- 1State Key Laboratory of Characteristic Chinese Medicine Resources in Southwest China, College of Pharmacy, Chengdu University of Traditional Chinese Medicine, Chengdu, China
- 2Library, Chengdu University of Traditional Chinese Medicine, Chengdu, China
- 3Institute of Interdisciplinary Medical Sciences, Shanghai University of Traditional Chinese Medicine, Shanghai, China
Puerarin, an isoflavone glycoside derived from Pueraria lobata (Willd.) Ohwi, has been identified as a pharmacologically active component with diverse benefits. A large number of experimental and clinical studies have demonstrated that puerarin is widely used in the treatment of a variety of diseases. Among them, cardiovascular diseases (CVDs) are the leading cause of death in the world, and therefore remain one of the most prominent global public health concerns. In this review, we systematically analyze the preclinical investigations of puerarin in CVDs, such as atherosclerosis, cardiac hypertrophy, heart failure, diabetic cardiovascular complications, myocardial infarction, stroke and hypertension. In addition, the potential molecular targets of puerarin are also discussed. Furthermore, we summarize the clinical trails of puerarin in the treatment of CVDs. Finally, the therapeutic effects of puerarin derivatives and its drug delivery systems are overviewed.
Introduction
Cardiovascular diseases (CVDs) remain a global health concern, as they are the number one cause of death in the world (Pardali et al., 2020). According to the World Heart Federation, 17.3 million people die from CVDs each year. By 2030, it is estimated that the number of deaths will reach 23.6 million (Smith et al., 2012). According to the American Heart Association’s methodology of projecting future care costs, by 2030, 40.5% of individuals in the United States will suffer from CVDs, with an estimated direct medical cost of $818 billion and indirect cost of $276 billion (Heidenreich et al., 2011). Therefore, effective treatment and the development of preventive intervention strategies to slow the progression of CVDs and reduce costs are momentous (Li et al., 2020). In terms of drug therapy, on the one hand, the demand for western medicine to control CVDs has not been met. On the other hand, natural product therapy has multiple targets and few adverse reactions. Thus, the latter has become an important research area for ameliorating CVDs (Hao et al., 2017; Varghese et al., 2018).
Kudzu root (Gegen in Chinese), the dried root of Pueraria lobata (Willd.) Ohwi (Figure 1A), is traditionally used as a traditional Chinese medicine (TCM) to promote blood circulation and increase blood flow (Zhang et al., 2013; Wong et al., 2011). Clinically, Gegen is mostly used in the prevention and treatment of CVDs and diabetes (Wong et al., 2011). Puerarin (C21H20O9; Figures 1B,C), an isoflavone glycoside, is the major bioactive ingredient of Gegen (Tian et al., 2016), which has been shown to be responsible for the pharmacological effects of Gegen in the cardiovascular system (Zhang et al., 2013). In addition, puerarin can also be isolated from several leguminous plants of the genus Pueraria, such as Pueraria tuberosa (Willd.) and Pueraria thomsonii Benth (Ahmad et al., 2020). Since the first isolation in the late 1950s, puerarin has been extensively studied for its effective and various pharmacological activities, including cardioprotection (Gao et al., 2006), vasodilation (Sun et al., 2007), anti-inflammation (Liu et al., 2013a), antioxidant (Jeon et al., 2020), attenuating insulin resistance (Zhang et al., 2010), neuroprotection (Zhao et al., 2020), alleviating pain (Wu et al., 2019), promoting bone formation (Yang et al., 2018a), inhibiting alcohol intake (Penetar et al., 2012) and anticancer (Hu et al., 2018). Experimental and clinical investigations have reported that puerarin is widely used in the treatment of CVDs, diabetes and its complications, Alzheimer’s disease, Parkinson’s disease, osteonecrosis, endometriosis and cancer (Zhou et al., 2014). In China, the injection form of puerarin, including Puerarin Injection (Figure 1D), Puerarin and Glucose Injection, as well as Puerarin and Sodium Chloride Injection, has been approved as a vasodilator for clinical treatment of coronary heart disease, angina, myocardial infarction (MI), retinal vein occlusion and sudden deafness (Zhang et al., 2013; Chen et al., 2018). In view of the pharmacological and therapeutic profile of puerarin in the cardiovascular system, this article reviews the cardiovascular actions of puerarin observed in experimental and clinical studies and the mechanisms underlying those effects. Moreover, the therapeutic utility of puerarin derivatives and its drug delivery systems are summarized.
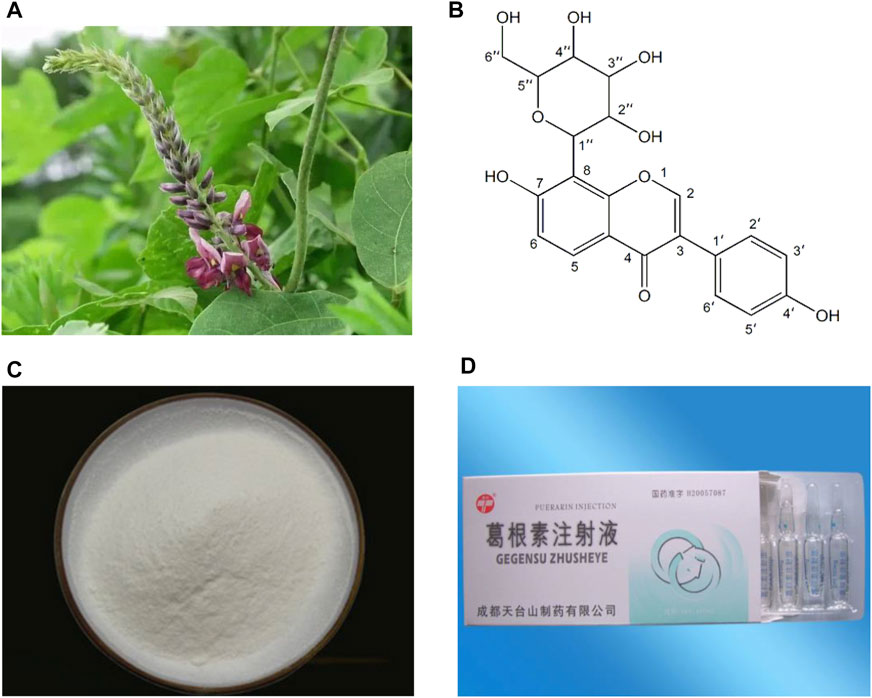
FIGURE 1. Pictures of Pueraria lobata (Willd.) Ohwi (A), chemical structure of puerarin (B), high purity puerarin crystalline powder (C) and Puerarin Injection (D).
Cadiovascular Effects of Puerrarin
Multiple studies based on animal models and cell lines have demonstrated that puerarin has strong cardiovascular effects on a variety of CVDs, such as atherosclerosis, cardiac hypertrophy, heart failure, diabetic cardiovascular complications, myocardial infarction, stroke and hypertension. The mechanisms are mainly related to reduction of inflammation, oxidative stress and apoptosis, and promotion of cardiac, endothelial and neurological function (Figure 2).
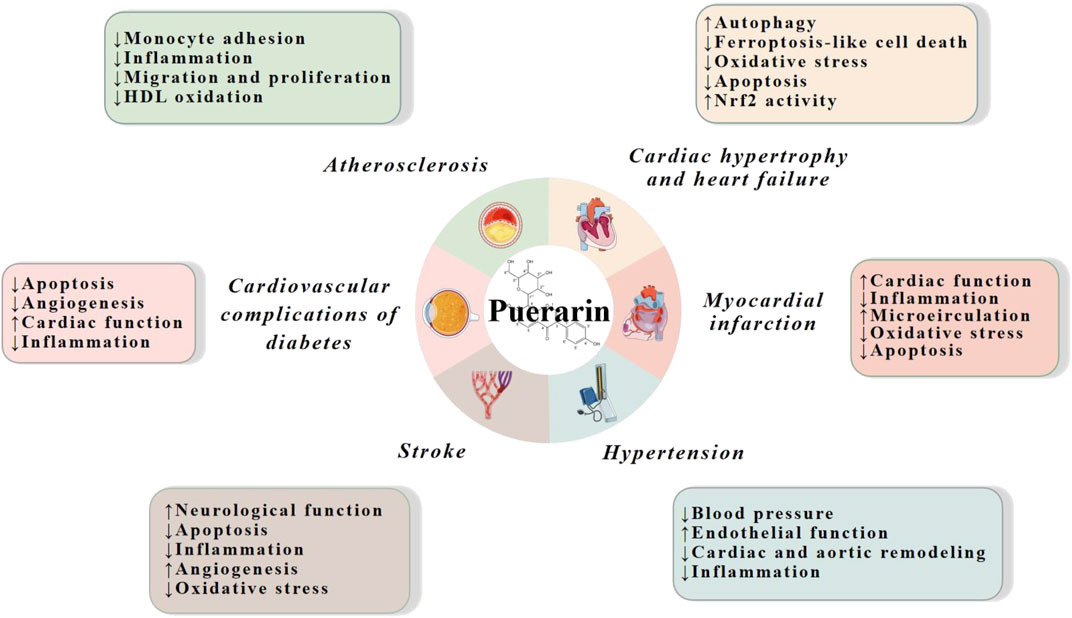
FIGURE 2. Cardiovascular actions of puerarin. HDL, high density lipoprotein; Nrf2, nuclear factor erythroid 2-related factor 2.
Atherosclerosis
Atherosclerosis, a chronic disease of the arterial wall, is the pathological basis of several diseases, such as cerebrovascular disease, coronary artery disease, acute coronary syndrome, MI and stroke (Weber and Noels, 2011). Recruitment of monocytes by activated endothelial cells to the vessel wall is an early event of atherogenesis (Mestas and Ley, 2008). The endothelium plays a vital role in controlling mass transportation, inhibiting thrombosis and modulating the growth and function of vascular smooth muscle cells (VSMCs) (Redmond et al., 2001; Kim et al., 2009). Numerous endothelial adhesion molecules including intercellular cell adhesion molecule-1 (ICAM-1), vascular cell adhesion molecule-1 (VCAM-1), selectin and integrin as well as extensive chemokines including interleukin-8 (IL-8) and monocyte chemoattractant protein-1 (MCP-1) mediate the adhesion of monocytes to vascular endothelium (Gerszten et al., 1999; Gerhardt and Ley, 2015; Wang et al., 2017). In this scenario, it has been shown that puerarin possesses the ability to modulate the expression of adhesion molecules and suppress monocyte endothelialial binding. In vitro puerarin (10 and 50 μM) decreased oxLDL-stimulated monocyte THP-1 adhesion to HUVECs and lowered the levels of adhesion-related proinflammatory chemokines (IL-8 and MCP-1) and endothelial adhesion molecules (ICAM-1 and VCAM-1). Furthermore, these protective effects were associated with activation of extracellular signal-regulated kinase 5/Kruppel-like factor 2 (ERK5/KLF2) pathway. Specifically, in vitro puerarin activated ERK5/KLF2 and then promoted the expression of endothelial nitric oxide synthase (eNOS) and thrombomodulin. Ex vivo the atherosclerotic lesions in both cross sections at aortic root and whole aorta of high fat-diet apolipoprotein E-deficient mice were markedly lessened by puerarin (100 mg/kg). Nevertheless, these alterations were reversed by XDM8-92 and BIX02189 (ERK5/KLF2 pathway inhibitors) (Deng et al., 2018).
Moreover, the migration and proliferation of VSMCs are important pathological components of atherosclerosis (Keramati et al., 2011; Yu et al., 2018). Intraperitoneal treatment of puerarin (50 and 100 mg/kg) facilitated re-endothelialization after balloon carotid denudation in rat and markedly prevented neointima formation. Besides, puerarin up-regulated the serum levels of nitric oxide (NO) and prostaglandin I2 (PGI2). In vitro puerarin strengthened late EPC and mature endothelial cell functions and inhibited the migration of VSMCs, suggesting that puerarin accelerated re-endothelialization by affecting vasodilator concentration and vascular cell functions (Cheng et al., 2013). In a rabbit model of fat diet-induced atherosclerosis, puerarin prevented the formation and development of atherosclerosis plaque and inhibited the reproduction and migration of VSMCs. Specifically, puerarin reduced the levels of total cholesterol, triglyceride and low density lipoprotein-cholesterol, while it increased high density lipoproteincholesterol production. Additionally, puerarin decreased whole blood viscosity and the expression of proliferation cell nuclear antigen and platelet-derived growth factor A protein levels of aorta (Bao et al., 2015). Besides, puerarin exerted less inhibitory effect on the high density lipoprotein oxidation induced by Cu2+ (Meng et al., 2004).
In addition to ameliorating vascular endothelial function and acting on smooth muscle cells, puerarin exerted anti-atherosclerotic activity through some other pathways. In a rat model of atherosclerosis, certain genes such as Pck1, Acaa2, DHCR7, Ascl1, Acad1, Acat1, and Nnat involved in lipid and energy metabolism were up-regulated, whereas they were down-regulated by puerarin. Besides, mitochondrial function in atherosclerosis rat aortas was meliorated by puerarin. Furthermore, peroxisome proliferator-activated receptor gamma (PPAR γ), retinoid X receptor and the corresponding downstream genes were up-regulated, whereas they were down-regulated by puerarin, suggesting that the therapeutic effect of puerarin on atherosclerosis is related to regulation of the PPAR pathway (Fu et al., 2014).
Cardiac Hypertrophy and Heart Failure
The initial development of cardiac hypertrophy is an adaptive response of the heart to physiological and pathological stimuli (Haq et al., 2000). Physiological hypertrophy is characterized by normal or augmented contractile function, and normal structure and organization of cardiac tissue. Pathological hypertrophy is related to systolic dysfunction, interstitial fibrosis, cardiac structural remodeling and myocardial fibrosis (Oka et al., 2014; Kamo et al., 2015). Over time, pathological hypertrophy eventually results in heart failure, which is a leading cause of death in patients with CVDs in the world (Li et al., 2014). In cardiac tissues, the activation of the transforming growth factor-beta (TGF-β) signaling pathway is associated with the development of myocyte hypertrophy (Villar et al., 2009). It has been evidenced that the expression and biological activity of TGF-β have changed in hypertrophic cardiomyocytes (van Wamel et al., 2002). In a mouse model of angiotensin II (Ang II)-induced heart hypertrophy, puerarin (100 mg/kg) ameliorated heart hypertrophy through up-regulation of miR-15b/195 expression and inhibition of non-canonical TGF-β signal members (TGF-β-activated-kinase 1 and p38). Similar results were obtained in Ang II-treated primary ventricular cardiomyocytes of neonatal C57BL/6J mice (Zhang et al., 2016).
Moreover, a number of studies have demonstrated that the modulation of ROS production and the regulation of nuclear factor erythroid 2-related factor 2 (Nrf2) activation by puerarin are associated with oxidative stress in cardiomyocyte hypertrophy. In vitro puerarin at 50 and 100 μM prevented ERK1/2 and c-Jun N-terminal kinase 1/2 (JNK1/2) phosphorylation, activator protein-1 binding activity, ROS level and nicotinamide adenine dinucleotide phosphate (NADPH) oxidase activity by disrupting Rac1 activation and membrane translocation of oxidase subunits (Gang et al., 2015). Nrf2 is a key master switch controlling the expression of antioxidant and protective enzymes (Erkens et al., 2015). Puerarin at 50 mg/kg attenuated abdominal aortic constriction-induced cardiac hypertrophy in rats via regulation of Nrf2. It increased Nrf2 and reduced Kelch-like ECH-associated protein 1 in the myocardium. Moreover, it elevated the nuclear accumulation of Nrf2 in parallel and the expression of associated downstream proteins (heme oxygenase 1, glutathione S-transferase P1 and NAD(P)H:quinone oxidoreductase 1). In vitro puerarin at 100 μM exerted anti-hypertrophic effect and up-regulated the metabolic enzymes UDP-glucuronosyltransferase 1A1 and 1A9 levels through activation of Nrf2 in Ang II-treated cardiomyocytes (Zhao et al., 2018). Additionally, dysregulated autophagy is related to hypertrophic process (Nakai et al., 2007). In both aortic banding rats and isoprenaline-induced H9c2 cells, puerarin blocked iron overload and lipid peroxidation (Liu et al., 2018). In addition, it ameliorated autophagy and prevented cardiomyocyte hypertrophy and apoptosis. Moreover, it activated the phosphorylation of 5′-adenosine monophosphate kinase (AMPK), while it prevented the level of mammalian target of rapamycin (mTOR) target proteins S6 ribosomal protein and 4E-binding protein 1 in vivo (Liu et al., 2015a).
Cardiovascular Complications of Diabetes
Diabetes Mellitus is an independent risk factor for CVDs (Yang et al., 2010a; Xie et al., 2020). The two predominant types of diabetes (type 1 and type 2) are both relevant to an increased risk of microvascular and macrovascular complications, the leading cause of morbidity and mortality in patients with diabetes (King et al., 2005; Forbes and Cooper, 2013). Major microvasculature defects evident in diabetes include diabetic retinopathy, diabetic kidney disease and diabetic neuropathy (Maric-Bilkan, 2017; Yang et al., 2017). Diabetic retinopathy is envisioned as a biomarker of generalized hyperglycemic damage in the microvasculature (Cheung and Wong, 2008). In addition to microvascular complications, macrovascular complications of diabetes include coronary heart disease, MI, stroke and peripheral vascular disease (Vinik and Flemmer, 2002; Chiha et al., 2012; Li et al., 2016; Ma, 2016). Additionally, both clinical and preclinical studies have demonstrated the existence of a specific diabetic cardiomyopathy independent of macrovascular complications (Davidoff et al., 2004; Huynh, Bernardo, McMullen and Ritchie, 2014). Clinically, diabetic cardiomyopathy is characterized by left ventricular hypertrophy and remodeling, and altered myocardial energy metabolism (Mizamtsidi et al., 2016). Increasing evidence suggests that puerarin is able to exert protective actions against these cardiovascular complications of diabetes.
Administration of puerarin at 140 mg/kg alleviated apoptosis of retinal pigment epithelial cells and lowered the mRNA expression of nitrotyrosine and inducible nitric oxide synthase (iNOS) and the protein expression of Fas/Fas ligand in Streptozotocin (STZ)-induced diabetic rats (Hao et al., 2012). Puerarin improved STZ-induced diabetic retinopathy by inhibiting the morphological changes of inner and outer nuclear layers via the down-regulation of vascular endothelial growth factor (VEGF) and hypoxia-inducible factor-1α (HIF-1α) expression (Teng et al., 2009). In retinal capillary endothelial cells (TR-iBRB2), puerarin (10, 25 and 50 μM) alleviated IL-1β-evoked leukocyte adhesion, the production of VCAM-1 and ICAM-1, mitochondrial dysfunction and cell apoptosis (Zhu et al., 2014). These results demonstrate that puerarin may be a potential therapeutic agent for the treatment of diabetic retinopathy.
In STZ-Nicotinamide-induced diabetic mice with permanent ligation of left anterior, puerarin promoted survival rate, restored cardiac function, augmented the expression and translocation of glucose transporter 4 and the phosphorylation of Akt, as well as decreased the expression of CD36 and PPAR α, suggesting that puerarin is able to improve cardiac dysfunction in diabetic patients after MI (Cheng et al., 2015). In diabetic rats treated with ischemia/reperfusion, puerarin (25, 50 and 100 mg/kg) decreased the myocardial infarct area and inflammatory response, while it elevated left ventricular developed pressure, NO level, caspase-3 activity, as well as the protein expression of phosphorylated-eNOS, VEGFA and Ang I, suggesting that puerarin may be an effective therapeutic candidate against cardiomyopathy in diabetic patients (Guo et al., 2018).
MI
MI is a condition of acute myocardial necrosis caused by the imbalance of coronary blood supply and myocardial demand (Liu et al., 2016a). Previous studies have shown that MI is caused by a complex set of pathological processes including inflammatory cell infiltration, an increase in free radical formation, apoptosis, irreversible DNA damage, etc (Liu et al, 2017a; Raish, 2017). Several animal models have shown that puerarin is effective in suppressing MI through multiple mechanisms. In a mouse model of isoproterenol-induced MI, puerarin reversed the typical electrocardiogram abnormal changes (Li et al., 2018). Puerarin combined treatment with tanshinone IIA blocked the release of inflammatory cells, modulated the expression of inflammatory cytokines (IL-1β, IL-6, IL-10 and iNOS), prevented M1 macrophages expression in the early stage of inflammation and increased M2 macrophages expression in myocardial ischemia mice. In addition, the combination of tanshinone IIA and puerarin inhibited inflammation by reducing toll-like receptor 4 protein expression and promoting CCAAT/enhancer-binding protein-β protein expression. Moreover, the combined application ameliorated hemodynamics through promoting cardiac function, decreasing myocardial cell damage and collagen synthesis, as well as preventing myocardial fibrosis and heart remodeling (Gao et al., 2019). In a rat model of MI, puerarin lessened infarct area in the heart, induced angiogenesis and increased the production of VEGF, HIF-1α and eNOS (Zhang et al., 2006). In a dog model of acute MI, puerarin suppressed the platelet aggregation and the blood viscosity, promoted the opening and formation of coronary collateral circulation and attenuated myocardial infarct area (Liu et al., 2000).
Furthermore, timely reperfusion is critical for the salvage of ischemic myocardium. However, reperfusion can also induce injury (Heusch, 2013). Accumulating evidence indicates that puerarin plays a protective role in myocardial ischemia/reperfusion injury. In a rat model of MI reperfusion injury, puerarin inhibited MI and MI reperfusion, reduced creatine kinase and methylene dioxyamphetamine, while it elevated superoxide dismutase (SOD) (Wenjun et al., 2015). In H9c2 cells exposed to ischemia-reperfusion, puerarin hoisted cell viability, inhibited apoptosis, lowered the levels of lactate dehydrogenase, ROS, MDA and NADPH oxidase 2, as well as increased the expression of miR-21 and the contents of SOD, catalase and glutathione peroxidase. Nevertheless, these actions were reversed through transfection of an miR-21 inhibitor, suggesting that the protective effects of puerarin against myocardial ischemia/reperfusion injury through suppressing apoptosis and oxidative stress via miR-21expression (Xu et al., 2019).
Stroke
Stroke is a leading cause of adult mortality and disability worldwide (Bonita et al., 2004; Sarikaya et al., 2015). It results from the loss of brain function due to an interruption of blood supply (Kalani et al., 2015), and can be compartmentalized into ischemic stroke and hemorrhagic stroke (Li et al., 2016). In addition, multiple mechanisms including inflammation, apoptosis, oxidative stress and other possible pathways are involved in the pathogenesis of stroke (Li et al., 2016). Intriguingly, it has been widely reported that puerarin is involved in neuroprotection, promotion of angiogenesis, anti-apoptosis, anti-oxidation and anti-inflammation, which is closely related to its prevention and treatment of stroke. In a rat model of middle cerebral artery occlusion, puerarin ameliorated neurological functions, attenuated infarct size, edema volume and autophagy in neurons, as well as augmented vascular remodeling after stroke (Xu et al., 2007; Wang et al., 2014; Wu et al., 2014; Hongyun et al., 2017). Moreover, it lessened the terminal deoxynucleotidyl transferase biotin-dUTP nick end labeling staining cells, enhanced the activation of erythropoietin activity, the expression of X-chromosome-linked inhibitor of apoptosis protein, the production of aspartate, glutamate, γ-aminobutyric acid (GABA) and taurine, the rate of glutamate/GABA, as well as lowered the levels of HIF-1α, iNOS, caspase-3 protein expression and tumor necrosis factor-α (TNF-α) mRNA expression (Xu et al., 2005; Xu et al., 2007; Chang et al., 2009; Gao et al., 2009). In another study, puerarin protected the brain neurocytes of rats in acute local ischemia brain injury by improving HSP70 expression. Meanwhile, it protected the brain neurocytes of rats in acute local cerebral ischemia/reperfusion injury by improving HSP70 expression and reducing the Fas expression (Pan and Li, 2008).
Combined treatment with catalpol and puerarin alleviated neurological deficiency induced by middle cerebral artery occlusion in rats. Moreover, catalpol and puerarin lessened infarct volume, water content in ischemic hemisphere, oxidative stress injury and apoptosis. Meanwhile, the combined treament promoted angiogenesis around the infarct of cortex and neurogenesis in the Hippocampal Dentate Gyrus, elevated regional cerebral blood flow and maintained vessel integrity (Liu et al., 2014a; Liu et al., 2017b). In another study, the combination of catalpol and puerarin restored neurological deficiency in motor, sense, balance and reflex, and protected neurovascular unit through suppression of oxidative stress and inflammatory responses and increase of certain protective factors in ischemic-reperfusion rats (Xue et al., 2016). In vitro in astrocytes after oxygen-glucose deprivation/reperfusion, puerarin reduced the level of pro-apoptosis factor and elevated the secretion of brain-derived neurotrophic factor (Wang et al., 2014). In cultured hippocampal neurons, it inhibited glutamate-stimulated apoptosis and necrosis (Xu et al., 2007). In human neutrophils in vitro, it suppressed respiratory bursts induced by formylMet-Leu-Phe (Chang et al., 2009). In oxygen-glucose deprivation-reoxygenation astrocytes, it inhibited apoptosis, modulated mRNA expression of abundant genes and impeded the olfactory transduction, janus kinase 2 and signal transducer and activator of transcription 3 pathways (Wei et al., 2017).
Hypertension
Hypertension is one of the most common diseases afflicting humans throughout the world (Ettner et al., 2012). Long-term elevated or unstable blood pressure increases the risk of vascular complications, including stroke, ischemic heart disease, congestive heart failure, renal disease and ocular fundus abnormalities (Shi et al., 2019). Increasing evidence indicates that puerarin has anti-hypertensive effect in a variety of animal models of hypertension. In spontaneously hypertensive rats, puerarin (40 and 80 mg/kg, i. p.) reduced SBP, diastolic blood pressure and heart rate, enhanced the levels of NO and cGMP and the expression of phosphorylated eNOS protein, and lowered angiotensin II type 1-receptor (AT1) and caveolin-1 levels (Shi et al., 2019). Besides, puerarin (25, 50 and100 mg/kg, i. p.) lowered the mRNA expression of TGF-β1 and Smad3, while it raised the mRNA expression of Smad7 (Zhang et al, 2011a). Treatment with high dose puerarin (200 mg/kg) up-regulated AT1 and ACE2 mRNA expression in kidney, while administration of puerarin at 100 mg/kg reduced the expression of AT1 and ACE2 mRNA in heart. Thereby, AT1 and angiotensin-converting enzyme 2 (ACE2) might be a positive feedback regulation (Ye et al., 2008). In Ang II-infused hypertensive rats, puerarin decreased SBP, aortic and left ventricular weigh, lowered aortic medial thickness and myocardial cell surface area, restored endothelium-dependent relaxation, increased the protein expression of phosphor-eNOS (Ser1177), as well as reduced the expression of gp91phox, p22phox, TGF-β1 and VCAM-1 (Li et al., 2017a). In two-kidney, one-clip renal hypertensive rats, it reduced the mean of left ventricular end diastolic pressure and the weight ratio of left ventricular mass (left ventricle plus septum) to body weight, the values of the which were 65.24 and 13.12%, respectively. In addition, it decreased the contents of apelin-36 in the plasma and left ventricle, the expression of apelin and angiotensin receptor-like protein J receptor (APJ) mRNA in left ventricle, and the levels of apelin and APJ peptides in left ventricle, the values of the which were 24.21, 49.40, 27.40, 45.66, 65.36 and 62.87%, respectively (Jin, et al., 2009). Furthermore, it decreased apelin mRNA and protein expression in ischemic and non-ischemic kidneys, while it increased APJ mRNA and protein expression in non-ischemic kidneys, and these effects were augmented by the combination of puerarin and felodipine (Huang et al., 2013). In another study, puerarin decreased SBP, diastolic blood pressure and heart rate, attenuated renal interstitial fibrosis, lowered the level of Ang II and the mRNA expression of ACE and AT1, while it increased the level of Ang (1–7) and the mRNA expression of ACE2 and Mas in kidney of renovascular hypertensive rats (Bai et al., 2013).
Others
In addition to the above-mentioned disorders, studies on experimental animals and cultured cells have shown that puerarin also plays a beneficial role in other CVDs. In chronic ischemia-induced vascular dementia rats, puerarin ameliorated hippocampal morphological changes, improved learning and memory function, promoted methyl-CpG binding protein 2 phosphorylation in the hippocampus, and lowered MDA, glutathione peroxidase and thiol production in the hippocampus and frontal cortex (Zhang et al., 2015; Wang et al., 2018). In hydrogen peroxide-treated SH-SY5Y cells, puerarin enhanced cell viability, scavenged ROS production and increased the production of Nrf2, FoxO1, FoxO3 and FoxO4 (Zhang et al., 2015). Additionally, it attenuated severe burn-stimulated acute myocardial injury via reduction of inflammation, neutrophil infiltration and oxidative stress in the heart of rats (Liu et al., 2015b). Puerarin at 2.5 mg/kg retarded CaPO4-induced aneurysm in mice. In vitro in TNF-α and CaPO4-stimulated macrophages, it reduced the Mmp9 mRNA expression, secreted protein production, as well as inhibited the production of ROS and the phosphorylation of inhibitor of I-κB, ERK and p38 (Tanaka et al., 2016). Besides, it lessened SBP and heart rate, eased pain, reduced mRNA and protein expression of P2X3 receptor (a member of P2X receptors family) in superior cervical ganglia, stellate ganglia and cervical dorsal root ganglia neurons, as well as down-regulated ATP-sensitive currents in superior cervical ganglia and cervical dorsal root ganglia neurons of myocardial ischemic rats (Liu et al., 2014b; Liu et al., 2014c). Combined administration of puerarin and Danshensu reduced ST elevation, ischemic size and serum contents of isoenzyme of creatine kinase, lactate dehydrogenase and malondialdehyde (MDA), while it up-regulated serum level of SOD in rats with acute ischemic myocardial injury (Wu et al., 2007).
Signal Transduction Pathways of Puerarin in CVDS
The cardiovascular effects of puerarin are associated with its regulation of multiple targets in the CVD-related signal pathways (Table 1; Figure 3). Among them, reports on the effects on sodium (Na+), potassium (K+) and calcium (Ca2+) channels, nuclear factor kappa B (NF-κB), PI3K/Akt, BCL-2 and BAX are more concentrated.
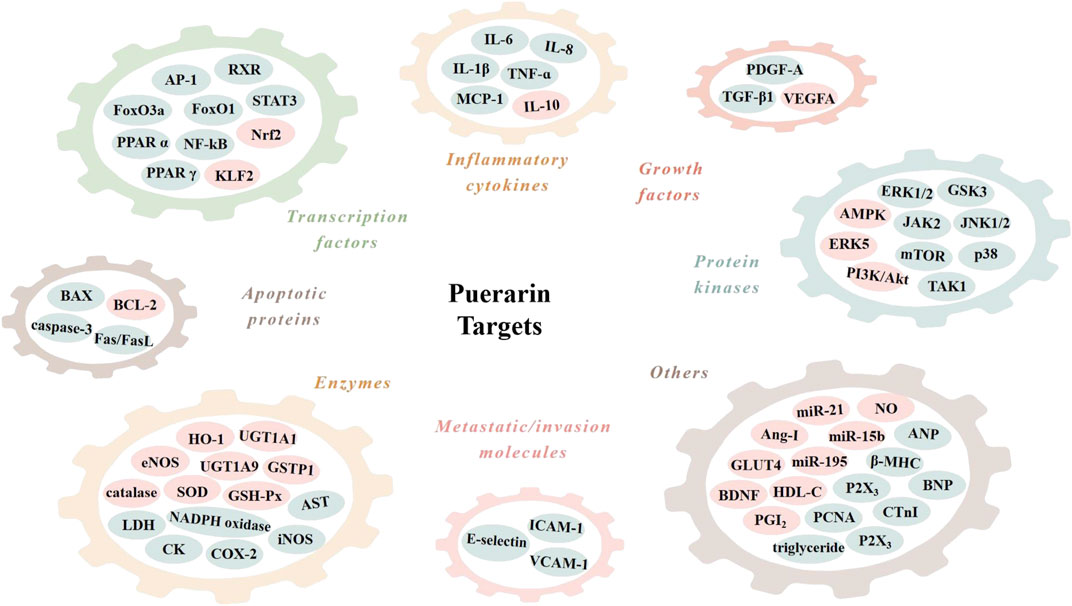
FIGURE 3. Molecular targets of puerarin. AMPK, adenosine monophosphate kinase; Ang-I, angiotensin I; ANP, atrial natriuretic peptide; AP-1, activator protein-1; AST, aspartate aminotransferase; BAX, BCL2-associated X protein; BCL-2, B cell lymphoma 2; BDNF; brain-derived neurotrophic factor; BNP, B-type natriuretic peptide; CK, creatine kinase; COX-2, cyclooxygenase-2; CTnI, cardiac troponin I; eNOS, endothelial nitric oxide synthase; ERK1/2, extracellular signal-regulated kinase 1/2; FasL, Fas ligand; FoxO1, forkhead box O1; GLUT4, glucose transporter 4; GSH-Px, glutathione peroxidase; GSK3, glycogen synthase kinase 3; GSTP1, glutathione S-transferase p 1; HDL-C, high density lipoproteincholesterol; HO-1, heme oxygenase 1; ICAM-1, intercellular cell adhesion molecule-1; IL-1β, interleukin-1β; iNOS, inducible nitric oxide synthase; JAK2, janus kinase 2; JNK1/2, c-Jun N-terminal kinase 1/2; KLF2, Kruppel-like factor 2; LDH, lactate dehydrogenase; MCP-1, monocyte chemoattractant protein-1; mTOR, mammalian target of rapamycin; NADPH oxidase, nicotinamide adenine dinucleotide phosphate oxidase; NF-κB, nuclear factor kappa B; Nrf2, nuclear factor erythroid 2-related factor 2; PCNA, proliferation cell nuclear antigen; PDGF-A, platelet-derived growth factor A; PGI2, prostaglandin I2; PPAR α, peroxisome proliferator-activated receptor α; RXR, retinoid X receptor; SOD, superoxide dismutase; STAT3, signal transducer and activator of transcription 3; TAK1, TGF-β-activated-kinase 1; TGF-β1, transforming growth factor-β1; TNF-α, tumor necrosis factor-α; UGT1A1, UDP-glucuronosyltransferase 1A1; VCAM-1, vascular cell adhesion molecule-1; VEGFA, vascular endothelial growth factor A; β-MHC, β-myosin heavy polypeptide.
Na+, K+ and Ca2+ channels
Na+ influx via slow Na+ channels may be associated with heart and brain hypoxic and ischemic damages (Ji and Wang, 1996). Inhibition of Ca2+-Na+ channels is considered to be an important indicator for the developments of potential anti-arrhythmia drugs (Zhang et al., 2003). Impaired K+ channel function in VSMCs is associated with a number of pathological conditions, including hypertension, ischemia/reperfusion, brain injury and diabetes. There are four major classes of K+ channels, including voltage-dependent K+, Ca2+-activated K+ (BKCa), ATP-sensitive K+ (KATP) and inward rectifier K+ (Kir) channels (Ko, 2008). Ca2+ influx causes an overall increase in cytoplasmic Ca2+ concentration resulting in vasoconstriction. Meanwhile, Ca2+ influx activates the local Ca2+ release event of ryanodine receptors, called Ca2+ sparks, which in turn activates nearby BKCa channels, causing hyperpolarized currents to counter vasoconstriction (Brenner et al., 2000). Accumulating evidence illustrates that puerarin can regulate the Na+ (Zhang et al., 2003), K+ (Miao et al., 2002; Findlay et al., 2008; Ravens and Cerbai, 2008) and Ca2+ (Guo, 2004) channels in cardiac myocytes, which may partly contribute to its anti-myocardial damage, anti-arrhythmic and anti-vasoconstrictive property.
In rat ventricular myocytes, puerarin exerted anti-myocardial ischemia and anti-arrhythmic action through preventing the cardiac Na+ channels (Zhang et al., 2003). In isolated rat heart, puerarin attenuated myocardial ischemia and reperfusion injury by suppressing mitochondrial permeability transition pore opening, activating the mitochondrial KATP channel (Gao et al., 2005; Gao et al., 2006) and BKCa channel opening, and elevating protein kinase C (Gao et al., 2007). In another study, puerarin at 2.4 mM time-dependently lowered L-type Ca2+ current of rat ventricular myocytes. Moreover, it increased the current-voltage curve of Ca2+ current, suggesting that puerarin provided protective effects on rat ventricular myocytes against cardiac damage and arrhythmia by suppression of L-type Ca2+ channel (Guo, 2004). Both in rat ventricular cells and Xenopus oocytes, puerarin restrained the Kir channel current (Zhang et al., 2011a). Additionally, the protective effect of puerarin on rat isolated cardiomyocytes against H2O2-stress was related to prevention of mitochondrial permeability transition pore opening and activation of mitochondrial BKCa channels (Yang et al., 2008). Puerarin exerted a vasorelaxant action on rat basilar artery rings pre-contracted by U46619. The mechanism was related to two pathways, one was an endothelium-dependent pathway associated with the production of NO, and the other was an endothelium-independent pathway involving the opening of K+ channels (Deng et al., 2012). Furthermore, puerarin at 0.1–1000 μM concentration-dependently exerted the vasodilatory action on noradrenaline bitartrate-induced rat thoracic aortac rings by activating large-conductance BKCa channels, especially BK-α+β1 channels (Sun et al., 2007). Puerarin at 50, 150 and 450 μM restrained the contraction of rat aortic rings stimulated by phenylephrine or KCl, and its action was in an endothelium-dependent manner. Meanwhile, the anti-vasoconstrictive effect of puerarin was exerted by triggering extracellular Ca2+ influx (Yan et al., 2009).
In the above studies, the specific ion channels that puerarin acts on were investigated by treatment with various channel inhibitors, such as 5-hydroxydecanoate (the mitochondrial specific KATP channel blocker) (Gao et al., 2005; Gao et al., 2006), paxilline and iberiotoxin (blockers of the BKCa channel) (Gao et al., 2007; Sun et al., 2007; Yang et al., 2008), barium (an open-channel inhibitor of Kir) (Zhang et al., 2011b) and three other K+ channel inhibitors, glibenclamide, tetraethylammonium and 4-aminopyridine (Yan et al., 2009; Deng et al., 2012).
NF-κB
The transcription factor NF-κB, a nuclear protein, was initial discovered by David Baltimore’s group in 1986 (Sen and Baltimore, 1986a; Sen and Baltimore, 1986b). NF-κB is a family of structurally related and functionally conserved dimeric proteins that consist of five members trapped in the cytoplasm under non-activated conditions: c-Rel, RelA (p65), RelB, p50/p105 and p52/p100 (Liu et al., 2013b; Ma and Hottiger, 2016). NF-κB binds to target DNA elements in the nucleus and positively regulates gene transcription involved in inflammatory and immune responses, cell growth control and apoptosis (Baldwin, 2001). Increasing evidences suggest that the cardiovascular regulatory activity of puerarin may involve the regulation of the NF-κB pathway.
In Dahl salt-sensitive hypertensive rats, puerarin ameliorated vascular insulin resistance and attenuated cardiovascular remodeling involving the suppression of NF-κB/JNK and ERK1/2 pathway (Tan et al., 2017). In Sprague-Dawley (SD) rats, administration of puerarin alleviated ischemia/reperfusion injury stimulated by 90 min of middle cerebral artery occlusion and followed by 2, 6, 12, 24 and 72 h reperfusion via suppressing the activation of NF-κB (Ding et al., 2007). In STZ-induced diabetic rats and in rat embryonic cardiomyoblast-derived H9c2 cells injured by high glucose in vitro, puerarin prevented diabetic cardiomyopathy through inhibiting inflammation via suppression of NF-kB activation (Yin et al., 2019). Moreover, it prevented Ang II-stimulated cardiac hypertrophy in C57BL/6J mice and in cultured cardiomyocytes via the redox-sensitive ERK1/2, p38 and NF-κB pathways (Chen et al., 2014). In Kunming mice, it inhibited isoprenaline-induced myocardial fibrosis through inhibiting TGF-β1 expression via activation of PPAR α/γ and subsequent blockade of NF-κB in myocardial tissue (Chen et al, 2012a). In addition, by reducing NF-κB activation, puerarin inhibted the inflammatory response in a rabbit model of atherosclerosis in vivo (Ji et al., 2016a) and in peripheral blood mononuclear cells of patients with stable angina pectoris in vitro (Yang et al., 2010b).
PI3K/Akt
PI3K play a vital role in the pathogenesis of CVDs via modulating several basic cellular functions, such as cell migration, translational responses and cell survival, and subsequently regulating certain essential biologic processes, such as vascular homeostasis, metabolism and thrombogenicity (Eisenreich and Rauch, 2011). PI3K mediates key signaling pathways through several downstream molecules, such as Akt, mTOR, 3-phosphoinositide-dependent kinase 1 and glycogen synthase kinase 3 (Cantley, 2002; Wymann et al., 2003). A large body of studies indicate that the PI3K/Akt signaling pathway may be involved in the cardiovascular protective activity of puerarin.
In a rat model of MI, puerarin enhanced serum NO content by increase of eNOS expression and Akt pathway (Zhang et al., 2008). In aortic banding mice, oral treatment with puerarin eased the progression of cardiac hypertrophy and ameliorated cardiac function by reduction of PI3K/Akt and JNK signaling (Yuan et al., 2014). Both in transgenic TG (fli1:EGFP) zebrafish and in cultured human endothelial cells, puerarin attenuated Aß40-induced vascular dysfunction via inhibition of NADPH oxidase-derived ROS overproduction and activation of the PI3K/Akt/eNOS pathway (Lu et al., 2014a). In neonatal rat cardiomyocytes isolated from the ventricular heart of 1–2 days old SD rats, puerarin retarded myocardial ischemia/reperfusion injury through reducing autophagy via the Akt signaling pathway (Tang et al., 2017). In daunorubicin-treated H9c2 cells, puerarin inhibited cell apoptosis by activating the PI3K/Akt pathway via the suppression of intracellular Ca2+ influx (Li et al., 2017b). In EA. hy926 endothelial cells, puerarin stimulated the phosphorylation of eNOS and the production of NO by provoking estrogen receptor-mediated PI3K/Akt- and Ca2+/calmodulin-dependent kinase II/AMPK-dependent signaling pathway (Hwang et al., 2011). Furthermore, it inhibited the onset of endothelial progenitor cell (EPC) senescence and promoted EPC proliferation in vitro, which might be mediated by the activation of telomerase via the PI3K/Akt pathway (Zhu et al., 2008). It was effective in abating osteoblastic differentiation of calcifying VSMCs as indicated by reduced alkaline phosphatase activity, osteocalcin secretion and Runx2 expression, which was related to targeting the estrogen receptor-mediated PI3K/Akt signaling pathway (Lu et al., 2014b). Additionally, it attenuated the neurocyte apoptosis and protected against cerebral ischemia/reperfusion injury in rats induced by Longa’s suture method possibly through the activation of the PI3K/Akt pathway (Han et al, 2012a). Combined treatment with catalpol and puerarin protected primary brain vascular endothelial cells from ischemic injury by promoting HIF-1α via activation of ERK and PI3K/Akt (Liu et al., 2017a). In the above studies, the PI3K inhibitor(s), such as LY294002 (Han et al, 2012b; Lu et al., 2014a) and wortmannin (Zhu et al., 2008), or the Akt inhibitor, such as API-2 (Tang et al., 2017) and 1L-6-hydroxymethyl-chiro-inositol 2-(R)-2-O-methyl-3-O-octadecylcarbonate (Lu et al., 2014b), was/were used to confirm that PI3K/Akt pathway does play a role in the cardiovascular effect of puerarin.
BCL-2 and BAX
BCL-2 family proteins, including both pro-life (e.g., BCL-2, BCL-XL) and pro-death (e.g., BAX, BAK) members, are the key regulators and executors of the intrinsic apoptosis pathway (van Delft and Huang, 2006; Singh et al., 2019). Apoptosis is a mechanism of programmed cell death and plays an important role in human health and disease (Volkmann et al., 2014). BAX and BAK regulate a cell to its programmed death by permeabilizing the mitochondrial outer membrane and then initiating the caspase cascade (Edlich, 2018). In vivo and in vitro studies suggest that the effect of puerarin on cardiovascular cell apoptosis may be related to its regulation of the expression of BCL-2 and BAX.
Puerarin attenuated apoptosis in rat middle cerebral artery occlusion brain by elevating the levels of cleaved caspase-3 and BAX and the ratio of BAX/BCL-2. Furthermore, puerarin inhibited apoptosis in primary cultured cortical rat astrocytes induced by oxygen-glucose deprivation/reperfusion injury via down-regulation of BAX level and BAX/BCL-2 rate and up-regulation of BCL-2 level and brain-derived neurotrophic factor expression (Wang et al., 2014). In CoCl2-damaged PC12 cells, combined administration of catalpol and puerarin inhibited apoptosis involving increase of BCL-2 level and decrease of BAX/BCL-2 ratio and caspase-3 level (Liu et al., 2014a). Puerarin treatment caused the same change trend on BCL-2 level and caspase-3 activity in rat H9c2 cardiomyocytes under H/R conditions (Xu et al., 2019). In rat pulmonary artery smooth muscle cells, puerarin induced apoptosis as indicated by down-regulated mitochondrial membrane potential and BCL-2 protein expression, cytochrome c release from the mitochondria, increased BAX protein expression and caspase-9 activation (Zhang et al, 2011c; Chen et al., 2012b). In subarachnoid hemorrhage mice, puerarin abated the neurological deficits, cerebral edema and blood-brain barrier disruption. In addition, puerarin elevated the ratio of BCL-2/BAX and the production of SOD and Sirt3, while it repressed the levels of cleaved caspase-3 and ROS (Zhang et al, 2019a). In SD rats, puerarin enhanced the learning-memory ability after global cerebral ischemia/reperfusion by attenuating apoptosis via elevation of BCL-2 expression (Wu et al., 2009).
Clinical Trials of Puerarin in CVDS
In addition to the cardiovascular benefits observed in cell-based and animal models, puerarin also exerted protective effects on patients with CVDs (Table 2). An open, controlled, randomized and parallel-group comparison trail (http://clinicaltrials.gov/, NCT02254655) on 119 subjects with a definite diagnose of active rheumatoid arthritis demonstrated that 24 weeks of intravenous treatment with puerarin reduced carotid intima-media thickness and its action might be related to the amelioration of insulin resistance, indicating that puerarin might be a potential therapeutic candidate against cardio-metabolic comorbidities in patients with active rheumatoid arthritis (Yang et al., 2018b). A clinical trail was carried out among 120 coronary artery disease patients with stable angina pectoris. Both treatment and control groups were treated with nitrate, Ca2+ antagonists, β-receptor blockers, statins and aspirin. Patients in the treatment group were given 28 consecutive days of combinative injection with puerarin. At the end of the study, the treatment group was better than the control group in terms of the total effective rate, the duration of angina pectoris, the improvement of the ST segment depression of electrocardiogram, the number of abnormal leads and the scores of Seattle angina questionnaire. Furthermore, there was a elevation of EPCs and NO levels and reduction of endothelin 1 (ET-1) expression and the levels of serum hypersensitive C-reactive protein, TNF-α and IL-6, and these alterations were more obvious in the treatment group than those in the control group (Zhang et al., 2019b). A study involving 388 patients with angina pectoris was conducted and it was found that the total efficacy of puerarin injection group and danshen injection group was 88.14% (171/194) and 61.86% (120/194), respectively. In addition, no obvious adverse reactions were noticed in both groups (Luo et al., 2012). A study carried out on 79 patients with anterior acute MI showed that the infarct size on the 28th day and the contents of granulocyte colony-stimulating factor, matrix metalloproteinase-9, IL-6 and TNF-α on the 7th day were reduced in the puerarin group (Xiao et al., 2005). A 2-weeks randomized controlled trial was conducted on 61 patients with acute MI. The results indicated that puerarin reduced plasma concentration of free fatty acids, suppressed inflammation and stabilized atherosclerotic plaques, and it was involved in the reduction of myocardial infarct size (Xiao et al., 2004). In another clinical trail, puerarin was demonstrated to be an effective therapeutic agent for cerebral vasospasm in patients after aneurysm subarachnoid hemorrhage. Puerarin treatment elevated the plasma concentration of NO, ET-1 and 6-keto-prostaglandin F1α, the mean velocity of middle cerebral artery, and the Glasgow outcome scale at discharge, while it reduced the plasma level of thromboxane B2 and the incidence of cerebral vasospasm (Wang et al., 2012). Recently, a randomized, double-blind, placebo-controlled, 2-way crossover trial (http://clinicaltrials.gov/, NCT03676296) on the effect of puerarin supplementation on CVD risk factors in men has been completed. The expected results are to obtain the short-term effect of puerarin on CVD risk factors in men, and provide evidence for the effect of puerarin on other potentially relevant risk factors, such as blood pressure, fasting blood glucose and testosterone, as well as some related biomarkers.
A Cochrane review revealed that there was no notable difference between the puerarin group and the control group in death or dependence of patients with acute ischemic stroke (OR, 0.81; 95% CI, 0.35–1.87) (Tan et al., 2008). Similarly, another Cochrane review concluded that there was insufficient evidence to assess the clinical efficacy of puerarin on the survival or dependence of patients with ischemic stroke (Liu et al., 2016b). However, a recent meta-analysis showed that the clinical effective rate of puerarin injection in the treatment of acute ischemic stroke was higher than the control drugs (RR 1.22, 95% CI 1.17 to 1.28, p < 0.001). Moreover, puerarin injection evidently ameliorated the neurological deficit (MD -3.69, 95% CI -4.67 to -2.71, p < 0.001). The hemorheology index and fibrinogen of puerarin injection were significantly lower than those of the control group. Collectively, it was suggested that puerarin injection might be more effective in the clinical treatment of acute ischemic stroke with relative safety. Nevertheless, the current evidence was not adequate in virtue of the poor methodological quality and short of sufficient safety data. The adverse reactions of puerarin injection in curing acute ischemic stroke included nausea, vomiting, and other mild gastrointestinal discomfort, facial flushing, dizziness and allergic reaction (Zheng et al., 2017).
The Derivatives and Drug Deliery Systems of Puerarin
The chemical structure of puerarin leads to low solubility and permeability, which results in poor oral absorption and low bioavailability. Simply increasing the dose will not effectively improve the bioavailability, and may lead to toxicity and side effects (Liu et al, 2016c). In view of this situation, a large number of studies are focused on developing derivatives or drug delivery systems to improve bioavailability and expand clinical applications. Among them, strategies include nanotechnologies and various preparation technologies (Zhang, 2019). In this section, only the derivatives and drug delivery systems of puerarin designed for cardiovascular applications are included. In a study, 7 puerarin derivatives (Figures 4A–G) were evaluated for their cardioprotective effect in the model of myocardial I/R injury of Langendorff isolated rat heart and the model of pituitrin-stimulatd SD rat myocardial ischemia, suggesting that the acylated modification of phenolic hydroxyl at C-7 in the puerarin molecule promoted the cardioprotective effect against myocardial ischemia and reperfusion injury, and the effect on the activity was related to the level of the bulk of the acylating agent (Feng et al., 2010). Puerarin derivative P1-EA (Figure 4H) and P2-EA (Figure 4I) were formed by substitution of the original phenolic hydroxyl in the puerarin molecules, which had higher lipophilicity and thus better permeabilized through the blood-brain barrier as compared to puerarin. It was resulted in an enhancement of protective activity against cerebral ischemic damage in a mouse model of ischemia/reperfusion-evoked dementia. Specifically, these two derivatives showed stronger suppression of inflammation (iNOS activity) and elevation of brain cortex Ca2+-Mg2+-ATPase activity (Ji et al., 2016b). Puerarin-7-O-glucuronide (Figure 4J), a water-soluble metabolite of puerarin, lessened intracellular superoxide level and improved total SOD activity, glutathione/glutathione disulfide ratio and total anti-oxidant capacity in xanthine oxidase/xanthine-induced neonatal rat cardiomyocytes. Similarly, it lowered superoxide production, NADPH oxidase activity and the expression of its subunits (p22phox and p47phox) in Ang II-stimulated neonatal rat cardiomyocytes. In addition, it lowered hypertrophic changes in Ang II-induced neonatal rat cardiomyocytes. Collectively, puerarin-7-O-glucuronide inhibited Ang II-induced cardiomyocyte hypertrophy in vitro via reduction of oxidative stress (Hou et al., 2017). In a rat model of cerebral ischemia/reperfusion injury, 3′-methoxy puerarin (Figure 4K) improved the cerebral tissue pathologic changes, enhanced the level of PGI2 in cerebral tissue and the activity of plasma tissue-type plasminogen activator, and lowered the activity of plasma plasminogen activator inhibitor and the mRNA expression of ET-1 in cerebral tissue (Zhao et al., 2007). Moreover, it ameliorated the symptoms of neurological deficit, lowered the infarct volume and the level of MDA in cortex, and up-regulated SOD activity (Han et al., 2009). Another study reported that 3′-methoxy puerarin protected acute cerebral infarction by inhibiting the levels of both excitatory amino acid (aspartate and glutamate) and inhibitory amino acid (taurine and GABA), and thereby regulated the dynamic changes of amino acids (Han et al, 2012b).
Nanoparticles containing puerarin and hydroxypropyl-β-cyclodextrin inclusion complex lowered infarction volume, reduced the brain infiltration of inflammatory cells and the neuronal pyknosis and karyolysis, alleviated cell apoptosis, and improved electroencephalogram in middle cerebral artery occlusion/reperfusion model rats (Tao et al., 2013). The drug concentrations in blood and brain for puerarin loaded poly (butylcyanoacrylate) nanoparticles (PBCN) were both higher than those for the free drug, suggesting the permeability of puerarin loaded PBCN coated with polysorbate 80 on the blood-brain barrier was higher than that of puerarin. Additionally, compared to free drug, intravenous administration of puerarin loaded PBCN showed greater protective activity on focal cerebral ischemic injury in rats, and its action was related to raising body weight and reducing neurological deficit scores, brain water content and the infarct volume (Zhao et al., 2013). In another study, a puerarin nanoparticle based on glycyrrhetinic acid-PEG-PBLA was synthesized and its protective effect against liver ischemia/reperfusion injury was evaluated in vivo. Synthesized glycyrrhetinic acid-PEG-PBLA improved the water solubility, bioactivity and litter half-life of puerarin and augmented the liver-targeted drug delivery. Moreover, pretreatment with puerarin/glycyrrhetinic acid-PEG-PBLA complex ameliorated liver dysfunction and oxidative stress via the toll-like receptor 4/NF-κB pathway, thus alleviating liver ischemia/reperfusion injury (Xiao et al., 2018). In a rat model of acute myocardial ischemia stimulated by coronary artery ligation, puerarin PEG-PLGA micelles obviously promoted the uptake of puerarin by cardiomyocytes, augmented the anti-acute myocardial ischemia activity of puerarin and lowered its dosage (Liu et al., 2019). RGD modified and PEGylated solid lipid nanoparticles loaded with puerarin (RGD/PEG-puerarin-SLN) elevated the bioavailability of puerarin, prolonged the retention time in vivo and increased the protective activity against acute myocardial ischemia injury. The areas under the concentration-time curve augmented from 52.93 (mg/mL•h) for free puerarin to 176.5 (mg/mL•h) for RGD/PEG-puerarin-SLN. T1/2 elevated from 0.73 h for free puerarin to 2.62 h for RGD/PEG-puerarin-SLN. Compared to other puerarin formulations, RGD/PEG-puerarin-SLN showed higher drug concentration in heart and plasma. Furthermore, the myocardial infarct size of RGD/PEG-puerarin-SLN was the lowest among all the formulations in acute MI model rats. The infarct size of RGD/PEG-puerarin-SLN, PEG-puerarin-SLN, puerarin-SLN and free puerarin was 6.2, 18.1, 28.3 and 36.0%, respectively (Dong et al., 2017). Similarly, the in vivo myocardial infarct therapy efficiency of puerarin-prodrug and tanshinone co-loaded SLN, puerarin-prodrug-SLN and tanshinone-SLN were evaluated in MI rats, which was 17 ± 1.9%, 31 ± 1.6% and 40 ± 2.2%, respectively. Additionally, SLN encapsulation attenuated the cytotoxicity of the drug and was a safer system. These results suggested that puerarin-prodrug contained, double drugs co-loaded SLN might be a candidate drug delivery system for cardioprotective drugs for the treatment of MI (Guo et al., 2019).
In addition to puerarin derivatives and its drug delivery systems, the modified crystal forms of puerarin also have better absorption and higher plasma drug concentration than puerarin. Puerarin-V, a new advantageous crystal form of puerarin, suppressed the typical ST segment depression and the production of myocardial injury markers, ameliorated ventricular wall infarction, and reduced the levels of IL-1β, IL-6 and TNF-α via regulation of the PPAR γ/NF-κB pathway in the isoproterenol-evoked MI mice. Furthermore, it attenuated cell apoptosis and lightened the production of inflammation cytokines in lipopolysaccharide-stimulated human coronary artery endothelial cells (Li et al., 2018).
Conclusion and Future Perspective
TCM has been used in China for thousands of years to treat various diseases. In recent decades, more and more studies have been carried out on TCM, and numerous impressive achievements have been achieved, such as artemisinin, a gift from TCM to the world (Tu, 2016). With the progress of biotechnology in component separation, more pharmacological active components have been isolated from TCM, and some of them have become commonly used drugs in clinic, such as berberine and curcumin. As an active ingredient of traditional herbal medicine Gegen, puerarin has been recognized to possess a wide range of pharmacological activities. Basic and clinical studies have indicated that puerarin is an effective therapeutic candidate against diabetes and its complications, osteonecrosis, cancer, Alzheimer’s disease, Parkinson’s disease and endometriosis. Furthermore, emerging studies over the past few decades have shown that puerarin plays a critical role in protecting humans and animals from CVDs, including atherosclerosis, cardiac hypertrophy, heart failure, diabetic cardiovascular complications, MI, stroke and hypertension. The efficacy of puerarin for treating multiple CVDs may be mainly mediated by modulation of Na+, K+ and Ca2+ channels, NF-κB, PI3K/Akt, BCL-2, and BAX. Furthermore, it may be related to the regulation of other targets such as PPAR, AMPK, AT1, ACE2, Nrf2, TNF-ɑ, IL-8, COX-2, and P2X3. Due to these cardiovascular protective effects, puerarin has been developed for clinical use. For example, Puerarin Injection, Puerarin and Glucose Injection, as well as Puerarin and Sodium Chloride Injection have been approved as clinical drugs for the treatment of certain CVDs in China. In other countries, a few clinical trials have been conducted to investigate the effect of puerarin on CVDs.
However, the solubility and permeability of puerarin are low, resulting in poor oral absorption and low bioavailability, which eventually limits its application in clinic. A simple increase in drug dose will not effectively improve bioavailability, and may cause side effects and toxicity. To overcome this obstacle, by modifying the structure of puerarin, a number of derivatives with better bioavailability have been developed, such as P1-EA, P2-EA, puerarin-7-O-glucuronide and 3′-methoxy puerarin. In addition, a variety of effective drug delivery systems of puerarin have been developed using nanotechnologies and multiple preparation technologies. Nevertheless, the toxicological data of puerarin derivatives and drug delivery systems are insufficient and need further research. Besides, other in-depth studies are required for puerarin development. The pathways underlying the cardiovascular properties of puerarin are complicated. Its direct molecular mechanism and key targets need to be further clarified. What’s more, further clinical trials with a larger sample size and rigorous design are needed to validate the current findings in the prevention and treatment of CVDs. With more intensive experimental and clinical studies on puerarin, its pharmacological mechanism will be revealed more definite, the forms of medication will be more diversified, and the clinical indications will be more extensive in the future. In this case, puerarin will also have the potential to become a safe and effective innovative Chinese medicine into the world.
Author Contributions
Y-XZ contributed to the drafting of the manuscript. HZ conducted various literature survey studies and prepared the initial draft of figures. CP designed, conceived and supervised process.
Funding
This work was supported by Xinglin Scholar Fund of Chengdu University of Traditional Chinese Medicine (YXRC2018006).
Conflict of Interest
The authors declare that the research was conducted in the absence of any commercial or financial relationships that could be construed as a potential conflict of interest.
Publisher’s Note
All claims expressed in this article are solely those of the authors and do not necessarily represent those of their affiliated organizations, or those of the publisher, the editors and the reviewers. Any product that may be evaluated in this article, or claim that may be made by its manufacturer, is not guaranteed or endorsed by the publisher.
References
Ahmad, B., Khan, S., Liu, Y., Xue, M., Nabi, G., Kumar, S., et al. (2020). Molecular Mechanisms of Anticancer Activities of Puerarin. Cancer Manag. Res. 12, 79–90. doi:10.2147/CMAR.S233567
Bai, S., Huang, Z. G., Chen, L., Wang, J. T., and Ding, B. P. (2013). Effects of Felodipine Combined with Puerarin on ACE2-Ang (1-7)-Mas axis in Renovascular Hypertensive Rat. Regul. Pept. 184, 54–61. doi:10.1016/j.regpep.2013.03.005
Baldwin, A. S. (2001). Series Introduction: the Transcription Factor NF-kappaB and Human Disease. J. Clin. Invest. 107, 3–6. doi:10.1172/JCI11891
Bao, L., Zhang, Y., Wei, G., Wang, Y., Ma, R., Cheng, R., et al. (2015). The Anti-atherosclerotic Effects of Puerarin on Induced-Atherosclerosis in Rabbits. Biomed. Pap. Med. Fac. Univ. Palacky Olomouc Czech Repub 159, 53–59. doi:10.5507/bp.2013.096
Bonita, R., Mendis, S., Truelsen, T., Bogousslavsky, J., Toole, J., and Yatsu, F. (2004). The Global Stroke Initiative. Lancet Neurol. 3, 391–393. doi:10.1016/S1474-4422(04)00800-2
Brenner, R., Peréz, G. J., Bonev, A. D., Eckman, D. M., Kosek, J. C., Wiler, S. W., et al. (2000). Vasoregulation by the Beta1 Subunit of the Calcium-Activated Potassium Channel. Nature 407, 870–876. doi:10.1038/35038011
Cantley, L. C. (2002). The Phosphoinositide 3-kinase Pathway. Science 296, 1655–1657. doi:10.1126/science.296.5573.1655
Chang, Y., Hsieh, C. Y., Peng, Z. A., Yen, T. L., Hsiao, G., Chou, D. S., et al. (2009). Neuroprotective Mechanisms of Puerarin in Middle Cerebral Artery Occlusion-Induced Brain Infarction in Rats. J. Biomed. Sci. 16, 9. doi:10.1186/1423-0127-16-9
Chen, C., Chen, C., Wang, Z., Wang, L., Yang, L., Ding, M., et al. (2012a). Puerarin Induces Mitochondria-dependent Apoptosis in Hypoxic Human Pulmonary Arterial Smooth Muscle Cells. PLoS One 7, e34181. doi:10.1371/journal.pone.0034181
Chen, G., Pan, S. Q., Shen, C., Pan, S. F., Zhang, X. M., and He, Q. Y. (2014). Puerarin Inhibits Angiotensin II-Induced Cardiac Hypertrophy via the Redox-Sensitive ERK1/2, P38 and NF-Κb Pathways. Acta Pharmacol. Sin 35, 463–475. doi:10.1038/aps.2013.185
Chen, R., Xue, J., and Xie, M. (2012b). Puerarin Prevents Isoprenaline-Induced Myocardial Fibrosis in Mice by Reduction of Myocardial TGF-Β1 Expression. J. Nutr. Biochem. 23, 1080–1085. doi:10.1016/j.jnutbio.2011.05.015
Chen, X., Yu, J., and Shi, J. (2018). Management of Diabetes Mellitus with Puerarin, a Natural Isoflavone from Pueraria Lobata. Am. J. Chin. Med. 46, 1771–1789. doi:10.1142/S0192415X18500891
Cheng, M., Li, X., Guo, Z., Cui, X., Li, H., Jin, C., et al. (2013). Puerarin Accelerates Re-endothelialization in a Carotid Arterial Injury Model: Impact on Vasodilator Concentration and Vascular Cell Functions. J. Cardiovasc. Pharmacol. 62, 361–368. doi:10.1097/FJC.0b013e31829dd961
Cheng, W., Wu, P., Du, Y., Wang, Y., Zhou, N., Ge, Y., et al. (2015). Puerarin Improves Cardiac Function through Regulation of Energy Metabolism in Streptozotocin-Nicotinamide Induced Diabetic Mice after Myocardial Infarction. Biochem. Biophys. Res. Commun. 463, 1108–1114. doi:10.1016/j.bbrc.2015.06.067
Cheung, N., and Wong, T. Y. (2008). Diabetic Retinopathy and Systemic Vascular Complications. Prog. Retin. Eye Res. 27, 161–176. doi:10.1016/j.preteyeres.2007.12.001
Chiha, M., Njeim, M., and Chedrawy, E. G. (20122012). Diabetes and Coronary Heart Disease: a Risk Factor for the Global Epidemic. Int. J. Hypertens. 2012, 697240. doi:10.1155/2012/697240
Davidoff, A. J., Davidson, M. B., Carmody, M. W., Davis, M. E., and Ren, J. (2004). Diabetic Cardiomyocyte Dysfunction and Myocyte Insulin Resistance: Role of Glucose-Induced PKC Activity. Mol. Cel Biochem 262, 155–163. doi:10.1023/b:mcbi.0000038231.68078.4b
Deng, Y., Lei, T., Li, H., Mo, X., Wang, Z., and Ou, H. (2018). ERK5/KLF2 Activation Is Involved in the Reducing Effects of Puerarin on Monocyte Adhesion to Endothelial Cells and Atherosclerotic Lesion in Apolipoprotein E-Deficient Mice. Biochim. Biophys. Acta Mol. Basis Dis. 1864, 2590–2599. doi:10.1016/j.bbadis.2018.04.021
Deng, Y., Ng, E. S., Yeung, J. H., Kwan, Y. W., Lau, C. B., Koon, J. C., et al. (2012). Mechanisms of the Cerebral Vasodilator Actions of Isoflavonoids of Gegen on Rat Isolated Basilar Artery. J. Ethnopharmacol 139, 294–304. doi:10.1016/j.jep.2011.11.021
Ding, M. P., Feng, F., and Hu, H. T. (2007). Effects of Puerarin on Expression of Nuclear Factor kappaB after Cerebral Ischemia/reperfusion in Rats. Zhongguo Zhong yao za zhi 32 (23), 2515–2518.
Dong, Z., Guo, J., Xing, X., Zhang, X., Du, Y., and Lu, Q. (2017). RGD Modified and PEGylated Lipid Nanoparticles Loaded with Puerarin: Formulation, Characterization and Protective Effects on Acute Myocardial Ischemia Model. Biomed. Pharmacother. 89, 297–304. doi:10.1016/j.biopha.2017.02.029
Edlich, F. (2018). BCL-2 Proteins and Apoptosis: Recent Insights and Unknowns. Biochem. Biophys. Res. Commun. 500, 26–34. doi:10.1016/j.bbrc.2017.06.190
Eisenreich, A., and Rauch, U. (2011). PI3K Inhibitors in Cardiovascular Disease. Cardiovasc. Ther. 29, 29–36. doi:10.1111/j.1755-5922.2010.00206.x
Erkens, R., Kramer, C. M., Lückstädt, W., Panknin, C., Krause, L., Weidenbach, M., et al. (2015). Left Ventricular Diastolic Dysfunction in Nrf2 Knock Out Mice Is Associated with Cardiac Hypertrophy, Decreased Expression of SERCA2a, and Preserved Endothelial Function. Free Radic. Biol. Med. 89, 906–917. doi:10.1016/j.freeradbiomed.2015.10.409
Ettner, R., Ettner, F., and White, T. (20122012). Secrecy and the Pathogenesis of Hypertension. Int. J. Fam. Med 2012, 492718. doi:10.1155/2012/492718
Feng, Z. Q., Wang, Y. Y., Guo, Z. R., Chu, F. M., and Sun, P. Y. (2010). The Synthesis of Puerarin Derivatives and Their Protective Effect on the Myocardial Ischemia and Reperfusion Injury. J. Asian Nat. Prod. Res. 12, 843–850. doi:10.1080/10286020.2010.505563
Findlay, I., Suzuki, S., Murakami, S., and Kurachi, Y. (2008). Physiological Modulation of Voltage-dependent Inactivation in the Cardiac Muscle L-type Calcium Channel: a Modelling Study. Prog. Biophys. Mol. Biol. 96, 482–498. doi:10.1016/j.pbiomolbio.2007.07.002
Forbes, J. M., and Cooper, M. E. (2013). Mechanisms of Diabetic Complications. Physiol. Rev. 93, 137–188. doi:10.1152/physrev.00045.2011
Fu, R., Zhang, Y., Guo, Y., Zhang, Y., Xu, Y., and Chen, F. (2014). Digital Gene Expression Analysis of the Pathogenesis and Therapeutic Mechanisms of Ligustrazine and Puerarin in Rat Atherosclerosis. Gene 552, 75–80. doi:10.1016/j.gene.2014.09.015
Gang, C., Qiang, C., Xiangli, C., Shifen, P., Chong, S., and Lihong, L. (2015). Puerarin Suppresses Angiotensin II-Induced Cardiac Hypertrophy by Inhibiting NADPH Oxidase Activation and Oxidative Stress-Triggered AP-1 Signaling Pathways. J. Pharm. Pharm. Scisociete Canadienne Des Sciences Pharmaceutiques, 18, 235–248. doi:10.18433/j3n318
Gao, L., Ji, X., Song, J., Liu, P., Yan, F., Gong, W., et al. (2009). Puerarin Protects against Ischemic Brain Injury in a Rat Model of Transient Focal Ischemia. Neurol. Res. 31 (4), 402–406. doi:10.1179/174313209X444017
Gao, Q., Pan, H. Y., Qiu, S., Lu, Y., Bruce, I. C., Luo, J. H., et al. (2006). Atractyloside and 5-hydroxydecanoate Block the Protective Effect of Puerarin in Isolated Rat Heart. Life Sci. 79, 217–224. doi:10.1016/j.lfs.2005.12.040
Gao, Q., Yang, B., Ye, Z. G., Wang, J., Bruce, I. C., and Xia, Q. (2007). Opening the Calcium-Activated Potassium Channel Participates in the Cardioprotective Effect of Puerarin. Eur. J. Pharmacol. 574, 179–184. doi:10.1016/j.ejphar.2007.07.018
Gao, Q., Pan, H. Y., Bruce, I. C., and Xia, Q. (2005). Improvement of Ventricular Mechanical Properties by Puerarin Involves Mitochondrial Permeability Transition in Isolated Rat Heart during Ischemia and Reperfusion. Conference Proceedings Annual Conference. Annual International Conference of the IEEE Engineering in Medicine and Biology Society. IEEE Engineering in Medicine and Biology Society, 5591–5594.
Gao, S., Li, L., Li, L., Ni, J., Guo, R., Mao, J., et al. (2019). Effects of the Combination of Tanshinone IIA and Puerarin on Cardiac Function and Inflammatory Response in Myocardial Ischemia Mice. J. Mol. Cel Cardiol 137, 59–70. doi:10.1016/j.yjmcc.2019.09.012
Gerhardt, T., and Ley, K. (2015). Monocyte Trafficking across the Vessel wall. Cardiovasc. Res. 107, 321–330. doi:10.1093/cvr/cvv147
Gerszten, R. E., Garcia-Zepeda, E. A., Lim, Y. C., Yoshida, M., Ding, H. A., Gimbrone, M. A., et al. (1999). MCP-1 and IL-8 Trigger Firm Adhesion of Monocytes to Vascular Endothelium under Flow Conditions. Nature 398, 718–723. doi:10.1038/19546
Guo, B. Q., Xu, J. B., Xiao, M., Ding, M., and Duan, L. J. (2018). Puerarin Reduces Ischemia/reperfusion-Induced Myocardial Injury in Diabetic Rats via Upregulation of Vascular Endothelial Growth Factor A/angiotensin-1 and Suppression of Apoptosis. Mol. Med. Rep. 17, 7421–7427. doi:10.3892/mmr.2018.8754
Guo, J., Xing, X., Lv, N., Zhao, J., Liu, Y., Gong, H., et al. (2019). Therapy for Myocardial Infarction: In Vitro and In Vivo Evaluation of Puerarin-Prodrug and Tanshinone Co-loaded Lipid Nanoparticulate System. Biomed. Pharmacother. 120, 109480. doi:10.1016/j.biopha.2019.109480
Guo, X. G., Chen, J. Z., Zhang, X., and Xia, Q. (2004). Effect of Puerarin on L-type Calcium Channel in Isolated Rat Ventricular Myocytes. Zhongguo Zhong yao za zhi 29 (3), 248–251.
Han, J., Wan, H., Ge, L., Zhu, Z., Guo, Y., Zhang, Y., et al. (2009). Protective Effect of 3'-methoxy Puerarin on Cerebral Ischemic Injury/Reperfusion in Rats. Zhongguo Zhong Yao Za Zhi 34 (11), 1422–1425.
Han, J., Wan, H., Li, J., and Ge, L. (2012b). Study on 3'-methoxy Puerarin to Dynamic Changes of Amino Acids in Rat Brain Ischemia-Reperfusion. Zhongguo Zhong Yao Za Zhi 37 (7), 1023–1027.
Han, J. Q., Yu, K. Y., and He, M. (2012a). Effects of Puerarin on the Neurocyte Apoptosis and P-Akt (Ser473) Expressions in Rats with Cerebral Ischemia/reperfusion Injury. Zhongguo Zhong Xi Yi Jie He Za Zhi 32 (8), 1069–1072.
Hao, L. N., Wang, M., Ma, J. L., and Yang, T. (2012). Puerarin Decreases Apoptosis of Retinal Pigment Epithelial Cells in Diabetic Rats by Reducing Peroxynitrite Level and iNOS Expression. Sheng Li Xue Bao 64 (2), 199–206.
Hao, P., Jiang, F., Cheng, J., Ma, L., Zhang, Y., and Zhao, Y. (2017). Traditional Chinese Medicine for Cardiovascular Disease: Evidence and Potential Mechanisms. J. Am. Coll. Cardiol. 69, 2952–2966. doi:10.1016/j.jacc.2017.04.041
Haq, S., Choukroun, G., Kang, Z. B., Ranu, H., Matsui, T., Rosenzweig, A., et al. (2000). Glycogen Synthase Kinase-3beta Is a Negative Regulator of Cardiomyocyte Hypertrophy. J. Cel Biol 151, 117–130. doi:10.1083/jcb.151.1.117
Heidenreich, P. A., Trogdon, J. G., Khavjou, O. A., Butler, J., Dracup, K., Ezekowitz, M. D., et al. (2011). Forecasting the Future of Cardiovascular Disease in the United States. Circulation, 123, 933–944. doi:10.1161/cir.0b013e31820a55f5
Heusch, G. (2013). Cardioprotection: Chances and Challenges of its Translation to the Clinic. Lancet 381, 166–175. doi:10.1016/S0140-6736(12)60916-7
Hongyun, H., Tao, G., Pengyue, Z., Liqiang, Y., and Yihao, D. (2017). Puerarin Provides a Neuroprotection against Transient Cerebral Ischemia by Attenuating Autophagy at the Ischemic Penumbra in Neurons but Not in Astrocytes. Neurosci. Lett. 643, 45–51. doi:10.1016/j.neulet.2017.02.009
Hou, N., Cai, B., Ou, C. W., Zhang, Z. H., Liu, X. W., Yuan, M., et al. (2017). Puerarin-7-O-glucuronide, a Water-Soluble Puerarin Metabolite, Prevents Angiotensin II-Induced Cardiomyocyte Hypertrophy by Reducing Oxidative Stress. Naunyn Schmiedebergs Arch. Pharmacol. 390, 535–545. doi:10.1007/s00210-017-1353-8
Hu, Y., Li, X., Lin, L., Liang, S., and Yan, J. (2018). Puerarin Inhibits Non-small Cell Lung Cancer Cell Growth via the Induction of Apoptosis. Oncol. Rep. 39, 1731–1738. doi:10.3892/or.2018.6234
Huang, Z. G., Bai, S., Chen, L., Wang, J. T., and Ding, B. P. (2013). Effect of Puerarin Combined with Felodipine on mRNA and Protein Expression of Apelin and APJ in Renovascular Hypertensive Rat. Zhongguo Zhong yao za zhi 38 (3), 381–385.
Huynh, K., Bernardo, B. C., McMullen, J. R., and Ritchie, R. H. (2014). Diabetic Cardiomyopathy: Mechanisms and New Treatment Strategies Targeting Antioxidant Signaling Pathways. Pharmacol. Ther. 142, 375–415. doi:10.1016/j.pharmthera.2014.01.003
Hwang, Y. P., Kim, H. G., Hien, T. T., Jeong, M. H., Jeong, T. C., and Jeong, H. G. (2011). Puerarin Activates Endothelial Nitric Oxide Synthase through Estrogen Receptor-dependent PI3-Kinase and Calcium-dependent AMP-Activated Protein Kinase. Toxicol. Appl. Pharmacol. 257, 48–58. doi:10.1016/j.taap.2011.08.017
Jeon, Y. D., Lee, J. H., Lee, Y. M., and Kim, D. K. (2020). Puerarin Inhibits Inflammation and Oxidative Stress in Dextran Sulfate Sodium-Induced Colitis Mice Model. Biomed. Pharmacother. 124, 109847. doi:10.1016/j.biopha.2020.109847
Ji, H. L., and Wang, T. Y. (1996). Puerarin Inhibits Tetrodotoxin-Resistant Sodium Current in Rat Dorsal Root Ganglion Neurons. Zhongguo yao li xue bao 17 (2), 115–118.
Ji, L., Du, Q., Li, Y., and Hu, W. (2016a). Puerarin Inhibits the Inflammatory Response in Atherosclerosis via Modulation of the NF-Κb Pathway in a Rabbit Model. Pharmacol. Rep. 68, 1054–1059. doi:10.1016/j.pharep.2016.06.007
Ji, Y., Jiang, P., and Yan, X. (2016b). Anticerebral Ischemia-Reperfusion Injury Activity of Synthesized Puerarin Derivatives. Biomed. Res. Int. 2016, 9821767. doi:10.1155/2016/9821767
Jin, G., Yang, P., Gong, Y., Fan, X., Tang, J., and Lin, J. (2009). Effects of Puerarin on Expression of Apelin and its Receptor of 2K1C Renal Hypertension Rats. Zhongguo Zhong yao za zhi 34 (24), 3263–3267.
Kalani, A., Kamat, P. K., Kalani, K., and Tyagi, N. (2015). Epigenetic Impact of Curcumin on Stroke Prevention. Metab. Brain Dis. 30, 427–435. doi:10.1007/s11011-014-9537-0
Kamo, T., Akazawa, H., and Komuro, I. (2015). Cardiac Nonmyocytes in the Hub of Cardiac Hypertrophy. Circ. Res. 117, 89–98. doi:10.1161/CIRCRESAHA.117.305349
Keramati, A. R., Singh, R., Lin, A., Faramarzi, S., Ye, Z. J., Mane, S., et al. (2011). Wild-type LRP6 Inhibits, whereas Atherosclerosis-Linked LRP6R611C Increases PDGF-dependent Vascular Smooth Muscle Cell Proliferation. Proc. Natl. Acad. Sci. U S A. 108, 1914–1918. doi:10.1073/pnas.1019443108
Kim, H. J., Yoo, E. K., Kim, J. Y., Choi, Y. K., Lee, H. J., Kim, J. K., et al. (2009). Protective Role of Clusterin/apolipoprotein J against Neointimal Hyperplasia via Antiproliferative Effect on Vascular Smooth Muscle Cells and Cytoprotective Effect on Endothelial Cells. Arterioscler Thromb. Vasc. Biol. 29, 1558–1564. doi:10.1161/ATVBAHA.109.190058
King, K. D., Jones, J. D., and Warthen, J. (2005). Microvascular and Macrovascular Complications of Diabetes Mellitus. Am. J. Pharm. Education 69, 1–10. doi:10.5688/aj690587
Ko, E. A., Han, J., Jung, I. D., and Park, W. S. (2008). Physiological Roles of K+ Channels in Vascular Smooth Muscle Cells. J. Smooth Muscle Res. 44 (2), 65–81. doi:10.1540/jsmr.44.65
Li, H., Gao, S., Ye, J., Feng, X., Cai, Y., Liu, Z., et al. (2014). COX-2 Is Involved in ET-1-Induced Hypertrophy of Neonatal Rat Cardiomyocytes: Role of NFATc3. Mol. Cel Endocrinol 382, 998–1006. doi:10.1016/j.mce.2013.11.012
Li, H., Sureda, A., Devkota, H. P., Pittalà, V., Barreca, D., Silva, A. S., et al. (2020). Curcumin, the golden Spice in Treating Cardiovascular Diseases. Biotechnol. Adv. 38, 107343. doi:10.1016/j.biotechadv.2019.01.010
Li, W., Lu, M., Zhang, Y., Xia, D., Chen, Z., Wang, L., et al. (2017b). Puerarin Attenuates the Daunorubicin-Induced Apoptosis of H9c2 Cells by Activating the PI3K/Akt Signaling Pathway via the Inhibition of Ca2+ Influx. Int. J. Mol. Med. 40, 1889–1894. doi:10.3892/ijmm.2017.3186
Li, W., Suwanwela, N. C., and Patumraj, S. (2016). Curcumin by Down-Regulating NF-kB and Elevating Nrf2, Reduces Brain Edema and Neurological Dysfunction after Cerebral I/R. Microvasc. Res. 106, 117–127. doi:10.1016/j.mvr.2015.12.008
Li, X., Lin, Y., Zhou, H., Li, Y., Wang, A., Wang, H., et al. (2017a1993). Puerarin Protects against Endothelial Dysfunction and End-Organ Damage in Ang II-Induced Hypertension. Clin. Exp. Hypertens. 39 (1), 58–64. doi:10.1080/10641963.2016.1200603
Li, X., Yuan, T., Chen, D., Chen, Y., Sun, S., Wang, D., et al. (2018). Cardioprotective Effects of Puerarin-V on Isoproterenol-Induced Myocardial Infarction Mice Is Associated with Regulation of PPAR-Υ/nf-Κb Pathway. Molecules 23, 3322. doi:10.3390/molecules23123322
Liu, B., Tan, Y., Wang, D., and Liu, M. (2016a). Puerarin for Ischaemic Stroke. Cochrane Database Syst. Rev. 2, CD004955. doi:10.1002/14651858.CD004955.pub3
Liu, B., Wu, Z., Li, Y., Ou, C., Huang, Z., Zhang, J., et al. (2015a). Puerarin Prevents Cardiac Hypertrophy Induced by Pressure Overload through Activation of Autophagy. Biochem. Biophys. Res. Commun. 464, 908–915. doi:10.1016/j.bbrc.2015.07.065
Liu, B., Zhao, C., Li, H., Chen, X., Ding, Y., and Xu, S. (2018). Puerarin Protects against Heart Failure Induced by Pressure Overload through Mitigation of Ferroptosis. Biochem. Biophys. Res. Commun. 497, 233–240. doi:10.1016/j.bbrc.2018.02.061
Liu, F., Rehmani, I., Esaki, S., Fu, R., Chen, L., de Serrano, V., et al. (2013b). Pirin Is an Iron-Ggdependent Redox Regulator of NF-Κb. Proc. Natl. Acad. Sci. U S A. 110, 9722–9727. doi:10.1073/pnas.1221743110
Liu, G., Liu, Z., and Yuan, S. (2016c). Recent Advances in Methods of Puerarin Biotransformation. Mini Rev. Med. Chem. 16 (17), 1392–1402. doi:10.2174/1389557516666160505114456
Liu, J., Sui, H., Zhao, J., and Wang, Y. (2017a). Osmotin Protects H9c2 Cells from Simulated Ischemia-Reperfusion Injury through AdipoR1/PI3K/AKT Signaling Pathway. Front. Physiol. 8, 611. doi:10.3389/fphys.2017.00611
Liu, Q., Lu, Z., and Wang, L. (2000). Restrictive Effect of Puerarin on Myocardial Infarct Area in Dogs and its Possible Mechanism. J. Tongji Med. Univ. 20 (1), 43–45. doi:10.1007/BF02887673
Liu, S., Ren, H. B., Chen, X. L., Wang, F., Wang, R. S., Zhou, B., et al. (2015b). Puerarin Attenuates Severe Burn-Induced Acute Myocardial Injury in Rats. Burns 41, 1748–1757. doi:10.1016/j.burns.2015.06.001
Liu, S., Yu, S., Xu, C., Peng, L., Xu, H., Zhang, C., et al. (2014b). Puerarin Alleviates Aggravated Sympathoexcitatory Response Induced by Myocardial Ischemia via Regulating P2X3 Receptor in Rat superior Cervical Ganglia. Neurochem. Int. 70, 39–49. doi:10.1016/j.neuint.2014.03.004
Liu, S., Zhang, C., Shi, Q., Li, G., Song, M., Gao, Y., et al. (2014c). Puerarin Blocks the Signaling Transmission Mediated by P2X3 in SG and DRG to Relieve Myocardial Ischemic Damage. Brain Res. Bull. 101, 57–63. doi:10.1016/j.brainresbull.2014.01.001
Liu, X., Mei, Z., Qian, J., Zeng, Y., and Wang, M. (2013a). Puerarin Partly Counteracts the Inflammatory Response after Cerebral Ischemia/reperfusion via Activating the Cholinergic Anti-inflammatory Pathway. Neural Regen. Res. 8, 3203–3215. doi:10.3969/j.issn.1673-5374.2013.34.004
Liu, X. Y., Jiang, Z. B., Luo, J., Li, J. H., and Hu, X. B. (2019). In Vitro Evaluation, Cellular Uptake and Anti-acute Myocardial Ischemia Effect of Puerarin PEG-PLGA Micelles. Zhongguo Zhong Yao Za Zhi 44 (11), 2244–2250. doi:10.19540/j.cnki.cjcmm.20190321.302
Liu, Y., Tang, Q., Shao, S., Chen, Y., Chen, W., and Xu, X. (2017b). Lyophilized Powder of Catalpol and Puerarin Protected Cerebral Vessels from Ischemia by its Anti-apoptosis on Endothelial Cells. Int. J. Biol. Sci. 13 (3), 327–338. doi:10.7150/ijbs.17751
Liu, Y., Xue, Q., Li, X., Zhang, J., Fu, Z., Feng, B., et al. (2014a). Amelioration of Stroke-Induced Neurological Deficiency by Lyophilized Powder of Catapol and Puerarin. Int. J. Biol. Sci. 10, 448–456. doi:10.7150/ijbs.8571
Liu, Y. T., Zhou, C., Jia, H. M., Chang, X., and Zou, Z. M. (2016b). Standardized Chinese Formula Xin-Ke-Shu Inhibits the Myocardium Ca(2+) Overloading and Metabolic Alternations in Isoproterenol-Induced Myocardial Infarction Rats. Sci. Rep. 6, 30208. doi:10.1038/srep30208
Lu, Q., Xiang, D. X., Yuan, H. Y., Xiao, Y., Yuan, L. Q., and Li, H. B. (2014b). Puerarin Attenuates Calcification of Vascular Smooth Muscle Cells. Am. J. Chin. Med. 42, 337–347. doi:10.1142/S0192415X14500220
Lu, X. L., Liu, J. X., Wu, Q., Long, S. M., Zheng, M. Y., Yao, X. L., et al. (2014a). Protective Effects of Puerarin against Aß40-Induced Vascular Dysfunction in Zebrafish and Human Endothelial Cells. Eur. J. Pharmacol. 732, 76–85. doi:10.1016/j.ejphar.2014.03.030
Luo, Z. K., Liu, Y., and Li, H. M. (2012). A Clinical Efficacy and Safety Study on Coronary Heart Disease and Angina Treatment with Puerarin Injection. Zhonghua Liu Xing Bing Xue Za Zhi 33 (6), 614–616.
Ma, B., and Hottiger, M. O. (2016). Crosstalk between Wnt/β-Catenin and NF-Κb Signaling Pathway during Inflammation. Front. Immunol. 7, 378. doi:10.3389/fimmu.2016.00378
Ma, R. C. (2016). Genetics of Cardiovascular and Renal Complications in Diabetes. J. Diabetes Investig. 7, 139–154. doi:10.1111/jdi.12391
Maric-Bilkan, C. (20171979). Sex Differences in Micro- and Macro-Vascular Complications of Diabetes Mellitus. Clin. Sci. 131, 833–846. doi:10.1042/cs20160998
Meng, F., Liu, R., Bai, H., Liu, B. W., and Liu, Y. (2004). Inhibitory Effect of Quercetin, Rutin and Puerarin on HDL Oxidation Induced by Cu2+. Sichuan Da Xue Xue Bao Yi Xue Ban 35, 836–838.
Mestas, J., and Ley, K. (2008). Monocyte-endothelial Cell Interactions in the Development of Atherosclerosis. Trends Cardiovasc. Med. 18, 228–232. doi:10.1016/j.tcm.2008.11.004
Miao, W. N., Shen, Y. J., and Zeng, X. R. (2002). Effect of Puerarin on K+ Channel of Isolated Ventricular Myocyte in guinea Pig. Zhongguo ying yong sheng li xue za zhi 18 (2), 155–158.
Mizamtsidi, M., Paschou, S. A., Grapsa, J., and Vryonidou, A. (2016). Diabetic Cardiomyopathy: a Clinical Entity or a Cluster of Molecular Heart Changes? Eur. J. Clin. Invest. 46, 947–953. doi:10.1111/eci.12673
Nakai, A., Yamaguchi, O., Takeda, T., Higuchi, Y., Hikoso, S., Taniike, M., et al. (2007). The Role of Autophagy in Cardiomyocytes in the Basal State and in Response to Hemodynamic Stress. Nat. Med. 13, 619–624. doi:10.1038/nm1574
Oka, T., Akazawa, H., Naito, A. T., and Komuro, I. (2014). Angiogenesis and Cardiac Hypertrophy: Maintenance of Cardiac Function and Causative Roles in Heart Failure. Circ. Res. 114, 565–571. doi:10.1161/CIRCRESAHA.114.300507
Pan, H. P., and Li, G. (2008). Protecting Mechanism of Puerarin on the Brain Neurocyte of Rat in Acute Local Ischemia Brain Injury and Local Cerebral Ischemia-Reperfusion Injury. Yakugaku Zasshi 128 (11), 1689–1698. doi:10.1248/yakushi.128.1689
Pardali, E., Dimmeler, S., Zeiher, A. M., and Rieger, M. A. (2020). Clonal Hematopoiesis, Aging, and Cardiovascular Diseases. Exp. Hematol. 83, 95–104. doi:10.1016/j.exphem.2019.12.006
Penetar, D. M., Toto, L. H., Farmer, S. L., Lee, D. Y., Ma, Z., Liu, Y., et al. (20122012). The Isoflavone Puerarin Reduces Alcohol Intake in Heavy Drinkers: a Pilot Study. Drug Alcohol Depend 126, 251–256. doi:10.1016/j.drugalcdep.2012.04.012
Raish, M. (2017). Momordica Charantia Polysaccharides Ameliorate Oxidative Stress, Hyperlipidemia, Inflammation, and Apoptosis during Myocardial Infarction by Inhibiting the NF-Κb Signaling Pathway. Int. J. Biol. Macromol 97, 544–551. doi:10.1016/j.ijbiomac.2017.01.074
Ravens, U., and Cerbai, E. (2008). Role of Potassium Currents in Cardiac Arrhythmias. Europace 10, 1133–1137. doi:10.1093/europace/eun193
Redmond, E. M., Cullen, J. P., Cahill, P. A., Sitzmann, J. V., Stefansson, S., Lawrence, D. A., et al. (2001). Endothelial Cells Inhibit Flow-Induced Smooth Muscle Cell Migration: Role of Plasminogen Activator Inhibitor-1. Circulation 103, 597–603. doi:10.1161/01.cir.103.4.597
Sarikaya, H., Ferro, J., and Arnold, M. (2015). Stroke Prevention-Mmedical and Lifestyle Measures. Eur. Neurol. 73, 150–157. doi:10.1159/000367652
Sen, R., and Baltimore, D. (1986a). Inducibility of Kappa Immunoglobulin Enhancer-Binding Protein NF-Kappa B by a Posttranslational Mechanism. Cell 47 (6), 921–928. doi:10.1016/0092-8674(86)90807-x
Sen, R., and Baltimore, D. (1986b). Multiple Nuclear Factors Interact with the Immunoglobulin Enhancer Sequences. Cell 46 (5), 705–716. doi:10.1016/0092-8674(86)90346-6
Shi, W., Yuan, R., Chen, X., Xin, Q., Wang, Y., Shang, X., et al. (2019). Puerarin Reduces Blood Pressure in Spontaneously Hypertensive Rats by Targeting eNOS. Am. J. Chin. Med. 47 (1), 19–38. doi:10.1142/S0192415X19500022
Singh, R., Letai, A., and Sarosiek, K. (2019). Regulation of Apoptosis in Health and Disease: the Balancing Act of BCL-2 Family Proteins. Nat. Rev. Mol. Cel Biol 20, 175–193. doi:10.1038/s41580-018-0089-8
Smith, S. C., Collins, A., Ferrari, R., Holmes, D. R., Logstrup, S., McGhie, D. V., et al. (2012). World Heart F, American Heart A, American College of Cardiology F, European Heart N and European Society of COur Time: a Call to Save Preventable Death from Cardiovascular Disease (Heart Disease and Stroke). J. Am. Coll. Cardiol. 60, 2343–2348. doi:10.1016/j.jacc.2012.08.962
Sun, X. H., Ding, J. P., Li, H., Pan, N., Gan, L., Yang, X. L., et al. (2007). Activation of Large-Conductance Calcium-Activated Potassium Channels by Puerarin: the Underlying Mechanism of Puerarin-Mediated Vasodilation. J. Pharmacol. Exp. Ther. 323, 391–397. doi:10.1124/jpet.107.125567
Tan, C., Wang, A., Liu, C., Li, Y., Shi, Y., and Zhou, M. S. (2017). Puerarin Improves Vascular Insulin Resistance and Cardiovascular Remodeling in Salt-Sensitive Hypertension. Am. J. Chin. Med. 45 (6), 1169–1184. doi:10.1142/S0192415X17500641
Tan, Y., Liu, M., and Wu, B. (2008). Puerarin for Acute Ischaemic Stroke. Cochrane Database Syst. Rev. 39, CD004955–2188. doi:10.1002/14651858.CD004955.pub2
Tanaka, T., Moriyama, T., Kawamura, Y., and Yamanouchi, D. (2016). Puerarin Suppresses Macrophage Activation via Antioxidant Mechanisms in a CaPO4-Induced Mouse Model of Aneurysm. J. Nutr. Sci. Vitaminol (Tokyo) 62 (6), 425–431. doi:10.3177/jnsv.62.425
Tang, H., Song, X., Ling, Y., Wang, X., Yang, P., Luo, T., et al. (2017). Puerarin Attenuates Myocardial Hypoxia/reoxygenation Injury by Inhibiting Autophagy via the Akt Signaling Pathway. Mol. Med. Rep. 15, 3747–3754. doi:10.3892/mmr.2017.6424
Tao, H. Q., Meng, Q., Li, M. H., Yu, H., Liu, M. F., Du, D., et al. (2013). HP-β-CD-PLGA Nanoparticles Improve the Penetration and Bioavailability of Puerarin and Enhance the Therapeutic Effects on Brain Ischemia-Reperfusion Injury in Rats. Naunyn Schmiedebergs Arch. Pharmacol. 386, 61–70. doi:10.1007/s00210-012-0804-5
Teng, Y., Cui, H., Yang, M., Song, H., Zhang, Q., Su, Y., et al. (2009). Protective Effect of Puerarin on Diabetic Retinopathy in Rats. Mol. Biol. Rep. 36, 1129–1133. doi:10.1007/s11033-008-9288-2
Tian, T., Cai, X., and Zhu, H. (2016). Puerarin, an Isoflavone Compound Extracted from Gegen (Radix Puerariae Lobatae), Modulates Sclera Remodeling Caused by Extremely Low Frequency Electromagnetic fields. J. Tradit Chin. Med. 36, 678–682. doi:10.1016/s0254-6272(16)30089-9
Tu, Y. (2016). Artemisinin-A Gift from Traditional Chinese Medicine to the World (Nobel Lecture). Angew. Chem. Int. Ed. Engl. 55, 10210–10226. doi:10.1002/anie.201601967
van Delft, M. F., and Huang, D. C. (2006). How the Bcl-2 Family of Proteins Interact to Regulate Apoptosis. Cell Res 16, 203–213. doi:10.1038/sj.cr.7310028
van Wamel, A. J., Ruwhof, C., van der Valk-Kokshoorn, L. J., Schrier, P. I., and van der Laarse, A. (2002). Stretch-induced Paracrine Hypertrophic Stimuli Increase TGF-Beta1 Expression in Cardiomyocytes. Mol. Cel Biochem 236, 147–153. doi:10.1023/a:1016138813353
Varghese, J. F., Patel, R., Singh, M., and Yadav, U. C. S. (2018). “Herbal Intervention in Cardiovascular Diseases,” in Functional Food and Human Health, 277–296. doi:10.1007/978-981-13-1123-9_14
Villar, A. V., Llano, M., Cobo, M., Expósito, V., Merino, R., Martín-Durán, R., et al. (2009). Gender Differences of Echocardiographic and Gene Expression Patterns in Human Pressure Overload Left Ventricular Hypertrophy. J. Mol. Cel Cardiol 46, 526–535. doi:10.1016/j.yjmcc.2008.12.024
Vinik, A., and Flemmer, M. (2002). Diabetes and Macrovascular Disease. J. Diabetes Complications 16, 235–245. doi:10.1016/s1056-8727(01)00212-4
Volkmann, N., Marassi, F. M., Newmeyer, D. D., and Hanein, D. (2014). The Rheostat in the Membrane: BCL-2 Family Proteins and Apoptosis. Cell Death Differ 21, 206–215. doi:10.1038/cdd.2013.153
Wang, D., Wang, Z., Zhang, L., and Wang, Y. (2017). Roles of Cells from the Arterial Vessel Wall in Atherosclerosis. Mediators Inflamm. 2017, 8135934. doi:10.1155/2017/8135934
Wang, H. Q., Zhang, M., Zhao, J. X., Wu, H. Q., Gao, Z., Zhang, G. L., et al. (2018). Puerarin Up-Regulates Methyl-CpG Binding Protein 2 Phosphorylation in Hippocampus of Vascular Dementia Rats. Chin. J. Integr. Med. 24, 372–377. doi:10.1007/s11655-018-2822-0
Wang, J. W., Gao, J. M., and Huang, Y. J. (2012). Effects of Puerarin on the Vascular Active Factor Related to Cerebral Vasospasm after Aneurysm Subarachnoid Hemorrhage. Zhongguo Zhong Xi Yi Jie He Za Zhi 32 (2), 164–167.
Wang, N., Zhang, Y., Wu, L., Wang, Y., Cao, Y., He, L., et al. (2014). Puerarin Protected the Brain from Cerebral Ischemia Injury via Astrocyte Apoptosis Inhibition. Neuropharmacology 79, 282–289. doi:10.1016/j.neuropharm.2013.12.004
Weber, C., and Noels, H. (2011). Atherosclerosis: Current Pathogenesis and Therapeutic Options. Nat. Med. 17, 1410–1422. doi:10.1038/nm.2538
Wei, S., Tong, J., Xue, Q., Liu, Y., and Xu, X. (2017). Effect of Puerarin on Transcriptome of Astrocyte during Oxygen-Glucose Deprivation/reoxygenation Injury. Mol. Cel Biochem 425, 113–123. doi:10.1007/s11010-016-2867-y
Wenjun, H., Jing, W., Tao, L., Ling, M., Yan, Y., Xiaorong, Z., et al. (2015). The Protective Effect of Puerarin on Myocardial Infarction Reperfusion Injury (MIRI): A Meta-Analysis of Randomized Studies in Rat Models. Med. Sci. Monit. 21, 1700–1706. doi:10.12659/MSM.894312
Wong, K. H., Li, G. Q., Li, K. M., Razmovski-Naumovski, V., and Chan, K. (2011). Kudzu Root: Traditional Uses and Potential Medicinal Benefits in Diabetes and Cardiovascular Diseases. J. Ethnopharmacol 134, 584–607. doi:10.1016/j.jep.2011.02.001
Wu, H. Q., Guo, H. N., Wang, H. Q., Chang, M. Z., Zhang, G. L., and Zhao, Y. X. (2009). Protective Effects and Mechanism of Puerarin on Learning-Memory Disorder after Global Cerebral Ischemia-Reperfusion Injury in Rats. Chin. J. Integr. Med. 15, 54–59. doi:10.1007/s11655-009-0054-4
Wu, L., Qiao, H., Li, Y., and Li, L. (2007). Protective Roles of Puerarin and Danshensu on Acute Ischemic Myocardial Injury in Rats. Phytomedicine 14, 652–658. doi:10.1016/j.phymed.2007.07.060
Wu, M., Liang, S., Ma, L., Han, Y., Zhang, X., and Xu, C. (2014). Effects of Delayed Puerarin Treatment in Long-Term Neurological Outcomes of Focal Ischemic Stroke in Rats. Indian J. Pharmacol. 46, 157–160. doi:10.4103/0253-7613.129305
Wu, Y., Chen, J., and Wang, R. (2019). Puerarin Suppresses TRPV1, Calcitonin Gene-Related Peptide and Substance P to Prevent Paclitaxel-Induced Peripheral Neuropathic Pain in Rats. Neuroreport 30, 288–294. doi:10.1097/WNR.0000000000001199
Wymann, M. P., Zvelebil, M., and Laffargue, M. (2003). Phosphoinositide 3-kinase Signalling-Wwhich Way to Target? Trends Pharmacol. Sci. 24, 366–376. doi:10.1016/S0165-6147(03)00163-9
Xiao, L. Z., Gao, L. J., and Ma, S. C. (2005). Comparative Study on Effects of Puerarin and Granulocyte colony-stimulating Factor in Treating Acute Myocardial Infarction. Zhongguo Zhong Xi Yi Jie He Za Zhi 25 (3), 210–213.
Xiao, L. Z., Huang, Z., Ma, S. C., Zen, Z., Luo, B., Lin, X., et al. (2004). Study on the Effect and Mechanism of Puerarin on the Size of Infarction in Patients with Acute Myocardial Infarction. Zhongguo Zhong Xi Yi Jie He Za Zhi 24 (9), 790–792.
Xiao, Y., Huang, J., Xu, J., Zeng, L., Tian, J., Lou, Y., et al. (2018). Targeted Delivery of Puerarin/glycyrrhetinic Acid-PEG-PBLA Complex Attenuated Liver Ischemia/reperfusion Injury via Modulating Toll-like Receptor 4/nuclear Factor-Κb Pathway. Ther. Deliv. 9 (4), 245–255. doi:10.4155/tde-2017-0106
Xie, Z., Li, Z., Dong, W., Chen, Y., Li, R., Wu, Y., et al. (2020). Metabolic Syndrome and Concomitant Diabetes Mellitus Are Associated with Higher Risk of Cardiovascular Comorbidity in Patients with Primary Glomerular Diseases: A Retrospective Observational Study. Clin. Cardiol. 43, 949–956. doi:10.1002/clc.23388
Xu, H. X., Pan, W., Qian, J. F., Liu, F., Dong, H. Q., and Liu, Q. J. (2019). MicroRNA-21 C-ontributes to the P-uerarin-induced C-ardioprotection via S-uppression of A-poptosis and O-xidative S-tress in a C-ell M-odel of I-schemia/reperfusion I-njury. Mol. Med. Rep. 20 (1), 719–727. doi:10.3892/mmr.2019.10266
Xu, X., Zhang, S., Zhang, L., Yan, W., and Zheng, X. (2005). The Neuroprotection of Puerarin against Cerebral Ischemia Is Associated with the Prevention of Apoptosis in Rats. Planta Med. 71, 585–591. doi:10.1055/s-2005-871261
Xu, X. H., Zheng, X. X., Zhou, Q., and Li, H. (2007). Inhibition of Excitatory Amino Acid Efflux Contributes to Protective Effects of Puerarin against Cerebral Ischemia in Rats. Biomed. Environ. Sci. 20 (4), 336–342.
Xue, Q., Liu, Y., He, R., Yang, S., Tong, J., Li, X., et al. (2016). Lyophilized Powder of Catalpol and Puerarin Protects Neurovascular Unit from Stroke. Int. J. Biol. Sci. 12, 367–380. doi:10.7150/ijbs.14059
Yan, L. P., Zhuang, Y. L., Chan, S. W., Chen, S. L., and Shi, G. G. (2009). Analysis of the Mechanisms Underlying the Endothelium-dependent Antivasoconstriction of Puerarin in Rat Aorta. Naunyn Schmiedebergs Arch. Pharmacol. 379, 587–597. doi:10.1007/s00210-008-0388-2
Yang, B., Gao, Q., Yao, H., and Xia, Q. (2008). Mitochondrial Mechanism of Cardioprotective Effect of Puerarin against H202-Stress in Rats. Zhongguo ying yong sheng li xue za zhi 24 (4), 399–404.
Yang, J. K., Wang, Y. Y., Liu, C., Shi, T. T., Lu, J., Cao, X., et al. (2017). Urine Proteome Specific for Eye Damage Can Predict Kidney Damage in Patients with Type 2 Diabetes: A Case-Control and a 5.3-Year Prospective Cohort Study. Diabetes care 40, 253–260. doi:10.2337/dc16-1529
Yang, M., Luo, Y., Liu, T., Zhong, X., Yan, J., Huang, Q., et al. (2018b). The Effect of Puerarin on Carotid Intima-media Thickness in Patients with Active Rheumatoid Arthritis: ARandomized Controlled Trial. Clin. Ther. 40, 1752–e1. doi:10.1016/j.clinthera.2018.08.014
Yang, W., Lu, J., Weng, J., Jia, W., Ji, L., Xiao, J., et al. (2010a). Prevalence of Diabetes Among Men and Women in China. N. Engl. J. Med. 362, 1090–1101. doi:10.1056/nejmoa0908292
Yang, X., Hu, W., Zhang, Q., Wang, Y., and Sun, L. (2010b). Puerarin Inhibits C-Reactive Protein Expression via Suppression of Nuclear Factor kappaB Activation in Lipopolysaccharide-Induced Peripheral Blood Mononuclear Cells of Patients with Stable Angina Pectoris. Basic Clin. Pharmacol. Toxicol. 107, 637–642. doi:10.1111/j.1742-7843.2010.00548.x
Yang, X., Yang, Y., Zhou, S., Gong, X., Dai, Q., Zhang, P., et al. (2018a). Puerarin Stimulates Osteogenic Differentiation and Bone Formation through the ERK1/2 and P38-MAPK Signaling Pathways. Curr. Mol. Med. 17, 488–496. doi:10.2174/1566524018666171219101142
Ye, X. Y., Song, H., and Lu, C. Z. (2008). Effect of Puerarin Injection on the mRNA Expressions of AT1 and ACE2 in Spontaneous Hypertension Rats. Zhongguo Zhong Xi Yi Jie He Za Zhi 28 (9), 824–827.
Yin, M. S., Zhang, Y. C., Xu, S. H., Liu, J. J., Sun, X. H., Liang, C., et al. (2019). Puerarin Prevents Diabetic Cardiomyopathy In Vivo and In Vitro by Inhibition of Inflammation. J. Asian Nat. Prod. Res. 21, 476–493. doi:10.1080/10286020.2017.1405941
Yu, M. H., Lin, M. C., Huang, C. N., Chan, K. C., and Wang, C. J. (2018). Acarbose Inhibits the Proliferation and Migration of Vascular Smooth Muscle Cells via Targeting Ras Signaling. Vascul Pharmacol. 103-105, 8–15. doi:10.1016/j.vph.2018.02.001
Yuan, Y., Zong, J., Zhou, H., Bian, Z. Y., Deng, W., Dai, J., et al. (2014). Puerarin Attenuates Pressure Overload-Induced Cardiac Hypertrophy. J. Cardiol. 63, 73–81. doi:10.1016/j.jjcc.2013.06.008
Zhang, G. Q., Hao, X. M., Dai, D. Z., Fu, Y., Zhou, P. A., and Wu, C. H. (2003). Puerarin Blocks Na+ Current in Rat Ventricular Myocytes. Acta Pharmacol. Sin 24 (12), 1212–1216.
Zhang, H., Zhang, L., Zhang, Q., Yang, X., Yu, J., Shun, S., et al. (2011a). Puerarin: a Novel Antagonist to Inward Rectifier Potassium Channel (Ik1). Mol. Cel Biochem 352, 117–123. doi:10.1007/s11010-011-0746-0
Zhang, J., Guo, W., Tian, B., Sun, M., Li, H., Zhou, L., et al. (2015). Puerarin Attenuates Cognitive Dysfunction and Oxidative Stress in Vascular Dementia Rats Induced by Chronic Ischemia. Int. J. Clin. Exp. Pathol. 8 (5), 4695–4704.
Zhang, L. (2019). Pharmacokinetics and Drug Delivery Systems for Puerarin, a Bioactive Flavone from Traditional Chinese Medicine. Drug Deliv. 26, 860–869. doi:10.1080/10717544.2019.1660732
Zhang, N. B., Huang, Z. G., Cui, W. D., and Ding, B. P. (2011b). Effects of Puerarin on Expression of Cardiac Smad3 and Smad7 mRNA in Spontaneously Hypertensive Rat. J. Ethnopharmacol 138, 737–740. doi:10.1016/j.jep.2011.10.013
Zhang, S., Chen, L., Zhou, Z., Fan, W., and Liu, S. (2019a). Effects of Puerarin on Clinical Parameters, Vascular Endothelial Function, and Inflammatory Factors in Patients with Coronary Artery Disease. Med. Sci. Monit. 25, 402–408. doi:10.12659/MSM.911108
Zhang, S., Chen, S., Shen, Y., Yang, D., Liu, X., Sun-Chi, A. C., et al. (2006). Puerarin Induces Angiogenesis in Myocardium of Rat with Myocardial Infarction. Biol. Pharm. Bull. 29, 945–950. doi:10.1248/bpb.29.945
Zhang, S. Y., Chen, G., Wei, P. F., Huang, X. S., Dai, Y., Shen, Y. J., et al. (2008). The Effect of Puerarin on Serum Nitric Oxide Concentration and Myocardial eNOS Expression in Rats with Myocardial Infarction. J. Asian Nat. Prod. Res. 10, 373–381. doi:10.1080/10286020801892250
Zhang, W., Liu, C. Q., Wang, P. W., Sun, S. Y., Su, W. J., Zhang, H. J., et al. (2010). Puerarin Improves Insulin Resistance and Modulates Adipokine Expression in Rats Fed a High-Fat Diet. Eur. J. Pharmacol. 649, 398–402. doi:10.1016/j.ejphar.2010.09.054
Zhang, X., Liu, Y., and Han, Q. (2016). Puerarin Attenuates Cardiac Hypertrophy Partly through Increasing Mir-15b/195 Expression and Suppressing Non-canonical Transforming Growth Factor Beta (Tgfβ) Signal Pathway. Med. Sci. Monit. 22, 1516–1523. doi:10.12659/msm.895877
Zhang, X., Zhao, F., Zhang, X., and Sheng, J. (2011c). Puerarin Induced Apoptosis of Pulmonary Artery Smooth Muscle Cell by Mitochondrial Pathway. Zhongguo Zhong yao za zhi 36 (16), 2255–2258.
Zhang, Y., Yang, X., Ge, X., and Zhang, F. (2019b). Puerarin Attenuates Neurological Deficits via Bcl-2/Bax/cleaved Caspase-3 and Sirt3/SOD2 Apoptotic Pathways in Subarachnoid Hemorrhage Mice. Biomed. Pharmacother. 109, 726–733. doi:10.1016/j.biopha.2018.10.161
Zhang, Z., Lam, T. N., and Zuo, Z. (2013). Radix Puerariae: an Overview of its Chemistry, Pharmacology, Pharmacokinetics, and Clinical Use. J. Clin. Pharmacol. 53, 787–811. doi:10.1002/jcph.96
Zhao, G. J., Hou, N., Cai, S. A., Liu, X. W., Li, A. Q., Cheng, C. F., et al. (2018). Contributions of Nrf2 to Puerarin Prevention of Cardiac Hypertrophy and its Metabolic Enzymes Expression in Rats. J. Pharmacol. Exp. Ther. 366, 458–469. doi:10.1124/jpet.118.248369
Zhao, L. X., Liu, A. C., Yu, S. W., Wang, Z. X., Lin, X. Q., Zhai, G. X., et al. (2013). The Permeability of Puerarin Loaded Poly(butylcyanoacrylate) Nanoparticles Coated with Polysorbate 80 on the Blood-Brain Barrier and its Protective Effect against Cerebral Ischemia/reperfusion Injury. Biol. Pharm. Bull. 36 (8), 1263–1270. doi:10.1248/bpb.b12-00769
Zhao, T., Han, J., Chen, Y., Wan, H., and Bie, X. (2007). The Mechanism of 3-methoxy Puerarin on Decreasing the Cerebral Ischemia-Reperfusion Injury in Rats. Asia Pac. J. Clin. Nutr. 16 Suppl 1 (Suppl. 1), 302–304.
Zhao, Y., Zhao, J., Zhang, X., Cheng, Y., Luo, D., Lee, S. M., et al. (2020). Botanical Drug Puerarin Promotes Neuronal Survival and Neurite Outgrowth against MPTP/MPP+-Induced Toxicity via Progesterone Receptor Signaling. Oxid Med. Cel Longev 2020, 7635291. doi:10.1155/2020/7635291
Zheng, Q. H., Li, X. L., Mei, Z. G., Xiong, L., Mei, Q. X., Wang, J. F., et al. (2017). Efficacy and Safety of Puerarin Injection in Curing Acute Ischemic Stroke: A Meta-Analysis of Randomized Controlled Trials. Medicine (Baltimore) 96, e5803. doi:10.1097/MD.0000000000005803
Zhou, Y. X., Zhang, H., and Peng, C. (2014). Puerarin: a Review of Pharmacological Effects. Phytother Res. 28, 961–975. doi:10.1002/ptr.5083
Zhu, J., Wang, X., Shang, Y., Xie, X., Zhang, F., Chen, J., et al. (2008). Puerarin Reduces Endothelial Progenitor Cells Senescence through Augmentation of Telomerase Activity. Vascul Pharmacol. 49, 106–110. doi:10.1016/j.vph.2008.07.002
Keywords: puerarin, cardiovascular diseases, mechanisms, targets, clinical trials
Citation: Zhou Y-X, Zhang H and Peng C (2021) Effects of Puerarin on the Prevention and Treatment of Cardiovascular Diseases. Front. Pharmacol. 12:771793. doi: 10.3389/fphar.2021.771793
Received: 07 September 2021; Accepted: 22 November 2021;
Published: 07 December 2021.
Edited by:
Donato Cappetta, University of Campania Luigi Vanvitelli, ItalyReviewed by:
Junping Zhang, First Teaching Hospital of Tianjin University of Traditional Chinese Medicine, ChinaBaochen Zhu, Beijing University of Chinese Medicine, China
Copyright © 2021 Zhou, Zhang and Peng. This is an open-access article distributed under the terms of the Creative Commons Attribution License (CC BY). The use, distribution or reproduction in other forums is permitted, provided the original author(s) and the copyright owner(s) are credited and that the original publication in this journal is cited, in accordance with accepted academic practice. No use, distribution or reproduction is permitted which does not comply with these terms.
*Correspondence: Cheng Peng, pengcchengd@163.com