- 1College of Grassland Science, Shanxi Agricultural University, Taigu, China
- 2State Key Laboratory of Vegetation and Environmental Change, Institute of Botany, The Chinese Academy of Sciences, Beijing, China
- 3College of Resources and Environment, University of Chinese Academy of Sciences, Beijing, China
Photosynthetic characteristics are widely used as indicators of plant responses to global environmental changes such as precipitation change and nitrogen (N) deposition increase. How different plant species respond physiologically to the future precipitation change combined with increasing N availability is largely unclear. A field experiment was conducted to study responses in seasonal and interannual leaf carbon (C) exchange of two dominant plant species, Leymus chinensis and Stipa grandis, to additional water (either as spring snow or as summer water) and N application in a semi-arid temperate steppe of China. Our results showed that spring snow and summer water addition both increased the maximum photosynthetic rate (Amax) of two dominant species. Such effect was likely caused by raised light saturation point, the maximum apparent quantum yield, stomatal conductance, and transpiration rate. The N application combined with spring snow or summer water addition both enhanced Amax of S. grandis in both experimental years, whereas N application only increased Amax of L. chinensis combined with summer water addition. Their responses were attributed to a concurrent increase in leaf N concentration (Nleaf) and decrease in leaf phosphorus (P) concentration (Pleaf), indicating that Nleaf and Pleaf affect photosynthetic characteristics to regulate leaf C exchange. Our results suggest that differentiated responses among different species in photosynthetic characteristics may lead to changes in ecosystem structure and functioning under increasing precipitation and N deposition.
Introduction
Ecosystem processes have been strongly modified by global change factors in terms of changes in both patterns and amount of precipitation and an increase in the amount of atmospheric nitrogen (N) deposition. Water and N are the two most limiting factors for plant growth and carbon (C) sequestration at both the leaf and ecosystem levels (Liang et al., 2020; Zhang et al., 2021). The two factors can also interact in intricate ways to influence the above-mentioned processes (Tang et al., 2017). By the end of the twenty-first century, rainfall is predicted to significantly increase in China with an increase of up to 30% in northern region (Cholaw et al., 2003; Chen, 2013) and snowfall is also predicted to greatly increase in the near future (Ma et al., 2012). Generally, rainfall is far more important than the snowfall in terms of their relative amount in the total precipitation in Inner Mongolia (Zhang et al., 2015). In addition, changes in precipitation amount will inevitably influence soil water content and nutrient availability, resulting in alteration in photosynthesis, and consequently affect plant growth (Davis et al., 1999; Ashraf and Harris, 2013). Besides, increasing N deposition due to industrialization globally and intensified use of N fertilizers, especially in agricultural systems will finally affect biogeochemical processes by changing soil N availability and other soil physicochemical properties (Galloway et al., 2008). Specifically, N deposition could induce water stress suppressing stomatal conductance and transpiration and further influence leaf C exchange (Zhang B. et al., 2017).
Leaf photosynthetic characteristics including photosynthetic capacity (i.e., the maximum photosynthetic rate, Amax), stomatal conductance, and transpiration rate can reflect plant responses to environmental changes and play fundamental roles in plant growth, which can further impact ecosystem structure and functioning (Xu et al., 2013). Plant chemistry, such as leaf N concentration (Nleaf) and leaf phosphorus (P) concentration (Pleaf), can be regarded as critical indicators for the response of different plants to those global change factors such as increasing N deposition and precipitation alteration (Reich, 2005). In addition, Nleaf and Pleaf are tightly correlated with the photosynthetic rate because they are important constituents for the synthesis of metabolic enzymes, chlorophyll, and other relevant photosynthetic apparatus (Gao et al., 2018; Zhong et al., 2018). Previous studies have shown that there are generally positive effects of N addition on plant photosynthesis (Zhang et al., 2008; Hou et al., 2019; Liang et al., 2020), although insignificant or even negative effects are also reported (Reich et al., 2003; Lin et al., 2017; Zhai et al., 2017). Therefore, it is highly needed to study the effects of N application on the seasonal and interannual variation in photosynthetic characteristics of different plant species, especially when combined with changes in water supply.
Dominant species generally determine community structure, function, and dynamics (Orwin et al., 2014; Mason et al., 2016), and they may have a broader range of physiology and therefore play a damping role on ecosystem fluctuation under future global changes (Norberg et al., 2001; Jump and Penuelas, 2005). In Inner Mongolia grasslands, Leymus chinensis and Stipa grandis are two dominant plant species in the typical steppe. They have distinct morphological and physiological traits with L. chinensis requiring relatively wetter and more fertile habitats than S. grandis (Chen et al., 2005). Therefore, the two species may respond differently to changes in water and N supply. Although the two species have established a stable balance by competing for limited resources when they co-exist in a community, changes in precipitation and N deposition may disturb and break this balance by affecting their relative competitiveness. Changes in their competitiveness inevitably impact their distribution, then reshape the community by shift in species composition, and, consequently, affect C sequestration and other processes at the ecosystem level (Walther et al., 2002; Still et al., 2003; Niu et al., 2006). Our previous study found that L. chinensis showed stronger responses than S. grandis in terms of abundance and biomass production under increasing precipitation and enhanced N availability in the semi-arid steppe (Zhang et al., 2021). However, little is known about the underlying mechanisms such as their responses in leaf photosynthetic characteristics of the two species under future scenarios of precipitation change and N deposition increase.
In this study, we would like to examine how plants, especially for the two dominant species (L. chinensis and S. grandis) in a typical steppe, respond to changes in precipitation regime either by increasing spring snow or by increasing summer rainfall interacted with N addition in terms of leaf C exchange capacity based on the construction of light response curves. We also aimed to understand what were the underlying mechanisms that would induce these responses. We hypothesized that (1) spring snow addition and summer water addition would have significantly different effects on leaf C exchange of the two species; (2) N addition would affect the leaf C exchange of the two species differently under spring snow and summer water addition; and (3) L. chinensis would respond stronger than S. grandis in the N uptake, photosynthetic characteristics, and would thus shift community structure and further influence ecosystem functioning under future global change scenarios.
Materials and Methods
Site Description
This study was conducted in a typical steppe located near the Inner Mongolia Grassland Ecosystem Research Station, Institute of Botany, Chinese Academy of Sciences (43°33′N and 116°40′E; 1,251 m above sea level). The studied typical steppe is primarily dominated by two grass species, namely Leymus chinensis and Stipa grandis. The regional monsoon climate in the study area is characterized by cold, dry winters and warm, wet summers. The long-term mean annual temperature (1970–2013) is 0.4°C (ranging from −24.1°C in January to 19.6°C in July). The mean annual precipitation is 333.3 mm (1982–2013), and the majority of precipitation occurs in the form of rainfall (91.9%, ~280.5 mm from May to September) with relatively small snowfall (8.1%) (Zhang et al., 2021).
Experimental Design and Treatments
A randomized block design was used in the experiment with treatments of N and water application. For this study, there were three replicates which were set up in the site and six treatments were involved. Therefore, totally 18 plots (5 m × 5 m) were included in this study with at least 1-m distance between adjacent plots. The six treatments were as follows: no water supply and no N application (control; N0W0), spring snow addition (N0W1), summer water addition (N0W2), N application (N1W0), spring snow and N application (N1W1), and summer water and N application (N1W2). Approximately 25 mm water equivalent of snow from the nearby area was evenly added to each spring snow plot in early March. Totally, 100 mm water from the nearby river was added to each summer rainfall plot yearly with a handheld sprinkler irrigation system, starting on June 15, 2010 (10 mm weekly). For N application, 10 g N m−2 was added to the plots in the form of urea in early July each year starting in 2009. This study was conducted for two growing seasons from 2012 to 2013.
Leaf C Exchange
Either the first or the second fully extended and intact leaf of each studied plant species was selected from each plot for leaf C exchange measurement. Measurements were taken between 08:00 am and 11:30 am once every 2 weeks from the end of May to the end of September with nine-time measurements across the years 2012 and 2013. Net photosynthetic rate (Pn), stomatal conductance, and transpiration rate were recorded using a LI-6400 portable photosynthesis system (Li-Cor Inc., Lincoln, NE, USA) with a red–blue LED source. Light response curves were obtained based upon Pn measurements along changes of photosynthetically active radiation (PAR) from 0 to 2,000 μmol m−2 s−1 (with the sequence of 2,000, 1,500, 1,200, 1,000, 800, 500, 300, 200, 150, 100, 50, 20, and 0 μmol m−2 s−1) using the built-in light source in the natural condition. Before the measurements, leaf was adapted to light under 2,000 μmol m−2 s−1 and then the data were logged in turn when the parameters were stable with ΔCO2 value fluctuation of <0.2 μmol mol−1 and the Pn value was stable at one after the decimal point. By fitting data to a quadratic equation (Equation 1), we determined the following physiological parameters of the two target plants, including the maximum photosynthetic rate (Amax) representing plant photosynthetic capacity, light saturation point representing the value of PAR when photosynthesis maintains relative high level without further change, light compensation point representing photosynthesis that will be higher than respiration beyond this value of PAR, the maximum apparent quantum yield representing the efficiency of light utilization in photosynthesis, and respiration (Long et al., 1993).
where Pn is the net photosynthetic rate (μmol CO2 m−2 s−1), Amax is the maximum photosynthetic rate (μmol CO2 m−2 s−1), AQE is the maximum apparent quantum yield of CO2 (μmol CO2 mol−1 photons), PAR is the photosynthetically active radiation (μmol m−2 s−1), k is the convexity coefficient (0 < k <1), and R is the rate of dark respiration (μmol CO2 m−2 s−1).
Soil Temperature and Soil Moisture
Soil temperature at 5-cm depth was recorded using the TH-212 portable intelligent data logger (Hichance Inc., Haidian, Beijing, China) along with the photosynthetic measurement. Simultaneously, soil moisture to 10-cm depth was determined using a TDR-200 probe (Spectrum Technologies Inc., Plainfield, IL, USA).
Leaf N and P Concentrations
To determine leaf N and P concentrations (Nleaf and Pleaf), fresh leaves of L. chinensis and S. grandis were collected during peak plant growth in August 2012 and 2013, oven-dried at 65°C for ~48 h, and then ground to a fine powder using a ball mill (Retsch MM 400; Retsch, Haan, Germany). The Nleaf was determined using an Elemental Analyzer Vario Macro Cube model with the dry combustion method (Elementar Analysensysteme GmbH, Germany). The Pleaf was determined using the molybdenum blue colorimetric method at 880 nm after digesting the samples in a mixture of concentrated sulfuric acid and hydrogen peroxide (Tan et al., 2013).
Statistical Analysis
Linear mixed models were used to determine the main effects of water, and N application and their interactive effects on the two dominant species (L. chinensis and S. grandis) in both growing seasons, separately. All response variables were checked for normality and homogeneity of model residues, and log10 or sqrt transformation was used if necessary.
We analyzed spring snow with or without N application treatments (N0W0, N0W1, N1W0, and N1W1) and summer water with or without N application treatments (N0W0, N0W2, N1W0, and N1W2) as different treatment levels, respectively. In the model, water including spring snow or summer water addition, and N application were treated as fixed terms. The sampling date was treated as a random term. The Amax, light saturation point, light compensation point, the maximum apparent quantum yield, respiration, stomatal conductance, and transpiration rate were treated as response variables. The response variable model was selected by comparing several models according to maximum-likelihood estimation with the lowest AIC value (West, 2009). The final model estimates reported were based on restricted maximum-likelihood estimates.
Two-way analysis of variance (ANOVA) was used to determine the main effects of various treatments on Nleaf and Pleaf. Student's t-tests were used to compare the differences between spring snow and summer water addition and the differences in N application between spring snow and summer water addition. The relationships of Amax with soil moisture, soil temperature, and precipitation (accumulated from previous November to October amount) were explored using curve fitting regression analysis. All data were analyzed using SPSS 23.0 for Windows (SPSS Inc., Chicago, IL, USA).
Results
Light Response Curves
By pooling data of measurements in each growing season, the average net photosynthetic rate (Pn) of the two species, L. chinensis and S. grandis, all showed a saturating response pattern to change of PAR, that is, increased rapidly with PAR when PAR was relatively low, but leveled off when PAR reached a saturation point regardless of treatments in the two growing seasons (Figure 1). The slope of light response curves of both plant species showed no differences when PAR was at the light limitation stage (≤ 200 μmol m−2 s−1). However, Pn of the two species differentiated when PAR was at the light saturation stage with summer water addition showing greater increasing effects than spring snow addition with or without N addition. Besides, the annual average Pn of L. chinensis was greater than that of S. grandis in 2012 (P < 0.001), but turns opposite in 2013 when the value of PAR was > 800 μmol m−2 s−1 (P < 0.01).
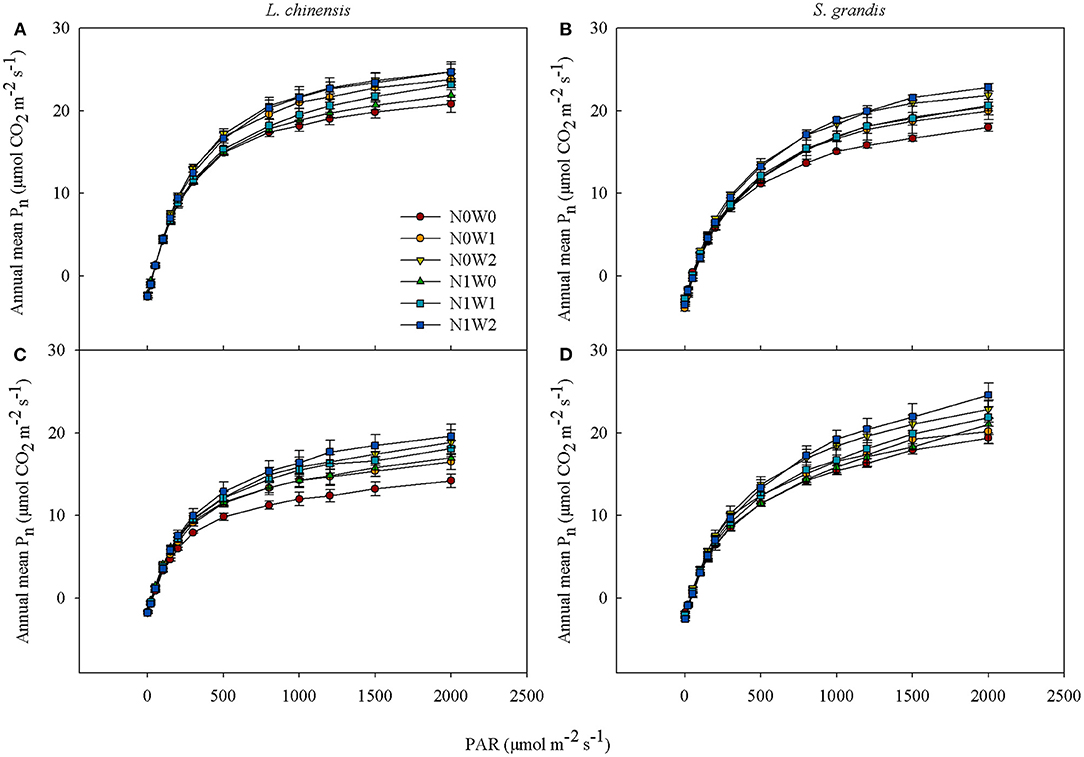
Figure 1. Average values of light response curves of Leymus chinensis (left panels) and Stipa grandis (right panels) across growing seasons in 2012 (A,B) and 2013 (C,D), respectively. Values represent mean ± SE (n = 3). Treatments are designated as follows: N0W0, no water supply and no N application (control); N0W1, spring snow addition; N0W2, summer water addition; N1W0, N application; N1W1, spring snow and N application; and N1W2, summer water and N application.
The effects of spring snow addition showed remarkable interannual variations in Amax, light saturation point, light compensation point, the maximum apparent quantum yield, and respiration of the two dominant species between the two growing seasons (Tables 1, 2). Spring snow addition significantly increased Amax of L. chinensis by 6.9% and that of S. grandis by 5.8% in 2012 and light saturation point of L. chinensis in 2013, but the effect was insignificant on other variables in either year (Figures 2, 3, Tables 1, 2). The effects of summer water addition on Amax of L. chinensis and S. grandis were more pronounced than spring snow addition in both growing seasons (Figures 4A,B). Specifically, summer water addition enhanced Amax of L. chinensis by 18.7% in 2012 and 20.3% in 2013 and increased that of S. grandis by 15.1% in 2012 and by 17.4% in 2013 (Figures 2A,B, 3A,B, Tables 1, 2). Summer water addition also increased light saturation point of L. chinensis and S. grandis in both years (Figures 2C,D, 3C,D, Tables 1, 2). Summer water addition decreased light compensation point of S. grandis, but had insignificant effects on that of L. chinensis in both years. Besides, summer water addition increased the maximum apparent quantum yield of L. chinensis, but had no significant effects on respiration of both species in either year (Figures 2E–J, 3E–J, Tables 1, 2).
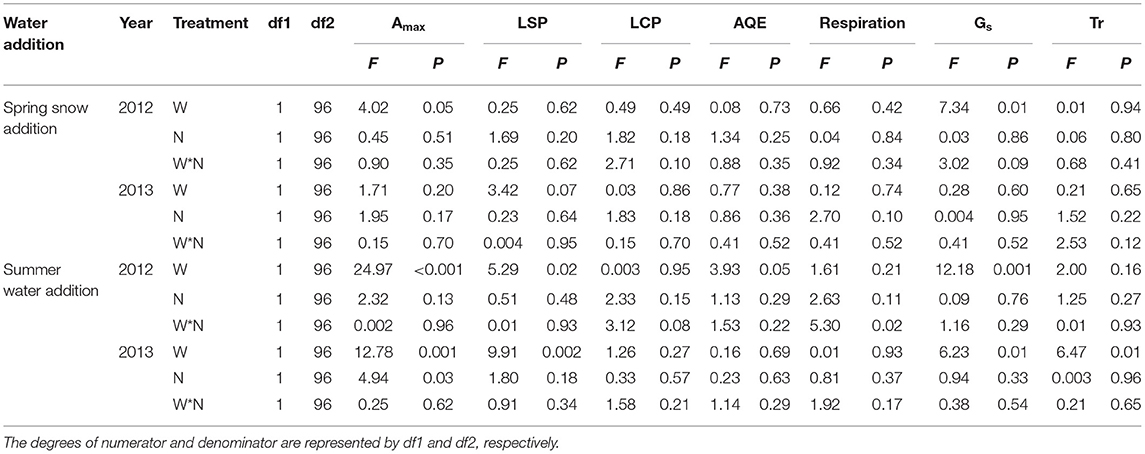
Table 1. Results (F- and P-values) of mixed-model analysis on the effects of water (W; including spring snow and summer water), N application (N), and their interactions on the maximum photosynthetic rate (Amax), light saturation point (LSP), light compensation point (LCP), maximum apparent quantum yield (AQE), respiration, stomatal conductance (Gs), and transpiration rate (Tr) of Leymus chinensis in 2012 and 2013.
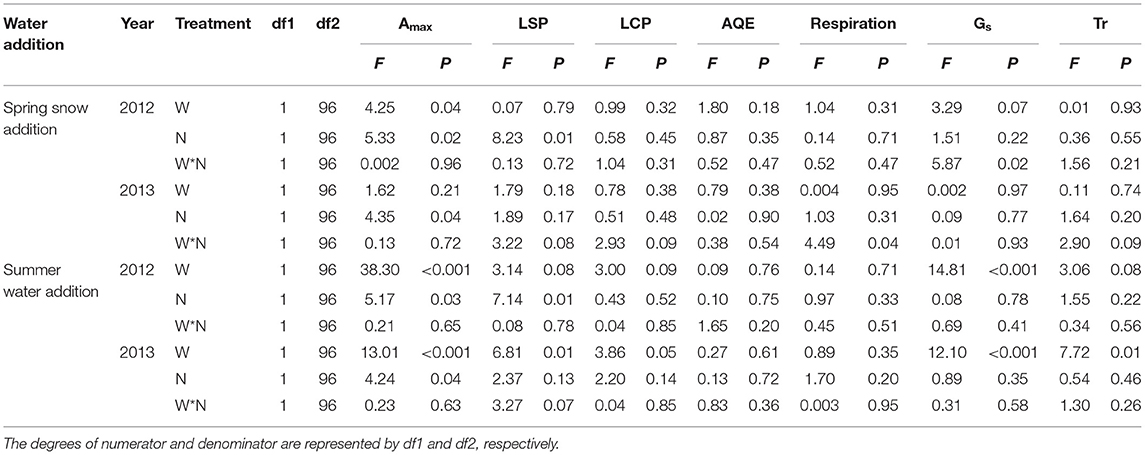
Table 2. Results (F- and P-values) of mixed-model analysis on the effects of water (W; including spring snow and summer water), N application (N), and their interactions on the maximum photosynthetic rate (Amax), light saturation point (LSP), light compensation point (LCP), maximum apparent quantum yield (AQE), respiration, stomatal conductance (Gs), and transpiration rate (Tr) of Stipa grandis in 2012 and 2013.
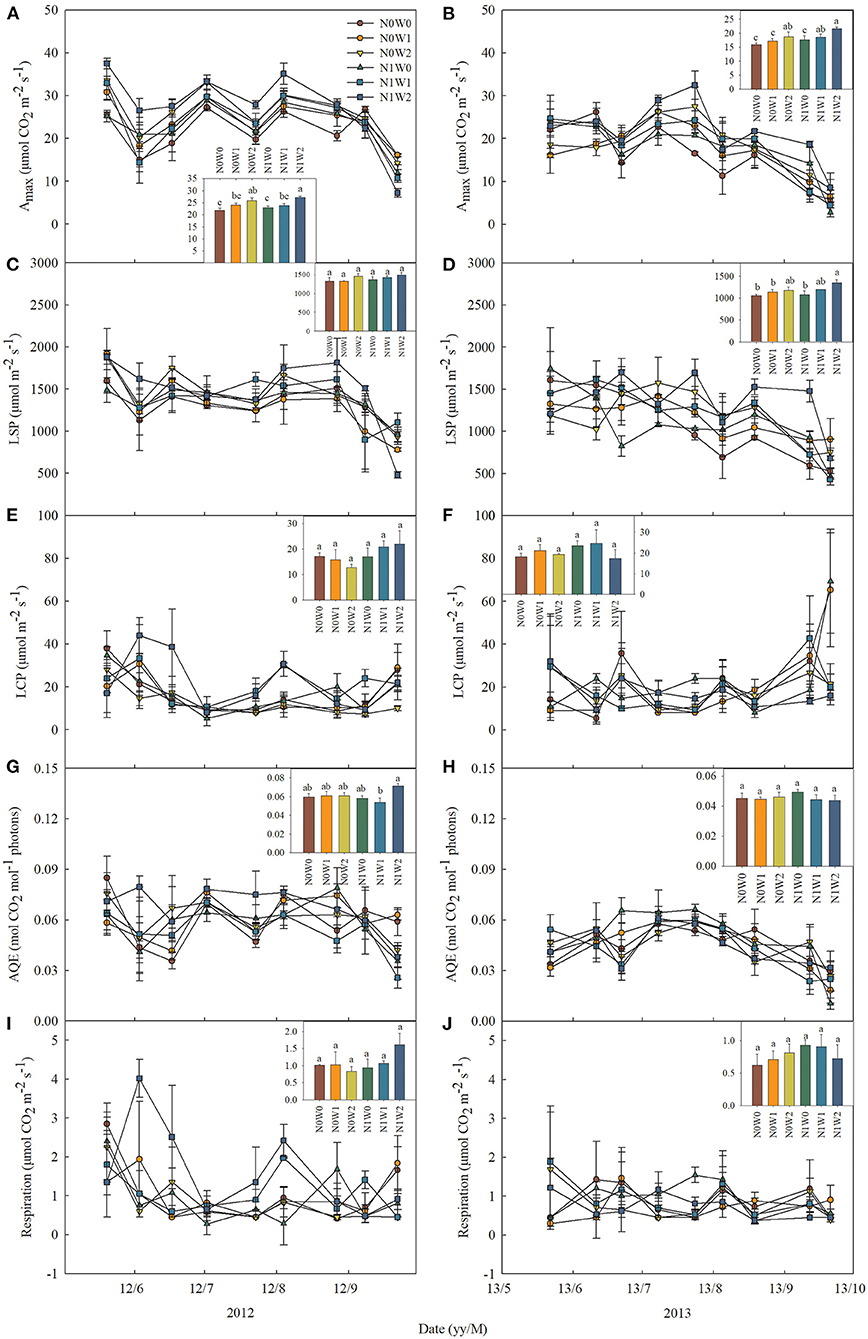
Figure 2. Seasonal dynamics of leaf photosynthetic characteristics of Leymus chinensis in 2012 (left panels) and 2013 (right panels), respectively, including (A,B) the maximum photosynthetic rate (Amax), (C,D) light saturation point (LSP), (E,F) light compensation point (LCP), (G,H) maximum apparent quantum yield (AQE), and (I,J) respiration. Inset figures show the average values across seasons with different letters indicating significant differences (P < 0.05) among the six treatments. Values represent mean ± SE (n = 3).
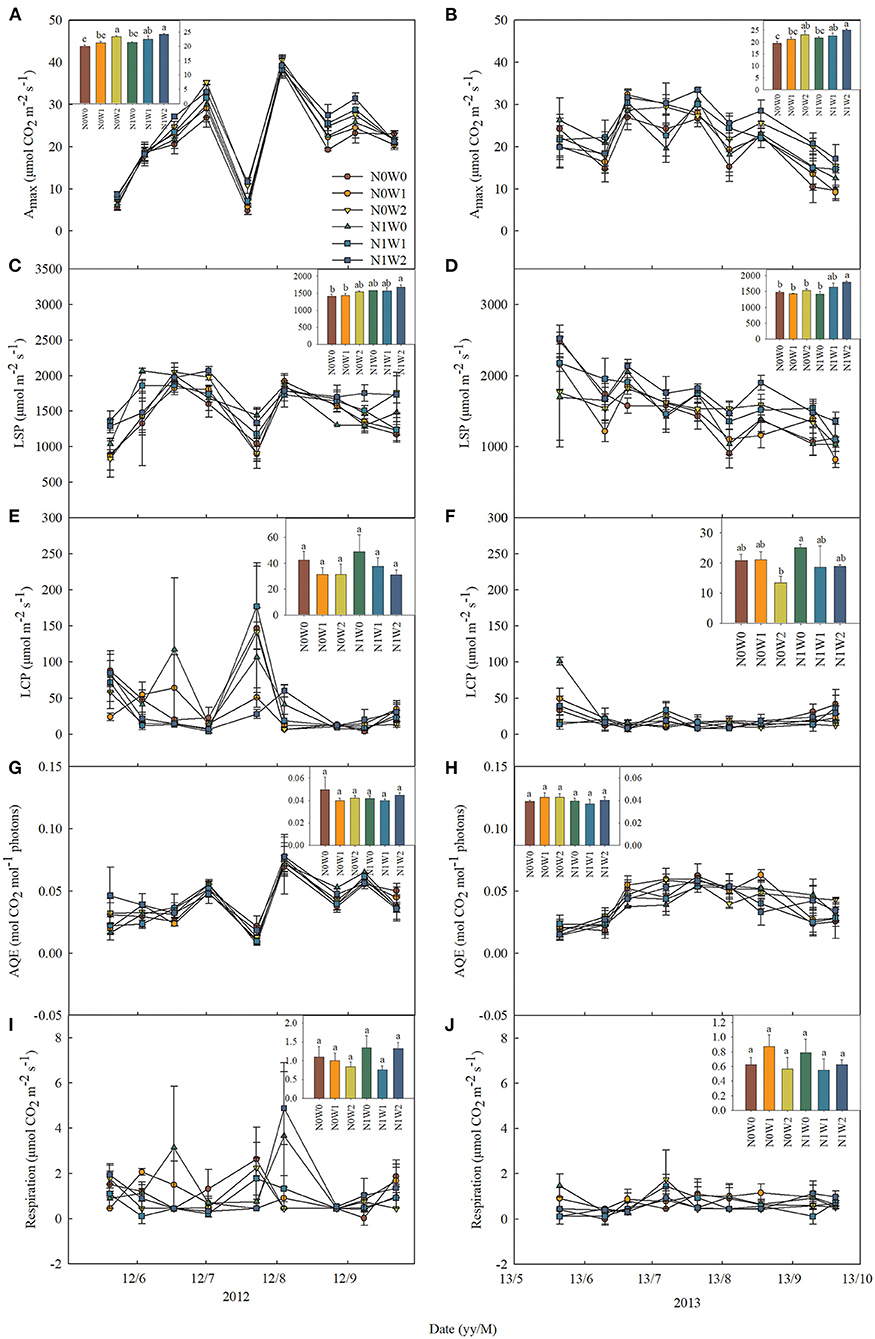
Figure 3. Seasonal dynamics of leaf photosynthetic characteristics of Stipa grandis in 2012 (left panels) and 2013 (right panels) including (A,B) the maximum photosynthetic rate (Amax), (C,D) light saturation point (LSP), (E,F) light compensation point (LCP), (G,H) maximum apparent quantum yield (AQE), and (I,J) respiration. Inset figures show the average values across seasons with different letters indicating significant differences (P < 0.05) among the six treatments. Values represent mean ± SE (n = 3).
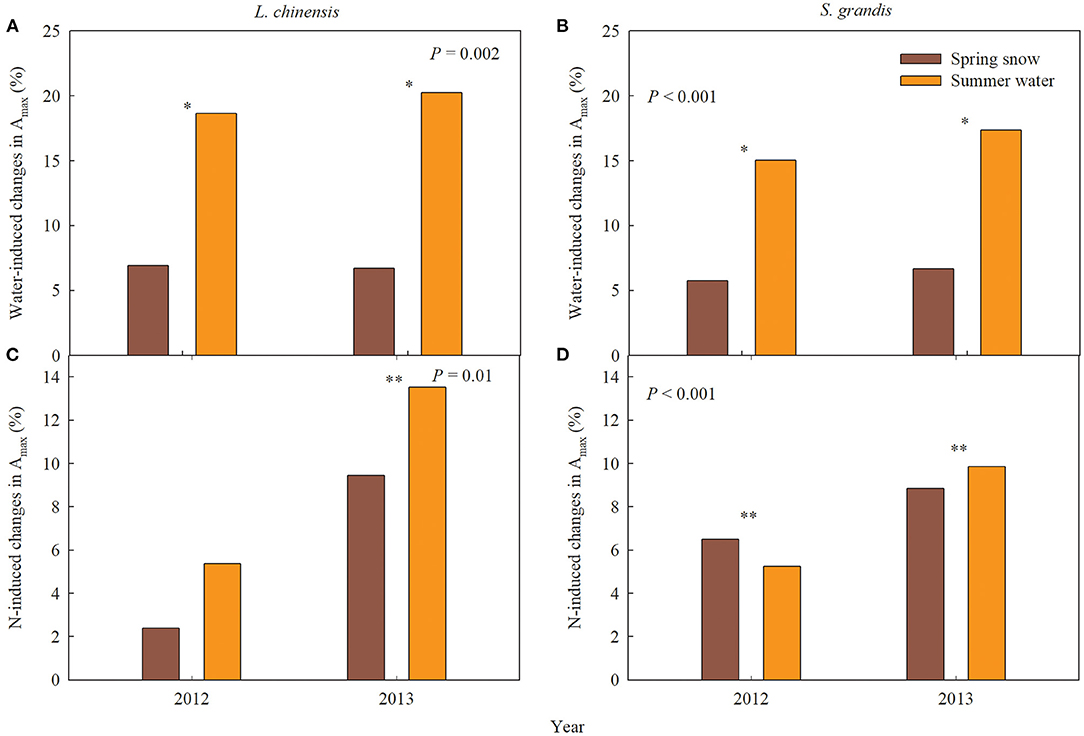
Figure 4. Water- and nitrogen (N)-induced changes in leaf carbon exchange of Leymus chinensis and Stipa grandis in 2012 and 2013, respectively. (A,B) Spring snow- and summer water-induced changes in the maximum photosynthetic rate (Amax); (C,D) N-induced changes in the maximum photosynthetic rate (Amax). P-values in the figures represent the difference between spring snow or summer water effects across 2 years. ** and * on each group represent significant differences in t-test, at P < 0.01 and P < 0.05 levels, respectively.
Applying N combined with spring snow addition increased the Amax of S. grandis by 6.5% in 2012 and by 8.8% in 2013 (Figure 4D). They also increased respiration of L. chinensis in 2013 and light saturation point of S. grandis in 2012, but had no significant effects on other photosynthetic parameters of the two species in both growing seasons (Figures 1, 2, Tables 1, 2). Applying N with summer water addition increased Amax of L. chinensis by 13.5% in 2013 and that of S. grandis by 5.2% in 2012 and by 9.9% in 2013. They also enhanced light saturation point of S. grandis in 2012 (Figures 2, 3, Tables 1, 2). The responses of Amax to N addition combined with summer water addition were stronger than with spring snow addition in 2013 for L. chinensis, but were weaker in 2012 for S. grandis (Figures 4C,D).
There were significant interactive effects between N addition and spring snow addition on respiration of S. grandis in 2013 and between N addition and summer water addition on respiration of L. chinensis in 2012.
Leaf Physiological Traits
Spring snow addition increased stomatal conductance of both species, but decreased Pleaf of S. grandis in 2012 and had no significant effects on other variables (Figures 5A–D, Tables 1, 2). Summer water addition increased stomatal conductance of both species, respectively, in 2012 and 2013 (Figures 5A,B). It also enhanced transpiration rate of L. chinensis in 2013 and that of S. grandis in 2012 and 2013 (Figures 5C,D, Tables 1, 2), but decreased Nleaf of L. chinensis in 2012 (Figures 5E,F, Table 3).
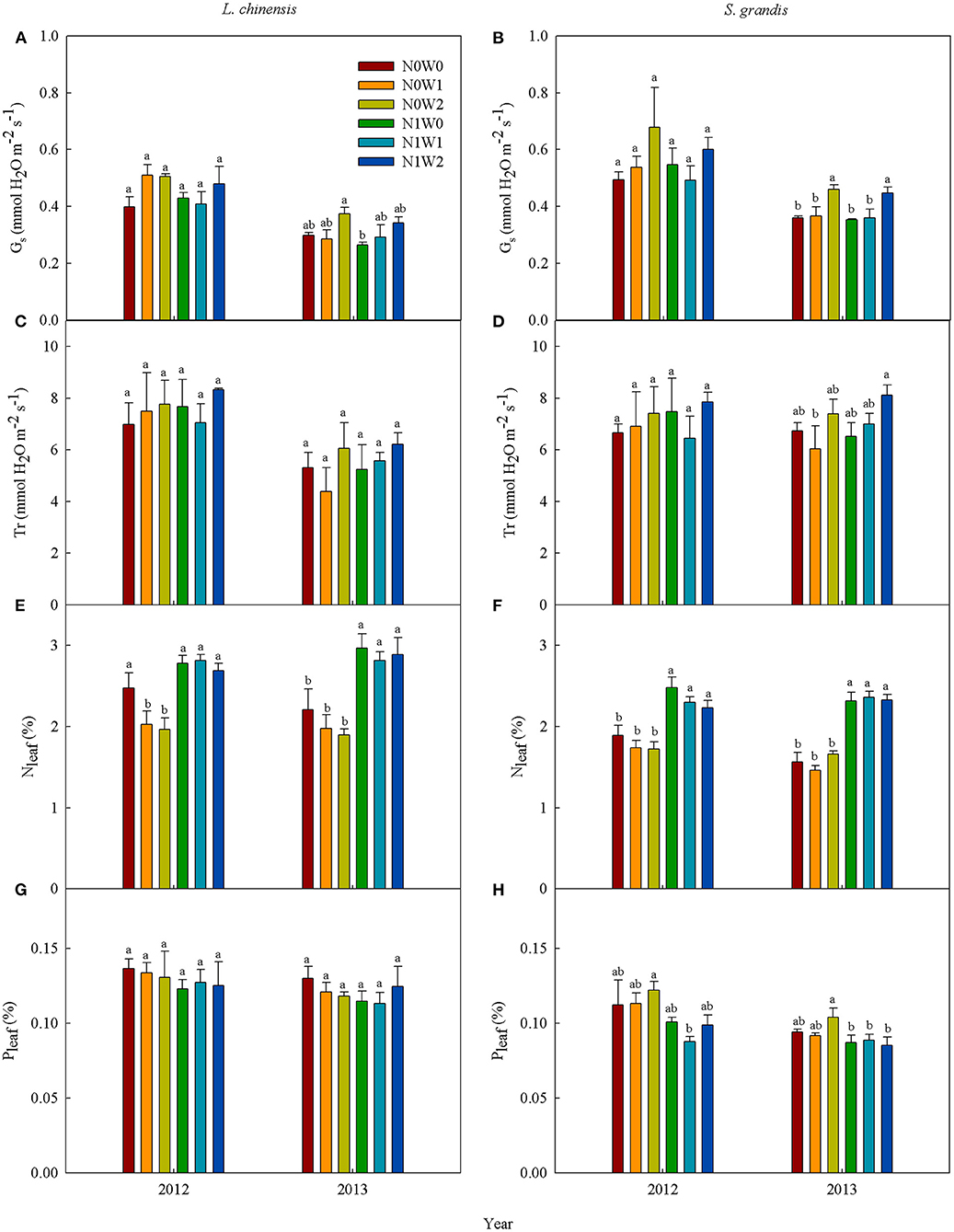
Figure 5. Mean values of leaf photosynthetic characteristics of Leymus chinensis (left panels) and Stipa grandis (right panels) across seasons in 2012 and 2013, respectively. (A,B) Stomatal conductance (Gs), (C,D) transpiration rate (Tr), (E,F) leaf N concentration (Nleaf), and (G,H) leaf P concentration (Pleaf). Different letters indicate significant differences (P < 0.05) among six treatments. Values represent mean ± SE (n = 3).
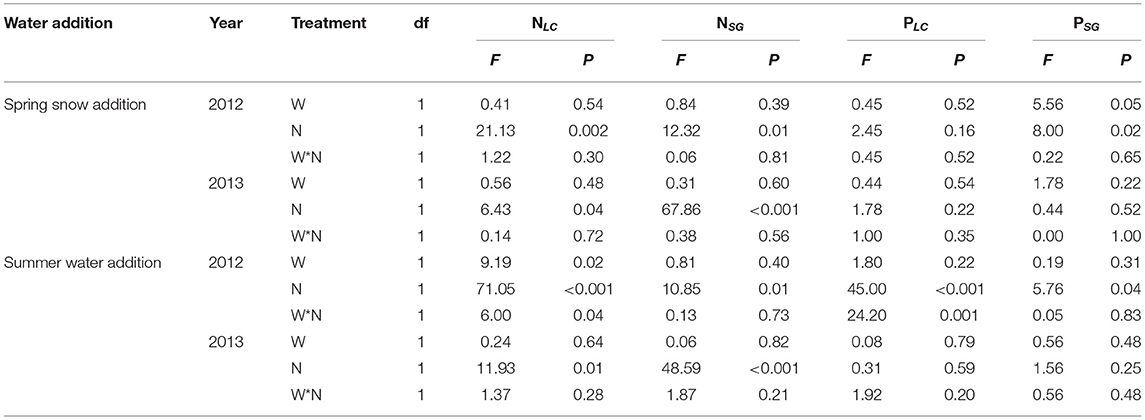
Table 3. Results (F- and P-values) of two-way ANOVA on the effects of water (W; including spring snow and summer water), N application (N), and their interactions on leaf N concentration (Nleaf, %) and leaf P concentration (Pleaf, %) of Leymus chinensis (NLC and PLC) and Stipa grandis (NSG and PSG) in 2012 and 2013.
When combined with spring snow addition, the application of N did not affect stomatal conductance and transpiration rate of either species in either growing season (Figures 5A,B, Tables 1, 2). Besides, N addition significantly increased Nleaf of both species, respectively, in 2012 and 2013, but decreased Pleaf of S. grandis significantly in 2012 (Figures 5E–H, Table 3).
When combined with summer rainfall, the application of N had insignificant effects on stomatal conductance and transpiration rate of the two species over the two growing seasons (Tables 1, 2). Besides, it enhanced Nleaf of L. chinensis and S. grandis in both years, but decreased Pleaf of L. chinensis and S. grandis in 2012 (Figures 5E–H).
There were significant interactions between spring snow and N application on the stomatal conductance of S. grandis and between summer water addition and N application on Nleaf and Pleaf of L. chinensis in 2012.
Effects of Abiotic Factors on Amax
The Amax of both species showed a logarithmic relationship with volumetric soil moisture in all treatments across 2012 and 2013, except N1W0 and N1W1 treatments for L. chinensis and N1W1 treatment for S. grandis in the later growing season (from end-August to end-September) (Figures 6A,C,E,G). The Amax of L. chinensis and S. grandis both showed an exponential growth relationship with soil temperature in all treatments across 2012 and 2013, except N1W0 treatment for L. chinensis and N0W0, N1W0, and N1W1 treatments for S. grandis in the later growing season (Figures 6B,D,F,H).
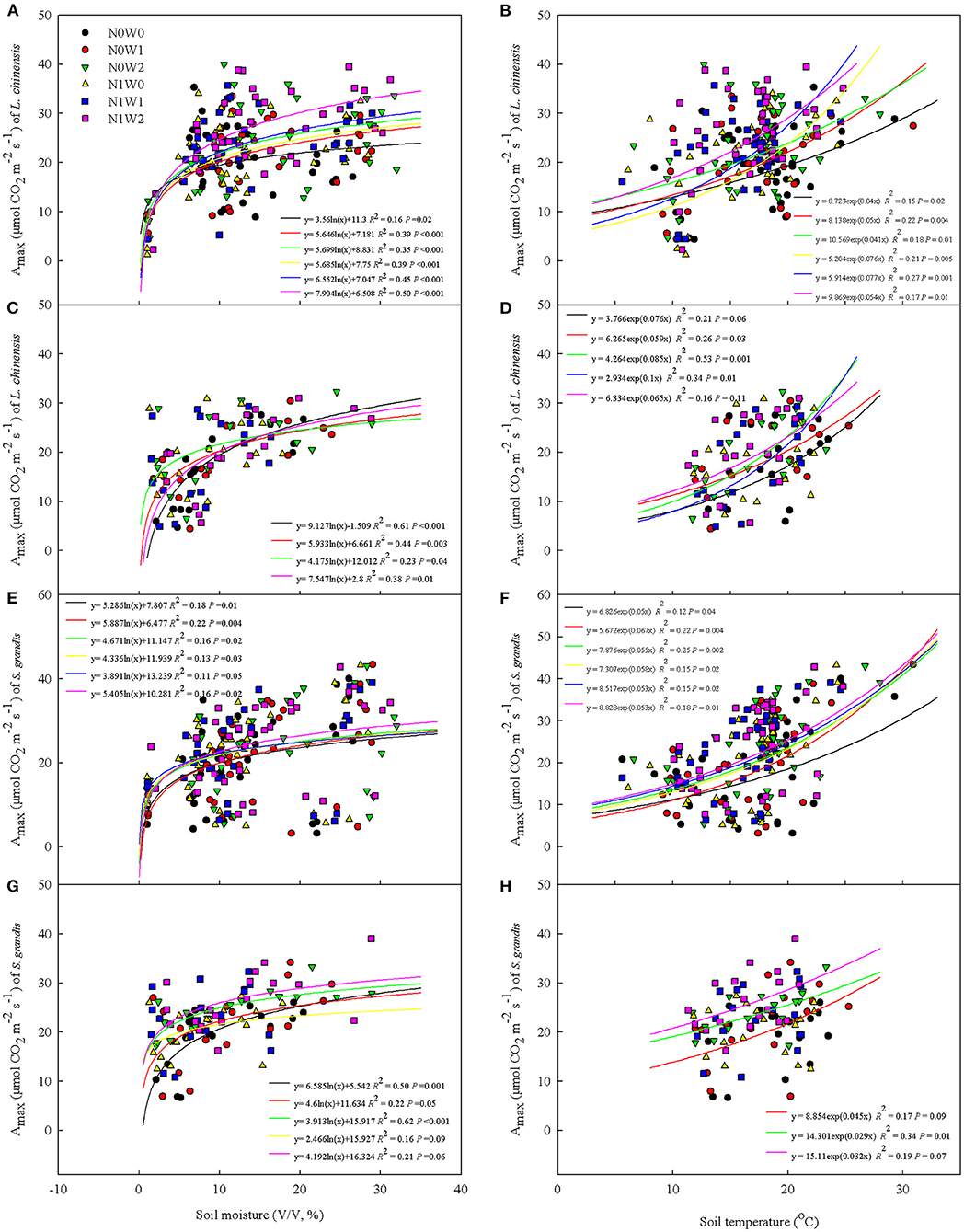
Figure 6. Relationships of the maximum photosynthetic rate (Amax) with soil moisture (left panels) and soil temperature (right panels) of two dominant species across 2012 and 2013. (A,B) Amax of Leymus chinensis in the early growing season (from end-May to mid-August), (C,D) Amax of Leymus chinensis in the later growing season (from end-August to end-September), (E,F) Amax of Stipa grandis in the early growing season (from end-May to mid-August), and (G,H) Amax of Stipa grandis in the later growing season (from end-August to end-September).
In addition, the Amax showed a significantly logarithmic relationship with increases in precipitation under spring snow and summer water addition treatments regardless of N application for L. chinensis, but only a marginally logarithmic relationship with precipitation for S. grandis under summer water addition with non-N application over the two growing seasons (Figure 7).
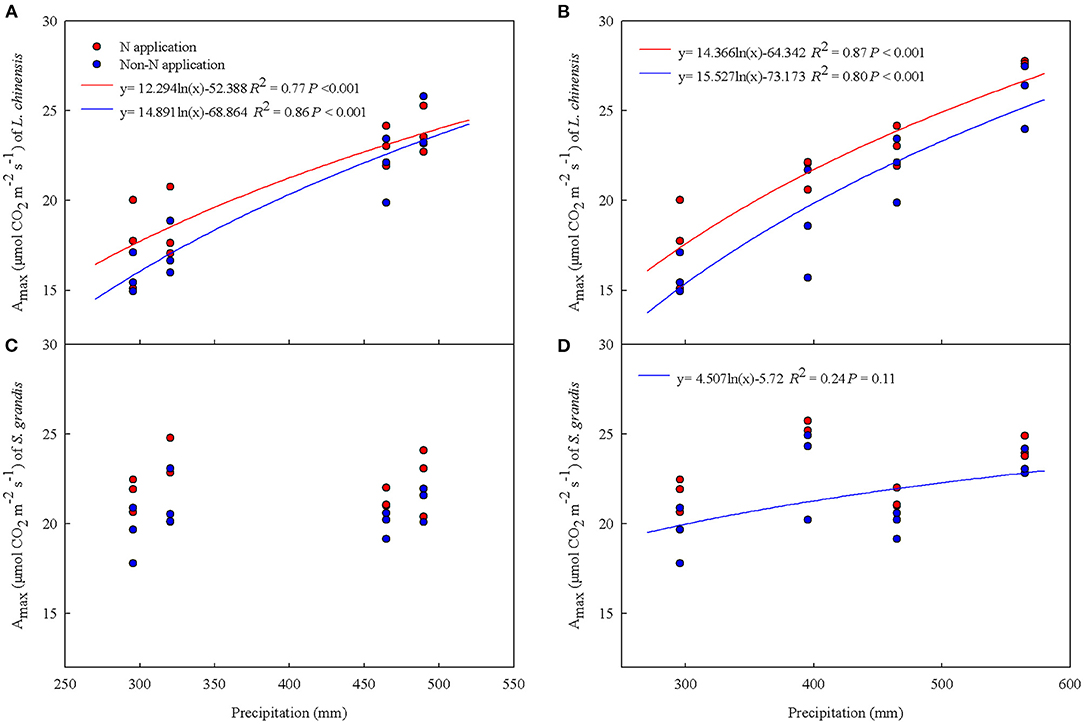
Figure 7. Relationships of the maximum photosynthetic rate (Amax) of Leymus chinensis and Stipa grandis with precipitation (from previous November to October amount) under water addition treatment with N application and non-N application across 2012 and 2013. (A) Amax of Leymus chinensis under spring snow addition treatment, (B) Amax of Leymus chinensis under summer water addition treatment, (C) Amax of Stipa grandis under spring snow addition treatment, and (D) Amax of Stipa grandis under summer water addition treatment.
Discussion
Effects of Precipitation Increase on Leaf C Exchange
Leaf photosynthetic characteristics reflect physiological adaptations of plants to changes in a specific environmental condition. Because water is a key limiting factor for plant growth, especially in arid and semi-arid areas, changes in precipitation (in the form of either spring snowfall or summer rainfall) are expected to affect plant growth via changing soil water availability and leaf photosynthetic characteristics such as Amax and stomatal conductance (Nippert et al., 2009). Most previous studies have demonstrated positive effects of increasing water supply on leaf photosynthesis (Song et al., 2016; Ali et al., 2018; Hui et al., 2018), although inconsistent results, ranging from being weak to negative, are also reported (Hartman et al., 2012; Yu et al., 2015). Besides, previous studies have also shown that the magnitude of changes in leaf photosynthetic characteristics induced by precipitation alteration depends closely on the amount of background precipitation (Fay et al., 2011; Zheng et al., 2011).
In this study, the effects on leaf C exchange varied remarkably during growing seasons with the form of the additional water either as spring snow or as summer water. Our results showed that the effect of increasing water on leaf photosynthetic characteristics was generally weaker for spring snow increase than for summer water increase. The most probable reason is that snowfall takes only a small part of the total annual precipitation in the study area. Besides, it occurs generally in the non-growing seasons, which mismatch the time of plant growth. A previous study showed that grassland was increasingly vulnerable to direct effects of climate extremes through affecting ecosystem function and phenology as changes in key traits of plant species (Knapp et al., 2020). We found that increases in spring snowfall and summer rainfall could significantly increase the capacity of both species' C assimilation by increasing light saturation point, stomatal conductance, and transpiration rate while decreasing light compensation point. Therefore, our findings highlight the importance that responses of both the species to future precipitation increase can be reflected via changes in key photosynthetic properties, which may finally affect ecosystem functioning and phenology.
In addition, previous studies showed that predicting responses of leaf C exchange and subsequent responses of semi-arid ecosystems C budget to precipitation required knowledge of timing of precipitation change (Craine et al., 2012; Post and Knapp, 2020). In this study, our results also showed that impacts of spring snow and summer water addition on leaf C exchange of the two dominant species varied among different years. The effects of spring snow addition were greater in 2012 when the background snowfall was around the long-term average value of the study area, but weaker in 2013 when the background snowfall was far greater than its average level. Similarly, the effects of summer water addition were weaker in 2012 when the background precipitation was higher than the long-term average of the study area, but stronger in 2013 when the background precipitation was at its average level. These results suggest that the enhancive effects of precipitation increase on leaf C exchange of the two dominant species depend highly on the amount of background precipitation with the effect that may be weakened when the background precipitation is high.
According to the results from a previous study, precipitation events affect ecological processes by soil moisture pulses in arid and semi-arid ecosystems (Post and Knapp, 2020), with soil moisture being able to explain the variation of leaf C assimilation as high as 52% (Fay et al., 2011). Our results also showed that there was a significantly positive relationship between Amax and soil moisture, which indicates that improvement in water supply on soil moisture could be an important underlying mechanism for the enhancement of the capacity of plant C assimilation by precipitation increase.
Effects of Additional N on Leaf C Exchange
Nitrogen supply affects plant growth and productivity, but the effect is more complex than expected and the mechanism on leaf photosynthetic characteristics is in fact not fully understood. Our results showed that N application along with spring snow addition increased the leaf C exchange capacity (Amax) of S. grandis and along with summer rainfall addition treatment significantly increased the leaf C exchange capacity of both species except the case for L. chinensis in 2012. The increasing effect of N addition might be a resultant of increasing light saturation point and Nleaf, but decreasing Pleaf as well, all of which were important physiological indicators of a plant's potential photosynthetic capacity (Luo et al., 2019). This also suggests that leaf C exchange of both the studied species is N-dependent and the N effect can be regulated by the water supply amount. A previous study showed that N application promoted phosphatase activity leading to P recycling stronger (Schleuss et al., 2020), which suggests that N addition aggravates the response of plant to P resulting in weakened response of leaf C exchange to N addition at our study site.
The significantly positive relationship between Amax and soil temperature partially explained the effect of N application on the capacity of plant C assimilation (Figure 6). With an increase in soil temperature and perhaps soil moisture as well, Amax was greater in N application than in non-N application plots for both species in the early growing season, but not later. These results suggest that N availability regulated water effects in the responses of plant performance mainly in the early growing season. In addition, the increasing effects of N application on leaf C exchange of the two species also changed when background precipitation varied (Figures 4C,D), indicating that the natural precipitation also adjusted the effect of N application, which might lead to limitation of other resources such as the availability of P in this semi-arid area.
Different Responses of Leaf C Exchange to Water and N Application
Different response patterns of the photosynthetic properties between the two dominant species under precipitation increase and N application can provide insights into the effects of climate changes on the structure of plant communities. Previous studies have shown that precipitation changes will significantly affect the plant photosynthesis and leaf level C exchange, which was corroborated in community-level CO2 exchange (Zhang R. et al., 2017), resulting in the variation of future population and community structure depending on the different magnitude of responses among the community compositional species (Silletti and Knapp, 2001; Nippert et al., 2009). In this study, although the patterns of light responding properties of the two species were similar to changes in spring snowfall and summer rainfall, the Amax of L. chinensis had an obviously higher increasing magnitude than that of S. grandis in both study years (Figures 4A,B), suggesting that the response of L. chinensis was stronger than that of S. grandis to change in precipitation in the study area. This may be because the relationships of Amax with soil moisture were more tightly related for L. chinensis than for S. grandis in both the early and later growing season. In addition, the Amax of L. chinensis was more tightly related with an increase in precipitation than that of S. grandis, suggesting that it is more conducive to the development of L. chinensis under the scenario of increasing precipitation in future. Moreover, responses of the Amax to N application were also greater for L. chinensis than for S. grandis under summer water addition treatment although only in 2013, while it was adverse under spring snow addition, suggesting that L. chinensis responses to N addition were more dependent on precipitation than S. grandis. The difference in the magnitude of responses between the two species may also result from their different adaptation strategies in terms of their rooting systems. S. grandis generally had fibrous rooting system distributing in relatively shallow soils, while L. chinensis showed strong rhizomatous rooting system which could distribute deeper in the soil profile (Chen et al., 2001; Zhang et al., 2021). Therefore, L. chinensis could be more beneficial than S. grandis for absorbing water and N resources from deeper soils, especially under the summer water addition. This corroborates results from previous studies which indicated that habitats for distribution of L. chinensis were generally wetter and more fertile than those of S. grandis (Chen et al., 2005).
Our results implicate that L. chinensis and S. grandis will respond differently to water and N availability increase due to their different root morphology and resource use strategy which corroborates the results of our earlier studies (Hasi et al., 2021; Zhang et al., 2021). Our previous work showed that the abundance and aboveground biomass of L. chinensis increased significantly under water addition with or without N application (Zhang et al., 2021), and this study suggests that physiological responses of that species may mirror those higher-order ecosystem responses. Therefore, different responses of two dominant species may lead to changes in the ecosystem structure and functioning when facing global change factors including both increasing precipitation and enhancive N deposition in this study area.
However, the sample size is relatively small in this study to fully explain the influencing mechanism of spring snow or summer water addition and N application on two dominant species leaf C exchange, although we tried to increase the monitoring frequency as much as possible. Therefore, more studies are needed to further explain the potential mechanism on the responses of plant performance to precipitation increase and N deposition in future.
Conclusion
Our results showed that summer water addition effects on leaf C exchange of L. chinensis and S. grandis were greater than spring snow addition. Correspondingly, N effects were more pronounced when combined with summer rainfall than spring snow addition by changing their key photo-physiological traits reflecting different adaptation strategies. This explains a physiological-based mechanism for potential transition in plant species composition as L. chinensis was more sensitive than S. grandis under water and N application. Therefore, differentiated responses of plant species in resource use and C assimilation may result in changes in community structure and further affect ecosystem functioning when facing global change scenarios such as increases in precipitation and N availability.
Data Availability Statement
The raw data supporting the conclusions of this article will be made available by the authors, without undue reservation.
Author Contributions
XZ conducted this study, made measurements, and wrote the draft of manuscript. PZ helped in the data analysis. JH initiated the experimental design and made final manuscript revision. All authors contributed to the article and approved the submitted version.
Funding
This study was financially supported by the National Natural Science Foundation of China (31800381 and 32071562), the Scientific and Technological Innovation Programs of Higher Education Institutions in Shanxi (2019L0366), the Research Project for Outstanding Doctor Work Award Fund in Shanxi Province (SXYBKY201746), the Science and Technology Innovation Fund of Shanxi Agriculture University (2017YJ11), and the Shanxi Province Graduate Education Innovation Project (2020BY054).
Conflict of Interest
The authors declare that the research was conducted in the absence of any commercial or financial relationships that could be construed as a potential conflict of interest.
Publisher's Note
All claims expressed in this article are solely those of the authors and do not necessarily represent those of their affiliated organizations, or those of the publisher, the editors and the reviewers. Any product that may be evaluated in this article, or claim that may be made by its manufacturer, is not guaranteed or endorsed by the publisher.
Acknowledgments
We thank the Inner Mongolia Grassland Ecosystem Research Station (IMGERS) for providing meteorological data in the study site. We also need to thank the subject editor and the three reviewers for their constructive and thoughtful comments which help us a lot in the manuscript revision.
References
Ali, S., Xu, Y., Jia, Q., Ma, X., Ahmad, I., Adnan, M., et al. (2018). Interactive effects of plastic film mulching with supplemental irrigation on winter wheat photosynthesis, chlorophyll fluorescence and yield under simulated precipitation conditions. Agr. Water Manage. 207, 1–14. doi: 10.1016/j.agwat.2018.05.013
Ashraf, M., and Harris, P. J. C. (2013). Photosynthesis under stressful environments: an overview. Photosynthetica 51, 163–190. doi: 10.1007/s11099-013-0021-6
Chen, H. P. (2013). Projected change in extreme rainfall events in China by the end of the 21st century using CMIP5 models. Chinese Sc. Bull. 58, 1462–1472. doi: 10.1007/s11434-012-5612-2
Chen, S., Bai, Y., Zhang, L., and Han, X. (2005). Comparing physiological responses of two dominant grass species to nitrogen addition in Xilin River Basin of China. Environ. Exp. Bot. 53, 65–75. doi: 10.1016/j.envexpbot.2004.03.002
Chen, S. H., Zhang, H., Wang, L. Q., Zhan, B. L., and Zhao, M. L. (2001). Root Systems of Grassland Plants in Northern China. Changchun: Jilin University Press.
Cholaw, B., Cubasch, U., Lin, Y. H., and Ji, L. R. (2003). The change of north China climate in transient simulations using the IPCC SRES A2 and B2 scenarios with a coupled atmosphere-ocean general circulation model. Adv. Atmos. Sci. 20, 755–766. doi: 10.1007/BF02915400
Craine, J. M., Nippert, J. B., Elmore, A. J., Skibbe, A. M., Hutchinson, S. L., and Brunsell, N. A. (2012). Timing of climate variability and grassland productivity. Proc. Natl. Acad. Sci. U.S.A. 109, 3401–3405. doi: 10.1073/pnas.1118438109
Davis, M. A., Wrage, K. J., Reich, P. B., Tjoelker, M. G., Schaeffer, T., and Muermann, C. (1999). Survival, growth, and photosynthesis of tree seedlings competing with herbaceous vegetation along a water-light-nitrogen gradient. Plant Ecol. 145, 341–350. doi: 10.1023/A:1009802211896
Fay, P. A., Blair, J. M., Smith, M. D., Nippert, J. B., Carlisle, J. D., and Knapp, A. K. (2011). Relative effects of precipitation variability and warming on tallgrass prairie ecosystem function. Biogeosciences 8, 3053–3068. doi: 10.5194/bg-8-3053-2011
Galloway, J. N., Townsend, A. R., Erisman, J. W., Bekunda, M., Cai, Z., Freney, J. R., et al. (2008). Transformation of the nitrogen cycle: recent trends, questions, and potential solutions. Science 320, 889–892. doi: 10.1126/science.1136674
Gao, L., Caldwell, C. D., and Jiang, Y. (2018). Photosynthesis and growth of camelina and canola in response to water deficit and applied nitrogen. Crop Sci. 58, 393–401. doi: 10.2135/cropsci2017.07.0406
Hartman, J. C., Nippert, J. B., and Springer, C. J. (2012). Ecotypic responses of switchgrass to altered precipitation. Funct. Plant Biol. 39, 126–136. doi: 10.1071/FP11229
Hasi, M., Zhang, X. Y., Niu, G. X., Wang, Y. L., Geng, Q. Q., Quan, Q., et al. (2021). Soil moisture, temperature and nitrogen availability interactively regulate carbon exchange in a meadow steppe ecosystem. Agr. Forest Meteorol. 304/305, 108389. doi: 10.1016/j.agrformet.2021.108389
Hou, W. F., Traenkner, M., Lu, J., Yan, J. Y., Huang, S. Y., Ren, T., et al. (2019). Interactive effects of nitrogen and potassium on photosynthesis and photosynthetic nitrogen allocation of rice leaves. BMC Plant Biol. 19, 302. doi: 10.1186/s12870-019-1894-8
Hui, D., Yu, C. L., Deng, Q., Dzantor, K. E., Zhou, S., Dennis, S., et al. (2018). Effects of precipitation changes on switchgrass photosynthesis, growth, and biomass: a mesocosm experiment. PLoS ONE 13, e0192555. doi: 10.1371/journal.pone.0192555
Jump, A. S., and Penuelas, J. (2005). Running to stand still: adaptation and the response of plants to rapid climate change. Ecol. Lett. 8, 1010–1020. doi: 10.1111/j.1461-0248.2005.00796.x
Knapp, A. K., Chen, A., Griffin-Nolan, R. J., Baur, L. E., Carroll, C. J. W., Gray, J. E., et al. (2020). Resolving the Dust Bowl paradox of grassland responses to extreme drought. Proc. Natl. Acad. Sci. U.S.A. 117, 22249–22255. doi: 10.1073/pnas.1922030117
Liang, X. Y., Zhang, T., Lu, X. K., Ellsworth, D. S., BassiriRad, H., You, C. M., et al. (2020). Global response patterns of plant photosynthesis to nitrogen addition: a meta-analysis. Glob. Change Biol. 26, 3585–3600. doi: 10.1111/gcb.15071
Lin, J., Wang, Y., Sun, S., Mu, C., and Yan, X. (2017). Effects of arbuscular mycorrhizal fungi on the growth, photosynthesis and photosynthetic pigments of Leymus chinensis seedlings under salt-alkali stress and nitrogen deposition. Sci. Total Environ. 576, 234–241. doi: 10.1016/j.scitotenv.2016.10.091
Long, S. P., Baker, N. R., and Raines, C. A. (1993). Analysing the responses of photosynthetic CO2 assimilation to long-term elevation of atmospheric CO2 concentration. Vegetatio 104/105, 33–45. doi: 10.1007/BF00048143
Luo, X. Z., Croft, H., Chen, J. M., He, L. M., and Keenan, T. F. (2019). Improved estimates of global terrestrial photosynthesis using information on leaf chlorophyll content. Glob. Change Biol. 25, 2499–2514. doi: 10.1111/gcb.14624
Ma, J., Wang, H., and Zhang, Y. (2012). Will boreal winter precipitation over China increase in the future? An AGCM simulation under summer “ice-free Arctic” conditions. Chinese Sci. Bull. 57, 921–926. doi: 10.1007/s11434-011-4925-x
Mason, N. W. H., Orwin, K., Lambie, S., Woodward, S. L., McCready, T., and Mudge, P. (2016). Leaf economics spectrum-productivity relationships in intensively grazed pastures depend on dominant species identity. Ecol. Evol. 6, 3079–3091. doi: 10.1002/ece3.1964
Nippert, J. B., Fay, P. A., Carlisle, J. D., Knapp, A. K., and Smith, M. D. (2009). Ecophysiological responses of two dominant grasses to altered temperature and precipitation regimes. Acta Oecol. 35, 400–408. doi: 10.1016/j.actao.2009.01.010
Niu, S., Zhang, Y., Yuan, Z., Liu, W., Huang, J., and Wan, S. (2006). Effects of interspecific competition and nitrogen seasonality on the photosynthetic characteristics of C3 and C4 grasses. Environ. Exp. Bot. 57, 270–277. doi: 10.1016/j.envexpbot.2005.06.004
Norberg, J., Swaney, D. P., Dushoff, J., Lin, J., Casagrandi, R., and Levin, S. A. (2001). Phenotypic diversity and ecosystem functioning in changing environments: a theoretical framework. Proc. Natl. Acad. Sci. U.S.A. 98, 11376–11381. doi: 10.1073/pnas.171315998
Orwin, K. H., Ostle, N., Wilby, A., and Bardgett, R. D. (2014). Effects of species evenness and dominant species identity on multiple ecosystem functions in model grassland communities. Oecologia 174, 979–992. doi: 10.1007/s00442-013-2814-5
Post, A. K., and Knapp, A. K. (2020). The importance of extreme rainfall events and their timing in a semi-arid grassland. J. Ecol. 108, 2431–2443. doi: 10.1111/1365-2745.13478
Reich, P. B. (2005). Global biogeography of plant chemistry: filling in the blanks. New Phytol. 168, 263–266. doi: 10.1111/j.1469-8137.2005.01562.x
Reich, P. B., Buschena, C., Tjoelker, M. G., Wrage, K., Knops, J., Tilman, D., et al. (2003). Variation in growth rate and ecophysiology among 34 grassland and savanna species under contrasting N supply: a test of functional group differences. New Phytol. 157, 617–631. doi: 10.1046/j.1469-8137.2003.00703.x
Schleuss, P. M., Widdig, M., Heintz-Buschart, A., Kirkman, K., and Spohn, M. (2020). Interactions of nitrogen and phosphorus cycling promote P acquisition and explain synergistic plant-growth responses. Ecology 101, e03003. doi: 10.1002/ecy.3003
Silletti, A. M., and Knapp, A. K. (2001). Responses of the codominant grassland species Andropogon gerardii and Sorghastrum nutans to long-term manipulations of nitrogen and water. Am. Midl. Nat. 145, 159–167. doi: 10.1674/0003-0031(2001)145[0159:ROTCGS]2.0.CO;2
Song, X., Wang, Y., and Lv, X. (2016). Responses of plant biomass, photosynthesis and lipid peroxidation to warming and precipitation change in two dominant species (Stipa grandis and Leymus chinensis) from North China Grasslands. Ecol. Evol. 6, 1871–1882. doi: 10.1002/ece3.1982
Still, C. J., Berry, J. A., Collatz, G. J., and DeFries, R. S. (2003). Global distribution of C3 and C4 vegetation: carbon cycle implications. Global Biogeochem. Cy. 17, 1006. doi: 10.1029/2001GB001807
Tan, Y. L., Chen, J., Yan, L. M., Huang, J. H., Wang, L. X., and Chen, S. P. (2013). Mass loss and nutrient dynamics during litter decomposition under three mixing treatments in a typical steppe in Inner Mongolia. Plant Soil 366, 107–118. doi: 10.1007/s11104-012-1401-6
Tang, B., Yin, C., Yang, H., Sun, Y., and Liu, Q. (2017). The coupling effects of water deficit and nitrogen supply on photosynthesis, WUE, and stable isotope composition in Picea asperata. Acta Physiol. Plan. 39, 148. doi: 10.1007/s11738-017-2451-4
Walther, G. R., Post, E., Convey, P., Menzel, A., Parmesan, C., Beebee, T. J. C., et al. (2002). Ecological responses to recent climate change. Nature 416, 389–395. doi: 10.1038/416389a
West, B. T. (2009). Analyzing longitudinal data with the linear mixed models procedure in SPSS. Eval. Health Prof. 32:207–228. doi: 10.1177/0163278709338554
Xu, W. Z., Deng, X. P., and Xu, B. C. (2013). Effects of water stress and fertilization on leaf gas exchange and photosynthetic light-response curves of Bothriochloa ischaemum L. Photosynthetica 51, 603–612. doi: 10.1007/s11099-013-0061-y
Yu, W. Q., Wang, Y. Q., Wang, Y. J., Zhang, H. L., Wang, B., and Liu, Y. (2015). Effect of precipitation condition on photosynthesis and biomass accumulation and referring to splash erosion status in five typical evergreen tree species in humid monsoon climatic region of subtropical hill-land. J. Cent. South Univ. 22, 3795–3805. doi: 10.1007/s11771-015-2924-9
Zhai, Z., Gong, J., Luo, Q., Pan, Y., Baoyin, T., Xu, S., et al. (2017). Effects of nitrogen addition on photosynthetic characteristics of Leymus chinensis in the temperate grassland of Nei Mongol, China. Chinese J. Plant Ecol. 41, 196–208. doi: 10.17521/cjpe.2016.0128
Zhang, B., Tan, X., Wang, S., Chen, M., Chen, S., Ren, T., et al. (2017). Asymmetric sensitivity of ecosystem carbon and water processes in response to precipitation change in a semi-arid steppe. Funct. Ecol. 31, 1301–1311. doi: 10.1111/1365-2435.12836
Zhang, R., Wu, J., Li, Q., Hanninen, H., Peng, C., Yao, H., et al. (2017). Nitrogen deposition enhances photosynthesis in Moso bamboo but increases susceptibility to other stress factors. Front. Plant Sci. 8, 1975. doi: 10.3389/fpls.2017.01975
Zhang, X., Hasi, M., Li, A., Tan, Y., Daryanto, S., Wang, L., et al. (2021). Nitrogen addition amplified water effects on species composition shift and productivity increase. J. Plant Ecol. 14, 816–828. doi: 10.1093/jpe/rtab034
Zhang, X., Tan, Y., Li, A., Ren, T., Chen, S., Wang, L., et al. (2015). Water and nitrogen availability co-control ecosystem CO2 exchange in a semiarid temperate steppe. Sci. Rep. 5, 15549. doi: 10.1038/srep15549
Zhang, Y., Niu, S., Xu, W., and Han, Y. (2008). Species-specific response of photosynthesis to burning and nitrogen fertilization. J. Integr. Plant Biol. 50, 565–574. doi: 10.1111/j.1744-7909.2008.00658.x
Zheng, S., Lan, Z., Li, W., Shao, R., Shan, Y., Wan, H., et al. (2011). Differential responses of plant functional trait to grazing between two contrasting dominant C3 and C4 species in a typical steppe of Inner Mongolia, China. Plant Soil 340, 141–155. doi: 10.1007/s11104-010-0369-3
Keywords: light response curve, nitrogen application, photosynthesis, snow addition, water addition, Leymus chinensis, Stipa grandis
Citation: Zhang X, Zhai P and Huang J (2022) Leaf Carbon Exchange of Two Dominant Plant Species Impacted by Water and Nitrogen Application in a Semi-Arid Temperate Steppe. Front. Plant Sci. 13:736009. doi: 10.3389/fpls.2022.736009
Received: 05 July 2021; Accepted: 31 March 2022;
Published: 02 May 2022.
Edited by:
Nick Smith, Texas Tech University, United StatesReviewed by:
Dany Moualeu-Ngangue, Leibniz University Hannover, GermanyAndrew J. Felton, Utah State University, United States
Maowei Liang, Peking University, China
Copyright © 2022 Zhang, Zhai and Huang. This is an open-access article distributed under the terms of the Creative Commons Attribution License (CC BY). The use, distribution or reproduction in other forums is permitted, provided the original author(s) and the copyright owner(s) are credited and that the original publication in this journal is cited, in accordance with accepted academic practice. No use, distribution or reproduction is permitted which does not comply with these terms.
*Correspondence: Xiaolin Zhang, alleenzhang@126.com; Jianhui Huang, jhhuang@ibcas.ac.cn
†These authors have contributed equally to this work and share first authorship