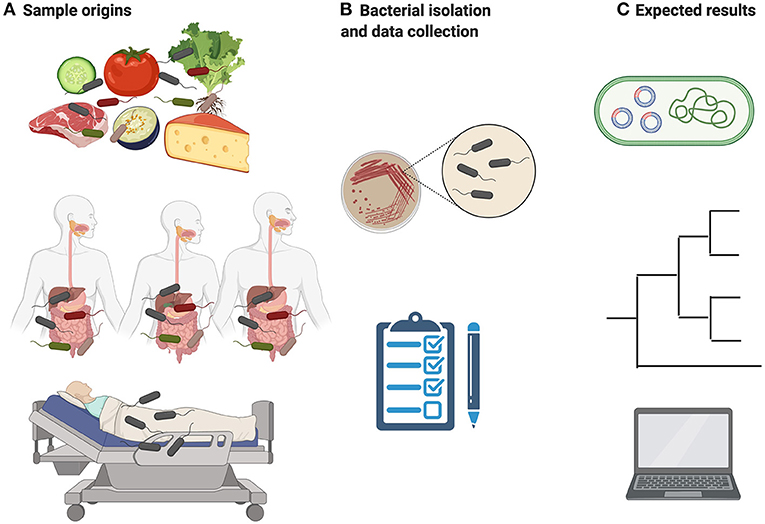
Figure 1. Schematic representation of use of whole genome sequencing to determine genetic relatedness of clinically relevant resistant Enterobacterales (CRRE) presence in food and humans. (A) Samples from three distinct origins including different food types, human samples of cohort participants colonized in the community and samples from hospitalized patients with a bloodstream infection. (B) Isolation of CRRE from the origins in (A) along with data collection. (C) Expected results include identification of mobile elements and phylogenetic relatedness on CRRE from these three origins, and prediction of risk factors using computational analysis.
In Chile, the Millennium Initiative for Collaborative Research on Bacterial Resistance (microb-r.org; which is a multi-institution research center focusing on the study of AMR) partnered with the Maule Cohort (MAUCO) (Ferreccio et al., 2016, 2020). Here, suitable conditions are given to study AMR and to use WGS of CRRE obtained from different food types and from human volunteers (10,000 adults inhabitants) from Molina county where the MAUCO cohort is located. MAUCO cohort's participants represent a unique opportunity to advance the current understanding on the public health consequences of the presence of CRRE in the food supply. Close genomic relatedness of CRRE from different sources could help to trace isolates from the food supply to human gastrointestinal tracts, to understand the movements of mobile elements as plasmids and resistance genes in CRRE from different food and water sources, and to infer phylogenetic relationships (Figure 1). Colonization is one aspect but clones that are capable of causing bloodstream infections can also be traced to gastrointestinal colonization and to food using WGS. Identification of clonal isolates in food, in colonized participants, and finally in bloodstream infections could possible close the current gap and provide important data to policy makers.
The isolation of CRRE from samples from three main compartments are necessary to obtain relevant data (Figure 1). Importantly, WGS of isolated CRRE along with metadata from the cohort participants obtained through, for instance questionnaires or participants records, could provide information on susceptible populations related to foodborne acquisition of CRRE. The elderly or immuno-compromised individuals are more susceptible to foodborne infections (Mohácsi-Farkas, 2016), and thus we expect that this is particularly important to them. Moreover, this approach can also include the impact of chronic diseases (e.g., diabetes, hypertension), along with risk factors such as antimicrobial therapy, patterns of food consumption, among others (Figure 1).
Discussion
The clinical interest in antimicrobial-resistant bacteria strikes at the hospitals where therapeutic options to treat infections caused by, for instance carbapenem resistant or ESBL producer Enterobacterias are limited (Rodriguez-Baño et al., 2018). Reports on the detection of bacteria resistant to clinically relevant antibiotics associated with food have increased worldwide, however, the outcome of CRRE in different food types and the potential public health impacts are currently unknown. Colonization with carbapenem resistant or ESBL-producing Enterobacterales has been described as one plausible outcome (Hölzel et al., 2018). The consumption of food harboring CRRE could further colonize the human gut—this will not necessarily cause or either be associated with a known foodborne disease. In addition, colonization could further worsen some conditions, e.g., recent evidence has shown that individuals colonized by multidrug resistant microorganisms are more likely to present systemic infections such as septicemia with a higher chance of treatment failure than not colonized ones (Ferreira et al., 2018). Several questions remain and important knowledge gaps need to be filled. These include a better understanding on the dose-response (Hölzel et al., 2018) and a genomic-based approach that better explains the distribution and closeness with resistant bacteria present in the human gastrointestinal tract with bacteria obtained from different food types.
Many antibiotics of human interest are used in domestic animals and may reach food and the environment, although AMR in food and the role in the overall AMR crisis are difficult to compare due to a lack of published studies or surveillance programs in animal, environmental, or food samples. For instance only a small fraction of food types have been studied as carriers of CRRE (Hudson et al., 2017; Schrijver et al., 2017). To avoid this problem, it is recommended to design studies with statistical support to determine the number of samples and genomes needed. It is also necessary to use methodologies that make relationships between what has been found in animals, food, and the environment with all the antecedents that currently exist in humans (the most common studied). Here, the use of study cohorts is recommended and can help to explain risk factors, the origin of some resistance plasmids or multidrug resistant bacteria, CRRE, etc. (Arcilla et al., 2017; Barría, 2018).
Genomic tools and longitudinal study design at a global level, can improve food safety and ensure a good surveillance system that is reliable, reproducible, and sustainable. This promotes collaborative studies among different regions or countries. Importantly, the developed countries could work together with underdeveloped countries, which do not have the financing or tools necessary to carry out this type of study and which are often suppliers of food of animal or vegetable origin (Errecart, 2015; Organización Naciones Unidas, 2019).
Finally, we believe that, in addition to the development of new antimicrobials, it is of extreme importance to carry out the follow-up and analysis of carbapenem-resistant and ESBL-producing Enterobacterales. The WGS, the generation of worldwide databases, and the publication of standard protocols for working with these pathogens in food are needed.
Data Availability Statement
The original contributions presented in the study are included in the article/supplementary material, further inquiries can be directed to the corresponding author/s.
Author Contributions
CD-G, FPÁ, and AM-S: wrote the manuscript. JM and SC: critically reviewed the manuscript. All authors contributed to the article and approved the submitted version.
Funding
This work was funded by FONDECYT 1181167 and by the ANID Millennium Science Initiative/Millennium Initiative for Collaborative Research on Bacterial Resistance, MICROB-R, NCN17_081.
Conflict of Interest
The authors declare that the research was conducted in the absence of any commercial or financial relationships that could be construed as a potential conflict of interest.
Acknowledgments
Figure 1 was created with BioRender.com.
References
Abdallah, H. M., Reuland, E. A., Wintermans, B. B., Al Naiemi, N., Koek, A., Abdelwahab, A. M., et al. (2015). Extended-spectrum β-lactamases and/or carbapenemases-producing Enterobacteriaceae isolated from retail chicken meat in Zagazig, Egypt. PLoS ONE 10:e0136052. doi: 10.1371/journal.pone.0136052
Allard, M. W., Bell, R., Ferreira, C. M., Gonzalez-Escalona, N., Hoffmann, M., Muruvanda, T., et al. (2018). Genomics of foodborne pathogens for microbial food safety. Curr. Opin. Biotechnol. 49, 224–229. doi: 10.1016/j.copbio.2017.11.002
Arcilla, M. S., van Hattem, J. M., Haverkate, M. R., Bootsma, M. C. J., van Genderen, P. J. J., Goorhuis, A., et al. (2017). Import and spread of extended-spectrum β-lactamase-producing Enterobacteriaceae by international travellers (COMBAT study): a prospective, multicentre cohort study. Lancet Infect. Dis. 17, 78–85. doi: 10.1016/S1473-3099(16)30319-X
Barría, R. M. (2018). “Introductory chapter: the contribution of cohort studies to health sciences,” in Cohort Studies in Health Sciences, ed R. Mauricio Barría (IntechOpen). doi: 10.5772/intechopen.80178
Ben-David, D., Kordevani, R., Keller, N., Tal, I., Marzel, A., Gal-Mor, O., et al. (2012). Outcome of carbapenem resistant Klebsiella pneumoniae bloodstream infections. Clin. Microbiol. Infect. 18, 54–60. doi: 10.1111/j.1469-0691.2011.03478.x
Bhutani, N., Muraleedharan, C., Talreja, D., Rana, S. W., Walia, S., Kumar, A., et al. (2015). Occurrence of multidrug resistant extended spectrum beta-lactamase-producing bacteria on iceberg lettuce retailed for human consumption. Biomed Res. Int. 2015:547547. doi: 10.1155/2015/547547
Botelho, L. A. B., Kraychete, G. B., Rocha, P. B., Da-Silva, A. P. D. S., Picão, R. C., Moreira, B. M., et al. (2020). CTX-M- And pAmpC-encoding genes are associated with similar mobile genetic elements in Escherichia coli isolated from different brands of Brazilian chicken meat. Microb. Drug Resist. 26, 14–20. doi: 10.1089/mdr.2019.0043
Brown, E., Dessai, U., Mcgarry, S., and Gerner-smidt, P. (2019). Use of whole-genome sequencing for food safety and public health in the United States. 16, 441–450. doi: 10.1089/fpd.2019.2662
Carmeli, Y., Harbarth, S., Kahlmeter, G., Kluytmans, J., Mendelson, M., Hospital, G. S., et al (2017). Global priority list of antibiotic-resistant bacteria to guide research, discovery, and development of new antibiotics. J. Med. Soc. 32:76. Available online at: https://www.who.int/medicines/publications/global-priority-list-antibiotic-resistant-bacteria/en/ (accessed April 30, 2021).
Chi, X., Berglund, B., Zou, H., Zheng, B., Börjesson, S., Ji, X., et al. (2019). Characterization of clinically relevant strains of extended-spectrum ß-lactamase-producing klebsiella pneumoniae occurring in environmental sources in a rural area of China by using whole-genome sequencing. Front. Microbiol. 10:211. doi: 10.3389/fmicb.2019.00211
Chioro, A., Coll-Seck, A. M., Høie, B., Moeloek, N., Motsoaledi, A., Rajatanavin, R., et al. (2015). Antimicrobial resistance: a priority for global health action. Bull. World Health Organ. 93:439. doi: 10.2471/BLT.15.158998
Clemente, L., Manageiro, V., Correia, I., Amaro, A., Albuquerque, T., Themudo, P., et al. (2019). Revealing mcr-1-positive ESBL-producing Escherichia coli strains among Enterobacteriaceae from food-producing animals (bovine, swine and poultry) and meat (bovine and swine), Portugal, 2010–2015. Int. J. Food Microbiol. 296, 37–42. doi: 10.1016/j.ijfoodmicro.2019.02.006
Dadgostar, P. (2019). Antimicrobial resistance: implications and costs. Infect. Drug Resist. 12, 3903–3910. doi: 10.2147/IDR.S234610
Errecart, V. (2015). Análisis del mercado mundial de carnes. Cere 1:35. Available online at: http://www.unsam.edu.ar/escuelas/economia/economia_regional/CERE-Mayo-2015.pdf (accessed April 30, 2021).
European Centre for Disease Prevention Control (ECDC). (2021). ECDC Strategic Framework for the Integration of Molecular and Genomic Typing Into European Surveillance and Multi-Country Outbreak Investigations 2019-2021. Available online at: https://www.ecdc.europa.eu/en/publications-data/ecdc-strategic-framework-integration-molecular-and-genomic-typing-european (accessed March 15, 2021).
Ferreccio, C., Huidobro, A., Cortés, S., Bambs, C., Toro, P., Van De Wyngard, V., et al. (2020). Cohort profile: the maule cohort (MAUCO). Int. J. Epidemiol. 49, 760–761. doi: 10.1093/ije/dyaa003
Ferreccio, C., Roa, J. C., Bambs, C., Vives, A., Corvalán, A. H., Cortés, S., et al. (2016). Study protocol for the Maule Cohort (MAUCO) of chronic diseases, Chile 2014-2024. BMC Public Health 16, 1–7. doi: 10.1186/s12889-015-2454-2
Ferreira, A. M., Moreira, F., Guimaraes, T., Spadão, F., Ramos, J. F., Batista, M. V., et al. (2018). Epidemiology, risk factors and outcomes of multi-drug-resistant bloodstream infections in haematopoietic stem cell transplant recipients: importance of previous gut colonization. J. Hosp. Infect. 100, 83–91. doi: 10.1016/j.jhin.2018.03.004
Garcia-Graells, C., Berbers, B., Verhaegen, B., Vanneste, K., Marchal, K., Roosens, N. H. C., et al. (2020). First detection of a plasmid located carbapenem resistant blaVIM-1 gene in E. coli isolated from meat products at retail in Belgium in 2015. Int. J. Food Microbiol. 324:108624. doi: 10.1016/j.ijfoodmicro.2020.108624
Ghafourian, S., Sadeghifard, N., Soheili, S., and Sekawi, Z. (2015). Extended spectrum beta-lactamases: definition, classification and epidemiology. Curr. Issues Mol. Biol. 17, 11–22. doi: 10.21775/cimb.017.011
Gona, F., Comandatore, F., Battaglia, S., Piazza, A., Trovato, A., Lorenzin, G., et al. (2020). Comparison of core-genome MLST, coreSNP and PFGE methods for Klebsiella pneumoniae cluster analysis. Microb. Genomics 6:e000347. doi: 10.1099/mgen.0.000347
Hassen, B., Abbassi, M. S., Ruiz-Ripa, L., Mama, O. M., Hassen, A., Torres, C., et al. (2020). High prevalence of mcr-1 encoding colistin resistance and first identification of blaCTX-M-55 in ESBL/CMY-2-producing Escherichia coli isolated from chicken faeces and retail meat in Tunisia. Int. J. Food Microbiol. 318:108478. doi: 10.1016/j.ijfoodmicro.2019.108478
Hayashi, W., Ohsaki, Y., Taniguchi, Y., Koide, S., Kawamura, K., Suzuki, M., et al. (2018). High prevalence of blaCTX-M-14 among genetically diverse Escherichia coli recovered from retail raw chicken meat portions in Japan. Int. J. Food Microbiol. 284, 98–104. doi: 10.1016/j.ijfoodmicro.2018.08.003
Hoang, T. A. V., Nguyen, T. N. H., Ueda, S., Le, Q. P., Tran, T. T. N., Nguyen, T. N. D., et al. (2017). Common findings of bla CTX-M-55-encoding 104–139 kbp plasmids harbored by extended-spectrum β-lactamase-producing Escherichia coli in pork meat, wholesale market workers, and patients with urinary tract infection in Vietnam. Curr. Microbiol. 74, 203–211. doi: 10.1007/s00284-016-1174-x
Hoffman, S. J., Outterson, K., Røttingen, J. A., Cars, O., Clift, C., Rizvi, Z., et al. (2015). An international legal framework to address antimicrobial resistance. Bull. World Health Organ. 93:66. doi: 10.2471/BLT.15.152710
Hoffmann, S., Devleesschauwer, B., Aspinall, W., Cooke, R., Corrigan, T., Havelaar, A., et al. (2017). Attribution of global foodborne disease to specific foods: Findings from a World Health Organization structured expert elicitation. PLoS ONE 12:e0183641. doi: 10.1371/journal.pone.0183641
Hölzel, C. S., Tetens, J. L., and Schwaiger, K. (2018). Unraveling the role of vegetables in spreading antimicrobial-resistant bacteria: a need for quantitative risk assessment. Foodborne Pathog. Dis. 15, 671–688. doi: 10.1089/fpd.2018.2501
Hudson, J. A., Frewer, L. J., Jones, G., Brereton, P. A., Whittingham, M. J., and Stewart, G. (2017). The agri-food chain and antimicrobial resistance: a review. Trends Food Sci. Technol. 69, 131–147. doi: 10.1016/j.tifs.2017.09.007
Huizinga, P., Van Den Bergh, M. K., Rossen, J. W., Willemsen, I., Verhulst, C., Savelkoul, P. H. M., et al. (2019). Decreasing prevalence of contamination with extended-spectrum beta-lactamaseproducing Enterobacteriaceae (ESBL-E) in retail chicken meat in the Netherlands. PLoS ONE 14:e0226828. doi: 10.1371/journal.pone.0226828
Jakobsen, L., Garneau, P., Bruant, G., Harel, J., Olsen, S. S., Porsbo, L. J., et al. (2012). Is Escherichia coli urinary tract infection a zoonosis? Proof of direct link with production animals and meat. Eur. J. Clin. Microbiol. Infect. Dis. 31, 1121–1129. doi: 10.1007/s10096-011-1417-5
Kaesbohrer, A., Bakran-Lebl, K., Irrgang, A., Fischer, J., Kämpf, P., Schiffmann, A., et al. (2019). Diversity in prevalence and characteristics of ESBL/pAmpC producing E. coli in food in Germany. Vet. Microbiol. 233, 52–60. doi: 10.1016/j.vetmic.2019.03.025
Keefer, A. B., Xiaoli, L., M'ikanatha, N. M., Yao, K., Hoffmann, M., and Dudley, E. G. (2019). Retrospective whole-genome sequencing analysis distinguished PFGE and drug resistance matched retail meat and clinical Salmonella isolates. bioRxiv. 165, 270–286. doi: 10.1101/356857
Kelly, A. M., Mathema, B., and Larson, E. L. (2017). Carbapenem-resistant Enterobacteriaceae in the community: a scoping review. Int. J. Antimicrob. Agents. 50, 127–134. doi: 10.1016/j.ijantimicag.2017.03.012
Kim, H. S., Chon, J. W., Kim, Y. J., Kim, D. H., Kim, M., Sang, and Seo, K. H. (2015). Prevalence and characterization of extended-spectrum-β-lactamase-producing Escherichia coli and Klebsiella pneumoniae in ready-to-eat vegetables. Int. J. Food Microbiol. 207, 83–86. doi: 10.1016/j.ijfoodmicro.2015.04.049
Kluytmans-van den bergh, M., Huizinga, P., Bonten, M. J., Bos, M., De Bruyne, K., Friedrich, A. W., et al. (2016). Presence of mcr-1-positive Enterobacteriaceae in retail chicken meat but not in humans in the Netherlands since 2009. Eurosurveillance 21:30149. doi: 10.2807/1560-7917.ES.2016.21.9.30149
Kovac, J., Bakker, H., Den, Carroll, L. M., and Wiedmann, M.. (2017). Precision food safety : a systems approach to food safety facilitated by genomics tools. Trends Anal. Chem. 96, 52–61. doi: 10.1016/j.trac.2017.06.001
Li, L., Ye, L., Yu, L., Zhou, C., and Meng, H. (2016). Characterization of extended spectrum -lactamase producing enterobacteria and methicillin-resistant Staphylococcus aureus isolated from raw pork and cooked pork products in South China. J. Food Sci. 81, M1773–M1777. doi: 10.1111/1750-3841.13346
Lipari, F. G., Hernández, D., Vilar,ó, M., Caeiro, J. P., and Saka, H. A. (2020). Caracterización clínica, epidemiológica y microbiológica de bacteriemias producidas por enterobacterias resistentes a carbapenems en un hospital universitario de Córdoba, Argentina. Rev. Chil. Infectol. 37, 362–370. doi: 10.4067/S0716-10182020000400362
Liu, B. T., and Song, F. J. (2019). Emergence of two Escherichia coli strains co-harboring mcr-1 and blaNDM in fresh vegetables from China. Infect. Drug Resist. 12:2627. doi: 10.2147/IDR.S211746
Liu, B. T., Song, F. J., Zou, M., Zhang, Q., Di, and Shan, H. (2017). High incidence of Escherichia coli strains coharboring mcr-1 and blaNDM from chickens. Antimicrob. Agents Chemother. 61, e02347–e02316. doi: 10.1128/AAC.02347-16
Liu, B. T., Zhang, X. Y., Wan, S. W., Hao, J. J., Jiang, R., De, and Song, F. J. (2018a). Characteristics of carbapenem-resistant Enterobacteriaceae in ready-to-eat vegetables in China. Front. Microbiol. 9:1147. doi: 10.3389/fmicb.2018.01147
Liu, C. M., Stegger, M., Aziz, M., Johnson, T. J., Waits, K., Nordstrom, L., et al. (2018b). Escherichia coli ST131-H22 as a foodborne uropathogen. MBio. 9, e00470–e00418. doi: 10.1128/mBio.00470-18
Logan, L. K., and Weinstein, R. A. (2017). The epidemiology of carbapenem-resistant Enterobacteriaceae: The impact and evolution of a global menace. J. Infect. Dis. 2215, S28–S36. doi: 10.1093/infdis/jiw282
Ludden, C., Moradigaravand, D., Jamrozy, D., Gouliouris, T., Blane, B., Naydenova, P., et al. (2020). A one health study of the genetic relatedness of Klebsiella pneumoniae and their mobile elements in the east of England. Clin. Infect. Dis. 70, 219–226. doi: 10.1093/cid/ciz174
Menichetti, F., and Tagliaferri, E. (2012). Antimicrobial resistance in internal medicine wards. Intern. Emerg. Med. 7, 271–281. doi: 10.1007/s11739-012-0828-3
Mohácsi-Farkas, C. (2016). Food irradiation: Special solutions for the immuno-compromised. Radiat. Phys. Chem. 129, 58–60. doi: 10.1016/j.radphyschem.2016.06.033
Montso, K. P., Dlamini, S. B., Kumar, A., Ateba, C. N., and Garcia-Perdomo, H. A. (2019). Antimicrobial resistance factors of extended-spectrum Beta-lactamases producing Escherichia coli and Klebsiella pneumoniae isolated from cattle farms and raw beef in North-West Province, South Africa. Biomed Res. Int. 2019:4318306. doi: 10.1155/2019/4318306
Nastasijevic, I., Milanov, D., Velebit, B., Djordjevic, V., Swift, C., Painset, A., et al. (2017). Tracking of Listeria monocytogenes in meat establishment using whole genome sequencing as a food safety management tool: a proof of concept. Int. J. Food Microbiol. 257, 157–164. doi: 10.1016/j.ijfoodmicro.2017.06.015
Nordmann, P., Naas, T., and Poirel, L. (2011). Global spread of carbapenemase producing Enterobacteriaceae. Emerg. Infect. Dis. 17:1791. doi: 10.3201/eid1710.110655
Nordstrom, L., Liu, C. M., and Price, L. B. (2013). Foodborne urinary tract infections: a new paradigm for antimicrobial-resistant foodborne illness. Front. Microbiol. 4:29. doi: 10.3389/fmicb.2013.00029
Organización Naciones Unidas (2019). Récord de Economí as Dependientes de la Exportación de Productos Básicos. Asuntos económicos. Available online at: https://news.un.org/es/story/2019/05/1455981 (accessed March 15, 2021).
Pehlivanlar Önen, S., Aslantaş, Ö., Sebnem, Yilmaz, E., and Kürekci, C. (2015). Prevalence of β-lactamase producing Escherichia coli from retail meat in Turkey. J. Food Sci. 80, M2023–M2029. doi: 10.1111/1750-3841.12984
Pijnacker, R., Dallman, T. J., Tijsma, A. S. L., Hawkins, G., Larkin, L., Kotila, S. M., et al. (2019). Articles an international outbreak of Salmonella enterica serotype enteritidis linked to eggs from Poland: a microbiological and epidemiological study. Lancet Infect Dis. 19, 778–786. doi: 10.1016/S1473-3099(19)30047-7
Pokharel, S., Shrestha, P., and Adhikari, B. (2020). Antimicrobial use in food animals and human health: time to implement ‘One Health’ approach. Antimicrob. Resist. Infect. Control. 9:181. doi: 10.1186/s13756-020-00847-x
Randall, L. P., Lodge, M. P., Elviss, N. C., Lemma, F. L., Hopkins, K. L., Teale, C. J., et al. (2017). Evaluation of meat, fruit and vegetables from retail stores in five United Kingdom regions as sources of extended-spectrum beta-lactamase (ESBL)-producing and carbapenem-resistant Escherichia coli. Int. J. Food Microbiol. 241, 283–290. doi: 10.1016/j.ijfoodmicro.2016.10.036
Rasmussen, M. M., Opintan, J. A., Frimodt-Møller, N., and Styrishave, B. (2015). Beta-lactamase producing Escherichia coli isolates in imported and locally produced chicken meat from Ghana. PLoS ONE 10:e0139706. doi: 10.1371/journal.pone.0139706
Rebbah, N., Messai, Y., Châtre, P., Haenni, M., Madec, J. Y., and Bakour, R. (2018). Diversity of CTX-M extended-spectrum β-Lactamases in Escherichia coli isolates from retail raw ground beef: First report of CTX-M-24 and CTX-M-32 in Algeria. Microb. Drug Resist. 24, 896–908. doi: 10.1089/mdr.2017.0171
Reuland, E. A., al Naiemi, N., Raadsen, S. A., Savelkoul, P. H. M., Kluytmans, J. A. J. W., and Vandenbroucke-Grauls, C. M. J. E. (2014). Prevalence of ESBL-producing Enterobacteriaceae in raw vegetables. Eur. J. Clin. Microbiol. Infect. Dis. 33, 1843–1846. doi: 10.1007/s10096-014-2142-7
Rodriguez-Baño, J., Gutiérrez-Gutiérrez, B., Machuca, I., and Pascual, A. (2018). Treatment of infections caused by Extended-Spectrum-Beta-Lactamase-, AmpC-, and carbapenemase-producing Enterobacteriaceae. Clin. Microbiol. Rev. 31, e00079–e00017. doi: 10.1128/CMR.00079-17
Saidani, M., Messadi, L., Soudani, A., Daaloul-Jedidi, M., Châtre, P., Ben Chehida, F., et al. (2018). Epidemiology, antimicrobial resistance, and extended-spectrum beta-lactamase-producing Enterobacteriaceae in clinical bovine mastitis in Tunisia. Microb. Drug Resist. 24, 1242–1248. doi: 10.1089/mdr.2018.0049
Salipante, S. J., SenGupta, D. J., Cummings, L. A., Land, T. A., Hoogestraat, D. R., and Cookson, B. T. (2015). Application of whole-genome sequencing for bacterial strain typing in molecular epidemiology. J. Clin. Microbiol. 53, 1072–1079. doi: 10.1128/JCM.03385-14
Schill, F., Abdulmawjood, A., Klein, G., and Reich, F. (2017). Prevalence and characterization of extended-spectrum β-lactamase (ESBL) and AmpC β-lactamase producing Enterobacteriaceae in fresh pork meat at processing level in Germany. Int. J. Food Microbiol. 257, 58–66. doi: 10.1016/j.ijfoodmicro.2017.06.010
Schrijver, R., Stijntjes, M., Rodríguez-Baño, J., Tacconelli, E., Babu Rajendran, N., and Voss, A. (2017). Review of antimicrobial resistance surveillance programmes in livestock and their meat in Europe, with a focus on antimicrobial resistance patterns in humans. Clin. Microbiol. Infect. 24, 577–590. doi: 10.1016/j.cmi.2017.09.013
Sugawara, Y., Hagiya, H., Akeda, Y., Aye, M. M., Myo Win, H. P., Sakamoto, N., et al. (2019). Dissemination of carbapenemase-producing Enterobacteriaceae harbouring bla NDM or bla IMI in local market foods of Yangon, Myanmar. Sci. Rep. 9:14455. doi: 10.1038/s41598-019-51002-5
Tadesse, D. A., Li, C., Mukherjee, S., Hsu, C. H., Bodeis Jones, S., Gaines, S. A., et al. (2018). Whole-genome sequence analysis of CTX-M containing Escherichia coli isolates from retail meats and cattle in the United States. Microb. Drug Resist. 24, 939–948. doi: 10.1089/mdr.2018.0206
Tekiner, I. H., and Özpinar, H. (2016). Occurrence and characteristics of extended spectrum beta-lactamases-producing Enterobacteriaceae from foods of animal origin. Brazilian J. Microbiol. 47, 444–451. doi: 10.1016/j.bjm.2015.11.034
Temkin, E., Adler, A., Lerner, A., and Carmeli, Y. (2014). Carbapenem-resistant Enterobacteriaceae: biology, epidemiology, and management. Ann. N. Y. Acad. Sci. 1323, 22–42. doi: 10.1111/nyas.12537
Touchon, M., Perrin, A., De Sousa, J. A. M., Vangchhia, B., Burn, S., O'Brien, C. L., et al. (2020). Phylogenetic background and habitat drive the genetic diversification of Escherichia coli. PLoS Genet. 16:e1008866. doi: 10.1371/journal.pgen.1008866
van Duin, D., and Doi, Y. (2017). The global epidemiology of carbapenemase-producing Enterobacteriaceae. Virulence 8, 460–469. doi: 10.1080/21505594.2016.1222343
Van Loon, K., Voor In'T Holt, A. F., and Vos, M. C. (2018). A systematic review and meta-analyses of the clinical epidemiology of carbapenem-resistant Enterobacteriaceae. Antimicrob. Agents Chemother. 62, e01730–e01717. doi: 10.1128/AAC.01730-17
Wang, S., Weller, D., Falardeau, J., Strawn, L. K., Fernando, O., Adell, A. D., et al. (2016). Food safety trends: from globalization of whole genome sequencing to application of new tools to prevent foodborne diseases. Trends Food Sci. Technol. 57, 188–198. doi: 10.1016/j.tifs.2016.09.016
World Health Organization (2020). (WHO) Antimicrobial Resistance. Available online at: https://www.who.int/news-room/fact-sheets/detail/antimicrobial-resistance (accessed February 8, 2021).
World Health Organization (2021). FAO/OIE/WHO Tripartite Collaboration on AMR. Food safrty. Available online at: https://www.who.int/foodsafety/areas_work/antimicrobial-resistance/tripartite/en/ (accessed February 4, 2021).
Ye, Q., Wu, Q., Zhang, S., Zhang, J., Yang, G., Wang, J., et al. (2018). Characterization of extended-spectrum β-lactamase-producing Enterobacteriaceae from retail food in China. Front. Microbiol. 9:1709. doi: 10.3389/fmicb.2018.01709
Zhang, Q., Lv, L., Huang, X., Huang, Y., Zhuang, Z., Lu, J., et al. (2019). Rapid increase in carbapenemase-producing Enterobacteriaceae in retail meat driven by the spread of the blaNDM-5-carrying IncX3 plasmid in China from 2016 to 2018. Antimicrob. Agents Chemother. 63, e00573–e00519. doi: 10.1128/AAC.00573-19
Keywords: antimicrobial resistance, genomics, clinically relevant bacteria, Escherichia coli, Klebsiella pneumoniae, food safety, resistant bacteria in food
Citation: Díaz-Gavidia C, Álvarez FP, Munita JM, Cortés S and Moreno-Switt AI (2021) Perspective on Clinically-Relevant Antimicrobial Resistant Enterobacterales in Food: Closing the Gaps Using Genomics. Front. Sustain. Food Syst. 5:667504. doi: 10.3389/fsufs.2021.667504
Received: 13 February 2021; Accepted: 12 April 2021;
Published: 14 May 2021.
Edited by:
Matthew D. Moore, University of Massachusetts, Amherst, United StatesReviewed by:
Yanhong Liu, United States Department of Agriculture (USDA), United StatesBen Davies Tall, United States Food and Drug Administration, United States
Séamus Fanning, University College Dublin, Ireland
Copyright © 2021 Díaz-Gavidia, Álvarez, Munita, Cortés and Moreno-Switt. This is an open-access article distributed under the terms of the Creative Commons Attribution License (CC BY). The use, distribution or reproduction in other forums is permitted, provided the original author(s) and the copyright owner(s) are credited and that the original publication in this journal is cited, in accordance with accepted academic practice. No use, distribution or reproduction is permitted which does not comply with these terms.
*Correspondence: Andrea I. Moreno-Switt, andrea.moreno@uc.cl