- 1Department of Nephrology, Union Hospital, Tongji Medical College, Huazhong University of Science and Technology, Wuhan, China
- 2Department of Neurology, Union Hospital, Tongji Medical College, Huazhong University of Science and Technology, Wuhan, China
- 3Department of Neurobiology, Institute of Brain Research, School of Basic Medical Sciences, Tongji Medical College, Huazhong University of Science and Technology, Wuhan, China
MicroRNAs (miRNAs) participate in the regulation of various important biological processes by regulating the expression of various genes at the post-transcriptional level. Podocytopathies are a series of renal diseases in which direct or indirect damage of podocytes results in proteinuria or nephrotic syndrome. Despite decades of research, the exact pathogenesis of podocytopathies remains incompletely understood and effective therapies are still lacking. An increasing body of evidence has revealed a critical role of miRNAs dysregulation in the onset and progression of podocytopathies. Moreover, several lines of research aimed at improving common podocytopathies diagnostic tools and avoiding invasive kidney biopsies have also identified circulating and urine miRNAs as possible diagnostic and prognostic biomarkers for podocytopathies. The present review mainly aims to provide an updated overview of the recent achievements in research on the potential applicability of miRNAs involved in renal disorders related to podocyte dysfunction by laying particular emphasis on focal segmental glomerulosclerosis (FSGS), minimal change disease (MCD), membranous nephropathy (MN), diabetic kidney disease (DKD) and IgA nephropathy (IgAN). Further investigation into these dysregulated miRNAs will not only generate novel insights into the mechanisms of podocytopathies, but also might yield novel strategies for the diagnosis and therapy of this disease.
Introduction
Podocytes are highly specialized, terminally differentiated glomerular visceral epithelial cells that are indispensable for the maintenance of an of intact glomerular filtration barrier in the kidney (Mathieson, 2011). Due to the restricted proliferation capacity, podocytes are especially vulnerable to a series of injurious stimuli, such as hyperglycemia, transforming growth factor β (TGF-β), angiotensin II, as well as adriamycin and puromycin, which could ultimately result in podocytes loss (Kang et al., 2010; Zhou and Liu, 2015). Glomerular podocytes are the primary target in majority of glomerular diseases (Arif et al., 2019), mainly including diabetic kidney diseases (DKD), focal segmental glomerulosclerosis (FSGS), minimal change disease (MCD), membranous nephropathy (MN), and IgA nephropathy (IgAN), leading to consequent albuminuria or proteinuria and subsequent renal function decline (Webster et al., 2017; Torban et al., 2019). Thus, preventing or reversing podocyte injury is an important strategy to treat podocyte-associated diseases. In the past decades we have witnessed dramatic advances in the understanding of podocyte biology as well as molecular mechanisms involved in podocyte injury (Mathieson, 2011; Lazzeri and Romagnani, 2015; Zhou and Liu, 2015; Kopp et al., 2020). Nevertheless, developing podocyte-specific targeted therapeutic strategies is still a great challenge. Currently, many pharmaceutical agents such as calcineurin inhibitors (CNIs), glucocorticosteroids (GCS), and mTOR inhibitors (mTORIs) have been reported to have protective effects against the podocyte injury. However, the side effects due to the non-specific nature of those agents pose a serious concern in the clinical practice (Mathieson, 2011; Kopp et al., 2020). Thus, identifying the key molecules that are implicated in different types of podocytopathies might offer important clues for the development of new therapeutic strategies for treating people with proteinuric kidney disease.
MicroRNAs (miRNAs) are a class of non-coding RNAs (ncRNAs) with an average length of 22 nucleotides, which have been first discovered by Lee et al., in 1993 (Lee et al., 1993; Yates et al., 2013). Although miRNAs do not have the ability to encode proteins, they are able to control the expression of their target genes at the post-transcriptional level (Borchert et al., 2006; Krol et al., 2010). Functionally, miRNAs mainly bind to the miRNA response elements (MREs) in the 3′-untranslated region (3′-UTR) of their target mRNAs, leading to mRNA degradation and/or mRNA translational inhibition (Borchert et al., 2006; Filipowicz et al., 2008; Krol et al., 2010; Yates et al., 2013). It has been predicted that over 60% of the human protein-coding genes are regulated by miRNAs (Friedman et al., 2009). Accordingly, as miRNAs are involved in the modulating the expression of entire gene networks, dysregulation of certain miRNAs can cause or contribute to a wide variety of human diseases (Gebeshuber et al., 2013; Badal et al., 2016; Treiber et al., 2019; Agbu and Carthew, 2021; Schober et al., 2021; Liu et al., 2022; Zhou et al., 2022). In addition to their critical roles in modulating gene expression, miRNAs have also been clinically used as promising non-invasive diagnostic, prognostic, and predictive biomarkers for several human diseases, such as malignant pleural mesothelioma, acral melanoma, clear cell renal carcinoma, HBV-related hepatocellular carcinoma and acute myocarditis (van Zandwijk et al., 2017; Treiber et al., 2019; Hong et al., 2020; Blanco-Domínguez et al., 2021). Previous studies have also indicated that miRNAs play pivotal roles in the development and progression of various glomerular diseases associated with podocyte dysfunction (Kato et al., 2009; Gebeshuber et al., 2013; Lazzeri and Romagnani, 2015; Trionfini and Benigni, 2017), indicating that they might also represent potential biomarkers and therapeutic targets for the diagnosis and treatment of podocytopathies. Moreover, conditional knockout of Dicer or Drosha, the key enzymatic regulators of miRNAs biogenesis and maturation, in a podocyte-specific manner results in progressive proteinuria and glomerulosclerosis, suggesting that miRNAs are also important genomic regulators of podocyte homeostasis (Shi et al., 2008; Zhdanova et al., 2011). Yet, the exact molecular mechanisms by which miRNAs modulate podocyte injury in podocytopathies still remains to be fully elucidated.
Although a large number of studies have demonstrated the critical roles of miRNAs in the occurrence and development of various podocytopathies, to the best of our knowledge, no previous systematic review has been performed to systematically generalize the involvement of miRNA dysregulation in podocytopathies. This review will mainly focus on the current understanding of pathogenic roles of miRNA dysregulation that links to podocyte injury in various podocytopathies and shed light on their use as potential biomarkers and treatment targets for this disease, which would benefit from further research.
Podocytopathies
Epidemiology and Etiology of Podocytopathies
Currently, despite the high incidence of podocytopathies, reliable epidemiological data on podocytopathies are still lacking (Kopp et al., 2020). The pathological diagnosis of podocytopathies is predominantly based on kidney biopsy, but many patients are not suitable for biopsy or lack of sufficient resources to perform biopsy, leading to an underestimation of the incidence of podocytopathies. In spite of the limitation, the prevalence of podocytopathies seems to be increasing globally, which is a leading cause of the increased prevalence of end-stage kidney disease (ESKD) (Rosenberg and Kopp, 2017). Currently, a number of key causes and risk factors have been revealed (Figure 1), mainly including genetic factors (Qiu et al., 2018), obesity (D'Agati et al., 2016), diabetes (Tonneijck et al., 2017), low nephron mass and nephron loss (Luyckx et al., 2017), immunological and/or soluble factors (Iijima et al., 2014; Kim et al., 2017; Colucci et al., 2019), vascular endothelial growth factor (VEGF) inhibition (Craici et al., 2014; Ollero and Sahali, 2015), infectious agents (Cohen et al., 2017; Nasr and Kopp, 2020) and various toxins (Rosenberg and Kopp, 2017; Puelles et al., 2019), that predispose individuals to development of podocytopathies. Alternatively, podocytopathies can be caused by a combination of diverse genetic and/or environmental risk factors that lead to podocyte damage, acting together to achieve a threshold effect for the development of proteinuria.
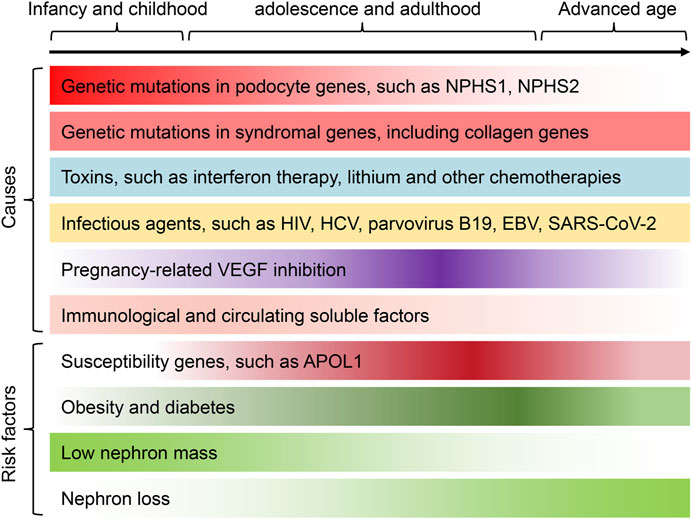
FIGURE 1. The underlying causes and risk factors of podocytopathies across the lifespan. A number of causes and risk factors have been revealed, mainly including genetic factors, immunological and/or soluble factors, VEGF inhibition, adaptive podocyte stress, infectious agents and various toxins, that predispose individuals to development of podocytopathies. Different risk factors and/or causes of podocytopathies can present at certain phases of life or be preferentially associated with gender and race. For instance, genetic causes of podocytopathies are more frequent in children and young adults. Podocytopathies associated with VEGF inhibition are more common in pregnant women. Among the major risk factors leading to the development of podocytopathies, nephron loss, severe obesity and diabetes are more frequently observed in adult middle-age patients, whereas low nephron mass is more frequent in adolescence or early adulthood. Besides, the susceptibility gene APOL1 is more prevalent in patients of Black adult. Finally, podocytopathies induced by various toxins and infectious agents can occur at all ages. The color gradient in each cause and risk factor represents the incidence of podocytopathies at different ages. APOL1, apolipoprotein L1; HCV, hepatitis C virus; EBV, Epstein–Barr virus; SARS-CoV-2, the virus that causes COVID-19.
Mechanisms and Pathophysiology of Podocytopathies
As terminally differentiated epithelial cells, the primary and secondary processes of podocytes cover the outer side of the glomerular basement membrane (GBM), constituting the glomerular filtration barrier (Wiggins, 2007; Mathieson, 2011). Foot process effacement (FPE) of podocytes is the earliest morphological patterns of podocyte injury, which is closely related to different degrees of proteinuria in the progress of various podocytopathies (Wiggins, 2007; Kopp et al., 2020). Although the effacement of podocytes foot process can be invertible, podocyte detachment from the GBM or death indicates inevitable podocyte loss, which can be mainly attributed to different unfavorable factors such as genetics, metabolism, toxicity or inflammation (Mundel and Shankland, 2002; Mundel and Reiser, 2010). Following damaged podocytes loss, the remaining podocytes adapt to expand to cover the newly denuded GBM, resulting in hypertrophy of remaining podocytes (Shankland, 2006). In addition, parietal epithelial cells (PECs), located in the inner portion of the Bowman capsule, are resident podocyte progenitors that can provide new podocytes following injury and loss (Ronconi et al., 2009; Eng et al., 2015). Despite these mechanisms play a beneficial role in the restoration of podocyte function and decrease proteinuria, they may be maladaptive or inefficient. Indeed, the capacity of hypertrophic podocytes is limited, which may be incapable to maintain the normal structure of podocyte foot processes, leading to enhanced local shear stresses that may subsequently trigger the process of podocyte detachment (Shankland, 2006; Fukuda et al., 2012). Furthermore, the differentiation of PECs into podocytes can be impaired by unfavorable factors (such as mechanical stress and proteinuria), ultimately resulting in inefficient podocyte regeneration or focal scar formation (Peired et al., 2013; Kopp et al., 2020). Nevertheless, despite certain progress has been achieved in exploring the molecular mechanisms and pathophysiology of podocytopathies, we are still far from fully understanding the pathogenesis of podocytopathies.
Diagnosis and Treatment of Podocytopathies
Currently, the diagnosis of podocytopathies is predominantly based on clinical manifestations combined with pathological findings. Renal biopsy is routinely conducted on all adults with nephrotic-range proteinuria to guide treatment and provide prognosis (Mathieson, 2011; Kopp et al., 2020). However, the decision whether and when to perform a kidney biopsy in children with persistent non-nephrotic proteinuria is still controversial (Hama et al., 2012; Leung et al., 2017). A detailed differential diagnosis of podocytopathies may demand a combination of personal and family history, clinical manifestations, histopathological testing, serological inspection, imaging examination and/or genetic analyses (Wiggins, 2007; Kopp et al., 2020). Podocytopathies can be regarded as a consequence of podocyte dysfunction induced by various genetic and/or environmental elements. The clinical syndromes associated with podocyte dysfunction mainly include the following several types: DKD, FSGS, MCD, MN, Alport’s syndrome, hypertensive, nephropathy (HTN), immune and inflammatory glomerulonephropathies (Imm/Inf GN) (Wiggins, 2007; Nagata, 2016).
Therapeutic approaches in the management of patients with podocytopathies presenting with massive proteinuria or nephrotic syndrome can vary depending on the population served and available resources. Children and adult patients with consistent non-nephrotic proteinuria are primarily managed with dietary salt restriction and renin-angiotensin system inhibitors (RASI) (Mathieson, 2011; Ayalon and Beck, 2015). Besides, low-dose thiazide diuretic will have an additive effect on the anti-proteinuric effect. Children and adolescent patients with new onset nephrotic syndrome in the absence of histological verification are usually treat with oral steroid therapy for two to three months (Zhao and Liu, 2020). Nevertheless, due to the heterogeneous clinical behavior and response to treatment with steroid, the dose and treatment duration for the steroid should be adjusted appropriately according to clinical response. In adult patients, kidney biopsy, laboratory inspections as well as renal imaging examinations preclude other glomerular disorders, thereby guiding the management of podocytopathies based on the underlying etiologies. Glucocorticoids are the first choice for the treatment of some cases with no specific cause can be ascertained (Kopp et al., 2020; Medjeral-Thomas et al., 2020). Patients with sub-nephrotic proteinuria are usually managed with maximal doses of RASI and followed longitudinally according to renal function and residual proteinuria. However, increasing evident suggests that, beyond the basic initial steroid therapy, a substantial proportion of patients with podocytopathies are in an urgent need of a personalized treatment plan to prevent unnecessary drug-related toxicity. Thus, more in-depth research is needed to get a systemic understanding of the underlying mechanisms of podocytopathies, so as to provide novel therapeutic strategies to combat this type of disease.
Role of MiRNAs in Podocytopathies
Increasing evidence indicates that miRNAs are critical regulators of gene expression in mammals, and the dysregulation of miRNAs is a common feature of various human diseases, including podocytopathies (Kato et al., 2009; Trionfini and Benigni, 2017; Fan et al., 2020). In the following sections, we will mainly focus on and summarize the current knowledge on the implications of miRNAs in the occurrence and development of podocytopathies as well as their potential value of clinical application in the diagnosis, treatment and prognosis of this disease.
MiRNAs in Focal Segmental Glomerulosclerosis
Focal segmental glomerulosclerosis (FSGS) is a group of clinicopathological syndromes sharing a common glomerular lesion and characterized by marked proteinuria and podocyte injury, which is the result of diverse insults directed to or inherent within the podocyte (D'Agati et al., 2011; Wei et al., 2011; Fogo, 2015). A broad range of factors such as genetic, virus infections, drugs, and circulating factors can contribute to the pathogenesis of FSGS, which can be broadly classified into primary (idiopathic) and secondary forms, with approximately 80% of cases being primary (D'Agati et al., 2011). Current therapeutic strategies for FSGS mainly focus on reduction of urinary protein excretion and preserving renal function (D'Agati et al., 2011; Rosenberg and Kopp, 2017). Approximately 40% of patients with primary FSGS who receive kidney transplantation eventually develop recurrent disease in the allograft (D'Agati et al., 2011). Thus, further elucidation of circulating-factor caused podocyte damage as well as understanding the mechanisms of podocyte stabilization may help to shed new light on the specific pathogenetic mechanisms involved in primary FSGS.
Recent years have witnessed a flourishing interest in exploring the underlying role of miRNAs in FSGS (Gebeshuber et al., 2013; Trionfini and Benigni, 2017). For example, Gebeshuber and colleagues performed the first study to investigate the role of specific miRNAs in FSGS (Gebeshuber et al., 2013). In this study the researchers originally identified miR-193a can be highly inducible by doxycycline in miR-193a transgenic mice under the control of a cytomegalovirus (CMV)-tet promotor, whereas the upregulation of miR-193a in transgenic mice induced by doxycycline resulted in rapidly progressing FSGS and death from kidney failure within 12 weeks in heterozygous mice and 6 weeks in homozygous mice, respectively. Mechanistical studies revealed that miR-193a may suppress the expression of the Wilms’ tumor protein (WT1), a transcription factor that is indispensable for maintaining podocyte differentiation and homeostasis. They concluded that the enhanced expression of miR-193a in FSGS unveiled a novel pathogenic mechanism for FSGS and may provide a novel approach to treat FSGS. Consistent with those findings, a recent study demonstrated that higher miR-193a level in urinary exosomes was related to higher probability of primary FSGS and indicated poor prognosis in children with nephrotic syndrome (Wang et al., 2021). In another study, the association between urinary miRNAs levels and disease activity and treatment response in patients with FSGS was also reported (Zhang et al., 2014). In order to identify urinary miRNA-based biomarkers for FSGS disease activity as well as predict patients responds to steroid therapy, Zhang and his colleagues performed miRNA profiling among patients with active FSGS (FSGS-A), FSGS in remission (FSGS-CR), and age- and sex-matched normal controls. A total of 54 candidate miRNAs were identified, among which the levels of miR-490, miR-196a, and miR-30a-5p in urine samples were confirmed to be significantly upregulated in FSGS-A patients compared with normal controls and FSGS-CR patients and could be used as sensitive indicators of disease activity. In addition, the same research group also found that plasma miR-186 level was significantly upregulated in FSGS patients with nephrotic proteinuria and declined with disease remission, which was positively correlated with urinary protein levels in patients with FSGS, implying the possibility of miR-186 as a specific biomarker for FSGS (Zhang et al., 2015). Whereas, another study showed that the miR-30 family were highly expressed in podocytes and downregulated in patients with FSGS, which protected podocytes against injury induced by deleterious factors (TGF-β, PAN, or LPS) through targeting Notch1 and p53 (Wu et al., 2014). And exogenous overexpression of miR-30a attenuated proteinuria and podocyte injury in PAN-treated rats (Wu et al., 2014). Wu et al. also demonstrated that miR-30s were consistently downregulated in podocytes of FSGS patients and PAN-treated rats, and identified that downregulation of miR-30s results in calcium/calcineurin signaling activation, thus leading to podocyte injury in FSGS (Wu et al., 2015). In addition, other studies have also reported the involvement of some other dysregulated miRNAs in FSGS, such as miR-135a (Yang et al., 2017), miR-155 (Ramezani et al., 2015), miR-206 (Guo et al., 2016), miR-150 (Qi et al., 2020), miR-106a (Xiao et al., 2018) and miR-146-5p (Williams et al., 2022).
Although great progress has been made in elucidating the critical roles of miRNAs in the initiation and progression of FSGS (Table 1), the impact of miRNAs on glomerular structure as well as podocyte foot process effacement have yet to be investigated in detail. Moreover, currently it is still difficult to figure out the exact molecular mechanisms involved in the regulation of urinary and plasma miRNAs expression levels under different states of FSGS. Alternatively, a better understanding of what mediates the podocytes injury in FSGS will likely lead to novel therapeutic strategies. Given the complexity of miRNA regulation, more in-depth studies are necessary to fully understand their contribution to FSGS.
miRNAs in Minimal Change Disease
Minimal change disease (MCD) is one of the main causes of nephrotic syndrome, which is typically defined as lacking significant visible glomerular structure alterations by light microscopy and the widespread effacement of podocyte foot processes on electron microscopy without electron-dense deposits (Floege and Amann, 2016). Although the exact etiology of MCD remains unclear, glomerular permeability factors are considered as important contributors to the pathogenesis of MCD (Clement et al., 2011). Current treatment of MCD predominantly relies on corticosteroids (Maas et al., 2016). However, steroid-sensitive individuals frequently develop recrudesce or steroid resistance, which eventually results in a part of patients requiring second-line steroid-sparing immunosuppression (Vivarelli et al., 2017). Steroid-resistance occurs in approximately 10%–30% of adult cases, which occurs more frequently and earlier in children (Waldman et al., 2007). Recently, several newer agents, such as rituximab, have been utilized in adult patients with MCD aiming to decrease the risk of adverse effects of steroid therapies. However, due to the extremely high cost, rituximab currently cannot replace steroid as a first-line treatment for MCD (Munyentwali et al., 2013). Moreover, in the pediatric setting, renal biopsy is usually not performed unless steroid-resistance is observed (Vivarelli et al., 2017). Hence, there is an urgent need for developing alternative strategies to treat MCD as well as identifying novel non-invasive methods to diagnose MCD.
Recent evidence has revealed that dysregulation of certain miRNAs is linked to MCD progression and clinical outcome, suggesting they might be considered as potential diagnostic markers and therapeutic targets for MCD. To date, numerous studies have demonstrated the critical role of miRNAs in FSGS progression. In this regard, published data on MCD is relatively scarce. One recent study has reported that exogenous overexpression of miR-499 could ameliorate MCD symptoms as well as attenuate the foot-process effacement of podocytes in PAN-induced MCD mouse model (Zhang et al., 2018). Mechanistical studies showed that miR-499 may exert a protective effect on podocytes by suppressing the expression of the catalytic calcineurin isoforms α (CnAα) and β (CnAβ), leading to a decreased activity of calcineurin signaling in podocytes. Zheng et al. identified that miR-27b aggravated PAN-induced podocyte dysfunction in a primary podocyte model through targeting inhibition of adenosine receptor 2B (Adora2b), an intracellular pro-survival protein (Zheng et al., 2018). Lu et al. proposed that miR-150 had the potential to differentiate MCD from other nephropathy subtypes (Lu et al., 2015). In addition, a growing body of evidence has indicated that dysregulation of certain miRNAs in body fluid of patients with MCD may serve as diagnostic markers and prognostic indicators, mainly including plasma levels of miR-192 (Cai et al., 2013), miR-30b, miR-30c, miR-34b, and miR-34c (Ramezani et al., 2015) and urine level of miR-1225-5p (Ramezani et al., 2015).
Although many miRNAs have been identified to be dysregulated in MCD (Table 2), the precise mechanism of action of these miRNAs in MCD pathology remains unclear due to the lack of direct in vivo experimental evidence. Furthermore, since researchers have proposed that MCD and FSGS are in fact different histological manifestations of the same disease processes, those miRNAs that were found to play a key role in FSGS may also be involved in the pathogenesis of MCD, which deserves to be further investigated.
MiRNAs in Membranous Nephropathy
Membranous nephropathy (MN) is defined as a complex pathological disorder of the glomeruli that occurs sporadically in all age groups (Couser, 2017). The deposition of immune complexes and the formation of membrane attack complexes contribute to the structural disturbances in podocytes, which ultimately lead to the development of massive proteinuria (Ronco et al., 2021). Clinically, MN often presents as nephrotic syndrome with variable outcomes, among which one-third of patients remit spontaneously, another third display variable degrees of persistent proteinuria without renal function exacerbation, while the remaining third develop to ESKD (Ronco et al., 2021). Cyclophosphamide-based therapy protocols have long been the standard therapy because they have been shown to prevent the occurrence of renal failure, but they put patients at a higher risk of developing cancer (Cattran and Brenchley, 2017). Besides, treatment plans with CD20-targeting agents are well tolerated, but patients achieve durable clinical remissions at low rates, and strong evidence of their effectiveness in preventing renal disease progression is still lacking (Ruggenenti et al., 2017; Ronco et al., 2021). Accordingly, developing new antigen-specific immunotherapies as well as identifying novel diagnostic and therapeutic targets for MN are of great importance for the development of effective therapeutic strategies to battle this disease.
With the continuous advancement of high-throughput sequencing technology and calculation methods, hundreds of dysregulated miRNAs have been identified and are proposed to potentially play significant roles in the pathogenesis of MN (Table 3). Nevertheless, only few miRNAs have been confirmed to be involved in MN. For instance, Sha et al. analyzed renal tissues from MN patients and found that miR-186 was significantly decreased in MN (Sha et al., 2015). In addition, they demonstrated that Ang II treatment significantly down-regulated the level of miR-186 in cultured podocytes, while ectopic expression of miR-186 attenuated Ang II-induced podocytes apoptosis. The expression levels of miR-217 were found to be consistently decreased in MN tissue and plasma by Li and her colleagues (Li et al., 2017). In vitro studies proposed that miR-217 silencing induced podocytes apoptosis through targeting tumor necrosis factor superfamily member 11 (TNFSF11). However, whether miR-217 could regulate TNFSF11 expression in vivo, and whether such relation between them could make a contribution to the pathology of MN, still require further investigation. Nonetheless, they proposed that absolutely quantifying plasma miR-217 could be an advantageous diagnostic biomarker for MN. Besides, another research also identified that miR-130a-5p was downregulated in the renal biopsy specimens from MN patients, which could attenuate Ang II induced-podocyte apoptosis through modulating PLA2R expression (Liu et al., 2018), however, this study also lacked in vivo experiments. Apart from these, some miRNAs, not yet experimentally confirmed, were proposed as potential diagnostic biomarkers for MN, including miR-98, miR-375, miR-7-5p, miR-615-3p, miR-577 (Chen et al., 2014), let-7a-5p, let-7c-5 (Barbagallo et al., 2019), miR-195-5p, miR-192-3p, miR-328-5p (Zhou et al., 2019), miR-106a and miR-19b (Wu et al., 2021), indicating that the exact role of miRNAs in the occurrence and the progression of MN is far less understood and remains an enigma.
Collectively, based on these current reports, targeting inhibition or ectopic expression of certain miRNAs in podocytes might yield new strategies for MN diagnosis, prevention, and therapy.
MiRNAs in Diabetic Kidney Disease
Diabetic kidney disease (DKD) is a major microvascular complication of diabetes mellitus and the leading cause of chronic kidney disease (CKD) and ESKD worldwide, which occurs in approximately one-third of type 1 diabetes mellitus (T1DM) patients and 40% of type 2 diabetes mellitus (T2DM) patients (Alicic et al., 2017). The global surge in DKD prevalence parallels the dramatic increase in the incidence of diabetes worldwide (de Boer et al., 2011). Progressive increase in proteinuria and progressive deterioration of renal function are major clinical manifestations of DKD (Doshi and Friedman, 2017). Despite current strategies for DKD management, mainly including lifestyle modification, intensive control of glycemic, blood pressure and lipid as well as albuminuria-reducing, have meaningfully improved outcomes for diabetes complications, DKD still poses a major risk factor for the development of ESKD (Alicic et al., 2017). Multiple lines of evidence have demonstrated that podocyte injury or loss plays a critical role in the development and progression of DKD, which ultimately leading to proteinuria and further renal damage (Mathieson, 2011; Reidy et al., 2014). Therefore, identifying the key molecules that may be involved in podocyte injury will provide new clues in developing novel diagnostic, therapeutic and prevention strategies for DKD.
Growing body of evidence suggests that dysregulation various of miRNAs may serve not only as diagnostic biomarkers but also as therapeutic targets for several malignant tumors (Beg et al., 2017; van Zandwijk et al., 2017). Furthermore, in recent years, with the deepening of researches, the roles of miRNAs in the pathogenesis of podocyte injury in DKD have received more attention. Many previous studies have systematically elucidated that the ectopic expression or knock down/out of indicated miRNAs could exert a significant effect on high glucose (HG) induced podocytes injury in vitro, as well as in rodent models of DKD. For example, ectopic expression of miRNA-23b in diabetic kidneys attenuated diabetes-induced podocyte injury, reduced proteinuria as well as effectively mitigated DKD progression in diabetic mice, indicating a protective role of miR-23b in DKD podocyte injury (Zhao et al., 2016). Another study by the same research group reported that miR-25 expression levels were significantly decreased in the sera of diabetic patients as well as in kidney tissues from diabetic mice (Liu et al., 2017). Further in vivo studies indicated that overexpression of miRNA-25 via intravenous injection of an miR-25 mimic was shown to ameliorate podocyte injury in diabetic mice. Mechanistic studies revealed that miRNA-25 exerted its protective role in DKD mainly through targeting inhibition of cell division cycle 42 (CDC42) expression, a downstream effector of Ras that can lead to congenital nephrotic syndrome and glomerulosclerosis. A more recent study also suggested the protective role of miRNA against podocyte injury in DKD. In this study, miR-10a and miR-10b were identified to be predominantly expressed in the kidney and significantly downregulated in podocytes under diabetic conditions, which acted as endogenous inhibitors of the NLRP3 inflammasome in DKD, thereby protecting podocytes against injury in DKD (Ding et al., 2021). Besides, other studies have also reported the protective role of miRNAs in against podocyte injury DKD, such as miR-146a (Lee et al., 2017), miR-29a (Lin et al., 2014), and miR-93 (Badal et al., 2016), which may act as potential therapeutic targets for DKD. While, Zhou et al. have demonstrated that up-regulated miR-27a exacerbated HG-induced podocytes injury in vitro and contributed to unfavorable renal function and increased podocyte injury in diabetic rats in vivo (Zhou et al., 2017). In addition, Kölling and colleagues suggested that therapeutic miR-21 silencing could ameliorate DKD in mice models (Kölling et al., 2017). Apart from these, other miRNAs also played detrimental roles in diabetes-induced podocytes injury, like miR-29c (Long et al., 2011), miR-182-5p (Ming et al., 2019), miR-20b (Wang et al., 2017), miR-503 (Zha et al., 2019), miR-193a (Mishra et al., 2018) and so on. In a word, dysregulation of many miRNAs indeed plays various harmful roles in the initiation and progression of podocyte injury in DKD (Table 4).
These lines of evidence suggest that miRNAs have dual roles in DKD-induced podocyte injury, targeting inhibition or ectopic expression of indicated miRNAs in podocytes would have protective effects against podocyte injury in DKD, thus deserving further investigation.
MiRNAs in IgA Nephropathy
IgA nephropathy (IgAN) is the most common primary glomerulonephritis worldwide (Roberts, 2014; Rodrigues et al., 2017). Patients with IgAN can present with a wide range of symptoms, from asymptomatic microscopic hematuria to rapidly progressive glomerulonephritis (Roberts, 2014; Rodrigues et al., 2017). Currently, there is still no specific treatment for IgAN and patients are managed with the aim of controlling blood pressure and maintaining renal function. The pathology of IgAN is mainly characterized by the deposition of pathogenetic polymeric IgA1-IgG immune complexes in the glomerular mesangium, proliferation of mesangial cells, increased synthesis of extracellular matrix and infiltration of many types of immune cells, including macrophages, monocytes and T cells (Lai et al., 2008; Lai et al., 2016). In addition, emerging evidence indicates that podocyte injury is singularly important in the pathogenesis of IgAN and has been considered as a key mechanism leading to the disease progression (Hill et al., 2011; Lai et al., 2016). Nevertheless, the precise molecular mechanisms underlying podocyte injury in IgAN have not been fully understood. Thus, it is urgent to identify novel key molecules underlying podocyte injury in IgAN that can potentially serve as early diagnostic biomarkers and/or therapeutic targets for IgAN.
Over the past decade, we have witnessed a flourishing of studies aimed at exploring the biological functions of miRNAs in the occurrence and development of IgAN (Szeto and Li, 2014). Hundreds of dysregulated miRNAs have been identified in IgAN, some of which have been proposed to play significant roles in the pathogenesis of IgAN. For example, Dai and his colleagues performed the first genome-wide analysis of miRNAs expression profiling in kidney biopsy samples from 11 patients with IgAN and 3 normal controls and found that the expression levels of miR-200c, miR-141, miR-205 and miR-192 were associated with disease severity and progression in IgAN patients (Dai et al., 2008). In another study, they further investigated miRNA expression in renal biopsy samples from six patients with IgAN and normal renal cortex samples from six patients with renal cancer by high-throughput sequencing technology and identified 11 upregulated miRNAs and 74 downregulated miRNAs in IgAN. Further bioinformatic analysis indicated that these dysregulated miRNAs were mainly involved in the regulation of the macro molecular metabolism, the nitrogen compound metabolic process and biosynthetic process (Tan et al., 2013). Although neither study further explored the exact roles of these dysregulated miRNAs in IgAN, they provided new directions for investigating the pathogenesis of IgAN. Another study found that miR-21 expression level was up-regulated remarkably in glomerular tissues of patients with IgAN compared to that of the healthy control people (Bao et al., 2014b). Further studies revealed that mesangial-derived cytokines could up-regulate miR-21 in podocytes and inhibition of miR-21 prevented fibrogenic activation in podocytes. Interestingly, the relationship between miR-21 and podocytopathy is inspired by the clinical study conducted by Kong et al., which had found that the actual level of urinary albumin excretion correlated with the urinary level of miR-21 (Kong et al., 2012), indicating miR-21 may be involved in the pathogenetic mechanisms linking albuminuria in IgAN. Besides, Guo et al. indicated that miR-200b/c/429 cluster alleviated inflammation in IgAN by targeting TNF-like weak inducer of apoptosis (TWEAK) (Guo and Liao, 2017). And Osamu et al. suggested that glomerular miR-26a expression decreased significantly in both IgAN mice and patients, which was closely linked to the progression of podocyte injury in IgAN (Ichii et al., 2014). In addition, other studies have also reported the involvement of some other dysregulated miRNAs in IgAN, such as miR-223 (Bao et al., 2014a), miR-590-3p (Zhai et al., 2019), miR-133a/b (Jin et al., 2018), miR-320 (Li et al., 2018), miR-100-3p, miR-877-3p (Liang et al., 2016), miR-23b (Li H. et al., 2021), miR-214-3p (Li Y. et al., 2021), and miR-150-5p (Pawluczyk et al., 2021). IgAN appears to be a systemic disease in which the kidneys are damaged as innocent bystanders (Wyatt and Julian, 2013). Serino et al., for the first time, analyzed their global miRNA expression profile in peripheral blood mononuclear cells (PBMCs) of seven IgAN patients and seven healthy participants and revealed a novel pathophysiological mechanism whereby upregulation of miR-148b contributed to the aberrant IgA1 glycosylation through inhibiting core 1, β1, 3-galactosyltransferase 1 (C1GALT1) mRNA expression, providing a potential pharmacologic target for IgAN (Serino et al., 2012). Intriguingly, this result was further confirmed in a recent study that miR-148b expression level was significantly up-regulated in tissues from IgAN patients and positively correlated with eGFR (Wen et al., 2018). Apart from these, many circulating or urine miRNAs, not yet experimentally confirmed, were proposed as potential diagnostic biomarkers for IgAN, like miR-146a, miR-155 (Wang et al., 2011), miR-148b and let-7b (Serino et al., 2016; Kouri et al., 2021), indicating that these circulating and urine miRNAs may have a good potential for diagnosing IgAN and they deserve to be fully exploited in the future.
Despite the limited number of available studies, some interesting miRNAs have been identified as potentially relevant to the pathogenesis of IgAN (Table 5). Although the above-mentioned studies shed new light on the pathogenesis of IgAN, these studies all included small sample sizes and their results had limited adjustment for multiple potential clinical confounders. Furthermore, there is currently no accepted standard protocol for functional assessment of identified candidate miRNAs. Therefore, further research is urgently needed to address those shortages.
Collectively, the above studies indicated that miRNAs may have played critical regulatory roles in the processes of podocytopathies (Figure 2), which may serve as promising diagnostic biomarkers as well as therapeutic targets clinically.
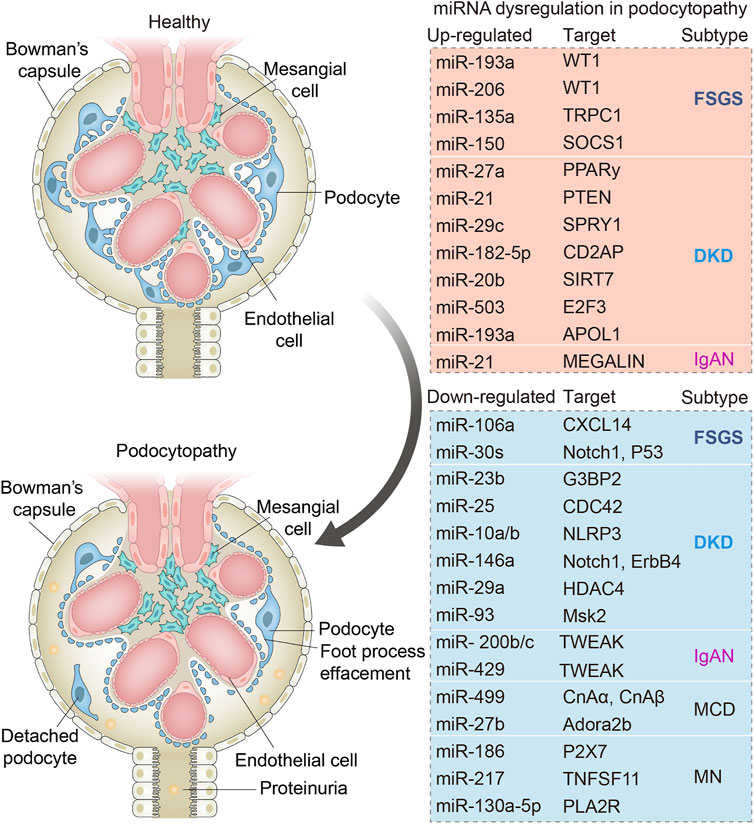
FIGURE 2. Schematic diagram showing the main dysregulated miRNAs and their corresponding downstream targets in various podocytopathies. Changes in miRNAs in podocytes occurring in focal segmental glomerulosclerosis (FSGS), minimal change disease (MCD), membranous nephropathy (MN), diabetic kidney diseases (DKD), and IgA nephropathy (IgAN). Dysregulation of indicated miRNAs contributes to podocyte injuries including podocyte foot process effacement and podocyte loss due to cell death or detachment from the glomerular basement membrane.
Potential Therapeutic Applications of MiRNAs
Mature miRNAs have unique characteristics, including short sequences as well as high homology across multiple vertebrate species, which make them potentially suitable as therapeutic targets for the treatment of various diseases. Currently, targeted manipulation of miRNAs in kidney can be achieved through exogenous delivery of specific miRNA inhibitors to block miRNA function or synthetic miRNA mimics to restore the expression level of a particular miRNA. However, no miRNAs are in clinical trials for podocytopathies at the moment. Whereas, to date, there are already some miRNA-based trials that have entered phase I or phase II clinical trials, showing significant clinical promise (Mellis and Caporali, 2018). This includes miR-16-based miRNA mimic for malignant pleural mesothelioma (van Zandwijk et al., 2017), miR-34a mimics in patients with advanced solid tumors (Beg et al., 2017; Hong et al., 2020), anti-miR-103 and anti-miR-107 for the treatment of type 2 diabetes and obesity (Trajkovski et al., 2011), miR-29a-3p mimics for scleroderma (Maurer et al., 2010), anti-miR-155 for cutaneous T-cell lymphoma and mycosis fungoides (Babar et al., 2012), anti-miR-122 for chronic hepatitis C virus (HCV) infection (Janssen et al., 2013), and anti-miR-21 for Alport syndrome (Gomez et al., 2015).
Many in vivo studies have focused on the therapeutic potential of manipulation of miRNAs in podocytopathies and promising therapeutic results in halting podocyte injury and ameliorating proteinuria have been achieved by knocking down miR-193a (Gebeshuber et al., 2013; Mishra et al., 2018), miR-150 (Qi et al., 2020), miR-27a (Zhou et al., 2017), and miR-21 (Kölling et al., 2017) or ectopic expressing miR-30s (Wu et al., 2014; Wu et al., 2015), miR-499 (Zhang et al., 2018), miR-27b (Zheng et al., 2018), miR-93 (Badal et al., 2016), miR-23b (Zhao et al., 2016), miR-25 (Liu et al., 2017), miR-10 (Ding et al., 2021) and miR-29a (Lin et al., 2014). Currently, efficient kidney in vivo transfection has been successfully achieved through intraperitoneal, intravenous or subcutaneous injection of indicated miRNA mimics or inhibitors (Trionfini et al., 2015; Henique et al., 2017). However, despite promising therapeutic applications, several obstacles must be conquered before miRNA-based therapies for podocytopathies can ultimately be translated into clinical practice, mainly including developing efficient methods to specific delivery of miRNA mimics or inhibitors to podocytes as well as avoiding the potential toxicity and adverse effects in other tissues and organs. Fortunately, so far, several strategies have been developed to partially overcome these limitations, such as using plasmids expressing indicated miRNAs or short-hairpin RNAs containing podocyte-specific promoters. In addition, the current standing diagnosis of podocytopathies predominately relays on kidney biopsy (Kopp et al., 2020), hence, there is an urgent need to develop novel non-invasive diagnostic methods for the detection and monitoring of such diseases. Emerging evidence suggests that many circulating or urine miRNAs are useful indicators of podocytopathies, such as miR-193a (Wang et al., 2021), miR-186 (Zhang et al., 2015), miR-192 (Cai et al., 2013), miR-217 (Li et al., 2017), and miR-146a (Lee et al., 2017). Therefore, measuring indicated miRNAs in the urine or plasma as a potential mechanism for monitoring accelerated podocyte loss non-invasively holds good potential for clinical application, which warrants further validation.
Conclusion
In this review, we summarized current literature related to the pathogenic role of miRNA dysregulation in the pathogenesis of podocytopathies. We majorly focused on narrating the roles and values of these dysregulated miRNAs in the occurrence, progression, clinical diagnosis, treatment and prognosis of podocytopathies, which may provide theory and clinical basis for the future clinical treatment and research of this rare disease. Over the past few decades our knowledge towards the critical role of podocytes in the onset and progression of proteinuric kidney disease has achieved tremendous progress. The importance of miRNAs in the field of podocytopathies is increasingly recognized as they allow researchers to gain a deeper understanding of podocytopathies pathways as well as provide a rationale for the development of novel diagnostic and possibly therapeutic strategies. Moreover, the strategies of miRNA-based therapy that either restores or abolishes miRNA expression and activity are very attractive, especially now that the several miRNA-targeted therapeutics have reached clinical development. By now, targeted manipulation of miRNA expression as an effective antiproteinuric therapy has been revealed only in experimental models of podocytopathies because many safety concerns have not been settled, from the delivery methods to the potential toxicity and adverse effects in other tissues and organs. Furthermore, due to the highly pleiotropic nature of miRNAs, it is unlikely that a single miRNA will be able to accurately diagnose and predict a certain disease. Given the involvement of many different miRNAs in podocytopathies, we firmly believe that a panel of miRNAs would potentially be more useful than a single miRNA as a biomarker. Collectively, further research in this area will continue to identify new therapeutic targets as well as sensitive and specific diagnostic biomarkers for podocytopathies.
Author Contributions
Conceptualization, XM and FL; validation, XM and FL; investigation, JC; writing—original draft preparation, FL and JC; writing—review and editing, XM, CL, and FL; visualization, FL and JC; supervision, XM, and CL; funding acquisition, XM. All authors contributed significantly to this review.
Funding
This work was supported by grants from the National Natural Science Foundation of China (No. 81974162, No. 81671066).
Conflict of Interest
The authors declare that the research was conducted in the absence of any commercial or financial relationships that could be construed as a potential conflict of interest.
Publisher’s Note
All claims expressed in this article are solely those of the authors and do not necessarily represent those of their affiliated organizations, or those of the publisher, the editors and the reviewers. Any product that may be evaluated in this article, or claim that may be made by its manufacturer, is not guaranteed or endorsed by the publisher.
References
Agbu P., Carthew R. W. (2021). MicroRNA-mediated Regulation of Glucose and Lipid Metabolism. Nat. Rev. Mol. Cell. Biol. 22 (6), 425–438. doi:10.1038/s41580-021-00354-w
Alicic R. Z., Rooney M. T., Tuttle K. R. (2017). Diabetic Kidney Disease. Clin. J. Am. Soc. Nephrol. 12 (12), 2032–2045. doi:10.2215/cjn.11491116
Arif E., Solanki A. K., Srivastava P., Rahman B., Fitzgibbon W. R., Deng P., et al. (2019). Mitochondrial Biogenesis Induced by the β2-adrenergic Receptor Agonist Formoterol Accelerates Podocyte Recovery from Glomerular Injury. Kidney Int. 96 (3), 656–673. doi:10.1016/j.kint.2019.03.023
Ayalon R., Beck L. H. (2015). Membranous Nephropathy: Not Just a Disease for Adults. Pediatr. Nephrol. 30 (1), 31–39. doi:10.1007/s00467-013-2717-z
Babar I. A., Cheng C. J., Booth C. J., Liang X., Weidhaas J. B., Saltzman W. M., et al. (2012). Nanoparticle-based Therapy in an In Vivo microRNA-155 (miR-155)-dependent Mouse Model of Lymphoma. Proc. Natl. Acad. Sci. U.S.A. 109 (26), E1695–E1704. doi:10.1073/pnas.1201516109
Badal S. S., Wang Y., Long J., Corcoran D. L., Chang B. H., Truong L. D., et al. (2016). miR-93 Regulates Msk2-Mediated Chromatin Remodelling in Diabetic Nephropathy. Nat. Commun. 7, 12076. doi:10.1038/ncomms12076
Bao H., Chen H., Zhu X., Zhang M., Yao G., Yu Y., et al. (2014a). MiR-223 Downregulation Promotes Glomerular Endothelial Cell Activation by Upregulating Importin α4 and α5 in IgA Nephropathy. Kidney Int. 85 (3), 624–635. doi:10.1038/ki.2013.469
Bao H., Hu S., Zhang C., Shi S., Qin W., Zeng C., et al. (2014b). Inhibition of miRNA-21 Prevents Fibrogenic Activation in Podocytes and Tubular Cells in IgA Nephropathy. Biochem. Biophysical Res. Commun. 444 (4), 455–460. doi:10.1016/j.bbrc.2014.01.065
Barbagallo C., Passanisi R., Mirabella F., Cirnigliaro M., Costanzo A., Lauretta G., et al. (2019). Upregulated microRNAs in Membranous Glomerulonephropathy Are Associated with Significant Downregulation of IL6 and MYC mRNAs. J. Cell. Physiology 234 (8), 12625–12636. doi:10.1002/jcp.27851
Beg M. S., Brenner A. J., Sachdev J., Borad M., Kang Y.-K., Stoudemire J., et al. (2017). Phase I Study of MRX34, a Liposomal miR-34a Mimic, Administered Twice Weekly in Patients with Advanced Solid Tumors. Invest. New Drugs 35 (2), 180–188. doi:10.1007/s10637-016-0407-y
Blanco-Domínguez R., Sánchez-Díaz R., de la Fuente H., Jiménez-Borreguero L. J., Matesanz-Marín A., Relaño M., et al. (2021). A Novel Circulating MicroRNA for the Detection of Acute Myocarditis. N. Engl. J. Med. 384 (21), 2014–2027. doi:10.1056/NEJMoa2003608
Borchert G. M., Lanier W., Davidson B. L. (2006). RNA Polymerase III Transcribes Human microRNAs. Nat. Struct. Mol. Biol. 13 (12), 1097–1101. doi:10.1038/nsmb1167
Cai X., Xia Z., Zhang C., Luo Y., Gao Y., Fan Z., et al. (2013). Serum microRNAs Levels in Primary Focal Segmental Glomerulosclerosis. Pediatr. Nephrol. 28 (9), 1797–1801. doi:10.1007/s00467-013-2434-7
Cattran D. C., Brenchley P. E. (2017). Membranous Nephropathy: Integrating Basic Science into Improved Clinical Management. Kidney Int. 91 (3), 566–574. doi:10.1016/j.kint.2016.09.048
Chen W., Lin X., Huang J., Tan K., Chen Y., Peng W., et al. (2014). Integrated Profiling of microRNA Expression in Membranous Nephropathy Using High-Throughput Sequencing Technology. Int. J. Mol. Med. 33 (1), 25–34. doi:10.3892/ijmm.2013.1554
Clement L. C., Avila-Casado C., Macé C., Soria E., Bakker W. W., Kersten S., et al. (2011). Podocyte-secreted Angiopoietin-Like-4 Mediates Proteinuria in Glucocorticoid-Sensitive Nephrotic Syndrome. Nat. Med. 17 (1), 117–122. doi:10.1038/nm.2261
Cohen S. D., Kopp J. B., Kimmel P. L. (2017). Kidney Diseases Associated with Human Immunodeficiency Virus Infection. N. Engl. J. Med. 377 (24), 2363–2374. doi:10.1056/NEJMra1508467
Colucci M., Carsetti R., Rosado M. M., Cascioli S., Bruschi M., Candiano G., et al. (2019). Atypical IgM on T Cells Predict Relapse and Steroid Dependence in Idiopathic Nephrotic Syndrome. Kidney Int. 96 (4), 971–982. doi:10.1016/j.kint.2019.04.006
Couser W. G. (2017). Primary Membranous Nephropathy. Clin. J. Am. Soc. Nephrol. 12 (6), 983–997. doi:10.2215/cjn.11761116
Craici I. M., Wagner S. J., Weissgerber T. L., Grande J. P., Garovic V. D. (2014). Advances in the Pathophysiology of Pre-eclampsia and Related Podocyte Injury. Kidney Int. 86 (2), 275–285. doi:10.1038/ki.2014.17
D'Agati V. D., Chagnac A., de Vries A. P. J., Levi M., Porrini E., Herman-Edelstein M., et al. (2016). Obesity-related Glomerulopathy: Clinical and Pathologic Characteristics and Pathogenesis. Nat. Rev. Nephrol. 12 (8), 453–471. doi:10.1038/nrneph.2016.75
D'Agati V. D., Kaskel F. J., Falk R. J. (2011). Focal Segmental Glomerulosclerosis. N. Engl. J. Med. 365 (25), 2398–2411. doi:10.1056/NEJMra1106556
Dai Y., Sui W., Lan H., Yan Q., Huang H., Huang Y. (2008). Microarray Analysis of Micro-ribonucleic Acid Expression in Primary Immunoglobulin A Nephropathy. Saudi Med. J. 29 (10), 1388–1393. doi:10.1007/s00296-008-0758-6
de Boer I. H., Rue T. C., Hall Y. N., Heagerty P. J., Weiss N. S., Himmelfarb J. (2011). Temporal Trends in the Prevalence of Diabetic Kidney Disease in the United States. Jama 305 (24), 2532–2539. doi:10.1001/jama.2011.861
Ding H., Li J., Li Y., Yang M., Nie S., Zhou M., et al. (2021). MicroRNA-10 Negatively Regulates Inflammation in Diabetic Kidney via Targeting Activation of the NLRP3 Inflammasome. Mol. Ther. 29 (7), 2308–2320. doi:10.1016/j.ymthe.2021.03.012
Doshi S. M., Friedman A. N. (2017). Diagnosis and Management of Type 2 Diabetic Kidney Disease. Clin. J. Am. Soc. Nephrol. 12 (8), 1366–1373. doi:10.2215/cjn.11111016
Eng D. G., Sunseri M. W., Kaverina N. V., Roeder S. S., Pippin J. W., Shankland S. J. (2015). Glomerular Parietal Epithelial Cells Contribute to Adult Podocyte Regeneration in Experimental Focal Segmental Glomerulosclerosis. Kidney Int. 88 (5), 999–1012. doi:10.1038/ki.2015.152
Fan Y., Chen H., Huang Z., Zheng H., Zhou J. (2020). Emerging Role of miRNAs in Renal Fibrosis. RNA Biol. 17 (1), 1–12. doi:10.1080/15476286.2019.1667215
Filipowicz W., Bhattacharyya S. N., Sonenberg N. (2008). Mechanisms of Post-transcriptional Regulation by microRNAs: Are the Answers in Sight? Nat. Rev. Genet. 9 (2), 102–114. doi:10.1038/nrg2290
Floege J., Amann K. (2016). Primary Glomerulonephritides. Lancet 387 (10032), 2036–2048. doi:10.1016/s0140-6736(16)00272-5
Fogo A. B. (2015). Causes and Pathogenesis of Focal Segmental Glomerulosclerosis. Nat. Rev. Nephrol. 11 (2), 76–87. doi:10.1038/nrneph.2014.216
Friedman R. C., Farh K. K.-H., Burge C. B., Bartel D. P. (2009). Most Mammalian mRNAs Are Conserved Targets of microRNAs. Genome Res. 19 (1), 92–105. doi:10.1101/gr.082701.108
Fukuda A., Chowdhury M. A., Venkatareddy M. P., Wang S. Q., Nishizono R., Suzuki T., et al. (2012). Growth-dependent Podocyte Failure Causes Glomerulosclerosis. J. Am. Soc. Nephrol. 23 (8), 1351–1363. doi:10.1681/asn.2012030271
Gebeshuber C. A., Kornauth C., Dong L., Sierig R., Seibler J., Reiss M., et al. (2013). Focal Segmental Glomerulosclerosis Is Induced by microRNA-193a and its Downregulation of WT1. Nat. Med. 19 (4), 481–487. doi:10.1038/nm.3142
Gomez I. G., MacKenna D. A., Johnson B. G., Kaimal V., Roach A. M., Ren S., et al. (2015). Anti-microRNA-21 Oligonucleotides Prevent Alport Nephropathy Progression by Stimulating Metabolic Pathways. J. Clin. Invest. 125 (1), 141–156. doi:10.1172/jci75852
Guo N., Guo J., Su D. (2016). MicroRNA-206 and its Down-Regulation of Wilms'Tumor-1 Dictate Podocyte Health in Adriamycin-Induced Nephropathy. Ren. Fail. 38 (6), 989–995. doi:10.3109/0886022x.2016.1165119
Guo Y., Liao Y. (2017). miR-200bc/429 Cluster Alleviates Inflammation in IgA Nephropathy by Targeting TWEAK/Fn14. Int. Immunopharmacol. 52, 150–155. doi:10.1016/j.intimp.2017.09.002
Hama T., Nakanishi K., Shima Y., Mukaiyama H., Togawa H., Tanaka R., et al. (2012). Renal Biopsy Criterion in Children with Asymptomatic Constant Isolated Proteinuria. Nephrol. Dial. Transplant. 27 (8), 3186–3190. doi:10.1093/ndt/gfr750
Henique C., Bollée G., Loyer X., Grahammer F., Dhaun N., Camus M., et al. (2017). Genetic and Pharmacological Inhibition of microRNA-92a Maintains Podocyte Cell Cycle Quiescence and Limits Crescentic Glomerulonephritis. Nat. Commun. 8 (1), 1829. doi:10.1038/s41467-017-01885-7
Hill G. S., Karoui K. E., Karras A., Mandet C., Van Huyen J.-P. D., Nochy D., et al. (2011). Focal Segmental Glomerulosclerosis Plays a Major Role in the Progression of IgA Nephropathy. I. Immunohistochemical Studies. Kidney Int. 79 (6), 635–642. doi:10.1038/ki.2010.466
Hong D. S., Kang Y.-K., Borad M., Sachdev J., Ejadi S., Lim H. Y., et al. (2020). Phase 1 Study of MRX34, a Liposomal miR-34a Mimic, in Patients with Advanced Solid Tumours. Br. J. Cancer 122 (11), 1630–1637. doi:10.1038/s41416-020-0802-1
Ichii O., Otsuka-Kanazawa S., Horino T., Kimura J., Nakamura T., Matsumoto M., et al. (2014). Decreased miR-26a Expression Correlates with the Progression of Podocyte Injury in Autoimmune Glomerulonephritis. PLoS One 9 (10), e110383. doi:10.1371/journal.pone.0110383
Iijima K., Sako M., Nozu K., Mori R., Tuchida N., Kamei K., et al. (2014). Rituximab for Childhood-Onset, Complicated, Frequently Relapsing Nephrotic Syndrome or Steroid-dependent Nephrotic Syndrome: a Multicentre, Double-Blind, Randomised, Placebo-Controlled Trial. Lancet 384 (9950), 1273–1281. doi:10.1016/s0140-6736(14)60541-9
Janssen H. L. A., Reesink H. W., Lawitz E. J., Zeuzem S., Rodriguez-Torres M., Patel K., et al. (2013). Treatment of HCV Infection by Targeting microRNA. N. Engl. J. Med. 368 (18), 1685–1694. doi:10.1056/NEJMoa1209026
Jin L.-W., Ye H.-Y., Xu X.-Y., Zheng Y., Chen Y. (2018). MiR-133a/133b Inhibits Treg Differentiation in IgA Nephropathy through Targeting FOXP3. Biomed. Pharmacother. 101, 195–200. doi:10.1016/j.biopha.2018.02.022
Kang Y. S., Li Y., Dai C., Kiss L. P., Wu C., Liu Y. (2010). Inhibition of Integrin-Linked Kinase Blocks Podocyte Epithelial-Mesenchymal Transition and Ameliorates Proteinuria. Kidney Int. 78 (4), 363–373. doi:10.1038/ki.2010.137
Kato M., Arce L., Natarajan R. (2009). MicroRNAs and Their Role in Progressive Kidney Diseases. Clin. J. Am. Soc. Nephrol. 4 (7), 1255–1266. doi:10.2215/cjn.00520109
Kim A. H. J., Chung J.-J., Akilesh S., Koziell A., Jain S., Hodgin J. B., et al. (2017). B Cell-Derived IL-4 Acts on Podocytes to Induce Proteinuria and Foot Process Effacement. JCI Insight 2 (21), e81836. doi:10.1172/jci.insight.81836
Kölling M., Kaucsar T., Schauerte C., Hübner A., Dettling A., Park J.-K., et al. (2017). Therapeutic miR-21 Silencing Ameliorates Diabetic Kidney Disease in Mice. Mol. Ther. 25 (1), 165–180. doi:10.1016/j.ymthe.2016.08.001
Kong A. P. S., Xiao K., Choi K. C., Wang G., Chan M. H. M., Ho C. S., et al. (2012). Associations between microRNA (miR-21, 126, 155 and 221), Albuminuria and Heavy Metals in Hong Kong Chinese Adolescents. Clin. Chim. Acta 413 (13-14), 1053–1057. doi:10.1016/j.cca.2012.02.014
Kopp J. B., Anders H.-J., Susztak K., Podestà M. A., Remuzzi G., Hildebrandt F., et al. (2020). Podocytopathies. Nat. Rev. Dis. Prim. 6 (1), 68. doi:10.1038/s41572-020-0196-7
Kouri N. M., Stangou M., Lioulios G., Mitsoglou Z., Serino G., Chiurlia S., et al. (2021). Serum Levels of miR-148b and Let-7b at Diagnosis May Have Important Impact in the Response to Treatment and Long-Term Outcome in IgA Nephropathy. Jcm 10 (9), 1987. doi:10.3390/jcm10091987
Krol J., Loedige I., Filipowicz W. (2010). The Widespread Regulation of microRNA Biogenesis, Function and Decay. Nat. Rev. Genet. 11 (9), 597–610. doi:10.1038/nrg2843
Lai K. N., Leung J. C. K., Chan L. Y. Y., Saleem M. A., Mathieson P. W., Lai F. M., et al. (2008). Activation of Podocytes by Mesangial-Derived TNF-α: Glomerulo-Podocytic Communication in IgA Nephropathy. Am. J. Physiology-Renal Physiology 294 (4), F945–F955. doi:10.1152/ajprenal.00423.2007
Lai K. N., Tang S. C. W., Schena F. P., Novak J., Tomino Y., Fogo A. B., et al. (2016). IgA Nephropathy. Nat. Rev. Dis. Prim. 2, 16001. doi:10.1038/nrdp.2016.1
Lazzeri E., Romagnani P. (2015). Differentiation of Parietal Epithelial Cells into Podocytes. Nat. Rev. Nephrol. 11 (1), 7–8. doi:10.1038/nrneph.2014.218
Lee H. W., Khan S. Q., Khaliqdina S., Altintas M. M., Grahammer F., Zhao J. L., et al. (2017). Absence of miR-146a in Podocytes Increases Risk of Diabetic Glomerulopathy via Up-Regulation of ErbB4 and Notch-1. J. Biol. Chem. 292 (2), 732–747. doi:10.1074/jbc.M116.753822
Lee R. C., Feinbaum R. L., Ambros V. (1993). The C. elegans Heterochronic Gene Lin-4 Encodes Small RNAs with Antisense Complementarity to Lin-14. Cell. 75 (5), 843–854. doi:10.1016/0092-8674(93)90529-y
Leung A. K., Wong A. H., Barg S. S. (2017). Proteinuria in Children: Evaluation and Differential Diagnosis. Am. Fam. Physician 95 (4), 248–254. Available at: https://www.aafp.org/pubs/afp/issues/2017/0215/p248.html
Li C., Shi J., Zhao Y. (2018). MiR‐320 Promotes B Cell Proliferation and the Production of Aberrant Glycosylated IgA1 in IgA Nephropathy. J. Cell. Biochem. 119 (6), 4607–4614. doi:10.1002/jcb.26628
Li H., Chen Z., Chen W., Li J., Liu Y., Ma H., et al. (2021a). MicroRNA-23b-3p Deletion Induces an IgA Nephropathy-like Disease Associated with Dysregulated Mucosal IgA Synthesis. J. Am. Soc. Nephrol. 32 (10), 2561–2578. doi:10.1681/asn.2021010133
Li J., Liu B., Xue H., Zhou Q. Q., Peng L. (2017). miR-217 Is a Useful Diagnostic Biomarker and Regulates Human Podocyte Cells Apoptosis via Targeting TNFSF11 in Membranous Nephropathy. BioMed Res. Int. 2017, 1–9. doi:10.1155/2017/2168767
Li Y., Xia M., Peng L., Liu H., Chen G., Wang C., et al. (2021b). Downregulation of miR-214-3p A-ttenuates M-esangial H-ypercellularity by T-argeting PTEN-mediated JNK/c-Jun S-ignaling in IgA N-ephropathy. Int. J. Biol. Sci. 17 (13), 3343–3355. doi:10.7150/ijbs.61274
Liang Y., Zhao G., Tang L., Zhang J., Li T., Liu Z. (2016). MiR-100-3p and miR-877-3p Regulate Overproduction of IL-8 and IL-1β in Mesangial Cells Activated by Secretory IgA from IgA Nephropathy Patients. Exp. Cell. Res. 347 (2), 312–321. doi:10.1016/j.yexcr.2016.08.011
Lin C.-L., Lee P.-H., Hsu Y.-C., Lei C.-C., Ko J.-Y., Chuang P.-C., et al. (2014). MicroRNA-29a Promotion of Nephrin Acetylation Ameliorates Hyperglycemia-Induced Podocyte Dysfunction. J. Am. Soc. Nephrol. 25 (8), 1698–1709. doi:10.1681/asn.2013050527
Liu D., Liu F., Wang X., Qiao Y., Pan S., Yang Y., et al. (2018). MiR-130a-5p Prevents Angiotensin II-Induced Podocyte Apoptosis by Modulating M-type Phospholipase A2 Receptor. Cell. Cycle 17 (21-22), 2484–2495. doi:10.1080/15384101.2018.1542901
Liu F., Chen J., Li Z., Meng X. (2022). Recent Advances in Epigenetics of Age-Related Kidney Diseases. Genes 13 (5), 796. doi:10.3390/genes13050796
Liu Y., Li H., Liu J., Han P., Li X., Bai H., et al. (2017). Variations in MicroRNA-25 Expression Influence the Severity of Diabetic Kidney Disease. J. Am. Soc. Nephrol. 28 (12), 3627–3638. doi:10.1681/asn.2015091017
Long J., Wang Y., Wang W., Chang B. H. J., Danesh F. R. (2011). MicroRNA-29c Is a Signature microRNA under High Glucose Conditions that Targets Sprouty Homolog 1, and its In Vivo Knockdown Prevents Progression of Diabetic Nephropathy. J. Biol. Chem. 286 (13), 11837–11848. doi:10.1074/jbc.M110.194969
Lu M., Wang C., Yuan Y., Zhu Y., Yin Z., Xia Z., et al. (2015). Differentially Expressed microRNAs in Kidney Biopsies from Various Subtypes of Nephrotic Children. Exp. Mol. Pathology 99 (3), 590–595. doi:10.1016/j.yexmp.2015.10.003
Luyckx V. A., Perico N., Somaschini M., Manfellotto D., Valensise H., Cetin I., et al. (2017). A Developmental Approach to the Prevention of Hypertension and Kidney Disease: a Report from the Low Birth Weight and Nephron Number Working Group. Lancet 390 (10092), 424–428. doi:10.1016/s0140-6736(17)30576-7
Maas R. J., Deegens J. K., Smeets B., Moeller M. J., Wetzels J. F. (2016). Minimal Change Disease and Idiopathic FSGS: Manifestations of the Same Disease. Nat. Rev. Nephrol. 12 (12), 768–776. doi:10.1038/nrneph.2016.147
Mathieson P. W. (2011). The Podocyte as a Target for Therapies-New and Old. Nat. Rev. Nephrol. 8 (1), 52–56. doi:10.1038/nrneph.2011.171
Maurer B., Stanczyk J., Jüngel A., Akhmetshina A., Trenkmann M., Brock M., et al. (2010). MicroRNA-29, a Key Regulator of Collagen Expression in Systemic Sclerosis. Arthritis & Rheumatism 62 (6), 1733–1743. doi:10.1002/art.27443
Medjeral-Thomas N. R., Lawrence C., Condon M., Sood B., Warwicker P., Brown H., et al. (2020). Randomized, Controlled Trial of Tacrolimus and Prednisolone Monotherapy for Adults withDe NovoMinimal Change Disease. Clin. J. Am. Soc. Nephrol. 15 (2), 209–218. doi:10.2215/cjn.06180519
Mellis D., Caporali A. (2018). MicroRNA-based Therapeutics in Cardiovascular Disease: Screening and Delivery to the Target. Biochem. Soc. Trans. 46 (1), 11–21. doi:10.1042/bst20170037
Ming L., Ning J., Ge Y., Zhang Y., Ruan Z. (2019). Excessive Apoptosis of Podocytes Caused by Dysregulation of microRNA‐182‐5p and CD2AP Confers to an Increased Risk of Diabetic Nephropathy. J Cell. Biochem. 120 (10), 16516–16523. doi:10.1002/jcb.28911
Mishra A., Ayasolla K., Kumar V., Lan X., Vashistha H., Aslam R., et al. (2018). Modulation of Apolipoprotein L1-microRNA-193a axis Prevents Podocyte Dedifferentiation in High-Glucose Milieu. Am. J. Physiology-Renal Physiology 314 (5), F832–f843. doi:10.1152/ajprenal.00541.2017
Mundel P., Reiser J. (2010). Proteinuria: an Enzymatic Disease of the Podocyte? Kidney Int. 77 (7), 571–580. doi:10.1038/ki.2009.424
Mundel P., Shankland S. J. (2002). Podocyte Biology and Response to Injury. J. Am. Soc. Nephrol. 13 (12), 3005–3015. doi:10.1097/01.asn.0000039661.06947.fd
Munyentwali H., Bouachi K., Audard V., Remy P., Lang P., Mojaat R., et al. (2013). Rituximab Is an Efficient and Safe Treatment in Adults with Steroid-dependent Minimal Change Disease. Kidney Int. 83 (3), 511–516. doi:10.1038/ki.2012.444
Nagata M. (2016). Podocyte Injury and its Consequences. Kidney Int. 89 (6), 1221–1230. doi:10.1016/j.kint.2016.01.012
Nasr S. H., Kopp J. B. (2020). COVID-19-Associated Collapsing Glomerulopathy: An Emerging Entity. Kidney Int. Rep. 5 (6), 759–761. doi:10.1016/j.ekir.2020.04.030
Ollero M., Sahali D. (2015). Inhibition of the VEGF Signalling Pathway and Glomerular Disorders. Nephrol. Dial. Transpl. 30 (9), 1449–1455. doi:10.1093/ndt/gfu368
Pawluczyk I. Z. A., Didangelos A., Barbour S. J., Er L., Becker J. U., Martin R., et al. (2021). Differential Expression of microRNA miR-150-5p in IgA Nephropathy as a Potential Mediator and Marker of Disease Progression. Kidney Int. 99 (5), 1127–1139. doi:10.1016/j.kint.2020.12.028
Peired A., Angelotti M. L., Ronconi E., la Marca G., Mazzinghi B., Sisti A., et al. (2013). Proteinuria Impairs Podocyte Regeneration by Sequestering Retinoic Acid. J. Am. Soc. Nephrol. 24 (11), 1756–1768. doi:10.1681/asn.2012090950
Puelles V. G., van der Wolde J. W., Wanner N., Scheppach M. W., Cullen-McEwen L. A., Bork T., et al. (2019). mTOR-Mediated Podocyte Hypertrophy Regulates Glomerular Integrity in Mice and Humans. JCI Insight 4 (18), e99271. doi:10.1172/jci.insight.99271
Qi H., Fu J., Luan J., Jiao C., Cui X., Cao X., et al. (2020). miR-150 Inhibitor Ameliorates Adriamycin-Induced Focal Segmental Glomerulosclerosis. Biochem. Biophysical Res. Commun. 522 (3), 618–625. doi:10.1016/j.bbrc.2019.11.096
Qiu C., Huang S., Park J., Park Y., Ko Y.-A., Seasock M. J., et al. (2018). Renal Compartment-specific Genetic Variation Analyses Identify New Pathways in Chronic Kidney Disease. Nat. Med. 24 (11), 1721–1731. doi:10.1038/s41591-018-0194-4
Ramezani A., Devaney J. M., Cohen S., Wing M. R., Scott R., Knoblach S., et al. (2015). Circulating and Urinary microRNA Profile in Focal Segmental Glomerulosclerosis: a Pilot Study. Eur. J. Clin. Invest. 45 (4), 394–404. doi:10.1111/eci.12420
Reidy K., Kang H. M., Hostetter T., Susztak K. (2014). Molecular Mechanisms of Diabetic Kidney Disease. J. Clin. Invest. 124 (6), 2333–2340. doi:10.1172/jci72271
Roberts I. S. D. (2014). Pathology of IgA Nephropathy. Nat. Rev. Nephrol. 10 (8), 445–454. doi:10.1038/nrneph.2014.92
Rodrigues J. C., Haas M., Reich H. N. (2017). IgA Nephropathy. Clin. J. Am. Soc. Nephrol. 12 (4), 677–686. doi:10.2215/cjn.07420716
Ronco P., Beck L., Debiec H., Fervenza F. C., Hou F. F., Jha V., et al. (2021). Membranous Nephropathy. Nat. Rev. Dis. Prim. 7 (1), 69. doi:10.1038/s41572-021-00303-z
Ronconi E., Sagrinati C., Angelotti M. L., Lazzeri E., Mazzinghi B., Ballerini L., et al. (2009). Regeneration of Glomerular Podocytes by Human Renal Progenitors. J. Am. Soc. Nephrol. 20 (2), 322–332. doi:10.1681/asn.2008070709
Rosenberg A. Z., Kopp J. B. (2017). Focal Segmental Glomerulosclerosis. Clin. J. Am. Soc. Nephrol. 12 (3), 502–517. doi:10.2215/cjn.05960616
Ruggenenti P., Fervenza F. C., Remuzzi G. (2017). Treatment of Membranous Nephropathy: Time for a Paradigm Shift. Nat. Rev. Nephrol. 13 (9), 563–579. doi:10.1038/nrneph.2017.92
Schober A., Blay R. M., Saboor Maleki S., Zahedi F., Winklmaier A. E., Kakar M. Y., et al. (2021). MicroRNA-21 Controls Circadian Regulation of Apoptosis in Atherosclerotic Lesions. Circulation 144 (13), 1059–1073. doi:10.1161/circulationaha.120.051614
Serino G., Pesce F., Sallustio F., De Palma G., Cox S. N., Curci C., et al. (2016). In a Retrospective International Study, Circulating miR-148b and Let-7b Were Found to Be Serum Markers for Detecting Primary IgA Nephropathy. Kidney Int. 89 (3), 683–692. doi:10.1038/ki.2015.333
Serino G., Sallustio F., Cox S. N., Pesce F., Schena F. P. (2012). Abnormal miR-148b Expression Promotes Aberrant Glycosylation of IgA1 in IgA Nephropathy. J. Am. Soc. Nephrol. 23 (5), 814–824. doi:10.1681/asn.2011060567
Sha W. G., Shen L., Zhou L., Xu D.-y., Lu G. Y. (2015). Down-regulation of miR-186 Contributes to Podocytes Apoptosis in Membranous Nephropathy. Biomed. Pharmacother. 75, 179–184. doi:10.1016/j.biopha.2015.07.021
Shankland S. J. (2006). The Podocyte's Response to Injury: Role in Proteinuria and Glomerulosclerosis. Kidney Int. 69 (12), 2131–2147. doi:10.1038/sj.ki.5000410
Shi S., Yu L., Chiu C., Sun Y., Chen J., Khitrov G., et al. (2008). Podocyte-selective Deletion of Dicer Induces Proteinuria and Glomerulosclerosis. J. Am. Soc. Nephrol. 19 (11), 2159–2169. doi:10.1681/asn.2008030312
Szeto C.-C., Li P. K.-T. (2014). MicroRNAs in IgA Nephropathy. Nat. Rev. Nephrol. 10 (5), 249–256. doi:10.1038/nrneph.2014.50
Tan K., Chen J., Li W., Chen Y., Sui W., Zhang Y., et al. (2013). Genome-wide Analysis of microRNAs Expression Profiling in Patients with Primary IgA Nephropathy. Genome 56 (3), 161–169. doi:10.1139/gen-2012-0159
Tonneijck L., Muskiet M. H. A., Smits M. M., van Bommel E. J., Heerspink H. J. L., van Raalte D. H., et al. (2017). Glomerular Hyperfiltration in Diabetes: Mechanisms, Clinical Significance, and Treatment. J. Am. Soc. Nephrol. 28 (4), 1023–1039. doi:10.1681/asn.2016060666
Torban E., Braun F., Wanner N., Takano T., Goodyer P. R., Lennon R., et al. (2019). From Podocyte Biology to Novel Cures for Glomerular Disease. Kidney Int. 96 (4), 850–861. doi:10.1016/j.kint.2019.05.015
Trajkovski M., Hausser J., Soutschek J., Bhat B., Akin A., Zavolan M., et al. (2011). MicroRNAs 103 and 107 Regulate Insulin Sensitivity. Nature 474 (7353), 649–653. doi:10.1038/nature10112
Treiber T., Treiber N., Meister G. (2019). Regulation of microRNA Biogenesis and its Crosstalk with Other Cellular Pathways. Nat. Rev. Mol. Cell. Biol. 20 (1), 5–20. doi:10.1038/s41580-018-0059-1
Trionfini P., Benigni A. (2017). MicroRNAs as Master Regulators of Glomerular Function in Health and Disease. J. Am. Soc. Nephrol. 28 (6), 1686–1696. doi:10.1681/asn.2016101117
Trionfini P., Benigni A., Remuzzi G. (2015). MicroRNAs in Kidney Physiology and Disease. Nat. Rev. Nephrol. 11 (1), 23–33. doi:10.1038/nrneph.2014.202
van Zandwijk N., Pavlakis N., Kao S. C., Linton A., Boyer M. J., Clarke S., et al. (2017). Safety and Activity of microRNA-Loaded Minicells in Patients with Recurrent Malignant Pleural Mesothelioma: a First-In-Man, Phase 1, Open-Label, Dose-Escalation Study. Lancet Oncol. 18 (10), 1386–1396. doi:10.1016/s1470-2045(17)30621-6
Vivarelli M., Massella L., Ruggiero B., Emma F. (2017). Minimal Change Disease. Clin. J. Am. Soc. Nephrol. 12 (2), 332–345. doi:10.2215/cjn.05000516
Waldman M., Crew R. J., Valeri A., Busch J., Stokes B., Markowitz G., et al. (2007). Adult Minimal-Change Disease: Clinical Characteristics, Treatment, and Outcomes. Clin. J. Am. Soc. Nephrol. 2 (3), 445–453. doi:10.2215/cjn.03531006
Wang G., Kwan B. C.-H., Lai F. M.-M., Chow K.-M., Li P. K.-T., Szeto C.-C. (2011). Elevated Levels of miR-146a and miR-155 in Kidney Biopsy and Urine from Patients with IgA Nephropathy. Dis. Markers 30 (4), 171–179. doi:10.3233/dma-2011-076610.1155/2011/304852
Wang L., Wang J., Wang Z., Zhou J., Zhang Y. (2021). Higher Urine Exosomal miR-193a Is Associated with a Higher Probability of Primary Focal Segmental Glomerulosclerosis and an Increased Risk of Poor Prognosis Among Children with Nephrotic Syndrome. Front. Cell. Dev. Biol. 9, 727370. doi:10.3389/fcell.2021.727370
Wang X., Lin B., Nie L., Li P. (2017). microRNA-20b Contributes to High Glucose-Induced Podocyte Apoptosis by Targeting SIRT7. Mol. Med. Rep. 16 (4), 5667–5674. doi:10.3892/mmr.2017.7224
Webster A. C., Nagler E. V., Morton R. L., Masson P. (2017). Chronic Kidney Disease. Lancet 389 (10075), 1238–1252. doi:10.1016/s0140-6736(16)32064-5
Wei C., El Hindi S., Li J., Fornoni A., Goes N., Sageshima J., et al. (2011). Circulating Urokinase Receptor as a Cause of Focal Segmental Glomerulosclerosis. Nat. Med. 17 (8), 952–960. doi:10.1038/nm.2411
Wen L., Zhao Z., Xiao J., Wang Z., He X., Birn H. (2018). Renal miR-148b Is Associated with Megalin Down-Regulation in IgA Nephropathy. Biosci. Rep. 38 (6), BSR20181578. doi:10.1042/bsr20181578
Wiggins R.-C. (2007). The Spectrum of Podocytopathies: a Unifying View of Glomerular Diseases. Kidney Int. 71 (12), 1205–1214. doi:10.1038/sj.ki.5002222
Williams A. M., Jensen D. M., Pan X., Liu P., Liu J., Huls S., et al. (2022). Histologically Resolved Small RNA Maps in Primary Focal Segmental Glomerulosclerosis Indicate Progressive Changes within Glomerular and Tubulointerstitial Regions. Kidney Int. 101 (4), 766–778. doi:10.1016/j.kint.2021.12.030
Wu J., Zheng C., Fan Y., Zeng C., Chen Z., Qin W., et al. (2014). Downregulation of microRNA-30 Facilitates Podocyte Injury and Is Prevented by Glucocorticoids. J. Am. Soc. Nephrol. 25 (1), 92–104. doi:10.1681/asn.2012111101
Wu J., Zheng C., Wang X., Yun S., Zhao Y., Liu L., et al. (2015). MicroRNA-30 Family Members Regulate Calcium/calcineurin Signaling in Podocytes. J. Clin. Invest. 125 (11), 4091–4106. doi:10.1172/jci81061
Wu L., Zhang X., Luo L., Li X., Liu Y., Qin X. (2021). Altered Expression of Serum miR‐106a, miR‐19b, miR‐17, and PTEN in Patients with Idiopathic Membranous Nephropathy. J. Clin. Lab. Anal. 35 (4), e23737. doi:10.1002/jcla.23737
Wyatt R. J., Julian B. A. (2013). IgA Nephropathy. N. Engl. J. Med. 368 (25), 2402–2414. doi:10.1056/NEJMra1206793
Xiao B., Wang L.-N., Li W., Gong L., Yu T., Zuo Q.-F., et al. (2018). Plasma microRNA Panel Is a Novel Biomarker for Focal Segmental Glomerulosclerosis and Associated with Podocyte Apoptosis. Cell. Death Dis. 9 (5), 533. doi:10.1038/s41419-018-0569-y
Yang X., Wu D., Du H., Nie F., Pang X., Xu Y. (2017). MicroRNA-135a Is Involved in Podocyte Injury in a Transient Receptor Potential Channel 1-dependent Manner. Int. J. Mol. Med. 40 (5), 1511–1519. doi:10.3892/ijmm.2017.3152
Yates L. A., Norbury C. J., Gilbert R. J. C. (2013). The Long and Short of microRNA. Cell. 153 (3), 516–519. doi:10.1016/j.cell.2013.04.003
Zha F., Bai L., Tang B., Li J., Wang Y., Zheng P., et al. (2019). MicroRNA‐503 Contributes to Podocyte Injury via Targeting E2F3 in Diabetic Nephropathy. J Cell. Biochem. 120 (8), 12574–12581. doi:10.1002/jcb.28524
Zhai Y., Qi Y., Long X., Dou Y., Liu D., Cheng G., et al. (2019). Elevated hsa‐miR‐590‐3p Expression Down‐regulates HMGB2 Expression and Contributes to the Severity of IgA Nephropathy. J. Cell. Mol. Med. 23 (11), 7299–7309. doi:10.1111/jcmm.14582
Zhang C., Zhang W., Chen H.-M., Liu C., Wu J., Shi S., et al. (2015). Plasma microRNA-186 and Proteinuria in Focal Segmental Glomerulosclerosis. Am. J. Kidney Dis. 65 (2), 223–232. doi:10.1053/j.ajkd.2014.07.013
Zhang K., Sun W., Zhang L., Xu X., Wang J., Hong Y. (2018). miR-499 Ameliorates Podocyte Injury by Targeting Calcineurin in Minimal Change Disease. Am. J. Nephrol. 47 (2), 94–102. doi:10.1159/000486967
Zhang W., Zhang C., Chen H., Li L., Tu Y., Liu C., et al. (2014). Evaluation of microRNAs miR-196a, miR-30a-5P, and miR-490 as Biomarkers of Disease Activity Among Patients with FSGS. Clin. J. Am. Soc. Nephrol. 9 (9), 1545–1552. doi:10.2215/cjn.11561113
Zhao B., Li H., Liu J., Han P., Zhang C., Bai H., et al. (2016). MicroRNA-23b Targets Ras GTPase-Activating Protein SH3 Domain-Binding Protein 2 to Alleviate Fibrosis and Albuminuria in Diabetic Nephropathy. J. Am. Soc. Nephrol. 27 (9), 2597–2608. doi:10.1681/asn.2015030300
Zhao J., Liu Z. (2020). Treatment of Nephrotic Syndrome: Going beyond Immunosuppressive Therapy. Pediatr. Nephrol. 35 (4), 569–579. doi:10.1007/s00467-019-04225-7
Zhdanova O., Srivastava S., Di L., Li Z., Tchelebi L., Dworkin S., et al. (2011). The Inducible Deletion of Drosha and microRNAs in Mature Podocytes Results in a Collapsing Glomerulopathy. Kidney Int. 80 (7), 719–730. doi:10.1038/ki.2011.122
Zheng Z., Hu H., Tong Y., Hu Z., Cao S., Shan C., et al. (2018). MiR-27b Regulates Podocyte Survival through Targeting Adenosine Receptor 2B in Podocytes from Non-human Primate. Cell. Death Dis. 9 (11), 1133. doi:10.1038/s41419-018-1178-5
Zhou G., Zhang X., Wang W., Zhang W., Wang H., Xin G. (2019). Both Peripheral Blood and Urinary miR-195-5p, miR-192-3p, miR-328-5p and Their Target Genes PPM1A, RAB1A and BRSK1 May Be Potential Biomarkers for Membranous Nephropathy. Med. Sci. Monit. 25, 1903–1916. doi:10.12659/msm.913057
Zhou L., Liu Y. (2015). Wnt/β-catenin Signalling and Podocyte Dysfunction in Proteinuric Kidney Disease. Nat. Rev. Nephrol. 11 (9), 535–545. doi:10.1038/nrneph.2015.88
Zhou W., Dong S., Chen Z., Li X., Jiang W. (2022). New Challenges for microRNAs in Acute Pancreatitis: Progress and Treatment. J. Transl. Med. 20 (1), 192. doi:10.1186/s12967-022-03338-2
Keywords: microRNA, podocytopathy, therapeutic target, focal segmental glomerulosclerosis, minimal change disease, membranous nephropathy, diabetic kidney disease, IgA nephropathy
Citation: Liu F, Chen J, Luo C and Meng X (2022) Pathogenic Role of MicroRNA Dysregulation in Podocytopathies. Front. Physiol. 13:948094. doi: 10.3389/fphys.2022.948094
Received: 19 May 2022; Accepted: 10 June 2022;
Published: 29 June 2022.
Edited by:
Vishal Patel, University of Texas Southwestern Medical Center, United StatesReviewed by:
Geetanjali Chawla, Regional Centre for Biotechnology (RCB), IndiaChen Yang, Affiliated Hospital of Guangdong Medical University, China
Copyright © 2022 Liu, Chen, Luo and Meng. This is an open-access article distributed under the terms of the Creative Commons Attribution License (CC BY). The use, distribution or reproduction in other forums is permitted, provided the original author(s) and the copyright owner(s) are credited and that the original publication in this journal is cited, in accordance with accepted academic practice. No use, distribution or reproduction is permitted which does not comply with these terms.
*Correspondence: Changqing Luo, luocq1021@163.com; Xianfang Meng, xfmeng@mails.tjmu.edu.cn
†These authors have contributed equally to this work and share first authorship