- 1School of Emergency Management, Jiangsu University, Zhenjiang, China
- 2School of the Environment and Safety Engineering, Jiangsu University, Zhenjiang, China
- 3Department of Botany, Pir Mehr Ali Shah-Arid University (PMAS), Rawalpindi, Pakistan
- 4Key Laboratory of Environmental Biotechnology, Research Center for Eco-Environmental Sciences, Chinese Academy of Sciences, Beijing, China
- 5Institute of Environmental Health and Ecological Security, School of Environment and Safety Engineering, Jiangsu University, Zhenjiang, China
- 6Division of Environmental Science and Ecological Engineering, Korea University, Seoul, Republic of Korea
- 7Jiangsu Collaborative Innovation Center of Technology and Material of Water Treatment, Suzhou University of Science and Technology, Suzhou, China
Drought stress can significantly affect plant growth and development. Biochar (BC) and plant growth-promoting rhizobacteria (PGPR) have been found to increase plant fertility and development under drought conditions. The single effects of BC and PGPR in different plant species have been widely reported under abiotic stress. However, there have been relatively few studies on the positive role of PGPR, BC, and their combination in barley (Hordeum vulgare L.). Therefore, the current study investigated the effects of BC from Parthenium hysterophorus, drought tolerant PGPR (Serratia odorifera), and the combination of BC + PGPR on the growth, physiology, and biochemical traits of barley plants under drought stress for two weeks. A total of 15 pots were used under five treatments. Each pot of 4 kg soil comprised the control (T0, 90% water), drought stress alone (T1, 30% water), 35 mL PGPR/kg soil (T2, 30% water), 2.5%/kg soil BC (T3, 30% water), and a combination of BC and PGPR (T4, 30% water). Combined PGPR and BC strongly mitigated the negative effects of drought by improving the shoot length (37.03%), fresh biomass (52%), dry biomass (62.5%), and seed germination (40%) compared to the control. The PGPR + BC amendment treatment enhanced physiological traits, such as chlorophyll a (27.9%), chlorophyll b (35.3%), and total chlorophyll (31.1%), compared to the control. Similarly, the synergistic role of PGPR and BC significantly (p< 0.05) enhanced the antioxidant enzyme activity including peroxidase (POD), catalase (CAT), and superoxide dismutase (SOD) to alleviate the toxicity of ROS. The physicochemical properties (N, K, P, and EL) of the soils were also enhanced by (85%, 33%, 52%, and 58%) respectively, under the BC + PGPR treatment compared to the control and drought stress alone. The findings of this study have suggested that the addition of BC, PGPR, and a combination of both will improve the soil fertility, productivity, and antioxidant defense systems of barley under drought stress. Therefore, BC from the invasive plant P. hysterophorus and PGPR can be applied to water-deficient areas to improve barley crop production.
1 Introduction
Barley (Hordeum vulgare L.) is an important winter cereal crop of the Poaceae family, grown in the arid and semi-arid regions of West Asia and North Africa (Hafez et al., 2020), and is a well-known quality food crop worldwide (Hafez et al., 2016). Approximately 25% of the world’s barley crop is malted or used as human food and 75% is used as animal feed (El-Hashash and El-Absy, 2019: Newton et al., 2011). Barley crops cover an area of 0.047 billion ha worldwide, and the cultivation of this plant produces approximately 0.147 billion tons (Scheitrum et al., 2020). It has a short life cycle, is resistant to saline and drought stress, and is ranked as the 4th most widely grown cereal crop after wheat, rice, and maize (Akhtar et al., 2021). Barley is a suitable crop for areas where irrigation is limited and is highly tolerant to many other biotic and abiotic stresses (Wiegmann et al., 2019), making it suitable for cultivation in challenging environments (Feiziasl, 2022).
One of the life threating and environmental stresses that effects plant growth, soil quality, and water availability is known as drought stress (Kilic, 2014). Drought presents a severe threat to humans and agriculture, with approximately 53 million people worldwide being affected by limited water availability (Elakhdar et al., 2022). Water scarcity and high temperatures are worldwide issues affecting the survival of agricultural crops and sustainable food production (Mertz-Henning et al., 2018). Severe drought stress results in inhibition of photosynthesis, metabolic disturbance, chlorosis, necrosis, and tissue damage (Jaleel et al., 2009). Drought stress reduces transpiration in plants, which is required for nutrient uptake from the soil and results in growth retardation and development (Çakmakçi et al., 2007). Plant turgidity, metabolism, and osmoregulation are inhibited in response to water-deficit conditions, leading to decreased crop production and development (Gordon et al., 2019). Crop response to drought stress is a critical issue. Therefore, there is a strong need to develop new methods to increase tolerance mechanism in plants (Ashoub et al., 2015). Two approaches are currently used to reduce the negative effects of drought stress on plant growth, that is, the application of plant growth-promoting rhizobacteria (PGPR) and biochar (BC) to soils. Both approaches are fast-growing, eco-friendly, and inexpensive in enhancing plant productivity under abiotic stress (Ullah et al., 2020).
Plant growth-promoting rhizobacteria are endophytic bacteria present in plant roots that promote plant growth and interact positively with plant and soil microorganisms (Forni et al., 2017). PGPR colonize the rhizosphere of plants to increase plant growth via direct or indirect mechanisms (Santoyo et al., 2021). Direct mechanisms include nutrient acquisition such as nitrogen fixation, P solubilization, and production of phytohormone. Meanwhile, indirect mechanisms inhibit the function of other pathogenic organism through biocontrol agents (Frampton et al., 2012) PGPR acts as a fertilizer for crop protection, soils structure, decomposition of organic matter and recycling of essential nutrients under abiotic stress, that is, drought stress (Kasim et al., 2016; Gouda et al., 2018). Under water deficit condition, PGPR promote root development in the soils (Kasim et al., 2016). In maize, it has been found that the application of PGPR is effective for plant growth and protection under drought stress (Khan et al., 2021), with positive roles in increasing crop production and yield (Akhtar et al., 2021). Akhtar et al. (2015) reported that PGP and BC enhanced physiological and reproductive responses in maize under saline conditions.
In agriculture, biochar (BC) is also known as “black gold” because it is produced from fossils and dead plant tissues. It increases essential minerals in the soil, including nitrogen (N), sulfur (S), phosphorous (P), carbon (C), and potassium (K) (Sánchez-Reinoso et al., 2020; Ullah et al., 2020). BC is used on farmlands to boost carbon and nitrogen levels (Pérez-Cabrera et al., 2022) and may sequester atmospheric carbon dioxide (CO2) (Feng et al., 2021). According to the EPA (2010), approximately 40% of greenhouse gases generated by of the addition of chemical fertilizers to soils can be reduced by adding BC (Schmidt et al., 2014). The highly porous structure and surface area of BC can alleviate the effects of drought because of its water retention capacity owing to adhesion and cohesion forces (Dempster et al., 2012). BC promotes water use efficiency because its structure consists of an oxygen functional group that stores more moisture in the soil (Suliman et al., 2017) Recently, it has been used to increase cowpea plant productivity, nutrient uptake, and antioxidant activity under drought stress (Farooq et al., 2021). Some reports have suggested that BC from Parthenium hysterophorus can be used as green muck, compost, and in soil bioremediation to enhance the physiological and biochemical characteristics of plants (El-Naggar et al., 2015; Tefera et al., 2022).
Parthenium hysterophorus is native to America, but has become invasive in Asia, Australia, and Africa (Javaid, 2010). P. hysterophorus was first introduced to India in 1955 and was later transported to Pakistan (Khan et al., 2012). This weed is among the top 10 plants that are harmful to agricultural systems (Rout and Callaway, 2012), and toxic to humans (Khan et al., 2012). Therefore, the positive management of this weed and biochar production are alternative ways to use it in the environment. However, BC production from this weed could be a useful strategy for controlling environmental stressors in agroecosystems (Masulili et al., 2010; Kumar et al., 2013). Biochar from P. hysterophorus can significantly enhance crop productivity and increase soil nutrients (Ahmad et al., 2021). Barley plants were found to be tolerant to salt stress following the application of BC and PGPR (Cardinale et al., 2015). The combined effect of PGPR and BC holds potential for Brassica napus in alleviating the toxic effects of drought, directly or indirectly, to promote physiological and biochemical responses (Lalay et al., 2022). Ain et al. (2023) observed a positive effect from P. hysterophorus biochar on the growth traits, physicochemical properties, and antioxidant activities of rice and wheat. Therefore, PGPR and BC may interact to enhance plant productivity and tolerance to various stressors. To date, no studies have yet reported the effects of the interactions between PGPR and BC on soil fertility or barley growth productivity. Therefore, the present study was conducted to investigate the effects of BC from P. hysterophorus and PGRP on the growth, physiology, and reproductive output of barley under drought stress conditions. We also evaluated the positive effects of PGPR and BC on soil fertility and nutrient uptake by barley under drought stress.
2 Materials and methods
2.1 Plant materials, soils analysis and treatment
This experiment was conducted at the PMAS Arid Agriculture University, Rawalpindi, Pakistan. Barley seeds were obtained from the Crop Science Institute, National Agricultural Center (NARC), Islamabad, Pakistan. The experimental design was completely randomized, with three replicates. Sodium hypochlorite (5% solution) was used for seed surface sterilization, and then the seeds were washed thoroughly with distilled water two to three times. The sterilized seeds were planted in pots containing soil collected from the botanical garden of the university. The soils were analyzed before and after the experiment using a protocol following the work of Akhtar et al. (2021). This included a suspension of water and soil at a 1:1 ratio, which was stirred and retained for 28 min. One hour later, the pH of the solution was measured using a pH meter.
To determine the total soil organic matter (SOM), 1.2 g of soil was mixed with K2Cr2O7 (10 mL) and 15 mL H2SO4 (concentrated). After 40 min, 250 mL of deionized water was added along with 8 mL of H3PO3 and 14 drops of phenolphthalein indicator for color (Ullah et al., 2020). Total nitrogen (N) was determined using the salicylic acid method. Total potassium (K) was determined by mixing 3 g of soil with 30 mL of ammonium acetate and shaking the mixture shaken for 5–8 min. The mixture was then centrifuged at 12000 rpm for 5 min. The supernatant was diluted with ammonium acetate, and readings were recorded on a flame photometer. The Olsen P method was used to determine the (P) (Ullah et al., 2020; Akhtar et al., 2021). The average soil pH ranged from 7 to 7.6, electrical conductivity (EC) 45 dS·m−1, total nitrogen in the form of nitrate, and organic content 0.55 mg·kg−1, available K 45 mg·kg−1, and available P 32 mg·kg−1 (Table 1).
Biochar was extracted from an invasive plant (P. hysterophorus) collected from the Bannu district, Slaima Sikander Khail Issaki, Bannu, KPK, Pakistan. Fresh biomass (leaves and stems) of P. hysterophorus was cut into small pieces, washed two to three times with distilled water and placed in a dryer for 3–5 d. The dry biomass were placed inside a furnace (350–450 °C) for 60 min and biochar obtained using the pyrolysis method (Mondal et al., 2016; Ain et al., 2023) (Figure 1). The physicochemical properties of the prepared biochar are listed in Table 1. We used a known drought-tolerant PGPR strain (accession number KC425221, Serratia odorifera) that had already been screened in the study by Bangash et al. (2013). The bacterial strain was grown in LB media (Luria–Bertani) and incubated for 3 d at 30 °C. The optical density was measured using a spectrophotometer at 530 nm (BMS VIS), and uniform colony forming units (108 CFU/mL) were obtained for seed inoculation (Ullah et al., 2020).
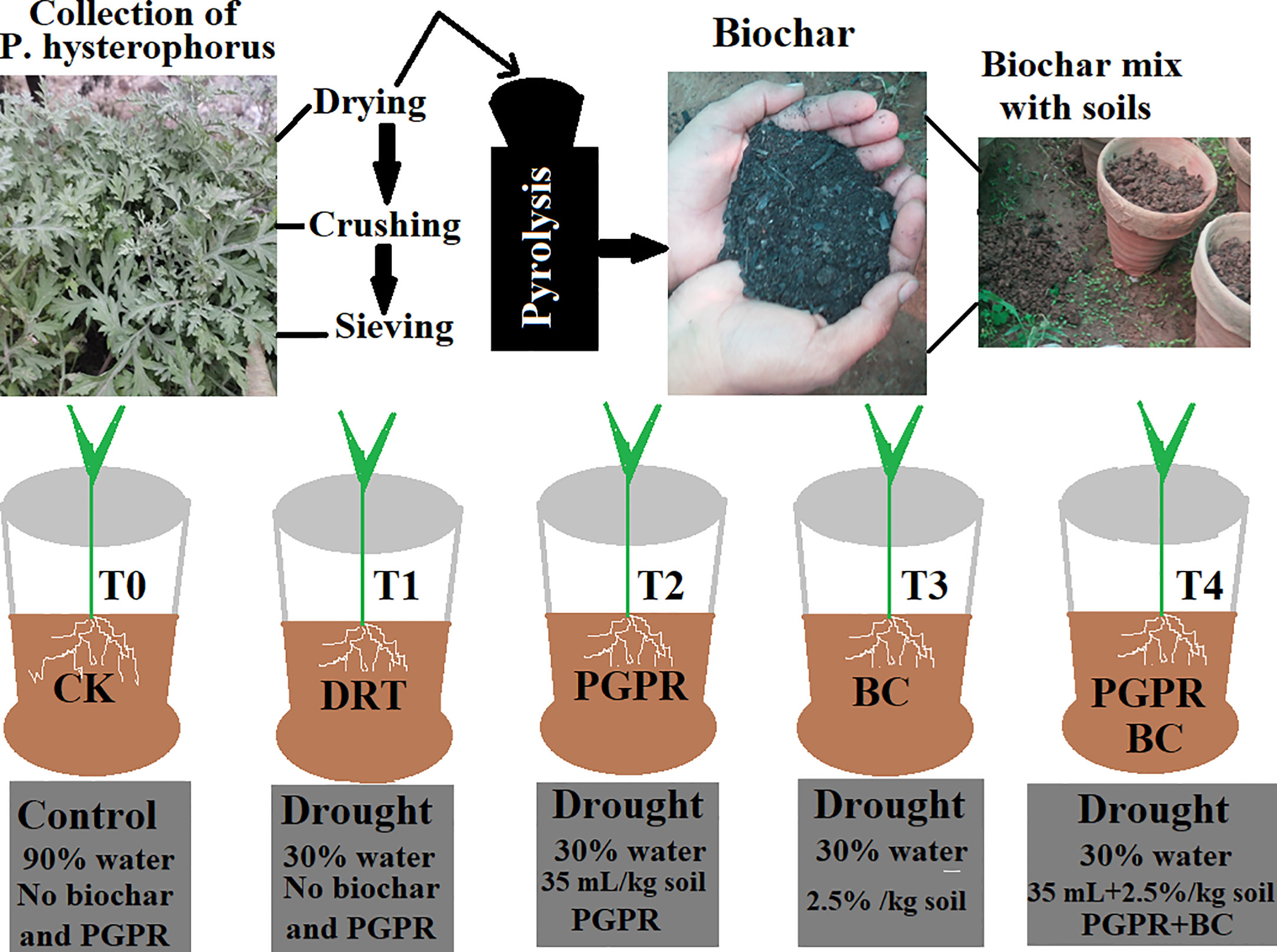
Figure 1 Schematic diagram for preparation of biochar from P. hysterophorus by pyrolysis method and detailed experimental design with treatments and control. T0 indicate control, T1 (only drought), T2 (drought and PGPR), T3 (drought and biochar) and T4 (drought combined with biochar and PGPR).
2.2 Study design
Five treatments were used to test the effects of PGPR and BC application on the barley seedlings. A total of 15 pots were used in this study. Three pots were used for each treatment and every pot was filled with 4 Kg soil. 4 kg soil mixed with 2.5% (100 g) ground biochar, and the PGPR strain was added during the inoculum method 35 mL/kg soil (108 CFU/mL), excluding the control and drought stress treatments. Barley seeds (15–20) were sown in each pot, with three replicates per treatment. Plants were watered with tap water and a standard NPK solution was applied as a fertilizer (Hafez et al., 2020). One month later, barley plants were subjected to drought conditions (30% water) for two weeks, except the three control pots (90% water) (Della Torre et al., 2021; Khan et al., 2021). The growth condition of the greenhouse were maintained at 20–25 °C, relative humidity 45–50%, with a controlled light environment at 14/10 h (Akhtar et al., 2021).
The experimental design consisted of 5 treatments × 3 replicates = 15 pots. The different treatments were organized as follows (Figure 1) (Tanveer et al., 2023). T0, control (no PGPR or BC); T1 = Drought (no PGPR or BC); T2, 35 mL PGPR/kg soil only; T3, 2.5% BC only; T4, 2.5% BC + 35 mL PGPR/kg soil. After treatment, all the plants were photographed, harvested, and stored at −80 °C for further analysis.
2.3 Growth and reproductive traits
For the seed germination assay, the germination percentage of barley plants was calculated using the following equation:
Different morphological parameters were measured for each plant, including the root length, shoot length, whole-plant fresh biomass, and whole-plant dry biomass (Rono et al., 2021).
2.4 Measurement of electrical conductivity
To measure the membrane stability index (MSI), leaf discs of each plant were placed in 10 mL of distilled water and heated in a water bath at 40 °C for 30 min. The electrical conductivity (EC) was measured for the same sample after being heated to 100 °C for 10 min (C1). The EC was then measured after the sample had been heated (C2). The MSI of each plant was calculated using the following formula (Khan et al., 2021):
2.5 Assessing the total chlorophyll content
Chlorophyll a, chlorophyll b, and total chlorophyll were assessed for each plant using the method described by Khan et al. (2020) and optimized by Arnon et al. (1949), with slight modifications. Fresh leaves were ground in 10 mL of 100% acetone and were then filtered using Whatman paper to collect the filter extract in a separate test tube. The filtrates were retained at 28 °C for 2–3 d in the dark and the absorbance were checked at 645 nm and 663 nm wavelengths respectively in the spectrophotometer (Thermo Scientific, EVO 60, Germany).
Chlorophyll a, chlorophyll b, and total chlorophyll were determined using the following formula (Khan et al., 2020):
2.6 Antioxidant enzyme activity
To examine the antioxidant enzyme activity for each plant treatment, we followed the protocol of Khattak et al. (2022) with slight modifications. Fresh barley leaves (0.4 g) from each treatment were ground with Tris buffer (NaH2PO4) and Na2HPO4 (pH 7) and mixed well before being placed in the centrifuge at 13000 rpm for 17 min at 4 °C. The pellets were removed and the supernatant was then used for enzyme extraction for various assays (POD, CAT, and SOD). The peroxidase (POD) activity was determined spectrophotometrically (Thermo Scientific, EVO 60) at 470 nm, with reaction mixture (1 mL 0.3% H2O2, 0.95 mL 0.2% guaiacol, 1 mL 50 mM PBS and 100100 µL). The enzyme activity was recorded every 30 s and at least six readings were obtained. The catalase (CAT) activity was assessed as described by Akhtar et al. (2021) with slight modifications. The reaction mixture contained 0.3% H202, 1.9 mL water, and 100 µL enzyme extract mixed together and recorded at 240 nm. For superoxide dismutase (SOD) activity, the protocol of Zulfiqar et al. (2022) was used. The reaction mixture contained 3 mL including PBS buffer (50 mM), nito-blue tetra azolium (70 µM), and methionine (13 mM) was mixed with 0.1 µM (EDTA) + 0.1 mL enzyme extract to form a solution. The absorption was checked at 560 nm using a spectrophotometer (Thermo Scientific, EVO 60).
2.7 Statistical analysis
Statistical analysis of the soil physicochemicals were analyzed using one-way ANOVA with Tukey’s test (p< 0.05) (IBMS, Amos, 21). The student’s t-test (p< 0.05) was used for pairwise comparisons among treatments with the control. All experimental data were obtained in triplicates. Significant differences at different p values (p< 0.05, one asterisk; p< 0.01 indicate double asterisk), standard deviations, and mean separations were conducted. Origin Pro 8 was used to design all the figures in this study.
3 Results
3.1 Physical and chemical properties of post harvested soils
The physical and chemical properties of the soils changed in response to the addition of BC, PGPR, or their combination. The pH and electrical conductivity (EC) of the soils increased with the addition of BC and PGPR (Table 2). All the soil nutrients, that is, total N, total P, and total K, significantly increased in the soils treated with BC alone, PGPR alone, and the combination of PGPR and BC. However, these essential nutrients decreased under drought conditions compared with the control (Table 2). The total organic matter (OM) of the post-harvested soils also showed a similar trend, because it significantly increased with the addition of biochar and PGPR but decreased under drought stress alone.
3.2 Effects of PGPR, biochar, and drought on growth and reproductive traits in barley
The growth of barley significantly increased with the amendment of PGPR, BC, and combined BC + PGPR compared to the control. However, it significantly decreased under drought alone (Figure 2A). Shoot length in barley was significantly elongated under the PGPR, BC, and PGPR + BC treatments, which was 37.03% higher in the PGPR + BC treatment than the control (Figure 2B). The shoot length of barley was significantly inhibited by drought treatment in the absence of PGPR and BC. The root length of the barley plants was substantially higher (35%) under the treatment with PGPR alone treatment compared to the control. However, there was no significant difference in the other treatments (Figure 2C).
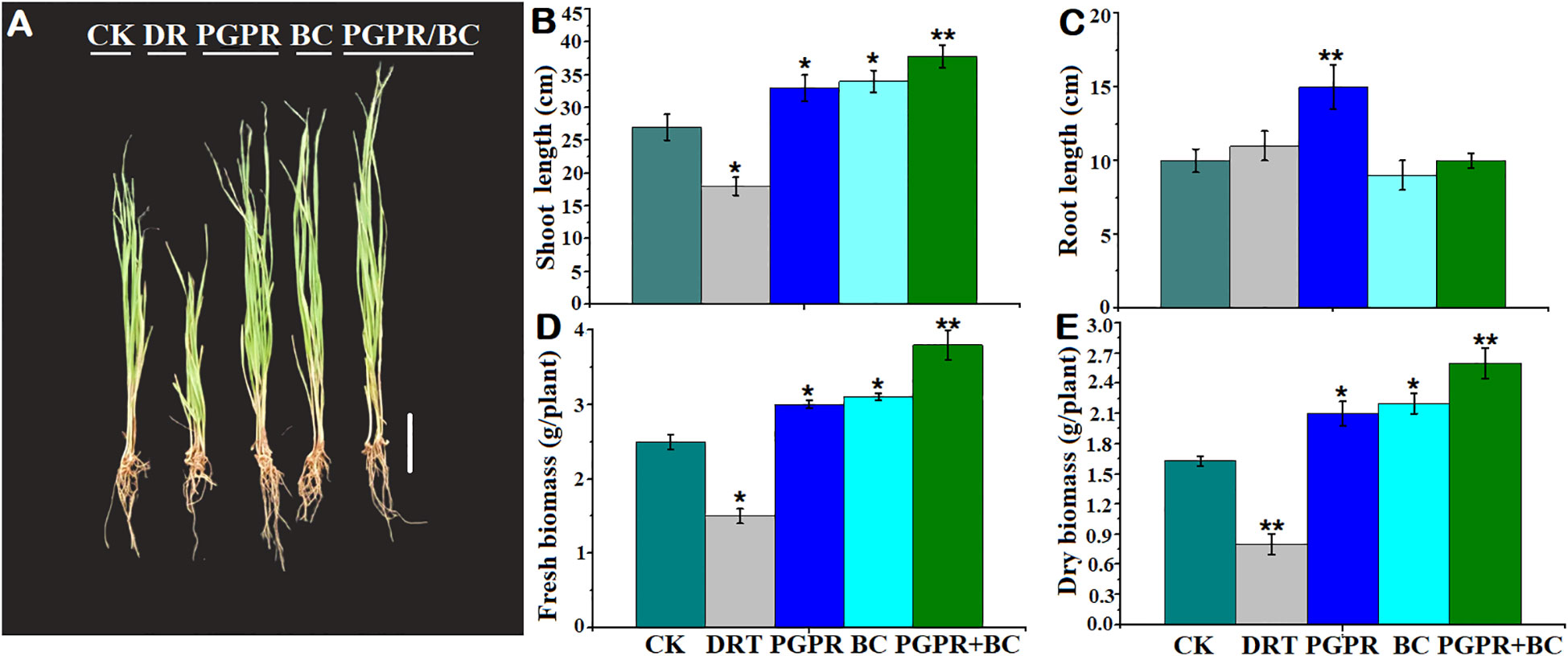
Figure 2 Growth response of barley with or without plant growth promoting rhizobacteria (PGPR), biochar (BC) and combined PGPR and BC (PGPR+BC) under drought (DRT) stress. (A) Plant phenotype, (B) Shoot length, (C) Root length, (D) Fresh biomass, (E) Dry biomass. The bars represent the standard deviation with 3 replicates. A single asterisk represents significant differences at p< 0.05, while two asterisks represent significant differences at p< 0.01 compared to the control (student t test).
Fresh biomass of the barley plants was significantly (p< 0.05) higher than the control by 20%, 24%, and 52% in the PGPR, BC, and PGPR + BC treatments, respectively. However, it decreased by 20% under drought stress alone (Figure 2D). A similar trend was observed for plant dry biomass, which was significantly higher under PGPR + BC treatment by 62.5% compared to the control. However, it decreased under the treatment with drought stress alone (Figure 2E).
The seed germination rate in the soils treated with BC was significantly higher by 42.4% (p< 0.05) that of the control (Figure 3). The second-highest seed germination rate was recorded in the BC combined with PGPR treatment (Figure 3). The seed germination rate in the PGPR alone treatment was slightly higher than that of the control (Figure 3). Under drought conditions, the seed germination rate was significantly lower than that of the control (p< 0.05, Figures 3A, B: Supplementary Data).
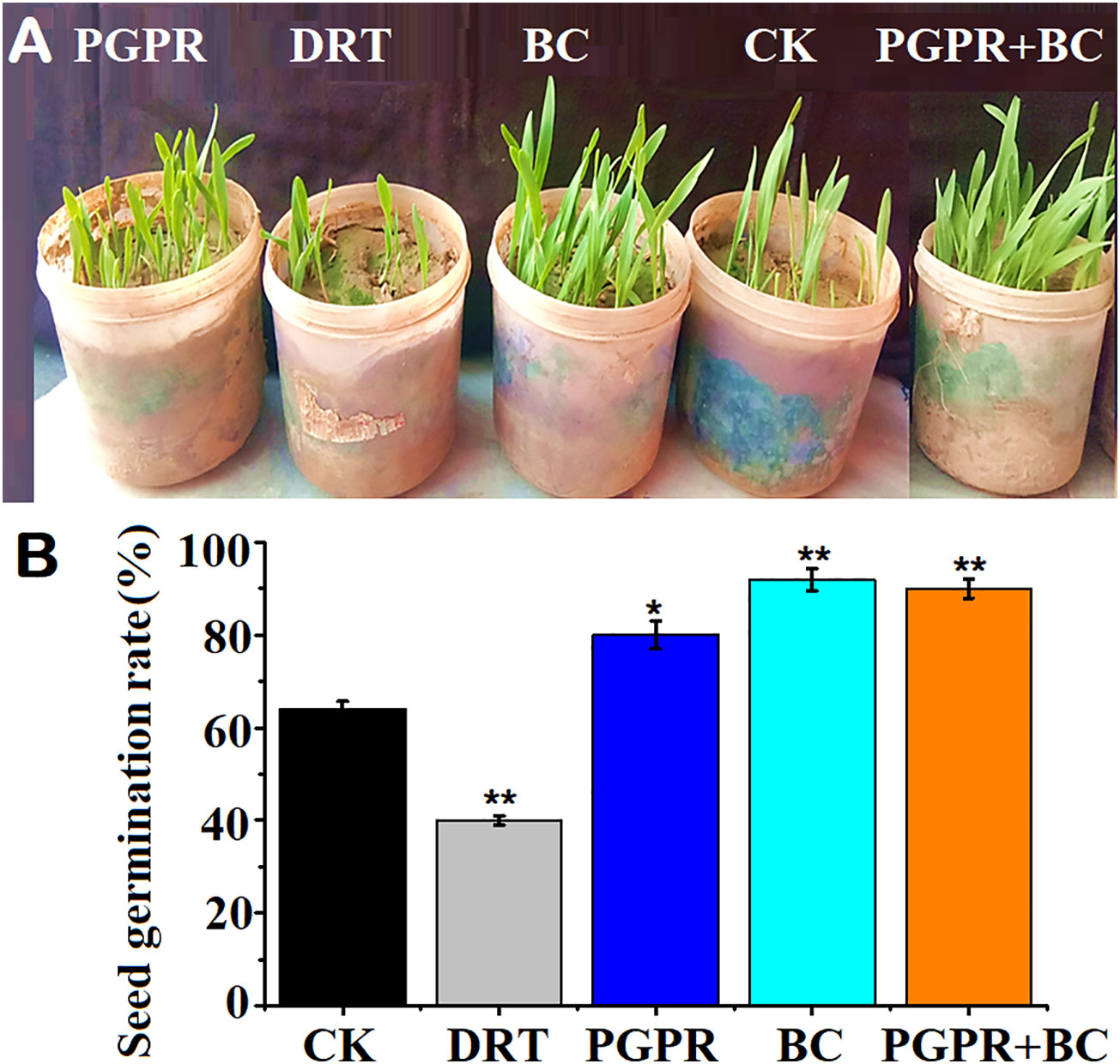
Figure 3 Seed germination assay of barley with or without plant growth promoting rhizobacteria (PGPR), biochar (BC) and PGPR+BC under drought stress. (A) Germinated seeds, (B) seed germination rates. The bars represent the standard deviation with 3 replicates. A single asterisk represents significant differences at p < 0.05, while two asterisks represent significant differences at p < 0.01 compared to the control (using student t test).
3.3 Effects of PGPR, biochar, and drought on physiological traits in barley
The chlorophyll-a (chl-a) content of the plants was significantly lower in the drought treatment than in the control. Meanwhile, the amendment of biochar and PGPR significantly enhanced chlorophyll-a in barley compared with the control (Figure 4A). A similar trend in chlorophyll b (chl-b) content was observed under the BC, PGPR, and combined BC + PGPR treatments compared to the control. However, it significantly decreased under drought stress alone (Figure 4B). The total chlorophyll content of the plants was significantly higher under the PGPR, BC, and PGPR + BC treatments than that of the control by 10.6%, 15.6%, and 31.1%, respectively. However, it decreased by 16.5% under the treatment with drought stress alone (Figure 4C).
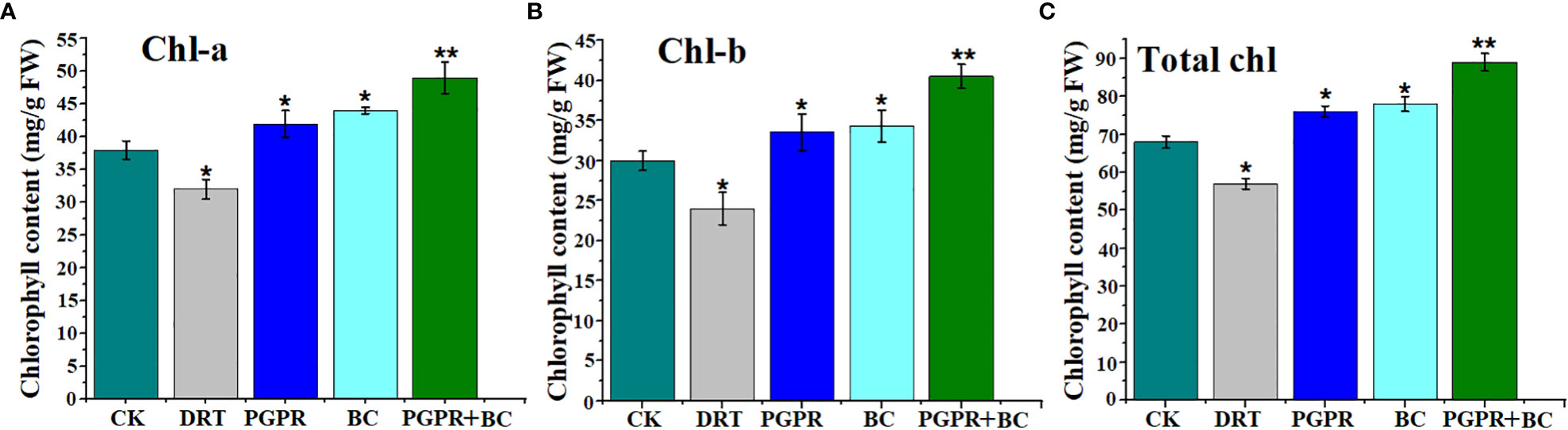
Figure 4 Chlorophyll content of barley plants with or without plant growth promoting rhizobacteria (PGPR), biochar (BC) and PGPR together with BC under drought stress. (A) Chlorophyll-a, (B) Chlorophyll-b, (C) total chlorophyll. The bars represent the standard deviation with 3 replicates. A single asterisk represents significant differences at p< 0.05, while two asterisks represent significant differences at p< 0.01 compared to the control (using student t test).
3.4 Total antioxidant activity in barley
The antioxidant enzymatic activities (POD, CAT, and SOD) of barley significantly increased in response to most treatments. However, they decreased significantly by 20%, 25%, and 25% respectively under conditions of drought stress alone compared to the control (Figures 5A–C). The highest POD, CAT, and SOD values were recorded (23.3, 100%, and 21%) in the combined BC + PGPR drought stress treatment (Figure 5). This suggests that BC and PGPR promote antioxidant enzyme activity to alleviate ROS toxicity.
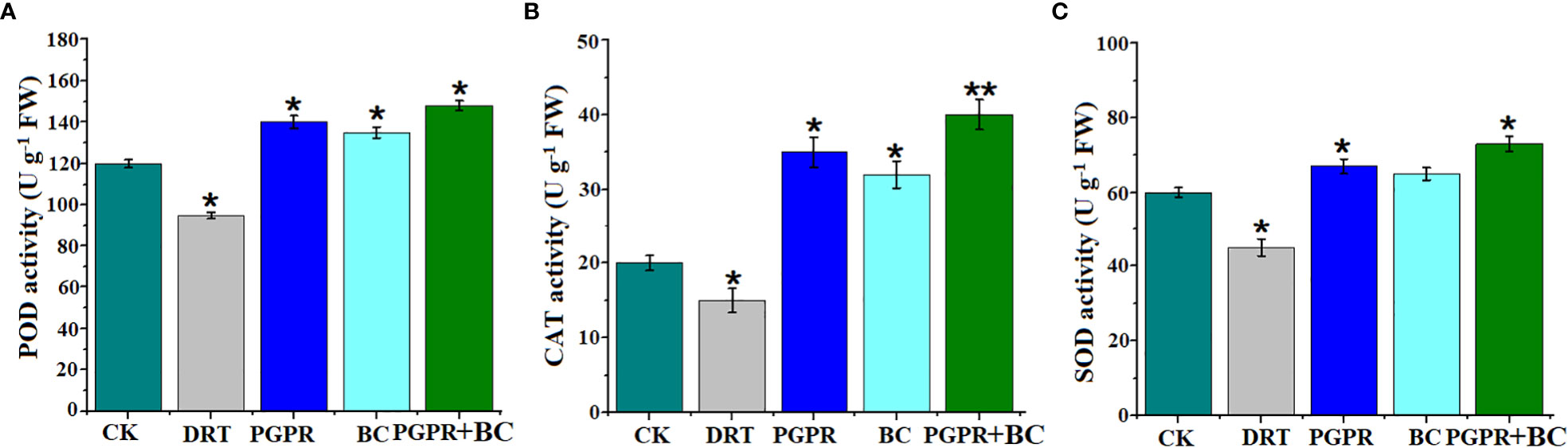
Figure 5 Antioxidant enzyme activity of barley plants in the control (well-watered), drought (alone), plant growth promoting rhizobacteria (PGPR with drought), biochar (BC with drought) and with PGPR and BC combined (with drought). (A) Peroxidase activity, (B) catalase activity, (C) superoxide dismutase activity. The bars represent the standard deviation with 3 replicates. A single asterisk represents significant differences at p< 0.05, while two asterisks represent significant differences at p< 0.01 compared to the control (using student t test).
3.5 Plant uptake activity
The uptake of nitrogen (N), potassium (K), and phosphorus (P) by barley under drought stress was examined. Soil nutrient uptake activities of barley plants were significantly enhanced under BC, PGR, and the combination of BC + PGPR (Figure 6). The highest (39.7%) total N uptake was recorded in the PGPR and BC combined treatment, whereas the lowest (7.1%) occurred under the drought stress treatment (Figure 6A). The total P content was highest under the PGPR + BC treatment (27.5%), and the second highest was observed under the BC alone treatment (23.5%). These were the only treatments higher than the control (Figure 6B). The total K uptake was highest (27%) under the BC + PGPR treatment and lowest (11.4%) under the treatment with drought stress (Figure 6C).
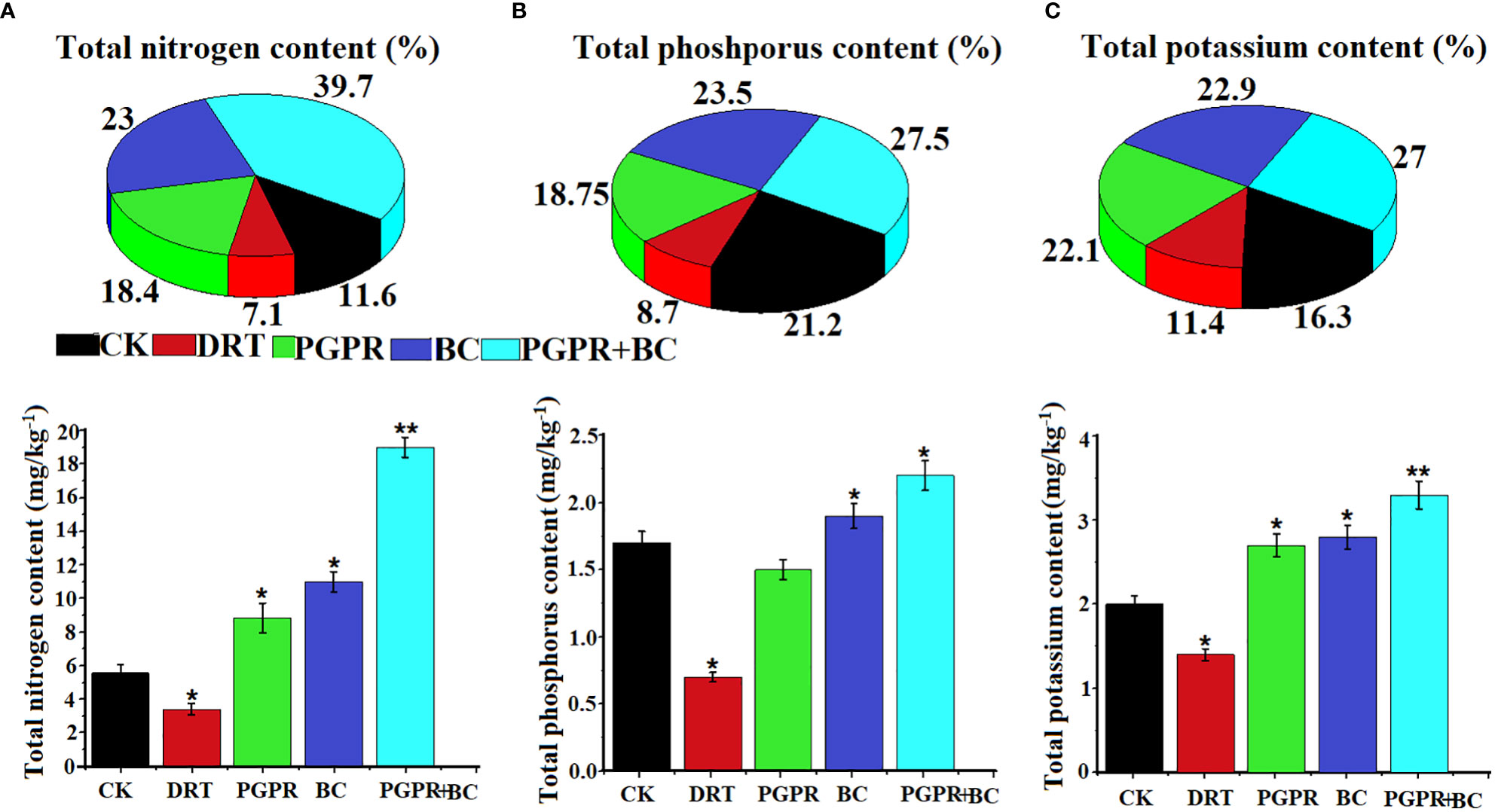
Figure 6 Nutrient uptake activity of barley plants in the control (well-watered no PGPR and BC), drought (alone), plant growth promoting rhizobacteria (PGPR with drought), biochar (BC with drought) and PGPR with BC (with drought). (A) Total nitrogen content, (B) total phosphorus content, and (C) Total potassium content. The bars represent the standard deviation with 3 replicates. A single asterisk represents significant differences among treatments at p< 0.05, while two asterisks represent significant differences at p< 0.01 compared to the control (using student t tests).
4 Discussion
The phenotypic, biological, agronomic, and physiological properties of plants are strongly affected by drought stress (Mertz-Henning et al., 2018). Water deficit is one of the global agricultural issue to restricts crop productivity and development, including plant growth, nutrient uptake, and antioxidant activity (Laird et al., 2010). Therefore, it is necessary to develop effective approaches for tolerance and resistance to drought stress. PGPR and BC activities can generally improve plant tolerance and productivity (Akhtar et al., 2021). PGPR can promote plant growth either directly or indirectly, that is, the direct mechanism of PGPR uses resource acquisition including essential minerals, ACC deaminase, auxin, nitrogen fixation, and phosphorus solubilization. Meanwhile, the indirect mechanism inhibits the function of other pathogenic organisms through biocontrol agents and cell wall degradation by enzyme production (Frampton et al., 2012). Different strains of PGPR have been proven to enhance plant growth, for example Azosprilillum, Bacillus, and Serratia (Akhtar et al., 2021: Bangash et al., 2013). BC is another strategy used for enhancing plant growth and development because it stores more water and increases nutrient uptake, which promotes the growth of the rhizosphere (Khan et al., 2021).
In the present study, we found that the combined treatment with PGPR (Serratia odorifera) and biochar (P. hysterophorus) enhanced soil organic content, total nitrogen, total phosphorus, and total electrical conductivity under drought stress. This was because BC has ash content, nutrient content, and increased holding capacity. These results have suggested that biochar and PGPR have a potential positive effect on soil fertility. Algal biochar and PGPR enhanced soil quality and organic matter, as well as total essential minerals. This is because algal BC has organic carbon that may enhance the bacterial population in the rhizosphere, which involves soil nitrification to promote total soil minerals (Ullah et al., 2020). Soil organic matter and essential minerals increase in soils because of the porous structure and ash content of the biochar, which enhanced the absorption ability of different cultivator (Yu et al., 2023). Ain et al. (2023) found that biochar from P. hysterophorus enhanced the organic matter and total nitrogen content in soils where rice plants were grown under drought and saline conditions. The potential effects of biochar on soil fertility, organic matter, and important minerals have been widely reported (Tak et al., 2013; Awad et al., 2019; Eissa, 2019).
In this study, we observed a positive correlation between PGPR and BC with increasing barley growth. The barley phenotypes were more vigorous when treated with BC, PGPR, or a combination of both compared to the control. Meanwhile, drought stress alone severely impacted the plants. The shoot length was significantly higher under the PGPR and BC treatments than under the control, which decreased the negative effects of drought stress alone (Figure 2). Therefore, it can be concluded that combining PGPR with BC significantly enhances plant growth under drought conditions. This result is in line with the findings of a previous study showing that the phenotypes of rapeseed improved when treated with biochar to promote growth under water-deficit conditions (Khan et al., 2021). Tomato plant phenotypes and shoot and root lengths significantly improved after amendment with biochar and PGPR, which significantly enhanced plant resistance to drought (Wang et al., 2021). Awad et al. (2019) highlighted that barley growth and development were enhanced after amendment with two different concentrations of biochar compared with the control treatment. In the present study, the root length was considerably longer under drought stress and PGPR treatments compared to BC. This suggested that water deficit could induce roots to grow deeper into the soils to enhance water uptake due to inoculation of the PGPR Serratia odorifera strain. This has the capability to form indole acetic acid (IAA) which is capable of increasing stimulation of amino cyclopropane carboxylic acid synthase that converts toxic ethylene to amino-cyclo-propane carboxylate (ACC). ACC may help convert the ethylene hormone to α-ketobutyrate and ammonia forms in the rhizosphere. Barley roots can become substantially elongated after decreased stimulation with ethylene (Bangash et al., 2013). BC from P. hysterophorus acts as a bodyguard for PGPR to enhance root proliferation. This provides essential nutrients and adjusts the water levels owing to its high surface area, increasing the electrolyte capacity and water-holding capacity (Hussain et al., 2021). This is supported by another study in which maize roots were elongated under drought stress when treated with PGPR and BC compared to the control (Ullah et al., 2020).
The seed germination rate of barley was significantly higher under biochar and the combination of BC and PGPR under drought stress (Saeed et al., 2022). These findings suggest that P. hysterophorus biochar would help to improve the barley plants during the early stages of seed germination under water-deficient conditions. This is because it may increase the soil essential nutrients (N, P, and K) owing to the porous and negatively charged structure to decrease soil leaching and enhance soil mineralization by directly aborting plant roots from the soil (Ain et al., 2023). It may also activate the metabolic system in the soils to activate PGPR inside the root zone. In this study, the PGPR strain may have increased the seed germination rate by increasing the production of essential growth hormones, that is, auxin and cytokinin, to enhance the production of enzyme activity. In this context, amylase can help to promote the levels of starch (Akhtar et al., 2021). The fresh and dry biomass of barley was significantly higher when treated with biochar and PGPR. Danish et al. (2020), reported the positive effects of ACC deaminase PGPR on maize for enhancement the maize development and biological response. Growth rate, chlorophyll content, and enzymatic activity of potato plants significantly increased with the inoculation of PGPR o resist the water scarcity condition (Batool et al., 2020). This is in line with the findings of the present study. Biochar from P. hysterophorus has been found to significantly improve seed germination, shoot height, and root length in maize plants (Kumar et al., 2013). This suggesting that there is some beneficial use for some invasive plant species in agriculture.
Plant physiological responses are important during abiotic stress (Khan et al., 2020) and chlorophyll levels can be used as an indicator for various abiotic stress in the plant (Wang et al., 2019). Water deficit condition, negatively affects the plant growth and physiological response (Mertz-Henning et al., 2018). However, it was previously found that the chlorophyll content in barley significantly increased under drought stress after amendment with BC (Tak et al., 2013). This is similar to the observations in the current study (Figure 4). Chlorophyll a and b were significantly higher in tomato plants following the application of PGPR (Batool et al., 2020), as well as in barley plants treated with BC (Awad et al., 2019). This suggests that BC and PGPR may benefit plant physiological responses. Rice plants have significantly enhanced chlorophyll content owing to the presence of PGPR, which promotes photosynthetic efficiency (Hafez et al., 2016). Our results have indicated that combining PGPR-tolerant strains with BC from P. hysterophorus enhances the ability of barley plants to survive under water-deficit conditions and can maintain photosynthetic pigments. The chlorophyll a, b and total chlorophyll content of the current proposed study significantly higher under BC and PGPR treatments because Chlorophyll is directly linked to the nitrogen content in the leaves. After the amendments of PGPR and BC, the photosynthetic rate in barley plants was enhanced because of increased N uptake activities from the soil via the xylem to close the stomata and prevent water loss under drought stress (Lalay et al., 2021). PGPR can prevent net photosynthetic activities by producing cytokinin hormones to close the stomata and enhance leaf cell division in the early growth stage. This then leads to an increase in the number of vascular bundles to promote leaf area (Sivasakthi et al., 2013; Hönig et al., 2018). Application of PGPR and BC may improve root growth, which, in turn, improves nutrient intake and ultimately increases photosynthetic activity.
The crop antioxidant defense system is triggered under water deficit conditions to absorb reactive oxygen radicals, which potentially affect numerous organelles in plants (Zhang et al., 2021). Antioxidant enzymes in barley plants may potentially mitigate the negative effects of drought stress by scavenging reactive oxygen species (ROS) and preventing cell damage (Gupta et al., 2015). The present findings have shown that catalase (CAT), peroxidase (POD), and superoxide dismutase (SOD) were significantly increased under drought stress following the addition of PGPR and BC. This alleviated the negative effects of ROS owing to PGPR-activated self-protective systems and the production of enzymes inside the plant (Figure 5). Our results are in line with those of other studies that found increased CAT, POD, and SOD activities under drought stress (Abid et al., 2019). This suggests that plants treated with PGPR and BC may produce ROS that protect the photosynthetic apparatus (Hafez et al., 2020). Barley plants showed decreased toxicity caused by reactive oxygen species after amendment with BC and chitosan, which boosted antioxidant enzyme activity. The findings of the current study are in line with those of Khan et al. (2021), who observed a positive role from biochar in reducing the toxic effects of drought stress supported by SOD, CAT, and POD enzyme activities.
Addition of biochar and PGPR increases the plant uptake of essential nutrients from the soil (Abdipour et al., 2019). Nutrient uptake in barley plants significantly increased after treatment with a range of biochar concentrations under drought stress (Awad et al., 2019). In the present study, we found that the uptake of total nitrogen, total potassium, and total phosphorus was significantly enhanced after amendment with biochar, PGPR, and a combination of both. This is because this PGPR strain has the capability to take up more nutrients from the soil by a direct mechanism to release the organic compound responsible for nutrient availability (Ullah et al., 2020). Therefore, PGPR and BC can enhance the uptake of essential nutrients under drought conditions. Yu et al. (2023) hypothesized that nitrogen uptake activity increases because of plant growth bacteria associated with the nitrogen cycle helping plants resist abiotic stress. In contrast, plants treated with biochar have enhanced uptake of total nitrogen, phosphorus, and potassium because they have negatively charged ions and cohesive forces that boost mineralization in soils (Laird et al., 2010). Mahmood (2022) also observed that more phosphorus was absorbed by plants treated with PGPR under high stress conditions.
5 Conclusion
The results of this study have shown that the positive role of the PGPR drought strain (Serratia odorifera) and BC from P. hysterophorus mitigated the negative effects of drought stress by improving plant growth, plant biomass, and seed germination. The combination of PGPR and BC significantly enhanced chlorophyll content and activated the antioxidant defense system in barley to resist drought stress. Furthermore, they improved the uptake of essential nutrients, that is, N, P, and K, as well as soil organic matter. Therefore, biochar and PGPR could play a key role in alleviating the negative effects of abiotic stress on agricultural crops. From this perspective, the application of BC and PGPR to other plants under different stress conditions should also be explored.
Data availability statement
The original contributions presented in the study are included in the article/Supplementary Material. Further inquiries can be directed to the corresponding authors.
Author contributions
Conceptualization: IK, FG, GL and D-LD. Funding acquisition: GL and D-LD. Data curation, investigation, and writing—original draft: FG, IK and SR. Methodology: IK. Writing —review and editing: SR and Z-CD. All authors contributed to the article and approved the submitted version.
Funding
This work was supported by the Open Project of Key Laboratory of Environmental Biotechnology, CAS (Grant No kf2020006), the Carbon Peak and Carbon Neutrality Technology Innovation Foundation of Jiangsu Province (BK20220030), the National Natural Science Foundation of China (32271587; 31800342), the Talent Project from the “Double-Entrepreneurial Plan” in Jiangsu Province, and the Senior Talent Foundation of Jiangsu University (18JDG039).
Conflict of interest
The authors declare that the research was conducted in the absence of any commercial or financial relationships that could be construed as a potential conflict of interest.
Publisher’s note
All claims expressed in this article are solely those of the authors and do not necessarily represent those of their affiliated organizations, or those of the publisher, the editors and the reviewers. Any product that may be evaluated in this article, or claim that may be made by its manufacturer, is not guaranteed or endorsed by the publisher.
Supplementary material
The Supplementary Material for this article can be found online at: https://www.frontiersin.org/articles/10.3389/fpls.2023.1175097/full#supplementary-material
References
Abdipour, M., Hosseinifarahi, M., Najafian, S. (2019). Effects of humic acid and cow manure biochar (CMB) in culture medium on growth and mineral concentrations of basil plant. Int. J. Hortic. Sci. Technol. 6, 27–38. doi: 10.22059/ijhst.2019.279022.287
Abid, R., Manzoor, M., De Oliveira, L. M., da Silva, E., Rathinasabapathi, B., Rensing, C., et al. (2019). Interactive effects of as, cd and zn on their uptake and oxidative stress in as-hyperaccumulator pteris vittata. Environ. pollut. 248, 756–762. doi: 10.1016/j.envpol.2019.02.054
Ahmad, A., Khan, W. U., Ali Shah, A., Yasin, N. A., Naz, S., Ali, A., et al. (2021). Synergistic effects of nitric oxide and silicon on promoting plant growth, oxidative stress tolerance and reduction of arsenic uptake in brassica juncea. Chemosphere 262, 128384. doi: 10.1016/j.chemosphere.2020.128384
Ain, Q. U., Shafiq, M., Nazir, A., Bareen, F. E. (2023). Fertilizer perspective of biochar and compost derived from biomass of parthenium hysterophorus in the rice wheat system in punjab, Pakistan. J. Plant Nutr. 46 (10), 2434–2450. doi: 10.1080/01904167.2022.2155555
Akhtar, S. S., Andersen, M. N., Naveed, M., Zahir, Z. A., Liu, F. (2015). Interactive effect of biochar and plant growth-promoting bacterial endophytes on ameliorating salinity stress in maize. Funct. Plant Biol. 42 (8), 770–781. doi: 10.1071/FP15054
Akhtar, N., Ilyas, N., Mashwani, Z.-u., Hayat, R., Yasmin, H., Noureldeen, A., et al. (2021). Synergistic effects of plant growth promoting rhizobacteria and silicon dioxide nano-particles for amelioration of drought stress in wheat. Plant Physiol. Biochem. 166, 160–176. doi: 10.1016/j.plaphy.2021.05.039
Arnon, D. I. (1949). Copper enzymes in isolated chloroplasts. polyphenoloxidase in beta vulgaris. Plant Physiol. 24 (1), 1. doi: 10.1104/pp.24.1.1
Ashoub, A., Baeumlisberger, M., Neupaertl, M., Karas, M., Brüggemann, W. (2015). Characterization of common and distinctive adjustments of wild barley leaf proteome under drought acclimation, heat stress and their combination. Plant Mol. Biol. 87, 459–471. doi: 10.1007/s11103-015-0291-4
Awad, M., Rekaby, S., Hegab, S., Eissa, M. (2019). Effect of biochar application on barley plants grown on calcareous sandy soils irrigated by saline water. Sci. J. Agric. Sci. 1 (2), 52–61. doi: 10.21608/sjas.2019.18618.1006
Bangash, N., Khalid, A., Mahmood, T., Siddique, M. T. (2013). Screening rhizobacteria containing ACC-deaminase for growth promotion of wheat under water stress. Pakistan J. Bot. 45, 91–96.
Batool, T., Ali, S., Seleiman, M. F., Naveed, N. H., Ali, A., Ahmed, K., et al. (2020). Plant growth promoting rhizobacteria alleviates drought stress in potato in response to suppressive oxidative stress and antioxidant enzymes activities. Sci. Rep. 10, 1–19. doi: 10.1038/s41598-020-73489-z
Çakmakçi, R., Dönmez, M. F., Erdoğan, Ü. (2007). The effect of plant growth promoting rhizobacteria on barley seedling growth, nutrient uptake, some soil properties, and bacterial counts. Turkish J. Agric. For. 31, 189–199. doi: 10.3906/tar-0701-8
Cardinale, M., Ratering, S., Suarez, C., Zapata Montoya, A. M., Geissler-Plaum, R., Schnell, S. (2015). Paradox of plant growth promotion potential of rhizobacteria and their actual promotion effect on growth of barley (Hordeum vulgare l.) under salt stress. Microbiol. Res. 181, 22–32. doi: 10.1016/j.micres.2015.08.002
Danish, S., Zafar-Ul-Hye, M., Hussain, S., Riaz, M., Qayyum, M. F. (2020). Mitigation of drought stress in maize through inoculation with drought tolerant ACC deaminase containing PGPR under axenic conditions. Pakistan J. Bot. 52, 49–60. doi: 10.30848/PJB2020-1(7
Della Torre, F., Ferreira, B. G., Lima, J. E., Lemos-Filho, J. P., Rossiello, R. O., França, M. G. (2021). Leaf morphophysiological changes induced by long-term drought in jatropha curcas plants explain the resilience to extreme drought. J. Arid. Environments 185, 104381. doi: 10.1016/j.jaridenv.2020.104381
Dempster, D. N., Jones, D. L., Murphy, D. V. (2012). Clay and biochar amendments decreased inorganic but not dissolved organic nitrogen leaching in soil. Arid. Soil Res. Rehabil. 50, 216–221. doi: 10.1071/SR11316
Eissa, M. A. (2019). Effect of cow manure biochar on heavy metals uptake and translocation by zucchini (Cucurbita pepo l). Arab. J. Geosci. 12, 1–10. doi: 10.1007/s12517-018-4191-1
Elakhdar, A., Solanki, S., Kubo, T., Abed, A., Elakhdar, I., Khedr, R., et al. (2022). Barley with improved drought tolerance: challenges and perspectives. Environ. Exp. Bot. 201, 104965. doi: 10.1016/j.envexpbot.2022.104965
El-Hashash, E. F., El-Absy, K. M. (2019). Barley (Hordeum vulgare l.) breeding. Adv. Plant Breed. Strat.: Cereals 5, 1–45. doi: 10.1007/978-3-030-23108-8_1
El-Naggar, A. H., Usman, A. R. A., Al-Omran, A., Ok, Y. S., Ahmad, M., Al-Wabel, M. I. (2015). Carbon mineralization and nutrient availability in calcareous sandy soils amended with woody waste biochar. Chemosphere 138, 67–73. doi: 10.1016/j.chemosphere.2015.05.052
Farooq, S., Hussain, M., Jabran, K., Hassan, W., Rizwan, M. S., Yasir, T. A. (2017). Osmopriming with CaCl2 improves wheat (Triticum aestivum L.) production under water-limited environments. Environ. Sci. Pollut. Res. 24, 13638–13649. doi: 10.1007/s11356-017-8957
Farooq, M., Romdhane, L., Rehman, A., Al-Alawi, A. K. M., Al-Busaidi, W. M., Asad, S. A., et al (2021). Integration of seed priming and biochar application improves drought tolerance in cowpea. J. Plant Growth Regul. 40, 1972–1980. doi: 10.1007/s00344-020-10245-7
Feiziasl, V. (2022). Assessment of water status and its critical stages in dryland barley genotypes (Hordeum vulgare) using crop water stress index (CWSI). J. Water Soil 36, 197–213. doi: 10.22067/JSW.2022.75527.1146
Feng, D., Guo, D., Zhang, Y., Sun, S., Zhao, Y., Shang, Q., et al. (2021). Functionalized construction of biochar with hierarchical pore structures and surface O-/N-containing groups for phenol adsorption. Chem. Eng. J. 410, 127707. doi: 10.1016/j.cej.2020.127707
Forni, C., Duca, D., Glick, B. R. (2017). Mechanisms of plant response to salt and drought stress and their alteration by rhizobacteria. Plant Soil 410, 335–356. doi: 10.1007/s11104-016-3007-x
Frampton, R. A., Pitman, A. R., Fineran, P. C. (2012). Advances in bacteriophage-mediated control of plant pathogens. Int. J. Microbiol. 2012, 11. doi: 10.1155/2012/326452
Gordon, R., Chapman, J., Power, A., Chandra, S., Roberts, J., Cozzolino, D. (2019). Mid-infrared spectroscopy coupled with chemometrics to identify spectral variability in Australian barley samples from different production regions. J. Cereal Sci. 85, 41–47. doi: 10.1016/j.jcs.2018.11.004
Gouda, S., Kerry, R. G., Das, G., Paramithiotis, S., Shin, H. S., Patra, J. K. (2018). Revitalization of plant growth promoting rhizobacteria for sustainable development in agriculture. Microbiol. Res. 206, 131–140. doi: 10.1016/j.micres.2017.08.016
Gupta, D. K., Palma, J. M., Corpas, F. J. (2015). Reactive oxygen species and oxidative damage in plants under stress. (Heidelberg: Springer), 1–22. doi: 10.1007/978-3-319-20421-5
Hafez, Y. M., Abdelaal, K. A. A., Eid, M. E., Mehiar, F. F. (2016). Morpho-physiological and biochemical responses of barley plants (Hordeum vulgare l.) against barley net blotch disease with application of non-traditional compounds and fungicides. Egypt. J. Biol. Pest Control 26, 261–268.
Hafez, Y., Attia, K., Alamery, S., Ghazy, A., Al-Doss, A., Ibrahim, E., et al. (2020). Beneficial effects of biochar and chitosan on antioxidative capacity, osmolytes accumulation, and anatomical characters of water-stressed barley plants. Agronomy 10, 1–18. doi: 10.3390/agronomy10050630
Hönig, M., Plíhalová, L., Husičková, A., Nisler, J., Doležal, K. (2018). Role of cytokinins in senescence, antioxidant defence and photosynthesis. Int. J. Mol. Sci. 19 (12), 4045. doi: 10.3390/ijms19124045
Hussain, S., Mumtaz, M., Manzoor, S. (2021). Foliar application of silicon improves growth of soybean by enhancing carbon metabolism under shading conditions. Plant Physiol. Biochem. 159, 43–52. doi: 10.1016/j.plaphy.2020.11.053
Jaleel, C. A., Manivannan, P., Wahid, A., Farooq, M., Al-Juburi, H. J., Somasundaram, R., et al. (2009). Drought stress in plants: a review on morphological characteristics and pigments composition. Int. J. Agric. Biol. 11, 100–105. doi. 08–305/IGC-DYT/2009/11–1–100–105
Javaid, A. (2010). Herbicidal potential of allelopathic plants and fungi against parthenium hysterophorus - a review. Allelopath. J. 25, 331–344.
Kasim, W. A., Gaafar, R. M., Abou-Ali, R. M., Omar, M. N., Hewait, H. M. (2016). Effect of biofilm forming plant growth promoting rhizobacteria on salinity tolerance in barley. Ann. Agric. Sci. 61, 217–227. doi: 10.1016/j.aoas.2016.07.003
Khan, Z., Khan, M. N., Zhang, K., Luo, T., Zhu, K., Hu, L. (2021). The application of biochar alleviated the adverse effects of drought on the growth, physiology, yield and quality of rapeseed through regulation of soil status and nutrients availability. Ind. Crops Prod. 171, 113878. doi: 10.1016/j.indcrop.2021.113878
Khan, H., Marwat, K. B., Hassan, G., Khan, M. A. (2012). Chemical control of parthenium hysterophorus l. at different growth stages in non-cropped area. Pakistan J. Bot. 44, 1721–1726.
Khan, I. U., Rono, J. K., Liu, X. S., Feng, S. J., Li, H., Chen, X., et al. (2020). Functional characterization of a new metallochaperone for reducing cadmium concentration in rice crop. J. Clean. Prod. 272, 123152. doi: 10.1016/j.jclepro.2020.123152
Khattak, W. A., He, J., Abdalmegeed, D., Hu, W., Wang, Y., Zhou, Z. (2022). Foliar melatonin stimulates cotton boll distribution characteristics by modifying leaf sugar metabolism and antioxidant activities during drought conditions. Physiol. Plant 174, e13526. doi: 10.1111/ppl.13526
Kilic, H. (2014). Additive main effects and multiplicative interactions (AMMI) analysis of grain yield in barley genotypes across environments. J. Agric. Sci. 20 (4), 337–344. doi: 10.1501/Tarimbil_0000001292
Kumar, S., Masto, R. E., Ram, L. C., Sarkar, P., George, J., Selvi, V. A. (2013). Biochar preparation from parthenium hysterophorus and its potential use in soil application. Ecol. Eng. 55, 67–72. doi: 10.1016/j.ecoleng.2013.02.011
Laird, D. A., Fleming, P., Davis, D. D., Horton, R., Wang, B., Karlen, D. L. (2010). Impact of biochar amendments on the quality of a typical Midwestern agricultural soil. Geoderma 158, 443–449. doi: 10.1016/j.geoderma.2010.05.013
Lalay, G., Ullah, S., Ahmed, I. (2022). Physiological and biochemical responses of Brassica napus L. to drought‐induced stress by the application of biochar and Plant Growth Promoting Rhizobacteria. Micros. Res. Tech. 85 (4), 1267–1281. doi: 10.1002/jemt.23993
Mahmood, Q. (2022). Sustainable plant nutrition under contaminated environments. Springer International Publishing AG. doi: 10.1007/978-3-030-91499-8
Masulili, A., Utomo, W. H., MS, S. (2010). Rice husk biochar for rice based cropping system in acid soil 1. the characteristics of rice husk biochar and its influence on the properties of acid sulfate soils and rice growth in West kalimantan, Indonesia. J. Agric. Sci. 2, 39. doi: 10.5539/jas.v2n1p39
Mertz-Henning, L. M., Ferreira, L. C., Henning, F. A., Mandarino, J. M. G., Santos, E. D., Oliveira, M. C. N. D., et al. (2018). Effect of water deficit-induced at vegetative and reproductive stages on protein and oil content in soybean grains. Agronomy 8, 3. doi: 10.3390/agronomy8010003
Mondal, S., Aikat, K., Halder, G. (2016). Biosorptive uptake of ibuprofen by chemically modified parthenium hysterophorus derived biochar: equilibrium, kinetics, thermodynamics and modeling. Ecol. Eng. 92, 158–172. doi: 10.1016/j.ecoleng.2016.03.022
Newton, A. C., Flavell, A. J., George, T. S., Leat, P., Mullholland, B., Ramsay, L., et al. (2011). Crops that feed the world 4. barley: a resilient crop? strengths and weaknesses in the context of food security. Food Secur. 3, 141–178. doi: 10.1007/s12571-011-0126-3
Pérez-Cabrera, C. A., Juárez-López, P., Anzaldo-Hernández, J., Alia-Tejacal, I., Valdez-Aguilar, L. A., Alejo-Santiago, G., et al. (2022). Biochar from sugarcane apices as a soil conditioner for greenhouse cultivation of ocimum basilicum var. thyrsif lora. Terra Latinoam. 40, 1–8. doi: 10.28940/terra.v40i0.1077
Rono, J. K., Le Wang, L., Wu, X. C., Cao, H. W., Zhao, Y. N., Khan, I. U., et al. (2021). Identification of a new function of metallothionein-like gene OsMT1e for cadmium detoxification and potential phytoremediation. Chemosphere 265, 129–136. doi: 10.1016/j.chemosphere.2020.129136
Rout, M. E., Callaway, R. M. (2012). Interactions between exotic invasive plants and soil microbes in the rhizosphere suggest that “everything is not everywhere”. Ann. Bot. 110, 213–222. doi: 10.1093/aob/mcs061
Saeed, M., Ilyas, N., Jayachandran, K., Shabir, S., Akhtar, N., Shahzad, A., et al. (2022). Advances in biochar and PGPR engineering system for hydrocarbon degradation: a promising strategy for environmental remediation. Environ. pollut. 305, 119282. doi: 10.1016/j.envpol.2022.119282
Sánchez-Reinoso, A. D., Ávila-Pedraza, E. Á., Restrepo-Díaz, H. (2020). Use of biochar in agriculture. Acta Biol. Colomb. 25, 327–338. doi: 10.15446/abc.v25n2.79466
Santoyo, G., Urtis-Flores, C. A., Loeza-Lara, P. D., Orozco-Mosqueda, M. D. C., Glick, B. R. (2021). Rhizosphere colonization determinants by plant growth-promoting rhizobacteria (Pgpr). Biol. (Basel). 10, 1–18. doi: 10.3390/biology10060475
Scheitrum, D., Schaefer, K. A., Nes, K. (2020). Realized and potential global production effects from genetic engineering. Food Pol. 93, 101882. doi: 10.1016/j.foodpol.2020.101882
Schmidt, H. P., Kammann, C., Niggli, C., Evangelou, M. W. H., Mackie, K. A., Abiven, S. (2014). Biochar and biochar-compost as soil amendments to a vineyard soil: influences on plant growth, nutrient uptake, plant health and grape quality. Agric. Ecosyst. Environ. 191, 117–123. doi: 10.1016/j.agee.2014.04.001
Sivasakthi, S., Kanchana, D., Usharani, G., Saranraj, P. (2013). Production of plant growth promoting substance by pseudomonas fluorescens and bacillus subtilis isolates from paddy rhizosphere soil of cuddalore district, Tamil nadu, India. Int. J. Microbiol. Res. 4, 227–233. doi: 10.5829/idosi.ijmr.2013.4.3.75171
Suliman, W., Harsh, J. B., Abu-Lail, N. I., Fortuna, A. M., Dallmeyer, I. (2017). The role of biochar porosity and surface functionality in augmenting hydrologic properties of a sandy soil. Sci. Total Environ. 574, 139–147. doi: 10.1016/j.scitotenv.2016.09.025
Tak, H. I., Ahmad, F., Babalola, O. O. (2013). Advances in the application of plant growth-promoting rhizobacteria in phytoremediation of heavy metals. Rev. Environ. Contam. Toxicol. 223, 33–52. doi: 10.1007/978-1-4614-5577-6_2
Tanveer, S., Akhtar, N., Ilyas, N., Sayyed, R. Z., Fitriatin, B. N., Perveen, K., et al. (2023). Interactive effects of pseudomonas putida and salicylic acid for mitigating drought tolerance in canola (Brassica napus l.). Heliyon 9, e14193. doi: 10.1016/j.heliyon.2023.e14193
Tefera, W., Seifu, W., Tian, S. (2022). Coconut shell-derived biochar and oyster shell powder alter rhizosphere soil biochemical properties and cd uptake of rice (Oryza sativa l.). Int. J. Environ. Sci. Technol. doi: 10.1007/s13762-022-04658-y
Ullah, N., Ditta, A., Khalid, A., Mehmood, S., Rizwan, M. S., Ashraf, M., et al. (2020). Integrated effect of algal biochar and plant growth promoting rhizobacteria on physiology and growth of maize under deficit irrigations. J. Soil Sci. Plant Nutr. 20, 346–356. doi: 10.1007/s42729-019-00112-0
Wang, Y., Li, W., Du, B., Li, H. (2021). Effect of biochar applied with plant growth-promoting rhizobacteria (PGPR) on soil microbial community composition and nitrogen utilization in tomato. Pedosphere 31, 872–881. doi: 10.1016/S1002-0160(21)60030-9
Wang, J., Wang, H., Chen, J., Zhang, S., Xu, J., Han, X., et al. (2019). Xylem development, cadmium bioconcentration, and antioxidant defense in populus × euramericana stems under combined conditions of nitrogen and cadmium. Environ. Exp. Bot. 164, 1–9. doi: 10.1016/j.envexpbot.2019.04.014
Wiegmann, M., Maurer, A., Pham, A., March, T. J., Al-Abdallat, A., Thomas, W. T. B., et al. (2019). Barley yield formation under abiotic stress depends on the interplay between flowering time genes and environmental cues. Sci. Rep. 9, 1–16. doi: 10.1038/s41598-019-42673-1
Yu, Y. Y., Xu, J., Gao, M. Z., Huang, T. X., Zheng, Y., Zhang, Y. Y., et al. (2023). Exploring plant growth promoting rhizobacteria potential for green agriculture system to optimize sweet potato productivity and soil sustainability in northern jiangsu, China. Eur. J. Agron. 142, 126661. doi: 10.1016/j.eja.2022.126661
Zhang, W., Pan, X., Zhao, Q., Zhao, T. (2021). Plant growth, antioxidative enzyme, and cadmium tolerance responses to cadmium stress in canna orchioides. Hortic. Plant J. 7, 256–266. doi: 10.1016/j.hpj.2021.03.003
Zulfiqar, B., Raza, M. A.S., Saleem, M. F., Aslam, M. U., Iqbal, R., Muhammad, F., et al. (2022). Biochar enhances wheat crop productivity by mitigating the effects of drought: Insights into physiological and antioxidant defense mechanisms. Plos One 17 (4), e0267819. doi: 10.1371/journal.pone.0267819
Keywords: tolerance, physicochemical, water stress, seed germination, soil nutrients
Citation: Gul F, Khan IU, Rutherford S, Dai Z-C, Li G and Du D-L (2023) Plant growth promoting rhizobacteria and biochar production from Parthenium hysterophorus enhance seed germination and productivity in barley under drought stress. Front. Plant Sci. 14:1175097. doi: 10.3389/fpls.2023.1175097
Received: 27 February 2023; Accepted: 15 May 2023;
Published: 08 June 2023.
Edited by:
Saqib Bilal, University of Nizwa, OmanReviewed by:
Fahim Nawaz, Muhammad Nawaz Shareef University of Agriculture, PakistanSalman Khan, Southern University of Science and Technology, China
Sadiq Naveed, Peking University, China
Copyright © 2023 Gul, Khan, Rutherford, Dai, Li and Du. This is an open-access article distributed under the terms of the Creative Commons Attribution License (CC BY). The use, distribution or reproduction in other forums is permitted, provided the original author(s) and the copyright owner(s) are credited and that the original publication in this journal is cited, in accordance with accepted academic practice. No use, distribution or reproduction is permitted which does not comply with these terms.
*Correspondence: Dao-Lin Du, daolindu@163.com; Guanlin Li, liguanlin@ujs.edu.com
†These authors have contributed equally to this work and share first authorship
‡These authors have contributed equally to this work