- 1Fundación Cardiovascular de Colombia, Bucaramanga, Colombia
- 2Research Group Innovación y Cuidado, Faculty of Nursing, Universidad Antonio Nariño, Neiva, Colombia
- 3Department of Epidemiology, Universidad Surcolombiana, Neiva, Colombia
- 4Department of Psychiatry, Massachusetts General Hospital, Harvard Medical School, Boston, MA, United States
- 5School of Medicine, Universidad de Santander, Bucaramanga, Colombia
Background: Upper limb motor impairment is one of the main complications of stroke, affecting quality of life both for the patient and their family. The aim of this systematic review was to summarize the scientific evidence on the safety and efficacy of Vagus Nerve Stimulation (VNS) on upper limb motor recovery after stroke.
Methods: A systematic review and meta-analysis of studies that have evaluated the efficacy or safety of VNS in stroke patients was performed. The primary outcome was upper limb motor recovery. A search of articles published on MEDLINE, CENTRAL, EBSCO and LILACS up to December 2021 was performed, and a meta-analysis was developed to calculate the overall effects.
Results: Eight studies evaluating VNS effects on motor function in stroke patients were included, of which 4 used implanted and 4 transcutaneous VNS. It was demonstrated that VNS, together with physical rehabilitation, increased upper limb motor function on average 7.06 points (95%CI 4.96; 9.16) as assessed by the Fugl-Meyer scale. Likewise, this improvement was significantly greater when compared to a control intervention (mean difference 2.48, 95%CI 0.98; 3.98). No deaths or serious adverse events related to the intervention were reported. The most frequent adverse events were dysphonia, dysphagia, nausea, skin redness, dysgeusia and pain related to device implantation.
Conclusion: VNS, together with physical rehabilitation, improves upper limb motor function in stroke patients. Additionally, VNS is a safe intervention.
Introduction
Stroke is a neurological condition caused by vascular problems such as cerebral infarction and/or intracerebral or subarachnoid hemorrhage (1). In 2019, more than 12 million strokes occurred worldwide, making it one of the leading causes of morbidity. Stroke is considered the second leading cause of mortality overall and one of the leading causes of disability worldwide, ranking first in people over 50 years of age (2). In the United States, stroke occurs in more than 7 million people, with a prevalence of 2.5 % (3).
Motor impairment occurs in 85% of patients with stroke, and it is considered one of the main problems resulting from this condition (4). Motor affectation in these subjects is characterized by a decreased capacity and strength of muscles, mainly of the upper extremities, diminishing the quality of life of both the patients and their families (5). Recovery of motor function occurs spontaneously during the 1st months after stroke (6) as a result of brain plasticity processes in the sensory and motor systems (7), however, 50 to 75% of these patients persist with significant motor sequelae limiting daily activities (8).
Efforts have been made to develop therapies that can improve motor impairment in stroke patients (9). Among these therapies are: constraint-induced movement (10), mirror therapy (11), and resistance training (12), however, these interventions have a low level of adherence (13) and the evidence supporting their effects is still weak (10–12). Recently, Vagus Nerve Stimulation (VNS) has been proposed as an intervention that could have beneficial effects in the recovery of motor function in these patients, since it contributes to the generation of adaptive neuroplasticity and the activation of neuromodulators that reduce brain inflammation (14, 15).
VNS consists in the activation of the vagus nerve using electrical current, either through the use of implants or extracorporeal electrodes. As the vagus nerve is composed mainly of afferent fibers, it allows the modulation of different brain structures receiving vagal afferent information, such as the nucleus of the solitary tract, locus coeruleus, raphe nuclei and the hypothalamus (16). In experimental animal stroke models, VNS has been shown to reduce infarct volume and improve neurological outcomes (17, 18). It has been proposed that one the mechanisms mediating these neuroprotective effects of VNS in acute cerebral ischemia is the modulation of the cholinergic anti-inflammatory pathway, and more specifically the α7 nicotinic acetylcholine receptor (α7nAChR) (19), a neurotransmitter gated ion channel expressed widely in the brain and on immune cells (20, 21). Activation of these receptors by the vagus nerve leads to a reduction in the release of pro-inflammatory cytokines (21), with beneficial effects on the reduction of infarct size and cerebral edema on experimental models of stroke (19).
In addition, it has been shown that VNS paired with motor training of the extremities, may upregulate cortical plasticity mechanisms that result in motor function recovery after a stroke (22). Following brain injuries affecting the motor or sensory cortices, nearby cortical regions partially regenerate to provide some of the lost functionality (23, 24). The size of the regenerated motor or sensory representation in surrounding cortical areas correlates with functional recovery, however the result gain in functionality is only a fraction of the observed pre-injury levels (24, 25). Previous studies have demonstrated that repeatedly pairing VNS with specific movements results in increased representation of these movements in the primary motor cortex (22). Further animal experiments have provided evidence that the administration of VNS paired with repeated movements of affected limbs after motor cortex damage is associated with a significant recovery of forelimb function that is superior to that observed with physical training alone (26, 27). These potentiating effects of VNS on cortical reorganization mechanisms may be related with the activation of nuclei such as locus coeruleus, raphe nuclei and nucleus basalis. These nuclei generate an increase in neuromodulators important in neuroplasticity, such as noradrenaline, serotonin, brain-derived neurotrophic factor and acetylcholine (28, 29). When these neurotransmitters are simultaneously released during neural activity related with motor rehabilitation, synaptic plasticity is promoted in motor-specific circuits (30). Thus, VNS paired with motor rehabilitation can cause an increased specific reorganization of the motor cortex, resulting in an enhanced motor recovery after cerebral ischemia (27).
VNS has mainly been administered by using implanted electrodes, but more recently, a non-invasive technique, known as transcutaneous VNS (cervical or auricular) has been proposed (31). VNS has traditionally required the implantation of an electrical pulse generator at the left subclavicular level, which is connected to electrodes in the left cervical branch of the vagus nerve (32). Its insertion is performed by a surgical procedure, which presents a higher risk of adverse events (33), the most frequent being dysphonia during stimulation, due to its proximity to the laryngeal nerve (34). On the other hand, transcutaneous VNS works through the placement of non-invasive electrodes on the neck or auricle for stimulation of the cervical or auricular branch of the vagus nerve, respectively (32). Transcutaneous VNS has a lower risk of adverse events, is reversible and easy to implement (32). In addition, experimental evidence suggests that the effects of transcutaneous VNS on brain function are comparable to those obtained with VNS (33). Diverse studies using electrical stimulation of the auricular branch of the vagus nerve in experimental models have shown a significant effect of this technique on the reduction of brain infarct volume (35–37). The magnitude of reduction in infarct size has been similar to the one reported for implanted VNS (18). In addition to these effects, transcutaneous VNS has shown to regulate other mechanisms that can promote recovery of neurological function after ischemic stroke (38, 39). These include upregulation of angiogenesis, which can improve perfusion of the tissue surrounding the injury promoting recovery (40), regulation of blood brain barrier permeability, which could improve cerebral edema after stroke (41), and inhibition of neuroinflammation resulting in neuroprotective effects against ischemic cerebral injuries (37). No animal studies have evaluated the effects of transcutaneous VNS paired with rehabilitation on the recovery of motor function after brain ischemic injury. However, multiple studies have shown beneficial effects of this technique on upregulation of mechanisms involved in neuroplasticity, such as upregulation of brain-derived neurotrophic factor (42). Transcutaneous VNS has also shown to improve axon regeneration and re-organization in experimental models of cerebral ischemia (37), suggesting that this technique may have similar effects to VNS on the mechanisms underlying its beneficial effects on motor recovery after a stroke.
There have been multiple clinical studies that have evaluated the safety and efficacy of implanted and transcutaneous VNS in the recovery of motor function after stroke (34, 43–49), and recently, meta-analyses have suggested that VNS has a positive effect on upper limb function in stroke patients (50–52), however these reviews did not evaluate the effect vagus nerve stimulation according to time since stroke. The aim of this systematic review is to summarize and analyze the scientific evidence of the safety and efficacy of both implanted and transcutaneous VNS for the management of upper limb motor impairment after stroke.
Methods
Search Strategy
The search was performed using the following databases: MEDLINE, CENTRAL, EBSCO and LILACS, without date restriction and was focused on studies conducted in humans. A combination of MeSH terms was used for the search, which were: ((Vagus Nerve Stimulation) OR (Vagus Nerve)) AND (Stroke). The search was conducted in December 2021 and was restricted to articles published in English or Spanish.
Selection Criteria
Studies of patients with acute or chronic stage stroke, where VNS was the intervention, compared to usual care or placebo stimulation, were included. The main outcome was the efficacy of VNS on upper limb motor recovery. Information on mild, moderate and severe adverse events of VNS was also collected to assess safety aspects. Clinical trials were included. Editorials, protocols, letters to the editor, commentaries, and case reports were excluded. Studies that only evaluated neuroplasticity mechanisms, neuromodulator production, cytokine inhibition, or brain infarct volume were also excluded. In order to include all relevant research, we reviewed the references of the included studies and also published abstracts from scientific conferences. In addition, we searched the clinicaltrial.gov website to identify clinical trials of VNS in stroke patients. The first author (JAR) independently reviewed the titles and abstracts for an initial assessment of eligibility criteria. Once the titles and abstracts were reviewed, JAR and DL reviewed the full-text articles to evaluate the inclusion of studies in the analysis. Discrepancies and doubts on the inclusion of articles were resolved by a third investigator (RG).
Data Extraction
Information from the articles was extracted by two reviewers (JAR, DL), using an established format containing the following variables: lead author, year of publication, outcome assessed, type of study, population, intervention assessed, comparison group, results in terms of primary and secondary outcomes, adverse event reporting, and stimulation parameters.
Assessment of Methodological Quality
We assessed the risk of bias of the included clinical trials using the Cochrane Collaboration's domain-based scale, which evaluates allocation concealment, randomization, blinding of participants and investigators, blinding in outcome assessment, selective outcome reporting, and incomplete outcome data.
Synthesis of Information
Qualitative analysis of each of the articles was performed, taking into account the characteristics of the studies, population, intervention, control group, outcomes and adverse event reporting.
Quantitative Analysis
An initial meta-analysis of 6 clinical trials was performed (three implanted and three transcutaneous VNS studies), where the mean difference in upper limb motor recovery between the active and control interventions was assessed. A second meta-analysis evaluated the average increase in motor recovery from baseline and included six clinical trials and one intervention study that had no comparison group, for a total of seven studies (three evaluating implanted VNS and four evaluating transcutaneous VNS). In each meta-analysis, the mean with its 95 % confidence interval was calculated. Care was taken not to duplicate data from clinical trials with more than one publication. A subgroup analysis was performed to determine the difference in the effects according to the VNS technique (implanted vs. transcutaneous) and the mean time since the stroke (more than 3 years versus <3 years). A random-effects model was used for the meta-analysis and heterogeneity was assessed using the I2 statistic, where I2 >60% was considered as significant heterogeneity. All analyses were performed in the RStudio program using the meta library.
Results
The database search yielded 1,316 records; after eliminating duplicates, 723 were selected for title and abstract review. Of these, 700 were excluded mainly because of study design and the lack of stroke as a studied event. In total, 25 research articles were reviewed in full. From these, eight articles met the eligibility criteria for the systematic review (Figure 1). The main reasons for exclusion were the evaluation of outcomes other than those stated in the selection criteria of this review (e.g., evaluation of physiological mechanisms of neuroplasticity or impact on cerebral infarct size).
Study Characteristics
Eight studies evaluating VNS were included, all of them published in English between 2016 and 2021, of which four used implanted and four transcutaneous VNS [cervical (n = 0), auricular stimulation (n = 4)]. The implanted VNS studies analyzed the efficacy of stimulation plus physical rehabilitation compared with patients who received physical rehabilitation therapies plus placebo stimulation or physical therapy alone (34, 47–49). The implanted VNS protocol in all evaluated studies had a duration of 18 sessions distributed over 6 weeks, where stimulation was administered in conjunction with rehabilitation training and used the following parameters: an amplitude of 0.8 mA, a pulse duration of 0.1 ms, a frequency of 30 Hz, and a duration of 0.5 seconds; with stimuli administered during each movement repetition. In general, all patients who received the intervention had a significant improvement in motor function, as assessed by the Fugl-Meyer scale (Table 1). This improvement in motor function persisted significantly up to 90 days after the end of the intervention in two studies (34, 49) (Table 1).
Regarding transcutaneous VNS, all studies used auricular stimulation. The study by Redgrave et al. (45) included 13 patients that had an anterior circulation ischemic stroke at least 3 months previously and had residual upper limb dysfunction. These subjects underwent 18 x 1-hour sessions over 6 weeks in which they received stimulation on the cymba conchae of the left ear concurrently with upper limb repetitive task practice (30–50 repetitions of 7–10 arm movements). Subjects received transcutaneous VNS with a frequency of 25 Hz, a pulse width of 0.1 ms, at maximum tolerated intensity (median intensity = 1.4 mA) during each movement repetition. This study found that transcutaneous VNS improved mean motor mobility at visit 18 (upper limb Fugl-Meyer mean increase = 17.1, SD 7.8), and that 10 patients (83 %) achieved a clinically relevant increase of >10 points on the Fugl-Meyer scale (45).
The study by Capone et al. (46) was a controlled clinical trial with a sample of 14 patients. Patients were randomized to robot-assisted physical therapy sessions associated with active transcutaneous auricular VNS or sham stimulation during 10 consecutive working days. Stimulation consisted of pulse trains lasting 20 s, with a pulse width of 0.3 ms and a frequency of 20 Hz, repeated every 5 min for 60 min. Patients in the transcutaneous auricular VNS group received the stimulation with electrodes placed in the left external acoustic meatus at the inner side of the tragus, whereas for those in the control group, electrodes were attached to the left ear lobe. The intensity of the stimulation was adjusted to a level between the detection and pain thresholds. Robotic-assisted therapy was delivered immediately after the end of real or sham transcutaneous VNS. In this study, the active intervention was found to improve upper extremity motor mobility (Fugl-Meyer scores) after 2 weeks of treatment as compared to the sham group (Mann-Whitney U = 5.00, p = 0.048) (34) (Table 1). Additionally, no adverse events were reported, and patients reported comfort and convenience during the intervention.
Wu et al. (43) evaluated the efficacy of transcutaneous auricular VNS in 10 patients with a history of ischemic stroke that occurred between 0.5 and 3 months compared with 11 patients that received sham stimulation. The active transcutaneous auricular VNS was delivered with electrodes fitted to the left cymba concha and consisted of pulse trains lasting 30 s, with a pulse width of 0.3 ms and a frequency of 20 Hz, repeated every 5 minutes for 30 minutes. The control group received sham stimulation (location= left cymba concha, intensity = 0 mA). Both groups received rehabilitation therapy performed after the end of the stimulation. In the study, the intervention group was found to significantly increase on FMA-UE score compared with the control group (6.9 [SD 1.85] vs. 3.18 [SD 1.17]); between group difference= 3.72, 95%CI 2.32; 5.12, p < 0.001) (Table 1).
Chang et al. (44) is the most recent study that evaluated the efficacy of the transcutaneous auricular VNS on the upper limb motor function. In this clinical trial, the authors included 34 patients with unilateral supratentorial chronic (>6 months) stroke. The intervention consisted of transcutaneous auricular VNS (location = left cymba concha, frequency = 30 Hz, pulse width = 0.3 ms, intensity = maximum tolerable level) with robotic therapy 3 days per week, for 3 weeks (nine sessions). The control group received sham stimulation (location = left cymba concha, intensity = 0 ma) with robotic therapy 3 days per week, for 3 weeks (nine sessions). The study found that at discharge, the FMA-UE score was increased in the intervention group compared with the control group (3.10 [SEM 0.57] vs. 2.86 [SEM 0.50]) (Table 1).
An initial meta-analysis was performed to evaluate the effects of active VNS vs. a control intervention on motor recovery after stroke. From the eight studies one was excluded because it did not have a control group (45), and one more (48) because reported data from clinical trials with a publication already included in the analysis. This meta-analysis revealed that motor recovery, as measured by change in the Fugl-Meyer assessment score, was significantly greater in the active group when compared to those subjects receiving the control intervention (mean difference 2.48, 95%CI 0.98; 3.98) (Figure 2). In a second meta-analysis, including the study that did not have a control group (45), it was observed that the intervention increased upper limb motor function (Fugl-Meyer scores) by an average of 7.06 (95%CI 4.96; 9.16) points when compared to baseline (Figure 3). An analysis by subgroups in meta-analysis #1 did not show clear differences (Figure 4). The analysis by subgroups in meta-analysis #2 showed that studies where transcutaneous VNS (Figure 5) was used or included participants with a lower average time since stroke (<3 years) (Figure 6) were associated with greater effects in motor recovery.
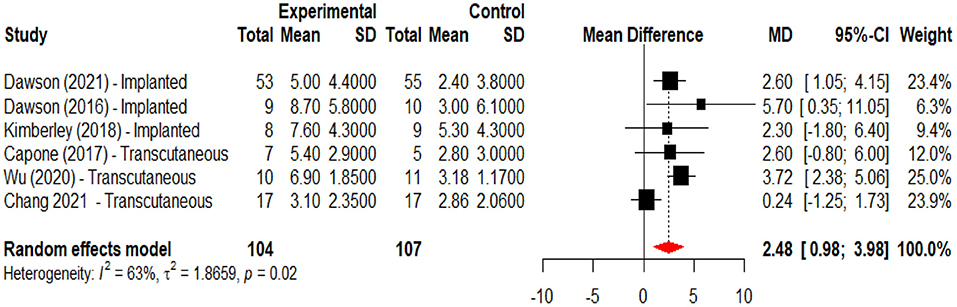
Figure 2. Forest plot for the meta-analysis of vagus nerve stimulation effects on upper limb motor function (FMA-UE score increase) when compared to a control intervention. Dawson et al. (34), Dawson et al. (47), and Kimberley et al. (47) used implanted stimulation, Capone et al. (46), Wu et al. (43), and Chang et al. (44) used transcutaneous stimulation.
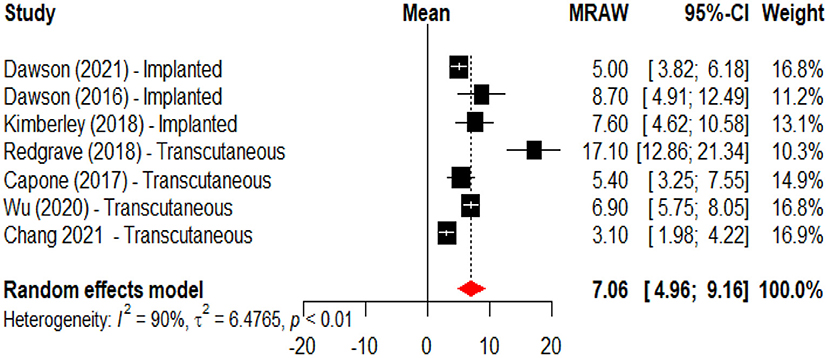
Figure 3. Forest plot for the meta-analysis of vagus nerve stimulation effects on upper limb motor function (FMA-UE score increase) when compared to baseline. Dawson et al. (34), Dawson et al. (47), and Kimberley et al. (47) used implanted VNS, Capone et al. (46), Redgrave et al. (45), Wu et al. (43), and Chang et al. (44) used transcutaneous stimulation.
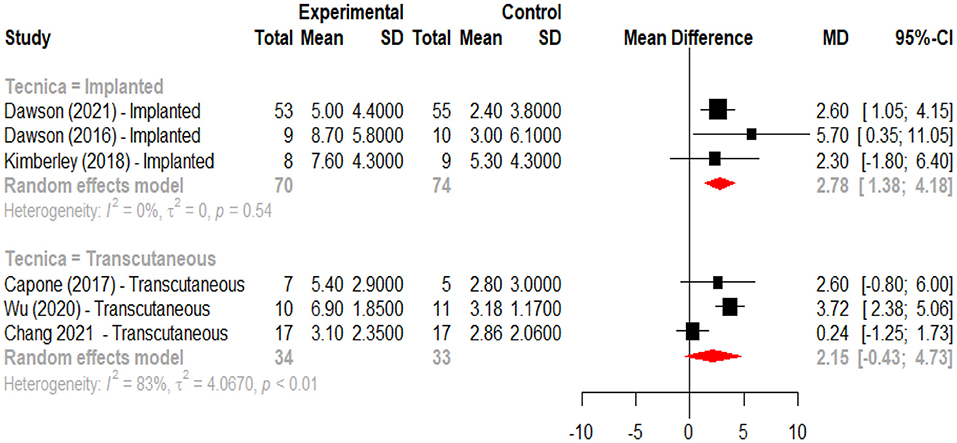
Figure 4. Forest plot for the meta-analysis of vagus nerve stimulation effects on upper limb motor function (FMA-UE score increase) when compared to a control intervention according to intervention modality (implanted vs. transcutaneous).
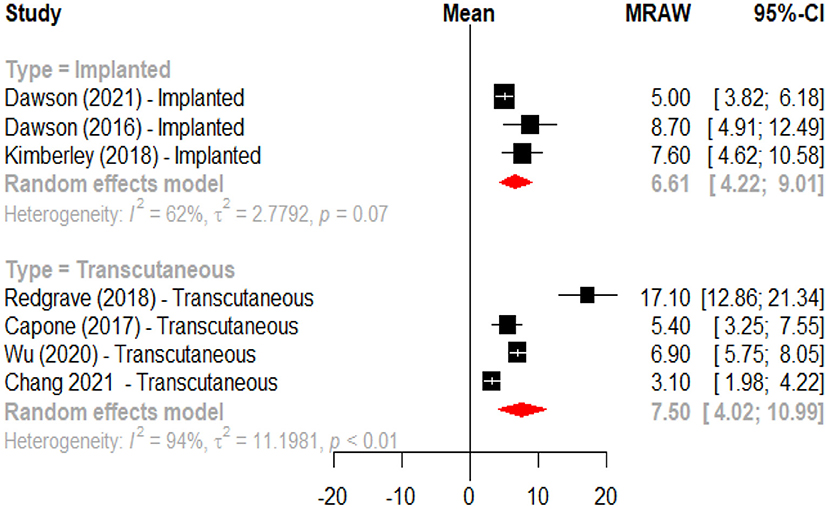
Figure 5. Forest plot for the meta-analysis of vagus nerve stimulation effects on upper limb motor function (FMA-UE score increase) when compared to baseline according to intervention modality (implanted vs. transcutaneous).
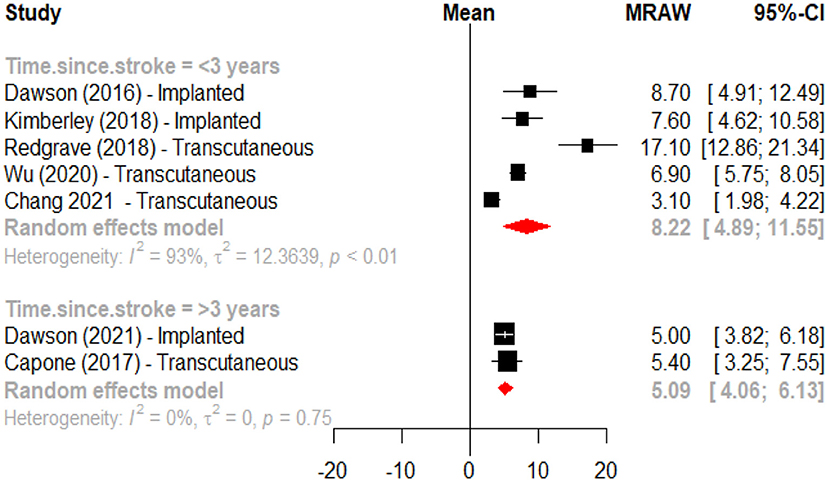
Figure 6. Forest plot for the meta-analysis of vagus nerve stimulation effects on upper limb motor function (FMA-UE score increase) when compared to baseline according to time since stroke (<3 years vs. ≥3 years).
Safety
No intervention-related deaths or serious adverse effects (AEs) were reported. The most frequent moderate AE associated with implanted VNS was left vocal cord paralysis associated with or without dysphonia (11.11 % in Dawson et al. and 5.88 % in Kimberley et al.) (47, 49); The most frequent moderate AE associated with the device implantation procedure, present in one patient, was surgical site infection requiring intravenous antibiotic treatment (49). The most frequent mild AEs related to stimulation therapy were, in order: dysphonia, dysphagia, nausea and dysgeusia. None of the mild AEs required changes in therapy protocol and all of them self-resolved during the follow-up period (34, 47, 49). Four studies reported AEs in transcutaneous VNS, observing fatigue, dizziness, ear pain, skin redness and tiredness (43, 45, 53). In the study by Capone et al. (transcutaneous VNS) there were no adverse events and patients did not report discomfort from the procedure (46). One study that monitored heart rate and blood pressure levels showed no clinically significant change throughout the treatment sessions (43).
Risk of Bias Assessment
The risk of bias assessment was applied to the six clinical trials (Dawson et al., Dawson et al., Kimberley et al., Capone, et al., Wu et al., Chang et al.) (34, 43, 44, 46, 47, 49) upon which the initial meta-analysis for the assessment of VNS efficacy for motor rehabilitation was based. All studies had low risk of bias in the selective reporting domain. The performance bias domain had the highest risk of bias with two studies (Dawson et al., Wu et al.) (43, 47) (Figure 7). In the clinical trial reported by Capone et al. (46), the risk of bias in the domains of randomization, and allocation concealment was unclear, and the risk of attrition bias was high (Figure 7).
Discussion
This systematic review and meta-analysis demonstrate that VNS is an effective therapy for upper limb motor recovery in stroke patients. Factors such as the VNS technique used and the time of intervention since the event seem to have an influence on the results obtained, with greater benefits if the stimulation is performed non-invasively and prior to 3 years after the event. However, the studies performed so far with transcutaneous stimulation have included a limited number of patients, therefore more evidence is needed before a definitive conclusion can be reached in this regard. The performed studies have shown a low rate of adverse events, so it can be concluded that VNS is a safe procedure for the management of this pathology. The incidence and severity of adverse events depend on whether the stimulation is performed with an implanted device or with a non-invasive technique, since the former has a higher risk of moderate adverse events such as vocal cord paralysis and surgical site infection associated with the implantation procedure, whereas for the transcutaneous technique the adverse events reported were all mild (e.g., fatigue, dizziness, ear pain and tiredness).
Previous clinical studies have demonstrated the efficacy of VNS for the treatment of migraine, anxiety symptoms, depression and epilepsy (54). In this systematic review, VNS together with physical rehabilitation was found to significantly improve upper limb motor function when compared with rehabilitation alone; a similar result to that reported in recently published meta-analyses (50–52). VNS together with physical therapy increases upper limb motor recovery of stroke patients by an average of 7 points in the Fugl-Meyer scale, which could be considered as a clinically significant response (55). In some of the reviewed studies with implanted VNS, a clinically significant response was found in 47 to 88% of patients up to 90 days after the end of in-clinic therapy, supporting potential sustained effects of the intervention on motor recovery after stroke (34, 49).
Results from this meta-analysis suggest that implementation of this intervention at earlier stages of the post-stroke recovery process could have a significantly greater effect in motor rehabilitation. Studies that in included participants where the intervention was, in average, initiated <3 years after the stroke had an estimated increase of eight points in the Fugl-Meyer scale after VNS and motor rehabilitation compared to an estimated increase of five points in the studies that included participants with an average of more than 3 years since the event. Only one study included patients in the sub-acute phase of stroke rehabilitation Wu et al. (43). This study found an average increase of 6.9 points in the Fugl-Meyer scale after 15 days of therapy, which increased to 7.7 points 4 weeks after therapy and was significantly greater than the change observed in the sham group. Given that most of the cortical reorganization processes are expected to occur during the sub-acute phase post stroke (56), this may be the optimal window of recovery to be modulated by the implementation of VNS in combination with physical therapy, however, future clinical studies with larger sample sizes will be necessary to confirm whether earlier administration of this intervention is associated with greater improvement in motor function.
This systematic review identifies several knowledge gaps that should be evaluated in further studies. First, although initial results from studies evaluating transcutaneous VNS are promising, more clinical trials evaluating this technique with larger sample sizes and appropriate control interventions are required to determine a more accurate effect size of this technique in motor recovery after stroke. Other variables that need to be studied include the definition of optimal stimulation parameters and treatment duration, as well as the appropriate timing for the combination of the stimuli with physical rehabilitation protocols. In addition, future studies will need to evaluate whether VNS has differential effects according to the compromised vascular region, severity of the lesion and stroke subtype (e.g., lacunar vs. non-lacunar) among other clinical characteristics that could impact the effectiveness of this intervention.
The systematic review and meta-analysis have some limitations that are important to mention. First, the number of clinical trials, was very low, and one of the included studies had no comparison group. A high statistical heterogeneity between studies was also identified. There are some sources of heterogeneity that could not be evaluated, for example, the day of primary outcome evaluation, physical rehabilitation protocol parameters, the severity of the lesion, and the vascular region affected by the stroke, among others.
We conclude that VNS together with physical rehabilitation improves upper limb motor function in stroke patients. Additionally, VNS is a safe intervention. More studies are needed to evaluate the efficacy and effectiveness of transcutaneous VNS in patients with stroke and to evaluate optimization of its effect according to the timing of the intervention and the use of more effective stimulation parameters.
Data Availability Statement
The original contributions presented in the study are included in the article/supplementary material, further inquiries can be directed to the corresponding author/s.
Author Contributions
JR-C independently reviewed the titles and abstracts for an initial assessment of study eligibility criteria. Once the titles and abstracts were reviewed. JR-C and DL-F reviewed the full-text articles to evaluate the inclusion of studies in the analysis. Discrepancies and doubts on the inclusion of articles were resolved by a third investigator RG. JR-C and RG wrote the initial version of the manuscript. JR-C, DL-F, SS-B, and FS-S reviewed the article and made significant contributions to the interpretation of results. All authors contributed to the article and approved the submitted version.
Funding
This research was conducted with support from the Ministry of Science, Technology and Innovation of Colombia Minciencias (Projects Nos. 656684368671, 656674555082, and 69/2021). Writing style review was supported by Universidad Antonio Nariño.
Conflict of Interest
The authors declare that the research was conducted in the absence of any commercial or financial relationships that could be construed as a potential conflict of interest.
Publisher's Note
All claims expressed in this article are solely those of the authors and do not necessarily represent those of their affiliated organizations, or those of the publisher, the editors and the reviewers. Any product that may be evaluated in this article, or claim that may be made by its manufacturer, is not guaranteed or endorsed by the publisher.
References
1. Sacco RL, Kasner SE, Broderick JP, Caplan LR, Connors JJ, Culebras A, et al. An updated definition of stroke for the 21st century: a statement for healthcare professionals from the American heart association/American stroke association. Stroke. (2013) 44:2064–89. doi: 10.1161/STR.0b013e318296aeca
2. Global Global burden of 369 diseases and injuries in 204 countries and territories 1990–2019: 1990–2019: a systematic analysis for the global burden of disease study 2019. Lancet. (2020) 396(10258):1204–22. doi: 10.1016/s0140-6736(20)30925-9
3. Benjamin EJ, Muntner P, Alonso A, Bittencourt MS, Callaway CW, Carson AP, et al. Heart disease and stroke statistics-2019 update: a report from the American heart association. Circulation. (2019) 139:e56–e528. doi: 10.1161/cir.0000000000000659
4. Nakayama H, Jørgensen HS, Raaschou HO, Olsen TS. Recovery of upper extremity function in stroke patients: the Copenhagen stroke study. Arch Phys Med Rehabil. (1994) 75:394–8. doi: 10.1016/0003-9993(94)90161-9
5. Opara JA, Jaracz K. Quality of life of post-stroke patients and their caregivers. J Med Life. (2010) 3:216–20.
6. Cramer SC. Repairing the human brain after stroke: I. Mechanisms of spontaneous recovery. Annals of neurology. (2008) 63:272–87. doi: 10.1002/ana.21393
7. Dancause N, Nudo RJ. Shaping plasticity to enhance recovery after injury. Progress Brain Res. (2011) 192:273–95. doi: 10.1016/b978-0-444-53355-5.00015-4
8. Kong KH, Chua KS, Lee J. Recovery of upper limb dexterity in patients more than 1 year after stroke: frequency, clinical correlates and predictors. NeuroRehabilitation. (2011) 28:105–11. doi: 10.3233/nre-2011-0639
9. Stinear C, Ackerley S, Byblow W. Rehabilitation is initiated early after stroke, but most motor rehabilitation trials are not: a systematic review. Stroke. (2013) 44:2039–45. doi: 10.1161/strokeaha.113.000968
10. Etoom M, Hawamdeh M, Hawamdeh Z, Alwardat M, Giordani L, Bacciu S, et al. Constraint-induced movement therapy as a rehabilitation intervention for upper extremity in stroke patients: systematic review and meta-analysis. Int J Rehabil Res Internationale Zeitschrift fur Rehabilitationsforschung Revue internationale de recherches de readaptation. (2016) 39:197–210. doi: 10.1097/mrr.0000000000000169
11. Nogueira N, Parma JO, Leão S, Sales IS, Macedo LC, Galvão A, et al. Mirror therapy in upper limb motor recovery and activities of daily living, and its neural correlates in stroke individuals: a systematic review and meta-analysis. Brain Res Bull. (2021) 177:217–38. doi: 10.1016/j.brainresbull.2021.10.003
12. Veldema J, Jansen P. Resistance training in stroke rehabilitation: systematic review and meta-analysis. Clin Rehabil. (2020) 34:1173–97. doi: 10.1177/0269215520932964
13. Page SJ, Levine P, Sisto S, Bond Q, Johnston MV. Stroke patients' and therapists' opinions of constraint-induced movement therapy. Clin Rehabil. (2002) 16:55–60. doi: 10.1191/0269215502cr473oa
14. van der Meij A, Wermer MJH. Vagus nerve stimulation: a potential new treatment for Ischaemic stroke. Lancet. (2021) 397:1520–1. doi: 10.1016/s0140-6736(21)00667-x
15. Ma J, Qiao P, Li Q, Wang Y, Zhang L, Yan LJ, et al. Vagus nerve stimulation as a promising adjunctive treatment for ischemic stroke. Neurochem Int. (2019) 131:104539. doi: 10.1016/j.neuint.2019.104539
16. Yuan H, Silberstein SD. Vagus nerve and vagus nerve stimulation, a comprehensive review: Part I. Headache. (2016) 56:71–8. doi: 10.1111/head.12647
17. Ay I, Lu J, Ay H, Gregory Sorensen A. Vagus nerve stimulation reduces infarct size in rat focal cerebral ischemia. Neurosci Lett. (2009) 459:147–51. doi: 10.1016/j.neulet.2009.05.018
18. Ay I, Sorensen AG, Ay H. Vagus nerve stimulation reduces infarct size in rat focal cerebral ischemia: an unlikely role for cerebral blood flow. Brain Res. (2011) 1392:110–5. doi: 10.1016/j.brainres.2011.03.060
19. Lu XX, Hong ZQ, Tan Z, Sui MH, Zhuang ZQ, Liu HH, et al. Nicotinic acetylcholine receptor alpha7 subunit mediates vagus nerve stimulation-induced neuroprotection in acute permanent cerebral ischemia by a7nAchR/JAK2 pathway. Med Sci Monit Int Med J Exp Clin Res. (2017) 23:6072–81. doi: 10.12659/msm.907628
20. Wang H, Yu M, Ochani M, Amella CA, Tanovic M, Susarla S, et al. Nicotinic acetylcholine receptor alpha7 subunit is an essential regulator of inflammation. Nature. (2003) 421:384–8. doi: 10.1038/nature01339
21. Bonaz B, Sinniger V, Pellissier S. Anti-inflammatory properties of the vagus nerve: potential therapeutic implications of vagus nerve stimulation. J Physiol. (2016) 594:5781–90. doi: 10.1113/jp271539
22. Porter BA, Khodaparast N, Fayyaz T, Cheung RJ, Ahmed SS, Vrana WA, et al. Repeatedly pairing Vagus nerve stimulation with a movement reorganizes primary motor cortex. Cereb Cort. (2012) 22:2365–74. doi: 10.1093/cercor/bhr316
23. Conner JM, Chiba AA, Tuszynski MH. The basal forebrain cholinergic system is essential for cortical plasticity and functional recovery following brain injury. Neuron. (2005) 46:173–9. doi: 10.1016/j.neuron.2005.03.003
24. Castro-Alamancos MA, Borrel J. Functional recovery of forelimb response capacity after forelimb primary motor cortex damage in the rat is due to the reorganization of adjacent areas of cortex. Neuroscience. (1995) 68:793–805. doi: 10.1016/0306-4522(95)00178-l
25. Ramanathan D, Conner JM, Tuszynski MH A. form of motor cortical plasticity that correlates with recovery of function after brain injury. Proc Natl Acad Sci USA. (2006) 103:11370–5. doi: 10.1073/pnas.0601065103
26. Hays SA, Ruiz A, Bethea T, Khodaparast N, Carmel JB, Rennaker RL. 2nd, et al. Vagus nerve stimulation during rehabilitative training enhances recovery of forelimb function after ischemic stroke in aged rats. Neurobiol Aging. (2016) 43:111–8. doi: 10.1016/j.neurobiolaging.2016.03.030
27. Engineer ND, Kimberley TJ, Prudente CN, Dawson J, Tarver WB, Hays SA. Targeted Vagus nerve stimulation for rehabilitation after stroke. Front Neurosci. (2019) 13:280. doi: 10.3389/fnins.2019.00280
28. Furmaga H, Carreno FR, Frazer A. Vagal nerve stimulation rapidly activates brain-derived neurotrophic factor receptor TrkB in rat brain. PLoS ONE. (2012) 7:e34844. doi: 10.1371/journal.pone.0034844
29. Jiang Y, Li L, Ma J, Zhang L, Niu F, Feng T, et al. Auricular vagus nerve stimulation promotes functional recovery and enhances the post-ischemic angiogenic response in an ischemia/reperfusion rat model. Neurochem Int. (2016) 97:73–82. doi: 10.1016/j.neuint.2016.02.009
30. Hays SA, Rennaker RL, Kilgard MP. Targeting plasticity with vagus nerve stimulation to treat neurological disease. Prog Brain Res. (2013) 207:275–99. doi: 10.1016/b978-0-444-63327-9.00010-2
31. Ventureyra EC. Transcutaneous vagus nerve stimulation for partial onset seizure therapy. A new concept Childs Nerv Syst. (2000) 16:101–2. doi: 10.1007/s003810050021
32. Yap JYY, Keatch C, Lambert E, Woods W, Stoddart PR, Kameneva T. critical review of transcutaneous vagus nerve stimulation: challenges for translation to clinical practice. Front Neurosci. (2020) 14:284. doi: 10.3389/fnins.2020.00284
33. Yuan H, Silberstein SD. Vagus nerve and Vagus nerve stimulation, a comprehensive review: part II. Headache. (2016) 56:259–66. doi: 10.1111/head.12650
34. Dawson J, Liu CY, Francisco GE, Cramer SC, Wolf SL, Dixit A, et al. Vagus nerve stimulation paired with rehabilitation for upper limb motor function after ischaemic stroke (VNS-REHAB): a randomised, blinded, pivotal, device trial. Lancet. (2021) 397:1545–53. doi: 10.1016/s0140-6736(21)00475-x
35. Ay I, Napadow V, Ay H. Electrical stimulation of the vagus nerve dermatome in the external ear is protective in rat cerebral ischemia. Brain Stimul. (2015) 8:7–12. doi: 10.1016/j.brs.2014.09.009
36. Zhao JJ, Wang ZH, Zhang YJ, Wang WJ, Cheng AF, Rong PJ, et al. The mechanisms through which auricular vagus nerve stimulation protects against cerebral ischemia/reperfusion injury. Neural Regenerat Res. (2022) 17:594–600. doi: 10.4103/1673-5374.320992
37. Li J, Zhang Q, Li S, Niu L, Ma J, Wen L, et al. α7nAchR mediates transcutaneous auricular vagus nerve stimulation-induced neuroprotection in a rat model of ischemic stroke by enhancing axonal plasticity. Neurosci Lett. (2020) 730:135031. doi: 10.1016/j.neulet.2020.135031
38. Li L, Wang D, Pan H, Huang L, Sun X, He C, et al. Non-invasive Vagus nerve stimulation in cerebral stroke: current status and future perspectives. Front Neurosci. (2022) 16:820665. doi: 10.3389/fnins.2022.820665
39. Baig SS, Kamarova M, Ali A, Su L, Dawson J, Redgrave JN, et al. Transcutaneous Vagus nerve stimulation (tVNS) in stroke: the evidence, challenges and future directions. Auton Neurosci. (2022) 237:102909. doi: 10.1016/j.autneu.2021.102909
40. Ma J, Zhang L, He G, Tan X, Jin X, Li C. Transcutaneous auricular vagus nerve stimulation regulates expression of growth differentiation factor 11 and activin-like kinase 5 in cerebral ischemia/reperfusion rats. J Neurol Sci. (2016) 369:27–35. doi: 10.1016/j.jns.2016.08.004
41. Yang Y, Yang LY, Orban L, Cuylear D, Thompson J, Simon B, et al. Non-invasive vagus nerve stimulation reduces blood-brain barrier disruption in a rat model of ischemic stroke. Brain Stimul. (2018) 11:689–98. doi: 10.1016/j.brs.2018.01.034
42. Li J, Zhang K, Zhang Q, Zhou X, Wen L, Ma J, et al. PPAR-γ mediates Ta-VNS-induced angiogenesis and subsequent functional recovery after experimental stroke in rats. Biomed Res Int. (2020) 2020:8163789. doi: 10.1155/2020/8163789
43. Wu D, Ma J, Zhang L, Wang S, Tan B, Jia G. Effect and safety of transcutaneous auricular Vagus nerve stimulation on recovery of upper limb motor function in subacute Ischemic stroke patients: a randomized pilot study. Neural Plast. (2020) 2020:8841752. doi: 10.1155/2020/8841752
44. Chang JL, Coggins AN, Saul M, Paget-Blanc A, Straka M, Wright J, et al. Transcutaneous auricular Vagus nerve stimulation (tAVNS) delivered during upper limb interactive robotic training demonstrates novel antagonist control for reaching movements following stroke. Front Neurosci. (2021) 15:767302. doi: 10.3389/fnins.2021.767302
45. Redgrave JN, Moore L, Oyekunle T, Ebrahim M, Falidas K, Snowdon N, et al. Transcutaneous auricular vagus nerve stimulation with concurrent upper limb repetitive task practice for poststroke motor recovery: a pilot study. J Stroke Cerebrovasc Dis: Off J Nat Stroke Assoc. (2018) 27:1998–2005. doi: 10.1016/j.jstrokecerebrovasdis.2018.02.056
46. Capone F, Miccinilli S, Pellegrino G, Zollo L, Simonetti D, Bressi F, et al. Transcutaneous Vagus nerve stimulation combined with robotic rehabilitation improves upper limb function after stroke. Neural Plast. (2017) 2017:7876507. doi: 10.1155/2017/7876507
47. Dawson J, Pierce D, Dixit A, Kimberley TJ, Robertson M, Tarver B, et al. Safety, feasibility, and efficacy of Vagus nerve stimulation paired with upper-limb rehabilitation after Ischemic stroke. Stroke. (2016) 47:143–50. doi: 10.1161/strokeaha.115.010477
48. Dawson J, Engineer ND, Prudente CN, Pierce D, Francisco G, Yozbatiran N, et al. Vagus nerve stimulation paired with upper-limb rehabilitation after stroke: one-year follow-up. Neurorehabil Neural Repair. (2020) 34:609–15. doi: 10.1177/1545968320924361
49. Kimberley TJ, Pierce D, Prudente CN, Francisco GE, Yozbatiran N, Smith P, et al. Vagus nerve stimulation paired with upper limb rehabilitation after chronic stroke. Stroke. (2018) 49:2789–92. doi: 10.1161/strokeaha.118.022279
50. Wei J, Zhang C, Wang JX, Sun FH, Xie YJ, Ou X, et al. The effect of VNS on the rehabilitation of stroke: a meta-analysis of randomized controlled studies. J Clin Neurosci: Off J Neurosurg Soc Au. (2020) 81:421–5. doi: 10.1016/j.jocn.2020.09.022
51. Zhao K, Yang J, Huang J, Zhao Z, Qu Y. Effect of vagus nerve stimulation paired with rehabilitation for upper limb function improvement after stroke: a systematic review and meta-analysis of randomized controlled trials. Int J Rehabil Res Internationale Zeitschrift fur Rehabilitationsforschung Revue internationale de recherches de readaptation. (2021) 3:509. doi: 10.1097/mrr.0000000000000509
52. Xie YL, Wang S, Wu Q, Chen X. Vagus nerve stimulation for upper limb motor impairment after ischemic stroke: a meta-analysis. Medicine. (2021) 100:e27871. doi: 10.1097/md.0000000000027871
53. Song GF, Wang HY, Wu CJ Li X, Yang FY A. retrospective study of transcutaneous Vagus nerve stimulation for post-stroke epilepsy. Medicine. (2018) 97:e11625. doi: 10.1097/md.0000000000011625
54. Yuan H, Silberstein SD. Vagus nerve and Vagus nerve stimulation, a comprehensive review: part III. Headache. (2016) 56:479–90. doi: 10.1111/head.12649
55. Page SJ, Fulk GD, Boyne P. Clinically important differences for the upper-extremity Fugl-Meyer Scale in people with minimal to moderate impairment due to chronic stroke. Phys Ther. (2012) 92:791–8. doi: 10.2522/ptj.20110009
56. Xia Y, Huang G, Quan X, Qin Q, Li H, Xu C, et al. Dynamic structural and functional reorganizations following motor stroke. Med Sci Monit Int Med J Exp Clin Res. (2021) 27:e929092. doi: 10.12659/msm.929092
Keywords: vagus nerve, vagus nerve stimulation, transcutaneous vagus nerve stimulation, stroke, rehabilitation
Citation: Ramos-Castaneda JA, Barreto-Cortes CF, Losada-Floriano D, Sanabria-Barrera SM, Silva-Sieger FA and Garcia RG (2022) Efficacy and Safety of Vagus Nerve Stimulation on Upper Limb Motor Recovery After Stroke. A Systematic Review and Meta-Analysis. Front. Neurol. 13:889953. doi: 10.3389/fneur.2022.889953
Received: 04 March 2022; Accepted: 13 June 2022;
Published: 01 July 2022.
Edited by:
Fivos Panetsos, Complutense University of Madrid, SpainReviewed by:
Daniel Gonzalez Nieto, Polytechnic University of Madrid, SpainEugenijus Kaniusas, Vienna University of Technology, Austria
Copyright © 2022 Ramos-Castaneda, Barreto-Cortes, Losada-Floriano, Sanabria-Barrera, Silva-Sieger and Garcia. This is an open-access article distributed under the terms of the Creative Commons Attribution License (CC BY). The use, distribution or reproduction in other forums is permitted, provided the original author(s) and the copyright owner(s) are credited and that the original publication in this journal is cited, in accordance with accepted academic practice. No use, distribution or reproduction is permitted which does not comply with these terms.
*Correspondence: Jorge A. Ramos-Castaneda, joramos98@uan.edu.co