- 1Laboratory of Applied Hydrology and Environment, Faculty of Sciences, University of Lomé, Lomé, Togo
- 2Laboratory of Animals Biology and Ecology, University of Joseph KI-ZERBO, Ouagadougou, Burkina Faso
- 3Faculty of Biosciences, University for Development Studies, Tamale, Ghana
Macroinvertebrate metrics are helpful tools for the assessment of water quality and overall aquatic ecosystem health. However, their degree of sensitivity and the most reliable metrics for the bioassessment program development are very poorly studied in Togo. This study aimed to test the sensitivity of metrics calculated at the family and genus levels. A total of 21 water quality parameters and macroinvertebrates' data were collected during three periods at 20 sampling sites within the Zio River. The canonical correspondence analysis (CCA), factor analysis (FA), and Spearman's correlation analysis were conducted on water quality parameters and macroinvertebrates' data. The results reveal that macroinvertebrate structure and composition were affected by water quality parameters related to human disturbances. In this study, three groups of macroinvertebrate communities were identified including sensitive taxa such as Ephemeroptera, Plecoptera, Trichoptera, and Odonata (EPTO) taxa; the resistant or resilient taxa such as Oligochaeta, Hirudinea, Diptera, and Pulmonates (OHDP) taxa; and tolerant taxa such as Prosobranchia, Bivalvia, Lepidoptera, Heteroptera, and Coleoptera (PBLHC). All the 13 macroinvertebrate-based metrics were found to be sensitive in the detection of water quality and human disturbance gradients. However, metrics related to EPTO and the tolerance measure [multimetric index of the Zio River basin (MMIZB), Average Score per Taxon (ASPT), and Biological Monitoring Working Party (BMWP)] are the most robust in discrimination of pressure gradients. This study reveals that macroinvertebrates are sensitive and can be used for the bioassessment program development at the order, family, or genera taxonomic level.
Introduction
Rivers are important ecosystems with high ecological value (Nguyen et al., 2018), and the health of these ecosystems is important for the human societies that depend on them (Dickens et al., 2018). Rivers are an important source of renewable water supply for human beings and freshwater ecosystems (Vorosmarty et al., 2010) and provide many goods and services such as domestic uses, navigation, recreational activities, and nursing grounds and food for many organisms (Berger et al., 2016). Until the late 1960s, the overriding interest in water has been on the available amount for consumption. Except when undesirable water quality conditions persist, the available water was considered acceptable for consumption. Only during the last three decades of the 20th century has water quality been deteriorated to the point where it is considered as important as managing for the availability of water (Abbasi and Abbasi, 2012).
Globally, human impact is changing the availability of freshwater (Rodell et al., 2018). Among freshwater ecosystems, streams and rivers are the most influenced or threatened by a range of anthropogenic stresses (Allan, 2004; Best and Darby, 2020). In the same line, Dudgeon et al. (2006) grouped the major threats to freshwater species under five interacting categories: overexploitation; water pollution; flow modification; destruction or degradation of habitat; and invasion by exotic species. The degree of these stressors led to a geographical variation in the threat to freshwater species with almost one in three freshwater species threatened with extinction worldwide (Collen et al., 2013), while estimates suggest that at least 10,000–20,000 freshwater species are extinct or at risk of extinction worldwide (Balian et al., 2008; Vorosmarty et al., 2010). For example, 1,116 freshwater migratory fish species are threatened and with 102 additional extinctions (Hogan, 2011). In tropical regions, particularly in Sub-Saharan countries, rivers are under pressure due to human activities that deteriorate water quality, limiting water availability for drinking and other uses (Traoré et al., 2016; Kaboré et al., 2018; Tampo, 2018; Chetty and Pillay, 2019; Agboola et al., 2020a). Furthermore, the river pollution problem, related to human disturbances through anthropogenic activities and urbanization (Azrina et al., 2006; Faridah et al., 2012; Edegbene et al., 2021), has many adverse impacts on river ecosystems that have required managers to increase water assessment efforts (Clifford and Tariro, 2005). Many African countries have concerns about the ecological status of their rivers and are increasing their investments on the restoration of degraded rivers (Smith et al., 2009; Fayiga et al., 2018; Wantzen et al., 2019).
In many studies, biological methods are valuable for determining natural and anthropogenic influences on water resources and habitats because biota responds to stressors from multiple spatial or temporal scales (Weigel and Robertson, 2007; Resende et al., 2010; He et al., 2020; Kurthen et al., 2020). In addition, the use of aquatic organisms in ecological studies has proven more effective than using environmental variables alone, because the aquatic community integrates structural and functional characteristics and reflects the health of the studied streams (Bonada et al., 2006; He et al., 2020). Accordingly, there is an increasing interest in the application of ecological thresholds for natural resource management (King and Baker, 2010; Forio et al., 2018). Several metrics and biotic indices are developed and used across the world (Barbour et al., 1996; Hering et al., 2006; Agboola et al., 2019; He et al., 2020; Ko et al., 2020. Gonçalves and Menezes (2011) argued that the use of biotic indices as a tool for river quality assessment was more useful in evaluating river health than the conventional national water quality assessment standard practices in many countries. Metcalfe (1989) and Alba-Tercedor (1996) on the other hand pointed at the disadvantage of the physicochemical assessment, which measures the water quality only at the time of sampling.
Among the river components, aquatic macroinvertebrates are the most sensitive to anthropogenic pressures (Nessimian et al., 2008; Mereta et al., 2012; Agboola et al., 2020a,b; Ko et al., 2020). Macroinvertebrate responses to the change in aquatic ecosystem condition is universally recognized, and their responses are used in indices to monitor freshwater ecosystem for integrity, aiding in decision making in management (Richter et al., 2003; Kaboré et al., 2016; Agboola et al., 2019; Tampo et al., 2020; Edegbene et al., 2021). Macroinvertebrates are commonly used in assemblages for biomonitoring because they integrate various desirable characteristics, such as ubiquity, different levels of tolerance to perturbations, and sampling cost-effectiveness (Mary, 1999; Dickens and Graham, 2002; Hering et al., 2006; Resh, 2008; Kenney et al., 2009; Li et al., 2012; Kaboré, 2016; Agboola et al., 2019; Ko et al., 2020).
The freshwater macroinvertebrate taxa vary in response to organic pollution; and thus, their diversity and composition have been used to make inferences about pollution loads (Kenney et al., 2009; Wan Abdul Ghani et al., 2018; Tampo et al., 2020). Generally, in natural pristine rivers, high abundances and richness of species could be found (Barbour et al., 1996, 1999). However, high impact due to human activities caused many changes of the assemblages and biodiversity of the river fauna (Wright et al., 1993; Pinel-Alloul et al., 1996). Despite the development and application of a variety of biotic indices, scores, and metrics based on macroinvertebrates for water quality and ecosystem health assessment in America and Europe, the literature provides little information on biological assessment and monitoring tools of freshwater ecosystems in Sub-Saharan Africa especially in West Africa (Adams, 1993; Dallas, 1997; Kaboré et al., 2016). In Togo, there is paucity of information on bioassessment of riverine ecosystems health, except for some works on hydrochemistry, macroinvertebrates as indicators, and multimetric index of the Zio River basin (MMIZB) carried out by Tampo et al. (2015, 2020) and Tampo (2018). The measure of macroinvertebrate sensitivity at different taxonomic scales is however very important for bioassessment (Bailey et al., 2001; Schmidt-Kloiber and Nijboer, 2004; Marshall et al., 2006; Metzeling et al., 2006; Jones, 2008; Costas et al., 2018) and essential for developing a biomonitoring program in Togo. Therefore, the aim of this study was to test the effectiveness and the robustness of a set of macroinvertebrate-based metrics in the Zio River.
Materials and Methods
Study Area and Sampling Sites
This study was undertaken in the main stem of the Zio River. It is located in a tropical climate between the latitude 6°5′ and 7°18′N (Figure 1) and includes three of the five ecoregions of Togo. The agriculture and other human activities described previously (Tampo et al., 2015, 2020; Tampo, 2018) are more intensive in the lower reaches of the Zio River. The river crosses some villages and the outskirts of the city of Lomé; and its water is the major source for domestic and agricultural purposes in rural and semi-urban areas. Moreover, the main activity in the basin is agriculture (maize, cassava, bean, yams, rice, etc.), fishing, and few industrial activities such as gravel washing and sand extraction (Bawa et al., 2018). According to Tampo (2018), the river can be subdivided into three sections, such as the upper section, the middle section, and the lower section, which is influenced by seawater during the low flow. Twenty sampling sites were selected across the study area, which included a range of impaired and unimpaired ecological states within the Zio River following anthropogenic disturbances (Tampo et al., 2020).
Sampling and Water Parameter Analyses
Twenty sites were sampled three times between 2013 and 2014 during different seasons (the rainy season, the dry season, and a transition period between the rainy season and dry season). Therefore, 60 samples were collected and 21 water quality parameters measured. At each site, the water quality parameters such as temperature, electrical conductivity (EC), dissolved oxygen (DO), and pH were measured in situ using Wissenschaftliche Technische Werkstätten (WTW) multipurpose water quality probe. After the in situ measurement, 1.5 L of water was taken in a plastic bottle and stored in a cool environment for analysis of chemical parameters in the laboratory within 48 h after collection. For microbiological analysis, samples were collected in borosilicate glassware of 500 ml. The chemical oxygen demand (COD) was determined by the potassium permanganate method, and biological oxygen demand (BOD) was determined by the 5 days' test according to respirometry method. Major and minor ions were determined by titration method (Ca2+, Mg2+, , and Cl−) and by UV-spectrophotometric method (, , , total iron, Mn2+, and ), while K+ and Na+ were determined by flame emission spectrophotometer. Total suspended solid (TSS) was determined by gravimetric method (dried at 105°C). All these parameters were measured in the Laboratory of Applied Hydrology and Environment (LAHE) of the Université de Lomé (Togo) with an accuracy ranking from 1 to 2% according to the standard methods as prescribed by AFNOR (1997) and Rodier et al. (2009).
Macroinvertebrate Collection and Identification
Benthic macroinvertebrates were sampled using a dip net (circular opening, 33 cm of diameter; mesh size, 320 μm) in lentic habitats and a Surber Sampler (rectangular opening, 20 cm × 25 cm; mesh size, 320 μm) for lotic habitats. At each site, substrate samples were taken and combined to one composite sample following protocol described by AFNOR (1997) and Rodier et al. (2009). All animals were identified at the family level and at the genus level for mollusk gastropod, Annelida, and insects using taxonomic manuals and keys (Durand and Levêque, 1981; Merritt and Cummins, 1996; Tachet et al., 2010).
Metric Selection
In this study, 13 metrics classified in three categories were used to assess the ecological status of the Zio River and to test their sensitivities (Table 1). These metrics were calculated using macroinvertebrate features (number of taxa, diversity indices, abundance, and tolerance score). The selection of these metrics was based on their simplicity and reliability for assessing the water quality of the river as well as their suitability to detect anthropogenic disturbances (Raburu et al., 2009; Jun et al., 2012; Nguyen et al., 2014; Kaboré et al., 2016; Agboola et al., 2019; Tampo et al., 2020). Table 1 indicates the metrics used and their definition.
Statistical Analysis
The canonical correspondence analysis (CCA) was applied in order to establish the relationship between water quality parameters and macroinvertebrate abundance and to identify water quality parameters affecting macroinvertebrate community. The analysis was performed under PAST software (version 3.0) based on dataset of water quality parameters and a dataset of abundance of macroinvertebrate community at phylum–class, subclass–order, and family and genus levels. In addition, we assessed the potential of macroinvertebrate taxa and metrics detected in this study to serve as bioindicators for the river's environmental condition investigated. Therefore, factor analysis (FA) and Spearman's correlation analysis between macroinvertebrate data and water quality variables were used, and with expert's consensus following Kaboré et al. (2016) and Tampo et al. (2015). The FA was performed using principal components as factor extraction method without any rotation. The factor loadings were considered for the explanation of correlations among variables and the detection of reliable metrics as indicators of water quality. Spearman's correlation and FA were computed using STATISTICA (version 7.0) for Windows.
Results
Status of Water Quality in the Zio River
The descriptive analysis gives an overview on the variation of water quality parameters in the Zio River during the three sampling periods (Table 2). EC expresses the degree of water mineralization and salinity. It varies in this study from 10,506 to 38.10 μS/cm, with high standard deviations (±1,755.07 μS/cm) indicating the large variability of EC from upstream to downstream of the Zio River. The pH ranged from 6.4 to 7.8, with the mean value varying from neutral toward a state of alkaline. The variation trends of most of major ions such as Ca2+, Mg2+, Na+, K+, , Cl−, and are similar to EC and in the range of natural water quality. The concentration of minor ions such as , , Fe, , and Mn2+ was below the detection limits in some sites and very low in other sites. The COD and the BOD are indicators of organic pollution in the water. COD values ranged from 4 to 30.7 mg/L with a mean of 8.68 mg/L, while BOD ranged from 2 to 20 mg/L with a mean value of 4.88 mg/L. The ratio COD/BOD is also an indicator of river pollution in terms of domestic or industrial effluents and biodegradability. This ratio ranges from 1.16 to 4.30 with a mean value of 1.92. The DO is one of the common parameters used to assess water quality and aquatic ecosystems' health. In the present study, the DO value varied from 0.6 to 12.8 mg/L. Many values recorded are high or close to 7 mg/L, showing that water in the Zio River can be qualified as good-to-excellent DO quality according to surface water standards except for a few polluted sites at the lower section of the river. The TSSs are defined as solids in the water including organic and inorganic materials that can be trapped by a filter. TSS ranged from 12 to 320 mg/L with a mean value and 75th percentile value of 108.6 and 158 mg/L, respectively.
Among microbiological parameters, total coliforms (TC) and fecal coliforms (FC) are often used as indicators of bacterial contamination in the water. Their presence is an indication of fecal contamination in water. In the present study, TC and FC range from 8 to 710 Cfu/100 ml and from 0 to 177 Cfu/100 ml, respectively. These values can express a risk of a bacterial contamination of water in the Zio River.
Sensitivity of Macroinvertebrate Community to the Water Quality
Sensitivity at Phylum–Class Level
The CCA indicates the environmental parameters that affect macroinvertebrate community at phylum–class level (Figure 2A). Table 3 shows the key indicator taxa and the total taxa identified at different taxonomic levels. The Supplementary Data in Appendix A indicate Spearman's correlation between water quality parameters and phylum–class abundance. From Figure 2A, phylum Annelida is affected by BOD, COD, and . Appendix A indicates that Annelida abundance is significantly and positively correlated with these parameters (r > 0.60; p < 0.05) and negatively correlated with DO (r < −70; p < 0.05). The significant correlation (r > 0.60; p < 0.05) of these parameters with TC and FC suggests that they can affect microbiological quality in the Zio River water. From Figure 2A, the class Crustacea is affected by EC and Cl and Na contents, which are core salinity parameters. Moreover, their abundance is significantly correlated with EC, Na, and Cl (r > 0.60; p < 0.05) and can suggest the degree of crustacean tolerance to the water salinity. From the results of CCA (Figure 2A) and the relationship (Spearman's correlation between water quality parameters and taxon abundance), this study identified at phylum–class level five taxa as indicators including three taxa as tolerant, one taxon as resistant, and one taxon as an indicator of water salinity (Table 3).
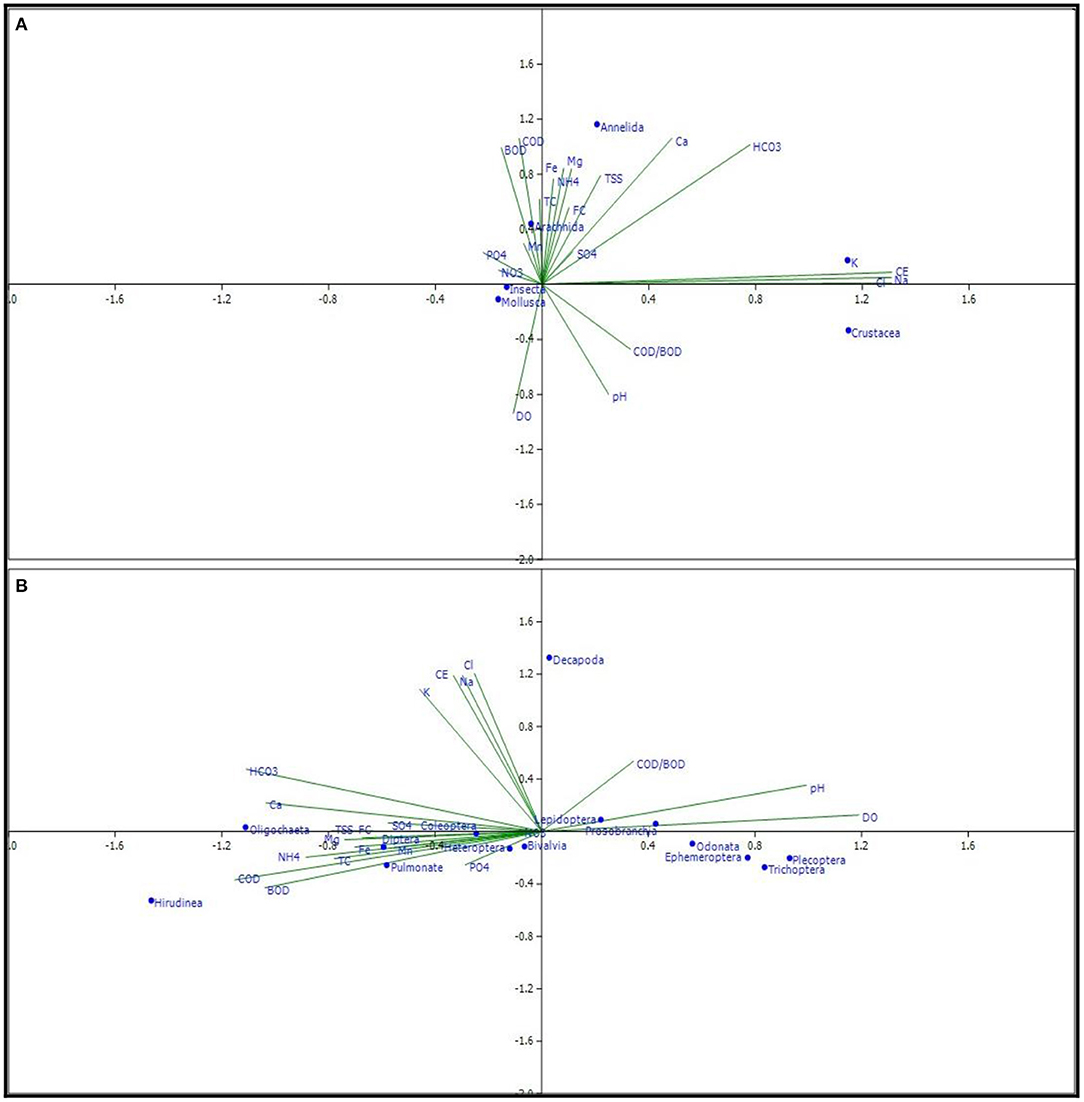
Figure 2. A canonical correspondence analysis (CCA) biplot showing the association between water quality parameters and abundance of macroinvertebrate phylum–class (A) and subclass–order (B) resolution.
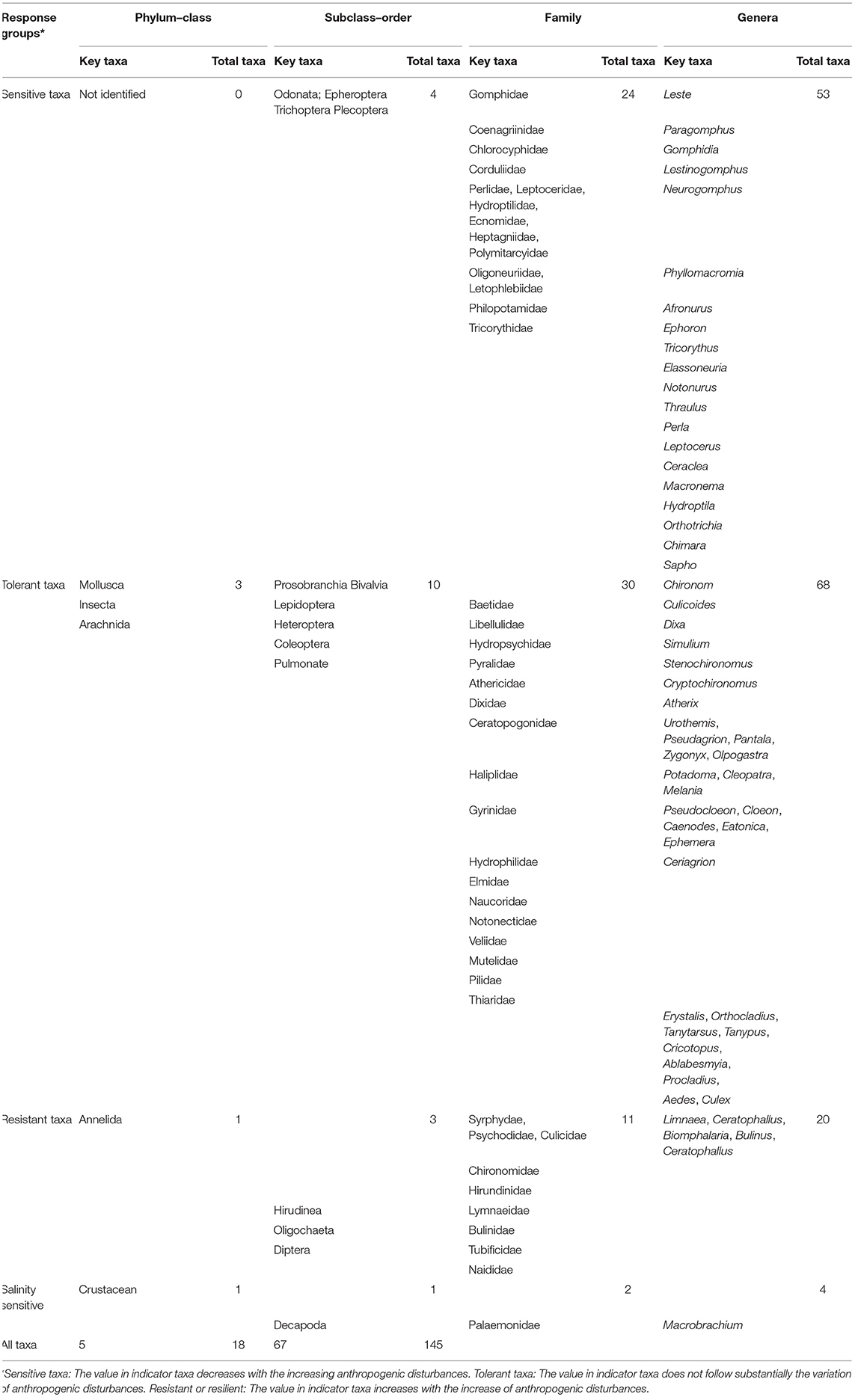
Table 3. Key taxa as indicated by their respective response groups for their specific taxonomic levels.
Response at Subclass–Order Level
The CCA indicates the environmental parameters that affect macroinvertebrate community at subclass–order level (Figure 2B). The Supplementary Data in Appendix B indicate Spearman's correlation between water quality parameters and subclass–order composition. The orders such as Ephemeroptera, Plecoptera, Trichoptera, and Odonata (EPTO) are significantly influenced by DO with a significant and positive correlation (r > 0.60; p < 0.05). Their abundances are negatively influenced by BOD, COD, TSS, and NH4 (r < −0.60; p < 0.05). The subclasses such as Hirudinea (Leeches) and Oligochaeta are positively affected by BOD, TSS, and NH4 (r > 0.60; p < 0.05) but negatively correlated with DO (r < −0.65; p < 0.05). The Pulmonates mollusks and Diptera are significantly correlated with FC and TC (r > 0.60; p < 0.05). From Figure 2B, EPTO are in an opposite trend in comparison with Oligochaeta, Hirudinea, Diptera, and Pulmonates (OHDP). Decapoda is significantly and positively correlated with EC, Na, K, and Cl and then positively correlated with the salinity. The other subclass–order such as Prosobranchia, Bivalvia, Lepidoptera, Heteroptera, and Coleoptera (PBLHC) do not reveal a significant association with water quality parameters. From Figure 2B and Spearman's correlation, at subclass–order resolution, four groups of macroinvertebrates can be distinguished as indicator taxa with different sensitivity to water quality parameters. The first group is composed of EPTO, which are sensitive to the decrease of DO, and to the increase of BOD, COD, TSS, and NH4. The second group is composed of OHDP, which has the opposite response in comparison with the first group. The third group is represented by Decapoda, which seems to be influenced by the salinity parameters (EC, Na, Cl, and K). The fourth group is composed of PBLHC, which does not show significant correlation with water quality parameters. The key indicator taxa and total number of taxa in each group at subclass–order resolution are mentioned in Table 3.
Response at Family Level
The CCA indicates the environmental parameters that affect macroinvertebrate community at the family level (Figure 3A). The Supplementary Data in Appendix C indicate Spearman's correlation between water quality parameters and families composition. In Figure 3A, except some few families (Baetidae, Caenidae, Libellulidae, and Hydropsychidae), most of the families are from EPTO orders, and other families such as Atyidae from Decapoda, and Elmidae and Gyrinidae from Coleoptera are also strongly affected by water quality parameters. The abundance of these families increases with the increasing DO but decreases with the increasing BOD, COD, NH4, TC, and FC concentrations. Spearman's correlation confirmed this trend by a significant correlation between the abundance of these families and DO (r > 0.50; p < 0.05), BOD, COD, NH4, FC, and TC (r < −0.50; p < 0.05). Therefore, the families such as Atyidae, Elmidae, and Gyrinidae and those from EPTO can be classified as sensitive taxa (Table 3). In contrast, the abundance of Syrphidae, Psychodidae, Culicidae, and Chironomidae from Diptera order; Hirudinidae from Hirudinea; Lymnaeidae and Bulinidae from Pulmonates; and Tubificidae and Naididae from Oligochaeta increases when BOD, COD, NH4, TC, and FC concentrations increase and decreases when DO concentration increases. The abundances of these taxa are significantly correlated with DO (r < −0.50; p < 0.05) and with BOD, COD, NH4, TC, and FC (r > 0.50; p < 0.05). These relationships suggest that the families such as Syrphidae, Psychodidae, Culicidae, Chironomidae, Hirudinidae, Lymnaeidae, Bulinidae, Tubificidae, and Naididae can be classified as resistant or resilient taxa (Table 3). According to Figure 3A, the abundance of Palaemonidae is influenced positively by EC, Na, and Cl and is significantly and positively correlated with these parameters (r > 0.55; p < 0.05). This family from Decapoda can be considered as an indicator taxon of water salinity. The remaining families' taxa mainly from PBLHC; some families' taxa from Diptera and Decapoda; and also the few families from EPTO did not reveal a significant relationship with water quality variables (r < |0.50|; p < 0.05). These taxa were classified as tolerant or indifferent taxa as indicated in Table 3.
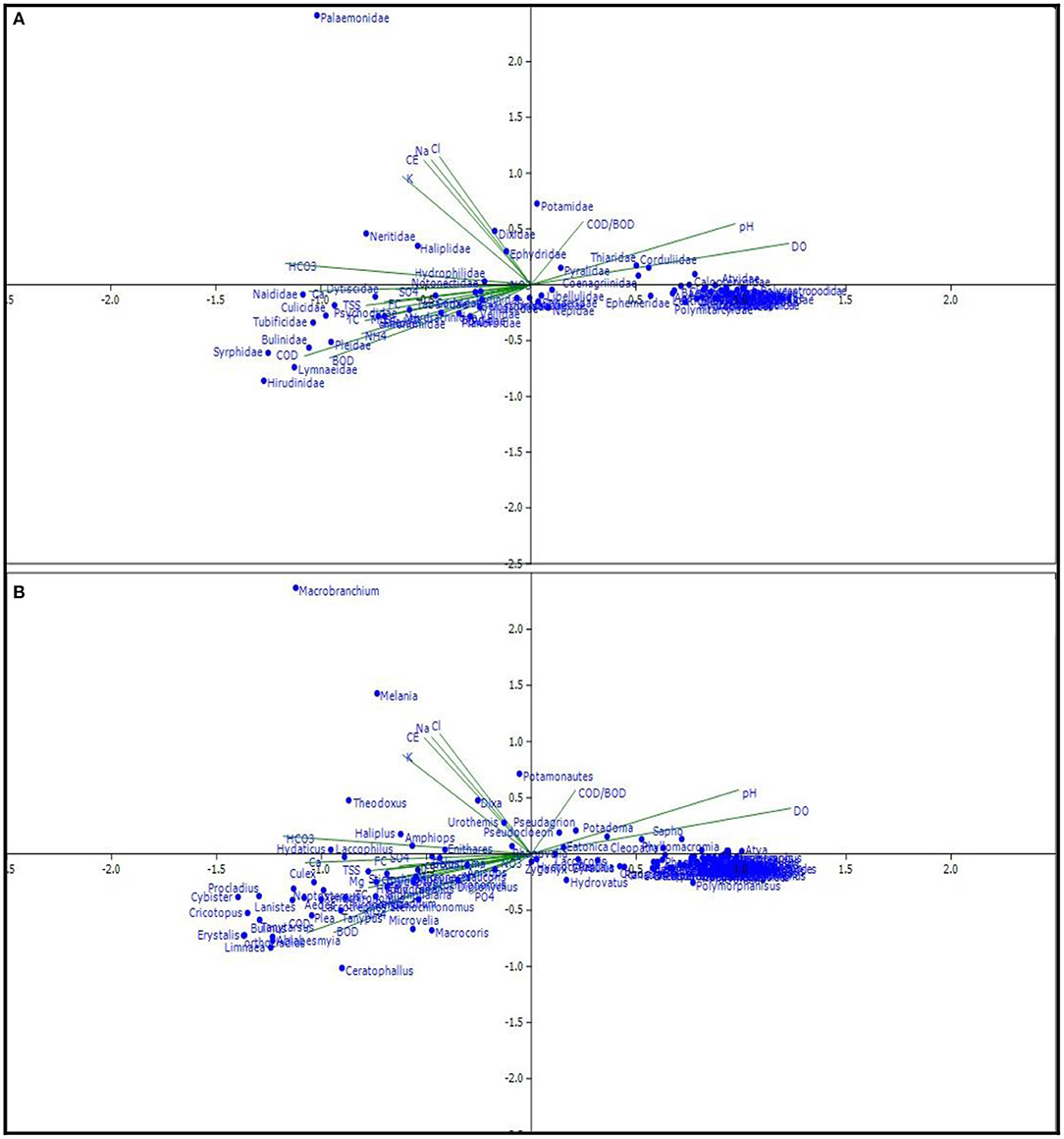
Figure 3. A canonical correspondence analysis (CCA) biplot showing the association between water quality parameters and abundance of macroinvertebrate family resolution (A) and genera resolution (B).
Response at Genus Level
The CCA indicates the environmental parameters that affect macroinvertebrate community at the genus level (Figure 3B). From Figure 3B and Table 3, it is seen that at the genus level, there is increase of sensitive taxa number for each group. Most of the genera from EPTO; genera Atya (Atyidae) from Decapoda, Limnius and Elmis (Elmidae) and Orectogyrus and Aulonogyrus (Gyrinidae) from Coleoptera; and some genera from Heteroptera such as Ranatra (Nepidae) and Hydrometra (Hydrometridae) were identified as sensitive taxa (Table 3). However, some genera from Ephemeroptera such as Pseudocloeon, Cloeon (Baetidae), Caenodes (Caenidae), Eatonica, and Ephemera (Ephemeridae) and others from Odonata such as Urothemis, Zygonyx, Olpogastra, Pantala (Libellulidae), and Pseudagrion (Coenagriinidae) are found to be tolerant taxa in this study. Some genera from Diptera are also identified as tolerant taxa (Chironomus, Culicoides, Dixa, Simulium, Stenochironomus, Cryptochironomus, Atherix, etc.), while other genera from Diptera are identified as resistant or resilient taxa (Erystalis, Orthocladius, Tanytarsus, Tanypus, Cricotopus, Ablabesmyia, Procladius, Aedes, Culex, etc.). The genera from Pulmonate (Limnaea, Ceratophallus, Biomphalaria, Bulinus, and Ceratophallus) were observed as resilient or resistant taxa, while the genera from Prosobranchia were distributed among tolerant taxa (Potadoma, Cleopatra, and Melania) and resilient taxa (Lanistes, Pila, and Theodoxus). Most of the genera from Coleoptera and Heteroptera identified here belong to tolerant and resistant taxa, with more genera from Heteroptera found in the tolerant group (Table 3). The genus Macrobrachium from Palaemonidae is positively influenced by salinity parameters and positively correlated with these parameters. In this study, it can be considered as an indicator taxon of water salinity (Table 3). The tolerant taxa were the most represented in terms of total number and at the different levels of identification. At the family level, 30 tolerant taxa, 24 sensitive taxa, 11 resistant taxa, and two taxa influenced by water salinity (salinity sensitive taxa) were identified. At the genus level, the same trend was observed, with 68 tolerant taxa, 53 sensitive taxa, 20 resistant taxa, and four salinity indicator taxa recorded (Table 3).
Response of Macroinvertebrate Metrics
The CCA indicates the association between water quality parameters and metrics (Figure 4). Table 4 presents the correlation coefficients (factor loadings) between observed variables (raw water quality parameters and macroinvertebrate metrics) and the latent or underlying variables (factors). The Supplementary Data in Appendix D indicate Spearman's correlation between water quality parameters and metrics. From Figure 4, three groups of metrics can be distinguished according to their relationship with water quality parameters. First, the metrics for taxonomic richness are composed of ETOF, ETOG, EPTF, and EPTG. These metrics are positively and significantly correlated with DO (r > 0.65, p < 0.05), but they are negatively and significantly correlated with EC, BOD, COD, TC, FC, Cl, Na, and NH4 (r < −0.60, p < 0.05). Then, the tolerant metrics are composed of ASPT and Biological Monitoring Working Party (BMWP) including MMIZB. These metrics are positively correlated with DO (r > 0.65, p < 0.05) and negatively correlated with EC, BOD, COD, TC, FC, and NH4 (r < −0.55, p < 0.05). Finally, the diversity indices are represented by Marg_G, Marg_F, Sha_H_F, and Sha_H_G, as well as the total number of family and genera that reveal a strong positive association with DO (r ≤ 0.5, p < 0.05) and significant negative correlation with EC, TC, Cl, Na, and NH4 (r ≥ −0.50, p < 0.05). From Table 4, the first factor is significantly and positively correlated with TSS, COD, BOD, TC, and NH4, but a significant negative association was observed with pH and DO. Therefore, the first factor can represent the water quality deterioration due to anthropogenic pressures. There is a positive association between the second factor and EC, Na, Cl, and K. This factor can represent the water salinity degree due mainly to mineralization processes or seawater. All the metrics tested in this study displayed a significant negative association with the first factor, but they did not reveal a significant association with the second factor. This result means that all metrics tested in this study are sensitive to water quality deterioration or anthropogenic disturbances affecting water quality.
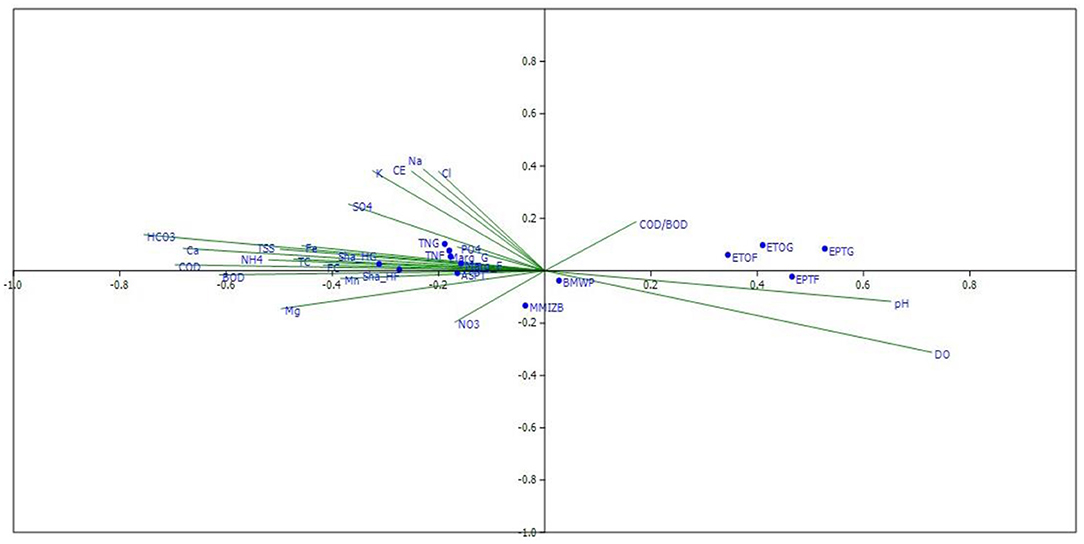
Figure 4. A canonical correspondence analysis (CCA) biplot showing the association between water quality parameters and macroinvertebrate metrics.
Discussions
Water Quality Parameters and Their Interpretation
Knowledge about water quality parameters is an important part of environmental monitoring and determining the condition of habitats. When water quality is poor, it affects not only aquatic life but also the aquatic ecosystem health. This section details with some parameters that affect water quality and aquatic ecosystem health. The values of EC show that more than 75% of water samples were in the range of natural freshwater, which varies from 0.5 to 1,500 μS/cm (Rodier et al., 2009). The high values of EC can affect freshwater organisms such as macroinvertebrate communities (Environmental Protection Agency of Ireland, 2001) as found in the present study; it is revealed that EC affects Palaemonidae taxon particularly the genus Macrobrachium. As a result, this taxon can detect the salinity or EC in the water of the Zio River. The pH has been considered as an important parameter in the ecology of aquatic macroinvertebrates (Thomsen and Friberg, 2002; Yuan, 2004). Benthic macroinvertebrates are sensitive to pH variation, and values below 5 or >9 are considered harmful (Yuan, 2004). The pH value found in the present study is in the range of natural water pH 6.5–8.5 according to some standards [U.S. Environmental Protection Agency (USEPA), 1980; Environmental Protection Agency of Ireland, 2001; Rodier et al., 2009; WHO, 2011]. However, according to Thomsen and Friberg (2002), the low pH values are associated with lower diversity of benthic macroinvertebrates and cause decreasing emergence rates of this community (Hall et al., 1980). The range of pH found in the present study is associated with high diversity of some macroinvertebrates such as EPTO taxa. The DO is an excellent indicator of water quality and one of the most sensitive to water quality degradation and therefore indicates the degree of water body self-purification (WHO, 1996; Sanchez et al., 2006; Makhoukh et al., 2011), whereas BOD indicates the quantity of biodegradable substances that mainly originate from organic matters into the water body due to human activities. In this study, the mean DO value observed in the range of saturated water (6–7 mg/L) with the 75th percentile value around 9.75 mg/L can indicate that most of the water samples can be classified from good to excellent quality according to international standards (Environmental Protection Agency of Ireland, 2001; Rodier et al., 2009). In the same vein, our study showed that DO and pH affect EPTO taxa found in the present study as sensitive taxa. Therefore, EPTO taxa or related metrics are able to detect variables such as DO and pH in the water of the Zio River.
The BOD value increases when DO value decreases and often indicates an organic pollution. We found that in the pristine rivers, this value is below 2 mg/L; and in moderately polluted rivers, it can range from 2 to 8 mg/L, although the rivers above 8 mg/L may be considered as severely polluted (Rejsek, 2002; Rodier et al., 2009). The COD is an indicative measurement of oxygen consumed by reducing substances (organic, nitrite, sulfide, ferrous salts, and others) in the water. It is also an important parameter of water quality assessment (Rejsek, 2002). The 75th percentile values and the mean values of BOD and COD indicate that the waters of this study can be considered as moderately polluted. The ratio COD/BOD is a meaningful parameter used to assess biodegradation in natural water. It is also used in environmental monitoring to assess the self-purification power of a river, which is the suitability for a river containing organic matter to transform this organic matter into mineral matter by natural processes (Mara and Pearson, 1999; Mara, 2004). A ratio of COD/BOD ranging from 1.5 to 2.5 indicates a good biodegradability and self-purification power (Mara et al., 2007; Rodier et al., 2009). Accordingly, the Zio River can be considered as a suitable river for self-purification process.
The TSS in water may cause pathogen problems, as the growth of anaerobic bacteria attached to suspended material can increase the risk of disease outbreaks and a significant portion of toxic organics (Rosewarne et al., 2014; Rono, 2017). In addition, the trace elements can be adsorbed onto organic matter present in water as suspended materials (Rono, 2017; Helmecke et al., 2020). TSS can also cause ecosystem disturbance through reduced water clarity, limiting photosynthesis and asphyxiation of some gill-breathing organism by clogging gills (Rodier et al., 2009; Rosewarne et al., 2014; Swinkels et al., 2014). The high levels of suspended solids can be considered as a form of pollution, which will have the effect of reducing the quality of the habitat for cold-water organisms (Rejsek, 2002; Rodier et al., 2009). All sampling sites within the Zio River contain acceptable concentration of TSS for aquatic ecosystem functioning life. Ammonium usually reflects a process of anaerobic degradation of nitrogenous organic matter and can be a good indicator of river pollution by urban effluents (Chapman and Kimstach, 1996). The range of values is near to natural water, but the standard deviation indicates a slight punctual source pollution of the study river. The present study revealed that COD, BOD, TSS, and affect OHDP taxa found here as resistant taxa. Thus, the findings from this study suggest that OHDP taxa or related metrics are able to detect COD, BOD, TSS, and in the water of the Zio River. Chloride is frequently associated with wastewater; and it is often included in assessments as an indicator of possible fecal contamination or as a measure of the amount of dispersion of wastewater discharges into the natural environment (WHO, 1996). In pristine freshwater, chloride concentrations are generally less than 10 mg/L and sometimes less than 2 mg/L (WHO, 1996; Rodier et al., 2009). Sodium concentration is often related to the nature of contact rock, evaporation, and seawater intrusion phenomenon. In this latter case, sodium content is often correlated with chloride content. The 75th percentile and mean values of sodium and chloride indicate that about 75% of water samples were not affected by sea intrusion, while 25% were affected as reported by Tampo (2018) at the lower reaches. TC and FC are parameters that indicate the possible fecal contamination of water. The recommended values in freshwater should be below 10 Cfu/100 ml. The values recorded here reveal a risk of fecal contamination at some sites. The Zio River water quality trend follows a gradient of pristine-to-poor water quality from the upper reaches to the lower reaches with a mosaic of good and poor water quality in the lower reaches based on proximity to anthropogenic pressure and saltwater intrusion (Tampo, 2018).
Response of Macroinvertebrates at Different Taxonomic Levels
In the present study, some phylum–class taxa could effectively indicate the ecological integrity associated with water quality. This could be explained by the fact that these phylum–classes were dominated by taxa with the same or very few different lifestyles that could point out a single category of pollution (Tachet et al., 2010). From this study, crustaceans were found to be good indicators of the freshwater salinity. Indeed, crustaceans were dominated by two families (Palaemonidae and Atyidae) and well known to be influenced by EC or water salinity (Attrill et al., 1999; Cuesta et al., 2006; N'Zi et al., 2008; Collocott et al., 2014). At the genus level, it was found that Macrobrachium from Palaemonidae was the most affected by salinity gradients and can be considered as the key indicator of water salinity. This corroborates the findings of other studies, which indicate that the life cycle or style of Macrobrachium is mainly related to estuary or water salinity (N'Zi et al., 2008; Collocott et al., 2014; Gangbe et al., 2016; Chen et al., 2018; Adam et al., 2019). Meanwhile, Mollusca taxon is associated with water eutrophication due to organic pollution. The findings revealed that phylum Annelida could be considered as generalists with high tolerance to stream degradation. At subclass–order level, the identified group of EPTO was found to be a sensitive group due to high number of sensitive taxa belonging to this group. This sensitivity of EPTO taxa is well known and described by earlier authors (Hilsenhof, 1988; Rodier et al., 2009; Tachet et al., 2010; Ko et al., 2020). The high abundance and diversity of EPTO taxa indicate good or excellent water quality with good ecological conditions (Tampo et al., 2015, 2020; Kaboré, 2016). Many metrics and multimetric indices calculated through EPTO taxa are widely used in biomonitoring programs as reported by many authors (Rodier et al., 2009; Mereta et al., 2012, 2013; Tampo et al., 2015, 2020; Kaboré et al., 2016). The results support other studies that found that the high abundance of Oligochaeta, Hirudinea, Pulmonates, and some Diptera often indicates organic pollution as seen in the present study. Globally, this has been seen with possible fecal contamination and substantial anthropogenic pressures (Hilsenhof, 1987; Rodier et al., 2009; Tachet et al., 2010; Tampo et al., 2020). This resistance to pollution of some macroinvertebrate groups can be explained by their lifestyle and the feeding strategy. For example, the Oligochaeta feed on detritus, bacteria, and dead remains of other aquatic organisms. Pulmonates are not only detritivores but also the intermediate host of many parasites (Tachet et al., 2010). According to Rodier et al. (2009), Oligochaeta and Leeches are indicators of polluted water and deteriorated habitat. Their number increase with the increase of eutrophication, which affects the overall ecosystem health (Kazanci et al., 2015). Within the insect fauna, Hilsenhof (1987) has demonstrated that Libellulidae, Lestidae, and Coenagrionidae from Odonata order are tolerant taxa. Furthermore, Rodier et al. (2009) showed that in a biomonitoring program based on a standardized global biotic index, Baetidae (algae grazer) and Caenidae from Ephemeroptera are resistant taxa, while Mary (1999) has reported some sensitive taxa in Heteroptera families. Here, we found within the EPTO order a notable diversity, with several families observed in good habitat conditions. This could be explained by the presence of families less tolerant or even sensitive to pollution. At the genus level, more taxa emerged as sensitive with specific indications. Some studies reported this increase of the sensitivity degree and the specific indication of macroinvertebrates when moving from phylum–class to species taxonomic level (Bailey et al., 2001; Jones, 2008; Menezes et al., 2011). However, according to the review paper of Jones (2008), many experts showed that the family level is sufficient or may be better in bioassessments even if genus and species taxonomic levels are required. The present study agrees with this consensus and shows that the family level is sufficient and better in the detection of nutrient pollution, organic pollution, and human disturbances and which pressures may drive shifts when using indices or metrics in macroinvertebrate communities. In addition to this, the present study showed that subclass and order levels are less sufficient of specific pollution but better for rapid evaluation of pollution at the global scale. These findings also agreed with those of other studies carried out in Africa (Dickens and Graham, 2002; Mereta et al., 2013; Elias et al., 2014; Kaboré et al., 2016; Dalu and Chauke, 2020; Ochieng et al., 2020; Tampo et al., 2020; Edegbene et al., 2021) in Asia (Blakely et al., 2014; Nguyen et al., 2014) and Europe (Poquet et al., 2009; Rodier et al., 2009; Costas et al., 2018) where these studies suggested the use of macroinvertebrates at the family level for the development of biomonitoring programs. The findings of the present study highlight the importance of using the family level for biomonitoring program in Togo and even elsewhere in Africa because of cost-effectiveness and the lack of systematic knowledge on macroinvertebrates.
Macroinvertebrate-Based Metrics
According to the results of the ordination (CCA and FA) and Spearman's correlation analysis, the metrics calculated at the family level and those calculated at the genus level were strongly correlated (r ≥ 0.80) and with the first factor (r ≥ |0.75|). This strong correlation is a redundant association between metrics calculated at family and genus levels. Thus, these two taxonomic levels can translate the same or common information. Our results also indicate that the taxonomic detail (family or genus level) does not substantially affect metrics used in bioassessment. These results are near the consensus that the lowest taxonomic level (species) is not always required for bioassessments (Jones, 2008). For example, in the case of differentiating impacted from unimpacted sites, some researchers showed that species-level identification was not always the best, but total richness and EPT richness performed better at family than at species, and family-level diversity measures also performed well (Schmidt-Kloiber and Nijboer, 2004; Schmidt-Kloiber et al., 2006). The negative correlation observed in the present study with all metrics confirmed the fact that they appear to be highly sensitive in the variation of water quality variables linked to the degree of human pressures. This sensitivity of macroinvertebrate metrics to the water quality and anthropogenic pressures is widely reported (Hering et al., 2006; Jun et al., 2012; Helson and Williams, 2013; Lakew and Moog, 2015; Kaboré et al., 2016; Aura et al., 2017; Tampo et al., 2020). The degree and the type of sensitivity of each one can explain the three groups of metrics identified by the CCA. In this way, reporting the sensitivity of EPTO group, many authors found that the diversity and abundance of this group increase with the increase of DO and with the decrease of nutrients, BOD, COD, and TSS (Mereta et al., 2013; Tampo et al., 2015; Kaboré et al., 2016). Thus, the EPTO group is the most suitable in the evaluation of water quality and aquatic ecosystems health and is used worldwide in bioassessments and monitoring programs (Armitage et al., 1983; Hilsenhof, 1987; Barbour et al., 1996, 1999; Schmidt-Kloiber et al., 2006; Rodier et al., 2009). The metrics related to tolerance measure (MMIZB; ASPT and BMWP) are also highly correlated with the first factor and some water quality parameters. This result revealed their sensitivity in water quality variation and mostly in the detection of anthropogenic pressures. Furthermore, in the previous studies, MMIZB revealed its sensitivity by discriminating impaired sites and unimpaired sites (Tampo et al., 2020). The two metrics ASPT and BMWP were also used in many studies as a single metric or integrated in a multimetric index for the monitoring of watersheds (Ferreira et al., 2011) for assessing water quality (Nguyen et al., 2014) and for assessing ecological integrity (Solimini et al., 2007; Lakew and Moog, 2015). That is likely because of their robust sensitivity and high discrimination power; the tolerance measure metrics are mostly recommended for ecological status evaluation under many conditions (Barbour et al., 1996; Hering et al., 2006). The total taxonomic number and the diversity indices were also calculated at family and genus levels. The results show that for the same metric of this group calculated, using family-level and genera-level data did not reveal a big difference about their relationship with water quality parameters and the first factor. Indeed, in bioassessments, richness is used to summarize biological condition, not to generate exhaustive taxon checklists, so coarse taxonomic resolution is often sufficient. For example, Marshall et al. (2006) reported only a 6% information loss when benthos data were rolled up to family from species. These results agree with those of several authors who found that correlations between ordination site-scores and environmental variables are slightly affected by taxonomic detail (Hewlett, 2000; Waite et al., 2000; Metzeling et al., 2006). In the same vein, Metzeling et al. (2006) reported that reducing taxonomic detail from species to family had little effect on ordination plots and gave similar principal axis correlations with environmental variables. Stronger evidence was provided by Hewlett's (2000) classification and ordination-based study of Australian stream sites. Even if the different taxonomic resolutions are known to influence the responses of macroinvertebrates through several mechanisms, our findings suggest that the use of macroinvertebrate metrics approach based on the family level has proven useful for Zio River's aquatic ecosystem monitoring.
Conclusion
In many developing countries across Africa such as Togo, rivers are threatened by intense agriculture (using fertilizers and pesticides), urbanization, and severe pollution, leading to habitat loss and water quality and negatively affecting aquatic organisms. With ongoing multiple pressures, the urgent need of biomonitoring tools is crucial for ensuring valuable biodiversity and water resource conservation. Along the Zio River gradient including several types of pressures, it has been demonstrated that macroinvertebrate indices or metrics have proven their sensitivity to water quality variation and human disturbance from order-level identification up to the genus level. Specifically, those related to EPTO taxa and tolerance were suitable in detection of water quality and human disturbances. The findings of this study confirm the importance of maintaining the family level for bioassessment and biomonitoring programs development in developing countries, especially in Sub-Saharan Africa, due to its cost-effectiveness and the lack of systematic knowledge on macroinvertebrates. However, the genus and species taxonomic levels are needed to improve the understanding of responses on the family level and the detection of specific pollution. We, therefore, recommend the use of the family level in metric and index formulation to monitor the Zio River.
Data Availability Statement
The original contributions presented in the study are included in the article/Supplementary Materials, further inquiries can be directed to the corresponding author.
Ethics Statement
The animal study was reviewed and approved by Wendengoudi Guenda, University of Joseph KI-ZERBO, Laboratory of Animals Biology and Ecology, 03 BP 7021 Ouagadougou 03, Burkina Faso.
Author Contributions
LT conceived the manuscript and wrote the paper with IK and EHA. LT and IK collected and analyzed the data. AO assisted LT in the laboratory analyses. LMB and GD-B supervised the laboratory analyses. EHA corrected the English language. LMB and GD-B supervised the study and contributed valuable comments to the manuscript. All authors contributed to the article and approved the submitted version.
Conflict of Interest
The authors declare that the research was conducted in the absence of any commercial or financial relationships that could be construed as a potential conflict of interest.
Publisher's Note
All claims expressed in this article are solely those of the authors and do not necessarily represent those of their affiliated organizations, or those of the publisher, the editors and the reviewers. Any product that may be evaluated in this article, or claim that may be made by its manufacturer, is not guaranteed or endorsed by the publisher.
Acknowledgments
We thank Prof. Guenda Wendengoudi, taxonomic specialist, for the support received during the stage of macroinvertebrate identification. We are also thankful to all the staff of the Laboratory of Applied Hydrology and Environment of Université de Lomé for their support during the fieldwork and laboratory analysis.
Supplementary Material
The Supplementary Material for this article can be found online at: https://www.frontiersin.org/articles/10.3389/frwa.2021.662765/full#supplementary-material
References
Abbasi, T., and Abbasi, S. A. (2012). Water Quality Indices. Amsterdam: Elsevier. doi: 10.1016/B978-0-444-54304-2.00016-6
Adam, C., Aristide, K. Y., Ebrima, N., and Tidiani, K. (2019). Diversity and spatial variation of benthic macroinvertebrates in the River Gambia estuary, West Africa. Int. J. Fish. Aqua. Stud. 7, 83–88
Adams, W. M. (1993). Indigenous use of wetlands and sustainable development in West Africa. Geog. J. 159, 209–218. doi: 10.2307/3451412
AFNOR (1997). Qualité de l'eau. Recueil des Normes Françaises Environnement. 2nd Edition, Tomes 1, 2, 3 et 4. Paris: AFNOR.
Agboola, O. A., Downs, C. T., and O'Brien, G. (2019). Macroinvertebrates as indicators of ecological conditions in the rivers of KwaZulu-Natal, South Africa. Ecol. Indic. 106:105465. doi: 10.1016/j.ecolind.2019.105465
Agboola, O. A., Downs, C. T., and O'Brien, G. (2020a). A multivariate approach to the selection and validation of reference conditions in KwaZulu-Natal Rivers, South Africa. Front. Environ. Sci. 8:584923. doi: 10.3389/fenvs.2020.584923
Agboola, O. A., Downs, C. T., and O'Brien, G. (2020b). Ecological risk of water resource use to the wellbeing of macroinvertebrate communities in the rivers of KwaZulu-Natal, South Africa. Front. Water. 2:584936. doi: 10.3389/frwa.2020.584936
Alba-Tercedor, J. (1996). “Aquatic macroinvertebrates and water quality of rivers,” in IV Simposio del Agua de Andalucía (SIAGA), vol 2, 203–213
Allan, J. D. (2004). Landscapes and riverscapes: the influence of land use on stream ecosystems. Annu. Rev. Ecol. Evol. Syst. 35, 257–284. doi: 10.1146/annurev.ecolsys.35.120202.110122
Armitage, P. D., Moss, D., Wright, J. F., and Furse, M. T. (1983). The performance of a new biological water quality score system based on macroinvertebrates over a wide range of unpolluted running-water sites. Water Res. 17, 333–347. doi: 10.1016/0043-1354(83)90188-4
Attrill, M. J., Power, M., and Thomas, R. M. (1999). Modelling estuarine Crustacea population fluctuations in response to physico-chemical trends. Mar. Ecol. Prog. Ser. 178, 89–99. doi: 10.3354/meps178089
Aura, C. M., Kimani, E., Musa, S., Kundu, R., and Njiru, J. M. (2017). Spatio-temporal macroinvertebrate multi-index of biotic integrity (MMiBI) for a coastal river basin: a case study of River Tana, Kenya. Ecohydrol. Hydrobiol. 17, 113–124. doi: 10.1016/j.ecohyd.2016.10.001
Azrina, M. Z., Yap, C. K., Rahim Ismail, A., Ismail, A., and Tan, S. G. (2006). Anthropogenic impacts on the distribution and biodiversity of benthic macroinvertebrates and water quality of the Langat River, Peninsular Malaysia. Ecotoxicol. Environ. Saf. 64, 337–347. doi: 10.1016/j.ecoenv.2005.04.003
Bailey, R. C., Norris, R. H., and Reynoldson, T. B. (2001). Taxonomic resolution of benthic macroinvertebrate communities in bioassessments. J. N. Am. Benthol. Soc. 20, 280–286. doi: 10.2307/1468322
Balian, E. V., Leveque, C., Segers, H., and Martens, K. (2008). The freshwater animal diversity assessment: an overview of the results. Hydrobiologia 595, 627–637. doi: 10.1007/s10750-007-9246-3
Barbour, M. T., Gerritsen, J., Griffith, G. E., Frydenborg, R., Mccarron, E., White, J. S., et al. (1996). A framework for biological criteria for Florida sites using benthic macroinvertebrates. J. North Am. Benthol. Soc. 15, 185–211. doi: 10.2307/1467948
Barbour, M. T., Gerritsen, J., Snyder, B. D., and Stribling, J. B. (1999). Rapid Bioassessment Protocols for Use in Streams and Wadeable Rivers: Periphyton, Benthic Macroinvertebrates and Fish, 2nd edition. Washington, DC: Environmental Protection Agency, Office of Water.
Bawa, L. M., Akakpo, W., Tampo, L., Kodom, T., Tchakala, I., Ameapoh, Y., et al. (2018). Assessment of seasonal and spatial variation of water quality in a coastal Basin: case of Lake Togo Basin. J. Sci. Eng. Res. 5, 117–132.
Berger, E., Haase, P., Oetken, M., and Sundermann, A. (2016). Field data reveal low critical chemical concentrations for river benthic invertebrates. Sci. Total Environ. 544, 864–873. doi: 10.1016/j.scitotenv.2015.12.006
Best, J., and Darby, S. E. (2020). The pace of human-induced change in large rivers: stresses, resilience, and vulnerability to extreme events. One Earth 2, 510–514. doi: 10.1016/j.oneear.2020.05.021
Blakely, T. J., Eikaas, H. S., and Harding, J. S. (2014). The Singscore: a macroinvertebrate biotic index for assessing the health of Singapore's streams and canals. Raffles Bull. Zool. 62, 540–548.
Bonada, N., Prat, N., Resh, V. H., and Statzner, B. (2006). Developments in aquatic insect biomonitoring: a comparative analysis of recent approaches. Annu. Rev. Entomol. 51, 495–523. doi: 10.1146/annurev.ento.51.110104.151124
Chapman, D., and Kimstach, V. (1996). Selection of Water Quality Variables. Water Quality Assessments: a Guide to the Use of Biota, Sediments and Water in Environment Monitoring, Chapman edition, 2nd ed. London: E and FN Spon, pp. 59–126. doi: 10.4324/NOE0419216001.ch3
Chen, Q. H., Chen, W. J., and Guo, Z. L. (2018). Caridean prawn (Crustacea, Decapoda) from Dongao Island, Guangdong, China. Zootaxa 4399, 315–328. doi: 10.11646/zootaxa.4399.3.2
Chetty, S., and Pillay, L. (2019). Assessing the influence of human activities on river health: a case for two South African rivers with differing pollutant sources. Environ. Monit. Assess. 191:168. doi: 10.1007/s10661-019-7308-4
Clifford, T., and Tariro, D. (2005). Land-use impacts on river water quality in lowveld sand river systems in south-east Zimbabwe. Land Use Water Resour. Res. 5, 3.1–3.10. doi: 10.22004/ag.econ.47961
Collen, B., Whitton, F., Dyer, E. E., Baillie, J. E. M., Cumberlidge, N., Darwall, W. R. T., et al. (2013). Global patterns of freshwater species diversity, threat and endemism. Global Ecol. Biogeogr. 23, 40–51. doi: 10.1111/geb.12096
Collocott, S. J., Vivier, L., and Cyrus, D. P. (2014). Prawn community structure in the subtropical Mfolozi–Msunduzi estuarine system, KwaZulu-Natal, South Africa. Afri. J. Aqua. Sci. 39, 127–140. doi: 10.2989/16085914.2014.925419
Costas, N., Pardo, I., Méndez-Fernández, L., Martínez-Madrid, M., and Rodríguez, P. (2018). Sensitivity of macroinvertebrate indicator taxa to metal gradients in mining areas in Northern Spain. Ecol. Indic. 93, 207–218. doi: 10.1016/j.ecolind.2018.04.059
Cuesta, J. A., Gonzalez-Ortegon, E., Rodriguez, A., Baldo, F., Vilas, C., and Drake, P. (2006). The decapod crustacean community of the Guadalquivir Estuary (SW Spain): seasonal and inter-year changes in community structure. Hydrobiologia 557, 85–95. doi: 10.1007/s10750-005-1311-1
Dallas, H. (1997). A preliminary evaluation of aspects of SASS (South Africa Scoring System) for rapid bioassessment of water quality in rivers. South. Afri. J. Aquat. Sci. 23, 79–94. doi: 10.1080/10183469.1997.9631389
Dalu, T., and Chauke, R. (2020). Assessing macroinvertebrate communities in relation to environmental variables: the case of Sambandou wetlands, Vhembe Biosphere Reserve. Appl. Water Sci. 10:6. doi: 10.1007/s13201-019-1103-9
Dickens, C., Cox, A., Johnston, R., Davison, S., Henderson, D., Meynell, P. J., et al. (2018). Monitoring the Health of the Greater Mekong's Rivers. Vientiane, Lao: CGIAR Research Program on Water, Land and Ecosystems (WLE).
Dickens, C. W., and Graham, P. (2002). The South African Scoring System (SASS) version 5 rapid bioassessment method for rivers. A. J. Aquat. Sci. 27, 1–10. doi: 10.2989/16085914.2002.9626569
Dudgeon, D., Arthington, A. H., Gessner, M. O., Kawabata, Z.-I., Knowler, D. J., Lévêque, C., et al. (2006). Freshwater biodiversity: importance, threats, status and conservation challenges. Biol. Rev. 81, 163–182. doi: 10.1017/S1464793105006950
Durand, J. R., and Levêque, C. (1981). Flore et Faune Aquatiques de l'Afrique Sahélo- Soudanienne, Tome 1 et Tome II. France: ORSTOM. I.R.D. n°44.
Edegbene, A. O., Odume, O. N., Arimoro, F. O., et al. (2021). Identifying and classifying macroinvertebrate indicator signature traits and ecological preferences along urban pollution gradient in the Niger Delta. Environ. Pollut. 281:117076. doi: 10.1016/j.envpol.2021.117076
Elias, J. D., Ijumba, J. N., Mgaya, Y. D., and Mamboya, F. A. (2014). Study on freshwater macroinvertebrates of some Tanzanian rivers as a basis for developing biomonitoring index for assessing pollution in tropical African regions. J. Ecosyst. 2014:985389. doi: 10.1155/2014/985389
Environmental Protection Agency of Ireland (2001). Parameters of Water Quality Interpretation and Standards. Ireland: EPA
Faridah, O., Alaa Eldin, M. E., and Ibrahim, M. (2012). Trend analysis of a tropical urban river water quality in Malaysia. J. Environ. Monit. 14, 3164–3173. doi: 10.1039/c2em30676j
Fayiga, A. O., Ipinmoroti, M. O., and Chirenje, T. (2018). Environmental pollution in Africa, environment. Dev. Sustain. 20, 41–73. doi: 10.1007/s10668-016-9894-4
Ferreira, W. R., Paiva, L. T., and Callisto, M. (2011). Development of a benthic multimetric index for biomonitoring of a neotropical watershed. Braz. J. Biol. 71, 15–25. doi: 10.1590/S1519-69842011000100005
Forio, M. A. E., Goethals, P. L. M., Lock, K., Asio, V., Bande, M., and Thas, O. (2018). Model-based analysis of the relationship between macroinvertebrate traits and environmental river conditions. Environ. Model. Softw. 106, 57–67. doi: 10.1016/j.envsoft.2017.11.025
Gangbe, L., Agadjihouede, H., Chikou, A., Senouvo, P., Mensah, G. A., and Laleye, P. (2016). Biologie et perspectives d'élevage de la crevette géante d'eau douce Macrobrachium vollenhovenii (Herklots, 1857). Int. J. Biol. Chem. Sci. 10, 573–598. doi: 10.4314/ijbcs.v10i2.11
Gonçalves, F. B., and Menezes, M. S. (2011). A comparative analysis of biotic indices that use macroinvertebrates to assess water quality in a coastal river of Paraná state, southern Brazil. Biota Neotrop. 11, 27–36. doi: 10.1590/S1676-06032011000400002
Hall, R. J., Likens, G. E., Fiance, S. B., and Hendrey, G. R. (1980). Experimental acidification of a stream in the Hubbard Brook experimental forest, New Hampshire. Ecology 61, 976–989. doi: 10.2307/1936765
He, S., Soininen, J., Chen, K., and Wang, B. (2020). Environmental factors override dispersal-related factors in shaping diatom and macroinvertebrate communities within stream networks in China. Front. Ecol. Evol. 8:141. doi: 10.3389/fevo.2020.00141
Helmecke, M., Fries, E., and Schulte, C. (2020). Regulating water reuse for agricultural irrigation: risks related to organic micro-contaminants. Environ. Sci. Eur. 32:4. doi: 10.1186/s12302-019-0283-0
Helson, J. E., and Williams, D. D. (2013). Development of a macroinvertebrate multimetric index for the assessment of low-land streams in the neotropics. Ecol. Indic. 29, 167–178. doi: 10.1016/j.ecolind.2012.12.030
Hering, D., Johnson, R. K., Kramm, S., Schmutz, S., Szoszkiewicz, K., and Verdonschot, P. F. M. (2006). Assessment of European streams with diatoms, macrophytes, macroinvertebrates and fish: a comparative metric-based analysis of organism response to stress. Fresh. Biol. 51, 1757–1785. doi: 10.1111/j.1365-2427.2006.01610.x
Hewlett, R. (2000). Implications of taxonomic resolution and sample habitat for stream classification at a broad geographic scale. J. N. Am. Benthol. Soc. 19, 352–361. doi: 10.2307/1468077
Hilsenhof, W. L. (1987). An improved biotic index of organic stream pollution. Great Lakes Entom. 20, 31–39.
Hilsenhof, W. L. (1988). Rapid field assessment of organic pollution with a family-level biotic index. J. N. Am. Benthol. Soc. 7, 65–68. doi: 10.2307/1467832
Hogan, Z. (2011). Review of freshwater fish. UNEP/CMS/Inf.10.33. Available online at: https://www.cms.int/sites/default/files/document/inf_33_freshwater_fish_eonly_0.pdf (accessed July 31, 2021).
Jones, F. C. (2008). Taxonomic sufficiency: the influence of taxonomic resolution on freshwater bioassessments using benthic macroinvertebrates. Environ. Rev. 16, 45–69. doi: 10.1139/A07-010
Jun, Y. C., Won, D. H., Lee, S. H., Kong, D. S., and Hwung, S. J. (2012). A multimetric benthic macroinvertebrate index for the assessment of stream biotic integrity in Korea. Int. J. Environ. Res. Public Health 9, 3599–3628. doi: 10.3390/ijerph9103599
Kaboré, I. (2016). Benthic invertebrate assemblages and assessment of ecological status of water bodies in the Sahelo- Soudanian area (Burkina Faso, West Africa), Thesis of University of Natural Resources and Life Sciences, Vienna, Austria. Available online at: https://zidapps.boku.ac.at/abstracts/download.php?property_id=107anddataset_id=14470 (accessed July 31, 2021).
Kaboré, I., Moog, O., Alp, M., Guenda, W., Koblinger, T., Mano, K., et al. (2016). Using macroinvertebrates for ecosystem health assessment in semi-arid streams of Burkina Faso. Hydrobiologia 766, 57–74. doi: 10.1007/s10750-015-2443-6
Kaboré, I., Moog, O., Ouéda, A., Sendzimir, J., Ouédraogo, R., Guenda, W., et al. (2018). Developing reference criteria for the ecological status of West African rivers. Environ. Monit. Assess. 190:2. doi: 10.1007/s10661-017-6360-1
Kazanci, N., Ekingen, P., Dügel, M., and Türkmen, G. (2015). Hirudinea (Annelida) species and their ecological preferences in some running waters and lakes. Int. J. Environ. Sci. Technol. 12, 1087–1096. doi: 10.1007/s13762-014-0574-3
Kenney, M. A., Sutton-Grier, A. E., Smith, R. F., and Gresens, S. E. (2009). Benthic macroinvertebrates as indicators of water quality: The intersection of science and policy. Terr. Arthropod Rev. 2, 99–128.
King, R. S., and Baker, M. E. (2010). Considerations for analyzing ecological community thresholds in response to anthropogenic environmental gradients. J. N. Am. Benthol. Soc. 29, 998–1008. doi: 10.1899/09-144.1
Ko, N. T., Suter, P., Conallin, J., Rutten, M., and Bogaard, T. (2020). Aquatic macroinvertebrate community changes downstream of the hydropower generating dams in myanmar-potential negative impacts from increased power generation. Front. Water. 2:573543. doi: 10.3389/frwa.2020.573543
Kurthen, A. L., He, F., Dong, X., Maasri, A., Wu, N., et al. (2020). Metacommunity structures of macroinvertebrates and diatoms in high mountain streams, Yunnan, China. Front. Ecol. Evol. 8:571887. doi: 10.3389/fevo.2020.571887
Lakew, A., and Moog, O. (2015). A multimetric index based on benthic macroinvertebrates for assessing the ecological status of streams and rivers in central and southeast highlands of Ethiopia. Hydrobiologia 751, 229–242. doi: 10.1007/s10750-015-2189-1
Li, F., Cai, Q., Qu, X., Tang, T., Wu, N., Fu, X., et al. (2012). Characterizing macroinvertebrate communities across China: large-scale implementation of a self-organizing map. Ecol. Indic. 23, 394–401. doi: 10.1016/j.ecolind.2012.04.017
Makhoukh, M., Sbaa, M., Berrahou, A., and Van, M. C. (2011). Contribution à l'etude physicochimique des eaux superficielles de l'oued moulouya (maroc oriental). Larh. J. 9, 149–169
Mara, D. D. (2004). Domestic Wastewater Treatment in Developing Countries. London: Earthscan Publications.
Mara, D. D., Mills, S. W., Pearson, H. W., and Alabaster, G. P. (2007). Waste stabilization ponds: a viable alternative for small community treatment systems. Water Environ. J. 6, 72–78. doi: 10.1111/j.1747-6593.1992.tb00740.x
Mara, D. D., and Pearson, H. W. (1999). A hybrid waste stabilization pond and wastewater storage and treatment reservoir system for wastewater reuse for both restricted and unrestricted irrigation. Water Res. 33, 591–594.
Marshall, J. C., Steward, A. L., and Harch, B. D. (2006). Taxonomic resolution and quantification of freshwater macroinvertebrate samples from an Australian dryland river: the benefits and costs of using species abundance data. Hydrobiologia 572, 171–194. doi: 10.1007/s10750-005-9007-0
Mary, N. (1999). caracterisations physicochimique et biologique des cours d'eau de la nouvelle-caledonie, proposition d'un indice biotique fonde sur l'etude des macroinvertebres benthiques. Thèse de doctorat,univ du Pacifique, Nouvelle Calédonie.
Menezes, S., Baird, D. J., and Soares, A. M. V. M. (2011). Beyond taxonomy: a review of macroinvertebrate trait-based community descriptors as tools for freshwater biomonitoring. J. Appl. Ecol. 47, 711–719. doi: 10.1111/j.1365-2664.2010.01819.x
Mereta, S. T., Boets, P., Bayih, A., Malu, A., Ephrem, Z., Sisay, A., et al. (2012). Analysis of environmental factors determining the abundance and diversity of macroinvertebrate taxa in natural wetlands of Southwest Ethiopia. Ecol. Info. 7, 52–61. doi: 10.1016/j.ecoinf.2011.11.005
Mereta, T. S., Boets, P., De Meester, L., and Goethals, P. L. M. (2013). Development of a multimetric index based on benthic macroinvertebrates for the assessment of natural wetlands in Southwest Ethiopia. Ecol. Indic. 29, 510–521. doi: 10.1016/j.ecolind.2013.01.026
Merritt, R. W., and Cummins, K. W. (1996). An Introduction to the Aquatic Insects of North America, 3rd Edition. Dubuque, IW: Kendall/Hunt Publishing Company
Metcalfe, J. L. (1989). Biological water quality assessment of running waters based on macroinvertebrate communities: history and present status in Europe. Environ. Pollut. 60, 101–139. doi: 10.1016/0269-7491(89)90223-6
Metzeling, L., Perriss, S., and Robinson, D. (2006). Can the detection of salinity and habitat simplification gradients using rapid bioassessment of benthic invertebrates be improved through finer taxonomic resolution or alternative indices? Hydrobiologia 572, 235–252. doi: 10.1007/s10750-005-9004-3
Nessimian, J. L., Venticinque, E. M., Zuanon, J., De Marco, P. Jr., Gordo, M., et al. (2008). Land use, habitat integrity, and aquatic insect assemblages in Central Amazonian streams. Hydrobiologia 614, 117–131. doi: 10.1007/s10750-008-9441-x
Nguyen, H. H., Everaert, G., Gabriels, W., Hoang, T. H., and Goethals, P. L. M. (2014). A multimetric macroinvertebrate index for assessing the water quality of the Cau river basin in Vietnam. Limnologica 45, 16–23. doi: 10.1016/j.limno.2013.10.001
Nguyen, T. H. T., Forio, M. A. E., Boets, P., Lock, K., Damanik Ambarita, M. N., Suhareva, N., et al. (2018). Threshold responses of macroinvertebrate communities to stream velocity in relation to hydropower dam: a case study from the Guayas River Basin (Ecuador). Water 10:1195. doi: 10.3390/w10091195
N'Zi, G. K., Gooré, B. G., Kouamélan, E. P., Koné, T., N'Douba, V., and Ollevier, F. (2008). Influence des facteurs environnementaux sur la répartition spatiale des crevettes dans un petit bassin ouest africain—rivière Boubo—Côte d'Ivoire, Tropicultura 26, 17–23.
Ochieng, H., Odong, R., and Okot-Okumu, J. (2020). Comparison of temperate and tropical versions of Biological Monitoring Working Party (BMWP) index for assessing water quality of River Aturukuku in Eastern Uganda. Glob. Ecol. Conserv. 23:e01183. doi: 10.1016/j.gecco.2020.e01183
Pinel-Alloul, B., M'ethot, G., Lapierre, L., and Willsie, A. (1996). Macrobenthic community as a biological indicator of ecological and toxicological factors in Lake Saint-Francois (Quebec). Environ. Pollut. 91, 65–87. doi: 10.1016/0269-7491(95)00033-N
Poquet, J. M., Alba-Tercedor, J., Punti, T., Sanchez-Montoya, M. M., Robles, S., Alvarez, M., et al. (2009). The MEDiterranean Prediction And Classi?cation System (MEDPACS): an implementation of the RIVPACS/ AUSRIVAS predictive approach for assessing Mediterranean aquatic macroinvertebrate communities. Hydrobiologia 623, 153–171.
Raburu, P. O., Masese, F. O., and Aura, M. C. (2009). Macroinvertebrate Index of Biotic Integrity (M-IBI) for monitoring rivers in the upper catchment of Lake Victoria Basin, Kenya. Aquat. Ecosyst. Health Manag. 12, 197–205. doi: 10.1080/14634980902907763
Rejsek, F. (2002). Analyse des eaux aspects réglémentaires et techniques, sciences et techniques de l'envionnement, Tome 2, édition aquitaine.
Resende, P. C., Resende, P., Pardal, M., Almeida, S., and Azeiteiro, U. (2010). Use of biological indicators to assess water quality of the Ul River (Portugal). Environ. Monit. Assess. 170, 535–544. doi: 10.1007/s10661-009-1255-4
Resh, V. H. (2008). Which group is best? Attributes of different biological assemblages used in freshwater biomonitoring programs. Environ. Monit. Assess. 138, 131–138. doi: 10.1007/s10661-007-9749-4
Richter, B. D., Mathews, R., Harrrison, D. L., and Wigington, R. (2003). Ecologically sustainable water management: Managing river flows for ecological integrity. Ecol. Appl.13, 206–224. doi: 10.1890/1051-0761(2003)013[0206:ESWMMR]2.0.CO;2
Rodell, M., Famiglietti, J. S., Wiese, D. N., Reager, J. T., Beaudoing, H. K., Landerer, F. W., et al. (2018). Emerging trends in global freshwater availability. Nature 557, 651–659. doi: 10.1038/s41586-018-0123-1
Rodier, J., Legube, B., and Merlet, N. (2009). L'Analyse de l'eau, 9e édition entièrement mise à jour.
Rono, A. K. (2017). Evaluation of TSS, BOD5, and TP in Sewage Effluent Receiving Sambul River. J. Pol. Eff. Cont. 5:2
Rosewarne, P. J., Svendsen, J. C., Mortimer, R. J. G., and Dunn, A. M. (2014). Muddied waters: suspended sediment impacts on gill structure and aerobic scope in an endangered native and an invasive freshwater crayfish, Hydrobiologia 722, 61–74. doi: 10.1007/s10750-013-1675-6
Sanchez, E., Colmenarejo, M. F., Vicente, J., Rubio, A., García, M. G., Travieso, L., et al. (2006). Use of the water quality index and dissolved oxygen deficit as simple indicators of watersheds pollution. Ecol. Indic. 7, 315–328. doi: 10.1016/j.ecolind.2006.02.005
Schmidt-Kloiber, A., Graf, W., Lorenz, A., and Moog, O. (2006). The AQEM/STAR taxalist- a pan-European macro-invertebrate ecological database and taxa inventory. Hydrobiologia 566, 325–342. doi: 10.1007/s10750-006-0086-3
Schmidt-Kloiber, A., and Nijboer, R. C. (2004). The effect of taxonomic resolution on the assessment of ecological water quality classes. Hydrobiologia 516, 269–283. doi: 10.1023/B:HYDR.0000025270.10807.10
Smith, K. G., Diop, M. D., Niane, M., and Darwall, W. R. T. (2009). The Status and Distribution of Freshwater Biodiversity in Western Africa Gland. IUCN: Switzerland and Cambridge, UK
Solimini, A. G., Bazzanti, M., Ruggiero, A., and Carchini, G. (2007). Developing a multimetric index of ecological integrity based on macroinvertebrates of mountain ponds in central Italy. Hydrobiologia 597, 109–123. doi: 10.1007/s10750-007-9226-7
Swinkels, L. H., Van de Ven, M. W. P. M., Stassen, M. J. M., Van der Velde, G., Lenders, H. J. R., and Smolders, A. J. P. (2014). Suspended sediment causes annual acute fish mortality in the Pilcomayo River (Bolivia). Hydrol. Process. 28, 8–15. doi: 10.1002/hyp.9522
Tachet, H., Richoux, P., Bournaud, M., and Usseglio-Polatera, P. (2010). Invertébrés d'eau douce, Systématique, Biologie, écologie. Paris: éditions CNRS.
Tampo, L. (2018). Hydrobiologie et Hydrochimie du bassin du Zio (Togo). Editions Universitaires Européenne.
Tampo, L., Lazar, I. M., Koboré, I., Oueda, A., Akpataku, V., et al. (2020). A multimetric index for assessment of aquatic ecosystem health based on macroinvertebrates for the Zio river basin in Togo. Limnologica 83:125783. doi: 10.1016/j.limno.2020.125783
Tampo, L., Ouéda, A., Nuto, Y., Kaboré, I., Bawa, L. M., Djaneye-Boundjou, G., et al. (2015). Using physicochemicals variables and benthic macroinvertebrates for ecosystem health assessment of inland rivers of Togo. Int. J. Inn. Appl. Stud. 12, 961–976.
Thomsen, A. G., and Friberg, N. (2002). Growth and emergence of the stonefly Leuctra nigra in coniferous forest streams with contrasting pH. Fresh. Biol. 47, 1159–1172. doi: 10.1046/j.1365-2427.2002.00827.x
Traoré, A. N., Mulaudzi, K., Chari, G. J. E., Foord, S. H., Mudau, L. S., Barnard, T. G., et al. (2016). The impact of human activities on microbial quality of rivers in the Vhembe District, South Africa. Int. J. Environ. Res. Public Health 13:817. doi: 10.3390/ijerph13080817
U.S. Environmental Protection Agency (USEPA) (1980). Protocol Development: Criteria and Standards for Potable Reuse and Feasible Alternatives. Washington, DC: USEPA.
Vorosmarty, C. J., McIntyre, P. B., Gessner, M. O., Dudgeon, D., Prusevich, A., Green, P., et al. (2010). Global threats to human water security and river biodiversity. Nature 467, 555–561. doi: 10.1038/nature09440
Waite, I. R., Herlihy, A. T., Larsen, D. P., and Klemm, D. J. (2000). Comparing strengths of geographic and non-geographic classifications of stream benthic macroinvertebrates in the Mid-Atlantic Highlands, USA. J. N. Am. Benthol. Soc. 19, 429–441. doi: 10.2307/1468105
Wan Abdul Ghani, W. M. H., Kutty, A. A., Mahazar, M. A., Al-Shami, S. A., and Hamid, S. A. (2018). Performance of biotic indices in comparison to chemical-based Water Quality Index (WQI) in evaluating the water quality of urban river. Environ. Monit. Assess. 190:297. doi: 10.1007/s10661-018-6675-6
Wantzen, K. M., Alves, C. B. M., Badiane, S. D., Bala, R., Blettler, M., Callisto, M., et al. (2019). Urban stream and wetland restoration in the global south—a DPSIR analysis. Sustainability 11:4975. doi: 10.3390/su11184975
Weigel, B. M., and Robertson, D. M. (2007). Identifying biotic integrity and water chemistry relations in nonwadeable rivers of Wisconsin: toward the development of nutrient criteria. Environ. Manag. 40, 691–708. doi: 10.1007/s00267-006-0452-y
WHO (1996). Water Quality Assessments—a Guide to Use of Biota, Sediments and Water in Environmental Monitoring—Second Edition. Geneva: WHO.
Wright, J., Furse, M., Armitage, P., and Moss, D. (1993). New procedures for identifying running-water sites subject to environmental stress and for evaluating sites for conservation, based on the macroinvertebrate fauna. Arch. Hydrol. 127, 319–326. doi: 10.1127/archiv-hydrobiol/127/1993/319
Keywords: sensitivity, metrics, ecological indicators, water quality, macroinvertebrates, taxonomic level
Citation: Tampo L, Kaboré I, Alhassan EH, Ouéda A, Bawa LM and Djaneye-Boundjou G (2021) Benthic Macroinvertebrates as Ecological Indicators: Their Sensitivity to the Water Quality and Human Disturbances in a Tropical River. Front. Water 3:662765. doi: 10.3389/frwa.2021.662765
Received: 01 February 2021; Accepted: 09 August 2021;
Published: 16 September 2021.
Edited by:
Gordon O'Brien, University of Mpumalanga, South AfricaReviewed by:
Letícia Santos De Lima, Federal University of Minas Gerais, BrazilMatthew Burnett, University of KwaZulu-Natal, South Africa
Copyright © 2021 Tampo, Kaboré, Alhassan, Ouéda, Bawa and Djaneye-Boundjou. This is an open-access article distributed under the terms of the Creative Commons Attribution License (CC BY). The use, distribution or reproduction in other forums is permitted, provided the original author(s) and the copyright owner(s) are credited and that the original publication in this journal is cited, in accordance with accepted academic practice. No use, distribution or reproduction is permitted which does not comply with these terms.
*Correspondence: Lallébila Tampo, charlestampo@gmail.com