- 1Institute of Crop and Nuclear Technology Utilization, Zhejiang Academy of Agricultural Sciences, Hangzhou, China
- 2College of Life and Environmental Science, Hangzhou Normal University, Hangzhou, China
Rice kernel quality has vital commercial value. Grain chalkiness deteriorates rice’s appearance and palatability. However, the molecular mechanisms that govern grain chalkiness remain unclear and may be regulated by many factors. In this study, we identified a stable hereditary mutant, white belly grain 1 (wbg1), which has a white belly in its mature grains. The grain filling rate of wbg1 was lower than that of the wild type across the whole filling period, and the starch granules in the chalky part were oval or round and loosely arranged. Map-based cloning showed that wbg1 was an allelic mutant of FLO10, which encodes a mitochondrion-targeted P-type pentatricopeptide repeat protein. Amino acid sequence analysis found that two PPR motifs present in the C-terminal of WBG1 were lost in wbg1. This deletion reduced the splicing efficiency of nad1 intron 1 to approximately 50% in wbg1, thereby partially reducing the activity of complex I and affecting ATP production in wbg1 grains. Furthermore, haplotype analysis showed that WBG1 was associated with grain width between indica and japonica rice varieties. These results suggested that WBG1 influences rice grain chalkiness and grain width by regulating the splicing efficiency of nad1 intron 1. This deepens understanding of the molecular mechanisms governing rice grain quality and provides theoretical support for molecular breeding to improve rice quality.
Introduction
Grain chalkiness describes the presence of opaque spots in the endosperm and has a vital role in determining rice quality. Rice grains with high transparency and low chalkiness are preferred by consumers, and varieties that produce this type of grain are popular with rice farmers and seed companies (Fitzgerald et al., 2009). However, rice varieties with high chalkiness grains are difficult to be popularized on a large scale, despite the high yield. Grain chalkiness not only affects the appearance and palatability of rice but also results in reduced yield after rice grain processing (Li et al., 2014; Misra et al., 2019). Therefore, clarification of the molecular mechanisms of rice chalkiness could contribute to the development of high-yield varieties with high quality to meet the market demand.
There are three types of rice grain chalkiness, dependent on where the chalkiness appears in the grain: white belly, white core, and white back (Zhou et al., 2009; Siebenmorgen et al., 2013). In the chalky part of the endosperm, starch granules are round and loosely packed, with many air gaps between starch granules, resulting in low light transmittance and a chalky appearance. In contrast to the chalky part, starch granules are polyhedral and densely packed in the transparent part (Guo et al., 2011; Li et al., 2014). Previous studies have shown that starch synthesis genes, storage protein biosynthesis regulation genes, and plastid and mitochondrion-associated genes may regulate chalkiness (Zhao et al., 2022).
In the starch synthesis pathway, the coordination and balance of various enzyme activities are an essential prerequisite for transparent grain formation. Weak or loss-of-function mutations of rice endosperm ADP-glucose pyrophosphorylase large subunit OsAGPL2 lead to various phenotypic mutations in grain type, ranging from white core to shrunken floury (Lee et al., 2007; Tuncel et al., 2014; Tang et al., 2016; Wei et al., 2017). The small subunit of ADP-glucose pyrophosphorylase qACE9 determines the size of the area of the chalky endosperm in rice (Gao et al., 2016). OsBT1 encodes an ADP-glucose transporter localized in the amyloplast envelope membrane. The osbt1 null mutant has a white core endosperm (Cakir et al., 2016; Li et al., 2017). RNA interference (RNAi)-repressed or knockout SSIIIa mutants had grains with white core endosperms (Ryoo et al., 2007; Zhang et al., 2011). Mutation of SSIIa via RNAi repression slightly increased grain chalkiness area, which resulted in a little white belly or white back. The kernels of SSIIa/SSIIIa double-repression mutant rice lines also had a chalky kernel appearance (Ryoo et al., 2007; Zhang et al., 2011). PHO1 encodes a plastidial α-glucan phosphorylase, and pho1 mutants have a white core or shrunken endosperm, which is temperature-dependent (Satoh et al., 2008). OsbZIP58, a bZIP transcription factor, was shown to directly bind to the promoters of six starch-synthesizing genes, including OsAGPL3, Wx, OsSSIIa, SBE1, OsBEIIb, and ISA2, to regulate their expression. Osbzip58 null mutants had white-bellied grains, with reduced starch accumulation in the grain belly region (Wang et al., 2013). RNAi repression or knockout of the nuclear transcription factor NF-YB1 decreased the filling rate of grains and resulted in a chalky grain phenotype (Xu et al., 2016; Bello et al., 2019). Knockout of NF-YC12 significantly reduced the accumulation of starch and increased grain chalkiness (Bello et al., 2019; Xiong et al., 2019). RSR1, an AP2/EREBP transcription factor, negatively regulates starch synthesis in the endosperm (Fu and Xue, 2010). The rsr1 mutant had altered starch granule morphology, resulting in a white core phenotype (Fu and Xue, 2010).
In seed storage protein biosynthesis, defects in post-transcriptional processing of storage proteins will lead to a complete floury endosperm phenotype (Wang et al., 2010; Fukuda et al., 2011; Tian et al., 2013; Ren et al., 2014; Fukuda et al., 2016; Wang et al., 2016). Recent studies found that some transcription factors and unfolded protein response (UPR) genes are involved in rice seed storage protein metabolism and regulation of grain chalkiness. OsbZIP50, OsBip1, OsBip2, and OsBip3 encode proteins that promote endoplasmic reticulum (ER) UPR, and rice lines that overexpress these UPR genes had various degrees of chalkiness. OsbZIP60 inhibits the expression of OsbZIP50, OsBip1, OsBip2, and OsBip3 to maintain ER homeostasis, and Osbzip60 showed high chalkiness (Yang et al., 2022b).
Defects in genes regulating plastid and mitochondrial metabolism can also lead to grain chalkiness. Mutation of the chloroplast-specific genes pyruvate orthophosphate dikinase FLO4, alanine aminotransferase LNUE1, and glyoxalase FLO15/OsGLYI7 resulted in white core endosperm phenotypes (Kang et al., 2005; You et al., 2019; Fang et al., 2022). OsGBP encodes a plastid-located granule-bound starch synthase binding protein with a carbohydrate-binding module 48 (CBM48) domain; mutation of this gene yields a white belly phenotype (Wang et al., 2020). In addition, the mutation of genes regulating plastid size and differentiation in the endosperm also results in increased grain chalkiness (Matsushima et al., 2014; Cai et al., 2018). The mitochondrion-associated pyruvate kinase OsPK3 physically forms heterodimers with two other PK isozymes, OsPK1 and OsPK4, which are involved in the regulation of grain filling; loss-of-function mutations in OsPK1, OsPK3, or OsPK4 led to chalky grains (Hu et al., 2020).
Previous studies have revealed that deficiency in mitochondrial energy supply leads to serious grain filling defects, with a floury rice grain phenotype (Hao et al., 2019; Wu et al., 2019; Yu et al., 2021). However, it remains unclear whether PPR protein and mitochondrial energy supply defects affect grain chalkiness. In this study, we addressed this question by generating a stable hereditary mutant, wbg1, which yielded a white belly phenotype. Our results confirmed that WBG1 encodes a mitochondrion-targeted P-type PPR protein and affects rice grain chalkiness by regulating the splicing efficiency of nad1 intron 1.
Materials and methods
Plant material and growth conditions
The wbg1 mutant was identified from a mutant pool created by treating the indica cultivar N22 seeds with N-methyl-N-nitrosourea (MNU). flo10 is a rice floury grain mutant that was identified in our previous study (Wu et al., 2019). Developing seeds and mature seeds were taken from the plants growing in paddy fields during the normal growing season.
Microscopy
Scanning electron microscopy (SEM) was used to observe grain phenotype. For this, mature brown grains of wild-type and wbg1 plants were transversely cut using a razor blade and sputter-coated with gold, followed by observation using a HITACHI S-3000N scanning electron microscope. For semi-thin section observation, a transverse section of developing endosperms approximately 1 mm thick was fixed in 2.5% (v/v) glutaraldehyde and 1% (w/v) paraformaldehyde. Samples were sectioned and observed as described previously (Wu et al., 2019).
Positional cloning, vector construction, and rice transformation
For WBG1 mapping, more than 120 polymorphic simple sequence repeat (SSR) and insertion–deletion (InDel) markers covering the whole rice genome were used. Molecular markers in the candidate regions were designed based on nucleotide polymorphisms identified between the indica cultivar 9311 and the japonica cultivar Nipponbare (https://ensembl.gramene.org/Oryza_indica/Location/Compara_Alignments). The WBG1 locus was narrowed to a 98.9-kb region on rice chromosome 3 using the primers listed in Supplementary Table 1. Annotations of predicted open reading frames (ORFs) were determined using genomic sequence NC_029258.1 (3639936 to 3738852) from the National Center for Biotechnology Information (https://www.ncbi.nlm.nih.gov/nuccore/NC_029258.1).
For genetic complementation tests, a 6.1-kb fragment containing the promoter and the full-length coding sequence of WBG1 was amplified using the primers listed in Supplementary Table 1. The fragment was cloned into the binary plasmid vector pCAMBIA1390, followed by introduction into Agrobacterium tumefaciens strain EHA105 and infection of the calli of wbg1. Positive transgenic lines were selected using the recombined vector-specific primers listed in Supplementary Table 1.
Blue native polyacrylamide gel electrophoresis and activity staining of NADH dehydrogenase
Blue native polyacrylamide gel electrophoresis (BN-PAGE) and activity staining of NADH dehydrogenase were performed as previously described (Wu et al., 2019).
RNA extraction, reverse transcriptase polymerase chain reaction, and quantitative RT-PCR analysis
RNA from grains at 9 days after fertilization (DAF) was extracted using an RNAprep Pure Plant Kit (Tiangen Co., Beijing, China) and reverse-transcribed using PrimeScript™ II Reverse Transcriptase (TaKaRa, Mountain View, CA, USA) with random hexamer primers. Reverse transcriptase polymerase chain reaction (RT-PCR) analyses of each splicing event in nad1 introns were performed using primers as previously described (Wu et al., 2019). The rice Actin gene was used as an internal control.
qRT-PCR analyses were performed using an ABI 7500 real-time PCR system with the SYBR Premix Ex Taq™ Kit (TaKaRa). qRT-PCR to assess the splicing efficiency of nad1 intron 1 and nad1 exon1 precursors was performed using the primers listed in Supplementary Table 1. The rice Ubiquitin gene was used as an internal control.
Measurement of total starch, amylose, and ATP content
The total starch content of wild-type and wbg1 mature grains was measured using a Total Starch Assay Kit (Megazyme, Bray, Ireland). The amylose content of wild-type and wbg1 mature grain was measured using the concanavalin A (Con A)-based method, described previously (Wu et al., 2019). ATP content of wild-type and wbg1 grains at 9 DAF were measured using a luciferin–luciferase ATP assay kit following the manufacturer’s protocol (Beyotime, Jiangsu, China).
Measurement of DNA density in agarose gel electrophoresis
Each lane of the gel was plotted using ImageJ software to obtain the value curve (https://imagej.net/software/imagej/). The areas under each resultant value curve were measured in pixels using the wand (tracing) tool in ImageJ.
Haplotypes analysis of WBG1
To analyze the haplotypes of WBG1 in controlling grain width, the full-length chromosome region of WBG1 (3,914 bp, Chr3: 3690005 to 3693919) was used to BLAST in RFGB database (https://www.rmbreeding.cn/). The resources of 1,649 indica and 795 japonica cultivars and the data of grain width (GW) were all collected from the RFGB database. The statistical analysis of phenotypic differences between haplotypes was obtained by using a methodology based on the one-way ANOVA-protected Tukey’s t-tests multiple pairwise comparisons indicated in https://www.rmbreeding.cn/Index/manual#haplotype.
Statistical analysis
GraphPad Prism 8.0 (http://www.graphpad.com/) was used for the statistical analysis. Student’s t-test was used to examine the experimental data.
Results
Phenotypic characterization of the wbg1 mutant
Rice grain chalkiness leads to reductions in rice grain quality and yield. To study the mechanism governing rice grain chalkiness, we treated the indica cultivar N22 grains with MNU and screened mutants for decreased grain quality and weight. Among them, a mutant with white belly phenotype was isolated and named white belly grain 1 (wbg1) (Figure 1A). Compared with the wild type, the grain length of wbg1 was unchanged, but grain width and thickness were significantly decreased (Figure 1B). The filling rate of wbg1 grains across the whole filling period was lower than that in the wild type, and the thousand-grain weight of wbg1 mature seeds was also decreased (Figures 1C, D). Starch measurement showed that the total starch and amylose contents were reduced in the wbg1 mutant (Figures 1E, F). Although wbg1 had decreased grain quality and weight, there was no significant difference in plant height when compared with the wild type (Figures 1G, H). Together, these results indicated that the mutation in wbg1 led to a decrease in grain filling rate and a white belly phenotype in mature grains.
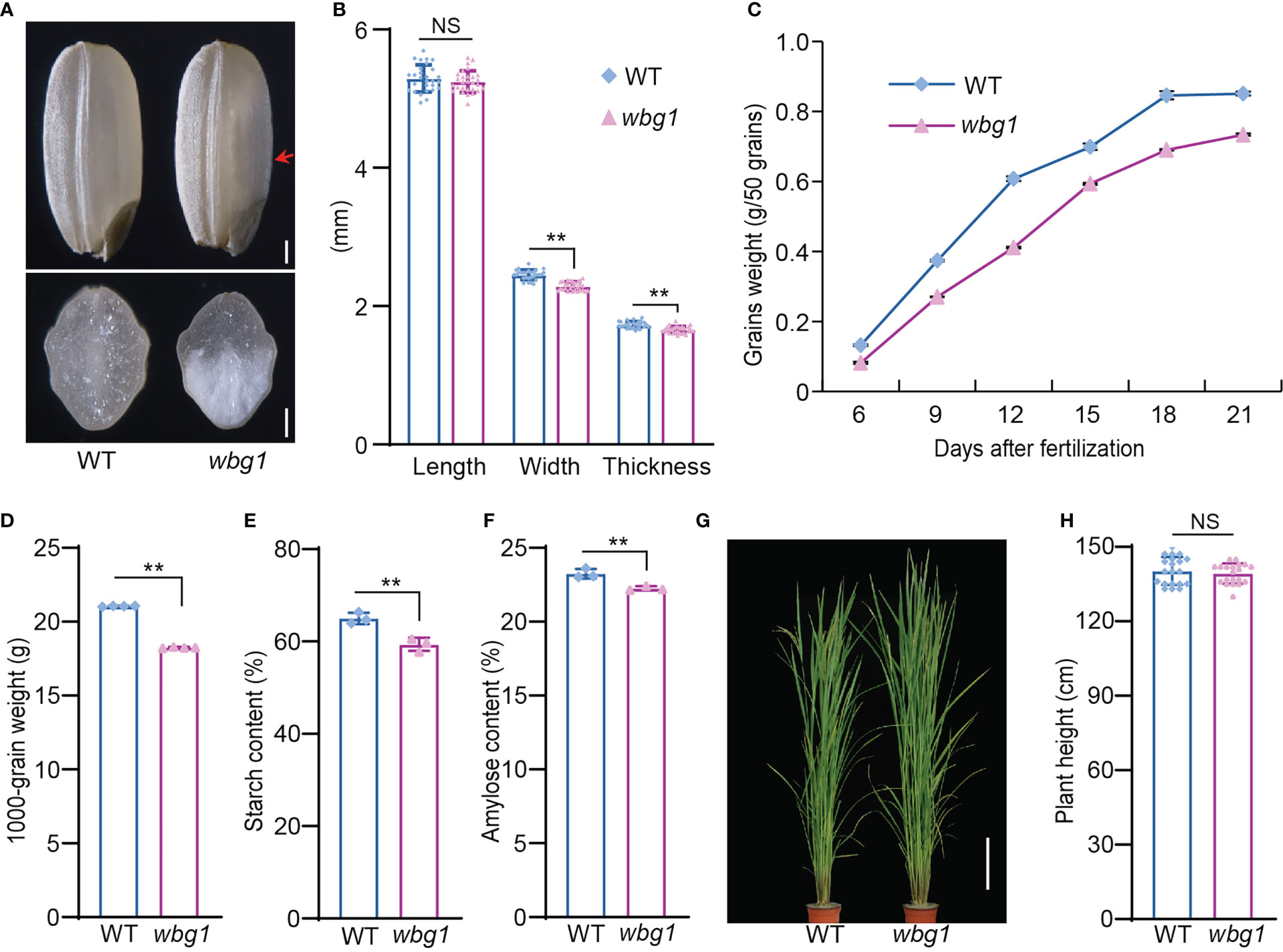
Figure 1 wbg1 phenotype. (A) The outer (upper panel) and cross-section (lower panel) phenotypes of wild-type (WT) and wbg1 grains. The belly side of the wbg1 grain is indicated by the red arrowhead. (B) Grain length, width, and thickness of WT and wbg1 grains. (C) Grain filling rates of WT and wbg1. (D) Thousand-grain weights of WT and wbg1. (E) Starch content of WT and wbg1 grains. (F) Amylose content of WT and wbg1 grains. (G) Plant phenotypes of WT and wbg1 at the grain-filling stage. Scale bar = 20 cm. (H) Plant heights of WT and wbg1 at the grain filling stage. Values are presented as mean ± standard deviation (SD) (**p < 0.01; Student’s t-test). NS, not significant.
Starch granules in the belly side of wbg1 grains were loosely packed
To investigate the mechanism of white belly formation in wbg1, scanning electron microscopy was performed to examine the belly and dorsal sides of wbg1 grains. The arrangement of starch granules on the dorsal side of wbg1 grains was consistent with that of the wild type, where starch granules were polyhedral and tightly packed. However, starch granules in the belly side of wbg1 grains were mostly oval or round and loosely arranged (Figures 2A–F). Semi-thin sections were cut to assess compound starch grains in the belly side of the developing endosperm in wbg1. The results showed that these starch grains were not tightly arranged and were fragile (Figures 2G, H). These results suggested that the wbg1 mutation resulted in the loose arrangement of starch granules in the belly side of the endosperm, which led to the white belly phenotype.
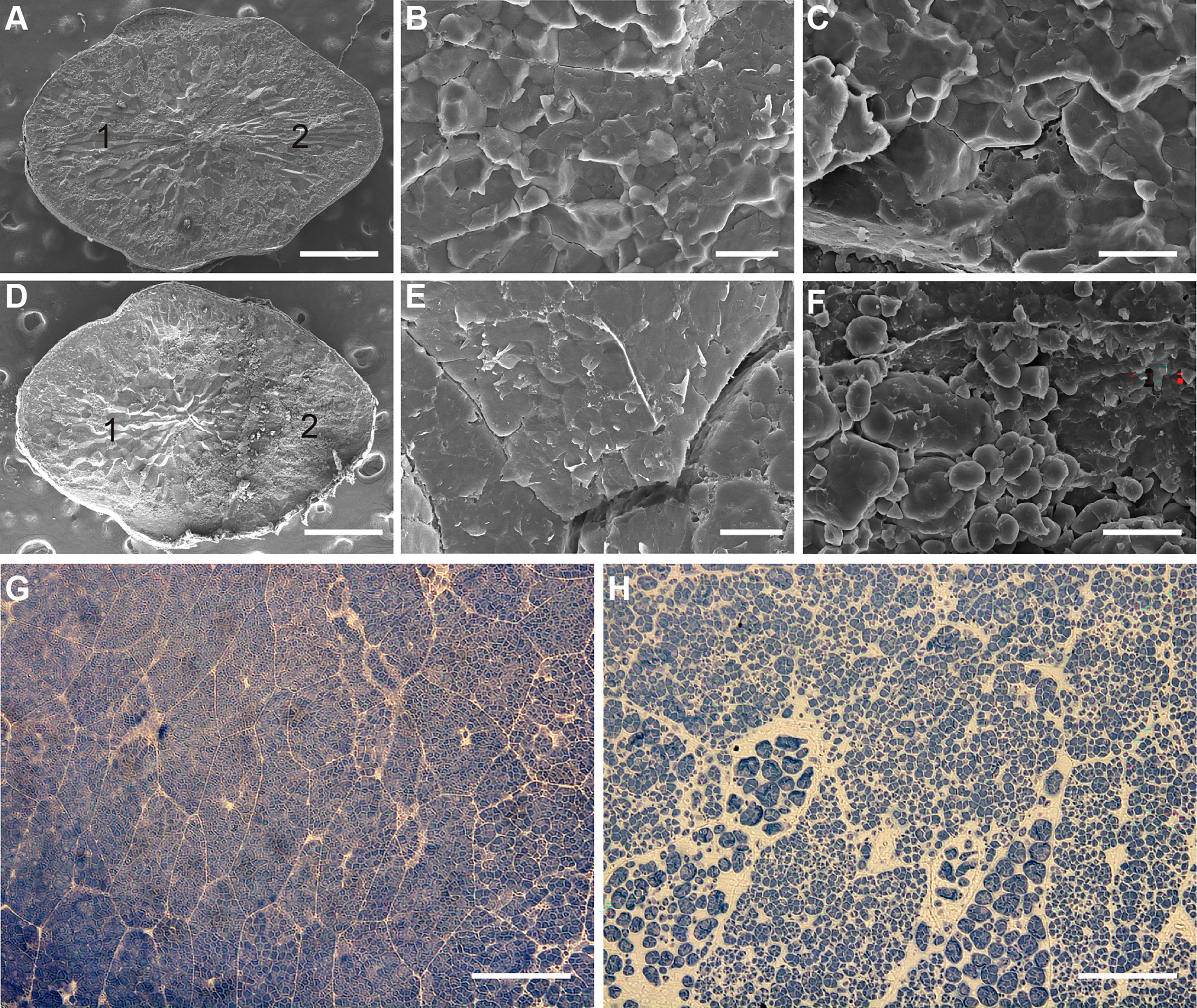
Figure 2 wbg1 endosperm morphology. (A–F) Scanning electron micrographs of transverse sections of wild-type (WT) (A–C) and wbg1 (D–F) endosperm. (A, D) Intact endosperm. “1” and “2” indicate the dorsal and belly sides of the endosperm, respectively. Scale bars, 0.5 mm. (B, E) Magnified region of the dorsal side of WT and wbg1 endosperm, respectively. Scale bars, 10 μm. (C, F) Magnified region of the belly side of WT and wbg1 endosperm, respectively. Scale bars, 10 μm. (G, H) Semi-thin sections of the belly side of WT and wbg1 endosperm, respectively. Scale bars, 10 μm.
wbg1 is a weak allelic mutant of FLO10
Of the 336 grains harvested from the heterozygous WBG1/wbg1 plant, 265 grains showed the wild-type phenotype, 71 grains showed the wbg1 phenotype, and the segregation ratio was consistent with 3:1 (χ2 = 3.02 < 3.84, p > 0.05), indicating that wbg1 phenotype is controlled by a single recessive nuclear gene. To identify the candidate gene responsible for the wbg1 phenotype, map-based cloning was carried out to isolate WBG1. An F2 mapping population was created by crossing wbg1 with the japonica rice cultivar Nipponbare. The mutation site in wbg1 was initially mapped to a 1,000-kb interval between the InDel markers I3-7 and I3-9 on chromosome 3. With the use of 1,143 individuals with the wbg1 phenotype, the WBG1 locus was narrowed down to a 98.9-kb genomic region containing 20 ORFs (Figure 3A). Sequencing analysis revealed that an 8-bp deletion was detected in the 10th ORF (Figure 3B), previously identified as FLO10 (Wu et al., 2019). The 8-bp deletion in wbg1 occurred 3,651-bp downstream of the start codon, resulting in a premature stop codon that generated a truncated protein harboring the N-terminal 1,218 amino acids.
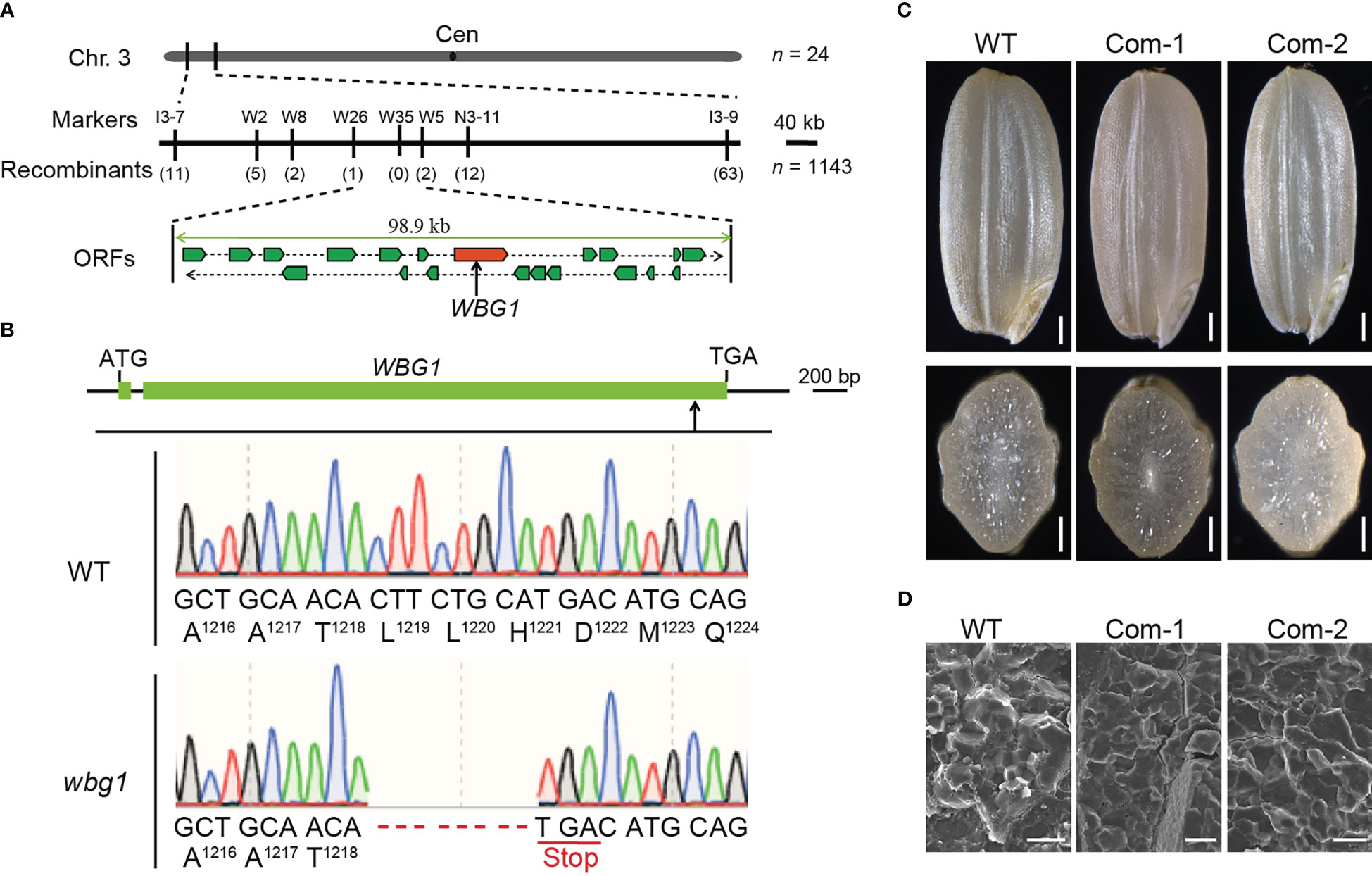
Figure 3 WBG1 map-based cloning and complementation tests. (A) Fine mapping of the WBG1 locus. The WBG1 locus was narrowed to a 98.9-kb region on rice chromosome 3 by using fine mapping primers listed in Supplementary Table 1. This region includes 20 predicted open reading frames (ORFs). The molecular markers and numbers of recombinants are indicated. Cen, centromere. (B) Exon/intron structure of WBG1 and the mutation site in wbg1. Green boxes indicate the two exons, and the line between the exons indicates the intron in WBG1. ATG and TGA indicate the start and stop codons, respectively. An eight-nucleotide deletion in the coding region of WBG1 results in a premature termination codon and protein truncation in wbg1. (C) Complementation of wbg1 by the introduction of a 6.1-kb fragment containing the 2.3-kb promoter and 3.8-kb full-length coding sequence of FLO10 into the wbg1 mutant. Scale bar, 0.5 mm. (D) Scanning electron microscopy (SEM) images of the belly side of grains from representative complemented lines. Scale bar, 10 μm.
To confirm whether the weak mutation in FLO10 was responsible for the wbg1 mutant phenotype, we introduced a 6.1-kb fragment containing the promoter region and the full-length coding sequence of FLO10 into the wbg1 mutant. Both the brown rice morphology and starch grain arrangement in wbg1 were restored (Figures 3C, D). Collectively, these results showed that a weak allelic mutation in FLO10 was responsible for the wbg1 white belly phenotype.
Splicing efficiency of mitochondrial nad1 intron 1 was decreased in wbg1
The flo10 mutant produces flo10 with only the N-terminal 151-amino-acid sequences and loss of all the predicted PPR motifs (Wu et al., 2019). However, the wbg1 mutant has 1218 amino acid residues with loss of the C-terminal 51 amino acid sequences containing two PPR motifs (Figure 4). In the flo10 mutant, the splicing efficiency of nad1 intron 1 was found to be substantially decreased, with a substantial accumulation of the precursor (Wu et al., 2019). To determine whether the splicing efficiency of nad1 intron 1 in wbg1 mutants was affected, we carried out RT-PCR and qRT-PCR experiments. The results of RT-PCR showed that the contents of nad1 intron 1 and mature nad1 mRNA in wbg1 decreased to approximately 50% (Figures 5A–C), which were further confirmed by qRT-PCR experiments (Figure 5D). The qRT-PCR experiments also indicated that nad1 exon 1 precursor was accumulated in the wbg1 mutant (Figure 5E). These results indicate that the two PPR motifs in the C-terminal, which are not present in wbg1, have an essential role in the WBG1 function.
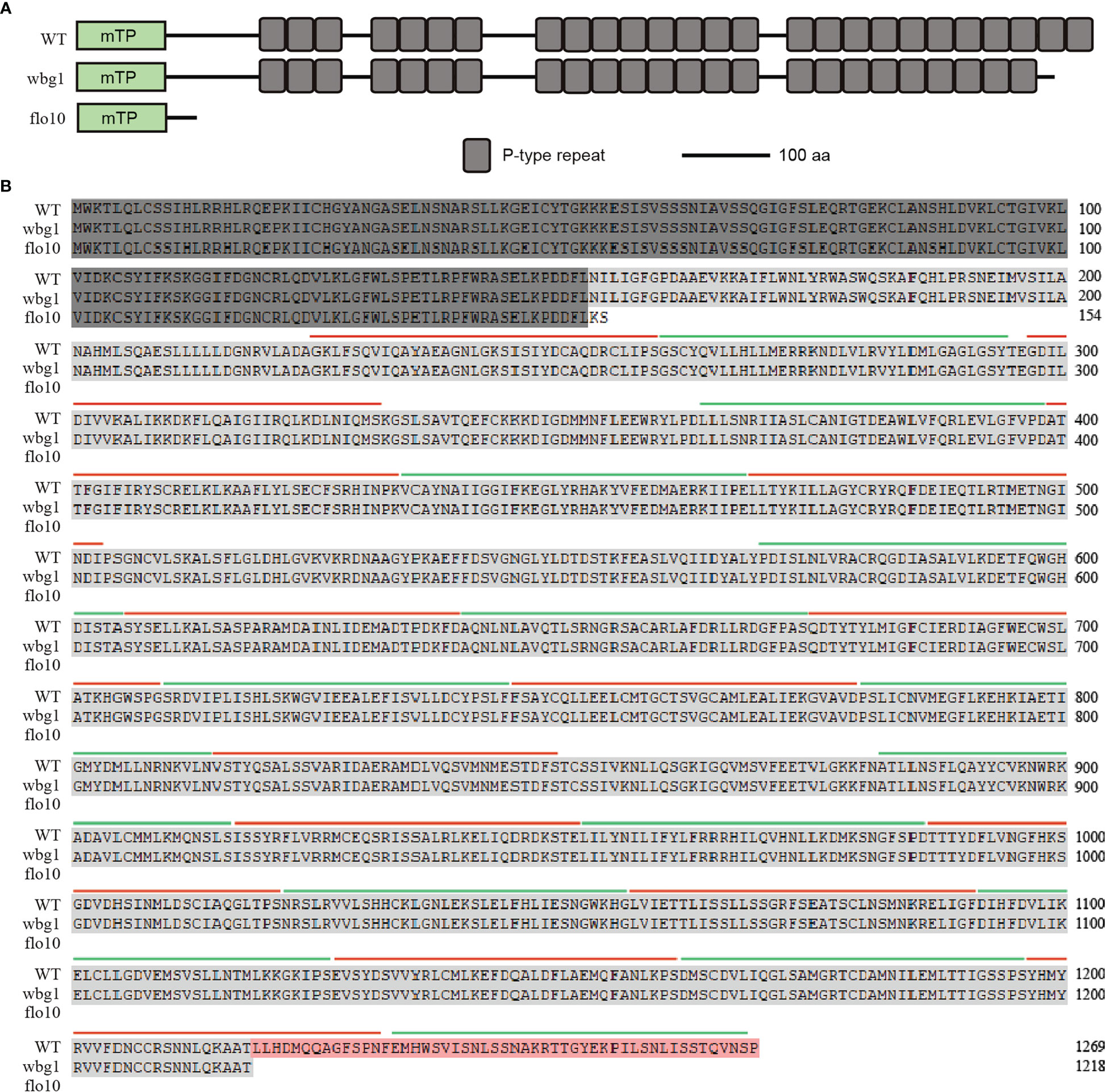
Figure 4 Schematic domain structure and amino acid sequence analysis of wbg1. (A) Schematic domain structure of WBG1, flo10, and wbg1 proteins. mTP, mitochondrion target peptide predicted by TargetP. WBG1/FLO10 comprises 1,269 amino acids with 26 predicted PPR repeats; the flo10 mutant lost all PPR motifs, while wbg1 only lost the last two PPR motifs at the C-terminal. (B) Sequences alignment of WBG1, wbg1, and flo10 proteins with predicted PPR repeat annotations. Amino acid residues present in all three sequences are indicated by dark gray shading. Light gray shading indicates the residual amino acids present in wbg1 and WBG1. The amino acid sequence at the C-terminal that is deleted in wbg1 but present in WBG1 is shaded orange. Each PPR repeat is indicated alternately by green and red lines.
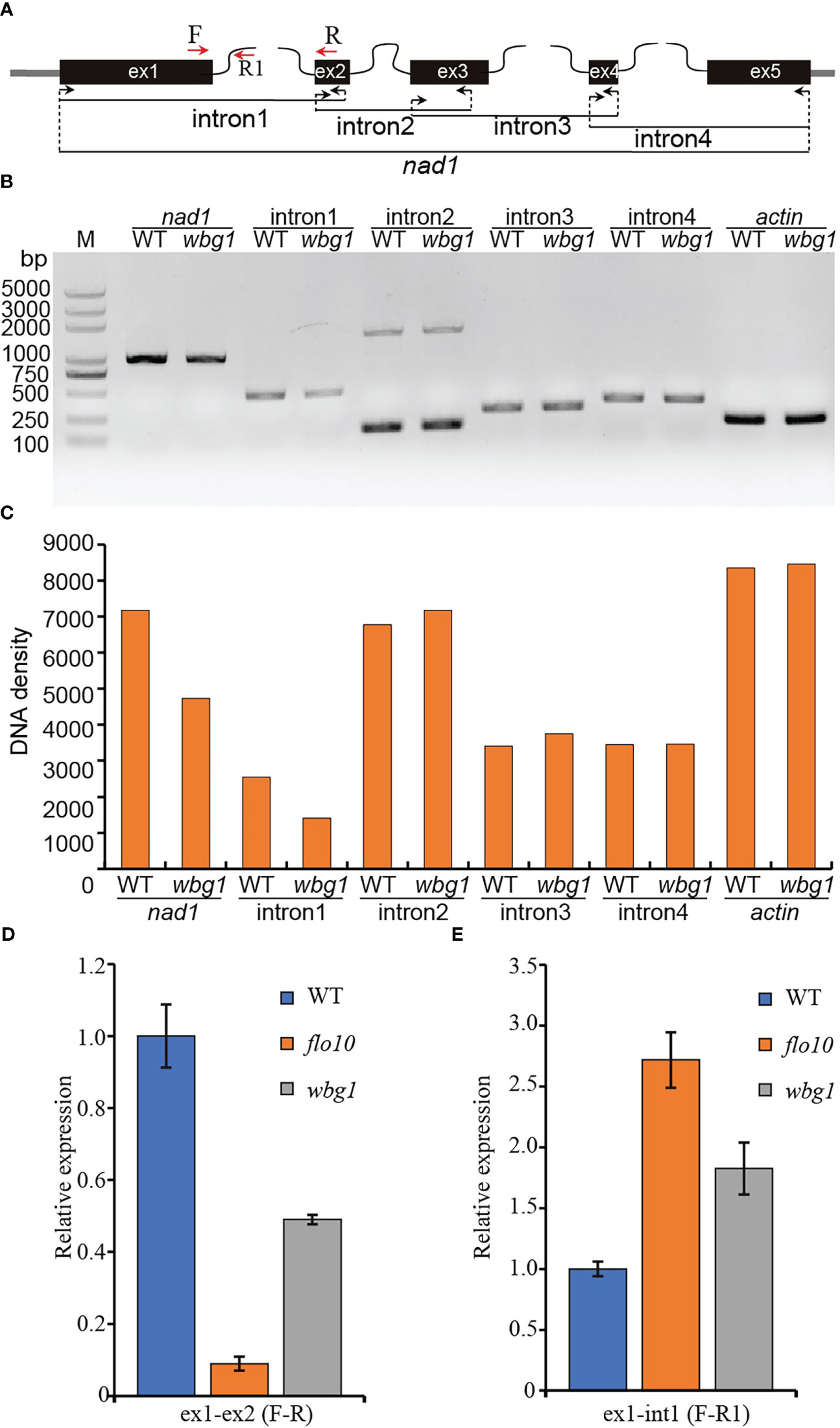
Figure 5 Splicing efficiency analysis of nad1 intron 1 in wbg1. (A) Schematic representation of the rice nad1 gene. (B) Reverse transcription polymerase chain reaction (RT-PCR) analysis of nad1 intron 1, 2, 3, and 4 splicing events in wild type (WT) and wbg1. The amplified fragments are indicated in panel (A) The rice Actin gene was used as an internal control. (C) DNA density of the amplified fragments in (B) was quantified using ImageJ software. (D, E) Quantitative RT-PCR measuring the splicing efficiency of nad1 intron 1 and nad1 exon 1 precursor. The PCRs were performed with the primer pairs indicated in panel (A) RNA was extracted from WT and wbg1 grains at 9 days after flowering (DAF). Representative results from three biological replicates are shown. Values are all mean ± standard deviation (SD).
The wbg1 mutant exhibits reduced respiratory chain complex I activity and ATP contents
nad1 (NADH dehydrogenase subunit 1) encodes the core subunit of respiratory chain complex I, and the reduced splicing efficiency of nad1 intron 1 leads to decreased nad1-translated protein content, which may affect the activity of respiratory chain complex I (Subrahmanian et al., 2016). BN-PAGE and in-gel NADH dehydrogenase activity staining were employed to investigate the changes in respiratory chain complex I activity in wbg1 mutant. Compared with that in the wild type, the activity of complex I in the wbg1 mutant was significantly reduced, but not to the extent observed in flo10 (Figure 6A). The transformation of WBG1 into the wbg1 mutant was able to restore the activity of respiratory chain complex I to wild-type levels. Given that ATP production in mitochondria is coupled with electron transfer via respiratory chain complex I in mitochondria, we measured ATP content in the developing endosperm of wbg1 and its complementary lines. The results showed that the ATP content in wbg1 was lower than that in the wild type but higher than in the flo10 mutant (Figure 6B). Transferring WBG1 into the wbg1 mutant was able to restore the ATP content in the developing endosperm to wild-type levels. Together, these results indicate that the grain chalkiness phenotype in wbg1 is caused by the reduction of mitochondrial respiratory chain complex I activity resulting from the deletion of the two PPR motifs.
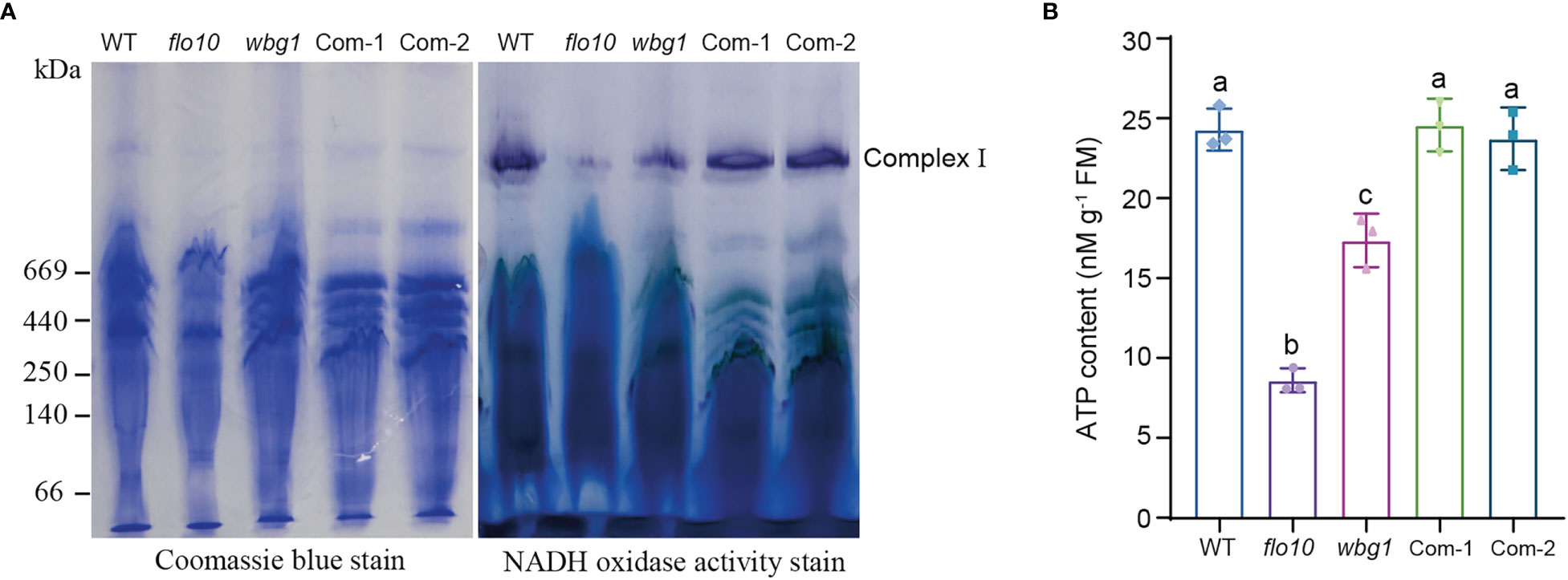
Figure 6 The deletion of two PPR motifs at the C-terminal of wbg1 reduced the activity of respiratory chain complex I in mitochondria. (A) Assembly and activity analysis of respiratory chain complex I in wild-type (WT) and flo10 and wbg1 mutants and complemented lines (Com-1 and Com-2). Left, Coomassie brilliant blue staining of mitochondrial respiratory chain complex I, separated using blue native gel. Right, in-gel staining of NADH dehydrogenase activity in respiratory chain complex (I) (B) ATP concentration in grains at 9 days after fertilization. Values are all mean ± SD from three biological replicates. a–c indicate significant differences by Student’s t-tests (p < 0.01).
WBG1 is associated with grain width
The wbg1 mutant had a significantly decreased grain width (Figure 1B), which may result from the arrested transport of assimilates from the dorsal to belly regions of the grain. To investigate the role of WBG1 in rice grain width, a genetic association analysis between WBG1 and grain width was performed in indica and japonica cultivars (https://www.rmbreeding.cn). The results showed that a total of 14 polymorphic sites were found, nine of which led to amino acid changes (Figure 7A). Based on the polymorphic sites, seven main haplotypes (referred to as Hap_1 to Hap_7) were observed in detail in both indica and japonica. A comparison of haplotype frequencies between cultivars indicated that Hap_6 and Hap_7 were common in japonica cultivars, with frequencies of 20.38% and 79.50%, respectively. Hap_1 to Hap_5 were almost exclusively found in indica cultivars with frequencies of 73.68%, 2.12%, 16.56%, 5.34%, and 0.49%, respectively (Figure 7B). Hap_6 and Hap_7, the two common haplotypes in japonica, had wider grains (3.408 and 3.243 mm, respectively), compared with Hap_1 to Hap_5 in indica, which had grain widths of 2.879, 2.724, 2.993, 2.929, and 2.933 mm, respectively (Figure 7C). Together, these data suggest that there was clear haplotype differentiation of WBG1 between indica and japonica rice varieties based on grain width.
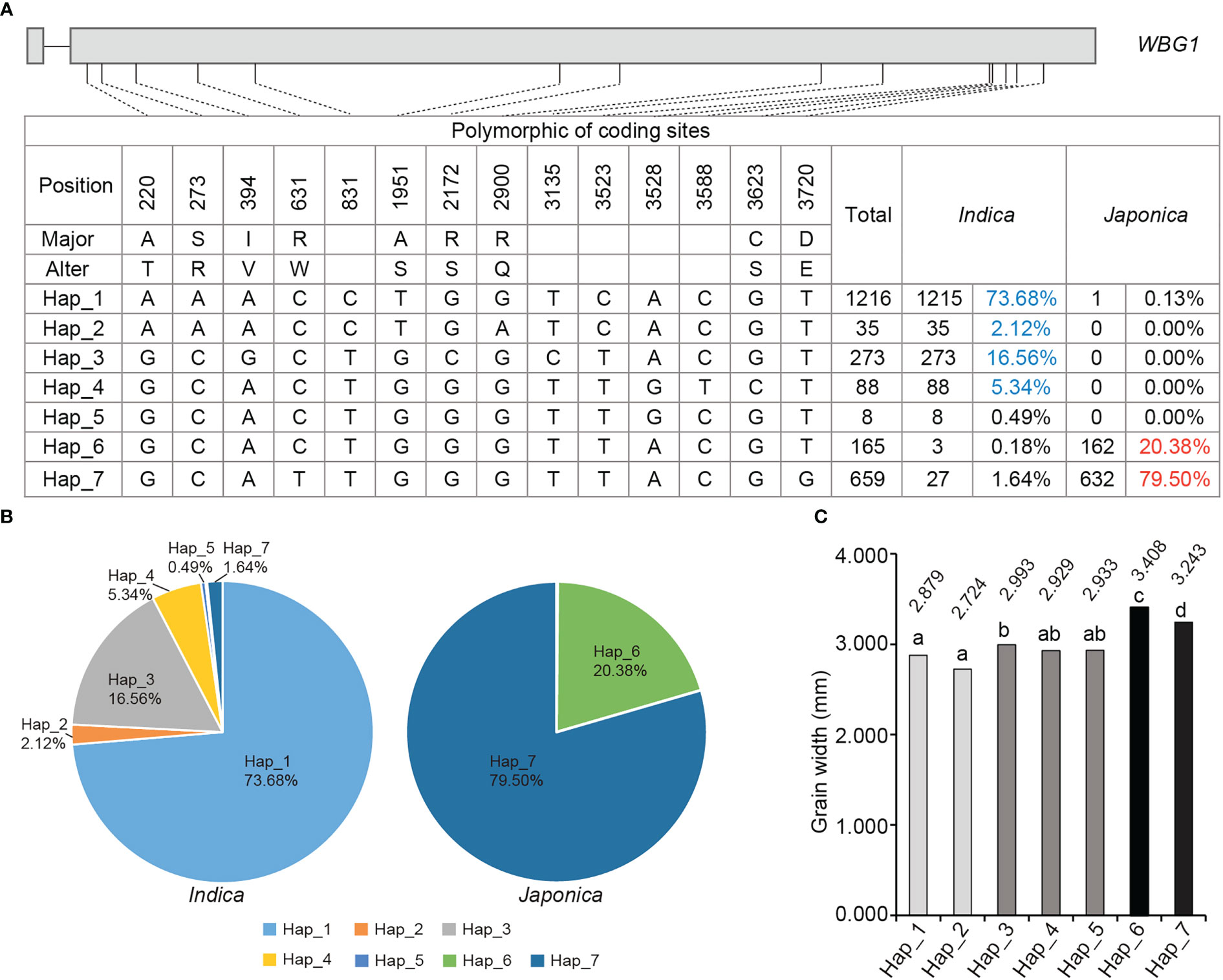
Figure 7 Haplotype analysis of WBG1 and haplotype association with rice grain width. (A) Polymorphic nucleotides in the WBG1 encoding region in indica and japonica cultivars. (B) Distribution of the proportion of seven haplotypes (Hap_1 to Hap_7) in indica (left) and japonica (right) cultivars. (C) Grain width statistics of the seven haplotypes. a–d indicate significant differences by Tukey’s t-tests (p < 0.01).
Discussion
Rice chalkiness is regulated by many genes, including those involved in starch synthesis and storage protein biosynthesis regulation, and plastid- and mitochondrion-associated genes (Zhao et al., 2022). PPR proteins form a large protein family in land plants and have functions in various processes, including cytoplasmic sterility, leaf and seed development, and stress responses (Li et al., 2021; Wang et al., 2021). The loss of function of some mitochondrion-associated PPR is known to lead to a substantial decline in grain filling rate and a floury endosperm (Hao et al., 2019; Wu et al., 2019; Yu et al., 2021; Yang et al., 2022a). However, the involvement of PPR proteins in the regulation of rice grain chalkiness has not been reported until now. In this study, we identified a stable hereditary mutant, wbg1, with mature grains that have a white belly phenotype (Figure 1A). The filling rate of wbg1 grains across the whole filling period was lower than that of the wild type, and the starch granules in the chalky part were loosely arranged (Figures 1C, 2F). Map-based cloning showed that wbg1 was a weak allelic mutant of FLO10 (Figure 3). The white belly observed in the wbg1 mutant is different from the floury endosperm resulting from previously identified defects in mitochondrion-associated PPR proteins. Our results indicate that mitochondrion-associated PPR proteins play an important role in the rice grain white belly phenotype.
wbg1 is a weak allele mutant of FLO10. In the wbg1 mutant, there were 8-bp deletions at the tail of WBG1, causing a premature translation and 51-amino-acid deletion at the C-terminal (Figure 3B). Different from the deletion of all 26 PPR motifs in the flo10 protein, the wbg1 only lost two PPR motifs at the C-terminal (Figure 4). Based on the recognition code of PPR motifs (Barkan et al., 2012; Yagi et al., 2013), the last two PPR motifs may recognize two RNA bases on the nad1 intron 1 precursor. Although the splicing efficiency of nad1 intron 1 in wbg1 is not as serious as that in flo10, it is reduced to approximately 50% (Figure 5). Thus, the recognition of the last two RNA bases on the nad1 intron 1 precursor by WBG1 plays an important role in nad1 intron 1 splicing.
Grain filling involves the transport of assimilates from the dorsal portion to the belly portion of grains. Comprehensive analyses, including 14C-labeled assimilate analyses, showed that sucrose moves via the symplastic pathway in the nucellar epidermis after unloading from the dorsal phloem and is taken up via the apoplastic pathway by aleurone cells into the endosperm (Oparka and Gates, 1981a; Oparka and Gates, 1981b; Yang et al., 2018). Previous studies have shown that the mutation in FLO10 leads to an abnormal aleurone layer with defective mitochondrial function, which may affect the transportation of filling assimilates into the developing endosperm, specifically leading to slow grain filling and a floury endosperm (Wu et al., 2019). In wbg1, we found that the activity of respiratory chain complex I and ATP content in the developing endosperm was reduced compared with the wild type, but not to the same extent as in the flo10 mutant (Figure 6). This indicates that mitochondrial activity depends on the level of mature nad1 mRNA, which is determined by the splicing efficiency of nad1 intron 1. Furthermore, the white belly phenotype in wbg1 was distinct from the floury phenotype in flo10 (Figure 1A), indicating that the WBG1 function may be associated with the transverse transportation of filling assimilates in the grain. The loss of the last two PPR motifs in wbg1 may lead to a reduction in the function of the aleurone layer, which contains a large number of mitochondria. This may result in further reductions in the transport efficiency of filling assimilates from the dorsal to the belly portion of the endosperm, leading to a lack of filling assimilates and resultant chalkiness in the belly. Based on these data, we propose a model for WBG1 regulation of grain chalkiness in rice (Figure 8). Further research could usefully focus on improving the efficiency of filling assimilate transport by increasing mitochondrial activity, which should decrease grain chalkiness and enable the cultivation of high-quality rice varieties to meet the market demand.
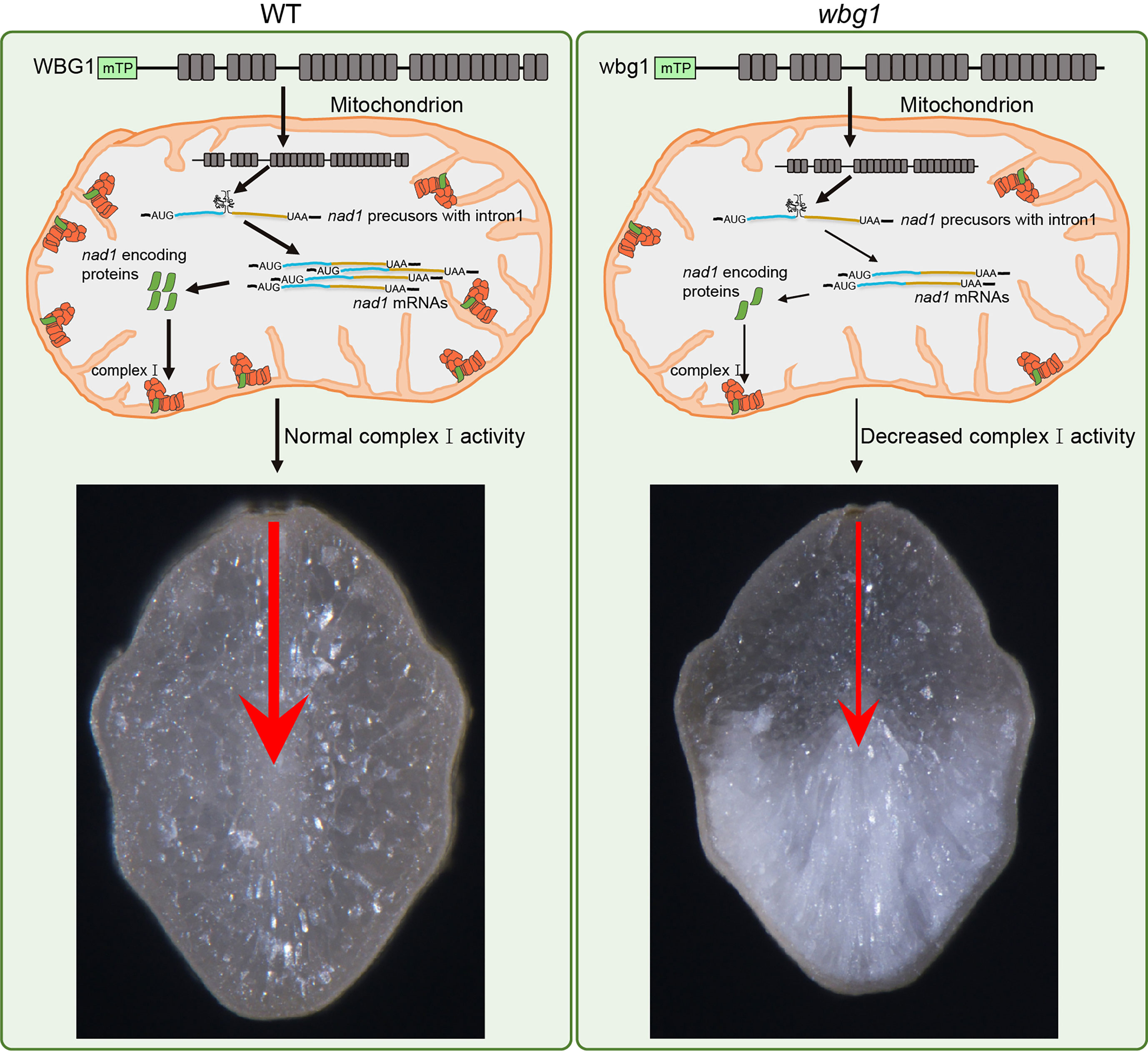
Figure 8 A proposed working model of WBG1 in regulating rice grain chalkiness. In wild-type (WT) (left), nad1 precursors with intron1 in mitochondria can be normally spliced by WBG1 and produce a certain level of nad1 mRNA. The nad1 mRNA encoded protein forms the required amount of mitochondrial complex I, thus maintaining normal mitochondrial function and keeping the transport efficiency of filling assimilates from grain dorsal to belly. mTP, predicted mitochondrion-targeted peptide. Each gray box represents a PPR motif. The blue bold line represents nad1 exon 1, the brown bold line represents nad1 exons 2–5, and the curved line between them represents nad1 intron1. In wbg1 mutant (right), the loss of two PPR motifs at the C-terminal of wbg1 leads to a reduction in splicing efficiency of nad1 intron1, resulting in a decrease in nad1 mRNA level. Then, the activity of complex I, which is composed of nad1 encoded protein, was decreased. Therefore, the transport efficiency of filling assimilates from the dorsal to belly is reduced, resulting in white belly grain.
Previous studies have shown that increased grain width results in grain belly chalkiness (Shi et al., 2019). In this study, we showed that WBG1 regulates grain filling: a weak mutation in WBG1 decreases the grain filling rate, leading to the formation of a white belly (Figures 1A, C). An analysis of the association between WBG1 encoding region haplotypes and grain width found clear haplotype differentiation between indica and japonica rice varieties, which was associated with grain width (Figure 7). These results indicate that the WBG1 haplotype may have an important role in grain width differentiation between indica and japonica rice, and WBG1 may have a function in the coordination of rice grain filling rate and grain width.
Data availability statement
The original contributions presented in the study are included in the article/Supplementary Material. Further inquiries can be directed to the corresponding authors.
Author contributions
MW, SY, and XZ planned and designed the experiments. MC and MW mapped the WBG1. MW, MC, RZ, and JY performed all other experiments. SY, GZ, and FY analyzed the data. MW and MC drafted the manuscript. All authors contributed to the article and approved the submitted version.
Funding
This work was supported by the National Natural Science Foundation of China (32101801) and the Natural Science Foundation of Zhejiang Province (LQ22C130001).
Conflict of interest
The authors declare that the research was conducted in the absence of any commercial or financial relationships that could be construed as a potential conflict of interest.
Publisher’s note
All claims expressed in this article are solely those of the authors and do not necessarily represent those of their affiliated organizations, or those of the publisher, the editors and the reviewers. Any product that may be evaluated in this article, or claim that may be made by its manufacturer, is not guaranteed or endorsed by the publisher.
Supplementary material
The Supplementary Material for this article can be found online at: https://www.frontiersin.org/articles/10.3389/fpls.2023.1136849/full#supplementary-material
References
Barkan, A., Rojas, M., Fujii, S., Yap, A., Chong, Y. S., Bond, C. S., et al. (2012). A combinatorial amino acid code for RNA recognition by pentatricopeptide repeat proteins. PloS Genet. 8, e1002910. doi: 10.1371/journal.pgen.1002910
Bello, B. K., Hou, Y., Zhao, J., Jiao, G., Wu, Y., Li, Z., et al. (2019). NF-YB1-YC12-bHLH144 complex directly activates Wx to regulate grain quality in rice (Oryza sativa l.). Plant Biotechnol. J. 17, 1222–1235. doi: 10.1111/pbi.13048
Cai, Y., Li, S., Jiao, G., Sheng, Z., Wu, Y., Shao, G., et al. (2018). OsPK2 encodes a plastidic pyruvate kinase involved in rice endosperm starch synthesis, compound granule formation and grain filling. Plant Biotechnol. J. 16, 1878–1891. doi: 10.1111/pbi.12923
Cakir, B., Shiraishi, S., Tuncel, A., Matsusaka, H., Satoh, R., Singh, S., et al. (2016). Analysis of the rice ADP-glucose transporter (OsBT1) indicates the presence of regulatory processes in the amyloplast stroma that control ADP-glucose flux into starch. Plant Physiol. 170, 1271–1283. doi: 10.1104/pp.15.01911
Fang, L., Ma, L., Zhao, S., Cao, R., Jiao, G., Hu, P., et al. (2022). Alanine aminotransferase (OsAlaAT1) modulates nitrogen utilization, grain yield, and quality in rice. J. Genet. Genomics 49, 510–513. doi: 10.1016/j.jgg.2022.02.028
Fitzgerald, M. A., McCouch, S. R., Hall, R. D. (2009). Not just a grain of rice: the quest for quality. Trends Plant Sci. 14, 133–139. doi: 10.1016/j.tplants.2008.12.004
Fu, F. F., Xue, H. W. (2010). Coexpression analysis identifies rice starch Regulator1, a rice AP2/EREBP family transcription factor, as a novel rice starch biosynthesis regulator. Plant Physiol. 154, 927–938. doi: 10.1104/pp.110.159517
Fukuda, M., Kawagoe, Y., Murakami, T., Washida, H., Sugino, A., Nagamine, A., et al. (2016). The dual roles of the golgi transport 1 (GOT1B): RNA localization to the cortical endoplasmic reticulum and the export of proglutelin and alpha-globulin from the cortical ER to the golgi. Plant Cell Physiol. 57, 2380–2391. doi: 10.1093/pcp/pcw154
Fukuda, M., Satoh-Cruz, M., Wen, L., Crofts, A. J., Sugino, A., Washida, H., et al. (2011). The small GTPase Rab5a is essential for intracellular transport of proglutelin from the golgi apparatus to the protein storage vacuole and endosomal membrane organization in developing rice endosperm. Plant Physiol. 157, 632–644. doi: 10.1104/pp.111.180505
Gao, Y., Liu, C., Li, Y., Zhang, A., Dong, G., Xie, L., et al. (2016). QTL analysis for chalkiness of rice and fine mapping of a candidate gene for qACE9. Rice (NY). 9, 41. doi: 10.1186/s12284-016-0114-5
Guo, T., Liu, X., Wan, X., Weng, J., Liu, S., Liu, X., et al. (2011). Identification of a stable quantitative trait locus for percentage grains with white chalkiness in rice (Oryza sativa). J. Integr. Plant Biol. 53, 598–607. doi: 10.1111/j.1744-7909.2011.01041.x
Hao, Y., Wang, Y., Wu, M., Zhu, X., Teng, X., Sun, Y., et al. (2019). A nucleus-localized PPR protein OsNPPR1 is important for mitochondrial function and endosperm development in rice. J. Exp. Bot. 70, 4705–4720. doi: 10.1093/jxb/erz226
Hu, L., Tu, B., Yang, W., Yuan, H., Li, J., Guo, L., et al. (2020). Mitochondria-associated pyruvate kinase complexes regulate grain filling in rice. Plant Physiol. 183, 1073–1087. doi: 10.1104/pp.20.00279
Kang, H. G., Park, S., Matsuoka, M., An, G. (2005). White-core endosperm floury endosperm-4 in rice is generated by knockout mutations in the C4-type pyruvate orthophosphate dikinase gene (OsPPDKB). Plant J. 42, 901–911. doi: 10.1111/j.1365-313X.2005.02423.x
Lee, S. K., Hwang, S. K., Han, M., Eom, J. S., Kang, H. G., Han, Y., et al. (2007). Identification of the ADP-glucose pyrophosphorylase isoforms essential for starch synthesis in the leaf and seed endosperm of rice (Oryza sativa l.). Plant Mol. Biol. 65, 531–546. doi: 10.1007/s11103-007-9153-z
Li, Y., Fan, C., Xing, Y., Yun, P., Luo, L., Yan, B., et al. (2014). Chalk5 encodes a vacuolar h+-translocating pyrophosphatase influencing grain chalkiness in rice. Nat. Genet. 46, 398–404. doi: 10.1038/ng.2923
Li, X., Sun, M., Liu, S., Teng, Q., Li, S., Jiang, Y. (2021). Functions of PPR proteins in plant growth and development. Int. J. Mol. Sci. 22, 11274. doi: 10.3390/ijms222011274
Li, S., Wei, X., Ren, Y., Qiu, J., Jiao, G., Guo, X., et al. (2017). OsBT1 encodes an ADP-glucose transporter involved in starch synthesis and compound granule formation in rice endosperm. Sci. Rep. 7, 40124. doi: 10.1038/srep40124
Matsushima, R., Maekawa, M., Kusano, M., Kondo, H., Fujita, N., Kawagoe, Y., et al. (2014). Amyloplast-localized SUBSTANDARD STARCH GRAIN4 protein influences the size of starch grains in rice endosperm. Plant Physiol. 164, 623–636. doi: 10.1104/pp.113.229591
Misra, G., Anacleto, R., Badoni, S., Butardo, V., Molina, L., Graner, A., et al. (2019). Dissecting the genome-wide genetic variants of milling and appearance quality traits in rice. J. Exp. Bot. 70, 5115–5130. doi: 10.1093/jxb/erz256
Oparka, K. J., Gates, P. (1981a). Transport of assimilates in the developing caryopsis of rice (Oryza sativa l.) : The pathways of water and assimilated carbon. Planta 152, 388–396. doi: 10.1007/BF00385354
Oparka, K. J., Gates, P. (1981b). Transport of assimilates in the developing caryopsis of rice (Oryza sativa l.) : Ultrastructure of the pericarp vascular bundle and its connections with the aleurone layer. Planta 151, 561–573. doi: 10.1007/BF00387436
Ren, Y., Wang, Y., Liu, F., Zhou, K., Ding, Y., Zhou, F., et al. (2014). GLUTELIN PRECURSOR ACCUMULATION3 encodes a regulator of post-golgi vesicular traffic essential for vacuolar protein sorting in rice endosperm. Plant Cell. 26, 410–425. doi: 10.1105/tpc.113.121376
Ryoo, N., Yu, C., Park, C. S., Baik, M. Y., Park, I. M., Cho, M. H., et al. (2007). Knockout of a starch synthase gene OsSSIIIa/Flo5 causes white-core floury endosperm in rice (Oryza sativa l.). Plant Cell Rep. 26, 1083–1095. doi: 10.1007/s00299-007-0309-8
Satoh, H., Shibahara, K., Tokunaga, T., Nishi, A., Tasaki, M., Hwang, S. K., et al. (2008). Mutation of the plastidial alpha-glucan phosphorylase gene in rice affects the synthesis and structure of starch in the endosperm. Plant Cell. 20, 1833–1849. doi: 10.1105/tpc.107.054007
Shi, C., Ren, Y., Liu, L., Wang, F., Zhang, H., Tian, P., et al. (2019). Ubiquitin specific protease 15 has an important role in regulating grain width and size in rice. Plant Physiol. 180, 381–391. doi: 10.1104/pp.19.00065
Siebenmorgen, T. J., Grigg, B. C., Lanning, S. B. (2013). Impacts of preharvest factors during kernel development on rice quality and functionality. Annu. Rev. Food Sci. Technol. 4, 101–115. doi: 10.1146/annurev-food-030212-182644
Subrahmanian, N., Remacle, C., Hamel, P. P. (2016). Plant mitochondrial complex I composition and assembly: A review. Biochim. Biophys. Acta 1857, 1001–1014. doi: 10.1016/j.bbabio.2016.01.009
Tang, X. J., Peng, C., Zhang, J., Cai, Y., You, X. M., Kong, F., et al. (2016). ADP-glucose pyrophosphorylase large subunit 2 is essential for storage substance accumulation and subunit interactions in rice endosperm. Plant Sci. 249, 70–83. doi: 10.1016/j.plantsci.2016.05.010
Tian, L., Dai, L. L., Yin, Z. J., Fukuda, M., Kumamaru, T., Dong, X. B., et al. (2013). Small GTPase Sar1 is crucial for proglutelin and alpha-globulin export from the endoplasmic reticulum in rice endosperm. J. Exp. Bot. 64, 2831–2845. doi: 10.1093/jxb/ert128
Tuncel, A., Kawaguchi, J., Ihara, Y., Matsusaka, H., Nishi, A., Nakamura, T., et al. (2014). The rice endosperm ADP-glucose pyrophosphorylase large subunit is essential for optimal catalysis and allosteric regulation of the heterotetrameric enzyme. Plant Cell Physiol. 55, 1169–1183. doi: 10.1093/pcp/pcu057
Wang, X., An, Y., Xu, P., Xiao, J. (2021). Functioning of PPR proteins in organelle RNA metabolism and chloroplast biogenesis. Front. Plant Sci. 12, 627501. doi: 10.3389/fpls.2021.627501
Wang, Y., Liu, F., Ren, Y., Wang, Y., Liu, X., Long, W., et al. (2016). GOLGI TRANSPORT 1B regulates protein export from the endoplasmic reticulum in rice endosperm cells. Plant Cell. 28, 2850–2865. doi: 10.1105/tpc.16.00717
Wang, Y., Ren, Y., Liu, X., Jiang, L., Chen, L., Han, X., et al. (2010). OsRab5a regulates endomembrane organization and storage protein trafficking in rice endosperm cells. Plant J. 64, 812–824. doi: 10.1111/j.1365-313X.2010.04370.x
Wang, W., Wei, X., Jiao, G., Chen, W., Wu, Y., Sheng, Z., et al. (2020). GBSS-BINDING PROTEIN, encoding a CBM48 domain-containing protein, affects rice quality and yield. J. Integr. Plant Biol. 62, 948–966. doi: 10.1111/jipb.12866
Wang, J. C., Xu, H., Zhu, Y., Liu, Q. Q., Cai, X. L. (2013). OsbZIP58, a basic leucine zipper transcription factor, regulates starch biosynthesis in rice endosperm. J. Exp. Bot. 64, 3453–3466. doi: 10.1093/jxb/ert187
Wei, X., Jiao, G., Lin, H., Sheng, Z., Shao, G., Xie, L., et al. (2017). GRAIN INCOMPLETE FILLING 2 regulates grain filling and starch synthesis during rice caryopsis development. J. Integr. Plant Biol. 59, 134–153. doi: 10.1111/jipb.12510
Wu, M., Ren, Y., Cai, M., Wang, Y., Zhu, S., Zhu, J., et al. (2019). Rice FLOURY ENDOSPERM10 encodes a pentatricopeptide repeat protein that is essential for the trans-splicing of mitochondrial nad1 intron 1 and endosperm development. New Phytol. 223, 736–750. doi: 10.1111/nph.15814
Xiong, Y., Ren, Y., Li, W., Wu, F., Yang, W., Huang, X., et al. (2019). NF-YC12 is a key multi-functional regulator of accumulation of seed storage substances in rice. J. Exp. Bot. 70, 3765–3780. doi: 10.1093/jxb/erz168
Xu, J. J., Zhang, X. F., Xue, H. W. (2016). Rice aleurone layer specific OsNF-YB1 regulates grain filling and endosperm development by interacting with an ERF transcription factor. J. Exp. Bot. 67, 6399–6411. doi: 10.1093/jxb/erw409
Yagi, Y., Hayashi, S., Kobayashi, K., Hirayama, T., Nakamura, T. (2013). Elucidation of the RNA recognition code for pentatricopeptide repeat proteins involved in organelle RNA editing in plants. PloS One 8, e57286. doi: 10.1371/journal.pone.0057286
Yang, J., Luo, D., Yang, B., Frommer, W. B., Eom, J. S. (2018). SWEET11 and 15 as key players in seed filling in rice. New Phytol. 218, 604–615. doi: 10.1111/nph.15004
Yang, H., Wang, Y., Tian, Y., Teng, X., Lv, Z., Lei, J., et al. (2022a). Rice FLOURY ENDOSPERM22, encoding a pentatricopeptide repeat protein, is involved in both mitochondrial RNA splicing and editing and is crucial for endosperm development. J. Integr. Plant Biol. doi: 10.1111/jipb.13402
Yang, W., Xu, P., Zhang, J., Zhang, S., Li, Z., Yang, K., et al. (2022b). OsbZIP60-mediated unfolded protein response regulates grain chalkiness in rice. J. Genet. Genomics 49, 414–426. doi: 10.1016/j.jgg.2022.02.002
You, X., Zhang, W., Hu, J., Jing, R., Cai, Y., Feng, Z., et al. (2019). FLOURY ENDOSPERM15 encodes a glyoxalase I involved in compound granule formation and starch synthesis in rice endosperm. Plant Cell Rep. 38, 345–359. doi: 10.1007/s00299-019-02370-9
Yu, M., Wu, M., Ren, Y., Wang, Y., Li, J., Lei, C., et al. (2021). Rice FLOURY ENDOSPERM 18 encodes a pentatricopeptide repeat protein required for 5' processing of mitochondrial nad5 messenger RNA and endosperm development. J. Integr. Plant Biol. 63, 834–847. doi: 10.1111/jipb.13049
Zhang, G., Cheng, Z., Zhang, X., Guo, X., Su, N., Jiang, L., et al. (2011). Double repression of soluble starch synthase genes SSIIa and SSIIIa in rice (Oryza sativa l.) uncovers interactive effects on the physicochemical properties of starch. Genome 54, 448–459. doi: 10.1139/g11-010
Zhao, D., Zhang, C., Li, Q., Liu, Q. (2022). Genetic control of grain appearance quality in rice. Biotechnol. Adv. 60, 108014. doi: 10.1016/j.biotechadv.2022.108014
Keywords: grain chalkiness, rice, wbg1, white belly, mitochondrion, grain width
Citation: Wu M, Cai M, Zhai R, Ye J, Zhu G, Yu F, Ye S and Zhang X (2023) A mitochondrion-associated PPR protein, WBG1, regulates grain chalkiness in rice. Front. Plant Sci. 14:1136849. doi: 10.3389/fpls.2023.1136849
Received: 03 January 2023; Accepted: 17 February 2023;
Published: 09 March 2023.
Edited by:
Hongxiang Ma, Yangzhou University, ChinaReviewed by:
Changquan Zhang, Yangzhou University, ChinaBianyun Yu, National Research Council Canada (NRC-CNRC), Canada
Copyright © 2023 Wu, Cai, Zhai, Ye, Zhu, Yu, Ye and Zhang. This is an open-access article distributed under the terms of the Creative Commons Attribution License (CC BY). The use, distribution or reproduction in other forums is permitted, provided the original author(s) and the copyright owner(s) are credited and that the original publication in this journal is cited, in accordance with accepted academic practice. No use, distribution or reproduction is permitted which does not comply with these terms.
*Correspondence: Xiaoming Zhang, zhangxiaoming@zaas.ac.cn; Shenghai Ye, shenghaiye@163.com