Bleeding During Veno-Venous ECMO: Prevention and Treatment
- 1Department of Anesthesiology and Critical Care, Faculty of Medicine, Medical Center—University of Freiburg, Freiburg im Breisgau, Germany
- 2Division of Pediatric Hematology and Oncology, Department of Pediatrics and Adolescent Medicine, Faculty of Medicine, Medical Center—University of Freiburg, Freiburg im Breisgau, Germany
Veno-venous extracorporeal membrane oxygenation (vvECMO) has become a routine treatment for severe lung failure in specialized centers. Spontaneous bleeding complications, however, are observed in 30–60% of patients during vvECMO treatment. Bleeding increases mortality by factors 2–3. Anticoagulation in combination with several acquired bleeding disorders caused by the mechanical pump and the foreign layer of the extracorporeal system contribute to the risk of bleeding. In this review, the mechanisms of the underlying pathologies and the route from diagnosis to treatment are described.
Introduction and History
Several intra- and extra pulmonary triggers can cause pulmonary inflammation leading to lung failure and consecutive acute respiratory distress (1). Today, the different etiologies and the severity are summarized as acute respiratory distress syndrome (ARDS) (2). When a severe form of ARDS develops, mechanical lung-protective (3) ventilation alone may not sufficiently supply patients with oxygen or adequately support them to eliminate carbon dioxide. Increasing pressure or oxygen concentration at the ventilator will probably aggravate the problem: the more invasive ventilator settings are adjusted, the more damage will be caused to the lungs (ventilator-induced lung injury, VILI) (4). To overcome this vicious circle, Hill and Bramson presented the first system for bedside extracorporeal carbon dioxide removal, the Bramson membrane lung in 1972 (5). The Bramson membrane lung was a huge machine connected to the patient in a veno-arterial approach to support him for 3 days. During this period, 10 units of packed red blood cells, 8 units of frozen plasma, and 13 units of platelet concentrates had to be transfused. In the 1970s, the idea of extracorporeal lung support was evaluated in clinical trials (6); however, it took several years to establish lung support as veno-venous rather than veno-arterial procedure (7). In the following years, “extracorporeal membrane oxygenation” (ECMO) was applied only in several specialized centers worldwide, because the invasive procedure to implant the cannulas, the thromboembolic complications, and the short half-life of the components prevented this procedure from becoming routine. The coincidence of two different events around the year 2010 paved the way for the renaissance of extracorporeal lung support: The worldwide influenza A H1N1 pandemic causing severe respiratory failure in thousands of patients (8) and the discovery of polymethylpentene (9) as ideal material to produce cheap, effective, and long-lasting fibers for oxygenator membranes (10). Veno-venous ECMO (vvECMO) provided a survival benefit for well-selected patients with severe ARDS during the H1N1 pandemic (8, 11). In a meta-analysis including two prospective randomized trials performed before the SARS-CoV2-pandemic, 90-day mortality was significantly lowered by vvECMO compared with conventional management in patients with severe ARDS (12).
Modern vvECMO systems drain venous blood from the inferior or superior cava vein or the right atrium by negative pressure, accelerate the blood in a centrifugal pump, and press the blood with positive pressure along a membrane made of hollow fibers in which gas exchange takes place (13–15). After oxygenation and decarboxylation, the blood is returned into the right atrium, right ventricle, or even in the pulmonary artery, defined by the type of cannulas used (10, 16–19). The same systems but with different cannulation sites may be applied in veno-arterial ECMO, used for heart, and lung support (20). As several other issues complicate perfusion, turbulences, and coagulation in veno-arterial systems leading to various additional aspects, this article exclusively focuses on coagulation disorders during vvECMO.
According to findings from Kalbhenn, Zieger et al. typical clinical bleeding at vvECMO can be attributed primarily to severe disorders of primary hemostasis (21–23). First described between 2014 and 2018 in intensive care patients at Freiburg University Medical Center, acquired von Willebrand syndrome (AVWS), thrombocytopenia, and platelet dysfunction are characteristic findings that can be detected in virtually all vvECMO patients. Secondary waste coagulopathy with a deficiency of fibrinogen and factor XIII becomes apparent. The blood flow through a vvECMO system generates negative pressures of up to minus 200 mmHg in the supplying tube system. A positive pressure is then generated in the pump which can be up to plus 200 mmHg depending on the tube diameter, hematocrit, and pump speed. In the pump, but also when flowing through oxygenators or by pressing the recirculated blood through the cannula which narrows for technical reasons, considerable turbulence and tensions are generated that affect the blood components. Shear forces unfold the von Willebrand factor (vWF) high-molecular-weight (HMW) multimers and thus present the A2 domain at which a specific metalloprotease [A disintegrin and metalloproteinase with a thrombospondin type 1 motif, member 13 (ADAMTS13)] attaches (24). The physiologic function of ADAMTS13 is the degradation of unfolded HMW vWF multimers. Excessive cleavage into vWF which is missing the high-molecular-weight (HMW) multimers and which shows a far less hemostatic activity occurs. The increased degradation leading to a significant reduction or complete loss of HMW vWF multimers is typical for “acquired von Willebrand syndrome” (AVWS). AVWS is a bleeding disorder similar to von Willebrand disease that occurs when there are deficiencies in VWF concentration, structure, or function as a result of acquired conditions (25). It is characterized by deficiency or complete loss of HMW vWF multimers increasing the risk of spontaneous bleeding from mucous membranes and, for example, catheter insertion sites (21, 26, 27). Routine coagulation analyses (e.g., international normalized ratio or activated partial thromboplastin time) are unsuited in detecting AVWS. The diagnosis of AVWS requires documentation of reduced vWF binding to either collagen (vWF collagen binding capacity, vWF: CB) or to platelet glycoprotein Ib receptors (vWF activity, vWF:A) in relation to vWF antigen (vWF: Ag). Test kits for quick determination of vWF: Ag and vWF: A meanwhile are commercially available and may routinely be provided by every clinical laboratory. In case of decreased vWF: CB/vWF: Ag or vWF: A/vWF: Ag ratio during vvECMO, AVWS is the most likely pathology. To confirm this diagnosis and for differentiation between AVWS and some types of inherited von Willebrand disease, however, multimer analysis by sodium dodecyl sulfate-agarose (SDS) gel electrophoresis is required (28, 29). SDS gel electrophoresis can only be performed in specialized coagulation laboratories and is relatively time-consuming.
The above mentioned pressure phenomena not only induce AVWS but also have impact on the relatively fragile platelet membrane inducing thrombocytolysis (“accelerated platelet destruction”) with consecutive thrombocytopenia (30–32). The mechanical destruction of the blood cells leads to the detachment of small membrane particles from platelets and also erythrocytes. These microparticles can present thrombogenic antigens and activate plasmatic coagulation (33, 34). Consecutively, this leads to the activation and accumulation of more platelets. It is not surprising that the higher the pump flow is set at the ECMO, the more pronounced is the platelet consumption (35, 36). Also contributing to thrombocytopenia is platelet consumption due to the activation of the platelets on the foreign materials of the tubing and oxygenators (57).
Besides the determination of platelet count, also platelet function was determined (37) in a subgroup of vvECMO patients (21). Basic platelet function test aggregometry was performed after stimulation of platelets with ristocetin, collagen, adenosine diphosphate (ADP), and epinephrine (38). Regardless of which of these substrates, a relevant hypoaggregation resulted which was still detectable days after ECMO explantation (21). This functional test was complemented by flow cytometry (39) to investigate the expression of several platelet receptors and secretion of α- and δ-platelet granules (40, 41) after staining of receptors with fluorescein-labeled monoclonal antibodies. In addition, vWF-binding capacity was determined using fluorescein-labeled monoclonal antibodies against vWF and fibrinogen. These investigations revealed highly reduced CD62 and CD63 expression (hinting to impaired α- and δ-granule secretion, respectively) and a reduced vWF-binding capacity (21). Taken together, these findings represent a reduced activatability of platelets which may be due to mechanical damage by ECMO or by exhaustion due to former activation of the platelets in the cannula or in the ECMO.
Anticoagulation and ECMO
Because of the increased risk of thrombosis within the extracorporeal system leading to malfunction and embolism, therapeutic anticoagulation was a paradigm not questioned during vvECMO for a long time (42). This was based on experiences from cardiac bypass surgery when patients without therapeutic anticoagulation had a larger waste of fibrinogen and platelet concentrates compared to those who were anticoagulated (43). Anticoagulation is established with unfractionated heparin (UFH) in the majority of patients (44). Modern cannulas, pumps, and membranes are coated with heparin or phosphorylcholine (45). A phosphorylcholine coating is considered to mimic the biological endothelial surface because phosphorylcholine is an integral component of the cell membrane (46). Phosphorylcholine coating (PHISIO) of tubes, cannula, oxygenator, and filter is associated with statistically unchanged vWF: ristocetin cofactor activity during cardiopulmonary bypass (47). Without doubt, the risk of bleeding when using anticoagulants is increased and has to be carefully weighed against the risk of thrombosis. Gail Annich focused on this dilemma in 2015 (48). The safe and effective use of low-molecular-weight heparin (LMWH) like enoxaparin and minimized anticoagulation concepts meanwhile have been proven in several studies (49, 50). As heparin-induced thrombocytopenia (HIT), a potential life-threatening reaction after recurrent exposition to heparin (51) may occur, and some centers use direct thrombin inhibitors like bivalirudin or argatroban instead of UFH or LMWH for anticoagulation. Independent of the type of anticoagulant, monitoring of anticoagulation still is sophisticated as acquired coagulation disorders may not be assessed by or influence routine laboratory markers (see below). Panigada et al. quoted that “ECMO […] does not change coagulation parameters” but “major bleedings occurred” in an observational study in 2016 (61). It is obvious that bleeding caused by ECMO was probably induced by factors not observed by routine coagulation tests such as prothrombin time or aPTT.
Bleeding During ECMO
Hemorrhagic diathesis has been described in nearly every ECMO patient. Registry data report bleeding complications in 22% (52) up to 45% (53) of vvECMO patients. Cannulation site and surgical site bleeding are the most frequent bleeding localizations, followed by pulmonary and spontaneous gastrointestinal hemorrhage. Registry data tend to underreport “minor” hemorrhage due to several reasons, and the Extracorporeal Life Support Organization (ELSO), for example, in 2014 defined bleeding to be documented not before a blood loss of ≥20 ml/kg bw/24 h or ≥10 ml/kg bw/24 h RBC transfused (42). Prospective trials assessing bleeding draw a different picture: epistaxis and hematuria complicated treatment of ECMO patients in even two-thirds of all patients in a cohort of patients with veno-arterial and veno-venous ECMO (54). Almost every third vvECMO patient suffered from relevant bleeding, and bleeding was associated with poor survival (54). While bleeding from cannula insertion sites and mucous membranes hints to impairment of primary hemostasis and can be controlled by local interventions, 4 to 19% of patients develop spontaneous intracranial bleeding with a survival rate of only 20% (55–60). In the aforementioned data, also patients with veno-arterial ECMO are partly included. In vvECMO according to ELSO registry data, intracranial bleeding seems to be more frequent compared to vaECMO (52). The exact incidence of intracranial bleeding is difficult to assess, as not all ECMO patients routinely receive cranial imaging and not all of the deceased undergo cerebral autopsy.
Transfusion
Continuous blood loss and hemolysis on ECMO also necessitate the transfusion of blood products. Transfusion thresholds, however, are mainly based on experts' opinions and differ between guidelines and recommendations. It is widely accepted that hemoglobin levels below 7–9 g/l should not be tolerated in adult vvECMO resulting in almost 90% of all patients receiving at least one packed red blood cell transfusion (RBC) during vvECMO (44, 52, 61). Treating anemia can improve oxygen delivery and may support coagulation at bleeding sites. Several studies, however, demonstrated that a restrictive threshold has acceptable outcomes in single-center cohorts (62). The recommended threshold to substitute platelet concentrates ranges from 50 to 100 thousand platelets/μl (44). Platelets are indispensable for primary hemostasis, but on the contrary platelet count does not necessarily correlate with platelet function; therefore, even with a platelet count above the aforementioned threshold, platelet transfusion may be reasonable in bleeding patients.
On the contrary, not indicated transfusions may be disadvantageous for patients: transfusions induce inflammatory cascades, have immunomodulatory effects, and are associated with the transmission or development of infections (63–66).
Steps to Control Bleeding
The “Treat Before They Bleed” Approach
As spontaneous intracranial hemorrhage—the most feared complication—should be avoided and blood transfusions should be restricted, early diagnosis, and treatment of acquired bleeding disorders should be performed before clinical bleeding can be observed. In our center, we established a diagnosis and treatment protocol for vvECMO patients (Table 1). This is mainly based on the key points such as prevention of bleeding, minimized anticoagulation, and correction of acquired coagulation disorders. The protocol and the treatment algorithm (Figure 1) at the author's department go far beyond what is recommended in current international guidelines (44). This is due to the high experience of this center and made possible by the good availability of a coagulation laboratory.
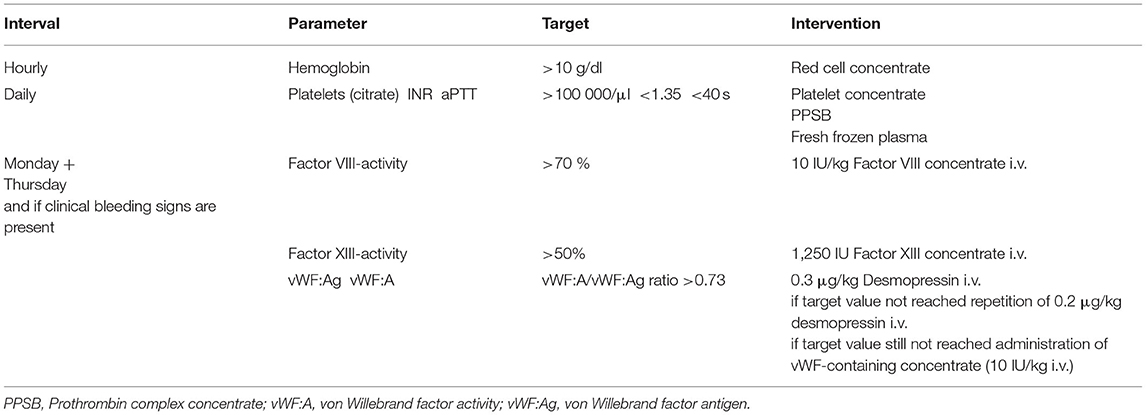
Table 1. Routine blood drawings for the determination and treatment of acquired bleeding disorders during vvECMO in our department.
Minimizing Anticoagulation
Bleeding diathesis may be enhanced by anticoagulants (56, 67). Annich pointed out the “precarious balance of hemostasis during ECMO therapy” (48), and Ranucci described anticoagulation in extracorporeal therapy as “navigating difficult seas between thrombosis and bleeding” (68).
Reducing anticoagulation in vvECMO to prophylactic doses is safe and feasible and reduces the risk of bleeding. Krueger et al. reported data of more than 60 patients on vvECMO who received enoxaparin in only prophylactic dose and did not develop thromboembolic events or bleeding episodes. In combination with correction of acquired coagulation disorders, there was no increase in thrombotic pump failure (49). Incidence of bleeding and need for blood transfusion with a mean of only 1,15 RBC units and 0,6 fresh frozen plasma units per ECMO day was relevantly lower compared to other reported cohorts (35, 69, 70). Meanwhile, concepts with low dose or even without anticoagulation have only been applied in a few centers (71, 72). Low-molecular-weight heparin seems to be the better anticoagulant compared to UFH with regard to the prevention of thromboembolic events (50).
Balancing Benefit and Risk With the Use of Anticoagulation and Transfusion in vvECMO
When patients begin to present with ongoing bleeding and transfusion of blood components becomes necessary during vvECMO, it should be critically revised whether the extracorporeal therapy still is beneficial for the patient. Probably, explantation of the vvECMO in favor of accepting a more invasive mechanical ventilation may be the less complicative approach.
Control of Acquired Disorders of Primary Hemostasis
When preconditions like INR, aPTT, blood pH, temperature, and calcium levels are optimized and patients clinically still bleed, transfusion of platelet concentrates is a reasonable approach. Depending on the medical center, a particular platelet count has to be determined as trigger for transfusion. In our center, for example, we chose a transfusion threshold of >100 000 /μl platelets analogous to national guidelines for the treatment of traumatic brain injury (73). If bleeding still occurs after platelet transfusion, it should be taken in account that the bleeding symptoms may be due to impaired platelet function. Objectivation of platelet function in clinical practice is difficult, as point-of-care systems may fail to detect ECMO-induced platelet dysfunction. Therefore, calculated transfusion of platelets may be effective to control bleeding even when platelet count is still >100 000 platelets/μl.
Adhesion of platelets to collagen presented in case of endothelial injury is promoted by the von Willebrand factor. As described above, vvECMO leads to acquired impairment of vWF function, so-called AVWS. For detection of AVWS, at least determination of vWF: Ag and vWF: A is necessary (29). In case of a reduced vWF: A/vWF: Ag ratio, AVWS is likely. First-line treatment is therapy with desmopressin (DDVAP) with 0,3 μg/kgbw to induce secretion of vWF multimers stored in endothelial cells (28, 74, 75). The next step is the substitution of vWF-containing concentrates. The most common drug is factor VIII/vWF concentrate derived from human plasma (76). A new option is recombinant von Willebrand factor concentrate (77).
Waste Coagulopathy
Diffuse activation of the coagulation system by platelet-derived microparticles, contact activation via factor XII, endothelial damage, and inflammation lead to waste coagulopathy (69, 78). In this context, deficiency of fibrinogen and/or factor XIII are common findings and may enhance bleeding diathesis (23, 35, 79–84). As fibrinogen is essential as well for clot formation as also for the activation of platelets, it is necessary to maintain normal fibrinogen levels by either transfusion of fresh frozen plasma (FFP) or fibrinogen concentrate. Clot firmness is dependent on cross-linking of fibrin with coagulation factor XIII (23, 79, 84–87). As many vvECMO patients present with factor XIII deficiency (23) which may enhance bleeding, substitution with factor XIII concentrates can be necessary to maintain clot firmness. In addition, hyper fibrinolysis should be diagnosed by viscoelastic tests like thrombelastography (88) and treated with infusion of tranexamic acid (89). Tranexamic acid may also be applied locally at bleeding sites.
Exchange of Components
As bio-coating of the extracorporeal circuit continuously is washed away and coagulation activating fragments accumulate in the oxygenator membrane, an important step to control ongoing bleeding and hemolysis during prolonged vvECMO is complete exchange of extracorporeal pump and/or oxygenator membrane (90, 91). Cannulas may be left in situ and connected to the new system in order to minimize the risk of cannulation.
Recombinant Factor VII
In case of uncontrollable bleeding during extracorporeal therapy despite optimization of the abovementioned preconditions, the careful use of recombinant factor VII has been described (92–94). This therapy has to be weighed carefully against the risk of acute clot formation within the extracorporeal system and possible thrombotic pump failure. Doses up to 60 μg/kgbw of recombinant factor VII seem to present an acceptable risk profile as ultima ratio option when all formerly described interventions have failed to control bleeding.
Hypercoagulopathy Following ECMO
Only hours after explantation of vvECMO, vWF: A, vWF: CB, and factor VIII activity not only recover, but also become overcorrected beyond values before vvECMO. These findings imply that the risk of thromboembolic events is particularly high after vvECMO decannulation which needs to be taken into account when planning appropriate anticoagulation (21, 78). According to a standardized protocol in our center, all patients receive a subcutaneous dose of 80 IU/kg bw enoxaparin 1 h after decannulation. According to the anti-Xa parameter (4 h later), the following doses of enoxaparin are adjusted aiming for an aXa of 0.5–0.8 IU/ml (therapeutic anticoagulation). Therapeutic anticoagulation with enoxaparin or rivaroxaban is maintained for at least 6 weeks (even if the patient is meanwhile discharged from the hospital).
Summary
- Treat before they bleed
- Decrease anticoagulation
- Control primary hemostasis
- Substitute wasted coagulation factors
- Consider membrane exchange and ECMO explantation
- Do not underestimate hypercoagulatory status after ECMO explantation.
Author Contributions
JK and BZ did the research of relevant part of articles cited. They performed literature search. JK wrote the primary version of the manuscript and drew the pictures and tables. BZ revised the manuscript. Both authors agreed to the final version of the manuscript. Both authors contributed to the article and approved the submitted version.
Funding
The article processing charge was partly funded by the Baden-Wuerttemberg Ministry of Science, Research and Art and the University of Freiburg in the funding programme Open Access Publishing.
Conflict of Interest
The authors declare that the research was conducted in the absence of any commercial or financial relationships that could be construed as a potential conflict of interest.
Publisher's Note
All claims expressed in this article are solely those of the authors and do not necessarily represent those of their affiliated organizations, or those of the publisher, the editors and the reviewers. Any product that may be evaluated in this article, or claim that may be made by its manufacturer, is not guaranteed or endorsed by the publisher.
Acknowledgments
The authors thank Christoph Benk and his team of perfusionists for their valuable support. They also appreciate the work of all physicians, nurses, and medical technical assistants in the coagulation laboratory of the Division of Pediatric Hematology and Oncology as well in the critical care unit of the Department of Anesthesiology and Critical Care at the University Medical Center, Freiburg.
References
1. Ashbaugh DG, Bigelow DB, Petty TL, Levine BE. Acute respiratory distress in adults. Lancet. (1967) 2:319–23. doi: 10.1016/S0140-6736(67)90168-7
2. Force ADT, Ranieri VM, Rubenfeld GD, Thompson BT, Ferguson ND, Caldwell E, et al. Acute respiratory distress syndrome: the Berlin definition. JAMA. (2012) 307:2526–33. doi: 10.1001/jama.2012.5669
3. Acute Respiratory Distress Syndrome N, Brower RG, Matthay MA, Morris A, Schoenfeld D, Thompson BT, et al. Ventilation with lower tidal volumes as compared with traditional tidal volumes for acute lung injury and the acute respiratory distress syndrome. N Engl J Med. (2000) 342:1301–8. doi: 10.1056/NEJM200005043421801
4. Slutsky AS, Ranieri VM. Ventilator-induced lung injury. N Engl J Med. (2013) 369:2126–36. doi: 10.1056/NEJMra1208707
5. Hill JD, O'Brien TG, Murray JJ, Dontigny L, Bramson ML, Osborn JJ, et al. Prolonged extracorporeal oxygenation for acute post-traumatic respiratory failure (shock-lung syndrome). Use of the Bramson membrane lung. N Engl J Med. (1972) 286:629–34. doi: 10.1056/NEJM197203232861204
6. Zapol WM, Snider MT, Hill JD, Fallat RJ, Bartlett RH, Edmunds LH, et al. Extracorporeal membrane oxygenation in severe acute respiratory failure. A randomized prospective study. JAMA. (1979) 242:2193–6. doi: 10.1001/jama.1979.03300200023016
7. Gattinoni L, Pesenti A, Mascheroni D, Marcolin R, Fumagalli R, Rossi F, et al. Low-frequency positive-pressure ventilation with extracorporeal CO2 removal in severe acute respiratory failure. JAMA. (1986) 256:881–6. doi: 10.1001/jama.1986.03380070087025
8. Australia New Zealand Extracorporeal Membrane Oxygenation Influenza I, Davies A, Jones D, Bailey M, Beca J, et al. Extracorporeal membrane oxygenation for 2009 influenza A(H1N1) acute respiratory distress syndrome. JAMA. (2009) 302:1888–95. doi: 10.1001/jama.2009.1535
9. Peek GJ, Killer HM, Reeves R, Sosnowski AW, Firmin RK. Early experience with a polymethyl pentene oxygenator for adult extracorporeal life support. ASAIO J. (2002) 48:480–2. doi: 10.1097/00002480-200209000-00007
10. Sidebotham D, Allen SJ, McGeorge A, Ibbott N, Willcox T. Venovenous extracorporeal membrane oxygenation in adults: practical aspects of circuits, cannulae, and procedures. J Cardiothorac Vasc Anesth. (2012) 26:893–909. doi: 10.1053/j.jvca.2012.02.001
11. Laudi S, Busch T, Kaisers U. Extracorporeal membrane oxygenation for ARDS due to 2009 influenza A(H1N1). JAMA. (2010) 303:941. doi: 10.1001/jama.2010.200
12. Combes A, Peek GJ, Hajage D, Hardy P, Abrams D, Schmidt M, et al. ECMO for severe ARDS: systematic review and individual patient data meta-analysis. Intensive Care Med. (2020) 46:2048–57. doi: 10.1007/s00134-020-06248-3
13. Nagaoka E, Someya T, Kitao T, Kimura T, Ushiyama T, Hijikata W, et al. Development of a disposable magnetically levitated centrifugal blood pump (MedTech Dispo) intended for bridge-to-bridge applications–two-week in vivo evaluation. Artif Organs. (2010) 34:778–83. doi: 10.1111/j.1525-1594.2010.01107.x
14. Hijikata W, Shinshi T, Asama J, Li L, Hoshi H, Takatani S, et al. A magnetically levitated centrifugal blood pump with a simple-structured disposable pump head. Artif Organs. (2008) 32:531–40. doi: 10.1111/j.1525-1594.2008.00576.x
15. Someya T, Kobayashi M, Waguri S, Ushiyama T, Nagaoka E, Hijikata W, et al. Development of a disposable maglev centrifugal blood pump intended for one-month support in bridge-to-bridge applications: in vitro and initial in vivo evaluation. Artif Organs. (2009) 33:704–13. doi: 10.1111/j.1525-1594.2009.00900.x
16. Gattinoni L, Carlesso E, Langer T. Clinical review: extracorporeal membrane oxygenation. Crit care. (2011) 15:243. doi: 10.1186/cc10490
17. Quintel M, Bartlett RH, Grocott MPW, Combes A, Ranieri MV, Baiocchi M, et al. Extracorporeal membrane oxygenation for respiratory failure. Anesthesiology. (2020) 132:1257–76. doi: 10.1097/ALN.0000000000003221
18. Pavlushkov E, Berman M, Valchanov K. Cannulation techniques for extracorporeal life support. Ann Transl Med. (2017) 5:70. doi: 10.21037/atm.2016.11.47
19. Lindholm JA. Cannulation for veno-venous extracorporeal membrane oxygenation. J Thorac Dis. (2018) 10:S606–12. doi: 10.21037/jtd.2018.03.101
20. Broman LM, Taccone FS, Lorusso R, Malfertheiner MV, Pappalardo F, Di Nardo M, et al. The ELSO maastricht treaty for ECLS nomenclature: abbreviations for cannulation configuration in extracorporeal life support—a position paper of the extracorporeal life support organization. Crit care. (2019) 23:36. doi: 10.1186/s13054-019-2334-8
21. Kalbhenn J, Schlagenhauf A, Rosenfelder S, Schmutz A, Zieger B. Acquired von Willebrand syndrome and impaired platelet function during venovenous extracorporeal membrane oxygenation: rapid onset and fast recovery. J Heart Lung Transplant. (2018) 37:985–91. doi: 10.1016/j.healun.2018.03.013
22. Kalbhenn J, Schmidt R, Nakamura L, Schelling J, Rosenfelder S, Zieger B. Early diagnosis of acquired von Willebrand Syndrome (AVWS) is elementary for clinical practice in patients treated with ECMO therapy. J Atheroscler Thromb. (2015) 22:265–71. doi: 10.5551/jat.27268
23. Kalbhenn J, Wittau N, Schmutz A, Zieger B, Schmidt R. Identification of acquired coagulation disorders and effects of target-controlled coagulation factor substitution on the incidence and severity of spontaneous intracranial bleeding during veno-venous ECMO therapy. Perfusion. (2015) 30:675–82. doi: 10.1177/0267659115579714
24. Baldauf C, Schneppenheim R, Stacklies W, Obser T, Pieconka A, Schneppenheim S, et al. Shear-induced unfolding activates von Willebrand factor A2 domain for proteolysis. J Thromb Haemost. (2009) 7:2096–105. doi: 10.1111/j.1538-7836.2009.03640.x
25. Stockschlaeder M, Schneppenheim R, Budde U. Update on von Willebrand factor multimers: focus on high-molecular-weight multimers and their role in hemostasis. Blood Coagul Fibrinolysis. (2014) 25:206–16. doi: 10.1097/MBC.0000000000000065
26. Franchini M, Mannucci PM. Acquired von Willebrand syndrome: focused for hematologists. Haematologica. (2020) 105:2032–7. doi: 10.3324/haematol.2020.255117
27. Leebeek FW, Eikenboom JC. Von Willebrand's disease. N Engl J Med. (2016) 375:2067–80. doi: 10.1056/NEJMra1601561
28. Federici AB, Budde U, Castaman G, Rand JH, Tiede A. Current diagnostic and therapeutic approaches to patients with acquired von Willebrand syndrome: a 2013 update. Semin Thromb Hemost. (2013) 39:191–201. doi: 10.1055/s-0033-1334867
29. Kalbhenn J, Glonnegger H, Büchsel M, Priebe H, Zieger B. Acquired Von Willebrand syndrome and desmopressin resistance during venovenous extracorporeal membrane oxygenation in patients with COVID-19: a prospective observational study. Crit Care Med. (2022). doi: 10.1097/CCM.0000000000005467. [Epub ahead of print].
30. Spragg RG, Hill RN, Wedel MK, Materson A, Moser KM. Platelet kinetics in venovenous membrane oxygenation. Trans Am Soc Artif Intern Organs. (1975) 21:171–7.
31. Chevuru SC, Sola MC, Theriaque DW, Hutson AD, Leung WC, Perez JA, et al. Multicenter analysis of platelet transfusion usage among neonates on extracorporeal membrane oxygenation. Pediatrics. (2002) 109:e89. doi: 10.1542/peds.109.6.e89
32. Anderson HL. 3rd, Cilley RE, Zwischenberger JB, Bartlett RH. Thrombocytopenia in neonates after extracorporeal membrane oxygenation. ASAIO Trans. (1986) 32:534–7. doi: 10.1097/00002216-198609000-00031
33. Van Der Meijden PE, Van Schilfgaarde M, Van Oerle R, Renne T. ten Cate H, Spronk HM. Platelet- and erythrocyte-derived microparticles trigger thrombin generation via factor XIIa. J Thromb Haemost. (2012) 10:1355–62. doi: 10.1111/j.1538-7836.2012.04758.x
34. Kailashiya J. Platelet-derived microparticles analysis: techniques, challenges and recommendations. Anal Biochem. (2018) 546:78–85. doi: 10.1016/j.ab.2018.01.030
35. Ang AL, Teo D, Lim CH, Leou KK, Tien SL, Koh MB. Blood transfusion requirements and independent predictors of increased transfusion requirements among adult patients on extracorporeal membrane oxygenation—a single centre experience. Vox Sang. (2009) 96:34–43. doi: 10.1111/j.1423-0410.2008.01110.x
36. Robinson TM, Kickler TS, Walker LK, Ness P, Bell W. Effect of extracorporeal membrane oxygenation on platelets in newborns. Crit Care Med. (1993) 21:1029–34. doi: 10.1097/00003246-199307000-00018
37. Born GV, Cross MJ. The aggregation of blood platelets. J Physiol. (1963) 168:178–95. doi: 10.1113/jphysiol.1963.sp007185
38. Koltai K, Kesmarky G, Feher G, Tibold A, Toth K. Platelet aggregometry testing: molecular mechanisms, techniques and clinical implications. Int J Mol Sci. (2017) 18:1803. doi: 10.3390/ijms18081803
39. Sbrana S, Della Pina F, Rizza A, Buffa M, De Filippis R, Gianetti J, et al. Relationships between optical aggregometry (type born) and flow cytometry in evaluating ADP-induced platelet activation. Cytometry B Clin Cytom. (2008) 74:30–9. doi: 10.1002/cyto.b.20360
40. Nolan JP, Jones JC. Detection of platelet vesicles by flow cytometry. Platelets. (2017) 28:256–62. doi: 10.1080/09537104.2017.1280602
41. Hickerson DH, Bode AP. Flow cytometry of platelets for clinical analysis. Hematol Oncol Clin North Am. (2002) 16:421–54. doi: 10.1016/S0889-8588(01)00017-X
42. ELSO. Anticoagulation Guideline. The Extracorporeal Life Support Organization (2014). Available online at: https://www.elso.org/portals/0/files/elsoanticoagulationguideline8-2014-table-contents.pdf (accessed April 18, 2022).
43. Begelman KM, Homer LD, Fletcher JR. Platelet and fibrinogen turnover during cardiopulmonary bypass without anticoagulation. Trans Am Soc Artif Intern Organs. (1979) 25:167–70. doi: 10.1097/00002480-197902500-00031
44. McMichael ABV, Ryerson LM, Ratano D, Fan E, Faraoni D, Annich GM. 2021 ELSO adult and pediatric anticoagulation guidelines. ASAIO J. (2022) 68:303–10. doi: 10.1097/MAT.0000000000001652
45. Pieri M, Turla OG, Calabro MG, Ruggeri L, Agracheva N, Zangrillo A, et al. A new phosphorylcholine-coated polymethylpentene oxygenator for extracorporeal membrane oxygenation: a preliminary experience. Perfusion. (2013) 28:132–7. doi: 10.1177/0267659112469642
46. De Somer F, Francois K, van Oeveren W, Poelaert J, De Wolf D, Ebels T, et al. Phosphorylcholine coating of extracorporeal circuits provides natural protection against blood activation by the material surface. Eur J Cardiothorac Surg. (2000) 18:602–6. doi: 10.1016/S1010-7940(00)00508-X
47. Albes JM, Stohr IM, Kaluza M, Siegemund A, Schmidt D, Vollandt R, et al. Physiological coagulation can be maintained in extracorporeal circulation by means of shed blood separation and coating. J Thorac Cardiovasc Surg. (2003) 126:1504–12. doi: 10.1016/S0022-5223(03)00958-9
48. Annich GM. Extracorporeal life support: the precarious balance of hemostasis. J Thromb Haemost. (2015) 13:S336–42. doi: 10.1111/jth.12963
49. Krueger K, Schmutz A, Zieger B, Kalbhenn J. Venovenous extracorporeal membrane oxygenation with prophylactic subcutaneous anticoagulation only: an observational study in more than 60 patients. Artif Organs. (2017) 41:186–92. doi: 10.1111/aor.12737
50. Gratz J, Pausch A, Schaden E, Baierl A, Jaksch P, Erhart F, et al. Low molecular weight heparin vs. unfractioned heparin for anticoagulation during perioperative extracorporeal membrane oxygenation: a single center experience in 102 lung transplant patients. Artif Organs. (2020) 44:638–46. doi: 10.1111/aor.13642
51. Greinacher A. CLINICAL PRACTICE. Heparin-induced thrombocytopenia. N Engl J Med. (2015) 373:252–61. doi: 10.1056/NEJMcp1411910
52. Willers A, Swol J, Buscher H, McQuilten Z, van Kuijk SMJ, Ten Cate H, et al. Longitudinal trends in bleeding complications on extracorporeal life support over the past two decades-extracorporeal life support organization registry analysis. Crit Care Med. (2022). doi: 10.1097/CCM.0000000000005466. [Epub ahead of print].
53. Nunez JI, Gosling AF, O'Gara B, Kennedy KF, Rycus P, Abrams D, et al. Bleeding and thrombotic events in adults supported with venovenous extracorporeal membrane oxygenation: an ELSO registry analysis. Intensive Care Med. (2022) 48:213–24. doi: 10.1007/s00134-021-06593-x
54. Aubron C, DePuydt J, Belon F, Bailey M, Schmidt M, Sheldrake J, et al. Predictive factors of bleeding events in adults undergoing extracorporeal membrane oxygenation. Ann Intensive Care. (2016) 6:97. doi: 10.1186/s13613-016-0196-7
55. Zangrillo A, Landoni G, Biondi-Zoccai G, Greco M, Greco T, Frati G, et al. A meta-analysis of complications and mortality of extracorporeal membrane oxygenation. Crit Care Resusc. (2013) 15:172–8.
56. Paden ML, Conrad SA, Rycus PT, Thiagarajan RR, Registry E. Extracorporeal life support organization registry report 2012. ASAIO J. (2013) 59:202–10. doi: 10.1097/MAT.0b013e3182904a52
57. Thiagarajan RR, Barbaro RP, Rycus PT, McMullan DM, Conrad SA, Fortenberry JD, et al. Extracorporeal life support organization registry international report 2016. ASAIO J. (2017) 63:60–7. doi: 10.1097/MAT.0000000000000475
58. Kasirajan V, Smedira NG, McCarthy JF, Casselman F, Boparai N, McCarthy PM. Risk factors for intracranial hemorrhage in adults on extracorporeal membrane oxygenation. Eur J Cardiothorac Surg. (1999) 15:508–14. doi: 10.1016/S1010-7940(99)00061-5
59. Luyt CE, Brechot N, Demondion P, Jovanovic T, Hekimian G, Lebreton G, et al. Brain injury during venovenous extracorporeal membrane oxygenation. Intensive Care Med. (2016) 42:897–907. doi: 10.1007/s00134-016-4318-3
60. Klinzing S, Wenger U, Stretti F, Steiger P, Rushing EJ, Schwarz U, et al. Neurologic injury with severe adult respiratory distress syndrome in patients undergoing extracorporeal membrane oxygenation: a single-center retrospective analysis. Anesth Analg. (2017) 125:1544–8. doi: 10.1213/ANE.0000000000002431
61. Raasveld SJ, Karami M, van den Bergh WM, Oude Lansink-Hartgring A, van der Velde F, Maas JJ, et al. RBC transfusion in venovenous extracorporeal membrane oxygenation: a multicenter cohort study. Crit Care Med. (2022) 50:224–34. doi: 10.1097/CCM.0000000000005398
62. Abbasciano RG, Yusuff H, Vlaar APJ, Lai F, Murphy GJ. Blood transfusion threshold in patients receiving extracorporeal membrane oxygenation support for cardiac and respiratory failure-a systematic review and meta-analysis. J Cardiothorac Vasc Anesth. (2021) 35:1192–202. doi: 10.1053/j.jvca.2020.08.068
63. Buddeberg F, Schimmer BB, Spahn DR. Transfusion-transmissible infections and transfusion-related immunomodulation. Best Pract Res Clin Anaesthesiol. (2008) 22:503–17. doi: 10.1016/j.bpa.2008.05.003
64. Isbister JP, Shander A, Spahn DR, Erhard J, Farmer SL, Hofmann A. Adverse blood transfusion outcomes: establishing causation. Transfus Med Rev. (2011) 25:89–101. doi: 10.1016/j.tmrv.2010.11.001
65. Spahn DR, Shander A, Hofmann A, Berman MF. More on transfusion and adverse outcome: it's time to change. Anesthesiology. (2011) 114:234–6. doi: 10.1097/ALN.0b013e3182054d3e
66. Shander A, Fink A, Javidroozi M, Erhard J, Farmer SL, Corwin H, et al. Appropriateness of allogeneic red blood cell transfusion: the international consensus conference on transfusion outcomes. Transfus Med Rev. (2011) 25:232–46.e53. doi: 10.1016/j.tmrv.2011.02.001
67. Annich GM, Zaulan O, Neufeld M, Wagner D, Reynolds MM. Thromboprophylaxis in extracorporeal circuits: current pharmacological strategies and future directions. Am J Cardiovasc Drugs. (2017) 17:425–39. doi: 10.1007/s40256-017-0229-0
68. Ranucci M. The conundrum of anticoagulation and hemostatic management in ECMO patients. Minerva Anestesiol. (2016) 82:147–8.
69. Esper SA, Levy JH, Waters JH, Welsby IJ. Extracorporeal membrane oxygenation in the adult: a review of anticoagulation monitoring and transfusion. Anesth Analg. (2014) 118:731–43. doi: 10.1213/ANE.0000000000000115
70. Rastan AJ, Lachmann N, Walther T, Doll N, Gradistanac T, Gommert JF, et al. Autopsy findings in patients on postcardiotomy Extracorporeal Membrane Oxygenation (ECMO). Int J Artif Organs. (2006) 29:1121–31. doi: 10.1177/039139880602901205
71. Fina D, Matteucci M, Jiritano F, Meani P, Lo Coco V, Kowalewski M, et al. Extracorporeal membrane oxygenation without therapeutic anticoagulation in adults: a systematic review of the current literature. Int J Artif Organs. (2020) 43:570–8. doi: 10.1177/0391398820904372
72. Olson SR, Murphree CR, Zonies D, Meyer AD, McCarty OJT, Deloughery TG, et al. Thrombosis and bleeding in Extracorporeal Membrane Oxygenation (ECMO) without anticoagulation: a systematic review. ASAIO J. (2021) 67:290–6. doi: 10.1097/MAT.0000000000001230
73. Schoeneberg C, Schilling M, Keitel J, Kauther MD, Burggraf M, Hussmann B, et al. [TraumaNetwork, trauma registry of the DGU(R), whitebook, S3 guideline on treatment of polytrauma/severe injuries—an approach for validation by a retrospective analysis of 2,304 patients (2002–2011) of a level 1 trauma centre]. Zentralbl Chir. (2017) 142:199–208. doi: 10.1055/s-0033-1360225
74. Budde U, Scheppenheim S, Dittmer R. Treatment of the acquired von Willebrand syndrome. Expert Rev Hematol. (2015) 8:799–818. doi: 10.1586/17474086.2015.1060854
75. Tiede A. Diagnosis and treatment of acquired von Willebrand syndrome. Thromb Res. (2012) 130 Suppl 2:S2–6. doi: 10.1016/S0049-3848(13)70003-3
76. Berntorp E, Archey W, Auerswald G, Federici AB, Franchini M, Knaub S, et al. A systematic overview of the first pasteurised VWF/FVIII medicinal product, haemate P/ humate -P: history and clinical performance. Eur J Haematol Suppl. (2008) 70:3–35. doi: 10.1111/j.1600-0609.2008.01049.x
77. Franchini M, Mannucci PM. Von Willebrand factor (Vonvendi(R)): the first recombinant product licensed for the treatment of von Willebrand disease. Expert Rev Hematol. (2016) 9:825–30. doi: 10.1080/17474086.2016.1214070
78. Kalbhenn J, Glonnegger H, Wilke M, Bansbach J, Zieger B. Hypercoagulopathy, acquired coagulation disorders and anticoagulation before, during and after extracorporeal membrane oxygenation in COVID-19: a case series. Perfusion. (2021) 36:592–602. doi: 10.1177/02676591211001791
79. Blome M, Isgro F, Kiessling AH, Skuras J, Haubelt H, Hellstern P, et al. Relationship between factor XIII activity, fibrinogen, haemostasis screening tests and postoperative bleeding in cardiopulmonary bypass surgery. Thromb Haemost. (2005) 93:1101–7. doi: 10.1160/TH04-12-0799
80. Doyle AJ, Hunt BJ. Current understanding of how extracorporeal membrane oxygenators activate haemostasis and other blood components. Front Med. (2018) 5:352. doi: 10.3389/fmed.2018.00352
81. Malfertheiner MV, Philipp A, Lubnow M, Zeman F, Enger TB, Bein T, et al. Hemostatic changes during extracorporeal membrane oxygenation: a prospective randomized clinical trial comparing three different extracorporeal membrane oxygenation systems. Crit Care Med. (2016) 44:747–54. doi: 10.1097/CCM.0000000000001482
82. Sorensen B, Larsen OH, Rea CJ, Tang M, Foley JH, Fenger-Eriksen C. Fibrinogen as a hemostatic agent. Semin Thromb Hemost. (2012) 38:268–73. doi: 10.1055/s-0032-1309287
83. Sorensen B, Tang M, Larsen OH, Laursen PN, Fenger-Eriksen C, Rea CJ. The role of fibrinogen: a new paradigm in the treatment of coagulopathic bleeding. Thromb Res. (2011) 128:S13–6. doi: 10.1016/S0049-3848(12)70004-X
84. Wettstein P, Haeberli A, Stutz M, Rohner M, Corbetta C, Gabi K, et al. Decreased factor XIII availability for thrombin and early loss of clot firmness in patients with unexplained intraoperative bleeding. Anesth Analg. (2004) 99:1564–9. doi: 10.1213/01.ANE.0000134800.46276.21
85. Dorgalaleh A, Rashidpanah J. Blood coagulation factor XIII and factor XIII deficiency. Blood Rev. (2016) 30:461–75. doi: 10.1016/j.blre.2016.06.002
86. Gerlach R, Raabe A, Zimmermann M, Siegemund A, Seifert V. Factor XIII deficiency and postoperative hemorrhage after neurosurgical procedures. Surg Neurol. (2000) 54:260–4. doi: 10.1016/S0090-3019(00)00308-6
87. Gorlinger K, Bergmann L, Dirkmann D. Coagulation management in patients undergoing mechanical circulatory support. Best Pract Res Clin Anaesthesiol. (2012) 26:179–98. doi: 10.1016/j.bpa.2012.04.003
88. Hellmann C, Schmutz A, Kalbhenn J. Bleeding during veno-venous ECMO cannot reliably be predicted by rotational thrombelastometry (ROTEM). Perfusion. (2018) 33:289–96. doi: 10.1177/0267659117746231
89. Moroz LA, Gilmore NJ. Fibrinolysis in normal plasma and blood: evidence for significant mechanisms independent of the plasminogen-plasmin system. Blood. (1976) 48:531–45. doi: 10.1182/blood.V48.4.531.531
90. Nagler B, Hermann A, Robak O, Schellongowski P, Buchtele N, Bojic A, et al. Incidence and etiology of system exchanges in patients receiving extracorporeal membrane oxygenation. ASAIO J. (2021) 67:776–84. doi: 10.1097/MAT.0000000000001332
91. Lubnow M, Philipp A, Foltan M, Bull Enger T, Lunz D, Bein T, et al. Technical complications during veno-venous extracorporeal membrane oxygenation and their relevance predicting a system-exchange—retrospective analysis of 265 cases. PLoS ONE. (2014) 9:e112316. doi: 10.1371/journal.pone.0112316
92. Repesse X, Au SM, Brechot N, Trouillet JL, Leprince P, Chastre J, et al. Recombinant factor VIIa for uncontrollable bleeding in patients with extracorporeal membrane oxygenation: report on 15 cases and literature review. Crit Care. (2013) 17:R55. doi: 10.1186/cc12581
93. Niebler RA, Punzalan RC, Marchan M, Lankiewicz MW. Activated recombinant factor VII for refractory bleeding during extracorporeal membrane oxygenation. Pediatr Crit Care Med. (2010) 11:98–102. doi: 10.1097/PCC.0b013e3181b0620b
Keywords: acquired von Willebrand syndrome, ARDS, ECMO, bleeding, thrombosis, prevention, treatment
Citation: Kalbhenn J and Zieger B (2022) Bleeding During Veno-Venous ECMO: Prevention and Treatment. Front. Med. 9:879579. doi: 10.3389/fmed.2022.879579
Received: 19 February 2022; Accepted: 27 April 2022;
Published: 23 May 2022.
Edited by:
Thierry Burnouf, Taipei Medical University, TaiwanCopyright © 2022 Kalbhenn and Zieger. This is an open-access article distributed under the terms of the Creative Commons Attribution License (CC BY). The use, distribution or reproduction in other forums is permitted, provided the original author(s) and the copyright owner(s) are credited and that the original publication in this journal is cited, in accordance with accepted academic practice. No use, distribution or reproduction is permitted which does not comply with these terms.
*Correspondence: Johannes Kalbhenn, johannes.kalbhenn@uniklinik-freiburg.de; orcid.org/0000-0001-7551-5082