Brain changes: aerobic exercise for traumatic brain injury rehabilitation
- 1Division of Medical Sciences, University of Victoria, Victoria, BC, Canada
- 2Brain Changes Initiative, Concord, ON, Canada
- 3KITE Research Institute, University Health Network, Toronto, ON, Canada
- 4Island Medical Program and Department of Cellular and Physiological Sciences, The University of British Columbia, Victoria, BC, Canada
Introduction: Traumatic Brain Injury (TBI) accounts for millions of hospitalizations and deaths worldwide. Aerobic exercise is an easily implementable, non-pharmacological intervention to treat TBI, however, there are no clear guidelines for how to best implement aerobic exercise treatment for TBI survivors across age and injury severity.
Methods: We conducted a PRISMA-ScR to examine research on exercise interventions following TBI in children, youth and adults, spanning mild to severe TBI. Three electronic databases (PubMed, PsycInfo, and Web of Science) were searched systematically by two authors, using keywords delineated from “Traumatic Brain Injury,” “Aerobic Exercise,” and “Intervention.”
Results: Of the 415 papers originally identified from the search terms, 54 papers met the inclusion criteria and were included in this review. The papers were first grouped by participants’ injury severity, and subdivided based on age at intervention, and time since injury where appropriate.
Discussion: Aerobic exercise is a promising intervention for adolescent and adult TBI survivors, regardless of injury severity. However, research examining the benefits of post-injury aerobic exercise for children and older adults is lacking.
1 Introduction
Traumatic brain injuries are a global health issue, with more than 27 million treated injuries being reported yearly (James et al., 2019). To put this figure in context, the number of reported head injuries is greater than the entire population of some countries, like Australia (25.69 million) (Australian Bureau of Statistics, 2023). Despite this being a global health issue, treating individuals with traumatic brain injury (TBI) remains challenging. TBIs are often
called snowflake injuries due to their unique etiology, severity, affected population, and the burden they place on families and the health care system in any country. Given how unique each TBI can be, finding the best course of treatment for individuals remains challenging. Ideal treatments should be lifestyle oriented, physically and financially accessible, tailorable, and easily implementable. While aerobic exercise may meet many of these criteria, it is currently unknown how effective it is as a treatment for TBI across age and injury severity.
Aerobic exercise can be defined as low-to-vigorous-intensity, repetitive physical exercise performed for extended periods, that produce an elevation in heart rate. The term “aerobic” highlights how the body uses oxygen to meet energy requirements through aerobic metabolism (McArdle et al., 2006; Plowman and Smith, 2007). Both subjective measures (relative perceived exertion, talk tests) and objective measures (heart rates, oxygen intake/output) are common ways of assessing an aerobic exercise prescription, with the gold standard being a maximal oxygen consumption test (Lee and Zhang, 2021). The current Government of Canada recommends that healthy adults aged 18–64 get at least 2.5 h per week of physical activity, focusing on aerobic activity in 10 + min sessions (Government of Canada, 2018). While these guidelines are helpful for general fitness, very few standardized recommendations exist for aerobic exercise following TBI, and most of the graded recommendations are based on mild TBI (Parachute, 2017).
It is worth exploring aerobic exercise as a rehabilitation method for brain injury for a number of reasons. First, it promotes physical health, and cardiovascular fitness. Additionally, it has been shown to promote cognitive and mental health (Ruegsegger and Booth, 2018), both of which can be impacted by TBI (Langlois et al., 2006; Haarbauer-Krupa et al., 2021). Aerobic exercise is a potentiator of neuroplasticity, the brain’s ability to rewire, reorganize and form new neural connections. In animal models, it has been extensively studied as a means to stimulate neurogenesis, the brain’s ability to form and integrate new neurons (van Praag et al., 1999; Farmer et al., 2004; Nokia et al., 2016). It has been shown to improve cognitive functions commonly impacted by TBI, including improving attention, memory and processing speed in children and adults (Khan and Hillman, 2014; Young et al., 2015), as well as reducing levels of depression and anxiety (Carek et al., 2011). Given that TBI-survivors have a higher likelihood of poverty (Young and Hughes, 2020) and disability (Hyder et al., 2007), the low cost and adaptable nature of aerobic exercise makes it an appealing therapeutic option.
An important consideration for brain injury interventions is that TBI outcomes can differ depending on the age at which the injury was sustained. Children are more likely to show initial improvements, only to later have disrupted brain development leading to cognitive and behavioral issues that manifest over time, potentially impacting academic performance and social relationships (Anderson et al., 2009). Adolescent children aged 12 to 17 may have a more complicated TBI experience, as this is a period of complex cognitive, hormonal and physical growth. For example, studies focused on TBI in adolescents have observed significant risks of further mental and physical health challenges (Ilie et al., 2014) and impaired socially adapted decision capacity, compared to non-brain-injured peers (Beauchamp et al., 2019). For adults, especially as they reach middle-to-older age, post-TBI challenges can span from difficulties with the return to work, early cognitive decline, and slowed recovery timelines (Rabinowitz et al., 2021). Despite these complexities, developing brains tend to have greater neuroplastic potential, and may be more receptive to post-injury intervention. Given these age-associated TBI outcomes and risks, it is essential to consider the affected individual’s age, as the injury’s implications and recovery trajectories differ across age groups.
Previous systematic review papers on the effects of aerobic exercise following traumatic brain injury have been limited by focusing on the severity of injury [i.e., concussion (Howell et al., 2019; Langevin et al., 2020)] or specific outcome measures [i.e., quality of life (O’Carroll et al., 2020) and cognition (McDonnell et al., 2011)]. To better understand how aerobic exercise may be used as a treatment following TBI, this systematic scoping review aims to encapsulate the literature examining aerobic exercise-based interventions following TBI, separated by injury severity and age. Given the diversities of the injury and the intervention, a scoping review was deemed an appropriate methodology to synthesize the vast body of evidence in this field. The aim is to present a comprehensive review of the literature on aerobic exercise as a post-TBI intervention, in order to better determine what interventions are supported and which areas require more investigation.
2 Methods
2.1 Registration
This scoping review was conducted in accordance with the Preferred Reporting for Systematic Reviews and Meta-analyses extension for scoping reviews (PRISMA-ScR) (Tricco et al., 2018) and was guided using pre-determined frameworks for scoping reviews (Arksey and O’Malley, 2005; Levac et al., 2010; Tricco et al., 2018). An overview of this framework includes: identifying the research question, relevant studies, study selection, data extraction/data charting, and summarizing and presenting the results. This review’s objectives, eligibility criteria, preliminary study characteristics, and indicator papers were determined before starting the study. In accordance with PRISMA-ScR, the protocol was not registered; however, is available by request.
2.2 Research question
What is the evidence surrounding aerobic exercise to improve cognitive, mental and physical health following all severities of traumatic brain injury in children, adolescents and adults?
2.3 Eligibility criteria
Inclusion and exclusion criteria were determined a priori and selected to capture focused results related to the research question (Supplementary Table 1).
2.4 Information sources
Three databases (i.e., Web of Science, PubMed, PsychInfo) were selected as digital search engines for this review paper. These databases were selected based on their missions to deliver quality clinical and medical materials. The first search occurred on May 01, 2023, and a final search was conducted on August 01, 2023. Two authors (TS and JM) conducted these searches individually and compared search results to ensure the reliability of the search terms.
2.5 Search
To identify eligible articles, four search term blocks were used (Supplementary Table 2). Four search blocks were used, each to assist in finding related articles: Traumatic Brain Injury, Aerobic Exercise, Intervention, Review. The first three blocks used AND modifiers between, while the fourth block used a NOT modifier to reduce the number of review papers included in the search results.
2.6 Selection of sources of evidence
The first and second authors individually exported the results into Zotero citations manager for screening and selection. To start, duplicate titles were removed, then titles and abstracts were screened for initial eligibility. The remaining papers were read in entirety, and full eligibility was assessed, as determined by the a priori inclusion criteria. The first and second author compared their final papers for inclusion, and any discrepancies were resolved by consensus. The number of papers at each step is available in Figure 1.
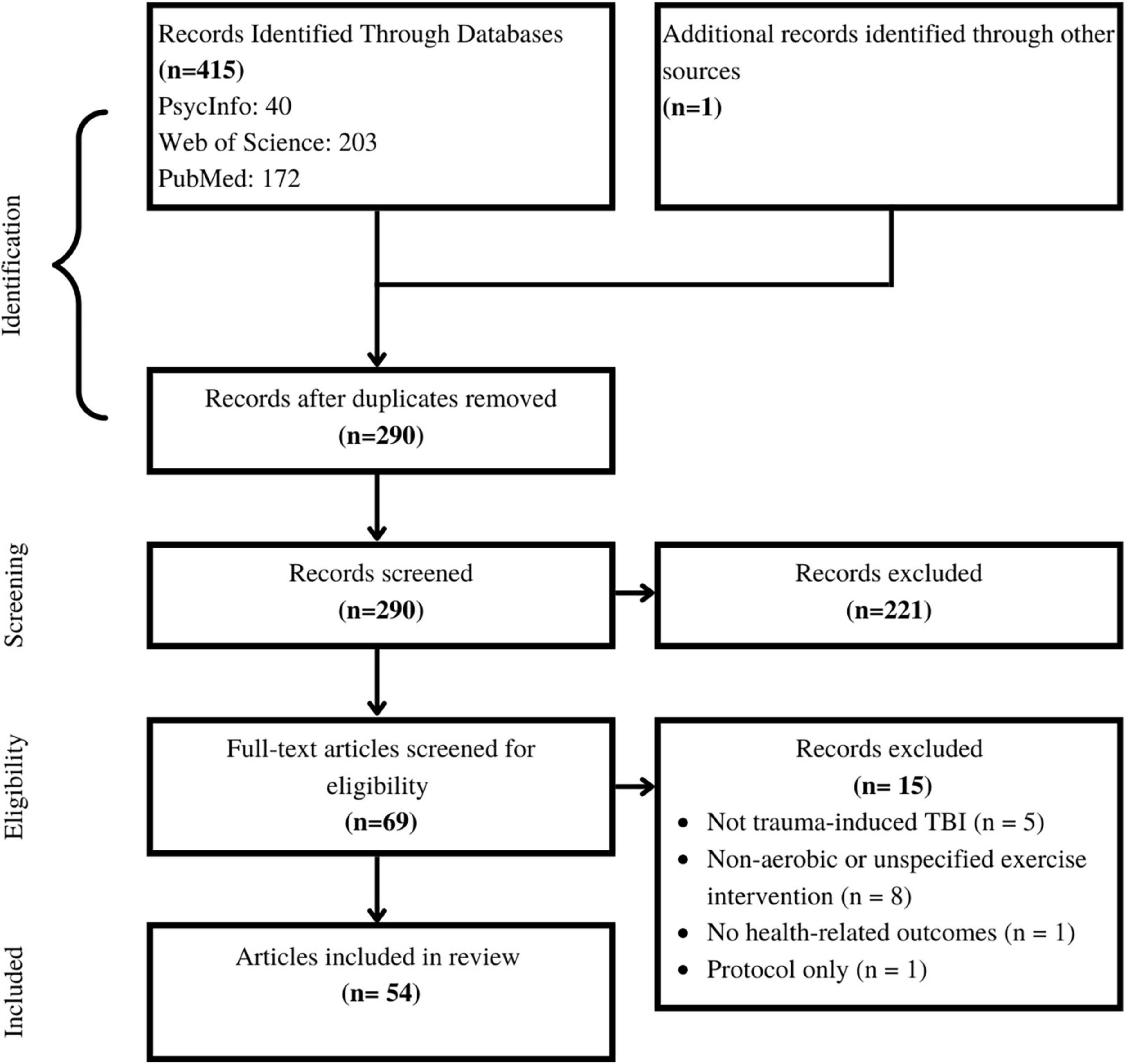
Figure 1. Selection of sources of evidence. The authors started with 415 papers. Following systematic duplication removal, screening, and eligibility assessment 54 papers were included in this review.
2.7 Data charting
Data charting was split between team members (TS, JM, MB, EE, CA, EG, HR, JB), and overseen and confirmed by TS and JM. Google Sheets was used to chart data, and specific variables of interest were identified before data charting. In addition to the standard variables (e.g., type of study, participant demographics, time since injury, intervention details), a notes and considerations section was added such that the charter was able to make note of anything pertinent in the study that was not immediately captured in the primary data charts. The results tables are simplified versions of the complete data charting used in this review.
2.8 Data items
The specific data items and rationale used in the data charting can be found in Supplementary Table 3.
2.9 Synthesis of results
Following the data charting, TS reviewed all charting and papers to ensure their alignment with the goals of the paper. While data charters made notes about considerations for each study, the authors did not conduct any formal critical appraisals or bias scoring in alignment with PRISMA-ScR protocols. However, should this scoping review inspire systematic reviews, the authors encourage including bias scoring or other critical metrics of the included data. Upon completion of data charting, papers were grouped based on participant age and severity of TBI, and a narrative summary was composed.
3 Results
3.1 Selection of sources as evidence
The first and second authors individually searched Web of Science, PubMed and PsychInfo databases, and found a total of 415 articles. After removing duplicate items, 290 articles were screened. After screening the title and abstract of each paper, 69 papers remained for full-text reading. Of the 69 papers, five were excluded due to wrong injury type, eight were excluded for not including an aerobic intervention, one was excluded as it did not contain primary research data, and one was excluded for not including any health-related outcome measures, leaving 54 papers in this review (Figure 1).
3.2 Characteristics of sources of evidence
Of the included articles, three overarching research designs emerged, including case series, randomized controlled trials, and non-randomized pre-post intervention studies. All citations were presented as primary, peer-reviewed articles, as defined in the inclusion and exclusion criteria. In studies encompassing multiple pre-defined age groups, the results were reported separately within each respective category if separate age-based analyses were conducted. However, if the age groups were combined, the study findings would be reported based on the category that corresponds to the mean age of the participants. Therefore, while thirty-four of the studies included adults (aged 18 +), 23 included adolescents (aged 12–17, inclusive), and five included children under 12, the main grouping of TBI severity could only be sub-sectioned into adults (n = 32) and adolescents (n = 22), given that all studies reported a mean age above 12.
3.3 Results of individuals sources of evidence
The following sections are divided by reported severity of injury (severe, mixed, mild, unspecified) and further subdivided by reported age group (adult, adolescent). Studies with similar outcome measures (mental health, physical health, cognitive health) are presented within these subcategories. Figure 2 provides an overview of the selected included articles.
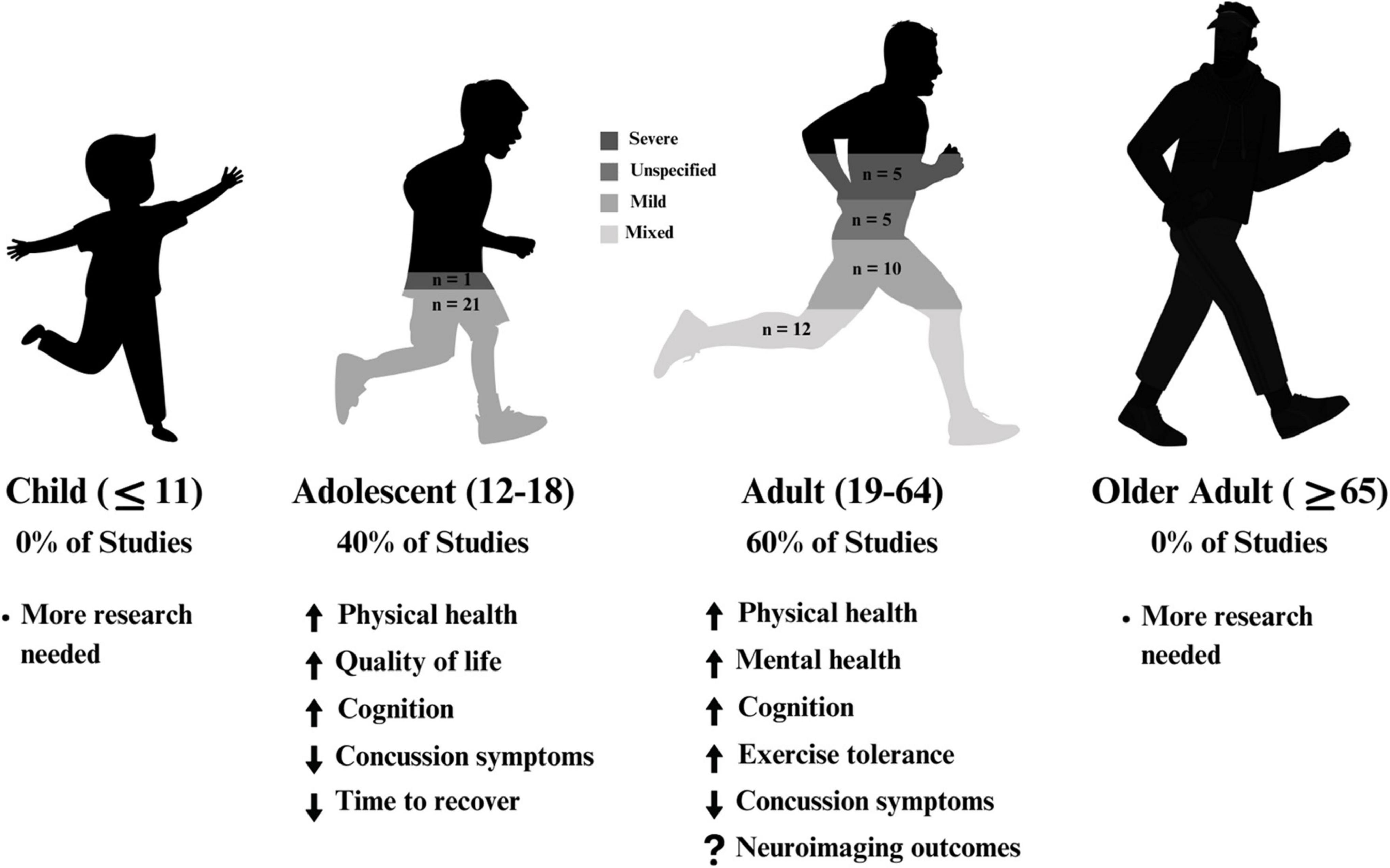
Figure 2. A summary of results across age within the included articles. A majority of studies focussed on adults (aged 19–64), and the remaining studies focussed on adolescents (aged 12–18). 57% of studies solely examined mild traumatic brain injury.
3.4 Severe brain injury
Six of the included studies exclusively studied individuals with severe brain injuries. Five papers examined adults (Hassett et al., 2009; Corral et al., 2014; Chanpimol et al., 2017; Curcio et al., 2020; Wender et al., 2021), while one presented a case report on an adolescent female (Tiwari et al., 2018). Full results are presented in Table 1.
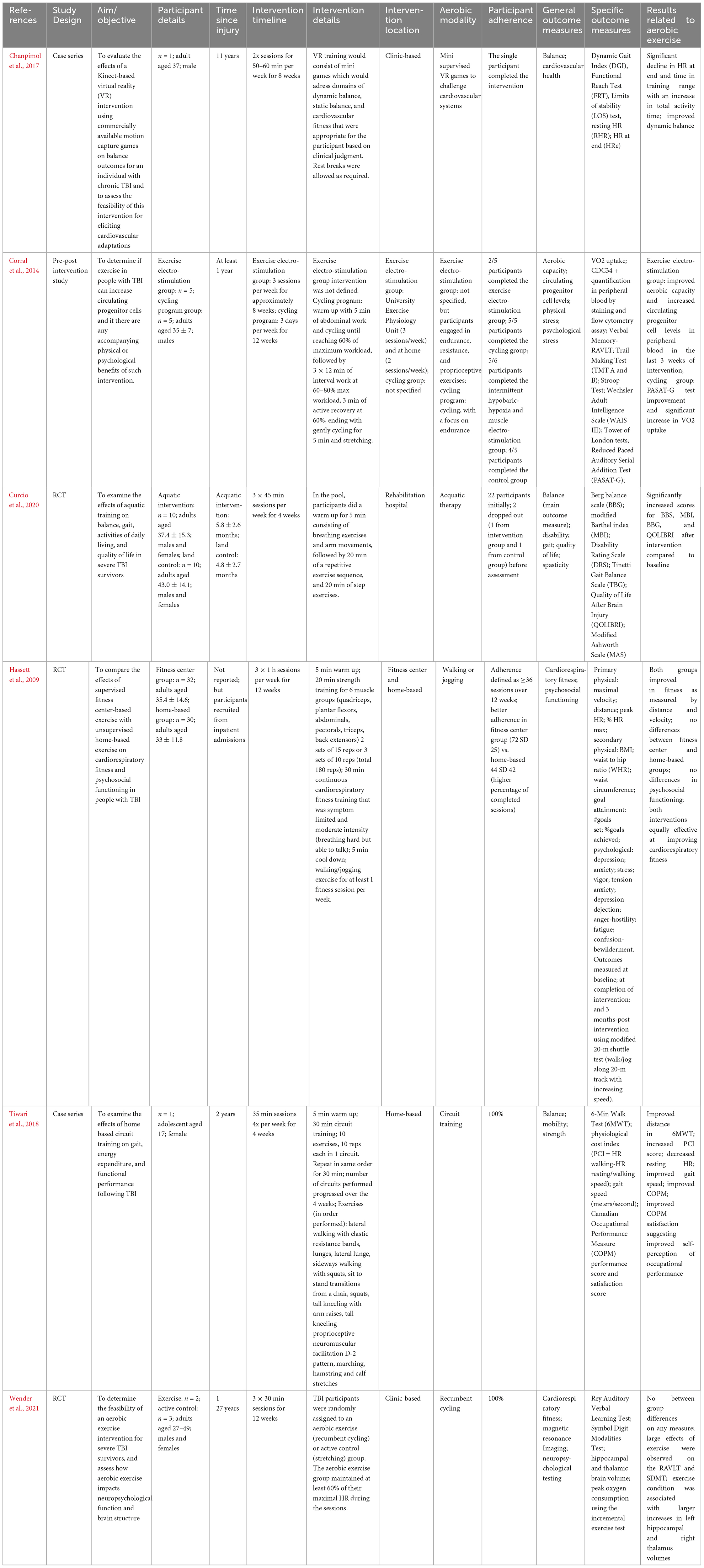
Table 1. Summary of the effects of aerobic exercise intervention following severe traumatic brain injury.
3.4.1 Adults
All studies included at least two time points (pre- and post-interventions). In most cases, individuals were adults when they sustained their TBI, with time since injury ranging across studies from unspecified inpatient admissions (Hassett et al., 2009) to 27 years post injury (Wender et al., 2021), however, most interventions occurred within 1–2 years post-injury. Intervention timelines ranged from 4-weeks to 12-weeks, typically 3 times per week for 30–60 min. Engagement in aerobic activity varied between studies; two used recumbent cycling for their aerobic component (Corral et al., 2014; Wender et al., 2021), one used a virtual reality platform (Chanpimol et al., 2017), one used aquatic therapy (Curcio et al., 2020), and the last used walking and jogging (Hassett et al., 2009). All studies were conducted in a clinic or otherwise supervised setting, and Hassett et al. (2009) also included a home-based exercise group.
Outcomes of interest across these studies included fitness, balance, quality of life, mental health, as well as brain structure and functions. Hassett et al. (2009), Corral et al. (2014), and Chanpimol et al. (2017) all observed improvements in measures of cardiovascular fitness across their interventions. Curcio et al. (2020) observed improvements in multiple measures of balance, and quality of life, while Wender et al. (2021) found large effects of exercise on measures of verbal memory and processing speed as compared to a stretching/toning control group. Additionally, Wender et al. (2021) reported greater increases in left hippocampal and right thalamic volumes, however this study included 2–3 individuals per group.
Some considerations of these studies include the limited definitions of aerobic or cardiovascular activity; only two studies included target heart rate or effort zones (Corral et al., 2014; Wender et al., 2021). Only one study included a home-based intervention and found decreased adherence in that group compared to clinic-based interventions. It is also important to consider the sample sizes in terms of adequate power and statistical analyses.
3.4.2 Adolescents
One case report examined the effects of aerobic exercise on a 17-year-old female who sustained their TBI in adolescence (2 years post-injury) (Tiwari et al., 2018). The home-based intervention included 4 × 35-min weekly sessions, for 4 weeks. This circuit-style intervention included a mixture of resistance exercises and cardiovascular training. Similar to adults with severe TBIs, researchers found improved cardiovascular health, quality of life, and increased gait speed.
3.5 Mixed injury severity
Twelve studies included mixed severities of mild, moderate and/or severe in their aerobic intervention studies (Bhambhani et al., 2005; Brown et al., 2005; Schwandt et al., 2012; Wise et al., 2012; Bellon et al., 2015; Chin et al., 2015, 2019; Weinstein et al., 2017; Morris et al., 2018; Ding et al., 2021; Romanov et al., 2021; Tomoto et al., 2022), all of which reported on adults aged 18–65. Full results can be found in Table 2.
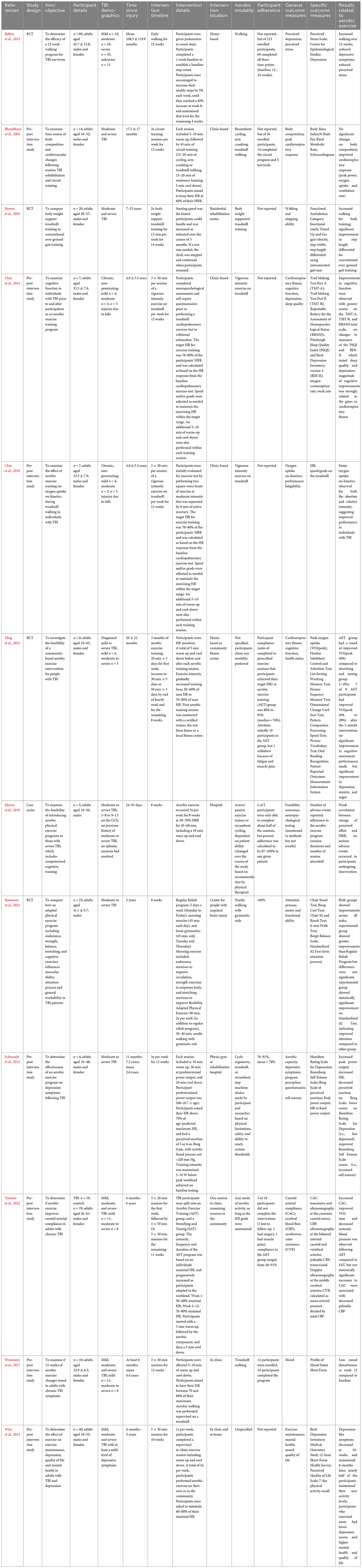
Table 2. Summary of the effects of aerobic exercise intervention following mixed-severity traumatic brain injury.
3.5.1 Adults
Eleven of twelve studies employed aerobic interventions at chronic time points post-injury, ranging from 6 months to 23 years (Bhambhani et al., 2005; Brown et al., 2005; Schwandt et al., 2012; Wise et al., 2012; Bellon et al., 2015; Chin et al., 2015, 2019; Weinstein et al., 2017; Ding et al., 2021; Romanov et al., 2021; Tomoto et al., 2022), while one study looked at individuals within 3 months of injury (Morris et al., 2018). Intervention timelines ranged between 4 to 14 weeks, with a minimum of two 15-min weekly sessions (Brown et al., 2005), and a maximum of daily activity (Bellon et al., 2015). Most studies employed supervised interventions, with only three involving a home-based component (Wise et al., 2012; Bellon et al., 2015; Ding et al., 2021). Half of the studies used walking or jogging as the aerobic intervention, typically done on a treadmill (Brown et al., 2005; Bellon et al., 2015; Chin et al., 2015, 2019; Weinstein et al., 2017; Romanov et al., 2021). Depending on the study and participant abilities, some interventions offered physical supports (i.e., walking poles, weight assistance). The other half of the studies used multiple types of exercise or allowed participants to choose their aerobic activity based on abilities and preferences (Bhambhani et al., 2005; Schwandt et al., 2012; Wise et al., 2012; Morris et al., 2018; Ding et al., 2021; Tomoto et al., 2022). Nine of twelve studies tracked participants’ heart rates to ensure aerobic zone adherence, all of which aimed for 60–80% of participants’ maximal heart rates.
Outcomes of interest varied between studies and included measures of mental health, physical health and abilities, cognition, quality of life, carotid arterial compliance, and feasibility of intervention. Eight studies included at least one measure of physical health or ability, all of which reported improvements across their respective interventions, most commonly improvements in VO2 max and heart rates (Bhambhani et al., 2005; Brown et al., 2005; Schwandt et al., 2012; Chin et al., 2015, 2019; Romanov et al., 2021; Tomoto et al., 2022). Six studies included measures related to mental health, with a majority (5/6) reporting improvements in their study-specific measures of stress, depression, quality of life and mood (Schwandt et al., 2012; Wise et al., 2012; Bellon et al., 2015; Weinstein et al., 2017; Chin et al., 2019; Ding et al., 2021). Of note, some studies used self-report for these metrics, while others used standardized inventories (see Table 2). Three studies included cognition-related measures, with Chin et al. (2015) reporting improvements in working memory performance and Romanov et al. (2021) finding improvements in attention. However, Chin et al. (2015) found no changes in cognitive performance across various other cognitive measures from pre- to post-intervention.
These studies should be reviewed with several considerations. First, it is hard to extrapolate if different effects of intervention would be observed if the studies focused on one severity of brain injury, given the heterogenous needs of adults with mild TBI compared to severe TBI. Many studies in this group were pilot studies, and were likely underpowered (Bhambhani et al., 2005; Brown et al., 2005; Schwandt et al., 2012; Chin et al., 2015, 2019; Weinstein et al., 2017; Morris et al., 2018; Ding et al., 2021; Tomoto et al., 2022), requiring follow-up with full studies. Further, several studies did not include control groups at all timepoints (Schwandt et al., 2012; Wise et al., 2012; Bellon et al., 2015; Chin et al., 2015; 2019; Weinstein et al., 2017; Morris et al., 2018; Ding et al., 2021). Intervention adherence also seemed to be a challenge, with adherence rates near 50% in two studies (Bhambhani et al., 2005; Bellon et al., 2015).
3.6 Mild brain injury
Ten studies specifically looked at aerobic interventions post-concussion in adults (Leddy et al., 2010; Leddy and Willer, 2013; Polak et al., 2015; Clausen et al., 2016; Adams and Moore, 2017; Dobney et al., 2017; Snyder et al., 2021; Varner et al., 2021; Hutchison et al., 2022; Langevin et al., 2022), and 21 studies focused on adolescents (Gagnon et al., 2016; Imhoff et al., 2016; Chrisman et al., 2017, 2021; Kurowski et al., 2017; Yuan et al., 2017; Chan et al., 2018; Hunt et al., 2018; McGeown et al., 2018; Micay et al., 2018; Bailey et al., 2019; Gladstone et al., 2019; Leddy et al., 2019a,b, 2021; Willer et al., 2019; Dobney et al., 2020; Howell et al., 2021, 2022; Chizuk et al., 2022; Shore et al., 2022). Results for adults are presented in Table 3.
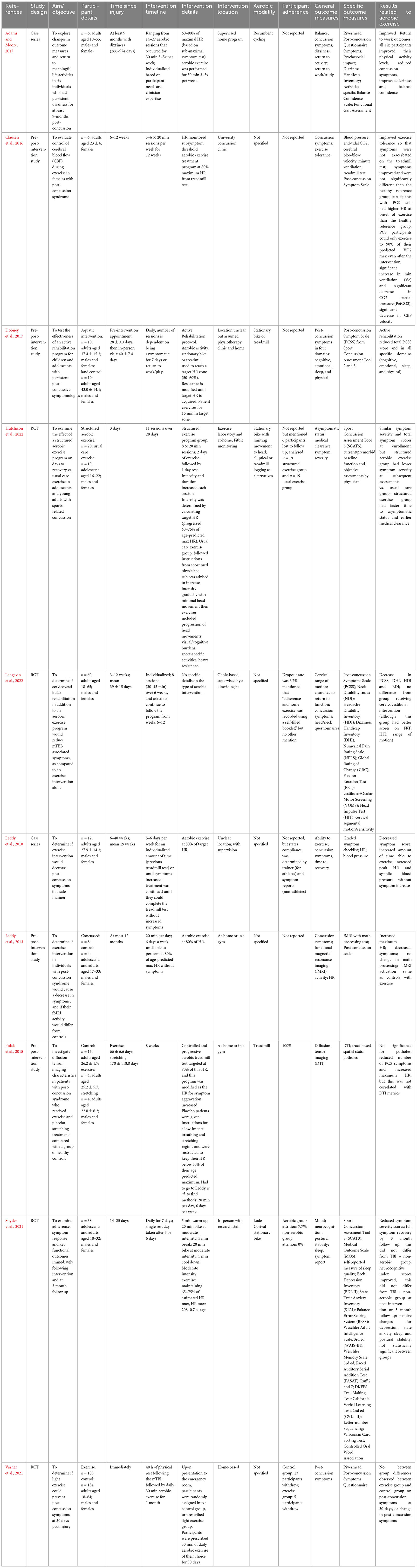
Table 3. Summary of the effects of aerobic exercise intervention following mild traumatic brain injury in adults.
3.6.1 Adults
Individuals were adults when they sustained their TBIs, with time since injury ranging from 48 h to 2.7 years. Five studies had set intervention lengths (ranging from 1 to 12 weeks), whereas the other studies took an individualized approach to the aerobic intervention duration. The type of aerobic modality performed was only specified in five of the ten studies. Aerobic modalities included recumbent cycling, elliptical and treadmill (Polak et al., 2015; Adams and Moore, 2017; Dobney et al., 2017; Snyder et al., 2021). Participants were assigned a target heart rate in eight of the ten studies, which ranged from 60–80% of maximum. Involvement of a home-based intervention was present in over half of the studies (6/10) (Leddy et al., 2013; Polak et al., 2015; Adams and Moore, 2017; Dobney et al., 2017; Varner et al., 2021; Hutchison et al., 2022), and the majority of studies included supervision throughout the interventions.
All ten studies examined concussion symptoms as an outcome measure. Other outcome measures varied between studies and included return to activity, exercise tolerance, cervical range of motion, head/neck questionnaires, diffusion tensor imaging, cognition, fMRI activity, sleep, and postural stability. Post-concussion symptoms were reduced following aerobic intervention in nine studies (Leddy et al., 2010; Leddy and Willer, 2013; Polak et al., 2015; Clausen et al., 2016; Adams and Moore, 2017; Dobney et al., 2017; Snyder et al., 2021; Hutchison et al., 2022; Langevin et al., 2022). Varner et al. (2021) found no significant change in post-concussion symptoms after 1 month of daily aerobic exercise that began 48 h post-injury compared to the control group. The two studies that examined exercise tolerance found an improvement in participants’ ability to exercise without symptom exacerbation post-intervention (Leddy et al., 2010; Clausen et al., 2016). In relation to brain imaging, Leddy et al. (2013) reported fMRI activation in TBI survivors after exercise to be the same as healthy exercise controls, and Polak et al. (2015) found no correlation between post-intervention symptom reduction and DTI metrics. Of note, both of the aforementioned reports were pilot studies (N = 4 concussed individuals in the intervention group), which must be considered when interpreting these data.
Considerations include inadequate comparison groups in some studies (Clausen et al., 2016; Adams and Moore, 2017; Dobney et al., 2017; Langevin et al., 2022). One study provided insufficient details on the aerobic exercise paradigm, and half of the studies did not specify the modality of aerobic exercise performed, affecting their reproducibility.
3.6.2 Adolescents
Most individuals were adolescents (12–18 years) upon sustaining their injury, with time since injury as short as three days up to 2.8 years post-injury (Hunt et al., 2018; Leddy et al., 2019a). Five studies included children (younger than 12 years) (Imhoff et al., 2016; Hunt et al., 2018; Dobney et al., 2020; Leddy et al., 2021; Howell et al., 2022), and two included adults (19 years and older) (McGeown et al., 2018; Howell et al., 2022), but since the average age within the study was in the adolescent range, and no age-separated analyses were done, results are presented in the adolescent section. Studies examining aerobic exercise interventions in adolescents post-concussion can be divided into two categories: early intervention (within 2 weeks post-injury), and chronic intervention for persistent symptoms (4 weeks or longer post-intervention). Due to the large number of studies in this section, the authors sub-grouped and presented the studies based on the above. Full results are presented in Tables 4, 5, respectively.
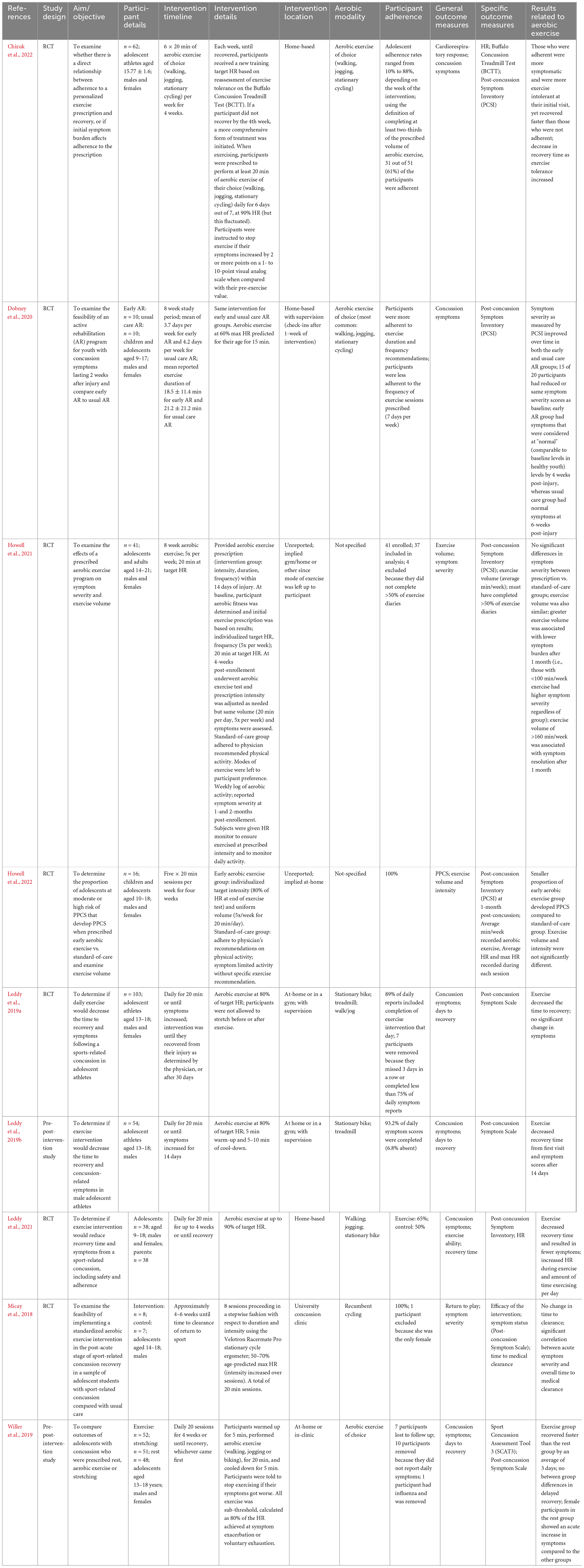
Table 4. Summary of the effects of aerobic exercise interventions within two-weeks following mild traumatic brain injury in adolescents.
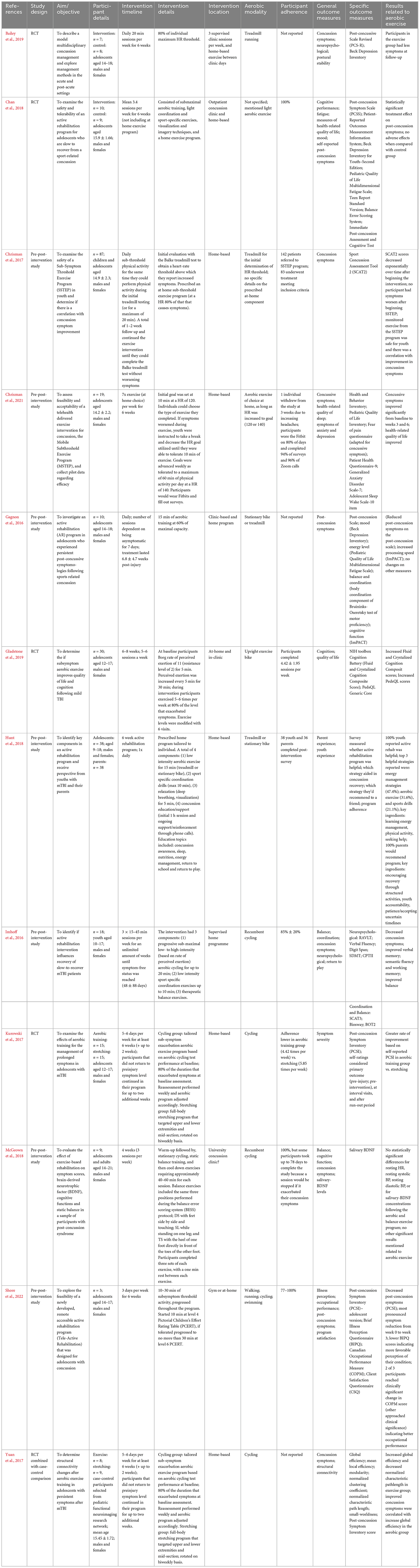
Table 5. Summary of the effects of aerobic exercise interventions implemented four weeks or longer following mild traumatic brain injury in adolescents.
3.6.2.1 Adolescents—Acute timepoint
Nine studies assessed adolescents with mTBI within 2-weeks of injury (64; 54,55,57; 89; 25; 37, 39; 18). Intervention timelines ranged from 4 to 8 weeks; however, several studies discontinued the intervention once return-to-play status was assumed. Most commonly, studies had participants engaging in daily aerobic exercise for 20 min (54,55,57; 89), or 20-min sessions 5 days per week (37, 39). The remaining studies examined 6x per week, 3x per week, 2x per week or personalized frequency for 15–20 min per session (64; 25; 18). All studies tracked heart rate and had participants exercising at 60–90% of their max heart rates during the exercise sessions. Eight studies allowed participants to choose their aerobic modality, if heart rate targets were met; most commonly participants engaged in jogging, walking or recumbent cycling. 64 had participants exclusively use recumbent cycling as an aerobic activity.
These particular studies showcased a distinct set of outcome measures. All studies were primarily centered on recovery from concussion, frequently evaluating parameters such as the duration of recovery, concussion symptoms, time taken to return to play, and participants’ ability to tolerate exercise. Of the eight studies that evaluated recovery time, seven found that implementing early exercise interventions reduced time to recover compared to standard care (Leddy et al., 2019a,b, 2021; Willer et al., 2019; Dobney et al., 2020; Howell et al., 2021; Chizuk et al., 2022). Additionally, Howell et al. (2022) reported that the early exercise group was less likely to develop persistent post-concussion symptoms compared to the usual treatment group.
Adherence to the exercise interventions ranged from 61 to 100% across studies, and interestingly, Chizuk et al. (2022) reported that adherent participants recovered faster than non-adherent participants, supporting the benefits of early exercise post-mTBI. While this set of studies represents the most cohesive group, most studies focus on sport-related concussions, therefore, these results may not be generalizable to wider populations.
3.6.2.2 Adolescents—Chronic timepoints
Twelve studies examined aerobic exercise for adolescents at least 1 month post injury who were experiencing at-least one persistent post-concussion symptom (Gagnon et al., 2016; Imhoff et al., 2016; Chrisman et al., 2017, 2021; Kurowski et al., 2017; Yuan et al., 2017; Chan et al., 2018; Hunt et al., 2018; McGeown et al., 2018; Bailey et al., 2019; Gladstone et al., 2019; Shore et al., 2022). A majority of these studies offered a mix of in-clinic and at-home interventions for 6 weeks (Chan et al., 2018; Hunt et al., 2018; Bailey et al., 2019; Chrisman et al., 2021; Shore et al., 2022), with up to an additional two weeks, making an 8-week intervention (Kurowski et al., 2017; Yuan et al., 2017; Gladstone et al., 2019). One study consisted of a four-week intervention (McGeown et al., 2018). In contrast, the remaining three studies offered the intervention until symptom resolution occurred (Gagnon et al., 2016; Imhoff et al., 2016; Chrisman et al., 2017). Intervention frequency ranged from 3 times per week (15–45 min) to 20 min daily. Ten studies included effort-based measures (e.g., Borg scale) or physiological measures (i.e., heart rate) to gauge aerobic status during exercise (Gagnon et al., 2016; Imhoff et al., 2016; Chrisman et al., 2017, 2021; Kurowski et al., 2017; Yuan et al., 2017; Chan et al., 2018; Bailey et al., 2019; Gladstone et al., 2019; Shore et al., 2022). Recumbent cycling was the exclusive aerobic modality in five studies (Imhoff et al., 2016; Kurowski et al., 2017; Yuan et al., 2017; McGeown et al., 2018; Gladstone et al., 2019), while five studies offered participants their choice of activity (Gagnon et al., 2016; Chan et al., 2018; Hunt et al., 2018; Chrisman et al., 2021; Shore et al., 2022). Two studies had participants engage in treadmill-based walking or running (Chrisman et al., 2017; Bailey et al., 2019).
While nine of twelve studies in this set examined concussion-related symptoms, many included additional measures of mental health, mood, cognition, intervention experience, balance, saliva-based protein analysis, illness perception and structural connectivity. A total of 100% of studies that examined concussion-related symptoms found improvements post-intervention; however, only five of these studies included appropriate comparison groups (Gagnon et al., 2016; Imhoff et al., 2016; Chrisman et al., 2017, 2021; Kurowski et al., 2017; Yuan et al., 2017; Chan et al., 2018; Bailey et al., 2019; Shore et al., 2022). In terms of cognition, Gladstone et al. (2019) found that exercise increased crystallized and fluid cognition composite scores, Gagnon et al. (2016) reported improved processing speed and no differences in balance, while Imhoff et al. (2016) reported improvements in multiple memory tasks and balance. McGeown et al. (2018) examined salivary brain-derived neurotrophic factor (BDNF), but did not find any improvements post-exercise. However, the authors suggest this can be attributed by the large variance in baseline levels and the variable intervention length. One study examined functional connectivity and found that participants in the exercise group had increased global efficiency and decreased normalized path length (Yuan et al., 2017). Only one study assessed attitudes and feasibility of the exercise intervention; 100% of the participants reported that the active rehabilitation was effective (Hunt et al., 2018).
Many considerations are prudent when assessing this group of studies. First, the sample sizes in ten of the twelve studies are below 30 individuals, which may indicate that a majority of these studies are underpowered. Additionally, only five studies included control or comparison groups, which helps determine effects related to the intervention.
3.7 Unspecified
Five studies did not specify the severity of traumatic brain injury in participants undergoing aerobic interventions, but referred to trauma-induced brain injury (Hoffman et al., 2010; Esquenazi et al., 2013; Kolakowsky-Hayner et al., 2017; Pennington et al., 2022; Tefertiller et al., 2022). Full results are presented in Table 6.
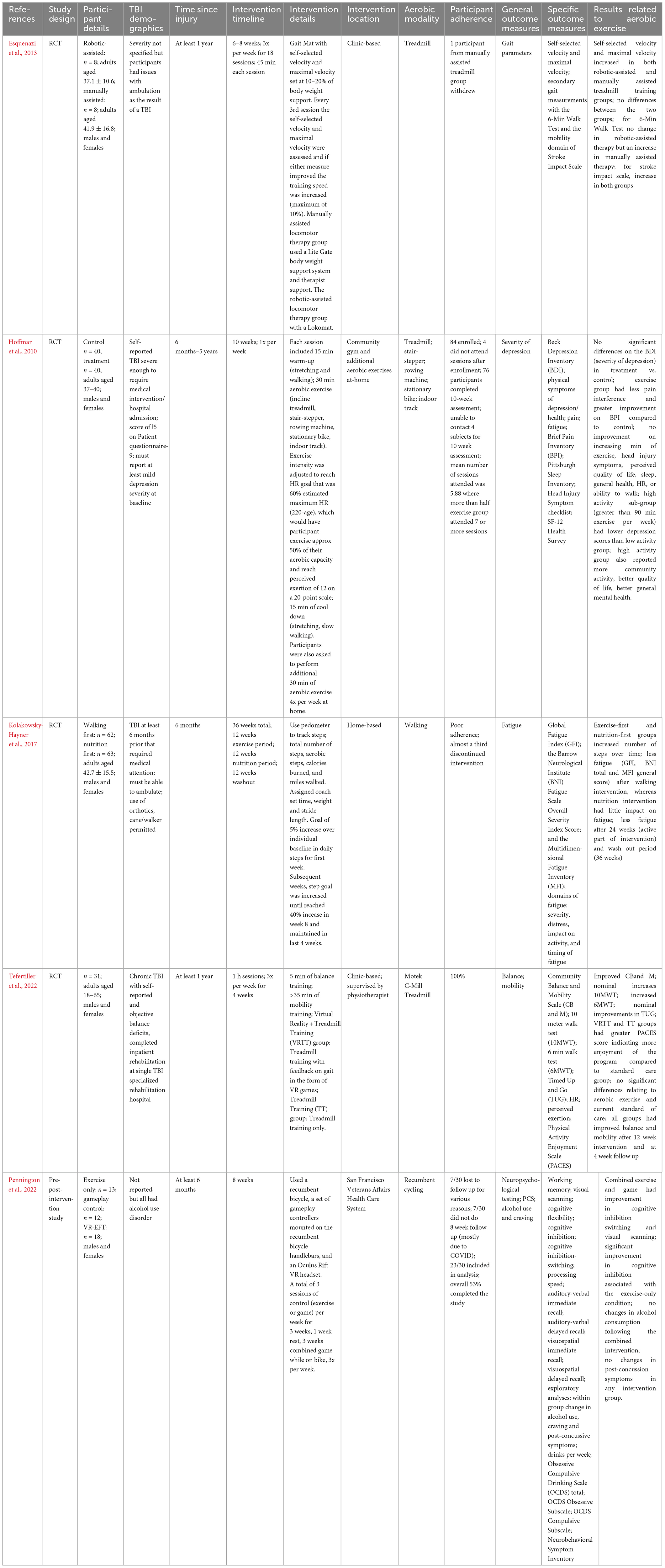
Table 6. Summary of the effects of aerobic exercise interventions following unspecified-severity traumatic brain injury.
All participants in these studies were adults, with time since injury ranging from 6 months to 5 years. Aerobic exercise interventions spanned from 4 to 12 weeks. Two of these interventions included home-based components and the other three were clinic-based. Aerobic modalities varied amongst studies, including the use of treadmills, stationary cycling, rowing machines, stair-steppers, indoor tracks, and outdoor walking. Four studies used a single modality of aerobic exercise, three of which had participants walking (two on the treadmill, one with home-based walking) and one study used stationary cycling (Esquenazi et al., 2013; Kolakowsky-Hayner et al., 2017; Pennington et al., 2022; Tefertiller et al., 2022). The study using stationary cycling also involved a combined intervention with virtual reality (Pennington et al., 2022).
Outcome measures assessed in these studies were gait, severity of depression, fatigue, balance, mobility, neuropsychological assessments, alcohol use, and post-concussion symptoms. Esquenazi et al. (2013) found that participants improved their mobility, along with self-selected and maximum velocity on the treadmill after the aerobic exercise intervention. Hoffman et al. (2010) found an improvement in pain after the exercise intervention, and lower post-intervention depression scores for the high-activity exercise group compared to the low-activity group. Participant fatigue was reduced after intervention in Kolakowsky-Hayner et al. (2017) and Tefertiller et al. (2022) found improved balance and mobility post-intervention which persisted at the 4-week follow-up. Improved cognitive inhibition was reported by Pennington et al. (2022); however, they found no change in alcohol use or post-concussion symptoms post-intervention.
It should be noted that there was low adherence to the home-based intervention in Kolakowsky-Hayner et al. (2017), with almost a third of participants discontinuing the intervention. Pennington et al. (2022) only had 53% of participants fully complete their in-person study, which included an 8-week follow-up. Additionally, target heart rate zones were only assigned to participants in one of the five studies (Hoffman et al., 2010).
4 Discussion
Fifty-four studies were included in this review, and first divided based on injury severity (i.e., severe, mixed, mild, unspecified), then subdivided based on age (i.e., adult, adolescent), and time since injury (i.e., acute, chronic) when applicable. The purpose of this review was to describe the breadth of aerobic interventions used to improve health-related outcomes post-TBI across the lifespan. However, it is worth noting that no studies exclusively examined aerobic exercise following moderate TBI, nor in children younger than 12, and therefore these groups are absent from the present review.
For adults, aerobic exercise post-TBI is a promising intervention, especially as no studies reported adverse effects for participants. However, it is worth considering how the impact of aerobic exercise may be influenced by factors such as the severity of the injury and time since injury. In studies focusing on severe brain injury, aerobic exercise seems to be effective at improving cardiovascular health and balance in TBI survivors, and shows promise for improving cognitive functions, like memory, which is supported by increased hippocampal volume post-intervention. In mixed and unspecified injury severity groups, again, aerobic exercise shows benefits related to cardiovascular and physical and fitness, cognition, and mental health. The studies focused on mild TBIs, including concussions, showed more consistent benefits for aerobic exercise interventions, particularly in symptom reduction and exercise tolerance. However the lesser-studied effects of these interventions on cognitive and neuroimaging measures are mixed. Overall, the body of evidence generally supports aerobic exercise as a safe, easily implementable intervention for adults post-TBI.
In adolescents, 21 studies examined aerobic exercise post-mild traumatic brain injury, and one study presented a case study post-severe TBI. From this cohort of studies, aerobic exercise is a safe and beneficial intervention for post-concussion adolescents, and can be effectively implemented as early as one-week post-injury. Overall, early implementation seems to promote recovery compared to standard care, and chronic implementation is effective at reducing concussion-related symptoms and supporting cognition and fitness.
While these findings are promising, there are several notable limitations in the present study and existing research. In the present study, while we aimed to conduct a comprehensive review, we could not include papers that did not have an official English translation. In the literature, although many studies showed positive results of aerobic exercise, an individual’s time since injury should be considered. Time since injury can play a role in physical recovery. For example, with severe brain injury, multiple physical injuries are common activity-limiting comorbidities (Chan et al., 2017). Several studies included assisted-physical activity, by use of walking supports, physical therapists present, and including non-balance dependent exercise (e.g., hand cranking), demonstrating that aerobic exercise is possible amongst other physical challenges. Neurologically, the post-TBI timeline is complex and may differ by injury severity. TBIs can cause cytotoxic cell death, neuronal damage, neural metabolic crisis, gliotic scarring, inflammation, and an increase in reactive oxygen species, all contributing to adverse outcomes. However, the post-injury timeline is also known to be one of neuroplasticity. It is suggested that neuroplasticity may occur in three phases post-injury (Sophie Su et al., 2016). During the first few days post-injury, increased cell death, neurometabolic strain and decreased cortical inhibitory pathways may signal for neuronal cells and glial cells to replace damaged ones and facilitate recovery, and the utilization of new neuronal networks (Burda and Sofroniew, 2014; Giza and Hovda, 2014; Sophie Su et al., 2016). In the weeks post-injury, synaptogenesis and axonal sprouting assist in the process (Sophie Su et al., 2016), while neural metabolism begins to stabilize (Wieloch and Nikolich, 2006). However, these previous perturbations from the brain’s typical state may induce “negative plasticity,” a perpetuating cycle of reduced brain plasticity, which can persist years after the injury (Figure 3; Tomaszczyk et al., 2014). This negative plasticity has been attributed to lack of use, reduced efficiency in processing sensory and perceptual information, and impaired control over neuromodulation, and is hypothesized to underlay long-term TBI-induced neurodegeneration (Tomaszczyk et al., 2014). Given that aerobic exercise promotes neuroplasticity, it seems especially important to include it in rehabilitation programs at all stages post injury, but it may have the largest benefits at more acute timepoints. It could also be possible that interventions implemented at chronic timepoints should occur at higher frequency, and for longer, to counteract the hypothesized negative plasticity.
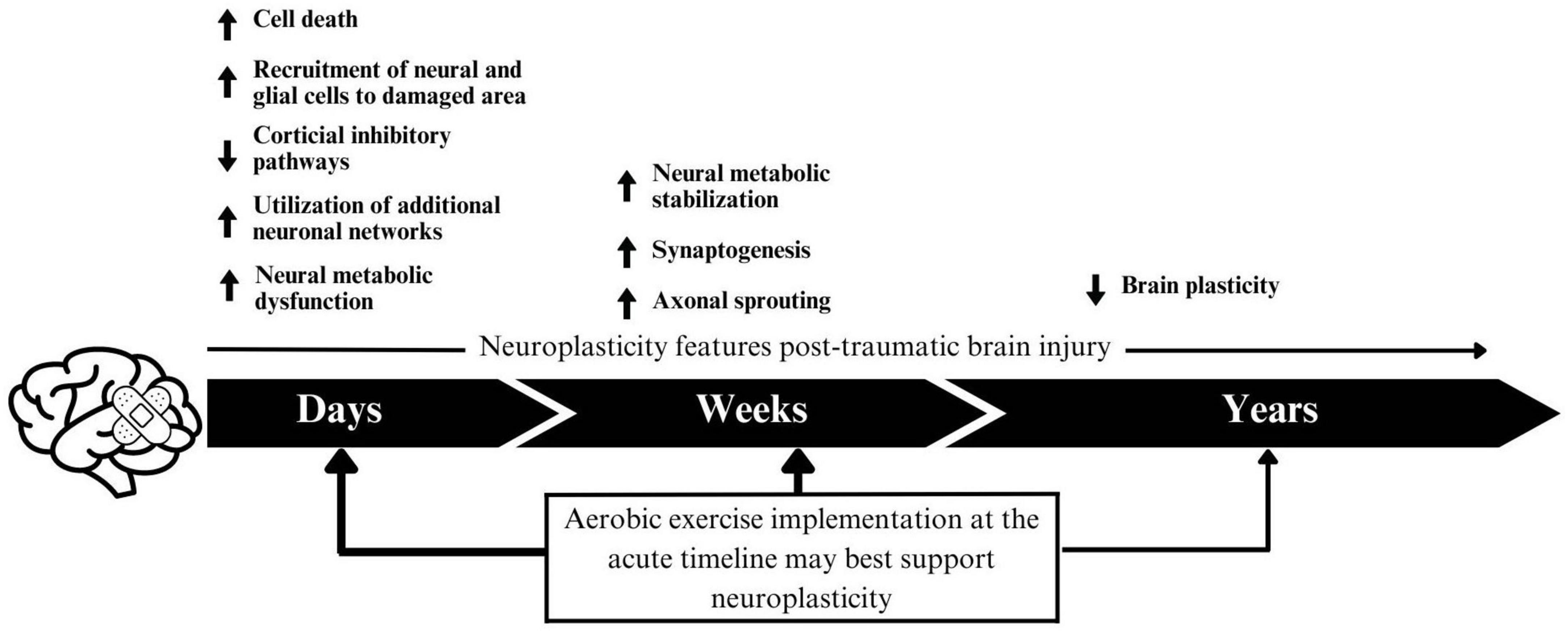
Figure 3. A proposed schematic of negative plasticity following traumatic brain injury. Arrow thickness indicates the proposed impact of intervention within that timeframe.
Common limitations in this cohort of studies include inadequate comparison groups, lack of details about the exercise programs, small sample sizes, and low adherence rates. While comparison groups are especially important for intervention-based studies, we recognize the many challenges that can occur in this population that can make having these groups challenging (e.g., recruitment windows, scheduling, participants engaging in multiple therapeutic interventions). The importance of adequate comparison groups (e.g., active control, wait-list control) allows us to draw more definitive conclusions about the intervention’s effects, which is especially important post-injury, given that time is an important factor in recovery. Studies which only include one group, or have small sample sizes are still valid and beneficial, and can lead to areas for future research, but they should be interpreted with discretion. Several studies in this review reported adherence rates near 50%, which may indicate challenges with study design, participant interest, feasibility, and accessibility of the intervention. In this set of studies, a mix of clinic-based and home-based studies reported low adherence, therefore, it may be important to offer both in-person and at-home options, such that participants can determine what would be best for them. Given these adherence challenges, it would be worthwhile to conduct feasibility studies in this area to better understand the contributing factors to this low adherence. Another challenge in this set of studies included limited details on aerobic activity, for example, unclear reporting of modality, duration, and frequency of sessions, and if participants had specific effort or heart rate targets. Accurate reporting of interventions allows future researchers to repeat and expand study designs that show promise. While these studies offer valuable insights, the common limitations underscore the necessity for well-structured, detailed research protocols, and robust adherence strategies.
This scoping review serves as a foundation for the application of post-traumatic brain injury aerobic exercise. Based on this review, we propose a methodological framework and a number of suggestions and areas for future research (Table 7). A key finding of this scoping review is the clear lack of research for children aged 12 years and younger, and elderly adults, aged 65 and over. Across all TBI severities, not one study exclusively studied children or elderly adults, despite the fact that these age groups are most at risk for TBIs (Government of Canada, 2020). Interventions at these time points are critical, as children are going through robust neurological development, while older adults typically fare worse after TBI than younger adults (LeBlanc et al., 2006; Araki et al., 2017). TBIs are one of the greatest causes of death and disability in these groups, and adapted aerobic exercise is a promising, and understudied research area. Physical abilities may play a role in this understudied area, but just because someone has reduced or different mobility, does not mean that aerobic exercise should be avoided. Weight-assisted walking, walking poles, water activities and hand cranking are some of the many ways in which researchers could accommodate younger and older age groups into the current body of literature. Another lacking area of research is specific to moderate TBI, or TBIs with a Glasgow Coma Scale rating of 9–13. While individuals with moderate TBIs were included in the research presented, they were either grouped in with severe TBI survivors or their injury severity was unspecified. By definition, moderate TBIs present uniquely upon examination, and survivors deserve tailored and specific research to support their recovery. One recommendation is to include severity-based sub-analyses of research to elicit any severity-specific findings.
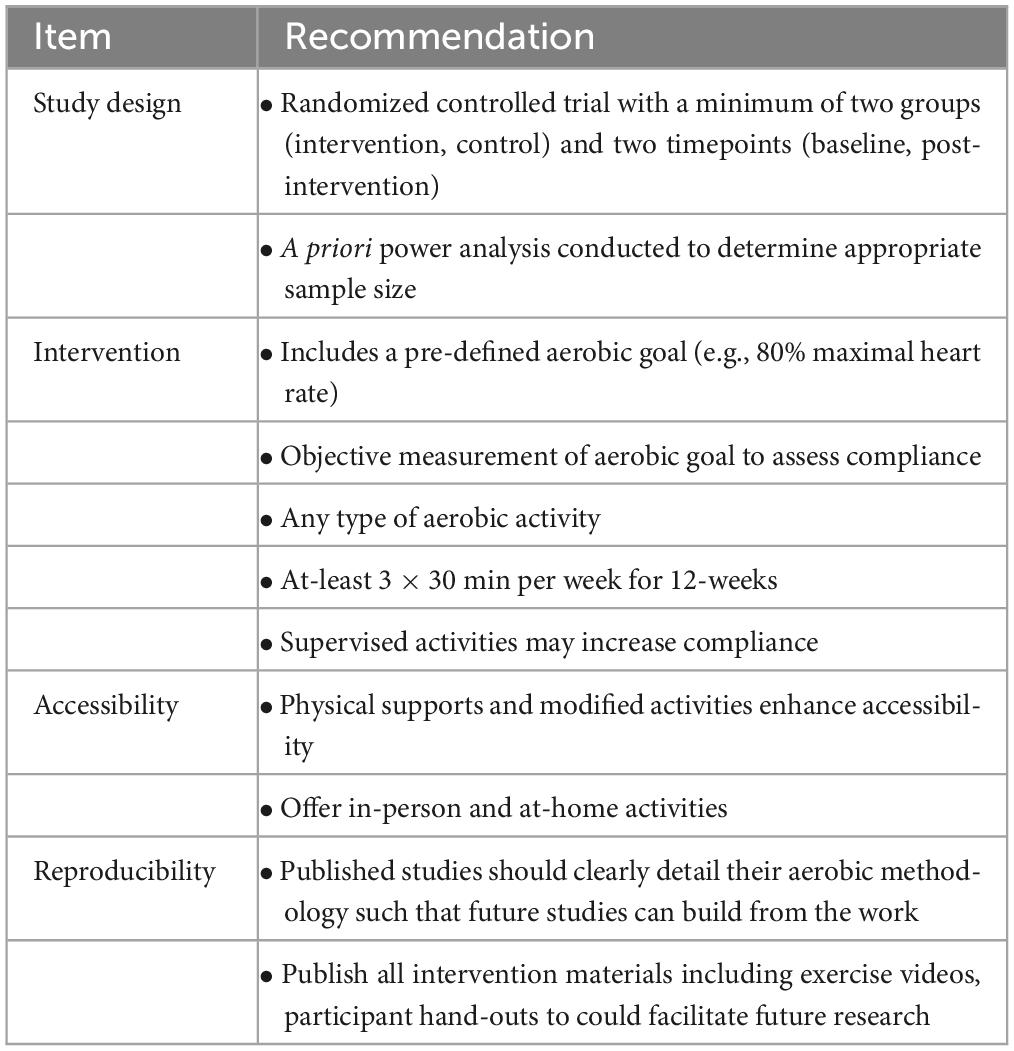
Table 7. Recommendations for future researchers using aerobic exercise as a post-traumatic brain injury intervention.
5 Conclusion
In conclusion, this scoping review presented the array of aerobic interventions used post-TBI across different life stages. It seems that while aerobic exercise post-TBI is a promising intervention area, it is influenced by various factors such as injury severity and the timing of the injury. Moreover, while this review suggests general consensus on the safety and effectiveness of aerobic exercise as a rehabilitative strategy post-TBI, it also highlights some significant gaps and limitations in the current research landscape.
Among these limitations, the inadequacy of comparison groups, small sample sizes, vague reporting on exercise programs, and low adherence rates emerged as key challenges. Despite these hurdles, it’s essential to acknowledge that studies with such limitations can still fuel future research and contribute to our understanding, albeit requiring careful interpretation.
Based on the present review, we suggest several avenues for future research. These include detailed and clear reporting of intervention design and execution, providing both in-clinic and at-home options for participants, and emphasizing the importance of power analysis in determining sample sizes. Furthermore, a focus on age-specific research is paramount given the glaring lack of studies on children aged 12 and under and elderly adults over 65. It is crucial to develop research strategies that are inclusive and accommodate individual abilities across the lifespan, especially considering the high risk of TBIs in these age groups. Also, more specific research on moderate TBIs, often overlooked in studies, is needed to ensure recovery support tailored to this group.
As such, this scoping review serves as a critical cornerstone for future research into the use of aerobic exercise post-TBI. By addressing the noted limitations and gaps in the existing body of knowledge, we can enhance our understanding of this field and optimize recovery strategies for TBI survivors across different ages and injury severities.
6 Transparency, rigor, and reproducibility
This review is part of a series of systematic reviews of Brain Changes Initiatives’ Brain Pillars of Health project. This scoping review was conducted according to PRISMA-ScR methodology and guidelines, and our findings are reported according to the PRISMA-ScR checklist. Our review was conducted by a multidisciplinary team including clinical experts in the field of brain injury, researchers and a survivor of brain injury. As reported in this scoping review, a range of aerobic exercise interventions exists for traumatic brain injury rehabilitation, however, there is a paucity of data specifically for children and older adults. Further, we assess intervention methods and study designs currently in use, and suggest standardization practices for future research. The search strategy is detailed in full in this manuscript. Full data charting sheets are available upon request.
Data availability statement
The original contributions presented in the study are included in the article/Supplementary material, further inquiries can be directed to the corresponding author.
Author contributions
TS: Conceptualization, Formal analysis, Methodology, Project administration, Visualization, Writing – original draft, Writing – review and editing. JM: Conceptualization, Formal analysis, Investigation, Methodology, Visualization, Writing – original draft, Writing – review and editing. MB: Formal analysis, Investigation, Writing – review and editing. EE: Formal analysis, Investigation, Writing – review and editing. CA: Formal analysis, Investigation, Writing – review and editing. EG: Investigation, Writing – review and editing. HR: Investigation, Writing – review and editing. JB: Investigation, Writing – review and editing. MG: Conceptualization, Funding acquisition, Resources, Writing – review and editing. JG: Conceptualization, Writing – review and editing. BC: Conceptualization, Funding acquisition, Resources, Writing – review and editing.
Funding
The author(s) declare financial support was received for the research, authorship, and/or publication of this article. This work was supported by a Special Purpose Grant, awarded from Branch Out Neurological Foundation to TS, with funds for this award provided by the Brain Changes Initiative. BC was supported by grants from CIHR.
Author disclosure
MG and Brain Changes Initiative (BCI)
MG is a physician, the founder of BCI, and a TBI survivor. In 2013, MG endured a critical motor vehicle accident, resulting in a severe traumatic brain injury. Following immediate life support, MG faced notable cognitive and physical impairments, casting doubt on returning to his previous medical schooling and active lifestyle. Through concerted efforts, including acute care and admittance to Toronto Rehabilitation Institute, he embarked on a profound recovery process, including Physiotherapy, Occupational Therapy, and Speech-Language Pathology. After his discharge, MG’s extensive research on neurogenesis and neuroplasticity helped structure a tailored rehabilitation program. With unwavering hard-work and commitment, he triumphantly completed his medical degree and residency at Western University’s Schulich School of Medicine. Now a licensed physician, MG has inaugurated Brain Changes Initiative, targeting transformative research, support, and advocacy in TBI, aiming to revolutionize the standard of care in Canada and worldwide. This initiative underscores the importance of tailored rehabilitation and continuous research in shaping a promising path for TBI survivors. To learn more about the Brain Changes Initiative’s story, research and funding opportunities visit: https://www.brainchanges.org/.
This review is the first commissioned piece in a series of systematic reviews for Brain Changes Initiative, focused on BCI’s six pillars of brain health: Aerobic Exercise, Cognitive Training, Sleep Hygiene, Mindfulness, Nutrition, and Limiting Harmful Exposures. MG is the founder of BCI and BRC and JG sit on BCI’s scientific advisory board. TSR is a volunteer for BCI.
Conflict of interest
The authors declare that the research was conducted in the absence of any commercial or financial relationships that could be construed as a potential conflict of interest.
The author(s) declared that they were an editorial board member of Frontiers, at the time of submission. This had no impact on the peer review process and the final decision.
Publisher’s note
All claims expressed in this article are solely those of the authors and do not necessarily represent those of their affiliated organizations, or those of the publisher, the editors and the reviewers. Any product that may be evaluated in this article, or claim that may be made by its manufacturer, is not guaranteed or endorsed by the publisher.
Supplementary material
The Supplementary Material for this article can be found online at: https://www.frontiersin.org/articles/10.3389/fnhum.2023.1307507/full#supplementary-material
References
Adams, J., and Moore, B. (2017). Return to meaningful activities after a multi-modal rehabilitation programme among individuals who experience persistent dizziness and debility longer than 9 months after sustaining a concussion: a case series. Physiother. Can. 69, 249–259. doi: 10.3138/ptc.2015-81ep
Anderson, V., Spencer-Smith, M., Leventer, R., Coleman, L., Anderson, P., Williams, J., et al. (2009). Childhood brain insult: can age at insult help us predict outcome? Brain 132, 45–56. doi: 10.1093/brain/awn293
Araki, T., Yokota, H., and Morita, A. (2017). Pediatric traumatic brain injury: characteristic features, diagnosis, and management. Neurol. Med. Chir. 57, 82–93.
Arksey, H., and O’Malley, L. (2005). Scoping studies: towards a methodological framework. Int. J. Soc. Res. Methodol. 8, 19–32. doi: 10.1080/1364557032000119616
Australian Bureau of Statistics (2023). Population | Australian Bureau of Statistics. Available online at: https://www.abs.gov.au/statistics/people/population (accessed June 22, 2023).
Bailey, C., Meyer, J., Briskin, S., Tangen, C., Hoffer, S., Dundr, J., et al. (2019). Multidisciplinary concussion management: A model for outpatient concussion management in the acute and post-acute settings. J. Head Trauma Rehabil. 34, 375–384. doi: 10.1097/HTR.0000000000000527
Beauchamp, M., Vera-Estay, E., Morasse, F., Anderson, V., and Dooley, J. (2019). Moral reasoning and decision-making in adolescents who sustain traumatic brain injury. Brain Inj. 33, 32–39. doi: 10.1080/02699052.2018.1531307
Bellon, K., Kolakowsky-Hayner, S., Wright, J., Huie, H., Toda, K., Bushnik, T., et al. (2015). A home-based walking study to ameliorate perceived stress and depressive symptoms in people with a traumatic brain injury. Brain Inj. 29, 313–319. doi: 10.3109/02699052.2014.974670
Bhambhani, Y., Rowland, G., and Farag, M. (2005). Effects of circuit training on body composition and peak cardiorespiratory responses in patients with moderate to severe traumatic brain injury. Arch. Phys. Med. Rehabil. 86, 268–276. doi: 10.1016/j.apmr.2004.04.022
Brown, T., Mount, J., Rouland, B., Kautz, K., Barnes, R., and Kim, J. (2005). Body weight-supported treadmill training versus conventional gait training for people with chronic traumatic brain injury. J. Head Trauma Rehabil. 20, 402–415. doi: 10.1097/00001199-200509000-00002
Burda, J., and Sofroniew, M. (2014). Reactive gliosis and the multicellular response to CNS damage and disease. Neuron 81, 229–248. doi: 10.1016/j.neuron.2013.12.034
Carek, P., Laibstain, S., and Carek, S. (2011). Exercise for the treatment of depression and anxiety. Int. J. Psychiatry Med. 41, 15–28. doi: 10.2190/PM.41.1.c
Chan, C., Iverson, G., Purtzki, J., Wong, K., Kwan, V., Gagnon, I., et al. (2018). Safety of active rehabilitation for persistent symptoms after pediatric sport-related concussion: A randomized controlled trial. Arch. Phys. Med. Rehabil. 99, 242–249. doi: 10.1016/j.apmr.2017.09.108
Chan, V., Mollayeva, T., Ottenbacher, K., and Colantonio, A. (2017). Clinical profile and comorbidity of traumatic brain injury among younger and older men and women: a brief research notes. BMC Res. Notes 10:371. doi: 10.1186/s13104-017-2682-x
Chanpimol, S., Seamon, B., Hernandez, H., Harris-Love, M., and Blackman, M. (2017). Using Xbox kinect motion capture technology to improve clinical rehabilitation outcomes for balance and cardiovascular health in an individual with chronic TBI. Arch. Physiother. 7:6. doi: 10.1186/s40945-017-0033-9
Chin, L., Chan, L., Drinkard, B., and Keyser, R. (2019). Oxygen uptake on-kinetics before and after aerobic exercise training in individuals with traumatic brain injury. Disabil. Rehabil. 41, 2949–2957. doi: 10.1080/09638288.2018.1483432
Chin, L., Keyser, R., Dsurney, J., and Chan, L. (2015). Improved cognitive performance following aerobic exercise training in people with traumatic brain injury. Arch. Phys. Med. Rehabil. 96, 754–759. doi: 10.1016/j.apmr.2014.11.009
Chizuk, H., Willer, B., Cunningham, A., Bezherano, I., Storey, E., Master, C., et al. (2022). Adolescents with Sport-Related Concussion Who Adhere to Aerobic Exercise Prescriptions Recover Faster. Med. Sci. Sports Exerc. 54, 1410–1416. doi: 10.1249/MSS.0000000000002952
Chrisman, S., Mendoza, J., Zhou, C., Palermo, T., Gogue-Garcia, T., Janz, K., et al. (2021). Pilot study of telehealth delivered rehabilitative exercise for youth with concussion: The mobile subthreshold exercise program (MSTEP). Front. Pediatr. 9:645814. doi: 10.3389/fped.2021.645814
Chrisman, S., Whitlock, K., Somers, E., Burton, M., Herring, S., Rowhani-Rahbar, A., et al. (2017). Pilot study of the Sub-Symptom Threshold Exercise Program (SSTEP) for persistent concussion symptoms in youth. NeuroRehabilitation 40, 493–499. doi: 10.3233/NRE-161436
Clausen, M., Pendergast, D., Willer, B., and Leddy, J. (2016). Cerebral blood flow during treadmill exercise is a marker of physiological postconcussion syndrome in female athletes. J. Head Trauma Rehabil. 31, 215–224. doi: 10.1097/HTR.0000000000000145
Corral, L., Conde, L., Guillamó, E., Blasi, J., Juncadella, M., Javierre, C., et al. (2014). Circulating progenitor cells during exercise, muscle electro-stimulation and intermittent hypobaric hypoxia in patients with traumatic brain injury: a pilot study. NeuroRehabilitation 35, 763–769. doi: 10.3233/NRE-141172
Curcio, A., Temperoni, G., Tramontano, M., De Angelis, S., Iosa, M., Mommo, F., et al. (2020). The effects of aquatic therapy during post-acute neurorehabilitation in patients with severe traumatic brain injury: a preliminary randomized controlled trial. Brain Inj. 34, 1630–1635. doi: 10.1080/02699052.2020.1825809
Ding, K., Tarumi, T., Tomoto, T., Bell, K., Madden, C., Dieppa, M., et al. (2021). A proof-of-concept trial of a community-based aerobic exercise program for individuals with traumatic brain injury. Brain Inj. 35, 233–240. doi: 10.1080/02699052.2020.1865569
Dobney, D., Grilli, L., Beaulieu, C., Straub, M., Galli, C., Saklas, M., et al. (2020). Feasibility of early active rehabilitation for concussion recovery in youth: A randomized trial. Clin. J. Sport Med. 30, 519–525. doi: 10.1097/JSM.0000000000000671
Dobney, D., Grilli, L., Kocilowicz, H., Beaulieu, C., Straub, M., Friedman, D., et al. (2017). Evaluation of an active rehabilitation program for concussion management in children and adolescents. Brain Inj. 31, 1753–1759. doi: 10.1080/02699052.2017.1346294
Esquenazi, A., Lee, S., Packel, A., and Braitman, L. (2013). A randomized comparative study of manually assisted versus robotic-assisted body weight supported treadmill training in persons with a traumatic brain injury. PM R. 5, 280–290. doi: 10.1016/j.pmrj.2012.10.009
Farmer, J., Zhao, X., van Praag, H., Wodtke, K., Gage, F., and Christie, B. (2004). Effects of voluntary exercise on synaptic plasticity and gene expression in the dentate gyrus of adult male Sprague-Dawley rats in vivo. Neuroscience 124, 71–79. doi: 10.1016/j.neuroscience.2003.09.029
Gagnon, I., Grilli, L., Friedman, D., and Iverson, G. L. (2016). A pilot study of active rehabilitation for adolescents who are slow to recover from sport-related concussion. Scand. J. Med. Sci. Sports 26, 299–306. doi: 10.1111/sms.12441
Giza, C., and Hovda, D. (2014). The new neurometabolic cascade of concussion. Neurosurgery 75, S24–S33. doi: 10.1227/NEU.0000000000000505
Gladstone, E., Narad, M., Hussain, F., Quatman-Yates, C., Hugentobler, J., Wade, S., et al. (2019). Neurocognitive and quality of life improvements associated with aerobic training for individuals with persistent symptoms after mild traumatic brain injury: secondary outcome analysis of a pilot randomized clinical trial. Front. Neurol. 10:1002. doi: 10.3389/fneur.2019.01002
Government of Canada (2018). Canada PHA of Physical Activity Tips for Adults (18-64 Years) education and awareness. Available online at: https://www.canada.ca/en/public-health/services/publications/healthy-living/physical-activity-tips-adults-18-64-years.html (accessed June 22, 2023).
Government of Canada (2020). Canada PHA of Injury in Review, 2020 Edition: Spotlight on Traumatic Brain Injuries across the Life Course. statistics. Available online at: https://www.canada.ca/en/public-health/services/injury-prevention/canadian-hospitals-injury-reporting-prevention-program/injury-reports/2020-spotlight-traumatic-brain-injuries-life-course.html (accessed July 26, 2023).
Haarbauer-Krupa, J., Pugh, M., Prager, E., Harmon, N., Wolfe, J., and Yaffe, K. (2021). Epidemiology of chronic effects of traumatic brain injury. J. Neurotrauma 38, 3235–3247. doi: 10.1089/neu.2021.0062
Hassett, L., Moseley, A., Tate, R., Harmer, A., Fairbairn, T., and Leung, J. (2009). Efficacy of a fitness centre-based exercise programme compared with a home-based exercise programme in traumatic brain injury: a randomized controlled trial. J. Rehabil. Med. 41, 247–255. doi: 10.2340/16501977-0316
Hoffman, J., Bell, K., Powell, J., Behr, J., Dunn, E., Dikmen, S., et al. (2010). A randomized controlled trial of exercise to improve mood after traumatic brain injury. PM R 2, 911–919. doi: 10.1016/j.pmrj.2010.06.008
Howell, D., Hunt, D., Aaron, S., Meehan, W., and Tan, C. (2021). Influence of aerobic exercise volume on postconcussion symptoms. Am. J. Sports Med. 49, 1912–1920. doi: 10.1177/03635465211005761
Howell, D., Taylor, J., Tan, C., Orr, R., and Meehan, W. (2019). The role of aerobic exercise in reducing persistent sport-related concussion symptoms. Med. Sci. Sports Exerc. 51, 647–652. doi: 10.1249/MSS.0000000000001829
Howell, D., Wingerson, M., Kirkwood, M., Grubenhoff, J., and Wilson, J. (2022). Early aerobic exercise among adolescents at moderate/high risk for persistent post-concussion symptoms: A pilot randomized clinical trial. Phys. Ther. Sport 55, 196–204. doi: 10.1016/j.ptsp.2022.04.010
Hunt, A., Laupacis, D., Kawaguchi, E., Greenspoon, D., and Reed, N. (2018). Key ingredients to an active rehabilitation programme post-concussion: perspectives of youth and parents. Brain Inj. 32, 1534–1540. doi: 10.1080/02699052.2018.1502894
Hutchison, M., Di Battista, A., Lawrence, D., Pyndiura, K., Corallo, D., and Richards, D. (2022). Randomized controlled trial of early aerobic exercise following sport-related concussion: Progressive percentage of age-predicted maximal heart rate versus usual care. PLoS One 17:e0276336. doi: 10.1371/journal.pone.0276336
Hyder, A., Wunderlich, C., Puvanachandra, P., Gururaj, G., and Kobusingye, O. (2007). The impact of traumatic brain injuries: a global perspective. NeuroRehabilitation 22, 341–353.
Ilie, G., Mann, R., Boak, A., Adlaf, E., Hamilton, H., Asbridge, M., et al. (2014). Suicidality, bullying and other conduct and mental health correlates of traumatic brain injury in adolescents. PLoS One 9:e94936. doi: 10.1371/journal.pone.0094936
Imhoff, S., Fait, P., Carrier-Toutant, F., and Boulard, G. (2016). Efficiency of an active rehabilitation intervention in a slow-to-recover paediatric population following mild traumatic brain injury: A Pilot Study. J. Sports Med. 2016:5127374. doi: 10.1155/2016/5127374
James, S., Theadom, A., and Ellenbogen, R. (2019). Global, regional, and national burden of traumatic brain injury and spinal cord injury, 1990-2016: a systematic analysis for the Global Burden of Disease Study 2016. Lancet Neurol. 18, 56–87. doi: 10.1016/S1474-4422(18)30415-0
Khan, N., and Hillman, C. (2014). The relation of childhood physical activity and aerobic fitness to brain function and cognition: a review. Pediatr. Exerc. Sci. 26, 138–146. doi: 10.1123/pes.2013-0125
Kolakowsky-Hayner, S., Bellon, K., Toda, K., Bushnik, T., Wright, J., Isaac, L., et al. (2017). A randomised control trial of walking to ameliorate brain injury fatigue: a NIDRR TBI model system centre-based study. Neuropsychol. Rehabil. 27, 1002–1018. doi: 10.1080/09602011.2016.1229680
Kurowski, B., Hugentobler, J., Quatman-Yates, C., Taylor, J., Gubanich, P., Altaye, M., et al. (2017). aerobic exercise for adolescents with prolonged symptoms after mild traumatic brain injury: An exploratory randomized clinical trial. J. Head Trauma Rehabil. 32, 79–89. doi: 10.1097/HTR.0000000000000238
Langevin, P., Frémont, P., Fait, P., Dubé, M., Bertrand-Charette, M., and Roy, J. (2020). Aerobic exercise for sport-related concussion: A systematic review and meta-analysis. Med. Sci. Sports Exerc. 52, 2491–2499. doi: 10.1249/MSS.0000000000002402
Langevin, P., Frémont, P., Fait, P., Dubé, M., Bertrand-Charette, M., and Roy, J. (2022). Cervicovestibular rehabilitation in adults with mild traumatic brain injury: A randomized clinical trial. J. Neurotrauma 39, 487–496. doi: 10.1089/neu.2021.0508
Langlois, J., Rutland-Brown, W., and Wald, M. (2006). The epidemiology and impact of traumatic brain injury: a brief overview. J. Head Trauma Rehabil. 21, 375–378. doi: 10.1097/00001199-200609000-00001
LeBlanc, J., de Guise, E., Gosselin, N., and Feyz, M. (2006). Comparison of functional outcome following acute care in young, middle-aged and elderly patients with traumatic brain injury. Brain Inj. 20, 779–790. doi: 10.1080/02699050600831835
Leddy, J., Cox, J., Baker, J., Wack, D., Pendergast, D., Zivadinov, R., et al. (2013). Exercise treatment for postconcussion syndrome: a pilot study of changes in functional magnetic resonance imaging activation, physiology, and symptoms. J. Head Trauma Rehabil. 28, 241–249. doi: 10.1097/HTR.0b013e31826da964
Leddy, J., Haider, M., Ellis, M., Mannix, R., Darling, S., Freitas, M., et al. (2019a). Early subthreshold aerobic exercise for sport-related concussion: A randomized clinical trial. JAMA Pediatr. 173, 319–325. doi: 10.1001/jamapediatrics.2018.4397
Leddy, J., Haider, M., Hinds, A., Darling, S., and Willer, B. S. A. (2019b). Preliminary Study of the Effect of Early Aerobic Exercise Treatment for Sport-Related Concussion in Males. Clin. J. Sport Med. 29, 353–360. doi: 10.1097/JSM.0000000000000663
Leddy, J., Kozlowski, K., Donnelly, J., Pendergast, D., Epstein, L., and Willer, B. (2010). A preliminary study of subsymptom threshold exercise training for refractory post-concussion syndrome. Clin. J. Sport Med. 20, 21–27. doi: 10.1097/JSM.0b013e3181c6c22c
Leddy, J., Master, C., Mannix, R., Wiebe, D., Grady, M., Meehan, W., et al. (2021). Early targeted heart rate aerobic exercise versus placebo stretching for sport-related concussion in adolescents: a randomised controlled trial. Lancet Child. Adolesc. Health 5, 792–799. doi: 10.1016/S2352-4642(21)00267-4
Leddy, J., and Willer, B. (2013). Use of graded exercise testing in concussion and return-to-activity management. Curr. Sports Med. Rep. 12, 370–376. doi: 10.1249/JSR.0000000000000008
Lee, J., and Zhang, X. (2021). Physiological determinants of VO2max and the methods to evaluate it: A critical review. Sci. Sports 36, 259–271. doi: 10.1016/j.scispo.2020.11.006
Levac, D., Colquhoun, H., and O’Brien, K. (2010). Scoping studies: advancing the methodology. Implement Sci. 5:69. doi: 10.1186/1748-5908-5-69
McArdle, W., Katch, F., and Katch, V. (2006). Essentials of Exercise Physiology. Philadelphia, PA: Lippincott Williams & Wilkins.
McDonnell, M., Smith, A., and Mackintosh, S. (2011). Aerobic exercise to improve cognitive function in adults with neurological disorders: a systematic review. Arch. Phys. Med. Rehabil. 92, 1044–1052. doi: 10.1016/j.apmr.2011.01.021
McGeown, J., Zerpa, C., Lees, S., Niccoli, S., and Sanzo, P. (2018). Implementing a structured exercise program for persistent concussion symptoms: a pilot study on the effects on salivary brain-derived neurotrophic factor, cognition, static balance, and symptom scores. Brain Inj. 32, 1556–1565. doi: 10.1080/02699052.2018.1498128
Micay, R., Richards, D., and Hutchison, M. (2018). Feasibility of a postacute structured aerobic exercise intervention following sport concussion in symptomatic adolescents: a randomised controlled study. BMJ Open Sport Exerc. Med. 4, e000404. doi: 10.1136/bmjsem-2018-000404
Morris, T., Costa-Miserachs, D., Rodriguez-Rajo, P., Finestres, J., Bernabeu, M., Gomes-Osman, J., et al. (2018). Feasibility of Aerobic Exercise in the Subacute Phase of Recovery From Traumatic Brain Injury: A Case Series. J. Neurol. Phys. Ther. 42, 268–275. doi: 10.1097/NPT.0000000000000239
Nokia, M., Lensu, S., Ahtiainen, J., Johansson, P., Koch, L., Britton, S., et al. (2016). Physical exercise increases adult hippocampal neurogenesis in male rats provided it is aerobic and sustained. J. Physiol. 594, 1855–1873. doi: 10.1113/JP271552
O’Carroll, G., King, S., Carroll, S., Perry, J., and Vanicek, N. (2020). The effects of exercise to promote quality of life in individuals with traumatic brain injuries: a systematic review. Brain Inj. 34, 1701–1713. doi: 10.1080/02699052.2020.1812117
Pennington, D., Reavis, J., Cano, M., Walker, E., and Batki, S. (2022). The impact of exercise and virtual reality executive function training on cognition among heavy drinking veterans with traumatic brain injury: a pilot feasibility study. Front. Behav. Neurosci. 16:802711. doi: 10.3389/fnbeh.2022.802711
Plowman, S., and Smith, D. (2007). Exercise Physiology for Health, Fitness, and Performance. Philadelphia, PA: Lippincott Williams & Wilkins.
Polak, P., Leddy, J., Dwyer, M., Willer, B., and Zivadinov, R. (2015). Diffusion tensor imaging alterations in patients with postconcussion syndrome undergoing exercise treatment: a pilot longitudinal study. J. Head Trauma Rehabil. 30, E32–E42. doi: 10.1097/HTR.0000000000000037
Rabinowitz, A., Kumar, R., Sima, A., Venkatesan, U., Juengst, S., O’Neil-Pirozzi, T., et al. (2021). Aging with traumatic brain injury: Deleterious effects of injury chronicity are most pronounced in later life. J. Neurotrauma 38, 2706–2713. doi: 10.1089/neu.2021.0038
Romanov, R., Mesarič, L., Perić, D., Vešligaj Damiš, J., and Petrova Filišič, Y. (2021). The effects of adapted physical exercise during rehabilitation in patients with traumatic brain injury. Turk. J. Phys. Med. Rehabil. 67, 482–489. doi: 10.5606/tftrd.2021.6145
Ruegsegger, G., and Booth, F. (2018). Health Benefits of Exercise. Cold Spring Harb. Perspect. Med. 8, a029694. doi: 10.1101/cshperspect.a029694
Schwandt, M., Harris, J., Thomas, S., Keightley, M., Snaiderman, A., and Colantonio, A. (2012). Feasibility and effect of aerobic exercise for lowering depressive symptoms among individuals with traumatic brain injury: a pilot study. J. Head Trauma Rehabil. 27, 99–103. doi: 10.1097/HTR.0b013e31820e6858
Shore, J., Hutchison, M., Nalder, E., Reed, N., and Hunt, A. (2022). Tele-Active Rehabilitation for adolescents with concussion: a feasibility study. BMJ Open Sport Exerc. Med. 8, e001277. doi: 10.1136/bmjsem-2021-001277
Snyder, A., Greif, S., Clugston, J., FitzGerald, D., Yarrow, J., Babikian, T., et al. (2021). The Effect of Aerobic Exercise on Concussion Recovery: A Pilot Clinical Trial. J. Int. Neuropsychol. Soc. 27, 790–804. doi: 10.1017/S1355617721000886
Sophie Su, Y., Veeravagu, A., and Grant, G. (2016). “Neuroplasticity after Traumatic Brain Injury,” in Traumatic Brain Injury, eds Y. Sophie Su, A. Veeravagu, G. Grant, and Translational Research (Boca Raton, FL: CRC Press).
Tefertiller, C., Ketchum, J., Bartelt, P., Peckham, M., and Hays, K. (2022). Feasibility of virtual reality and treadmill training in traumatic brain injury: a randomized controlled pilot trial. Brain Inj. 36, 898–908. doi: 10.1080/02699052.2022.2096258
Tiwari, D., Daly, C., and Alsalaheen, B. (2018). Home-based circuit training program for an adolescent female with severe traumatic brain injury: A case report. Physiother. Theory Pract. 34, 137–145. doi: 10.1080/09593985.2017.1370750
Tomaszczyk, J., Green, N., Frasca, D., Colella, B., Turner, G., Christensen, B., et al. (2014). Negative neuroplasticity in chronic traumatic brain injury and implications for neurorehabilitation. Neuropsychol. Rev. 24, 409–427. doi: 10.1007/s11065-014-9273-6
Tomoto, T., Le, T., Tarumi, T., Dieppa, M., Bell, K., Madden, C., et al. (2022). Carotid Arterial Compliance and Aerobic Exercise Training in Chronic Traumatic Brain Injury: A Pilot Study. J. Head Trauma Rehabil. 37, 263–271. doi: 10.1097/HTR.0000000000000722
Tricco, A., Lillie, E., Zarin, W., O’Brien, K., Colquhoun, H., Levac, D., et al. (2018). PRISMA Extension for Scoping Reviews (PRISMA-ScR): Checklist and Explanation. Ann. Intern. Med. 169, 467–473. doi: 10.7326/M18-0850
van Praag, H., Christie, B., Sejnowski, T., and Gage, F. (1999). Running enhances neurogenesis, learning, and long-term potentiation in mice. Proc. Natl. Acad. Sci. U. S. A. 96, 13427–13431. doi: 10.1073/pnas.96.23.13427
Varner, C., Thompson, C., de Wit, K., Borgundvaag, B., Houston, R., and McLeod, S. (2021). A randomized trial comparing prescribed light exercise to standard management for emergency department patients with acute mild traumatic brain injury. Acad. Emerg. Med. 28, 493–501. doi: 10.1111/acem.14215
Weinstein, A., Chin, L., Collins, J., Goel, D., Keyser, R., and Chan, L. (2017). Effect of Aerobic Exercise Training on Mood in People With Traumatic Brain Injury: A Pilot Study. J. Head Trauma Rehabil. 32, E49–E56. doi: 10.1097/HTR.0000000000000253
Wender, C., Sandroff, B., Krch, D., Wylie, G., Cirnigliaro, C., Wecht, J., et al. (2021). The preliminary effects of moderate aerobic training on cognitive function in people with TBI and significant memory impairment: a proof-of-concept randomized controlled trial. Neurocase 27, 430–435. doi: 10.1080/13554794.2021.1990964
Wieloch, T., and Nikolich, K. (2006). Mechanisms of neural plasticity following brain injury. Curr. Opin. Neurobiol. 16, 258–264. doi: 10.1016/j.conb.2006.05.011
Willer, B., Haider, M., Bezherano, I., Wilber, C., Mannix, R., Kozlowski, K., et al. (2019). Comparison of Rest to Aerobic Exercise and Placebo-like Treatment of Acute Sport-Related Concussion in Male and Female Adolescents. Arch. Phys. Med. Rehabil. 100, 2267–2275. doi: 10.1016/j.apmr.2019.07.003
Wise, E., Hoffman, J., Powell, J., Bombardier, C., and Bell, K. (2012). Benefits of exercise maintenance after traumatic brain injury. Arch. Phys. Med. Rehabil. 93, 1319–1323. doi: 10.1016/j.apmr.2012.05.009
Young, J., Angevaren, M., Rusted, J., and Tabet, N. (2015). Aerobic exercise to improve cognitive function in older people without known cognitive impairment. Cochrane Database Syst. Rev. 2015:CD005381. doi: 10.1002/14651858.CD005381.pub4
Young, J., and Hughes, N. (2020). Traumatic brain injury and homelessness: From prevalence to prevention. Lancet Public Health 5, e4–e5. doi: 10.1016/S2468-2667(19)30225-7
Yuan, W., Wade, S., Quatman-Yates, C., Hugentobler, J., Gubanich, P., and Kurowski, B. (2017). Structural connectivity related to persistent symptoms after mild tbi in adolescents and response to aerobic training: Preliminary investigation. J. Head Trauma Rehabil. 32, 378–384. doi: 10.1097/HTR.0000000000000318
Keywords: traumatic brain injury, aerobic exercise, TBI intervention, aerobic intervention, concussion
Citation: Snowden T, Morrison J, Boerstra M, Eyolfson E, Acosta C, Grafe E, Reid H, Brand J, Galati M, Gargaro J and Christie BR (2023) Brain changes: aerobic exercise for traumatic brain injury rehabilitation. Front. Hum. Neurosci. 17:1307507. doi: 10.3389/fnhum.2023.1307507
Received: 04 October 2023; Accepted: 28 November 2023;
Published: 20 December 2023.
Edited by:
Yu Huang, Soterix Medical Inc., United StatesReviewed by:
Juliet Haarbauer Krupa, Centers for Disease Control and Prevention (CDC), United StatesPatricia Rzezak, University of São Paulo, Brazil
Copyright © 2023 Snowden, Morrison, Boerstra, Eyolfson, Acosta, Grafe, Reid, Brand, Galati, Gargaro and Christie. This is an open-access article distributed under the terms of the Creative Commons Attribution License (CC BY). The use, distribution or reproduction in other forums is permitted, provided the original author(s) and the copyright owner(s) are credited and that the original publication in this journal is cited, in accordance with accepted academic practice. No use, distribution or reproduction is permitted which does not comply with these terms.
*Correspondence: Brian R. Christie, brain64@uvic.ca