- 1Shaanxi Academy of Traditional Chinese Medicine, Xi’an, Shaanxi, China
- 2Jiangsu Provincial Key Laboratory of Cardiovascular and Cerebrovascular Medicine, School of Pharmacy, Nanjing Medical University, Nanjing, Jiangsu, China
- 3Xi’an Institute for Food and Drug Control, Xi’an, Shaanxi, China
- 4School of Food and Biological Engineering, Jiangsu University, Zhenjiang, Jiangsu, China
There are about 20 species of Porana Burm. f. worldwide in tropical and subtropical Asia, Africa and neighboring islands, Oceania, and the Americas. In China, India, and other places, this genus enjoys a wealth of experience in folk applications. Nevertheless, the chemical composition of only five species has been reported, and 59 compounds have been isolated and identified, including steroids, coumarins, flavonoids, quinic acid derivatives, and amides. Pharmacological studies revealed that extracts from this genus and their bioactive components exhibit anti-inflammatory, analgesic, antioxidant, anti-gout, anti-cancer, and anti-diabetic effects. Although this genus is abundant, the development of its pharmacological applications remains limited. This review will systematically summarize the traditional and current uses, chemical compositions, and pharmacological activities of various Porana species. Network analysis was introduced to compare and confirm its output with current research progress to explore the potential targets and pathways of chemical components in this genus. We hope to increase understanding of this genus’s medicinal value and suggest directions for rational medicinal development.
1 Introduction
There are more than 20 species of Porana Burm. f. worldwide in tropical and subtropical Asia, Africa and neighboring islands, Oceania, and the Americas. Fifteen species are displayed in Table 1 (for more information, see http://www.plantsoftheworldonline.org or www.theplantlist.org). The global distribution of Porana plants based on the Global Biodiversity Information Facility (https://www.gbif.org/) and the herbarium diagrams of three mainstream species are shown in Figure 1.
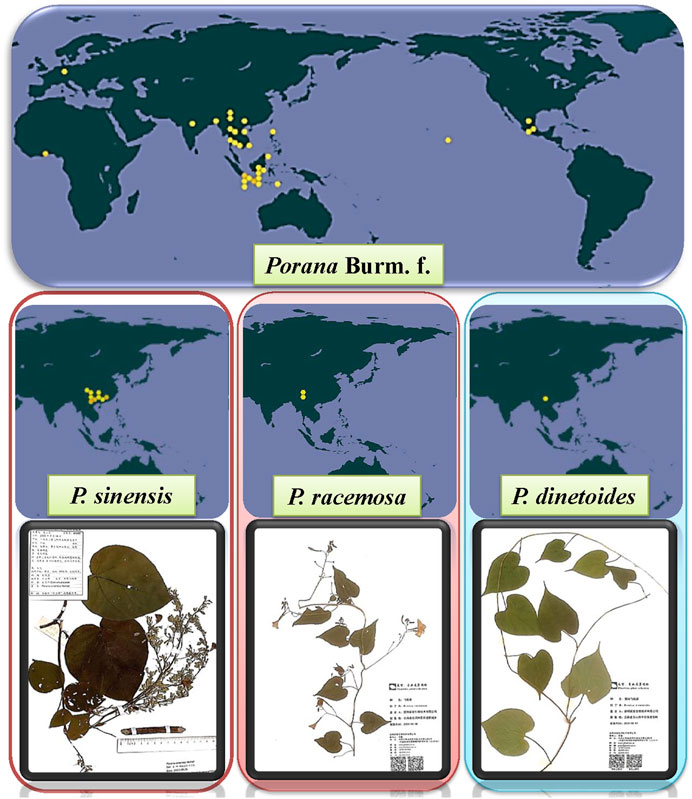
FIGURE 1. The global distribution of Porana Burm. f., and the plant specimens of P. sinensis, P. racemosa, and P. dinetoides.
Porana plants are vines, woody, herbaceous, or climbing shrubs. Their ovate leaves are mostly cordate at the base, with petioles. The inflorescence morphology of Porana plants is divided into racemes or panicles, with some single-flower forms. Their bracts are leaflike, small and subulate, or absent. Their corollas are neatly arranged, presenting white, reddish, and some lavender. The ovaries are primarily glabrous. Some are one-celled, containing two ovules, while some are one-to two-celled, containing two to four ovules. Their stigmas are spherical, each connecting to the ovary by one style. Capsules of Porana plants are relatively small, sub-globose to oblong, dehiscent in two petals, or not dehiscent. Porana plants usually have only one spherical and glabrous seed (Chen et al., 2004).
The medicinal records of Porana plants are extensive. Porana paniculata Roxb. has been used in folk medicine to treat pain and inflammation in Ayurveda and India (Kumar et al., 2015). Porana sinensis Hemsl. is a direct substitute for commercial Dingongteng medicinal materials and is known for its therapeutic effect on rheumatoid arthritis and bruises (Ren et al., 2019). According to the National Compendium of Chinese Herbal Medicine, the whole plant of Porana racemosa Roxb. is used to treat colds and indigestion (Guoqiang, 2014), while its stems and roots are used to treat rheumatism (Liu and Li, 1997). Research on the phytochemistry of Porana plants focuses on Porana discifera C.K.Schneid., P. racemosa, P. sinensis, Porana spectabilis Kurz, and Porana duclouxii Gagnep. & Courchet; 59 compounds have been isolated from Porana plants, including 14 steroids, six coumarins, seven flavonoids, six quinic acid derivatives, and three amides (Zhu et al., 2007; Li et al., 2013; Ding et al., 2014; Chen et al., 2015; Xue et al., 2019). Pharmacological studies revealed that the extracts of Porana plants and their bioactive compounds treat arthritis (Dou et al., 2013), gout (Chen et al., 2014; Du et al., 2020), inflammation (Wu et al., 2016; Xue et al., 2019), and cancer (Huang et al., 2019).
Although Porana has a wide range of medicinal uses, and its extracts and bioactive compounds show excellent efficacy, current research remains limited, complicating the investigation of its chemical components, pharmacological activities, quality control, and safety. Therefore, it is critical to perform a systematic literature review on Porana to promote rational medicinal development.
2 Methodology
An extensive search of studies was conducted from scientific journals (original research, reviews, and short communications), books, and reports from internationally recognized databases (Web of Science, PubMed, ScienceDirect, China National Knowledge Infrastructure, and Google Scholar). The following keywords were selected: “Porana,” “pharmacology,” “ethnopharmacology,” “compound,” “phytotherapy,” “biological activity,” “substitute,” “toxicity,” and “quality control.” The bibliographies of all selected articles were scanned to seek additional relevant articles.
3 Traditional uses
The medicinal parts of P. sinensis are canes, which have been used to substitute for the endangered traditional Chinese medicine Dinggongteng (Erycibes caulis) in China (Xue et al., 2017). Dinggongteng is a traditional Chinese folk medicine, first recorded in the Supplement to Medica, which recorded the effect of dispelling wind and strengthening the waist (Shang, 2004). The National Collection of Chinese Herbal Medicine, the Dictionary of Chinese Herbal Medicine, and the Chinese Materia Medica have documented Dinggongteng, which dispels wind and dampness, relaxes tendons, activates collaterals, reduces swelling, and relieves pain. The traditional clinical application of Dinggongteng has been to treat rheumatoid arthritis, bruises, and other diseases, according to the 2020 edition of the Chinese Pharmacopoeia. With E. caulis as the main medicinal material, and more than ten Chinese patent medicines have been developed, including Feng Liaoxing Rheumatism Dieda Liquor and Tengluoning Capsule (Fan et al., 2021; Peng et al., 2021). Dinggongteng is often combined with Cinnamomi ramulus, Ephedrae herba, Angelicae sinensis radix, and other medicinal materials. Wu et al. (2005) investigated the commercial medicinal materials in Guangxi, the main production area for E. caulis, as well as Shanghai, Jiangsu, Zhejiang, and other places, and found that the wild resources of Erycibe obtusifolia Benth. and Erycibe schmidtii Craib could no longer meet the demand for clinical medication. P. sinensis has already become a mainstream substitute for E. caulis on the market. The widespread application of P. sinensis has promoted the sustainable utilization of the endangered traditional Chinese medicine E. caulis while accumulating evidence for the effectiveness and safety of P. sinensis.
P. racemosa is also a traditional folk medicine of the Dai, Yi, and Tujia nationalities in China, and its whole herb is the medicinal part (Fang et al., 2007). According to the National Compendium of Chinese Herbal Medicine, the whole plant of P. racemosa relieves the surface, eliminates food accumulation, and is primarily used for colds and food accumulation (Editorial Board, 1975). Its stem and root treat rheumatism (Liu and Li, 1997). In the treatment of cold and fever, it is often used in combination with Peucedanum praeruptorum and Periliae fructus, while in the treatment of food accumulation, it is often used in combination with Crataegi fructus and Serissa serissoides (Fang et al., 2007). In Guangxi Province, P. spectabilis is used to treat uterine prolapse, with its whole herb as the medicinal part (Li et al., 1985). P. spectabilis contains scopoletin, ethyl caffeate, and other compounds (Zhu et al., 2001); however, no pharmacodynamic study has been reported. According to the Chinese Materia Medica, the root of Porana mairei Gagnep. relieves cough and asthma (Editorial Board, 2009).
In summary, Porana plants are used as folk medicines. The genus has received increasing attention due to the widespread use of P. sinensis as a substitute for E. caulis.
4 Chemical compositions of Porana plants
Based on literature reports and our previous research, we concluded that the research on the phytochemical constituents of this genus focused on P. discifera, P. racemosa, P. sinensis, P. spectabilis, and P. duclouxii. Fifty-nine compounds have been isolated from Porana species, including 14 steroids, six coumarins, seven flavonoids, six quinic acid derivatives, three amides, and 23 other compounds. These compounds are displayed in Table 2 according to their chemical name, chemical type, and their original plants. The structural formulas of these compounds are shown in Figure 2.
4.1 Steroids
Fourteen steroids have been isolated from Porana species, of which 12 were isolated from P. discifera, including compounds 1–10 (Zhu et al., 2000) and 12–13 (Yu et al., 2003); four were found in P. racemosa, including compounds 11–14 (Liu and Li, 1997; Wang, 2003; Li et al., 2004); two were found in P. sinensis, including compounds 12–13 (Zhang et al., 2006). Compounds 1–10 are phytoecdysteroids, natural polyhydroxylated compounds with a four-ringed skeleton, usually comprising 27 carbon atoms or 28–29 carbon atoms with the characteristic 7-en-6 ketone on the steroid nucleus (Tarkowská and Strnad, 2016). Phytoecdysteroids are a class of natural steroids with insect ecdysis activity. They also exhibit extensive pharmacological effects on higher animals, including hypoglycemia, wound repair, and immune regulation (Taha-Salaime et al., 2019; Yusupova et al., 2019). Compounds 1–7 have no anti-inflammatory, sedative, anti-convulsant, or anti-cerebra-hypoxic activities in animal testing with Kunming mice (Zhu et al., 2000). Most steroids reported in Porana species have been found in P. discifera. In this case, several issues need to be addressed. Are these compounds also present in other plants of this genus, and can they be used as the chemical indicators of the Porana Burm. f.? Answering these questions must address the biological activity of steroids among the pharmacological activities of this genus.
4.2 Coumarins
Three coumarin compounds have been isolated from P. racemosa, including compounds 15–17 (Li et al., 2004). Four coumarin compounds have been found in P. sinensis, including compounds 15–16 (Zhang et al., 2006) and 18–19 (Xue et al., 2019). Three coumarin compounds have been reported in P. discifera, including compounds 15–16 and 20 (Yu et al., 2003). Two coumarin compounds are found in P. spectabilis, including compounds 15–16 (Zhu et al., 2001). The coumarins obtained from Porana plants are simple coumarins, and compounds 15 and 16 have been found in four species; these are thought to be the primary pharmacodynamic substances and chemical indicators of E. caulis (Chen et al., 2014; Chen et al., 2020). Therefore, compounds 15 and 16 are essential for applying P. sinensis as a substitute for E. caulis.
4.3 Flavonoids
Six flavonoids have been isolated from P. racemosa, including compounds 21–23 (Li et al., 2004) and 25–27 (Wang, 2003). Two flavonoids were found in P. discifera, including compounds 24–25 (Yu et al., 2003). Flavonoids are very common in plants. According to reports, no characteristic flavonoid has been found in this genus; this might be due to the lack of reports on the chemical constituents of Porana plants. However, several characteristic isoflavones, pterocarpans, and rotenoids were identified in Erycibes plants (Peng et al., 2021). Based on this, we speculate that flavonoids might be the components differentiating Porana from Erycibes. Considering flavonoids’ excellent biological activity, exploring such compounds should not be ignored.
4.4 Quinic acid derivatives
Six quinic acid derivatives have been reported in the Porana species, including compounds 28–33 (Chen et al., 2013; Chen et al., 2019; Chen et al., 2020), all from P. sinensis. Our fingerprint study has revealed that Porana dinetoides C.K.Schneid., P. racemosa, and P. duclouxii also contained quinic acid derivatives (Figure 3). Because many quinic acid derivatives have been detected in fingerprints, this group of compounds can be used as chemical markers for quality control, and this potential deserves further evaluation.
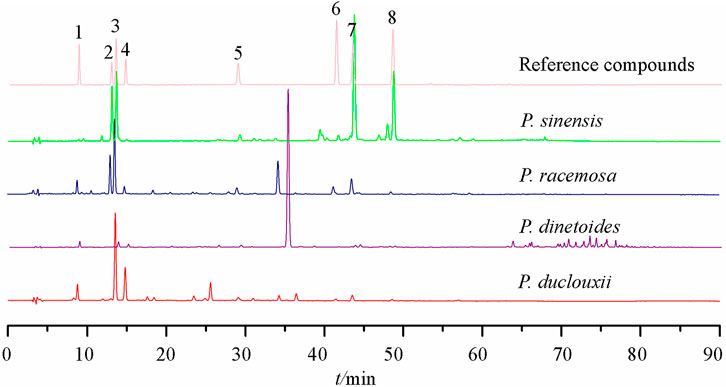
FIGURE 3. HPLC fingerprints of four species of genus Porana 1) 5-O-caffeoylquinic acid; 2) Scopolin; 3) Chlorogenic acid; 4) 4-O-caffeoylquinic acid; 5) Scopoletin; 6) 3,5-dicaffeoylquinic acid; 7) 3,4-dicaffeoylquinic acid; 8) 4,5-dicaffeoylquinic acid.
4.5 Amides
Three amides have been isolated from Porana plants, among which compound 34 has been found in P. racemosa (Li et al., 2004) and compounds 35–36 have been found in P. sinensis (Zhang et al., 2006). The chemical structures of the three amides are similar. It was reported that compound 36 has better activity than compound 35 in inhibiting nitric oxide (NO) release from lipopolysaccharide (LPS)-induced RAW 264.7 cells, suggesting that introducing a methoxy group at the two-position reduces the anti-inflammatory activity of these compounds (Zheng et al., 2018).
4.6 Other compounds
Twenty-three compounds were found in Porana species, including one lignin (compound 38), one monoterpenoid (compound 55), two sesquiterpenes (compound 42, 54), three triterpenoids (compound 46, 47, 57), one benzoquinone (compound 49), seven phenols (compounds 44, 45, 48, 50, 51, 53, 59), one stilbene (compound 58), five glycosides compounds (compound 37, 39–41, 52), one chromone (compound 43), and one cyclohexanol (compound 56). There are many phenolic acids and their derivatives in Porana plants. Resin glycosides are characteristic of constituents in Convolvulaceae, and three such components (compounds 39–41) have been isolated from Porana plants (Ding et al., 2014). Compounds 39–41 all have a common trisaccharide moiety and (11S)-hydroxytetradecanoic acid or (3S,11S)-dihydroxytetradecanoic acid as the aglycone. These 23 compounds have not shown any regularity. There is no evidence to assess the importance of these compounds regarding quality control or biological activity.
In summary, only five species of Porana plants have been reported, with a total of 59 compounds to date. Combined with the literature reports and fingerprints, phenolic acids and coumarins are widely represented in this genus. Phytoecdysteroids and resin glycosides have specific characteristics; however, their distribution is narrow in this genus. This finding suggests that there might be substantial differences in the chemical compositions of these plants, and a phytochemical study of other species needs to be performed urgently.
5 Pharmacological activities of Porana plants
5.1 Network analysis of Porana plants
Because the research on this genus is not systematic, to maximize its medicinal value, we first predicted its targets based on its chemical components using network analysis. Using follow-up comparisons with reported pharmacological research results, the pharmacological effects of this genus were explored.
5.1.1 Enrichment of critical targets
The two-dimensional structures of all 59 compounds found in Porana plants were identified in the PubChem database (https://pubchem.ncbi.nlm.nih.gov/search/), their sdf files were downloaded, and they were imported into the Swiss Target Prediction database (http://www.swisstargetprediction.ch/) to predict their targets (Gfeller et al., 2014). After removing the duplicate targets, the potential targets were obtained. We obtained 713 targets in this manner.
5.1.2 The construction and topological parameter analysis of a protein-protein interaction network
All 713 targets obtained in section 5.1.1 were imported into the STRING platform (https://string-db.org/) to construct a PPI network. The topological parameters of the PPI network were calculated and analyzed using Cytoscape 3.6.0. The critical targets were determined with greater values of the degree, closeness centrality, and betweenness centrality than the mean value. This analysis revealed that the mean degree of potential target nodes was 39.5, the mean value of closeness centrality was 0.4326, and the mean value of betweenness centrality was 0.0019. The output was 135 targets with a higher value than the corresponding mean.
5.1.3 Kyoto encyclopedia of genes and genomes pathway enrichment analysis
To explore the related signaling pathways of the 135 targets obtained in Section 5.1.2, the targets were imported into DAVID (https://david.ncifcrf.gov/home.jsp), with the species limited to humans. KEGG pathway enrichment analysis was performed to identify the relevant signaling pathways. After removing specific diseases such as prostate cancer, viral carcinogenesis, glioma, or other irrelevant items, with p < 0.01 as the screening condition, the top 20 most significant pathways were selected for the subsequent enrichment analysis using R language software (Supplementary Table S1). As shown in Figure 4, the abscissa (enrichment) of the bubble chart represents the ratio of the core targets involved in each pathway to the total number of targets in the pathway; the size of the bubble represents the number of core targets involved in the pathway; the color ranges from red to green, indicating that the p-value is from small to large, and deeper redness indicates the higher significance of the pathway.
5.1.4 The construction and analysis of the compound-target-pathway network
According to the top 20 pathways of gene enrichment in the KEGG pathway enrichment analysis, the potential targets and the corresponding components enriched in these pathways were outputted. The data table of the C-T-P was imported into Cytoscape 3.6.0 to construct the C-T-P network with a total of 148 nodes (20 pathways, 73 targets, 55 components) and 772 edges. Then the Network Analyzer was used to calculate the topology parameters of the C-T-P network, while a Degree Sorted Circle Layout was applied to lay out nodes. The C-T-P network topology parameters were also analyzed using Network Analyzer, and the results are displayed in Supplementary Table S2. The mean degree of the 55 differentially active components was 7.29, the mean value of closeness centrality was 0.3505, and the mean value of betweenness centrality was 0.0067. Three network topology parameters with 17 components were higher than the corresponding mean value (compounds 1–5, 7, 16–17, 23–25, 34–36, 45, 48, and 50). The mean degree of the 73 potential target nodes was 10.58, the mean value of closeness centrality was 0.3732, and the mean value of betweenness centrality was 0.0122. Three network topology parameters of 20 targets were higher than the corresponding mean value (MAPK1, PIK3CA, AKT1, MAP2K1, MAPK3, EGFR, MMP2, PRKCA, ESR2, GSK3B, MAPK14, ESR1, PIK3R1, NRAS, SRC, PTGS2, MMP9, TNF, KDR, and ADORA3). The mean degree of the 20 pathways was 18.55, the mean value of closeness centrality was 0.4054, and the mean betweenness centrality was 0.0254. Three network topology parameters of six signaling pathways were higher than the corresponding mean value (PI3K-Akt, HIF-1, estrogen, MAPK, chemokine, and the thyroid hormone signaling pathway).
The results of the network analysis revealed 17 active compounds in Porana species, including six steroids, three flavonoids, three amides, two coumarins, and three organic acid esters. In the follow-up quality control study, critical research should be carried out on the actual content of these compounds. Coumarins are widely distributed in Porana species, presenting in P. sinensis, P. racemosa, P. discifera, and P. spectabilis. Taking coumarin scopolin as an example, its targets include GSK3B, EGFR, MAPK1, IL2, HSPA8, MMP9, HK1, GAPDH, TNF, ADORA3, acting on PI3K-Akt, HIF-1, estrogen, MAPK, and other signaling pathways. Scopolin promotes the differentiation of osteoblasts and inhibits the decrease of bone mineral density, participating in osteoporosis treatment (Park et al., 2020), possibly associated with the regulation of the estrogen pathway. Intraperitoneal injection of scopolin alleviates the symptoms of adjuvant arthritis in rats by inhibiting inflammatory responses and angiogenesis (Pan et al., 2009); the mechanisms might involve the PI3K-Akt, HIF-1, and MAPK signaling pathways (Park et al., 2015; Qu et al., 2016; Yang et al., 2018).
Porana plants are widely used in traditional Chinese and Indian medicine to relieve inflammation and pain and to treat rheumatoid arthritis. Recent studies demonstrated that the PI3K-Akt pathway inhibits apoptosis in chondrocytes, and modulation of the pathway might be a potential target for the therapy of rheumatic arthritis (Malemud, 2015). HIF-1α increases the production of inflammatory cytokines and promotes angiogenesis in rheumatic arthritis patients (Park et al., 2015). We reported that the 40% ethanol extract of P. sinensis alleviates rheumatoid arthritis by regulating the PI3K-Akt and HIF-1 signaling pathways (Hu et al., 2022).
P. racemosa is another plant in the genus Porana with well-documented medicinal applications, which could be used for the treatment of colds. The results of network analysis revealed its primary active components to be scopolin, umbelliferone, eupatilin, and quercetin, which act on AKT1, EGFR, MAPK1, NFκB1, PIK3R1, SRC, TNF, and other targets to regulate PI3K-Akt, MAPK, and the chemokine signaling pathway, indicating the main involvement of inflammatory pathway.
MAPK participates in cell proliferation, differentiation, transformation, and apoptosis regulation through phosphorylation of nuclear transcription factors, cytoskeletal proteins, and enzymes (Yeung et al., 2018). PI3K-Akt regulates survival, cell growth, differentiation, cellular metabolism, and cytoskeletal reorganization of cells. Modification of this pathway is strongly implicated in the pathogenesis of most cancers (Malemud, 2015). The treatment of cancers is not a traditional application of Porana plants. Due to the regulatory effect of compounds on multiple anti-cancer pathways, the genus Porana has excellent application prospects in anti-cancer drugs.
The targeting pathway of the chemical constituents of Porana species supports the application of this genus in the treatment of rheumatoid arthritis, colds, and cancer. However, the application of Porana plants in treating these diseases needs to be verified in animal and clinical trials.
5.2 Pharmacological activities of the extracts of Porana plants
For the extracts, various preparation methods lead to significant differences in chemical composition and bioactivities. When reviewing the pharmacological effects of Porana extracts, we focused on the following to facilitate identifying the reasons for the differences in pharmacological effects: plant origin and part, extraction methods, quality control methods, biological activities, and screening models (Table 3). Because in vitro studies of extracts have not considered systemic absorption or metabolism of active compounds, the results of these studies might be biased.
5.2.1 Anti-inflammatory and analgesic effects
In a previous study, our group adopted the xylene-induced mouse ear swelling model, the formalin-induced inflammation model, and the carrageenan-induced mice air pouch inflammation model to investigate the anti-inflammatory activity of 40% ethanol extracts of P. sinensis (extract 1). We also applied the mouse acetic acid writhing model and the formalin-induced pain model to investigate its analgesic effects (Chen et al., 2013). We found that the oral administration of extract 1 (570 and 285 mg/kg) inhibits ear swelling in mice by 39.0% and 29.5%, respectively, and the induced inflammation in formalin mice by 37.3% and 30.8%, respectively. In the carrageenan-induced mice air pouch inflammation model, extract 1 significantly inhibits the synthesis of PGE2. Extract 1 significantly reduces the number of writings in mice and relieves phase II pain in the formalin-induced pain model. The 80% methanol ultrasonic extract of P. sinensis (extract 2) inhibits LPS-induced RAW 264.7 release of NO at 25, 50, and 100 μg/ml, with inhibition of iNOS, COX-2, and IL-6 mRNA expression (Xue et al., 2017). However, this study lacked a positive control. COX-2 is a critical enzyme that catalyzes the conversion of arachidonic acid to prostaglandins, and this study confirmed the inhibitory effect of extract 1 on PGE2 synthesis. We reported that 40% ethanol extract of P. sinensis (extract 3) inhibits the release of inflammatory mediators (NO, TNF-α, IL-1β, and IL-6) in LPS-induced RAW 264.7 cells (Hu et al., 2022). Extract 3 attenuates the severity, pathological changes, and release of cytokines (IL-6 and HIF-1α) during rheumatoid arthritis progression by regulating the PI3K/Akt and HIF-1 pathways (Hu et al., 2022).
There are many studies on the anti-inflammatory and analgesic efficacy of the extract of P. sinensis in vitro and in vivo. Compared with methotrexate, aspirin, and other positive control drugs, these extracts’ anti-inflammatory and analgesic effects are insignificant. Except for P. sinensis, species such as P. spectabilis have been recorded for the treatment of chest pain in folk medicine (Li et al., 1985); however, no experimental verification has been reported.
5.2.2 Anti-oxidant activity
As a chronic inflammatory autoimmune disease, rheumatoid arthritis is closely related to oxidative stress (Peng et al., 2021). The 60% ethanol extract (extract 4) of P. paniculata presented good anti-oxidant activity in DPPH assay, superoxide anion scavenging activity assay, nitric oxide scavenging activity assay, hydrogen peroxide scavenging assay and metal chelating activity (Kumar et al., 2015). In the superoxide anion scavenging assay, extract 4 exhibited more robust activity than the positive control butylated hydroxyanisole. In the hydrogen peroxide scavenging assay, extract 4 (IC50: 25.65 μg/ml) performed almost as well as gallic acid (IC50: 24.29 μg/ml). Our group also tested ten batches of 80% methanol extract (extract 5) of P. sinensis, all of which showed good DPPH˙ scavenging activity, with IC50 values ranging from 211 to 439 μg/ml (Chen et al., 2020). However, the above-mentioned test method for anti-oxidant activity is based on chemical reaction in vitro, which is far from practical. Therefore, it is necessary to explore the antioxidant activity in vivo to clarify the molecular mechanisms of its antioxidant activity.
5.2.3 Anti-gout effect
In a previous study, we applied the strategy of network analysis combined with experimental verification to study the mechanism of the 40% ethanol extract of P. sinensis (extract 6) against gout. Extract 6 (0.25, 0.5, 1.0 g/g) dose-dependently reduced joint swelling in rats with monosodium urate (MSU) crystal-induced gout arthritis, with decreased serum MDA and IL-1β levels, and increased serum SOD, TGF-β, and IL-4 levels. By mediating the TLR2-MyD88 signaling pathway, it regulates the release of inflammatory factors and oxygen free radicals to prevent and treat gouty arthritis (Du et al., 2020). Because xanthine oxidase is a target for gout treatment, we tested the xanthine oxidase inhibitory activity of ten batches of the 80% methanol extract of P. sinensis (extract 7), revealing its good activity, with IC50 values ranging from 26.7 to 45.5 mg/ml (Chen et al., 2020). The treatment of gout-related diseases is not traditionally applied to the genus Porana. Although the in vitro and in vivo experiments demonstrated the anti-gout potential of P. sinensis, it remains needs to be verified by clinical research. In addition, due to the different extraction methods of these extracts, the active components of anti-gout should be clarified in the future.
5.2.4 Toxicity
Only acute toxicity of P. sinensis has been reported. No mice died with a single intragastric 40% ethanol extract of P. sinensis at 5 g/kg. The weights, behaviors, and anatomical examinations showed no apparent abnormalities within 14 days (Chen et al., 2013). However, because it is a medicinal plant, acute toxicity evaluation is insufficient, and chronic toxicity tests and clinical safety evaluations of Porana plants need to be performed.
In summary, the current research on the medicinal effects of Porana species concentrates on P. sinensis. Although Porana is widely distributed, its medicinal value is limited. Especially for P. racemosa, which enjoys abundant folk medicinal records and good development prospects, its systematic pharmacodynamic and clinical research is lacking. For the pharmacological study of the extract, to clarify its pharmacodynamic components, chemical analysis is required. Some studies have not provided quality control on the extracts, which would affect the reliability of these studies.
5.3 Pharmacological activities of the active constituents of Porana plants
To further analyze the pharmacological activities of this genus, we followed the pharmacological studies of compounds in this genus and discussed their correlation with the results of our network analysis. The results are summarized in Table 4.
5.3.1 Anti-inflammatory and analgesic effects
The results of long-term folk medicinal and network analysis indicated that anti-inflammatory and analgesic effects are the primary medicinal effects of Porana plants. The intraperitoneal injection of scopoletin (compound 15, 1, 5, 10 mg/kg) reduced serum levels of NO, TNF-α, and PGE2 of carrageenan-induced paw edema mice, and the protein expression of iNOS and COX-2 (Chang et al., 2012). Scopoletin reduced the amount of writhing in the mouse acetate writhing model and formalin-induced pain in the late phase. The anti-inflammatory and analgesic effects of scopoletin (10 mg/kg) are equivalent to that of indomethacin (10 mg/kg) (Chang et al., 2012). However, scopoletin was given by intraperitoneal injection, which would limit its application. In the carrageenan-induced mouse model of pleurisy, intraperitoneal injection of scopoletin (1 mg/kg) reduced serum NO, TNF-α, and IL-1β levels and inhibited p65, p38 phosphorylation in mouse lungs (Pereira dos Santos Nascimento et al., 2016). Dou et al. (2013) reported that scopoletin (15, 30, 60 μmol/L) significantly inhibited the production of IL-6 in fibroblast-like synoviocytes induced by IL-1β and the phosphorylation of p38, ERK, PKC, and CREB. These findings suggest that scopoletin might play a role by mediating the MAPK/PKC/CREB pathways. It should be noted that this study lacks a positive control. P38 MAPK is relevant to human inflammatory disease, and inhibition of p38 phosphorylation reduces gene expression of many inflammatory mediators (Dou et al., 2013). The regulatory effect of scopoletin on the MAPK signaling pathway is consistent with the results of network analysis. These findings suggest that scopoletin exerts anti-inflammatory and analgesic effects through multiple targets and pathways, indicating its good medicinal potential (Parama et al., 2022). However, due to the instability of scopoletin under physiological media and poor water solubility, its oral bioavailability is only 6.0%, severely restricting its medicinal application (Sakthivel et al., 2022). With the rapid development of pharmaceutical technology, new drug delivery systems have introduced possible applications of scopoletin in recent years. For example, there is a formulation of soluplus-based micelles for scopoletin, which increases its absorption, bioavailability, and tissue distribution 33-fold (Zeng et al., 2017). Pan et al. (2009) reported that intraperitoneal injection of scopolin (compound 16, 50, and 100 mg/kg) alleviated the symptoms of adjuvant-induced arthritis in rats by inhibiting the expression of IL-6, VEGF, and FGF-2 in rat synovial tissue. Li et al. (2019) established an LC-MS/MS method for the simultaneous determination of scopolin and scopoletin in rat biomatrices, while the bioavailability of scopolin was exceptionally low.
There are also many reports on umbelliferone’s anti-inflammatory and analgesic activities (compound 17) and isofraxidin (compound 20). Oral administration of umbelliferone (20 and 40 mg/kg) for 28 days led to significant decreases in ear thickness, spleen size and weight, and serum levels of IgE, IgG1, IgG2a, TNF-α, and IL-4. There were also decreases in mast cell infiltration on 2,4-dinitrochlorobenzene and house dust mite extract-treated mice (Lim et al., 2019). Umbelliferone reduced the secretion of pro-inflammatory cytokines and chemokines in TNF-α/IFN-γ-treated HaCaT cells via the regulation of the MAPK, IkB-α/NF-κB, and STAT1 signaling pathways (Lim et al., 2019). There are many reports on isofraxidin in the treatment of osteoarthritis (Jin et al., 2018; Wang and Wang, 2021). For example, isofraxidin (1, 10, and 50 μmol/L) blocked IL-1β-stimulated production of NO and PGE2, inhibited the expression of COX-2, iNOS, MMP-1, MMP-3, MMP-13, ADAMTS-4 and -5, and suppressed IL-1β-induced IκB-α degradation and NF-κB activation in human osteoarthritis chondrocytes (Lin et al., 2018); it should be noted that there was no positive control group in this study. Pharmacokinetic studies demonstrated in vivo its rapid absorption after oral applications (Majnooni et al., 2020).
The HPLC fingerprints of the Porana plants (Figure 3) showed that quinic acid derivatives frequently appear in different Porana species. The anti-inflammatory, analgesic-related pharmacodynamics of chlorogenic acid has been reported in many studies and associated with the NF-κB, MAPK, and JNK/AP-1 signaling pathways; they have also been associated with the downregulation of TNF-α, COX-2, and PGE2 (Bagdas et al., 2020). Xue et al. (2019) applied the method of D101 macroporous resin to track the anti-inflammatory components in P. sinensis; Compounds 31–33 inhibited NO/iNOS and PGE2/COX-2 pathways, and the nuclear translocation of NF-κB was also blocked. Wu et al. (2016) reported that compounds 31–33 reduce mouse ammonia liquor-induced acute airway inflammation by reducing the total leukocytes in bronchoalveolar lavage fluid. Among these three compounds, 4,5-dicaffeoylquinic acid exhibited the most potent effect, suggesting that the structure-activity relationship requires further elaboration.
Seven flavonoids have been isolated from Porana species. Eupatilin (compound 23) and quercetin (compound 25) present diverse anti-inflammatory activities. Eupatilin exerts anti-inflammatory effects by regulating NF-κB (Choi et al., 2011), TLR4/MyD88 (Fei et al., 2019a), AMPK (Zhou et al., 2018), and by suppressing osteoclast differentiation (Kim et al., 2015), inhibiting oxidative stress (Ali et al., 2017). Although eupatilin has broad bioactivity, its oral bioavailability is only 2.7% (Wang et al., 2018a). Quercetin is a broad-spectrum anti-inflammatory and analgesic substance without specificity. Considering the folk medicinal application of Porana plants, we only focused on its application in arthritis. Quercetin decreased serum TNF-a, IL-1β, IL-17, and MCP-1 levels in a collagen-induced mouse arthritis model (Haleagrahara et al., 2017). The authors claimed that quercetin produces better activity than methotrexate, which might not be accurate due to the different doses and routes of administration (quercetin, Po with 30 mg/kg; methotrexate, Ip with 0.5 mg/kg). MCP-1 (chemokine ligand 2) has a critical role in inflammation (Singh et al., 2021). These studies confirmed the regulatory effect of Porana plants on the chemokine pathway in network analysis. Yuan et al. (2020) found that quercetin reduces neutrophil infiltration and promotes apoptosis in activated neutrophils; however, this study did not provide the dosage of quercetin and the positive control dexamethasone.
β-Ecdysterone (compound 1) inhibited 3-methyladenine-induced apoptosis of chondrocytes, downregulated PI3K, p-AKT1, p-mTOR, p-p70S6K, and caspase-3 expression, and activated autophagy in chondrocytes in a rat model of monoiodoacetate-induced osteoarthritis (Tang et al., 2020). N-trans-feruloyltyramine (compound 35) strongly suppressed mRNA expression of COX-2 and iNOS via suppression of AP-1 and the JNK signaling pathway in LPS-induced RAW 264.7 cells (Jiang et al., 2015).
There are several anti-inflammatory and analgesic active ingredients in Porana species, including coumarins, quinic acid derivatives, flavonoids, steroids, and amides. The results of the components (scopolin, umbelliferone, eupatilin, quercetin, N-trans-feruloyltyramine) pathways (PI3K-Akt, HIF-1, MAPK, chemokine) in our network analysis are consistent with the results of the literature review, which suggests the potential of Porana species in the treatment of arthritis. However, it should be noted that, although components such as scopoletin and eupatilin show good anti-inflammatory and analgesic effects, their bioavailability is relatively low. Further structural modification is needed, or new drug delivery systems should be developed to improve their bioavailability.
5.3.2 Anti-gout effect
Intraperitoneal injection of scopoletin (compound 15, 100, and 200 mg/kg) significantly lowered the number of neutrophils and mononuclear phagocytes of MSU-induced inflammation in a mouse air pouch model. The secretion of IL-1β, TNF-α, IL-6, PGE2, and NO were suppressed by scopoletin (30–300 μmol/L) at the transcriptional level in MSU-stimulated RAW 264.7 cells, mediated by the suppression of NF-κB activation and blockade of the MAPK signal pathway (Yao et al., 2012). In our previous study, we also found that scopoletin (4.9 mg/kg) inhibited the production of serum MDA, IL-1β, and TGF-1β, promoted the release of SOD and IL-4 and inhibited the expression of TLR2 and MyD88 mRNA in rat joint synovium (Du et al., 2020). In another study, we found that 3,4-dicaffeoylquinic acid (compound 31, IC50: 0.32 mmol/L), 4,5-dicaffeoylquinic acid (compound 32, IC50: 0.26 mmol/L), and 3,5-dicaffeoylquinic acid (compound 33, IC50: 0.21 mmol/L) exhibited weak xanthine oxidase inhibitory activity (Positive control: allopurinol, IC50: 0.01 mmol/L) (Chen et al., 2014), partially explaining the phytochemistry of anti-gout activity.
In summary, scopoletin plays an anti-gout role primarily by regulating inflammatory pathways, and quinic acid derivatives have xanthine oxidase inhibitory activity. Due to a large amount of anti-inflammatory, analgesic, antioxidant, and xanthine oxidase-inhibiting ingredients, the genus Porana has excellent application prospects as anti-gout therapies. However, only P. sinensis has been reported to treat acute gouty arthritis. Therefore, the anti-gout efficacy of other species in this genus must be further explored.
5.3.3 Anti-cancer activity
Scopoletin (compound 15) inhibited the growth of cervical cancer cell lines, including DoTc2, SiHa, HeLa, and C33A cells, with the IC50 values ranging from 7.5 to 25 μmol/L. The apoptotic cell death in HeLa cells induced by scopoletin involved the upregulation of Bax, caspase 3, 8, and 9, the downregulation of Bcl-2, and the blockade of the PI3K/Akt pathway. Scopoletin also caused cell cycle arrest at the G2/M phase and inhibited cell migration (Tian et al., 2019). Umbelliferone (compound 17) exerted anti-cancer effects on various cells and animal models through induction of apoptosis, cell cycle arrest, reduction of cell proliferation, and inhibition of the release of inflammatory factors. For example, treating human renal carcinoma cells with umbelliferone-induced dose-dependent decreases in Ki67, MCM2, Bcl-2, CDK2, CyclinE1, CDK4, and CyclinD1 and an increase in Bax (Wang et al., 2019). Isofraxidin (compound 20, 5–80 μmol/L) significantly bate cell proliferation, induced cell apoptosis, and decreased the expression of anti-apoptotic protein Bcl-2 in human colorectal cancer cell lines (HT-29 and SW-480). Isofraxidin blocks the Akt pathway via inhibition expression of p-Akt (Shen et al., 2017).
There are many reports on the anti-cancer properties of quinic acid derivatives in Porana species. 5-O-caffeoylquinic acid (compound 30) abrogated mitogen-stimulated invasion but not proliferation in p53 wild-type A549 and p53-deficient H1299 NSCLC cells. The anti-invasive activity of 5-O-caffeoylquinic acid in A549 cells might be mediated by the inactivation of the p70S6K-dependent signaling pathway (In et al., 2016). Chlorogenic acid (compound 28) inhibited the proliferation of U2OS, Saos-2, and MG-63 osteosarcoma cells (50, 100, 200 μmol/L) (Sapio et al., 2020). This compound also participates in the apoptosis of 4T1 breast cancer tumors in BALB/c mice, involving the increase of the Bax/Bcl-2 ratio and the genes for p53 and caspase-3 (Changizi et al., 2021); it inhibits the viability of HCT116 and HT29 colon cancer cell lines associated with the induction of cell cycle arrest at the S phase and the suppression of extracellular signal-related kinase activation (Hou et al., 2017). These findings suggest that caffeoylquinic acids exhibit relatively broad anti-cancer activity, with targeted cancer types including lung cancer, osteosarcoma, breast cancer, and colon cancer. Chlorogenic acid inhibits cell proliferation and blocks the cell cycle; however, 5-O-caffeoylquinic acid does not inhibit cell proliferation. As isomers, the difference in antiproliferative effect between these two compounds deserves further explanation.
The flavonoid eupatilin (compound 23) inhibits the viability and proliferation of glioma cells by arresting the cell cycle at the G1/S phase. Eupatilin disrupts the structure of the cytoskeleton and affects F-actin depolymerization via the p-LIMK/cofilin pathway (Fei et al., 2019b). However, this study did not report a proapoptotic effect of eupatilin on glioma, which was inconsistent with other studies. Eupatilin (12.5, 25, 50 μmol/L) inhibits the proliferation, metastasis, and spread of prostate cancer cells through modulation of PTEN and NF-κB signaling (Serttas et al., 2021); it blocks the proliferation of esophageal cancer TE1 cells associated with the inhibition of the Akt and ERK pathways (Wang et al., 2018b). Another flavonoid, 4ʹ-hydroxywogonin (compound 24), reduced the viability and suppressed the proliferation of SW620 colorectal cancer cells angiogenesis by disrupting PI3K/Akt signaling, while the expression of VEGF-A decreased dose-dependently (Sun et al., 2018). Based on this study, it could be presumed that the anti-angiogenic activity of PI3K inhibitors was at least partially mediated by their capacity to reduce VEGF levels.
N-trans-feruloyltyramine (compound 35) inhibits the proliferation of HepG2 cells with an IC50 of 194 ± 0.894 μmol/L, which was significantly lower than the positive control taxol (IC50: 26 ± 0.128 μmol/L) (Gao et al., 2019). Comparing the results on HepG2 and LO2 cells revealed that N-trans-feruloyltyramine might have selective cytotoxic effects.
In summary, there are many anti-cancer active components in Porana plants, including coumarins, quinic acid derivatives, and flavonoids. Of these, scopoletin, umbelliferone, chlorogenic acid, and eupatilin have many reports on their anti-cancer activity. These components are widely distributed in nature and are not specific. Since the related research mostly stays at the level of in vitro research, and more in vivo research and clinical studies are needed.
5.3.4 Anti-diabetic activity
In the streptozotocin-induced diabetic mice model, scopoletin (compound 15, 0.01 g/100 g diet) reduced blood glucose and glycated hemoglobin, glucose intolerance, hepatic lipid accumulation and downregulated hepatic gene expression of triglyceride and cholesterol synthesis and inflammation (TLR4, MyD88, NF-κb1, TNF-α, and IL-6). These results suggest that scopoletin protects against diabetes-induced steatosis and inflammation by inhibiting lipid biosynthesis and the TLR4-MyD88 pathway (Choi et al., 2017). However, this was a single-dose study with substantial differences in the dosage of the positive control metformin (0.5 g/100 g diet) and scopoletin, which cannot be used for comparison. In another study, scopoletin (1 mg/kg) reduced blood glucose, insulin, and lipid levels in high-fructose diet-induced type 2 diabetes, involving the activation of IRS1, PI3K, and Akt phosphorylation (Kalpana et al., 2019). Scopoletin inhibited the activity of α-glucosidase and α-amylase and reduced postprandial blood glucose levels in streptozotocin-induced diabetes mice. Unfortunately, the IC50 value of scopoletin was 85.12 and 37.36 μmol/L for α-glucosidase and α-amylase, which were lower than acarbose (Jang et al., 2018), indicating that its potential is limited. Another study reported that scopoletin stimulated insulin secretion via a K+ATP channel-dependent pathway in INS-1 pancreatic β cells (Park et al., 2022). Scopoletin could play a role in treating diabetes by stimulating insulin secretion, inhibiting α-glucosidase and α-amylase, and downregulating triglyceride and cholesterol synthesis and inflammation. However, the inhibitory effect of scopoletin on α-glucosidase and α-amylase would be weaker than that of the positive control drug acarbose.
There are many reports on the efficacy and mechanism of chlorogenic acid (compound 28), quercetin (compound 25), and rutin (compound 27) in the treatment of diabetes. For example, quercetin stimulated insulin secretion (Kittl et al., 2016), alleviated ferroptosis in pancreatic cells (Li et al., 2020), and ameliorated diabetic encephalopathy through the SIRT1/ER stress pathway (Hu et al., 2020). Rutin decreased carbohydrate absorption from the small intestine, inhibited tissue gluconeogenesis, increased tissue glucose uptake, stimulated insulin secretion from beta cells, and protected pancreatic islets against degeneration (Ghorbani, 2017). Chlorogenic acid prevented diabetic nephropathy (Bao et al., 2018), rescued sensorineural auditory function, attenuated insulin resistance, and modulated glucose uptake (Hong et al., 2017).
In summary, many anti-diabetic ingredients are found in Porana plants, including coumarins, quinic acid derivatives, and flavonoids. The content of coumarins and quinic acid derivatives is relatively high in the genus Porana, suggesting that this genus could be used to treat diabetes. The network analysis shows that the pathways regulated by the chemical components of Porana plants play an essential role in diabetes treatment. For example, the PI3K/Akt pathway damaged in various body tissues leads to obesity and type 2 diabetes as the result of insulin resistance; in turn, insulin resistance exacerbates the PI3K/Akt pathway, forming a vicious circle (Huang et al., 2018). The progression of diabetes and its complications can be prevented or treated by modulating HIF-1 expression or activity (Catrina and Zheng, 2021). However, apart from the pharmacological or clinical studies of these compounds, there are no reports on the application of Porana plants in diabetes treatment; relevant research needs to be performed.
5.3.5 Other activities
Alkorashy et al. (2020) used transcriptomic methods to study the effect of scopoletin (compound 15) on the phagocytosis of stimulated U937-derived macrophages. Scopoletin enhanced the phagocytic activity, involving the downregulation of seven genes (CDC42, FCGR1A/FCGR1C, ITGA9, ITGB3, PLCE1, RHOD, and RND3) and upregulation of five genes (DIRAS3, ITGA1, PIK3CA, PIK3R3, and PLCD1). These results provide a basis for applying scopoletin in treating cancer progression and metastasis, autoimmune disorders, pelvic organ prolapse, and cystic fibrosis. ITGB3 is upregulated in pelvic organ prolapse disorders in women, and the downregulation of these genes supports the folk medicinal application of P. spectabilis in the treatment of uterine prolapse. Scopoletin also acts as an anti-fungal phytocompound against a multidrug-resistant strain of Candida tropicalis, with properties affecting planktonic and biofilm forms of this pathogen (Lemos et al., 2020).
6 Conclusion
The genus Porana is abundant in natural resources and is widely distributed in Asia, Africa, Oceania, America, and other regions. In China and India, this genus has several medicinal records. Currently, only the chemical composition, efficacy, and quality control of P. sinensis have been systematically reported, while the medicinal value of other species in this genus has not yet been explored. Therefore, we systematically reviewed this genus’s traditional and current use, chemical compositions, and pharmacological activities. We applied network analysis to predict the key targets and pathways of chemical components in this genus to clarify the research status of Porana species and highlight the directions for the rational medicinal development of this genus.
Regarding chemical components, only five species of genus Porana have been reported, with 59 compounds isolated and identified, including steroids, coumarins, flavonoids, quinic acid derivatives, and amides. Combined with the fingerprints (Figure 3), coumarins and quinic acid derivatives are widely distributed in this genus, while steroids have only been reported in P. discifera. Because the research on chemical constituents is the forerunner of medicinal value development, the phytochemical study of other species in this genus needs to be performed.
In terms of pharmacological effects, the extracts of Porana plants exhibit anti-inflammatory, analgesic, antioxidant, and anti-gout activities. However, studies on the pharmacological effects of Porana plants are focused on P. sinensis, and there are few pharmacological studies on other species. Especially for plants with extensive folk medicinal records (such as P. racemosa), detailed pharmacodynamic research needs to be performed. The chemical constituents of Porana present anti-inflammatory, analgesic, anti-gout, anti-cancer, and diabetes treatment activities. Gout and diabetes treatment are not the traditional medicinal applications of Porana plants. However, this genus contains chemical substances with appropriate biological activities. Therefore, we speculate that this genus has the potential to develop in the direction of anti-gout and anti-diabetes. Future research needs to investigate different species’ anti-gout and anti-diabetic efficacy, explain their mechanism of action, and systematically elucidate their active components.
Network analysis showed that steroids, flavonoids, amides, coumarins, and other components maybe be relevant for anti-inflammatory, analgesic, anti-gout, anti-cancer, and diabetes treatment activities of Porana plants. Their targets include GSK3B, EGFR, MAPK1, IL2, HSPA8, MMP9, HK1, GAPDH, TNF, ADORA3, and their pathways include PI3K-Akt, HIF-1, estrogen, and MAPK. The enriched targets and pathways are consistent with the results of our literature review.
In summary, Porana plants are abundant in natural resources and are widely recorded in folk medicine; nevertheless, the study of their medicinal value is limited. Research on the systematic chemical constituents of this genus is urgently needed. Anti-inflammatory, analgesic, anti-gout, anti-cancer, and diabetes treatments are critical directions for future study.
Author contributions
YP and YL: original and final drafting, editing, revision, and figure editing; YY, YG, HR, JH, and WL: figures, tables and review of the literature; XC: network analysis; HT and ZC: revised the draft and final editing.
Funding
This work was financially supported by the National Natural Science Foundation of China [grant numbers 81973419]; Key Research and Development Program of Shaanxi [grant number 2019ZDLSF04-07, 2022SF-315]; Shaanxi Administration of Traditional Chinese Medicine Projects [grant number 2022-SLRH-YQ-003, 2021-PY-003].
Acknowledgments
Thanks for all institutions that provided the funding.
Conflict of interest
The authors declare that the research was conducted in the absence of any commercial or financial relationships that could be construed as a potential conflict of interest.
Publisher’s note
All claims expressed in this article are solely those of the authors and do not necessarily represent those of their affiliated organizations, or those of the publisher, the editors and the reviewers. Any product that may be evaluated in this article, or claim that may be made by its manufacturer, is not guaranteed or endorsed by the publisher.
Supplementary material
The Supplementary Material for this article can be found online at: https://www.frontiersin.org/articles/10.3389/fphar.2022.998965/full#supplementary-material
Abbreviations
Akt, protein kinase B; COX-2, cyclooxygenase-2; C-T-P, compound-target-pathway; FGF-2, fibroblast growth factor 2; HIF, hypoxia inducible factor; HPLC, high performance liquid chromatography; Ig, intragastric administration; IL-6, interleukin 6; iNOS, inducible nitric oxide synthase; Ip, intraperitoneal injection; KEGG, Kyoto Encyclopedia of Genes and Genomes; LPS, lipopolysaccharide; MAPK, mitogen-activated protein kinases; MDA, malonic dialdehyde; MSU, monosodium urate; MyD88, myeloid differentiation factor 88; NF-κB, nuclear transcription factor-κB; NO, nitric oxide; PGE2, prostaglandin E2; PI3K, phosphoinositide 3-kinase; PPI, protein-protein interaction; SOD, superoxide dismutase; STAT, signal transducer and activator of transcription; TLR2, toll-like receptor 2; TNF-α, tumor necrosis factor-α; VEGF, vascular endothelial growth factor.
References
Ali, M. Y., Seong, S. H., Reddy, M. R., Seo, S. Y., Choi, J. S., and Jung, H. A. (2017). Kinetics and molecular docking studies of 6-formyl umbelliferone isolated from Angelica decursiva as an inhibitor of cholinesterase and BACE1. Molecules 22 (10), 1604. doi:10.3390/molecules22101604
Alkorashy, A. I., Doghish, A. S., Abulsoud, A. I., Ewees, M. G., Abdelghany, T. M., Elshafey, M. M., et al. (2020). Effect of scopoletin on phagocytic activity of U937-derived human macrophages: Insights from transcriptomic analysis. Genomics 112 (5), 3518–3524. doi:10.1016/j.ygeno.2020.03.022
Bagdas, D., Gul, Z., Meade, J. A., Cam, B., Cinkilic, N., and Gurun, M. S. (2020). Pharmacologic overview of chlorogenic acid and its metabolites in chronic pain and inflammation. Curr. Neuropharmacol. 18 (3), 216–228. doi:10.2174/1570159X17666191021111809
Bao, L., Li, J., Zha, D., Zhang, L., Gao, P., Yao, T., et al. (2018). Chlorogenic acid prevents diabetic nephropathy by inhibiting oxidative stress and inflammation through modulation of the Nrf2/HO-1 and NF-ĸB pathways. Int. Immunopharmacol. 54, 245–253. doi:10.1016/j.intimp.2017.11.021
Catrina, S. B., and Zheng, X. (2021). Hypoxia and hypoxia-inducible factors in diabetes and its complications. Diabetologia 64 (4), 709–716. doi:10.1007/s00125-021-05380-z
Chang, T.-N., Deng, J.-S., Chang, Y.-C., Lee, C.-Y., Jung-Chun, L., Lee, M.-M., et al. (2012). Ameliorative effects of scopoletin from Crossostephium chinensis against inflammation pain and its mechanisms in mice. Evidence-Based Complementary Altern. Med. 2012, 1–10. doi:10.1155/2012/595603
Changizi, Z., Moslehi, A., Rohani, A. H., and Eidi, A. (2021). Chlorogenic acid induces 4T1 breast cancer tumor's apoptosis via p53, Bax, Bcl‐2, and caspase‐3 signaling pathways in BALB/c mice. J. Biochem. Mol. Toxicol. 35 (2), e22642. doi:10.1002/jbt.22642
Chen, Z., Liao, L., Yang, Y., Zhang, Z., and Wang, Z. (2015). Different fingerprinting strategies to differentiate Porana sinensis and plants of Erycibe by high‐performance liquid chromatography with diode array detection, ultra high performance liquid chromatography with tandem quadrupole mass spectrometry, and chemometrics. J. Sep. Sci. 38 (2), 231–238. doi:10.1002/jssc.201400861
Chen, Z., Liao, L., Zhang, Z., Wu, L., and Wang, Z. (2013). Comparison of active constituents, acute toxicity, anti-nociceptive and anti-inflammatory activities of Porana sinensis Hemsl., Erycibe obtusifolia Benth. and Erycibe schmidtii Craib. J. Ethnopharmacol. 150 (2), 501–506. doi:10.1016/j.jep.2013.08.059
Chen, Z., Tao, H., Liao, L., Zhang, Z., and Wang, Z. (2014). Quick identification of xanthine oxidase inhibitor and antioxidant from Erycibe obtusifolia by a drug discovery platform composed of multiple mass spectrometric platforms and thin‐layer chromatography bioautography. J. Sep. Sci. 37 (16), 2253–2259. doi:10.1002/jssc.201400342
Chen, Z., Wang, M., Yang, Y., Cui, X., Hu, J., Li, Y., et al. (2020). Promotion of a quality standard for Porana sinensis Hemsl. based on the efficacy‐oriented Effect‐Constituent Index. Biomed. Chromatogr. 34 (2), e4726. doi:10.1002/bmc.4726
Chen, Z., Wang, M., Yang, Y., Du, X., Zhang, Z., and Li, Y. (2019). Qualitative and quantitative analysis of Porana sinensis Hemsl by UHPLC‐Q‐Exactive MS, TLC autographic method and DART‐MS. Phytochem. Anal. 30 (3), 311–319. doi:10.1002/pca.2814
Choi, E.-J., Lee, S., Chae, J.-R., Lee, H.-S., Jun, C.-D., and Kim, S.-H. (2011). Eupatilin inhibits lipopolysaccharide-induced expression of inflammatory mediators in macrophages. Life Sci. 88 (25-26), 1121–1126. doi:10.1016/j.lfs.2011.04.011
Choi, R. Y., Ham, J. R., Lee, H. I., Cho, H. W., Choi, M. S., Park, S. K., et al. (2017). Scopoletin supplementation ameliorates steatosis and inflammation in diabetic mice. Phytother. Res. 31 (11), 1795–1804. doi:10.1002/ptr.5925
Ding, W.-B., Zhang, D.-G., Liu, C.-J., Li, G.-H., and Li, Y.-Z. (2014). Resin glycosides from Porana duclouxii. J. Asian Nat. Prod. Res. 16 (2), 135–140. doi:10.1080/10286020.2013.864281
Dou, Y., Tong, B., Wei, Z., Li, Y., Xia, Y., and Dai, Y. (2013). Scopoletin suppresses IL-6 production from fibroblast-like synoviocytes of adjuvant arthritis rats induced by IL-1β stimulation. Int. Immunopharmacol. 17 (4), 1037–1043. doi:10.1016/j.intimp.2013.10.011
Du, X., Zhao, L., Yang, Y., Zhang, Z., Hu, J., Ren, H., et al. (2020). Investigation of the mechanism of action of Porana sinensis Hemsl. against gout arthritis using network pharmacology and experimental validation. J. Ethnopharmacol. 252, 112606. doi:10.1016/j.jep.2020.112606
Editorial Board, C.M.M (2009). Chinese Materia Medica. Shanghai: Shanghai Scientific & Technical Publishers.
Editorial Board, N.C.C.H.M (1975). National Compendium of Chinese herbal medicine. Beijing: People's Medical Publishing House.
Fan, L., Wu, L., Yu, X.-H., Chen, Y.-B., Lin, L., and Li, S.-G. (2021). The ethnopharmacology, phytochemistry, pharmacology and toxicology of the genus Erycibe (Convolvulaceae). J. Ethnopharmacol. 278, 114312. doi:10.1016/j.jep.2021.114312
Fang, Z., Zhao, H., and Zhao, J. (2007). Tujia medicinal records. Beijing: China Medical Science and Technology Press.
Fei, X., Chen, C., Kai, S., Fu, X., Man, W., Ding, B., et al. (2019a). Eupatilin attenuates the inflammatory response induced by intracerebral hemorrhage through the TLR4/MyD88 pathway. Int. Immunopharmacol. 76, 105837. doi:10.1016/j.intimp.2019.105837
Fei, X., Wang, J., Chen, C., Ding, B., Fu, X., Chen, W., et al. (2019b). Eupatilin inhibits glioma proliferation, migration, and invasion by arresting cell cycle at G1/S phase and disrupting the cytoskeletal structure. Cancer Manag. Res. 11, 4781–4796. doi:10.2147/CMAR.S207257
Gao, X., Wang, C., Chen, Z., Chen, Y., Santhanam, R. K., Xue, Z., et al. (2019). Effects of N-trans-feruloyltyramine isolated from laba garlic on antioxidant, cytotoxic activities and H2O2-induced oxidative damage in HepG2 and L02 cells. Food Chem. Toxicol. 130, 130–141. doi:10.1016/j.fct.2019.05.021
Gfeller, D., Grosdidier, A., Wirth, M., Daina, A., Michielin, O., and Zoete, V. (2014). SwissTargetPrediction: A web server for target prediction of bioactive small molecules. Nucleic Acids Res. 42 (W1), W32–W38. doi:10.1093/nar/gku293
Ghorbani, A. (2017). Mechanisms of antidiabetic effects of flavonoid rutin. Biomed. Pharmacother. 96, 305–312. doi:10.1016/j.biopha.2017.10.001
Guoqiang, W. (2014). “Dinggongteng,” in The compilation of national Chinese herbal medicine. 3rd edition (Beijing: People's Medical Publishing House).
Haleagrahara, N., Miranda-Hernandez, S., Alim, M. A., Hayes, L., Bird, G., and Ketheesan, N. (2017). Therapeutic effect of quercetin in collagen-induced arthritis. Biomed. Pharmacother. 90, 38–46. doi:10.1016/j.biopha.2017.03.026
Hong, B. N., Nam, Y. H., Woo, S. H., and Kang, T. H. (2017). Chlorogenic acid rescues sensorineural auditory function in a diabetic animal model. Neurosci. Lett. 640, 64–69. doi:10.1016/j.neulet.2017.01.030
Hou, N., Liu, N., Han, J., Yan, Y., and Li, J. (2017). Chlorogenic acid induces reactive oxygen species generation and inhibits the viability of human colon cancer cells. Anticancer. Drugs 28 (1), 59–65. doi:10.1097/CAD.0000000000000430
Hu, J., Zhao, L., Li, N., Yang, Y., Qu, T., Ren, H., et al. (2022). Investigation of the active ingredients and pharmacological mechanisms of Porana sinensis Hemsl. Against rheumatoid arthritis using network pharmacology and experimental validation. PLoS One 17 (3), e0264786. doi:10.1371/journal.pone.0264786
Hu, T., Shi, J.-J., Fang, J., Wang, Q., Chen, Y.-B., and Zhang, S.-J. (2020). Quercetin ameliorates diabetic encephalopathy through SIRT1/ER stress pathway in db/db mice. Aging 12 (8), 7015–7029. doi:10.18632/aging.103059
Huang, S., Wang, L.-L., Xue, N.-N., Li, C., Guo, H.-H., Ren, T.-K., et al. (2019). Chlorogenic acid effectively treats cancers through induction of cancer cell differentiation. Theranostics 9 (23), 6745–6763. doi:10.7150/thno.34674
Huang, X., Liu, G., Guo, J., and Su, Z. (2018). The PI3K/AKT pathway in obesity and type 2 diabetes. Int. J. Biol. Sci. 14 (11), 1483–1496. doi:10.7150/ijbs.27173
In, J.-K., Kim, J.-K., Oh, J. S., and Seo, D.-W. (2016). 5-Caffeoylquinic acid inhibits invasion of non-small cell lung cancer cells through the inactivation of p70S6K and Akt activity: Involvement of p53 in differential regulation of signaling pathways. Int. J. Oncol. 48 (5), 1907–1912. doi:10.3892/ijo.2016.3436
Jang, J. H., Park, J. E., and Han, J. S. (2018). Scopoletin inhibits α-glucosidase in vitro and alleviates postprandial hyperglycemia in mice with diabetes. Eur. J. Pharmacol. 834, 152–156. doi:10.1016/j.ejphar.2018.07.032
Jiang, Y., Yu, L., and Wang, M.-H. (2015). N-trans-feruloyltyramine inhibits LPS-induced NO and PGE2 production in RAW 264.7 macrophages: Involvement of AP-1 and MAP kinase signalling pathways. Chem. Biol. Interact. 235, 56–62. doi:10.1016/j.cbi.2015.03.029
Jin, J., Yu, X., Hu, Z., Tang, S., Zhong, X., Xu, J., et al. (2018). Isofraxidin targets the TLR4/MD-2 axis to prevent osteoarthritis development. Food Funct. 9 (11), 5641–5652. doi:10.1039/c8fo01445k
Kalpana, K., Sathiya Priya, C., Dipti, N., Vidhya, R., and Anuradha, C. V. (2019). Supplementation of scopoletin improves insulin sensitivity by attenuating the derangements of insulin signaling through AMPK. Mol. Cell. Biochem. 453 (1), 65–78. doi:10.1007/s11010-018-3432-7
Kim, J., Kim, Y., Yi, H., Jung, H., Rim, Y. A., Park, N., et al. (2015). Eupatilin ameliorates collagen induced arthritis. J. Korean Med. Sci. 30 (3), 233–239. doi:10.3346/jkms.2015.30.3.233
Kittl, M., Beyreis, M., Tumurkhuu, M., Fürst, J., Helm, K., Pitschmann, A., et al. (2016). Quercetin stimulates insulin secretion and reduces the viability of rat INS-1 beta-cells. Cell. Physiol. biochem. 39 (1), 278–293. doi:10.1159/000445623
Kumar, A. S., Reddy, J. R., and Gupta, V. R. M. (2015). In vitro antioxidant activity of Porana paniculata and Ipomoea quamoclit-two ethnomedicinally important plants of Convolvulaceae family. Br. J. Pharm. Res. 5 (4), 286–293. doi:10.9734/bjpr/2015/12226
Lemos, A. S., Florêncio, J. R., Pinto, N. C., Campos, L. M., Silva, T. P., Grazul, R. M., et al. (2020). Antifungal activity of the natural coumarin scopoletin against planktonic cells and biofilms from a multidrug-resistant Candida tropicalis strain. Front. Microbiol. 11, 1525. doi:10.3389/fmicb.2020.01525
Li, B.-G., Chen, B., Wang, D.-Y., Ye, Q., and Zhang, G.-L. (2004). A novel C30 sterol from Porana racemosa. Acta Bot. Sin. 46 (3), 375–378. doi:10.3321/j.issn:1672-9072.2004.03.018
Li, B., Chen, Y., and Li, S. (2013). Progress in chemical constituents and pharmaceutical activities of Porana genus plants. Sci. Technol. Rev. 31 (11), 74–79. doi:10.3981/j.issn.1000-7857.2013.11.011
Li, B., Lu, M., Chu, Z., Lei, S., Sun, P., Xiong, S., et al. (2019). Evaluation of pharmacokinetics, bioavailability and urinary excretion of scopolin and its metabolite scopoletin in Sprague Dawley rats by liquid chromatography–tandem mass spectrometry. Biomed. Chromatogr. 33 (12), e4678. doi:10.1002/bmc.4678
Li, B., Tan, D., and Fang, D. (1985). A preliminary study on the folk medicinal plants of the Zhuang Nationality in Guangxi. Guangxi Med. J. 4, 171–172.
Li, D., Jiang, C., Mei, G., Zhao, Y., Chen, L., Liu, J., et al. (2020). Quercetin alleviates ferroptosis of pancreatic β cells in type 2 diabetes. Nutrients 12 (10), 2954. doi:10.3390/nu12102954
Lim, J.-y., Lee, J.-H., Lee, D.-H., Lee, J.-H., and Kim, D.-K. (2019). Umbelliferone reduces the expression of inflammatory chemokines in hacat cells and dncb/dfe-induced atopic dermatitis symptoms in mice. Int. Immunopharmacol. 75, 105830. doi:10.1016/j.intimp.2019.105830
Lin, J., Li, X., Qi, W., Yan, Y., Chen, K., Xue, X., et al. (2018). Isofraxidin inhibits interleukin-1β induced inflammatory response in human osteoarthritis chondrocytes. Int. Immunopharmacol. 64, 238–245. doi:10.1016/j.intimp.2018.09.003
Liu, K., and Li, S. (1997). Study on the chemical constituents of Huangwulong. Hunan J. Traditional Chin. Med. 13 (6), 46.
Majnooni, M. B., Fakhri, S., Shokoohinia, Y., Mojarrab, M., Kazemi-Afrakoti, S., and Farzaei, M. H. (2020). Isofraxidin: Synthesis, biosynthesis, isolation, pharmacokinetic and pharmacological properties. Molecules 25 (9), 2040. doi:10.3390/molecules25092040
Malemud, C. J. (2015). The PI3K/Akt/PTEN/mTOR pathway: A fruitful target for inducing cell death in rheumatoid arthritis? Future Med. Chem. 7 (9), 1137–1147. doi:10.4155/fmc.15.55
Pan, R., Dai, Y., Gao, X., and Xia, Y. (2009). Scopolin isolated from Erycibe obtusifolia Benth stems suppresses adjuvant-induced rat arthritis by inhibiting inflammation and angiogenesis. Int. Immunopharmacol. 9 (7-8), 859–869. doi:10.1016/j.intimp.2009.02.019
Parama, D., Girisa, S., Khatoon, E., Kumar, A., Alqahtani, M. S., Abbas, M., et al. (2022). An overview of the pharmacological activities of scopoletin against different chronic diseases. Pharmacol. Res. 179, 106202. doi:10.1016/j.phrs.2022.106202
Park, E., Kim, J., Jin, H.-S., Choi, C. W., Choi, T. H., Choi, S., et al. (2020). Scopolin attenuates osteoporotic bone loss in ovariectomized mice. Nutrients 12 (11), 3565. doi:10.3390/nu12113565
Park, J. E., Kim, S. Y., and Han, J. S. (2022). Scopoletin stimulates the secretion of insulin via a KATP channel-dependent pathway in INS-1 pancreatic beta cells. J. Pharm. Pharmacol. 74, 1274–1281. doi:10.1093/jpp/rgab143
Park, S. Y., Lee, S. W., Kim, H. Y., Lee, W. S., Hong, K. W., and Kim, C. D. (2015). HMGB1 induces angiogenesis in rheumatoid arthritis via HIF‐1α activation. Eur. J. Immunol. 45 (4), 1216–1227. doi:10.1002/eji.201444908
Peng, Y., Tao, H., Yang, Y., Gao, Y., Ren, H., Hu, J., et al. (2021). Chemical compositions, pharmacological activities, quality control studies of Erycibes plants, and the development of their substitutes. Phytother. Res. 35 (8), 4049–4074. doi:10.1002/ptr.7070
Pereira dos Santos Nascimento, M. V., Arruda-Silva, F., Gobbo Luz, A. B., Baratto, B., Venzke, D., Mendes, B. G., et al. (2016). Inhibition of the NF-κB and p38 MAPK pathways by scopoletin reduce the inflammation caused by carrageenan in the mouse model of pleurisy. Immunopharmacol. Immunotoxicol. 38 (5), 344–352. doi:10.1080/08923973.2016.1203929
Qu, Y., Wu, J., Deng, J.-X., Zhang, Y. P., Liang, W. Y., Jiang, Z. L., et al. (2016). MicroRNA-126 affects rheumatoid arthritis synovial fibroblast proliferation and apoptosis by targeting PIK3R2 and regulating PI3K-AKT signal pathway. Oncotarget 7 (45), 74217–74226. doi:10.18632/oncotarget.12487
Ren, H., Yang, Y., Cui, X., Hu, J., Meng, X., and Chen, Z. (2019). Recent advances and prospects of Porana sinensis. Res. Pract. Chin. Med. 33 (3), 81–86.
Sakthivel, K. M., Vishnupriya, S., Priya Dharshini, L. C., Rasmi, R. R., and Ramesh, B. (2022). Modulation of multiple cellular signalling pathways as targets for anti-inflammatory and anti-tumorigenesis action of Scopoletin. J. Pharm. Pharmacol. 74 (2), 147–161. doi:10.1093/jpp/rgab047
Sapio, L., Salzillo, A., Illiano, M., Ragone, A., Spina, A., Chiosi, E., et al. (2020). Chlorogenic acid activates ERK1/2 and inhibits proliferation of osteosarcoma cells. J. Cell. Physiol. 235 (4), 3741–3752. doi:10.1002/jcp.29269
Serttas, R., Koroglu, C., and Erdogan, S. (2021). Eupatilin inhibits the proliferation and migration of prostate cancer cells through modulation of PTEN and NF-κB signaling. Anticancer. Agents Med. Chem. 21 (3), 372–382. doi:10.2174/1871520620666200811113549
Shen, P., Wang, H.-G., Li, M.-M., Ma, Q.-Y., Zhou, C.-W., Pan, F., et al. (2017). Isofraxidin inhibited proliferation and induced apoptosis via blockage of Akt pathway in human colorectal cancer cells. Biomed. Pharmacother. 92, 78–85. doi:10.1016/j.biopha.2017.05.065
Singh, S., Anshita, D., and Ravichandiran, V. (2021). MCP-1: Function, regulation, and involvement in disease. Int. Immunopharmacol. 101, 107598. doi:10.1016/j.intimp.2021.107598
Sun, D., Zhang, F., Qian, J., Shen, W., Fan, H., Tan, J., et al. (2018). 4′-hydroxywogonin inhibits colorectal cancer angiogenesis by disrupting PI3K/AKT signaling. Chem. Biol. Interact. 296, 26–33. doi:10.1016/j.cbi.2018.09.003
Taha-Salaime, L., Davidovich-Rikanati, R., Sadeh, A., Abu-Nassar, J., Marzouk-Kheredin, S., Yahyaa, Y., et al. (2019). Phytoecdysteroid and clerodane content in three wild Ajuga species in Israel. ACS Omega 4 (1), 2369–2376. doi:10.1021/acsomega.8b03029
Tang, Y., Mo, Y., Xin, D., Zeng, L., Yue, Z., and Xu, C. (2020). β-ecdysterone alleviates osteoarthritis by activating autophagy in chondrocytes through regulating PI3K/AKT/mTOR signal pathway. Am. J. Transl. Res. 12 (11), 7174–7186.
Tarkowská, D., and Strnad, M. (2016). Plant ecdysteroids: Plant sterols with intriguing distributions, biological effects and relations to plant hormones. Planta 244 (3), 545–555. doi:10.1007/s00425-016-2561-z
Tian, Q., Wang, L., Sun, X., Zeng, F., Pan, Q., and Xue, M. (2019). Scopoletin exerts anticancer effects on human cervical cancer cell lines by triggering apoptosis, cell cycle arrest, inhibition of cell invasion and PI3K/AKT signalling pathway. J. BUON 24 (3), 997–1002.
Wang, D. (2003). Study on the chemical constituents of Gladiolus gandavensis and Porana racemosa. Doctorate. Chin. Acad. Sci.
Wang, W., and Wang, B. (2021). Isofraxidin inhibits receptor activator of nuclear factor-κB ligand–induced osteoclastogenesis in bone marrow–derived macrophages isolated from Sprague–Dawley rats by regulating NF-κB/NFATc1 and Akt/NFATc1 signaling pathways. Cell Transpl. 30, 096368972199032. doi:10.1177/0963689721990321
Wang, X., Huang, S., Xin, X., Ren, Y., Weng, G., and Wang, P. (2019). The antitumor activity of umbelliferone in human renal cell carcinoma via regulation of the p110γ catalytic subunit of PI3Kγ. Acta Pharm. 69 (1), 111–119. doi:10.2478/acph-2019-0004
Wang, X., Ren, J., Zhu, S., Ren, G., Wang, L., Chen, X., et al. (2018a). Pharmacokinetics and tissue distribution of eupatilin and its metabolite in rats by an HPLC-MS/MS method. J. Pharm. Biomed. Anal. 159, 113–118. doi:10.1016/j.jpba.2018.06.037
Wang, X., Zhu, Y., Zhu, L., Chen, X., Xu, Y., Zhao, Y., et al. (2018b). Eupatilin inhibits the proliferation of human esophageal cancer TE1 cells by targeting the Akt-GSK3β and MAPK/ERK signaling cascades. Oncol. Rep. 39 (6), 2942–2950. doi:10.3892/or.2018.6390
Wu, L., Zhu, E., Zhang, Z., and Wang, Z. (2005). Investigating original plant of Caulis Erycibes in Guangxi and identifying mainstream variety of Caulis Erycibes in market. Chin. Traditional Herb. Drugs 36 (9), 1398–1400.
Wu, Q.-z., Zhao, D.-x., Xiang, J., Zhang, M., Zhang, C.-f., and Xu, X.-h. (2016). Antitussive, expectorant, and anti-inflammatory activities of four caffeoylquinic acids isolated from Tussilago farfara. Pharm. Biol. 54 (7), 1117–1124. doi:10.3109/13880209.2015.1075048
Xue, Q., Fan, H., Li, K., Yang, L., Sun, L., and Liu, Y. (2017). Comparative evaluations on phenolic antioxidants of nine adulterants and anti-inflammation of four alternatives with their original herb Erycibe schmidtii. RSC Adv. 7 (81), 51151–51161. doi:10.1039/c7ra10767f
Xue, Q., Yin, P., Li, K., Fan, H., Yang, L., Cao, X., et al. (2019). Identification of bioactive phenolics from Porana sinensis Hemsl. stem by UPLC-QTOF-MS/MS and the confirmation of anti-inflammatory indicators using LPS-induced RAW264. 7 cells. Inflammopharmacology 27 (5), 1055–1069. doi:10.1007/s10787-018-00558-1
Yang, G., Chang, C.-C., Yang, Y., Yuan, L., Xu, L., Ho, C.-T., et al. (2018). Resveratrol alleviates rheumatoid arthritis via reducing ROS and inflammation, inhibiting MAPK signaling pathways, and suppressing angiogenesis. J. Agric. Food Chem. 66 (49), 12953–12960. doi:10.1021/acs.jafc.8b05047
Yao, X., Ding, Z., Xia, Y., Wei, Z., Luo, Y., Feleder, C., et al. (2012). Inhibition of monosodium urate crystal-induced inflammation by scopoletin and underlying mechanisms. Int. Immunopharmacol. 14 (4), 454–462. doi:10.1016/j.intimp.2012.07.024
Yeung, Y. T., Aziz, F., Guerrero-Castilla, A., and Arguelles, S. (2018). Signaling pathways in inflammation and anti-inflammatory therapies. Curr. Pharm. Des. 24 (14), 1449–1484. doi:10.2174/1381612824666180327165604
Yu, R., Xu, Q., Bogang, L., and Zhang, G. (2003). Chemical consitituents of Prana discifera. Nat. Prod. Res. Dev. 15 (5), 405–407.
Yuan, K., Zhu, Q., Lu, Q., Jiang, H., Zhu, M., Li, X., et al. (2020). Quercetin alleviates rheumatoid arthritis by inhibiting neutrophil inflammatory activities. J. Nutr. Biochem. 84, 108454. doi:10.1016/j.jnutbio.2020.108454
Yusupova, U. Y., Usmanov, D., and Ramazonov, N. S. (2019). Phytoecdysteroids from the plant dianthus helenae. Chem. Nat. Compd. 55 (2), 393–394. doi:10.1007/s10600-019-02701-y
Zeng, Y-C., Li, S., Liu, C., Gong, T., Sun, X., Fu, Y., et al. (2017). Soluplus micelles for improving the oral bioavailability of scopoletin and their hypouricemic effect in vivo. Acta Pharmacol. Sin. 38 (3), 424–433. doi:10.1038/aps.2016.126
Zhang, C., Zhang, Z., Zhang, M., and Wang, Z. (2006). Studies on chemical constituents in stems of Porana sinensis Hemsl. Chin. Pharm. J. 41 (2), 94–96. doi:10.3321/j.issn:1001-2494.2006.02.004
Zheng, X., Chen, F., Liang, Q., Lu, Y., and Zhou, G. (2018). Amide constituents from the root of Lycium yunnanense kuang and their anti-inflammatory activity. Nat. Prod. Res. Dev. 30, 603–609. doi:10.16333/j.1001-6880.2018.4.012
Zhou, K., Cheng, R., Liu, B., Wang, L., Xie, H., and Zhang, C. (2018). Eupatilin ameliorates dextran sulphate sodium-induced colitis in mice partly through promoting AMPK activation. Phytomedicine 46, 46–56. doi:10.1016/j.phymed.2018.04.033
Zhu, W.-M., Zhao, Q., Li, S.-L., and Hao, X.-J. (2007). Sesquiterpenoids from Hedychium yunnanense and Porana discifera, and the structural revision of two sesquiterpenoids from Laggera pterodonta. J. Asian Nat. Prod. Res. 9 (3), 277–283. doi:10.1080/10286020600703385
Zhu, W. (2001). A preliminary study on the chemistry of five medicinal plant resources. Doctorate. Chin. Acad. Sci. 13 (5), 1–4.
Zhu, W., Yang, X., He, H., and Hao, X. (2000). Phytoecdysones from Porana discifera. Acta Bot. Yunnanica 22 (3), 351–357. doi:10.3969/j.issn.2095-0845.2000.03.018
Keywords: Porana burm. f., traditional use, phytochemistry, network analysis, pharmacological activity
Citation: Peng Y, Li Y, Yang Y, Gao Y, Ren H, Hu J, Cui X, Lu W, Tao H and Chen Z (2022) The genus Porana (Convolvulaceae) - A phytochemical and pharmacological review. Front. Pharmacol. 13:998965. doi: 10.3389/fphar.2022.998965
Received: 20 July 2022; Accepted: 10 October 2022;
Published: 18 October 2022.
Edited by:
Wei Peng, Chengdu University of Traditional Chinese Medicine, ChinaReviewed by:
Mahdi Moridi Farimani, Shahid Beheshti University, IranJun Yu Liu, Chengdu University of Traditional Chinese Medicine, China
Copyright © 2022 Peng, Li, Yang, Gao, Ren, Hu, Cui, Lu, Tao and Chen. This is an open-access article distributed under the terms of the Creative Commons Attribution License (CC BY). The use, distribution or reproduction in other forums is permitted, provided the original author(s) and the copyright owner(s) are credited and that the original publication in this journal is cited, in accordance with accepted academic practice. No use, distribution or reproduction is permitted which does not comply with these terms.
*Correspondence: Hongxun Tao, thxshutcm@163.com; Zhiyong Chen, chenzhiyong0612@sina.com
†These authors have contributed equally to this work and share first authorship