- 1Department of Atmospheric, Ocean and Earth Sciences, George Mason University, Fairfax, VA, United States
- 2The Nature Conservancy, Arlington, VA, United States
This paper presents a synthesis of evidence and implementation gaps in the application of nature-based solutions (NbS) in agricultural landscapes that contribute to reduce trade-offs between food production, climate change and conservation objectives. The literature and data surveyed relies primarily in peer-reviewed sources and is organized around an overview of NbS science and applications in agricultural landscapes in major biomes. To date, the focus of NbS applications in food production has been predominantly for carbon sequestration, water quality, and disaster-risk management objectives while documented examples of NbS benefits in agricultural production are sparse. Conservation applications of NbS appear to show evidence of effectiveness across multiple objectives in biodiversity, land, soil and water. Evidence and analysis of NbS to meet climate change mitigation targets has surged in recent years driven by global community efforts. Overall, considerable scientific work remains to refine and reduce the uncertainty of NbS benefit estimates across production, climate and conservation objectives, and resilience implications. However, delaying implementation of NbS in agricultural landscapes would likely increase the costs to meet agricultural production, climate, conservation and other societally beneficial goals, while degrading the capacity of natural systems to continue to provide these and other ecosystem services.
1 Introduction
In recent years, considerable progress has been made in the area of nature-based solutions (NbS) that improve ecosystem functions of environments and landscapes affected by anthropogenic practices and interventions, while enhancing livelihoods and other social and cultural functions. This has opened a portfolio of NbS options that offer a pragmatic way forward for simultaneously addressing climate, conservation, and socioeconomic objectives while maintaining healthy and productive land and water systems. NbS can mimic natural processes and build on operational land-water management concepts that aim to simultaneously improve water availability and quality and raise agricultural productivity (Griscom et al., 2017; Sonneveld et al., 2018; Muller et al., 2021). NbS can involve conserving or rehabilitating natural ecosystems and/or the enhancement or the creation of natural processes in modified or artificial ecosystems (Dewi et al., 2013; WWAP, 2018; Adewopo, 2019). For instance, in agricultural landscapes, NbS can be applied for soil health, soil moisture, carbon mitigation (through soil and forestry), downstream water quality protections, biodiversity benefits as well as agricultural production and supply chains to achieve net-zero environmental impacts while achieving food and water security and meet climate goals.
Many examples in the literature on NbS practices have focused on highlighting the resilience trade-offs in production vs. conservation, e.g., sparing vs. sharing (Franklin and Mortensen, 2012), intensification vs. sustainable production (Matocha et al., 2012), agriculture vs. forestry (Adewopo, 2019), production forest vs. regeneration forest (Meyfroidt and Lambin, 2009; Dewi et al., 2013), short-term economic gains vs. long-term environmental benefits (Meyfroidt, 2018), among others. Integration across multiple objectives and identifying potential tradeoffs between them remaons a challenge in these investigations. This review article focuses on the application of NbS in agricultural landscapes (Muller et al., 2021) that contribute to reducing these deep uncertainty trade-offs between food production, conservation objectives and climate change. Specifically, this article provides a brief synthesis of the evidence and knowledge gaps in applications of NbS to agricultural landscapes toward achieving co-benefits in food production, climate change goals, and conservation of land, water, and biodiversity.
2 Methods
To define and classify NbS in agricultural landscapes, several typology efforts employed in the literature have been drawn upon. The Food and Agricultural Organization (FAO) has promoted a typology for NbS based on levels of human intervention (Eggermont et al., 2015; Sonneveld et al., 2018). Griscom et al. (2017) have defined Natural Climate Solutions which capture several NbS applications that intersect climate change mitigation and agriculture, while providing co-benefits in land, water, and biodiversity. This review provides a broader context that is representative of major efforts in the international community centered on the application of NbS to a variety of global issues (e.g., IPCC for climate change, IPBES for biodiversity, among others).
The literature and data surveyed relies primarily in peer-reviewed sources and is organized around an overview of NbS science and applications in agricultural landscapes in major biomes (forests, grasslands and croplands, and wetlands). These sources have been complemented with selected gray literature sources that provide evidence-based case study applications. For peer-reviewed literature, focus has been placed on the web of science, google scholar and science direct portals. Additionally, gray literature sources were obtained from organized literature outlets such as the World Overview of Conservation Approaches and Technologies (WOCAT), which focuses on documenting case study applications and best practices on NBS for sustainable land management. The Economics of Ecosystems and Biodiversity (TEEB) synthesis reports (TEEB, 2018a,b) were consulted to complement this review along the lines of economic considerations of ecosystem services in agriculture and food production. FAO's recent extensive report on The State of the World's Biodiversity for Food and Agriculture (FAO, 2019) was drawn from as a source of information linking biodiversity conservation to ecosystem services, provision of food security, resilience of food systems and support of livelihoods in agriculture.
This process yielded a significant body of peer-reviewed and gray literature and data sources with NbS applications across agricultural landscapes for a variety of objectives. Because of this abundance in available published work, this review has been structured by separately grouping the co-benefits provided by NbS into: (i) agricultural production; (ii) conservation (biodiversity, land, water); (iii) climate change; and (iv) socioeconomic considerations of NbS in agricultural landscapes. Synergies and trade-offs in resilience of these systems (production, land, water, biodiversity, climate, socioeconomics) has been noted in some of the sources surveyed to highlight NbS as a connector across multiple co-benefits; this occurs particularly in the climate-related references, which often encompass conservation and other co-benefits.
3 NbS and agricultural production
Case studies and quantification of co-benefits of NbS in agricultural landscapes have had a dominant focus on carbon sequestration, water quality, and disaster-risk management (Cohen-Shacham et al., 2016; FOLU, 2019), while specific examples of NbS benefits in agricultural production are sparse. For instance, in the Special Report on Climate Change and Land (IPCC, 2019) while forestry and water management are featured among the five NbS response options on “land management”, none explicitly stated agriculture, and in the same report, urban agriculture is reported under management of supply rather than of land, focusing NbS away from agricultural landscapes.
Examples of experiences in implementation of NbS in agricultural landscapes do suggest however a variety of co-benefits specific to resilience in agricultural production. For instance, some production-oriented practices make use of the multiple ecosystem functions of trees, plants and (wild or domesticated) animals for agricultural production, while minimizing the negative environmental impacts of the production (Daryanto et al., 2018) such as regenerative agriculture and conservation agriculture. Other documented practices are aimed at retaining or increasing available nutrients or improving the microclimate. For example, trees in alley cropping can provide shade among other roles, e.g., tree crops for food and fodder production, perennial alley crops, trees for crop facilitation via shade, within-system tree diversity (Wolz and DeLucia, 2018).
Many resilience-oriented practices drawing on agroecological principles (Altieri, 1992; FAO, 2018) or collectively referred to as climate-smart agriculture (FAO, 2013; Rosenstock et al., 2019), would also fall into this category. Specifically, in agroforestry and sloping agriculture land technologies, in addition to production contributions, plants may also perform NbS functions if, for example, planted as grass strips, or nitrogen-fixing legumes used as green mulch and fruit trees, planted along contours (Aguiar et al., 2015; McIvor et al., 2017; Are et al., 2018).
When agricultural species play the role of vegetation in NbS, multiple functions are rendered. For example, grass strips control soil erosion and return crop yields (Rosenstock et al., 2019) and vetiver grass can act as phytoremediation to trap phosphorous (Huang et al., 2019) while providing cut for animal feed. The efficiency of a catch crop also depends on physical elements, such as slope gradient (Novara et al., 2019) and root structure. Some research has reported on microterraces and built terraces as NbS for agriculture (Zuazo et al., 2011; Liu et al., 2018). In northern India for example, simple weed strips and weed mulch also created microterraces, which resulted in reduced soil erosion and higher yields (Lenka et al., 2017).
Other experiences are illustrative of agriculture-derived resilience co-benefits of NbS. For instance, trees in croplands or agroforestry (Gliessman, 2006) are an increasingly prominent example of a working landscape practice that can provide multiple economic, cultural and ecological benefits (FAO, 2005; World Agroforestry Centre, 2008). Agroforestry's diversified cropping systems mimicking natural forests form an important part of indigenous food production systems around the world and are also being used as a contemporary agricultural best management practice in non-traditional contexts. These systems tend to be resilient, productive, pest resistant, nutrient-conserving, and biodiverse, providing multiple economic, cultural and ecological benefits (Ewel, 1999). For example, they can provide fuelwood, cultivated foods, timber and medicinal plants for local communities (Junsongduang et al., 2013; Thaman, 2014), while also supporting high levels of biodiversity (Jose, 2009; Asfaw and Lemenih, 2010). These systems have also been shown to reduce sediment and nutrient runoff into adjacent watercourses and enhance carbon sequestration and storage (Montagnini and Nair, 2004; Bruun et al., 2009). Agroforestry systems also support a diversity of wild foods and provide pollinator habitat, both of which can help to combat malnutrition and micronutrient deficiencies (Johns, 2003; Steyn et al., 2006; DeClerck et al., 2011; Chaplin-Kramer et al., 2014; Ellis et al., 2015). A subset of agroforestry, silvopasture, integrates trees with pasture with the intention of increasing pasture quality and producing fodder while also protecting soils and vegetation.
Another type of NbS in agricultural landscapes, conservation agriculture, is defined by a combination of conservation tillage, crop rotations, and cover crops has gained traction in many parts of the world. In some regions, variations on the principles of conservation agriculture have been part of traditional agricultural systems for generations. Friedrich et al. (2012) reviews conservation agriculture applications around the world, with the greatest concentrations by far in United States, Brazil, Argentina, Australia, and Canada. The broad extent of this adoption has been cited as evidence of its implicit benefits for farmers (Brouder and Gomez-Macpherson, 2014).
There is evidence that conservation agriculture increases soil organic matter and a range of associated processes including improved sediment retention; however, crop yield outcomes vary based on practices employed, climate, crop type, and biophysical conditions (Palm et al., 2014). Available evidence on actual changes in crop yields suggests that conservation agriculture has the greatest potential to increase crop yields when implemented as a set of integrated practices in rainfed systems in water-limited or water-stressed regions, including potentially on millions of hectares in Sub-Saharan Africa and South Asia. Decisions to adopt conservation agriculture practices can go beyond immediate changes in crop yield, though. For example, a review of farmer adoption of conservation agriculture, identified reduction in farm operation costs, nutrient use and efficiency, water savings, and crop yield stability as additional factors beyond increased crop yield that motivated adoption (FAO, 2011).
Intensification of agriculture is a common policy intervention worldwide, both from a perspective of increased production and conservation perspectives, the latter in terms of the millions of hectares of forests which otherwise would be converted into farmland, provision of ecosystem services, and of some 590 billion tons of carbon prevented from being released into the atmosphere (Burney and Lobell, 2010). Rockström et al. (2017) describe the conditions and the elements of mainstreaming resilient agricultural intensification to reposition agriculture from being the major driver of global environmental change to a major contributor to the transition to sustainability through incorporating double objectives of increasing yields and enhancing the ecosystem services.
While a detailed characterization of agriculture-derived resilience provided by NbS, particularly in socioeconomic terms, remains to be done, it is anticipated that upscaling NbS implementation will need to adapt practices and strategies to the local biophysical, economic, and socio-cultural context and work to integrate local knowledge for effective results. Where they do so, existing sustainable agricultural systems can be supported, and less resilient practices shifted toward mutually beneficial outcomes for farmers and broader society.
4 NbS and conservation in agricultural landscapes
The recognition of NbS toward conservation co-benefits has been increasingly documented in the literature in recent years. For consistency, a taxonomy of conservation actions developed by the International Union for Conservation of Nature (IUCN) and the Conservation Measure Partnership (IUCN-CMP, 2006) was used to connect literature on NbS with a known set of conservation activities. These activities represent specific conservation, restoration, and improved land management actions that practitioners may take that are nature-based. Following this approach, co-benefits in biodiversity are defined as any increases in alpha, beta, and/or gamma diversity as described in the Convention on Biological Diversity (United Nations, 1992). For freshwater conservation, co-benefits relate to NbS applications in water resources management (e.g., flood and drought management), extreme weather events, developing drought-tolerant crops, choosing tree species and forestry practices less vulnerable to storms and fires, and other similar activities. Interventions here include source water protection, water flow regulation, water quality, and protection from droughts and flooding. Soil-related conservation co-benefits are characterized by improvement in metrics of soil quality that enhance productivity, maintain nutrient cycling, and improve plant growth (Shukla et al., 2006) as well as the improved potential food provision and reduced soil erosion services described in the Millennium Ecosystem Assessment (2005).
Various agricultural landscape approaches have been documented to achieve multiple conservation goals from ecological intensification of crop production with biodiversity focus (Garibaldi et al., 2019) to ecosystem services within payment-for-ecosystem-services (PES) schemes (Holt et al., 2016). A key objective with NbS practices in this category has been to ensure ecological connectivity of conservation agriculture on field-units across larger landscapes (Karabulut et al., 2019). Furthermore, species diversity plays important roles for recovery after disaster and preventive disaster risk reduction, such as mangroves protecting against storm surges (van Noordwijk et al., 2019).
Other cases in the literature illustrate the integration of practices to connect patches in the landscape. A number of cases across Europe implemented agrobiodiversity approaches, where permanent grassland and crop diversification within ecological focus areas involved a certain percent of arable land set aside to be used for field margins, hedges, trees, fallow land, landscape features, biotopes, buffer strips, and afforested areas (Delbaere et al., 2014). Similarly, connectivity was achieved with ecological infrastructure such as woodland hedges, grass strips, wildflower strips, and field margins (Rosas-Ramos et al., 2018). In Pakistan, an example of NbS practices include crop rotation, intercropping, agroforestry, crop diversification, live fencing, and wind barriers by trees (Shah et al., 2019). These examples illustrate a combination of practices that build up multiple conservation objectives, and also contribute to climate change emissions reduction.
Other NbS efforts have focused on source water protection in the provision of climate and conservation co-benefits (Vogl et al., 2017; Abell et al., 2019; Kroeger et al., 2019). Source water protection has broad geographic relevance for achieving multiple conservation objectives. An analysis of return on investment in NbS in watersheds around the world was performed as part of assessing enabling conditions for multiple climate and conservation objectives (biodiversity, water, soil). This analysis focused on estimation of potential water quality treatment savings (reduction in concentrations of sediments and Phosphorus) relative to upstream watershed NbS implementation costs. This information was used to classify “high-opportunity” watersheds for investments in NbS for source water protection. Figure 1 shows results generated from this analysis.
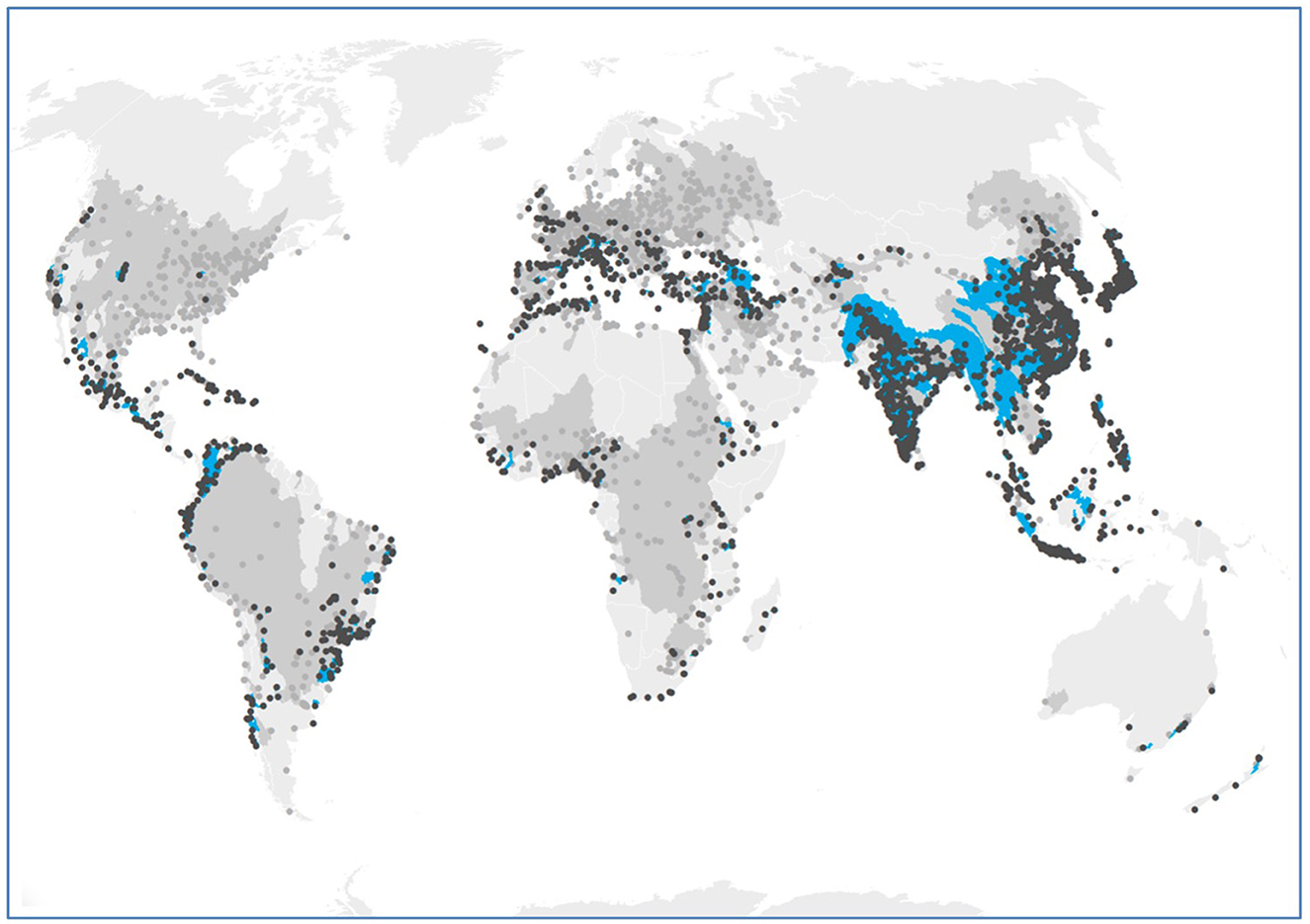
Figure 1. High opportunity watersheds for investments in nature-based solutions for protection of water sources (blue, highest priority; dark gray, all watersheds analyzed; black, recipient locations fed by water source); generated from data in Abell et al. (2019).
In a recent review, Miralles-Wilhelm et al. (2023) explore the concept of resilience in watersheds, linking ecological, social, engineering, and integrative approaches to define and apply systems thinking across multiple co-benefits. In this review, knowledge gaps are identified through an assessment of this literature and compilation of a set of research questions through stakeholder engagement activities. A research agenda describing key areas of inquiry such as identifying resilience variables and their interactions, leveraging watershed natural properties, processes, and dynamics to facilitate and enable resilience through application of NbS; analytical methods and tools including monitoring, modeling, metrics, and scenario planning, and their applications to watersheds at different spatial and temporal scales, and infusing resilience concepts as core values in watershed management. Given the spatial coincidence of watersheds and agricultural landscapes around the world, some of the concepts in this review are applicable to this work.
5 NbS and climate change goals
The intensity of global efforts toward mitigating the effects of climate change through reduction of emissions of greenhouse gases, and more recently through carbon sequestration, have resulted in an increased focus on NbS for climate mitigation. These global efforts have yielded a rich amount of literature that characterizes NbS in agricultural landscapes with mitigation in a much more specific and quantitative way relative to conservation co-benefits as discussed above. This is in part due to the fact that climate mitigation has a clear global goal (e.g., limiting to 1.5 or 2 degrees the increase in mean global temperature) and that vast resources in research have been invested over the past 3 decades (e.g., IPCC, the World Climate Research Program, and other global, regional and national efforts).
Griscom et al. (2017) provide an in-depth analysis of NbS for climate change mitigation with a particular focus on agricultural landscapes and avoidance/reduction of emissions through carbon sequestration. This investigation reports an overall summary of the potential of NbS toward climate mitigation potential as 23.8 PgCO2e y−1 (95% CI 20.3–37.4) at a 2030 reference year (Figure 2). This estimate is not constrained by costs, but it is constrained by a global land cover scenario with safeguards for meeting increasing human needs for food and fiber. It also assumes no reduction in existing cropland area but does allow for grazing lands in forested ecoregions to be reforested, consistent with agricultural intensification and diet change scenarios. This potential value is also constrained by excluding activities that would either negatively impact biodiversity (e.g., replacing native non-forest ecosystems with forests) or have carbon benefits that are offset by net biophysical warming (e.g., albedo effects from expansion of boreal forests).
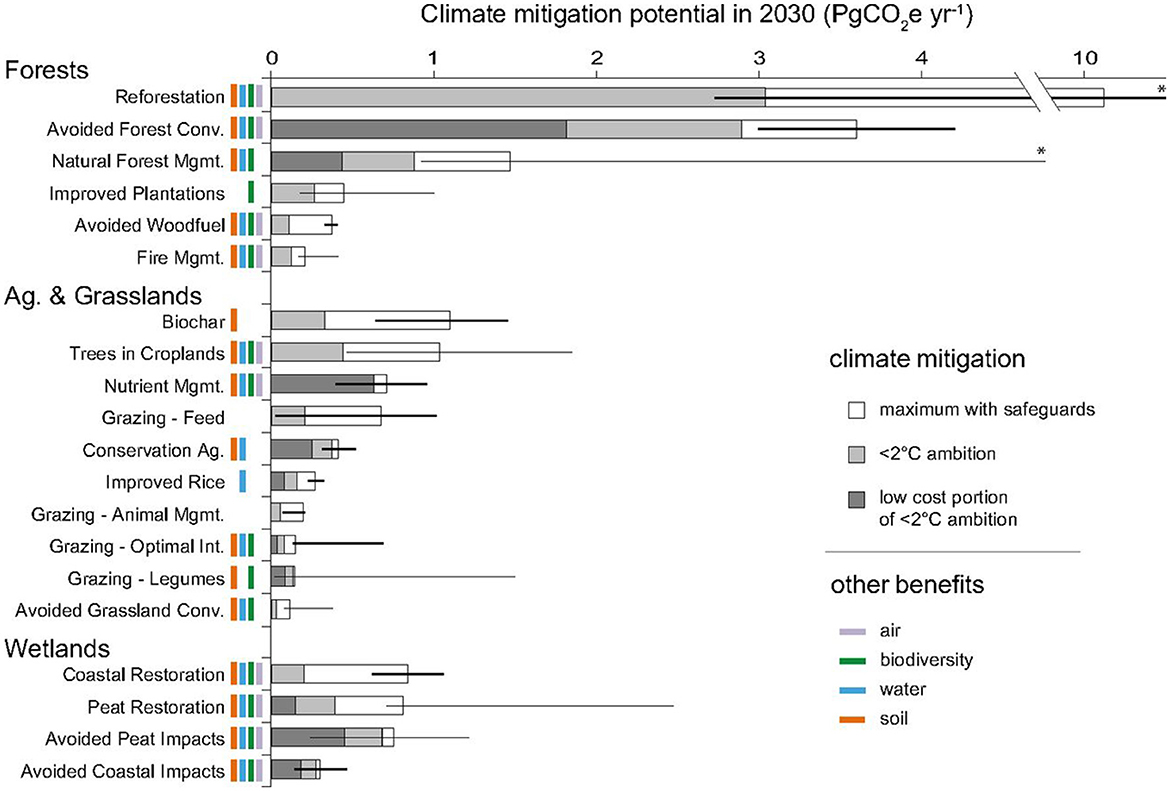
Figure 2. Climate change mitigation potential of NbS in agricultural landscapes; maximum mitigation potential with safeguards has been estimated for the reference year 2030. Dark-colored portions of bars represent cost-effective mitigation levels assuming a global ambition to hold warming to <2°C (<100 USD MgCO2e−1 y−1). Light-colored portions of bars indicate medium (<50 USD MgCO2e−1 y−1) and low-cost (<10 USD MgCO2e−1 y−1) portions of <2°C levels. Wider error bars indicate empirical estimates of 95% confidence intervals, while narrower error bars indicate estimates derived from expert elicitation. Conservation co-benefits linked with each NbS are indicated by colored bars for biodiversity, water (quantity and quality), soil (quality), and air (quality). Asterisks indicate truncated error bars. Source: Griscom et al. (2017).
This analysis includes the tradeoff between costs and benefits of NbS implementation for climate mitigation. This is approached through an analysis of published information on the fraction of maximum mitigation potential that offers a cost-effective contribution to meeting the Paris Climate Agreement goal of limiting warming to below 2°C. This limit of <2°C is referenced in the literature as a cost-effective level of mitigation equivalent to a marginal abatement cost not greater than ~100 USD MgCO −1 as of 2030. This ensures that the marginal (per unit) cost of emissions reductions from NbS does not exceed the marginal benefit of avoiding carbon emissions. The marginal benefit of emissions reductions is represented by estimates of the social cost of carbon, which is the value to society of the avoided marginal damage of CO2 emissions due to climate change and is obtained through welfare-maximizing emissions pricing models (Tol, 2005; Nordhaus, 2014). The social cost of carbon in 2030 is estimated to be 82–260 USD MgCO2e−1 to meet the 1.5–2°C climate target (Dietz and Stern, 2015). This value is consistent with estimates for the avoided cost to society from holding warming to below 2°C (Canadell and Raupach, 2008; Meinshausen et al., 2009).
The proportion of climate mitigation toward a <2°C outcome that could be achieved at low cost was also assessed as part of this analysis. A marginal cost threshold of ~10 USD MgCO2e−1 was used for this purpose, consistent with the current cost of emission reductions efforts underway and current prices on existing carbon markets. The review of published data also reveals that more than one-third of the <2°C cost effective levels for NbS are low cost (<10 USD MgCO2e−1, total of 4.1 PgCO2e y−1; Figure 2). The “low-cost” and “cost-effective” carbon sequestration opportunities compare favorably with cost estimates for emerging technologies, most notably bioenergy with carbon capture and storage (BECCS)—which range from ~40 USD MgCO −1 to over −121,000 USD per MgCO2. Furthermore, large-scale BECCS is largely untested and likely to have significant impacts on water use, biodiversity, and other ecosystem services.
6 Socioeconomic considerations
Although there is a vast body of literature body documenting studies of different types of compensations for land use conversion and PES, few of such studies include NbS. In one study, Geussens et al. (2019) investigated Uganda farmers' willingness to accept eight agricultural practices (some of which intersect with the NBS focus of this review), e.g., minimum tillage, mulching, contouring, trenches, grass strips, agroforestry, and riverbank protection) under nine different compensation levels, or PES contracts. This study had two important lessons for NbS. First, it showed that the biggest difference between willing and reluctant PES-adopters, concerned their perceived benefits of NbS implementation. Their preferences depended not only on the intervention, but also on the compensation level, and whether they received community funds or individual compensation. Second, among the considerations that the project designers had to make were willingness to adopt vs. the reduced effectiveness of practices when they were too scattered; because of this, a minimum number of farmers were required. The willingness to accept was high when the need for a different solution had reached a certain threshold, such as severity of degradation (the Uganda example), or when farmers have run out of other viable options. Ultimately, PES schemes would benefit land uses with high ecosystem values by combining marketable and non-marketable ecosystem services, such as biomass production and groundwater, soil quality, carbon sequestration, and other co-benefits (Kay et al., 2019).
Adoption of NbS in agriculture is found to be dependent on perceived benefits to livelihoods. Several studies suggest that farmers may not adopt NbS despite having witnessed ecosystem benefits, because of increased initial costs, labor inputs, or customs and preferences (Chapman and Darby, 2016; McWilliam and Balzarova, 2017; Cerdà et al., 2018). A combination of economic, attitudinal and farm structural factors are relevant and apply to a range of situations from riparian buffer zones in the EU (Buckley et al., 2012) to coastal zone management in Southeast Asia (Joffre et al., 2015).
Studies such as these suggest that benefits from NbS will be prioritized differently by different groups of people. While there may be diverse societal interests among the immediate beneficiaries, such as land users and landowners, those interests may also need to be negotiated with direct and indirect, and often disconnected, ecosystem services to the wider society. Many of the people living in the most exposed areas have vital knowledge about its ecosystems (Simelton and Dam, 2014; van der Wolf et al., 2016). Despite this, poverty and immediate needs can drive farmers to put pressure on already degraded ecosystems through unsustainable practices (IFAD, 2013). NbS design should be guided by inclusiveness, local needs, knowledge and aspirations as an integral part of the solutions (Richards, 2011). Improvements of agroecosystem functions need to be made clear as livelihoods improvements (Gawith and Hodge, 2019).
Intuitively, NbS interventions need to make an economic argument for adoption, from the perspectives of both farmers and decision makers; however, scarce literature includes economic assessments of NbS practices in agricultural landscapes. The exception is the climate mitigation literature focusing on land-based approaches, which has received increasing attention in the literature. Among the few published studies on economic valuation of NbS in agriculture are cost-effective assessments of management approaches to reduce sediment loads and agriculture runoff (Gikas et al., 2018; Irwin et al., 2018). A study in Tunisia (Mtibaa et al., 2018) found that while contour ridges alone halved the sediment yield, the most cost-effective option was a combination of practices, including buffer strips, conversion to orchard, and grass strip cropping. Similarly, Gikas et al. (2018) showed that two low-cost options with plants in constructed wetlands, performed better when combined with bio-mixtures containing coconut fiber for bio-purification. Other estimates, such as those by Irwin et al. (2018) related the improvement in water quality from reduced agriculture runoff with an associated value for residents and recreation users. Here, ten percent improved water quality resulted in a lifetime cost benefit ratio of 2.9.
This shortcoming in economic assessments can be explained by a few reasons. First, there are difficulties in correctly evaluating ecosystem values. For example, the effects and valuation of agroforestry ecosystem services were clearer at the farm/plot scale, whereas attribution easily gets blurred in mixed land uses at landscape scale. Second, there are difficulties extrapolating results from smaller empirical studies, e.g., the role of pollinator services for global scale food production. To overcome this, Melathopoulos et al. (2015) devised an approach to estimate values of pollinator services from three different assumptions: (i) the degree of dependency of crops on pollinators; (ii) pollinators need different habitats and pollinate different crops (wild vs. domesticated) hence the cost to retain them will vary; (iii) the degree of alignment of the price of the ecosystem service with the risk, e.g., the value depends on the probability of a collapse. Third, underlying economic assumptions of gray vs. green infrastructure depend on how risk, investment costs and value of losses are calculated. For instance, Onuma and Tsuge (2018) tried to determine when green infrastructure is preferable to gray for disaster risk reduction. They did this by developing parameters to compare the two options in view of hazard, population potentially affected, and associated vulnerability. Although their focus was not primarily on agriculture, similar valorization principles can have applications for NbS in agricultural landscapes. For example, gray infrastructure is designed as defense to one particular natural hazard and breaks at a certain magnitude, while mixing gray with green infrastructure as back-up can be more durable. Additionally, costs are often lower for recovering green infrastructure after an event. Lastly, NbS interventions need to consider surrounding land use change, such as increasing land rents on intensive agriculture land, which will likely drive costs for conservation and carbon credit compensations (Phelps et al., 2013). Adding a long-term lens is as critical as the probability of a practice itself to contribute to ecosystem recovery.
7 Discussion
Advancing implementation of NbS in agricultural landscapes toward improved resilience of climate, conservation an socioeconomic objectives needs to emphasize gains in agricultural production and socioeconomic benefits to farmers; this is an area of opportunity for future analytical work on the general topic of NbS. With the exception of a limited number of sources exist in the literature that are largely focused on local case study applications (e.g., Current and Scherr, 1995; Grieg-Gran et al., 2005; Corbera et al., 2007; Turpie et al., 2008; Pascual et al., 2010; Hegde and Bull, 2011; Zheng et al., 2013), most published studies that are included in this review have stopped short of doing the economic analysis of NbS benefits (outside of climate and conservation, which have been done by the climate and conservation science communities rather than the agricultural science one). However, the analysis could be done to make estimates of these gains and benefits at a global scale of NbS implementation. Given the conservation and adaptation benefits documented for NbS, it is likely that economic benefits to farmers would be realized by NbS implementation, and further work would systematically quantify them.
The conjunctive realization of multiple resilience co-benefits through the implementation of NbS in agricultural landscapes is an area of active research and experimentation in the field; a myriad of new approaches continue to be investigated and tested. For instance, recent research reviewed in Backer et al. (2018) has demonstrated that inoculating plants with plant-growth promoting rhizobacteria (PGPR) can be an effective strategy to stimulate crop growth. Furthermore, these strategies can improve crop tolerance for the abiotic stresses (e.g., drought, heat, and salinity) likely to become more frequent as climate change conditions continue to develop. This discovery has resulted in multifunctional PGPR-based formulations for commercial agriculture, to minimize the use of synthetic fertilizers and agrochemicals.
Another example that has been receiving increased attention lately is ecosystem services provided by insects. Examples include not only pollination, but also other services such as dung burial, pest control, and wildlife nutrition. A recent review of the value of ecosystem services provided by insects provides estimations of the value of each service on projections of losses that would accrue if insects were not functioning at their current level (Losey and Vaughan, 2006). This source estimates the annual value of these ecological services provided in the United States to be at least $57 billion, an amount that justifies greater investment in the conservation of these services.
Many of these innovative NbS approaches fall under the umbrella of bioprospecting, i.e., the exploration of biodiversity for new resources of social and commercial value (Barrett and Lybbert, 2000; Beattie et al., 2011). It is carried out by a wide range of established industries in the food production sector such as agriculture as well as a wide range of comparatively new ones such as aquaculture. Much contemporary bioprospecting has multiple goals, including the conservation of biodiversity, the sustainable management of natural resources and economic development. With respect to NbS in agricultural landscapes, the science aspects of bioprospecting continue to evolve in three vital ways. First, the discovery of new ecosystem services provided by biodiversity (such as the ones provided in this review). Second, carrying out field studies to confirm and quantify the co-benefits of these NbS. Third, demonstrating the value of millions of mostly microscopic species to local, regional and global economic activities.
With respect to climate change, the quantification of potential contribution of NbS to meeting global goals is conservative in three ways. First, payments for ecosystem services other than carbon sequestration have not been analyzed in the literature reviewed and could spur cost-effective implementation of these solutions beyond the levels identified in this document. NbS can enhance conservation benefits which have high monetary values. Improved human health from dietary shifts toward plant-based foods reduce healthcare expenses and further offset implementation costs (Springmann et al., 2016). Second, these findings are conservative because this review only includes activities where data available in the literature are sufficiently robust for global extrapolation. For example, no-till agriculture (Conservation Agriculture), improved manure management in concentrated animal feed operations (Nutrient Management), adaptive multi paddock grazing (Grazing), and soil carbon emissions that may occur with the conversion of forests to pasture (Avoided Forest Conversion) are excluded from the NbS reviewed here. Future research may reveal a robust empirical basis for including such activities and fluxes within these pathways. Third, the Paris Agreement and more recent efforts in climate goals focus on limiting warming to “well below 2°C” and pursuing “efforts to limit the temperature increase to 1.5°C”. Additional investment in all mitigation efforts (i.e., beyond ~100 USD/MgCO2e), including NbS, would be warranted to keep warming to well-below 2°C, or to 1.5°C, particularly if a likelier chance of success is desired.
Feedbacks from climate change on terrestrial carbon stocks are uncertain in the scientific literature. Increases in temperature, drought, fire, and pest outbreaks could negatively impact photosynthesis and carbon storage, while CO2 fertilization has positive effects. Unchecked climate change could reverse terrestrial carbon sinks by midcentury and erode the long-term climate benefits of NbS. Thus, climate change puts terrestrial carbon stocks at risk. Cost-effective implementation of NbS, by increasing terrestrial carbon stocks, would slightly increase (by 4%) the stocks at risk by 2050. However, the risk of net emissions from terrestrial carbon stocks is less likely under a <2°C scenario. As such, overall NbS slightly increase the total risk exposure, yet it will be a large component of any successful effort to mitigate climate change and thus help mitigate this risk. Further, most NbS pathways can increase resilience to climate impacts. Rewetting wetlands reduces the risk of peat fires. Reforestation that connects fragmented forests reduces exposure to forest edge disturbances. Fire management increases resilience to catastrophic fire. On the other hand, some of our pathways assume intensification of food and wood yields—and some conventional forms of intensification can reduce resilience to climate change. All of these challenges underscore the urgency of aggressive, simultaneous implementation of mitigation from both nature-based solutions and fossil fuel emissions reductions, as well as the importance of implementing NbS and land use intensification in locally appropriate ways with best practices that maximize resilience.
Overall, considerable scientific work remains to refine and reduce the uncertainty of NbS benefit estimates and resilience implications. Recent work (DeFries et al., 2015; Wood et al., 2015; Reguero et al., 2018; Oldfield et al., 2019; Bossio et al., 2020) has focused on aspects of improved quantification of ecosystem services in agricultural landscapes, particularly in generating evidence of transforming agricultural practices toward multiple co-benefits. Work also remains to refine methods for implementing pathways in socially and culturally responsible ways while enhancing resilience and improving food security for a growing human population. However, delaying implementation of NbS in agricultural landscapes reviewed here would likely increase the costs to meet agricultural production, climate, conservation and other societally beneficial goals, while degrading the capacity of natural systems to continue to provide other ecosystem services.
Author contributions
FM-W designed and conducted this review, organized the literature database, wrote the manuscript, read, and approved the submitted version.
Funding
Funding for this work was provided under project P118989 by The Nature Conservancy.
Acknowledgments
The content of this manuscript has been presented in part in the FAO and The Nature Conservancy report (Miralles-Wilhelm, 2021).
Conflict of interest
FM-W was employed by The Nature Conservancy.
Publisher's note
All claims expressed in this article are solely those of the authors and do not necessarily represent those of their affiliated organizations, or those of the publisher, the editors and the reviewers. Any product that may be evaluated in this article, or claim that may be made by its manufacturer, is not guaranteed or endorsed by the publisher.
References
Abell, R., Vigerstol, K., Higgins, H., Kang, S., Karres, N., Lehner, B., et al. (2019). Freshwater biodiversity conservation through source water protection: quantifying the potential and addressing the challenges. Aquatic Conserv: Mar. Freshw. Ecosyst. 1–17. doi: 10.1002/aqc.3091
Adewopo, J. (2019). “Smallholder maize-based systems. A piece of the puzzle for sustaining food security in Nigeria,” in Multifunctional Land Uses in Africa. Sustainable Food Security Solutions, eds E. Simelton, and M. Ostwald (London: Routledge). Available online at: https://core.ac.uk/download/pdf/224976759 (accessed December 05, 2023).
Aguiar, T. R. Jr, Rasera, K., Parron, L. M., Brito, A. G., and Ferreira, M. T. (2015). Nutrient removal effectiveness by riparian buffer zones in rural temperate watersheds: the impact of no-till crops practices. Agric. Water Manag. 149, 74–80. doi: 10.1016/j.agwat.2014.10.031
Altieri, M. (1992). Agroecological foundations of alternative agriculture in California Agriculture. Ecosyst. Environ. 39, 23–53. doi: 10.1016/0167-8809(92)90203-N
Are, K. S., Oshunsanya, S. O., and Oluwatosin, G. A. (2018). Changes in soil physical health indicators of an eroded land as influenced by integrated use of narrow grass strips and mulch. Soil Till. Res. 184, 269–280. doi: 10.1016/j.still.2018.08.009
Asfaw, B., and Lemenih, M. (2010). Traditional agroforestry systems as a safe haven for woody plant species: a case study from a topo-climatic gradient in South Central Ethiopia. Forests Trees Livelihoods 19, 359–377. doi: 10.1080/14728028.2010.9752678
Backer, R., Rokem, J. S., Ilangumaran, G., Lamont, J., Praslickova, D., Ricci, E., et al. (2018). Plant growth-promoting rhizobacteria: context, mechanisms of action, and roadmap to commercialization of biostimulants for sustainable agriculture. Front. Plant Sci. 9, 1473. doi: 10.3389/fpls.2018.01473
Barrett, C., and Lybbert, T. (2000). Is bioprospecting a viable strategy for conserving tropical ecosystems? Working Paper Series in Environmental and Resource Economics. Ecol. Econ. 34, 293–300 doi: 10.1016/S0921-8009(00)00188-9
Beattie, A., Hay, M., Magnusson, B., deNys, R., Smeather, J., and Vincent, J. (2011). Ecology and bioprospecting. Aust. Ecol. 36, 341–356. doi: 10.1111/j.1442-9993.2010.02170.x
Bossio, D. A., Cook-Patton, S. C., Ellis, P. W., Fargione, J., Sanderman, J., Smith, P., et al. (2020). The role of soil carbon in natural climate solutions. Nat. Sustain. 3, 391–398. doi: 10.1038/s41893-020-0491-z
Brouder, S. M., and Gomez-Macpherson, H. (2014). The impact of conservation agriculture on smallholder agricultural yields: a scoping review of the evidence. Agric. Ecosyst. Environ. 187, 11–32. doi: 10.1016/j.agee.2013.08.010
Bruun, T. B., De Neergaard, A., Lawrence, D., and Ziegler, A. D. (2009). Environmental consequences of the demise in swidden cultivation in Southeast Asia: carbon storage and soil quality. Hum. Ecol. 37, 375–388. doi: 10.1007/s10745-009-9257-y
Buckley, C., Hynes, S., and Mechan, S. (2012). Supply of an ecosystem service—Farmers' willingness to adopt riparian buffer zones in agricultural catchments. Environ. Sci. Policy 24, 101–109. doi: 10.1016/j.envsci.2012.07.022
Burney, J. S., and Lobell, D. (2010). Greenhouse gas mitigation by agricultural intensification. PNAS 107, 12052–12057. doi: 10.1073/pnas.0914216107
Canadell, J. G., and Raupach, M. R. (2008). Managing forests for climate change mitigation. Science 320, 1456–1457. doi: 10.1126/science.1155458
Cerdà, A., Rodrigo-Comino, J., Giménez-Morera, A., Novara, A., Pulido, M., Kapović-Solomun, M., et al. (2018). Policies can help to apply successful strategies to control soil and water losses. The case of chipped pruned branches (CPB) in Mediterranean citrus plantations. Land Use Policy 75, 734–745. doi: 10.1016/j.landusepol.2017.12.052
Chaplin-Kramer, R., Dombeck, E., Gerber, J., Knuth, K. A., Mueller, N. D., Mueller, M., et al. (2014). Global malnutrition overlaps with pollinator-dependent micronutrient production. Proc. R. Soc. B Biol. Sci. 281, 20141799. doi: 10.1098/rspb.2014.1799
Chapman, A., and Darby, S. (2016). Evaluating sustainable adaptation strategies for vulnerable mega-deltas using system dynamics modelling: rice agriculture in the Mekong Delta's An Giang Province, Vietnam. Sci. Total Environ. 559, 326–338. doi: 10.1016/j.scitotenv.2016.02.162
Cohen-Shacham, E., Walters, G., Janzen, C., and Maginnis, S. (2016). Nature-Based Solutions to Address Global Societal Challenges. Gland: IUCN. p. xiii + 97. Available online at: https://www.researchgate.net/publication/307608144_Nature-based_Solutions_to_address_global_societal_challenges (accessed December 05, 2023).
Corbera, E., Kosoy, N., and Martínez Tuna, M. (2007). Equity implications of marketing ecosystem services in protected areas and rural communities: case studies from Meso-America. Glob. Environ. Chang. 17, 365–380. doi: 10.1016/j.gloenvcha.2006.12.005
Current, D., and Scherr, S. J. (1995). Farmer costs and benefits from agroforestry and farm forestry projects in Central America and the Caribbean: implications for policy. Agroforest. Syst. 30, 87–103. doi: 10.1007/BF00708915
Daryanto, S., Fu, B., Wang, L., Jacinthe, P.-A., and Zhao, W. (2018). Quantitative synthesis on the ecosystem services of cover crops. Earth Sci. Rev. 185, 357–373. doi: 10.1016/j.earscirev.2018.06.013
DeClerck, F. A., Fanzo, J., Palm, C., and Remans, R. (2011). Ecological approaches to human nutrition. Food Nutr. Bull. 32, S41–S50. doi: 10.1177/15648265110321S106
DeFries, R., Fanzo, J., Remans, R., Palm, C., Wood, S., and Anderman, T. (2015). Metrics for land-scarce agriculture. Science 349, 238–240. doi: 10.1126/science.aaa5766
Delbaere, B., Mikos, V., and Pulleman, M. (2014). European policy review: functional agrobiodiversity supporting sustainable agriculture. J. Nat. Conserv. 22, 193–194. doi: 10.1016/j.jnc.2014.01.003
Dewi, S., van Noordwijk, M., Ekadinata, A., and Pfund, J.-L. (2013). Protected areas within multifunctional landscapes: squeezing out intermediate land use intensities in the tropics? Land Use Policy 30, 38–56. doi: 10.1016/j.landusepol.2012.02.006
Dietz, S., and Stern, N. (2015). Endogenous growth, convexity of damage and climate risk: how Nordhaus' framework supports deep cuts in carbon emissions. Econ. J. 125, 574–620. doi: 10.1111/ecoj.12188
Eggermont, H., Estelle, B., Azevedo, J. M. N., Beumer, V., Brodin, T., Claudet, J., et al. (2015). Nature-based Solutions: new Influence for Environmental Management and Research in Europe. GAIA 24/4:243–248. doi: 10.14512/gaia.24.4.9
Ellis, A. M., Myers, S. S., and Ricketts, T. H. (2015). Do pollinators contribute to nutritional health? PLoS ONE 10, e114805. doi: 10.1371/journal.pone.0114805
Ewel, J. J. (1999). Natural systems as models for the design of sustainable systems of land use. Agroforest. Syst. 45, 1–21. doi: 10.1023/A:1006219721151
FAO (2005). Realizing the Economic Benefits of Agroforestry: Experiences, Lessons and Challenges, FAO, State of the World's Forests (2005). p. 88–97. Available online at: http://www.fao.org/3/y5574e/y5574e09.pdf (accessed December 05, 2023).
FAO (2011). What is Conservation Agriculture? Training Guide for Extension Agents and Farmers in Eastern Europe and Central Asia. Available online at: http://www.fao.org/3/i7154en/i7154en.pdf (accessed December 05, 2023).
FAO (2013). Climate-Smart Agriculture - Sourcebook. Rome: Food and Agriculture Organization of the United Nations. Available online at: http://www.fao.org/3/i3325e/i3325e.pdf (accessed December 05, 2023).
FAO (2018). The 10 Elements of Agroecology Guiding the Transition to Sustainable Food and Agricultural Systems. Rome: Food and Agriculture Organization of the United Nations, 15. Available online at: http://www.fao.org/3/i9037en/i9037en.pdf (accessed December 05, 2023).
FAO (2019). “The State of the World's Biodiversity for Food and Agriculture,” in FAO Commission on Genetic Resources for Food and Agriculture Assessments, eds J. Bélanger, and D. Pilling (Rome), 572. Available online at: http://www.fao.org/3/CA3129EN/ca3129en.pdf (accessed December 05, 2023).
FOLU (2019). Growing Better: Ten Critical Transitions to Transform Food and Land Use, The Global Consultation Report of the Food and Land Use Coalition September 2019. Food and Land Use Coalition.
Franklin, E. J., and Mortensen, D. A. (2012). A comparison of land-sharing and land-sparing strategies for plant richness conservation in agricultural landscapes. Ecol. Appl. 22, 459–471. doi: 10.1890/11-0206.1
Friedrich, T., Derpsch, R., and Kassam, A. (2012). Overview of the Global Spread of Conservation Agriculture. Field Actions Science Reports Special Issue 6. Available online at: https://journals.openedition.org/factsreports/1941 (accessed December 05, 2023).
Garibaldi, L. A., Pérez-Méndez, N., Garratt, M. P. D., Gemmill-Herren, B., Miguez, F. E., and Dicks, L. V. (2019). Policies for ecological intensification of crop production. Trends Ecol. Evol. 34, 282–286. doi: 10.1016/j.tree.2019.01.003
Gawith, D., and Hodge, I. (2019). Focus rural land policies on ecosystem services, not agriculture. Nat. Ecol. Evol. 3, 1136–1139. doi: 10.1038/s41559-019-0934-y
Geussens, K., Van den Broeck, G., Vanderhaegen, K., Verbist, B., and Maertens, M. (2019). Farmers' perspectives on payments for ecosystem services in Uganda. Land Use Policy 84, 316–327. doi: 10.1016/j.landusepol.2019.03.020
Gikas, G. D., Pérez-Villanueva, M., Tsioras, M., Alexoudis, C., Pérez-Rojas, G., Masís-Mora, M., et al. (2018). Low-cost approaches for the removal of terbuthylazine from agricultural wastewater: Constructed wetlands and biopurification system. Chem. Eng. J. 335, 647–656. doi: 10.1016/j.cej.2017.11.031
Gliessman, S. R. (2006). Agroecology: The Ecology of Sustainable Food Systems. 2nd Edn. CRC Press. Available online at: https://www.researchgate.net/publication/233138094_Agroecology_The_Ecology_of_Food_Systems (accessed December 05, 2023).
Grieg-Gran, M., Porras, I., and Wunder, S. (2005). How can market mechanisms for forest environmental services help the poor?: preliminary lessons from Latin America. World Dev. 33, 1511–1527. doi: 10.1016/j.worlddev.2005.05.002
Griscom, B. W., Adams, J., Ellis, P. W., Houghton, R. A., Lomax, G., Miteva, D. A., et al. (2017). Natural climate solutions. Proc. Natl. Acad. Sci. U. S. A. 114, 11645–11650. doi: 10.1073/pnas.1710465114
Hegde, R., and Bull, G. Q. (2011). Performance of an agro-forestry based Payments-for-environmental-services project in Mozambique: a household level analysis. Ecol. Econ. 71, 122–130. doi: 10.1016/j.ecolecon.2011.08.014
Holt, A. R., Alix, A., Thompson, A., and Maltby, L. (2016). Food production, ecosystem services and biodiversity: we can't have it all everywhere. Sci. Total Environ. 573, 1422–1429. doi: 10.1016/j.scitotenv.2016.07.139
Huang, Z., Oshunsanya, S. O., Li, Y., Yu, H., and Are, K. S. (2019). Vetiver grass hedgerows significantly trap P but little N from sloping land: evidenced from a 10-year field observation. Agric. Ecosyst. Environ. 281, 72–80. doi: 10.1016/j.agee.2019.05.005
IFAD (2013). Smallholders, Food Security, and the Environment. Rome: International Fund for Agricultural Development. Available online at: https://www.ifad.org/documents/38714170/39135645/smallholders_report.pdf/133e8903-0204-4e7d-a780-bca847933f2e (accessed December 05, 2023).
IPCC (2019). Climate Change and Land. IPCC Special Report on Climate Change, Desertification, Land Degradation, Sustainable Land Management, Food Security, and Greenhouse Gas Fluxes in Terrestrial Ecosystems. Available online at: https://www.ipcc.ch/site/assets/uploads/2019/08/4.-SPM_Approved_Microsite_FINAL.pdf (accessed December 05, 2023).
Irwin, N. B., Irwin, E. G., Martin, J. F., and Aracena, P. (2018). Constructed wetlands for water quality improvements: benefit transfer analysis from Ohio. J. Environ. Manag. 206, 1063–1071. doi: 10.1016/j.jenvman.2017.10.050
IUCN-CMP (2006). Unified Classification of Conservation Actions, Version 1.0. Available online at: https://fosonline.org/wp-content/uploads/2010/06/IUCN-CMP_Unified_Actions_Classification_2006_06_01.pdf (accessed December 05, 2023).
Joffre, O. M., Bosma, R. H., Bregt, A. K., van Zwieten, P. A. M., Bush, S. R., and Verreth, J. A. J. (2015). What drives the adoption of integrated shrimp mangrove aquaculture in Vietnam? Ocean Coast. Manag. 114, 53–63. doi: 10.1016/j.ocecoaman.2015.06.015
Johns, T. (2003). Plant biodiversity and malnutrition: simple solutions to complex problems. Afr. J. Food Agric. Nutr. Dev. 3, 45–52. doi: 10.4314/ajfand.v3i1.19134
Jose, S. (2009). Agroforestry for ecosystem services and environmental benefits: an overview. Agroforest. Syst. 76, 1–10. doi: 10.1007/s10457-009-9229-7
Junsongduang, A., Balslev, H., Inta, A., Jampeetong, A., and Wangpakapattanawong, P. (2013). Medicinal plants from Swidden fallows and sacred forest of the Karen and the Lawa in Thailand. J. Ethnobiol. Ethnomed. 9, 44. doi: 10.1186/1746-4269-9-44
Karabulut, A. A., Udias, A., and Vigiak, O. (2019). Assessing the policy scenarios for the Ecosystem Water Food Energy (EWFE) nexus in the Mediterranean region. Ecosyst. Serv. 35, 231–240. doi: 10.1016/j.ecoser.2018.12.013
Kay, S., Graves, A., Palma, J. H. N., Moreno, G., Roces-Díaz, J. V., Aviron, S., et al. (2019). Agroforestry is paying off – Economic evaluation of ecosystem services in European landscapes with and without agroforestry systems. Ecosyst. Serv. 36, 100896. doi: 10.1016/j.ecoser.2019.100896
Kroeger, T., Klemz, C., Boucher, T., Fisher, J., Acosta, E., Cavassani, A. T., et al. (2019). Returns on investment in watershed conservation: application of a best practices analytical framework to the Rio Camboriú Water Producer program, Santa Catarina, Brazil. Sci. Total Environ. 657, 1368–1381. doi: 10.1016/j.scitotenv.2018.12.116
Lenka, N. K., Satapathy, K. K., Lal, R., Singh, R. K., Singh, N. A. K., Agrawal, P. K., et al. (2017). Weed strip management for minimizing soil erosion and enhancing productivity in the sloping lands of north-eastern India. Soil Till. Res. 170, 104–113. doi: 10.1016/j.still.2017.03.012
Liu, H., Yao, L., Lin, C., Wang, X., Xu, W., and Wang, H. (2018). 18-year grass hedge effect on soil water loss and soil productivity on sloping cropland. Soil Till. Res. 177, 12–18. doi: 10.1016/j.still.2017.11.007
Losey, J., and Vaughan, M. (2006). The economic value of ecological services provided by insects. BioScience 56,4. doi: 10.1641/0006-3568(2006)56-311:TEVOES-2.0.CO;2
Matocha, J., Schroth, G., Hills, T., and Hole, D. (2012). Integrating Climate Change Adaptation and Mitigation Through Agroforestry and Ecosystem Conservation. Dordrecht: Springer Science+Business Media. Available online at: https://link.springer.com/chapter/10.1007/978-94-007-4676-3_9 (accessed December 05, 2023).
McIvor, I., Youjun, H., Daoping, L., Eyles, G., and Pu, Z. (2017). Agroforestry: Conservation Trees and Erosion Prevention, Reference Module in Food Science. Elsevier. Available online at: https://www.researchgate.net/publication/323739443_Agroforestry_Conservation_Trees_and_Erosion_Prevention (accessed December 05, 2023).
McWilliam, W., and Balzarova, M. (2017). The role of dairy company policies in support of farm green infrastructure in the absence of government stewardship payments. Land Use Policy 68, 671–680. doi: 10.1016/j.landusepol.2017.08.030
Meinshausen, M., Meinshausen, N., Hare, W., Raper, S. C. B., Frieler, K., Knutti, R., et al. (2009). Greenhouse-gas emission targets for limiting global warming to 2 degrees C. Nature 458, 1158–1162. doi: 10.1038/nature08017
Melathopoulos, A. P., Cutler, G. C., and Tyedmers, P. (2015). Where is the value in valuing pollination ecosystem services to agriculture? Ecol. Econ. 109, 59–70. doi: 10.1016/j.ecolecon.2014.11.007
Meyfroidt, P. (2018). Trade-offs between environment and livelihoods: bridging the global land use and food security discussions. Global Food Sec. 16, 9–16. doi: 10.1016/j.gfs.2017.08.001
Meyfroidt, P., and Lambin, E. F. (2009). Forest transition in Vietnam and displacement of deforestation abroad. Proc. Natl Acad. Sci. U. S. A. 106, 16139–16144. doi: 10.1073/pnas.0904942106
Millennium Ecosystem Assessment (2005). Ecosystems and Human Well-being: Synthesis. Washington, DC. Available online at: https://www.millenniumassessment.org/documents/document.356.aspx.pdf (accessed December 05, 2023).
Miralles-Wilhelm, F. (2021). Nature-Based Solutions in Agriculture—Sustainable Management and Conservation of Land, Water, and Biodiversity. FAO and The Nature Conservancy. doi: 10.4060/cb3140en
Miralles-Wilhelm, F., Matthews, J., Karres, N., Abell, R., Dalton, J., Dalton, S., et al. (2023). Emerging theme and future directions in watershed resilience research. Water Sec. 18. doi: 10.1016/j.wasec.2022.100132
Montagnini, F., and Nair, P. K. R. (2004). Carbon sequestration: an underexploited environmental benefit of agroforestry systems. Agroforest. Syst. 61, 281–295. doi: 10.1007/978-94-017-2424-1_20
Mtibaa, S., Hotta, N., and Irie, M. (2018). Analysis of the efficacy and cost-effectiveness of best management practices for controlling sediment yield: a case study of the Joumine watershed, Tunisia. Sci. Total Environ. 616–617, 1–16. doi: 10.1016/j.scitotenv.2017.10.290
Muller, L., Eulenstein, F., Dronin, N. M., Mirschel, W., McKensie, B. M., Antrop, M., et al. (2021). “Agricultural landscapes: history, status and challenges,” in Exploring and Optimizing Agricultural Landscapes. Innovations in Landscape Research, eds L. Mueller, V. G. Sychev, N. M. Dronin, and F. Eulenstein (Cham: Springer). doi: 10.1007/978-3-030-67448-9_9
Nordhaus, W. (2014). Estimates of the social cost of carbon: concepts and results from the DICE- 2013R model and alternative approaches. J. Assoc. Environ. Resour. Econ. 1, 273–312. doi: 10.1086/676035
Novara, A., Minacapilli, M., Santoro, A., Rodrigo-Comino, J., Carrubba, A., Sarno, M., et al. (2019). Real cover crops contribution to soil organic carbon sequestration in sloping vineyard. Sci. Total Environ. 652, 300–306. doi: 10.1016/j.scitotenv.2018.10.247
Oldfield, E., Bradford, M., and Wood, S. (2019). Global meta-analysis of the relationship between soil organic matter and crop yields. Soil 5, 15–32. doi: 10.5194/soil-5-15-2019
Onuma, A., and Tsuge, T. (2018). Comparing green infrastructure as ecosystem-based disaster risk reduction with gray infrastructure in terms of costs and benefits under uncertainty: a theoretical approach. Int. J. Disast. Risk Reduct. 32, 22–28. doi: 10.1016/j.ijdrr.2018.01.025
Palm, C., Blanco-Canqui, H., DeClerck, F., Gatere, L., and Grace, P. (2014). Conservation agriculture and ecosystem services: an overview. Agric. Ecosyst. Environ. 187, 87–105. doi: 10.1016/j.agee.2013.10.010
Pascual, U., Muradian, R., Rodriquez, L. C., and Duraiappah, A. (2010). Exploring the links between equity and efficiency in payments for environmental services: a conceptual approach. Ecol. Econ. 69, 1237–1244. doi: 10.1016/j.ecolecon.2009.11.004
Phelps, J., Carrasco, L., Webb, E., Koh, L. P., and Pascual, U. (2013). Agricultural intensification escalates future conservation costs. Proc. Natl Acad. Sci. U. S. A. 110, 7601–7606. doi: 10.1073/pnas.1220070110
Reguero, B. G., Beck, M. W., Bresch, D. N., Calil, J., and Meliane, I. (2018). Comparing the cost effectiveness of nature-based and coastal adaptation: A case study from the Gulf Coast of the United States. PLoS ONE 13, e0192132. doi: 10.1371/journal.pone.0192132
Richards, M. (2011). Social and Biodiversity Impact Assessment (SBIA) Manual for REDD+ Projects: Part 2 – Social Impact Assessment Toolbox. Climate, Community & Biodiversity Alliance and Forest Trends with Rainforest Alliance and Fauna & Flora International. Washington, DC. Available online at: https://www.forest-trends.org/publications/social-and-biodiversity-impact-assessment-sbia-manual-for-redd-projects-2/ (accessed December 05, 2023).
Rockström, J., Williams, J., Daily, G., Noble, A., Matthews, N., Gordon, L., et al. (2017). Sustainable intensification of agriculture for human prosperity and global sustainability. Ambio 46, 4. doi: 10.1007/s13280-016-0793-6
Rosas-Ramos, N., Baños-Picón, L., Tobajas, E., de Paz, V., Tormos, J., Asís, J., et al. (2018). Value of ecological infrastructure diversity in the maintenance of spider assemblages: a case study of Mediterranean vineyard agroecosystems. Agric. Ecosyst. Environ. 265, 244–253, doi: 10.1016/j.agee.2018.06.026
Rosenstock, T., Nowak, A., and Girvetz, E. (2019). The Climate-Smart Agriculture Papers. Investigating the Business of a Productive, Resilient and Low Emission Future. Springer International Publishing. Aailable online at: https://link.springer.com/chapter/10.1007/978-3-319-92798-5_1 (accessed December 05, 2023).
Shah, S. I. A., Zhou, J., and Shah, A. A. (2019). Ecosystem-based Adaptation (EbA) practices in smallholder agriculture; emerging evidence from rural Pakistan. J. Clean. Prod. 218, 673–684. doi: 10.1016/j.jclepro.2019.02.028
Shukla, M. K., Lal, R., and Ebinger, M. (2006). Determining soil quality indicators by factor analysis. Soil Tillage Res. 87, 194–204. doi: 10.1016/j.still.2005.03.011
Simelton, E., and Dam, V. B. (2014). Farmers in NE Viet Nam rank values of ecosystems from seven land uses. Ecosyst. Serv. 9, 133–138. doi: 10.1016/j.ecoser.2014.04.008
Sonneveld, B. G., Merbis, J. S., Alfarra, M. D. A., Ünver, O., and Arnal, M. A. (2018). Nature-Based Solutions for Agricultural Water Management and Food Security. FAO Land and Water Discussion Paper no. 12. Rome: FAO, 66. Available online at: http://www.fao.org/3/CA2525EN/ca2525en.pdf (accessed December 05, 2023).
Springmann, M., Godfray, H. C. J., Rayner, M., and Scarborough, P. (2016). Analysis and valuation of the health and climate change cobenefits of dietary change. Proc. Natl. Acad. Sci. U. S. A. 113, 4146–4151. doi: 10.1073/pnas.1523119113
Steyn, N. P., Nel, J. H., Nantel, G., Kennedy, G., and Labadarios, D. (2006). Food variety and dietary diversity scores in children: are they good indicators of dietary adequacy? Public Health Nutr. 9, 644–650. doi: 10.1079/PHN2005912
TEEB (2018a). Scientific and Economics Foundations Report. Available online at: http://teebweb.org/agrifood/scientific-and-economic-foundations-report/~ttp://teebweb.org/agrifood/scientific-and-economic-foundations-report/ (accessed December 05, 2023).
TEEB (2018b). Measuring What Matters in Agriculture and Food Systems: A Synthesis of the Results and Recommendations of TEEB for Agriculture and Food's Scientific and Economic Foundations Report. Available online at: https://teebweb.org/our-work/agrifood/reports/measuring-what-matters-synthesis/ (accessed December 05, 2023).
Thaman, R. R. (2014). Agrodeforestation and the loss of agrobiodiversity in the Pacific Islands: a call for conservation. Pac. Conserv. Biol. 20. doi: 10.1071/PC140180
Tol, R. (2005). The marginal damage costs of carbon dioxide emissions: an assessment of the uncertainties. Energy Policy 33, 2064–2074. doi: 10.1016/j.enpol.2004.04.002
Turpie, J. K., Marais, C., and Blignaut, J. N. (2008). The working for water programme: Evolution of a payments for ecosystem services mechanism that addresses both poverty and ecosystem service delivery in South Africa. Ecol. Econ. 65, 788–798. doi: 10.1016/j.ecolecon.2007.12.024
United Nations (1992). Convention on Biological Diversity. Available online at: http://www.cbd.int/doc/legal/cbd-en.pdf (accessed December 05, 2023).
van der Wolf, J., Jassogne, L., Gilgram, G., and Vaast, P. (2016). Turning local knowledge on agroforestry into an online decision-support tool for tree selection in smallholders' farms. Exp. Agric. 55, 1–17. doi: 10.1017/S001447971600017X
van Noordwijk, M., Hairiah, K., Tata, H., and Lasco, L. (2019). “How can agroforestry be part of disaster risk management?,” in Sustainable Development Through Trees on Farms: Agroforestry in its Fifth Decade (Bogor: World Agroforestry (ICRAF) Southeast Asia Regional Program), 251–267. Available online at: http://apps.worldagroforestry.org/downloads/Publications/PDFS/BC19021.pdf (accessed December 05, 2023).
Vogl, A., Bryant, B., Hunink, J., Wolny, S., Apse, C., and Droogers, P. (2017). Valuing investments in sustainable land management in the Upper Tana River basin, Kenya J. Environ. Manag. 195, 78–91. doi: 10.1016/j.jenvman.2016.10.013
Wolz, K. J., and DeLucia, E. H. (2018). Alley cropping: Global patterns of species composition and function. Agric. Ecosyst. Environ. 252, 61–68. doi: 10.1016/j.agee.2017.10.005
Wood, S., Karp, D., DeClerck, F., Kremen, C., Naeem, S., and Palm, C. (2015). Functional traits in agriculture: agrobiodiversity and ecosystem services. Trends Ecol. Evol. 30. doi: 10.1016/j.tree.2015.06.013
World Agroforestry Centre (2008). Annual Report 2007-2008: Agroforestry for Food Security and Healthy Ecosystems. Nairobi: ICRAF. Available online at: http://www.worldagroforestry.org/downloads/Publications/PDFS/RP15815.pdf (accessed December 05, 2023).
WWAP (2018). The United Nations World Water Development Report 2018: Nature-Based Solutions for Water. Paris, UNESCO; United Nations World Water Assessment Programme/UN-Water.
Zheng, H., Robinson, B. E., Liang, Y.-C., Polasky, S., Ma, D.-C., Wang, F.-C., et al. (2013). Benefits, costs, and livelihood implications of a regional payment for ecosystem service program. Proc. Natl. Acad. Sci. U. S. A. 110, 16681–16686. doi: 10.1073/pnas.1312324110
Zuazo, V. H. D., Pleguezuelo, C. R. R., Peinado, F. J. M., de Graaff, J., Martínez, J. R. F., and Flanagan, D. C. (2011). Environmental impact of introducing plant covers in the taluses of terraces: implications for mitigating agricultural soil erosion and runoff. Catena 84, 79–88. doi: 10.1016/j.catena.2010.10.004
Keywords: nature-based solutions, agriculture, resilience, conservation, climate change, socioeconomics
Citation: Miralles-Wilhelm F (2023) Nature-based solutions in agricultural landscapes for reducing tradeoffs between food production, climate change, and conservation objectives. Front. Water 5:1247322. doi: 10.3389/frwa.2023.1247322
Received: 25 June 2023; Accepted: 29 November 2023;
Published: 19 December 2023.
Edited by:
John H. Matthews, Alliance for Global Water Adaptation (AGWA), United StatesReviewed by:
Prakash Kumar Jha, Mississippi State University, United StatesTemesgen Gashaw, Bahir Dar University, Ethiopia
Copyright © 2023 Miralles-Wilhelm. This is an open-access article distributed under the terms of the Creative Commons Attribution License (CC BY). The use, distribution or reproduction in other forums is permitted, provided the original author(s) and the copyright owner(s) are credited and that the original publication in this journal is cited, in accordance with accepted academic practice. No use, distribution or reproduction is permitted which does not comply with these terms.
*Correspondence: Fernando Miralles-Wilhelm, fmiralle@gmu.edu