Exogenous melatonin treatment affects ascorbic acid metabolism in postharvest ‘Jinyan’ kiwifruit
- 1College of Agronomy, Jiangxi Agricultural University, Nanchang, China
- 2Jiangxi Key Laboratory for Postharvest Technology and Non-destructive Testing of Fruits & Vegetables, Nanchang, China
Ascorbic acid (AsA) is an important nutritious substance in fruits, and it also can maintain the biological activity of fruits during storage. This research investigated the effect of exogenous melatonin (MT) on AsA metabolism in postharvest kiwifruit. Our results indicated that exogenous MT delayed the decrease of fruit firmness and titratable acid (TA), inhibited the increase of soluble solids content (SSC), reduced the respiration rate and ethylene production, and maintained a higher AsA content in kiwifruit during storage. The high expression of L-galactose pathway key genes in the early storage and regeneration genes in the later storage maintained the AsA content in postharvest kiwifruit. MT treatment enhanced the expression levels of AsA biosynthesis (AcGME2, AcGalDH, and AcGalLDH) and regeneration (AcGR, AcDHAR, and AcMDHAR1) genes. Meanwhile, the expression of the degradation gene AcAO was inhibited in MT-treated kiwifruits.
Introduction
Kiwifruit (Actinidia spp.) is famous for its high content of ascorbic acid (AsA), which is considered to be one of its key nutritional characteristics. During the postharvest process, AsA content decreases with the ripening and senescence of kiwifruit. As a rich small molecule substance, AsA is closely related to the stress resistance, antioxidant capacity, cell division and photosynthesis of plants. Due to gene mutations encoding enzymes in AsA biosynthesis, humans cannot synthesize AsA by themselves, so they obtain AsA from foods (1).
In higher plants, the L-galactose pathway is a critical metabolic pathway for AsA biosynthesis. At present, the genes involved in this pathway had been identified, cloned and verified from different kiwifruit varieties (2, 3). GDP-D-mannose 3′, 5′-epimerase (GME) catalyzes the conversion of GDP-D-mannose to GDP-L-galactose, and GME transcripts were associated with the peak of AsA content in four genotypes of kiwifruit (4). After GME expression was silenced, the level of AsA in tomato decreased significantly (5). GDP-L-galactose phosphorylase (GGP) can catalyze the conversion of GDP-L-galactose to L-galactose-1-phosphate. The AsA content of kiwifruit was significantly higher in Actinidia eriantha than Actinidia rufa, probably because the GGP transcripts in Actinidia eriantha were significantly higher than Actinidia rufa (6). L-galactose dehydrogenase (GalDH) catalyzes the oxidation of L-galactose to L-galactose-1,4-lactone, and L-galactose 1,4-lactone dehydrogenase (GalLDH) is the last enzyme in AsA synthesis. GalLDH catalyzes the conversion of L-galactose 1,4-lactone to AsA and plays a critical role in the accumulation of AsA in plants. In plants, the activity and transcription level of GalLDH were positively correlated with AsA content (7). The accumulation of AsA was closely related to GalLDH activity during fruit development of kiwifruit, and the change trend of GalLDH activity was similar to that of AsA accumulation in kiwifruit (8). Meanwhile, the L-gulose pathway, D-galacturonate pathway and myo-inositol pathway are also involved in AsA synthesis in plants (9–11).
AsA can be regenerated by the AsA-glutathione cycle. Ascorbate peroxidase (APX) and ascorbate oxidase (AO) are used as electron donors to reduce hydrogen peroxide (H2O2) and superoxide anion (O2–), respectively, resulting in production of monodehydroascorbate (MDHA). Monodehydroascorbate reductase (MDHAR) and dehydroascorbate reductase (DHAR) can convert MDHA to AsA (9). The reaction of AsA catalyzed by AO was delayed in carrots treated with a pulsed electric field (PEF); therefore, high-energy PEF treatment maintained the stability of AsA (12). In transgenic plants, AsA content was increased by DHAR overexpression, indicating that AsA content can be increased by enhancing the AsA cycle (13). TaMDHAR is a gene encoding MDHAR, and knock out of TaMDHAR in wheat resulted in decreased MDHAR activity and lower AsA content (14).
In plants, melatonin (MT) was first discovered in 1995 (15). Since then, the function and role of MT in plants have been widely studied. For postharvest fruit preservation, many studies have reported that MT has an effect on delaying senescence and is expected to become a new preservative. MT treatment inhibited the expression of the ethylene synthesis genes ACS and ACO in pear fruits and reduced the ethylene production rate, thereby delayed fruit senescence (16). As a signaling molecule, MT can enhance enzymatic and non-enzymatic antioxidant systems, which can scavenge reactive oxygen species (ROS) in fruits during storage. The non-enzymatic antioxidants included glutathione (GSH), AsA, polyphenols and so on. The AsA content of sweet cherries was increased by MT treatment (17). At present, the effects of exogenous MT treatment on postharvest kiwifruit had been reported. MT could improve the antioxidant capacity of kiwifruit then alleviated chilling injury of the fruit (18). MT also inhibited the respiration rate and ethylene production of kiwifruit and delayed fruit senescence, meanwhile the content of ethanol and acetaldehyde were reduced in the later storage of fruit (19). In addition, exogenous MT treatment significantly increased the AsA content of kiwifruit during storage, but the specific mechanism is not very clear currently. Therefore, the effects of exogenous MT on the AsA content, AsA synthesis and transcription level of cyclic transformation genes in kiwifruit during postharvest storage were studied, which will provide a basis for the regulation of storage quality in postharvest kiwifruit.
Materials and methods
Plant material and treatment
‘Jinyan’ kiwifruit (Actinidia chinensis Planch) were harvested from Kiwifruit Germplasm Resource Nursery, Fengxin County, Jiangxi Province (28.68° N, 115.31° E) and transported to Jiangxi Key Laboratory for Postharvest Technology and Non-destructive Testing of Fruits & Vegetables on 27 October 2021. The orchard is a commercial orchard belonging to the subtropical humid climate, sandy red soil, pH 5.5–6.5. ‘Jinyan’ kiwifruit are 6-year-old vines which grafted onto rootstock of A. arguta (Siebold et Zucc.) Planch. ex Miq. The soluble solids content (SSC) of kiwifruit were about 8% at harvest. After 1 day (d) of sweating, fruits with moderate size, consistent maturity, no infection by pests and mechanical damage were selected for further experiments.
According to our previous experiments (data not published), the fruits were dipped in 0.1 mmol/L MT for 10 min, and distilled water as a control. Both the control and MT treatments were repeated three times, with 200 fruits per replicate. Then, the fruits were dried naturally and placed at 20 ± 1°C and 85–90% relative humidity. During kiwifruit storage, 15 fruits per treatment were selected randomly to determine fruit firmness, SSC, respiration rate and ethylene production rate every 2 days. The remaining samples were frozen and placed at −80°C for further measurement.
Determination of firmness and soluble solids content
After removing the peel, two symmetrical points on the equatorial region of each fruit were randomly selected to measure fruit firmness by a TMS-Touch texture analyzer, the probe diameter was 8 mm, penetrated the fruits at a speed of 5 mm s–1 to a depth of 10 mm, 15 fruits were measured for each treatment, and the results were expressed in newton (N). SSC was determined by PAL-1 digital refractometer and repeated three times per treatment; the results were expressed as %.
Determination of respiration rate and ethylene production
The respiration rate was measured by a GXH-3051H infrared carbon dioxide fruit and vegetable respiration tester (Jun-Fang-Li-Hua Technology-Research Institute, Beijing, China) with 1,040 μL/L CO2 as the calibration standard. The carrier gas was CO2-removed air, the gas flow rate was 0.5 L/min, and the respiration rate was calculated as mg CO2⋅kg–1⋅h–1 of five fruits per replicate for a total of three replicates.
For ethylene production measurement, nine fruits per replicate were placed in a 0.8 L closed gas collector for 2 h, and 1 mL of top air was extracted from each container and injected into a Gas Chromatography (GC-2014, Shimadzu, Japan) through a rubber diaphragm and column (DANI, Italy). Detector temperature was 200°C, column temperature was 130°C, current velocity of N2 was 5.0 mL min–1. The ethylene production was calculated as ng⋅kg–1⋅s–1.
Determination of titratable acid and ascorbic acid
For TA analysis, 10 g kiwifruit was grinded to ground and diluted with 20 mL distilled water in a triangular bottle, bathed in 80°C water for 30 min. After cooling and centrifugation, supernatant was used by titration with 0.1 M sodium hydroxide and TA was expressed in % (20). The AsA content was measured as previously reported with a slight modification (21), 3 g of fruits tissue was grinded with 10 mL 0.05 mol L–1 oxalic acid-EDTA, the centrifuged at 9,000 × g for 40 min and the supernatant was collected. Then, 2 mL supernatant was mixed with 3 mL oxalic acid-EDTA, 0.5 mL of metaphosphoric acid-acetic acid, and 1 mL of 5% H2SO4. The mixture was watered at 80°C for 15 min then cooled to room temperature, and the absorbance was measured at 760 nm using AsA as standard. The AsA content was showed as mg⋅100 g–1, and the experiment was repeated three times.
Real-time quantitative PCR
RNA extraction was performed using an RNA extraction kit (TIANGEN). The extracted RNA integrity was tested by 1% agarose gel electrophoresis, and the RNA concentration was determined by evaluating the A260/A280 ratio with a UV spectrophotometer. cDNA was obtained using a YEASEN reverse transcription kit (YEASEN Biotech Co., Ltd.) strictly following the manufacturer’s instructions. Gene expression analysis was performed using a CFX96TM Real-Time System (Bio-Rad, USA) and a Takara kit (TB Green® Premix Ex TaqTM). The total qRT-PCR reaction system was 10 μl, which included 0.3 μl positive and negative gene-specific primers respectively, 5.0 μl TB Green and 3.4 μl ddH2O and 1.0 μl cDNA. The relative expression of the gene was normalized to actin (EF063572) and expressed as 2–ΔΔCt according to previous methods (22, 23), and the reaction was repeated three times.
Statistical analysis
The experiment was conducted using a completely randomized design. Excel 2016 was used for data statistics, figures were created by GraphPad Prism 8.0 software, and SPSS 26.0 statistical software was used to analyze the significant differences between the control and MT treatments by two-way ANOVA at p < 0.05 (*) or p < 0.01 (**).
Results
Effect of melatonin treatment on firmness and soluble solids content
The fruit firmness of the two groups decreased continuously under normal temperature storage. MT treatment significantly delayed the decrease in fruit firmness, and there were extremely significant differences between MT treatment and control fruits at 2–8 days of storage (Figure 1A). Meanwhile, the SSC increased in the two groups at all times, and MT delayed the increase of SSC (Figure 1B).
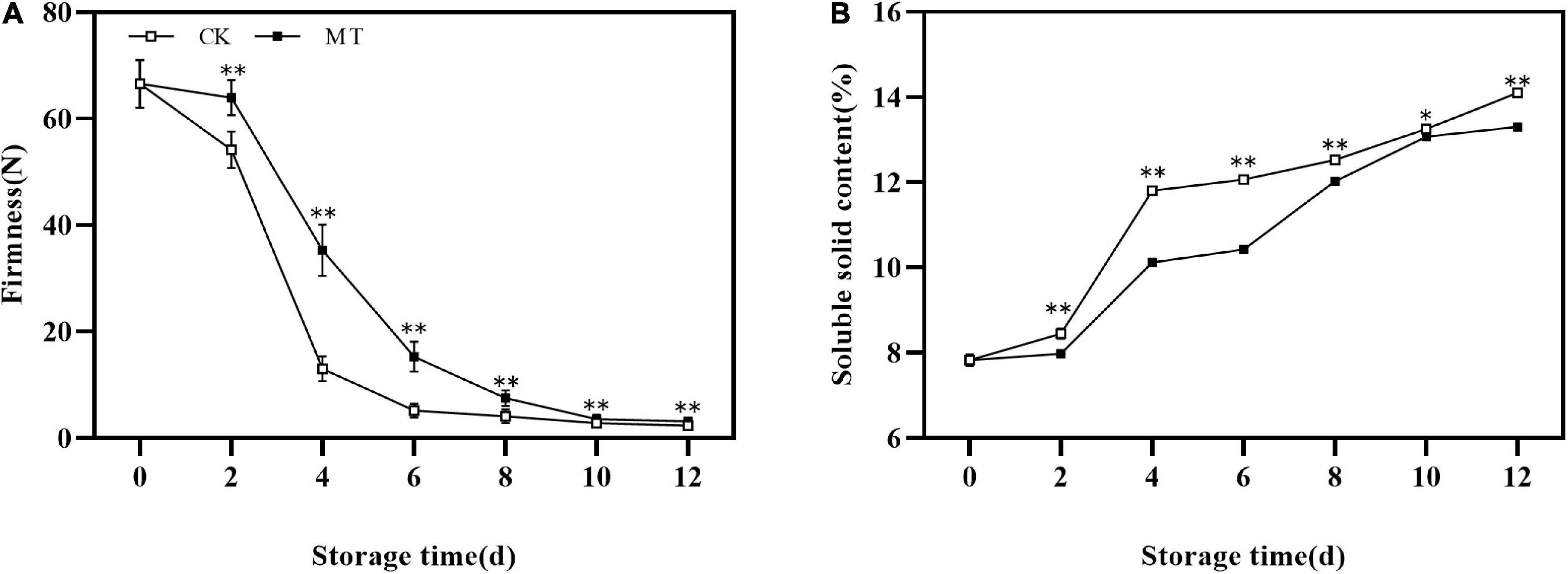
Figure 1. Effects of melatonin (MT) treatment on the firmness (A) and soluble solids content (SSC) (B) of ‘Jinyan’ kiwifruit. Data are presented as the means ± SE (n = 3). Asterisks indicate significant differences between the control and MT treatments (*P < 0.05; **P < 0.01).
Effects of melatonin treatment on respiratory rate and ethylene production
During storage, the changes in the respiration rate and ethylene production of fruits were similar, first increased and then decreased with obvious peaks. MT delayed and inhibited the peak of respiration rate and ethylene production, the peak values were only 66.65 and 52.24% of those in the control group, respectively (Figure 2).
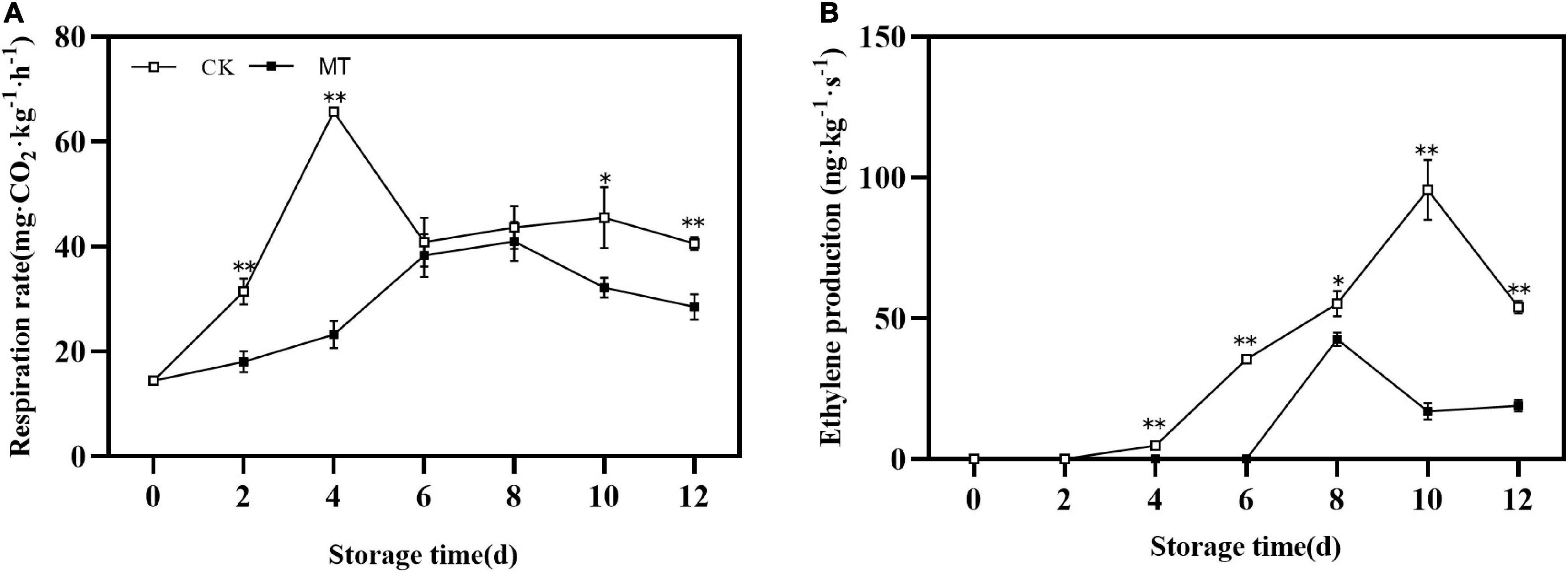
Figure 2. Effects of melatonin (MT) treatment on respiration rate (A) and ethylene production (B) of ‘Jinyan’ kiwifruit. Data are presented as the means ± SE (n = 3). Asterisks indicate significant differences between the control and MT treatments (*P < 0.05; **P < 0.01).
Effects of melatonin treatment on titratable acid and ascorbic acid
During the whole storage process, the TA content of kiwifruit showed a decreasing trend. MT treatment delayed the decrease in TA of fruits (Figure 3A). The AsA content in the fruits first increased and then decreased, with the peak of MT treatment group appeared later than that of the control group and the peak value of the MT treatment group was 1.17 times that of the control group. At the same time, the AsA content of the treatment group was significantly higher than that of the control group during storage except at 2 days (Figure 3B).
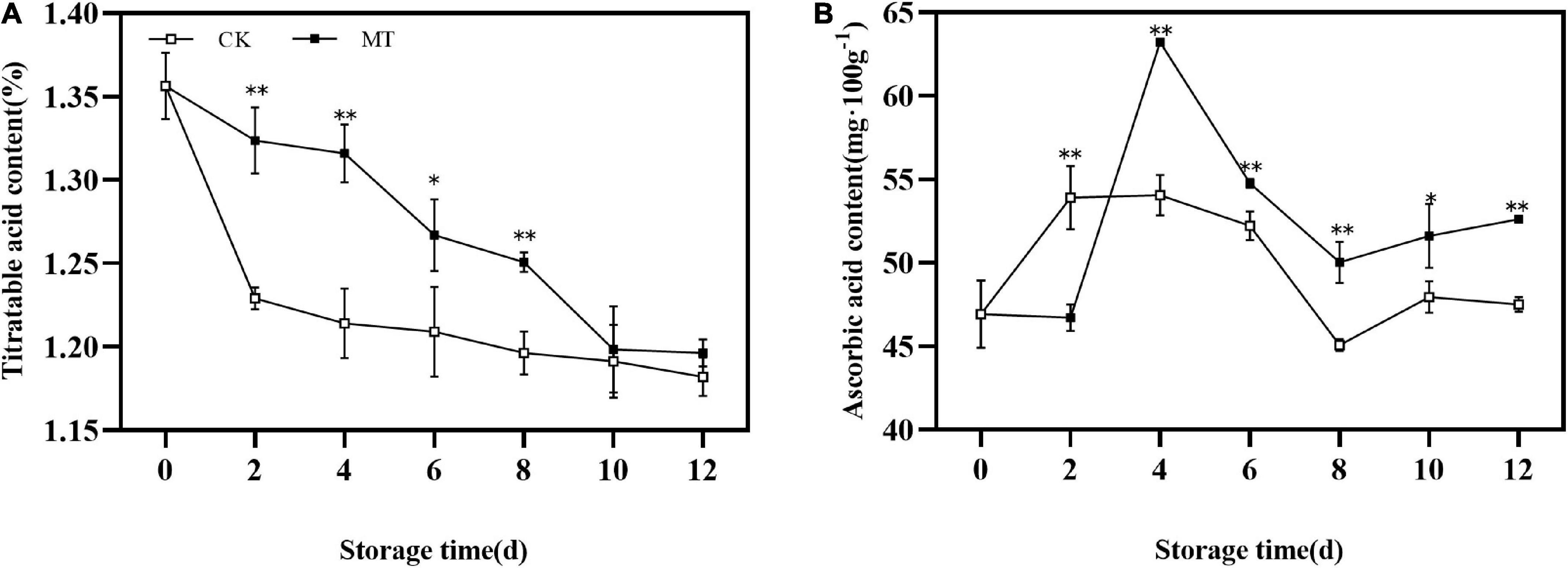
Figure 3. Effects of melatonin (MT) treatment on the titratable acid (TA) (A) and ascorbic acid content (B) of ‘Jinyan’ kiwifruit. Data represent the means ± SE (n = 3). Asterisks indicate significant differences between the control and MT treatments (*P < 0.05; **P < 0.01).
Effect of melatonin treatment on gene expression of ascorbic acid biosynthesis
The genes involved in the AsA synthesis process showed different trends. The expression levels of AcGME2, AcGalDH, and AcGalLDH in the MT treatment group were significantly higher than those in the control group, while the expression levels of AcPMI2, AcPMM, AcGMP1, AcGME1, and AcGGP1 were also higher in the MT treatment group at some time points. There was no significant difference in AcPGI4 or AcGMP4 expression between the two groups, but the expression levels of AcGPP1, AcGPP2, AcMIOX1, and AcGalUR, genes in the inositol pathway and D-galactose aldehyde pathway, were lower in the MT treatment group than in the control group (Figure 4).
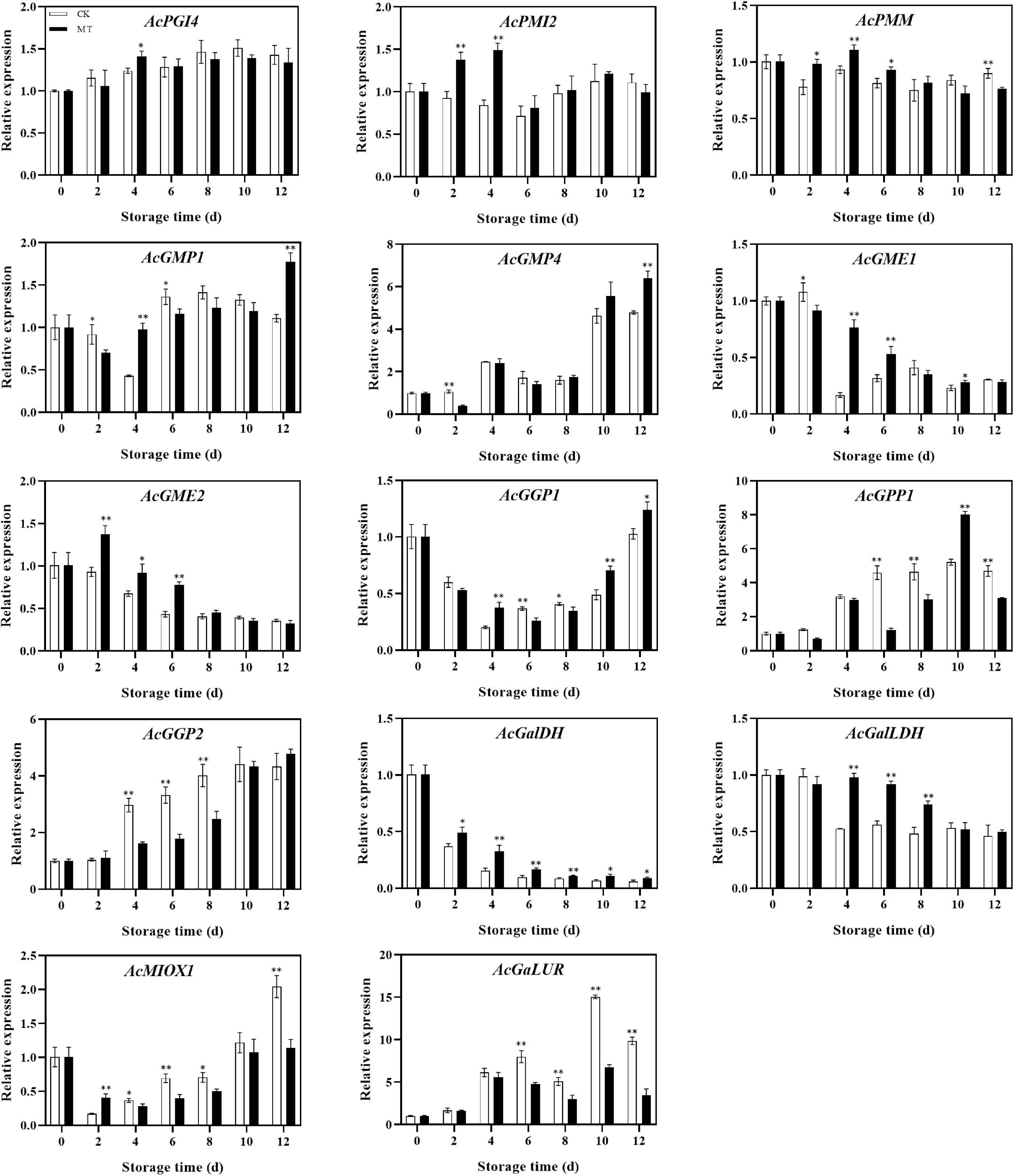
Figure 4. Effects of melatonin (MT) treatment on expression levels of genes involved in ascorbic acid biosynthesis of ‘Jinyan’ kiwifruit. Data represent the means ± SE (n = 3). Asterisks indicate significant differences between the control and MT treatments (*P < 0.05; **P < 0.01).
Effect of melatonin treatment on gene expression of ascorbic acid recycling and degradation
MT treatment upregulated the expression of recycling genes, including AcGR, AcMDHAR1, AcMDHAR2, and AcDHAR. The expression levels of AcMDHAR1 and AcDHAR were significantly higher in the MT treatment group than in the control group except at 2 days of storage, and the expression levels gradually increased in both groups. In regards to degradation genes, MT treatment upregulated AcAPX1 expression but downregulated AcAO expression. The expression levels of AcAO in the MT treatment group were only 17.43 and 58.24% of those of the control group at 4 and 6 days of storage, respectively (Figure 5).
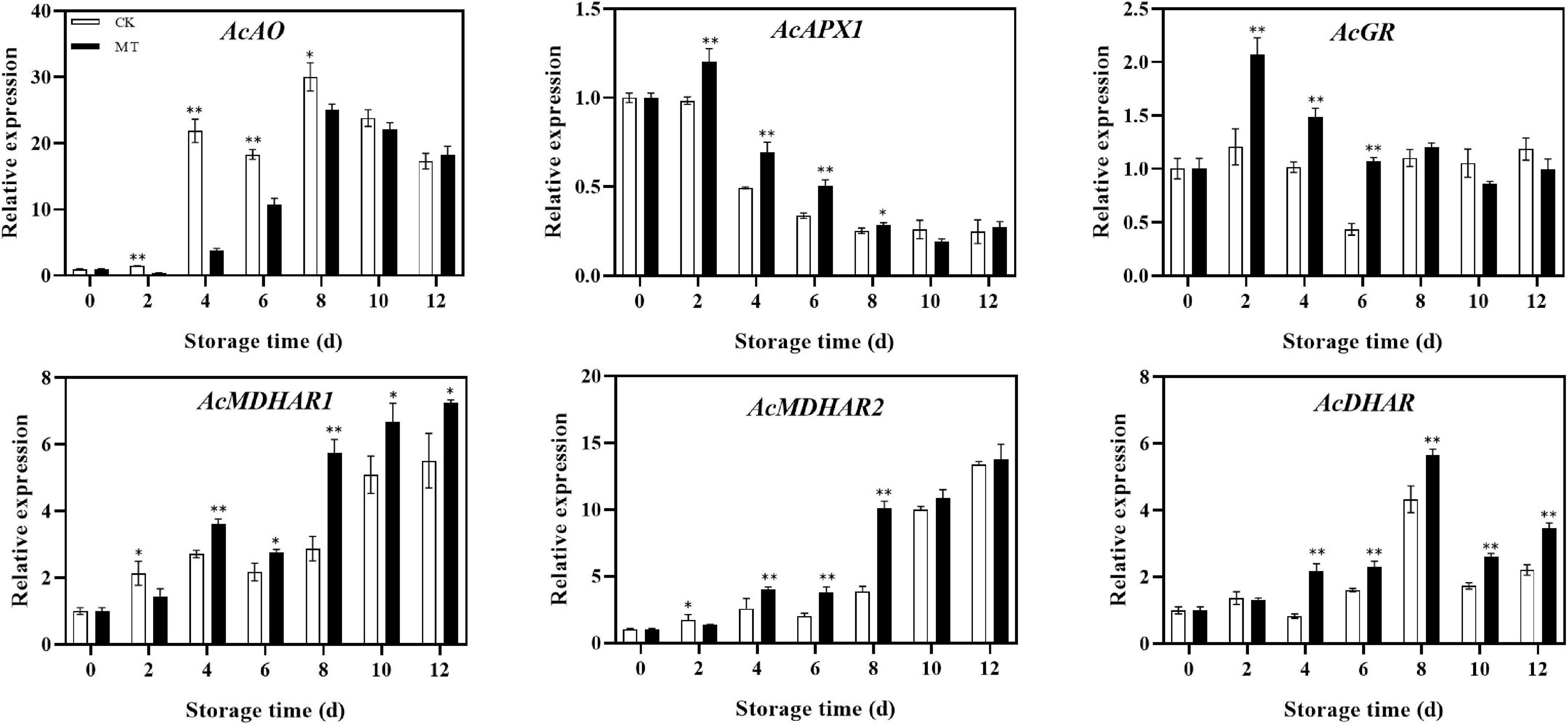
Figure 5. Effects of melatonin (MT) treatment on expression of genes involved in ascorbic acid recycling and degradation of ‘Jinyan’ kiwifruit. Data represent the means ± SE (n = 3). Asterisks indicate significant differences between the control and MT treatments (*P < 0.05; **P < 0.01).
Discussion
The application of MT on peach and kiwifruit could delay the decline in fruit firmness and SSC (19, 24). The main reason was that MT inhibited the expression of ethylene biosynthesis genes, then the respiration rate and ethylene production were suppressed in fruit (19). Similar results were obtained in this study. As an important antioxidant in plants, AsA participates in the defense of plants against oxidative stress and affects the stability of the cell membrane. MT treatment can increase antioxidant capacity and the AsA content in postharvest fruits, which was confirmed in kiwifruit and pear (18, 25). In this study, MT treatment increased the AsA content of kiwifruit during postharvest storage and improved the antioxidant capacity of kiwifruit, and maintain the stability of membrane lipids, thereby delaying fruit senescence.
AsA accumulation was regulated by L-galactose pathway-related genes. GME and GGP coexpression significantly increased the AsA concentration in Arabidopsis (26). The transcriptional trends of GME and GGP were similar during kiwifruit (Actinidia eriantha) development and had a significant correlation with AsA levels (8). However, the expression level of GGP first decreased and then increased in this experiment. The transcriptional expression level was the lowest when the AsA content reached the peak, which may be related to the feedback regulation mechanism of AsA. Laing et al. (27) reported the feedback regulation mechanism of AsA in Arabidopsis thaliana. When the AsA concentration was high, uORFs began to express and inhibit the translation of GGP, and GGP translation was normal at lower AsA contents. Meanwhile, the expression of GPP was negatively correlated with AsA content. This is similar to a study in peach and kiwifruit (28, 29). The expression of GPP in the L-galactose pathway is not consistent with AsA accumulation. The correlation between GPP and AsA content was also different in various varieties of kiwifruit. The expression level of GPP was basically consistent with the AsA content in kiwifruit (Actinidia deliciosa), while the correlation between GPP expression and AsA content was low in kiwifruit (Actinidia eriantha) (6, 8). In this experiment, MT treatment enhanced the expression levels of AcGME2, AcGaLDH, and AcGalLDH, which could be one of reasons for higher AsA content in MT-treated fruits. However, the finding that some synthetic genes were negatively correlated with AsA content needs to be further explored.
In this experiment, the expression levels of AcMIOX and AcGaLUR, two key genes in the D-galacturonate pathway and myo-inositol pathway, increased during the late storage period and were inhibited by MT treatment at most stages of storage. GaLUR expression was significantly higher in the 1-Methylcyclopropene (1-MCP) treatment group than in the control group in the regulation of the AsA metabolic pathway by 1-MCP (29). However, D-galacturonic acid was found to be released during pectin hydrolysis in MT-treated strawberry. MT could maintain fruit firmness and cell integrity, resulting in decreased release of D-galacturonic acid from the cell wall and ultimately affecting AsA synthesis in strawberry (30), which may be the result of lower expression of AcGaLUR in kiwifruit treated with MT. The expression level of MIOX in kiwifruit (Actinidia eriantha) was not significantly correlated with AsA content, indicating that the inositol pathway may had little effect on AsA accumulation in kiwifruit (Actinidia eriantha) (8). The specific synthesis of the myo-inositol pathway in kiwifruit still needs further study.
Compared to AsA biosynthesis, the recycling and degradation pathway of AsA may be more important in maintaining AsA content in postharvest fruits. The expression levels of AcDHAR and AcMDHAR1 were upregulated during storage, and MT treatment significantly promoted their expression, which may be the reason for the higher level of AsA content in MT-treated kiwifruits at the later stage of storage. Exogenous MT has also been reported to improve the enzyme activities of DHAR and MDHAR in kiwifruit leaves, thereby delaying their senescence (31). The previous studies showed that the AsA content was increased by 1.4- and 1.9-fold in transgenic Arabidopsis and tomato with DHAR overexpression, respectively, and overexpression of the MDHAR gene increased the AsA content by nearly twofold in transgenic tobacco (32–34). For the key degradation gene of AsA, AO, we obtained a similar result to that of a previous report (29). MT treatment downregulated the expression level of AcAO, and the effect was remarkable in the middle storage period. Compared with AsA biosynthesis genes, the expression level of AcAO gene had a higher fold difference. Therefore, the AsA degradation pathway may play a more important role than the AsA biosynthesis pathway on maintaining the AsA content of postharvest fruits. At the same time, the increase in AcAO gene expression was the reason for the gradual decrease in AsA during kiwifruit storage. In this experiment, the expression of AcAPX1 and AcGR was stimulated by MT treatment. MT inhibited the accumulation of O2–, H2O2, and malondialdehyde (MDA) and maintained relative membrane permeability by increasing the activities of superoxide dismutase (SOD), catalase (CAT), APX, glutathione reductase (GR), and lipoxygenase (LOX) (19, 21). APX and GR can scavenge reactive oxygen species in fruits, thereby maintaining the stability of the fruit cell membrane. Exogenous MT could increase the content of endogenous MT in fruits (35), and endogenous MT, as a free radical scavenger, also plays a positive role in the antioxidant capacity of fruits, which may be a major reason for the function of MT in delaying fruit senescence during storage. At the same time, whether there was a positive correlation between endogenous MT and AsA content in fruit remains to be further investigated.
Conclusion
In summary, AsA content in fruits are regulated by multiple pathways and genes. The high expression level of key genes in the L-galactose pathway at the early stage and circulating transformation genes at the later stage maintained the AsA content in postharvest kiwifruits. Meanwhile, MT treatment promoted the expression level of biosynthesis and regeneration genes. It inhibited the expression level of degradation genes. Therefore, MT treatment maintained a higher AsA content and delayed postharvest fruit senescence during kiwifruit storage. However, the synergistic effect of different pathway genes on AsA metabolism requires further study.
Data availability statement
The original contributions presented in this study are included in the article/Supplementary material, further inquiries can be directed to the corresponding author.
Author contributions
ZL: investigation, methodology, formal analysis, data curation, and writing—original draft. JZh: investigation and methodology. MX: conceptualization and validation. JZe: formal analysis and data curation. JC: supervision and project administration. MC: conceptualization, writing—review and editing, project administration, and funding acquisition. All authors contributed to the article and approved the submitted version.
Funding
This work was supported by the National Natural Science Foundation of China (32160399) and Jiangxi Province Key Research and Development Program (20192ACB60002).
Conflict of interest
The authors declare that the research was conducted in the absence of any commercial or financial relationships that could be construed as a potential conflict of interest.
Publisher’s note
All claims expressed in this article are solely those of the authors and do not necessarily represent those of their affiliated organizations, or those of the publisher, the editors and the reviewers. Any product that may be evaluated in this article, or claim that may be made by its manufacturer, is not guaranteed or endorsed by the publisher.
Supplementary material
The Supplementary Material for this article can be found online at: https://www.frontiersin.org/articles/10.3389/fnut.2022.1081476/full#supplementary-material
References
1. Li Y, Schellhorn H. New developments and novel therapeutic perspectives for vitamin C. J Nutr. (2007) 137:2171–84. doi: 10.1093/jn/137.10.2171
2. Li J, Li M, Liang D, Cui M, Ma F. Expression patterns and promoter characteristics of the gene encoding Actinidia deliciosa L-galactose-1-phosphate phosphatase involved in the response to light and abiotic stresses. Mol Biol Rep. (2013) 40:1473–85. doi: 10.1007/s11033-012-2190-y
3. Li J, Li M, Liang D, Ma F, Lei Y. Comparison of expression pattern, genomic structure, and promoter analysis of the gene encoding GDP-l-galactose phosphorylase from two Actinidia species. Sci Hortic. (2014) 169:206–13. doi: 10.1016/j.scienta.2014.02.024
4. Li J, Cui M, Li M, Wang X, Liang D, Ma F. Expression pattern and promoter analysis of the gene encoding GDP-d-mannose 3’, 5’-epimerase under abiotic stresses and applications of hormones by kiwifruit. Sci Hortic. (2013) 150:187–94. doi: 10.1016/j.scienta.2012.11.008
5. Gilbert L, Alhagdow M, Nunes-Nesi A, Quemener B, Guillon F, Bouchet B, et al. GDP-D-mannose 3, 5-epimerase (GME) plays a key role at the intersection of ascorbate and non-cellulosic cell-wall biosynthesis in tomato. Plant J. (2009) 60:499–508. doi: 10.1111/j.1365-313X.2009.03972.x
6. Li M, Ma F, Liang D, Li J, Wang Y. Ascorbate biosynthesis during early fruit development is the main reason for its accumulation in kiwi. PLoS One. (2010) 5:e14281. doi: 10.1371/journal.pone.0014281
7. Ishikawa T, Dowdle J, Smirnoff N. Progress in manipulating ascorbic acid biosynthesis and accumulation in plants. Physiol Plant. (2006) 126:343–55. doi: 10.1111/j.1399-3054.2006.00640.x
8. Liao G, Jinyin Chen L, He Y, Li X, Lv Z, Yi S, et al. Three metabolic pathways are responsible for the accumulation and maintenance of high AsA content in kiwifruit (Actinidia eriantha). BMC Genomics. (2021) 22:13. doi: 10.1186/S12864-020-07311-5
9. Davey M, Van Montagu M, Inzé D, Sanmartin M, Kanellis A, Smirnoff N, et al. Plant L-ascorbic acid: chemistry, function, metabolism, bioavailability and effects of processing. J Sci Food Agric. (2000) 80:825–60. (20000515)80: 73.0.CO;2-6 doi: 10.1002/(SICI)1097-0010
10. Valpuesta V, Botella M. Biosynthesis of L-ascorbic acid in plants: new pathways for an old antioxidant. Trends Plant Sci. (2004) 9:573–7. doi: 10.1016/j.tplants.2004.10.002
11. Wolucka B, Van Montagu M. GDP-mannose 3′, 5′-epimerase forms GDP-L-gulose, a putative intermediate for the de novo biosynthesis of vitamin C in plants. J Biol Chem. (2003) 278:47483–90. doi: 10.1074/jbc.M309135200
12. Leong S, Oey I, Burritt D. A novel strategy using pulsed electric fields to modify the thermostability of ascorbic acid oxidase in different carrot cultivars. Food Bioprocess Technol. (2015) 8:811–23. doi: 10.1007/s11947-014-1448-x
13. Chen Z, Young T, Ling J, Chang S, Gallie D. Increasing vitamin C content of plants through enhanced ascorbate recycling. Proc Natl Acad Sci USA. (2003) 100:3525–30. doi: 10.1073/pnas.0635176100
14. Feng H, Wang X, Zhang Q, Fu Y, Feng C, Wang B, et al. Monodehydroascorbate reductase gene, regulated by the wheat PN-2013 miRNA, contributes to adult wheat plant resistance to stripe rust through ROS metabolism. Biochim Biophys Acta Gene Regul Mech. (2014) 1839:1–12. doi: 10.1016/j.bbagrm.2013.11.001
15. Hattori A, Migitaka H, Iigo M, Itoh M, Yamamoto K, Ohtani-Kaneko R, et al. Identification of melatonin in plants and its effects on plasma melatonin levels and binding to melatonin receptors in vertebrates. Biochem Mol Biol Int. (1995) 35:627–34.
16. Zhai R, Liu J, Liu F, Zhao Y, Liu L, Fang C, et al. Melatonin limited ethylene production, softening and reduced physiology disorder in pear (Pyrus communis L.) fruit during senescence. Postharvest Biol Technol. (2018) 139:38–46. doi: 10.1016/j.postharvbio.2018.01.017
17. Wang F, Zhang X, Yang Q, Zhao Q. Exogenous melatonin delays postharvest fruit senescence and maintains the quality of sweet cherries. Food Chem. (2019) 301:125311. doi: 10.1016/j.foodchem.2019.125311
18. Jiao J, Jin M, Liu H, Suo J, Yin X, Zhu Q, et al. Application of melatonin in kiwifruit (Actinidia chinensis) alleviated chilling injury during cold storage. Sci Hortic. (2022) 296:110876. doi: 10.1016/j.scienta.2022.110876
19. Cheng J, Zheng A, Li H, Huan C, Jiang T, Shen S, et al. Effects of melatonin treatment on ethanol fermenation and ERF expression in kiwifruit cv. Bruno during postharvest. Sci Hortic. (2022) 293:110696. doi: 10.1016/j.scienta.2021.110696
20. Huang Q, Wang C, Zhang Y, Chen C, Chen J. Gum arabic edible coating reduces postharvest decay and alleviates nutritional quality deterioration of ponkan fruit during cold storage. Front Nutr. (2021) 8:717596. doi: 10.3389/fnut.2021.717596
21. Wang Q, Ding T, Zuo J, Gao L, Fan L. Amelioration of postharvest chilling injury in sweet pepper by glycine betaine. Postharvest Biol Technol. (2016) 112:114–20. doi: 10.1016/j.postharvbio.2015.07.008
22. Wang Q, An X, Xiang M, Chen X, Luo Z, Fu Y, et al. Effects of 1-MCP on the physiological attributes, volatile components and ester-biosynthesis-related gene expression during storage of ‘Jinyan’ kiwifruit. Horticulturae. (2021) 7:381. doi: 10.3390/horticulturae7100381
23. Livak K, Schmittgen T. Analysis of relative gene expression data using real-time quantitative PCR and the 2–ΔΔCT method. Methods. (2001) 25:402–8. doi: 10.1006/meth.2001.1262
24. Gao H, Zhang Z, Chai H, Cheng N, Yang Y, Wang D, et al. Melatonin treatment delays postharvest senescence and regulates reactive oxygen species metabolism in peach fruit. Postharvest Biol Technol. (2016) 118:103–10. doi: 10.1016/j.postharvbio.2016.03.006
25. Zheng H, Liu W, Liu S, Liu C, Zheng L. Effects of melatonin treatment on the enzymatic browning and nutritional quality of fresh-cut pear fruit. Food Chem. (2019) 299:125116. doi: 10.1016/j.foodchem.2019.125116
26. Bulley S, Rassam M, Hoser D, Otto W, Schünemann N, Wright M, et al. Gene expression studies in kiwifruit and gene over-expression in Arabidopsis indicates that GDP-L-galactose guanyltransferase is a major control point of vitamin C biosynthesis. J Exp Bot. (2009) 60:765–78. doi: 10.1093/jxb/ern327
27. Laing W, Martínez-Sánchez M, Wright M, Bulley S, Brewster D, Dare A, et al. An upstream open reading frame is essential for feedback regulation of ascorbate biosynthesis in Arabidopsis. Plant Cell. (2015) 27:772–86. doi: 10.1105/tpc.114.133777
28. Imai T, Ban Y, Terakami S, Yamamoto T, Moriguchi T. L-Ascorbate biosynthesis in peach: cloning of six L-galactose pathway-related genes and their expression during peach fruit development. Physiol Plant. (2009) 136:139–49. doi: 10.1111/j.1399-3054.2009.01213.x
29. Zhang Y, Wang K, Xiao X, Cao S, Chen W, Yang Z, et al. Effect of 1-MCP on the regulation processes involved in ascorbate metabolism in kiwifruit. Postharvest Biol Technol. (2021) 179:111563. doi: 10.1016/j.postharvbio.2021.111563
30. Liu C, Zheng H, Sheng K, Liu W, Zheng L. Effects of melatonin treatment on the postharvest quality of strawberry fruit. Postharvest Biol Technol. (2018) 139:47–55. doi: 10.1016/j.postharvbio.2018.01.016
31. Liang D, Shen Y, Ni Z, Wang Q, Lei Z, Xu N, et al. Exogenous melatonin application delays senescence of kiwifruit leaves by regulating the antioxidant capacity and biosynthesis of flavonoids. Front Plant Sci. (2018) 9:426. doi: 10.3389/fpls.2018.00426
32. Eltelib H, Fujikawa Y, Esaka M. Overexpression of the acerola (Malpighia glabra) monodehydroascorbate reductase gene in transgenic tobacco plants results in increased ascorbate levels and enhanced tolerance to salt stress. S Afr J Bot. (2012) 78:295–301. doi: 10.1016/j.sajb.2011.08.005
33. Huang M, Xu Q, Deng X. L-Ascorbic acid metabolism during fruit development in an ascorbate-rich fruit crop chestnut rose (Rosa roxburghii Tratt). J Plant Physiol. (2014) 171:1205–16. doi: 10.1016/j.jplph.2014.03.010
34. Li Q, Li Y, Li C, Yu X. Enhanced ascorbic acid accumulation through overexpression of dehydroascorbate reductase confers tolerance to methyl viologen and salt stresses in tomato. Czech J Genet Plant Breed. (2012) 48:74–86. doi: 10.17221/100/2011-CJGPB
Keywords: kiwifruit, melatonin, ascorbic acid, gene expression, metabolism
Citation: Luo Z, Zhang J, Xiang M, Zeng J, Chen J and Chen M (2022) Exogenous melatonin treatment affects ascorbic acid metabolism in postharvest ‘Jinyan’ kiwifruit. Front. Nutr. 9:1081476. doi: 10.3389/fnut.2022.1081476
Received: 27 October 2022; Accepted: 18 November 2022;
Published: 02 December 2022.
Edited by:
Yifen Lin, Fujian Agriculture and Forestry University, ChinaReviewed by:
Zheng Xianbo, Henan Agricultural University, ChinaFariborz Habibi, University of Florida, United States
Copyright © 2022 Luo, Zhang, Xiang, Zeng, Chen and Chen. This is an open-access article distributed under the terms of the Creative Commons Attribution License (CC BY). The use, distribution or reproduction in other forums is permitted, provided the original author(s) and the copyright owner(s) are credited and that the original publication in this journal is cited, in accordance with accepted academic practice. No use, distribution or reproduction is permitted which does not comply with these terms.
*Correspondence: Ming Chen, mingchen@jxau.edu.cn