The role of diseases in unifying the health of global estuaries
- 1Department of Biology, Stanford University, Stanford, CA, United States
- 2Department of Earth System Science, Stanford University, Stanford, CA, United States
Establishing a universal indicator of estuary health is an ongoing challenge for coastal ecology that is made more pressing due to the threat that climate change and anthropogenic activity pose to them. Historically, estuarine health was measured through basic physical, chemical, and biological traits, which have been used to routinely monitor estuaries for more than 30 years. However, it is unclear if they are dynamic enough to accurately assess ecosystem health changes driven by the pressures of climate change and anthropogenic activity. Measuring estuarine health indicators and noting incipient indicators relevant to the evolving threats of climate- and anthropogenic-related stressors on estuarine ecosystems is vital for safeguarding them into the future. Monitoring the presence and vitality of disease in estuarine ecosystems may prove to be a significant indicator of estuarine health. Here we review 22 years of literature (354 papers) to identify the role of marine diseases as critical indicators of long-term estuary health compared to traditional methods, with the goal of identifying a key indicator and underlying unifier of other health metrics. As indicators of both general ecosystem health and of multiple other stressors, diseases play a disproportionately significant role in estuary health in the face of climate- and anthropogenic-related stressors. Marine diseases are a unifier of structural and functional estuary health indicators and must be observed and modeled further.
1 Introduction
1.1 Effects of climate change and anthropogenic activity on estuaries
The ongoing climate crisis and anthropogenic stressors represent significant threats to global coastal zones (Whitfield and Elliott, 2002; Robb, 2014; Cloern et al., 2016; Peng et al., 2021). Estuaries are among the most important environments of the coastal zone that are at the interface of terrestrial and marine habitats, which create some of the most biologically productive environments on earth (Montagna et al., 2012). These are complex, yet critical ecotones for coastal integrity (White and Kaplan, 2017; Bradley, 2017; Liu et al., 2021). As biodiversity hotspots, they provide up to $5.4 trillion (1.8 x 108 hectares globally at 28,916 2007$/ha) in multiple ecosystem services (i.e., habitat provisioning, nutrient regulation, storm surge attenuation, etc.) that support human life near and far from the coast (Cyrus and Forbes, 1996; Turner et al., 2006; Costanza et al., 2014a; Bradley, 2017). More than half of the global human population lives within 100 km of the coast with more people moving toward coastlines every year (Niemi et al., 2004). Increased coastal and watershed development has led to more frequent, anthropogenically-driven loading of toxic substances, overload of nutrients and subsequent algal blooms, hypoxia, beach closures, and damage to coastal fisheries (Costanza et al., 2014b). Further, climate change has led to changes in pH levels, a rise in sea levels, losses of coastal wetlands and estuaries, and saltwater intrusion into coastal aquifers (Mastrocicco et al., 2019). Both climate change and anthropogenic sources of stress have a negative impact on estuarine health.
The combination of anthropogenic and climate change related stress drives a significant need to develop robust measure of estuarine health. However, coastal resources have primarily been monitored on a stressor-by-stressor basis (e.g., hypoxia, nutrient loading, or dissolved oxygen). Previous research has individualistically measured the physical, chemical, biological, and aesthetic elements of estuaries and the anthropogenic modifications they can undergo, but little research has made interdisciplinary links among and between forms of modification (Cooper, 2001; Paerl et al., 2006). To fully measure the complexities of coastal systems, studies demonstrating interdisciplinary measures of estuary health will help the field of coastal ecology establish primary indicators of estuary health in the context of rapidly shifting environmental conditions.
1.2 Measures of estuary health
Substantial challenges persist in the integration of estuarine health indicators, which makes comparing outcomes of indicators difficult. Estuary health is an ecologically complex concept that can encompass multiple environmental parameters with direct and indirect effects (Turpie et al., 2013; Bradley, 2017). Indicators of estuary health often aggregate multiple variables into one metric with a strong emphasis on faunal benthic populations (e.g., clams, shrimps, and worms) due their sensitivity to environmental conditions (Table 1). For example, the AZTI marine biotic index (AMBI) is a tool to quantitatively assess estuary health based on sample counts of benthic fauna that has been used on every continent, except for Antarctica (Bazairi et al., 2005; Medeiros, 2012; Ranasinghe and Salas, 2012; Wenqian et al., 2012). However, it is unclear if these existing indicators are dynamic enough to accurately assess the sometimes rapid effects of anthropogenic and climate change driven modification such as acidification or the development of hypoxic zones (Bianchi and Allison, 2009; Cai et al., 2021) with studies sometimes showing divergent conclusions on different aspects of the same system (Teixeira et al., 2012; Valença and Santos, 2012; Seibel and Childress, 2013; Borja et al., 2019).
In recent decades, ocean conditions (e.g., pH and salinity changes, nonnative species invasions and hypoxia) have shifted dramatically due to climate and anthropogenic stressors (He and Silliman, 2019). Many of these conditions have led to lower environmental resilience, which has resulted in more frequent marine disease outbreaks (Graham et al., 2021; Groner et al., 2021; Thorstad et al., 2021). Diseases are underlying indicators of many critical aspects of marine health, while also providing insights into the relationship between indicators and resulting effects (Lafferty et al., 2008; Groner et al., 2016; Harvell, 2019). For instance, global connectivity through trade has allowed diseases to enter new regions with little resistance or chance of resilience (Abelson et al., 2020). This has shed light on marine diseases as a possible key relevant estuary health indicator (Harvell, 1999; Lafferty, 2004).
1.3 The role of marine diseases and keystone species interactions in estuaries
Monitoring infectious diseases as indicators of estuarine health has recently gained attention given their impact on both host and non-host populations of flora and fauna (Harvell et al., 2002). Parasitism, the mechanism by which diseases are spread by pathogens and parasites (Lafferty and Mordecai, 2016), is the most common consumer strategy in estuaries. Parasites can control host population densities, regulate critical keystone species, influence competitive outcomes (i.e., species invasions), and regulate vital ecosystem engineers via trait- or density-mediated effects (Behrens, 2004; Miura et al., 2006; Kuris et al., 2008; Lafferty et al., 2008; Rossiter, 2013; Broecke et al., 2019). Estuarine food webs are closely linked to parasites as they are prey in over half of trophic interactions in global estuaries (Hechinger et al., 2011; Byers, 2020). Nonetheless, research on the causes and dynamics of disease spread and outbreaks in estuaries is lacking (McLaughlin et al., 2020). Studying marine disease dynamics offer a unique opportunity to link functional and structural indicators of estuary health in the context of the influences of climate and anthropogenic stressors on estuaries. In this context, interactions between marine diseases and keystone species could be critical for assessing estuarine health. Estuarine keystone species help define and support the function and structure of entire ecosystems, which make their response to parasitic interactions critical for overall ecosystem health (Chadwick, 2021). Keystone species disproportionately affect the abundance and distribution of other species within an ecosystem; meaning their central functional role can influence complex dynamics. When a disease impacts the abundance, structure, behavior, or resilience of a keystone species in an estuary, trophic cascading effects can lead to long-term changes in ecosystem structure and function (Castello et al., 1995; Eviner and Hawkes, 2008; Preston et al., 2016; Schultz, 2016; Harvell, 2019; Tuohy et al., 2020). For example, their devastating effects on estuarine keystone species have already been documented to include three species of California abalone (Crosson et al., 2014), two species of Caribbean coral (Precht et al., 2002), and one species of west coast sea star (Montecino-Latorre et al., 2016). In each case infectious disease introduction and spread were driven and facilitated by a rapid change in environmental conditions, compared to the historical record. A deeper understanding of the integrated structure and function of an estuary, the role of keystone flora and fauna, and the relationship between estuary health and climate change are key relationships that must be further explored to protect, manage, and conserve estuaries in the 21st century. In this review, we seek to understand how estuarine health has been measured in the past with a focus on the frequency of pathogens as an indicator of estuarine health.
2 Methods
2.1 Systematic quantitative literature review
We conducted a systematic, quantitative literature review on estuary health studies published between 2000 to 2022 during February to September of 2022. This range was selected to analyze the literature at the turn of the 21st century, which aligns with increased documentation of marine disease outbreaks as a prominent part of the literature (Tracy, 2019). We searched four large databases for relevant publications (i.e., Google Scholar, NOAA National Centers for Coastal Ocean Science, Web of Science, and PubMed) using 14 sets of search terms related to marine diseases and estuary health starting with the keyword “health” in combination with the following terms: ‘marine’, ‘estuary’, ‘intertidal zone’, ‘keystone estuary species’, ‘estuary disease’, ‘marine disease’, ‘intertidal zone disease’, ‘estuary resilience’, ‘climate change and estuaries’, ‘marine ecotone’. Note that in our results (Section 3), we use “pathogens” as a proxy for marine disease dynamics because pathogens have clearer definition in the current literature (Ward and Lafferty, 2004; Balloux and van Dorp, 2017).
The initial search resulted in 3,107 relevant publications in total (Figure 1). An additional 46 primary sources were found within the cited literature of five relevant reviews, which resulted in a total of 3,153 studies (Orth et al., 2006; Lafferty et al., 2008; Groner et al., 2016; Harvell, 2019; McLaughlin et al., 2020). We removed 1,679 duplicate publications, which left a total of unique 1,474 publications. Publications included in our final review centered on marine health by focusing on a minimum of one assessment type of the Estuary Health Index (i.e., Heydorn’s assessments, Ramm’s Community Degradation Index, Cooper’s Estuarine Health Index, CERM’s Index of Physical Health, Van Driel’s Estuary Habitat Integrity Index, Whitfield’s qualitative assessment, or Harrison & Whitfield’s Estuarine Fish Community Index; Turpie et al., 2013). Thematic relevance of each paper’s abstracts, methods, and discussions/conclusions sections were determined using the text analysis software Google Cloud Natural Language Processing (NLP) with the REST API (Natsiavas et al., 2017; Ozturk et al., 2022). This software was chosen because it provides real-time analysis of insights stored in unstructured text, especially in the medical and health sciences (Google Cloud, 2023). Using thousands of pre-trained classifications, we fed in the 14 sets of key search terms into the natural language processing model and asked for all publications relevant on these bases.
A total of 1,120 publications were excluded due to key words focusing on either non-marine systems or on diseases that primary originate and circle within the human community, as well as for other reasons such as focus on economic impacts (e.g., property damage and business losses), beach closures, or harmful algal blooms, all of which are not relevant for or do not meet our eligibility criteria. Although keystone species have been frequently studied, we use the software to analyze their frequency in the context of ecosystem health. The relevance review resulted in a final total of 351 publications that fit our criteria for selection. After selecting these studies, we ran the natural language processing model on both groups of papers once again, for verification. We ran the software on all 1,120 publications that were excluded, for verification, and on all 351 papers included in the final model, to ensure that we had selected the most pertinent pool of publications for our purposes. An additional systematic search of the literature was conducted in February of 2023, to account for new publications prior to manuscript submission, netting 3 more publications for a total of 354 publications. Additional information gathered from the final publications included the type of study (i.e., genetic, experimental, review, observational, or modeling studies), the period of documentation (i.e., the year of publication), and core themes of the literature (i.e., human stressors, climate, economic, and ecological impacts).
The resulting studies were broken down into six estuary health indicator categories (i.e., hydrologic alteration, landcover/land use change (LCLUC), chemical pollutants, nutrient pollution, invasive species, and pathogens) to analyze temporal trends in estuarine health studies (Turpie et al., 2013; NOAA, 2022a). We further dissected each category with relation to four primary, complex natural and anthropogenic factors that have influenced humans to manage ecosystems across time (i.e., human stressors, climate change, economic impacts, and ecological impacts; Hoegh-Guldberg and Bruno, 2010; Chi et al., 2018). We ensured that any category of health measured in our study could fit into one of these four overarching factors (Moss et al., 2010). Study type were categorized into experimental, observational, review, genetics, or modeling based-studies (Röhrig et al., 2009) (Table 1).
Finally, we documented the number of health studies that addressed keystone species of estuarine ecosystems. We compared this to studies that focused specifically on keystone species and estuary ‘natural processes’, specifically on their ‘general role’ in estuary ecosystems, or specifically on ‘environmental or anthropogenic factors’. For the last category, studies were distinguished based on whether the key term “health” was discussed in the context of environmental or anthropogenic factors. Mann-Kendall tests were performed to assess the significance of trends over time.
3 Results
3.1 Most prominent health issues in estuaries
Over the past 22 years, estuarine health studies focused on pathogen dynamics have increased in their share of estuarine health literature more rapidly compared to other types of studies (Figure 2). Prior to 2007 there were a total seven studies focused on pathogens, however, there has been a year after year increase up to 2022 with 30 being published in that year. Invasive species studies show a similar increasing temporal trend (Mann-Kendall p-value < 0.05) with an increase in number studies of 23 between 2000-2022. Hydrologic alteration and LCLUC experienced similar temporal declines in relevance with each declining by 12 and 15 studies between 2000-2022, respectively. Finally, chemical, and nutrient pollution both have increasing temporal trends with chemical pollutants becoming the most common study type by 2022.
Pathogen focused studies were weighted primarily towards genetics, review, and experimental studies, with near to no observational or modeling studies (Figure 3). Pathogens also observed several gaps in the literature across time, such as during 2001, 2003, 2004, 2007, 2010, and 2017. We observed the highest number of studies pertaining to pathogens between 2018 to 2022. This category is also experiencing an increasing variety of studies being conducted in more recent years, especially because there is now groundwork laid out for observational and modeling studies to occur (Mann-Kendall p-value < 0.05). We observed that draining, damming, dredging, and filling demonstrated a mix of study types throughout the twenty-two years, with no gaps throughout the years as well. Habitat conversion and habitat loss demonstrated more modeling studies between 2000 to 2012; toxic substances showed more weight towards modeling, genetics, and experimental studies; nutrient pollution and eutrophication showcased more observational studies throughout the years; and invasive species demonstrated a mix of all types of studies with observational and review studies occurring consistently throughout time. All these categories also demonstrated no temporal gaps in their appearance in the literature.
3.2 Pathogens and complex natural and anthropogenic factors and their relationship to ecosystem management across time
We further analyzed the potential drivers for the change in study prevalence over time. Presently, economic impacts are driving the most increase in pathogen related studies, but human stressors, ecological impacts, and climate change are all slowly increasingly over time as well (Figure 4). Compared to other categories, we observed that economic impact studies were driving several of the conversations in the literature far more than human stressors, climate change, or ecological impacts (with an economic impact studies Mann-Kendall significance of p < 0.05). Out 354 studies we analyzed, only 75 focused specifically on keystone species from 2000-2022 (Figure 5). Only five percent of those total studies focused on keystone species and health, while keystone species in the primary context of natural processes (32 percent), of their general ecosystem role (39 percent), or of environmental or anthropogenic stressors (24 percent) made up most of the studies that focused specifically on keystone species in context of economic impact.
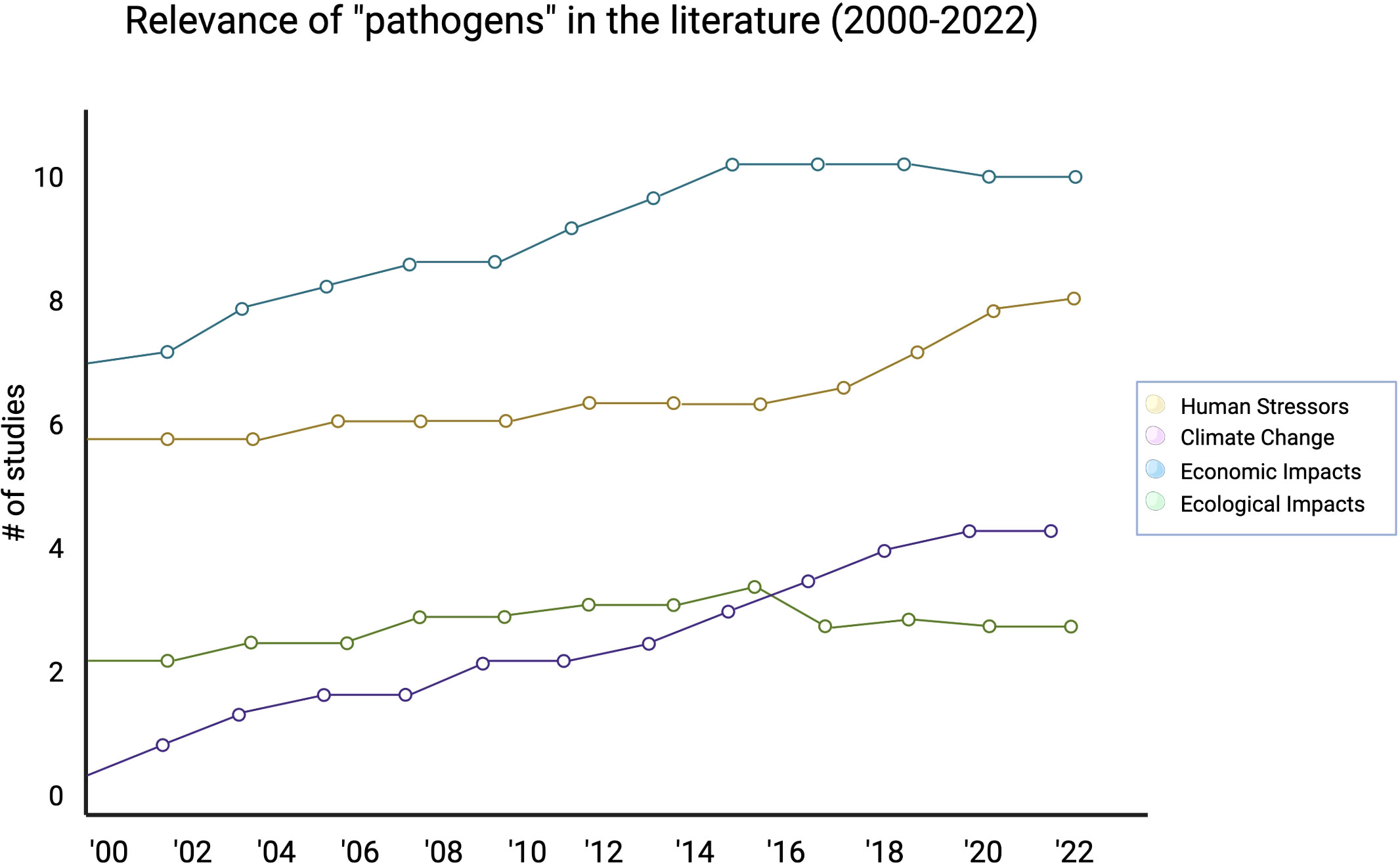
Figure 4 A closer look at potential drivers for the change in “pathogen” study prevalence across time.
3.3 The broader picture
The increasing frequency and lack of predictability of climate and anthropogenic stressors can serve as a call to action for increasing the understanding of estuarine health and its integration across multiple components of the system. Although estuary health has been quantified through a variety of indicators (Turpie et al., 2013), our understanding of the role of pathogens in this dynamic remains poorly studied. Even in the early 2000s, the study of pathogens in estuary ecosystems was largely missing (Table 1). It wasn’t until roughly 2010 that we noticed a spike in the literature for pathogen dynamics in estuaries. Even so, studies show heavy bias towards commercially important systems, such as pathogens that result from and impact aquaculture. We also observed a greater need for more quantitative studies of estuary health, given a tilt primarily towards review and experimental studies, historically. The publications from this study also made note of the need for repeated quantitative ecological assessments (i.e., interannual or annual surveys, drone-based data surveys, etc.) to detect and measure estuary health trends (Cai et al., 2021). Giari and Lafferty both also note, on separate occasions, that pathogens have also had historic influences on the biodiversity, ecosystem makeup, evolution, and functionality of estuaries, and therefore, understanding their role in estuary health is going to be critical for the long-term well-being of estuaries. There is a need to reconsider and adjust quantitative indicators that are relevant to the emerging threats of climate- and anthropogenic-related influences on estuarine ecosystems. Marine diseases (pathogens) are a key indicator and underlying unifier of health metrics for estuaries and are going to be vital to our understanding of coastal and estuary responses in the face of climate change and anthropogenic stressors.
4 Discussion
Marine diseases are a unifier of structural and functional estuary health indicators that must be studied further. Our review of twenty-two years of literature unveiled disproportionate research on more traditional ecological indicators of estuary health and minimal updates in the indices used to measure estuary health in the face of climate change and anthropogenic stressors. Several studies highlighted urgent needs for practitioners to incorporate climate change adaptation into conservation and management in accordance with well-researched frameworks (Chevillot et al., 2019; O’Regan et al., 2021). Our review also found a significant increase in pathogen inclusion in the literature since 2007, and are becoming a vital indictor of estuary health (Couch and Fournie, 2021). Still, the studies since 2007 are primarily review- and experimental-based and the increased literature calls for more modeling and observational studies going forward. Our recommendation is that the best way to begin incorporating pathogen dynamics into future literature is to begin with a focus on keystone species, given their disproportionately influential roles with regards to ecosystem stability, resilience, and health.
We observed that estuaries were most often studied in the context economic impacts (Figure 3). Apart from disease, the different indicators of estuary health had consistent presence in the literature. Disease was sporadically studied as a driver of estuary health change with a significant increases in pathogen-based studies occurring after 2007 (Lafferty and Mordecai, 2016). Further, the initial increase was largely driven by review and experimental studies with a subsequent wave of increases due to observational and modeling studies. This may have been motivated by the new literature being generated for marine-based diseases, which can use published data for parameterization and interpretation (Hoenig et al., 2017; Trochta et al., 2022). Finally, our data show that studies do not often make the connection between pathogen dynamics and keystone species.
As we have attempted to show, the disease literature lags the more salient environmental changes of its time. Although marine diseases (pathogens) are no different in that capacity, they are of particular concern and urgent importance because they play incredibly dynamic roles in the environment (Sobocinski et al., 2022). Estuaries are not only complex ecotones that glean insight into the nature of freshwater and saltwater ecosystems in the face of climate change, but they can serve the same purpose with regards to their connection between the most fundamental of ecosystems - land and sea. They are incredibly unique in this regard, so their very richness coinciding with anthropogenic habitation is a significant threat to both terrestrial and marine environments. At the same time, they can therefore provide many answers to underlying questions of climate change and anthropogenic stress.
4.1 Estuarine health literature dynamics in the context of environmental change
The emphasis of estuarine health in the literature tends to follow the most pressing environmental challenges of that time (Zoffoli et al., 2020). Until the last few decades, many estuary habitats in North America were drained and developed for agriculture and urban expansion (Abrahim and Parker, 2002; Yang and Liu, 2005). In the United States alone, 38 percent of estuaries and wetlands associated with coastal regions have been converted for urban expansion or permanently lost (NOAA, 2022b). The effect of this history is highlighted in our results, with most studies focusing on impacts of draining, damming, dredging, and filling of estuaries in the context of habitat loss and conversion. Nutrient pollution has been one of the most important threats to the water quality of estuaries with resulting eutrophication contributing to harmful algal blooms and anoxic conditions that impacts wild and commercial flora and fauna (Fowles et al., 2018). Invasive species were also a large part of the literature (Figure 1), especially given that estuaries and coasts are particularly susceptible, for instance through shipping and boating (Ruiz et al., 2000), aquarium trade (Padilla and Williams, 2004), and aquaculture (Williams and Grosholz, 2008). While each of the previously mentioned estuary health indicators have been in the literature consistently over our period of observation, pathogen outbreaks appears to have increased with more frequent environmental disease outbreaks since the early 2000s (Patz et al., 2000). The reason this is notable is because pathogen outbreaks can cause severe, large-scale, and sometime irrecoverable damage to already complex ecosystems like estuaries (Lafferty, 2004). Previously not considered in most estuary health indices (Table 1), pathogen dynamics play important roles in ecosystems than we previously understood. Studies indicate that they will be an area of ongoing concern with increasingly severe climate change and anthropogenic stress (Rama et al., 2022). We notice the pathogens category underwent the most significant growth in the number of studies conducted over the last twenty-two years of the six observed categories, from 0 in 2000 to nearly 35 by 2022. Marine disease (pathogen) dynamics make indirect appearances in about 38 percent of the overall 354 studies simply because of the ubiquitous nature of pathogen dynamics (Lafferty et al., 2008; Lafferty and Mordecai, 2016). Although these numbers are still relatively minimal in the large scheme of estuary health, they motivate more research into the potentially unique role pathogen dynamics play between structural and functional components of estuary health (Harvell, 2019).
4.2 Understanding how pathogens integrate into the current literature
Our review indicates that marine diseases inclusion in the literature has increased since 2007 and are becoming vital indictors of estuary health. Currently, economic losses are the primary driver of pathogen inclusion in the literature both prior to and following the growing body of disease literature (Kennish, 2002; Malham K, et al., 2014). Pathogens were included in the literature as paired with disease outbreaks resulting in mass die-offs of commercial fish catch, losses in employment opportunities, and threats to coastal blue carbon storage, water quality, flood protection, and coastal infrastructure (Chesney et al., 2000; Hoagland et al., 2002; Short, 2004; Thronson and Quigg, 2008; de Jesús Crespo et al., 2019; Thorhaug et al., 2019). Opportunistic marine pathogens are ubiquitous in the environment and cause disease in immune-compromised or stressed hosts. Although this has shown that disease can be a natural part of all ecosystems, an increasing number of cases show shifts in the balance between the host, pathogen, and the environment. Just like mass die-offs of commercial fish, mass die-offs of wild marine populations can also persist. Furthermore, the rapid changes occurring in our world’s oceans right now, coupled with other anthropogenic stressors, will likely lead to more opportunistic diseases in the marine environment (Burge et al., 2013).
Human stressors were the next major category that pathogen-based studies were categorized under, which is associated with economic losses (Elliott and Whitfield, 2011). Studies discussed pathogens and disease outbreaks in the context of anthropogenic activities increasing fecal bacteria loads, pathogenic viruses and nutrients in rivers, and estuaries and coastal areas being targets of point and diffuse sources such as sewage discharges and agricultural runoff (Kennish, 2022). Studies also discussed this in the context of a variety of physical, chemical, and biological processes (often disturbed or influenced by humans) inducing the co-flocculation of microorganisms, with mineral particles and organic matter resulting in the likelihood of pathogenic outbreaks (Malham et al., 2014). This is critical, as anthropogenic stressors are leading to rising temperatures, eutrophication, ocean acidification, habitat destruction and loss of biodiversity, and extreme weather events, which are in turn leading to disease outbreaks in marine environments (Sobocinski et al., 2022).
Studies with a focus on climate change increased in frequency after 2010 (Weber, 2016), which may indicate a shift in research priorities (Scanes et al., 2020a). Several studies analyze data from long-term monitoring programs, noticing unusual rates of temperature change, warming, and acidification. It has been noted that the response of estuaries to disease outbreaks in the face of climate change can be dependent on estuary morphology (Gillanders et al., 2022). Studies found that lagoonal and riverine estuaries are both acidifying and warming at rates faster than historically seen, partially due to shallow average depths and limiting oceanic exchanges (Scanes et al., 2020b). Finally, multiple studies related to climate also noted that models, measured on an order of magnitude faster than global ocean predictions and atmospheric models, may not be as useful for predicting the response of estuaries to climate change – an incredibly critical observation for modeling and management (Ruggiero et al., 2010; Scanes et al., 2020b; Chilton et al., 2021; Lonsdale et al., 2022). This means that predicting the response of estuaries to global climate change remains speculative. We severely need a new generation of indicators for marine waters (Niemi et al., 2004).
The studies produced since 2007 are primarily review- and experimental-based, however more modeling and observational studies are needed going forward to fully capture the effect of pathogens in estuaries. Data that quantify the impacts of environmental parameters on a disease or diseases in estuary flora and fauna can provide new and importantly, critical, insights into how disease interacts with host populations. More observational and modeling studies can offer linkages between disease patterns and disease outbreaks in the spotlight of climate change and larger climate controlling processes. Greater research focused on modeling and observations might reveal more detail on the strong effect pathogens have on population levels in estuaries, which might be critical if keystone species are affected. Few observational or modeling studies, especially long-term studies, exist to describe these relationships (Lafferty et al., 2008; Bushek et al., 2012). If we are going to successfully manage complex ecosystems like estuaries, we will need to better understand the impetus for and types of pathogen-based research in estuarine systems.
4.3 The role of keystone species in the literature
Our recommendation is that the best way to begin incorporating pathogen dynamics into future literature is to initially focus on keystone species, given their disproportionate influence on ecosystem stability, resilience, and health (Table 2). When a pathogen or disease outbreak stresses the function, structure, or resilience of a keystone species in an estuary, there are bound to be long-term impacts or potential changes to ecosystem structure and/or function (Castello et al., 1995; Schultz, 2016; Graham et al., 2021). Of the documented literature, 21 percent mention keystone species with 9 percent of those 74 studies focusing on interactions between parasites and keystone species. Changes in the abundance of keystone species due to a disease outbreak can have major cascading effects in ecosystem function and community composition. This has already been documented with sea otters in coastal California being affected by pathogens (Ostfeld et al., 2008) and the rocky intertidal zone starfish Pisaster ochraceus (Hajishengallis et al., 2012) falling victim to pathogenic disease outbreaks. There is indication in the literature that if the relationship between keystone species and the microbial ecology of an environment could be elucidated further, there could be essential insights made into the structure of marine pathogenic communities and their interplay with their environment and hosts (Estes and Palmisano, 1974; Ostfeld et al., 2008; Hajishengallis et al., 2012; Danilović et al., 2022). Such thematic findings, along with the dearth of literature on this interaction, highlight a crucial need for significantly more study.
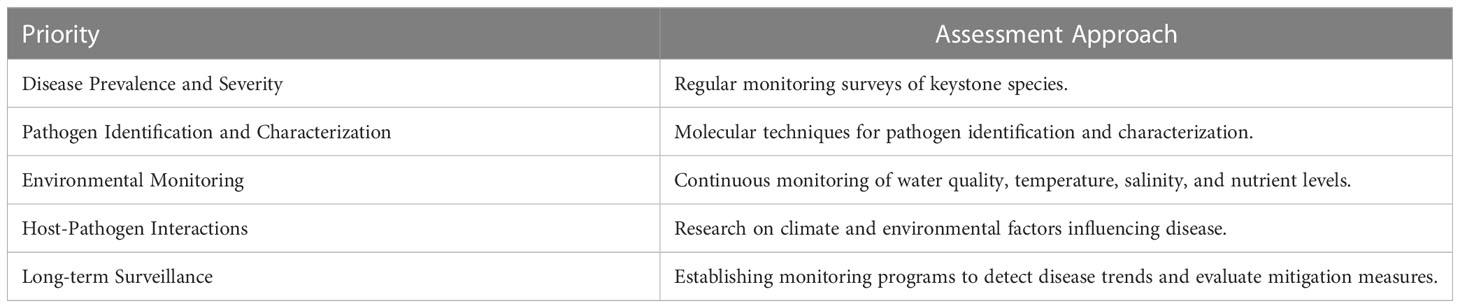
Table 2 Representation of how the identified priorities can be utilized in the assessment of estuarine health.
4.4 Future monitoring
Through a quantitative systematic literature review, we have examined the ecological hypothesis that pathogen dynamics have experienced an upward trend over the past two decades. Based on the key conclusions drawn from this analysis, we propose that forthcoming marine health monitoring programs should place pathogen dynamics at the core of their surveillance efforts, recognizing it as a central and unifying factor in assessing the well-being of global estuaries. Considering the intricate impacts of global climate change on diseases, comprehensively tracking, and comprehending the layered dynamics of marine diseases, including their occurrence patterns, will be crucial for conserving ecosystems that face increasing anthropogenic and climate-related stressors.
Based on the comprehensive review of literature on marine diseases and their relationship with global estuaries, we have identified several key priorities and approaches that should be considered in the development of future high-quality marine health monitoring programs. These priorities are crucial for assessing and safeguarding the health of estuarine ecosystems. Here, we present a list of these priorities along with a suggested table that demonstrates their potential use in the assessment of estuarine health:
1. Disease Occurrence and Distribution Mapping:
• Develop a standardized approach to map the occurrence and distribution of marine diseases within estuaries.
• Utilize spatial analysis techniques to identify disease hotspots and track changes over time.
• Establish a database to collect and share disease occurrence data across estuaries globally.
2. Long-term Monitoring of Disease Trends:
• Implement long-term monitoring programs to assess temporal trends in disease prevalence and intensity.
• Utilize standardized sampling protocols and diagnostic techniques for consistent and comparable data collection.
• Analyze trends to identify patterns, seasonality, and potential drivers of disease dynamics.
3. Multi-disciplinary Approaches:
• Foster collaborations between researchers, practitioners, and stakeholders from different disciplines (e.g., marine ecology, public health, climate science) to gain a comprehensive understanding of disease dynamics.
• Integrate socio-economic and ecological data to assess the impacts of diseases on estuarine communities and ecosystems.
4. Early Warning Systems:
• Develop early warning systems that incorporate environmental and biological indicators to predict disease outbreaks.
• Establish real-time monitoring networks for continuous data collection and timely response to emerging disease events.
5. Ecosystem Health Indicators:
• Identify and monitor key indicator species and ecological parameters that reflect the overall health of estuaries.
• Link disease occurrence and severity to ecosystem health indicators, providing a holistic assessment of estuarine well-being.
To facilitate a better understanding of these priorities, we have compiled a table (Table 1) that outlines their respective utilization in the assessment of estuarine health. This table serves as a practical tool for designing and implementing effective monitoring programs. By integrating these priorities into estuarine health assessments, monitoring programs can effectively track and respond to the evolving dynamics of marine diseases. Moreover, these efforts should be carried out within the broader context of understanding the impacts of climate change on disease dynamics, and how they intersect with other anthropogenic stressors. This comprehensive approach will enable the conservation and management of estuarine ecosystems under increasing threats from both natural and human-induced stressors.
Author contributions
Conceptualization, KT. Methodology, KT. Formal analyses, KT. Writing-original draft preparation, KT. Writing-all other drafts, KT. Writing-review and editing, KT, EWJ. Supervision, EWJ. All authors contributed to the article and approved the submitted version.
Funding
The lead author was supported by the National Science Foundation (NSF) Graduate Research Fellowship Program. The statements, findings, conclusions, and recommendations are those of the authors and do not necessarily reflect the views of NSF.
Acknowledgments
We would like to thank Dr. Elizabeth Hadly for her support in reviewing this manuscript. We would also like to thank the insights we received from the National Estuarine Research Reserve and the National Parks Service with regards to estuary management and diseases along United States coastlines. We would also like to thank key mentors Dr. Drew Harvell, Dr. Kevin Lafferty, and Dr. Sandy Wyllie-Echeverria on their guidance and support, along with faculty members Dr. Kabir Peay, Dr. Rob Dunbar, and Dr. Barnabas Daru.
Conflict of interest
The authors declare that the research was conducted in the absence of any commercial or financial relationships that could be construed as a potential conflict of interest.
Publisher’s note
All claims expressed in this article are solely those of the authors and do not necessarily represent those of their affiliated organizations, or those of the publisher, the editors and the reviewers. Any product that may be evaluated in this article, or claim that may be made by its manufacturer, is not guaranteed or endorsed by the publisher.
Supplementary material
The Supplementary Material for this article can be found online at: https://www.frontiersin.org/articles/10.3389/fmars.2023.1185662/full#supplementary-material
References
Abelson A., Reed D. C., Edgar G. J., Smith C. S., Kendrick G. A., Orth R. J., et al. (2020). Challenges for restoration of coastal marine ecosystems in the anthropocene. Front. Mar. Sci. 7. doi: 10.3389/fmars.2020.544105
Abrahim G., Parker R. (2002). Heavy-metal contaminants in tamaki estuary: impact of city development and growth, Auckland, new Zealand. Env. Geol 42, 883–890. doi: 10.1007/s00254-002-0593-0
Balloux F., van Dorp L. (2017). Q&A: what are pathogens, and what have they done to and for us? BMC Biol. 15, 91. doi: 10.1186/s12915-017-0433-z
Bazairi H., Bayed A., Hily C. (2005). Structure and bioassessment of benthic communities of a lagoonal ecosystem of the Atlantic Moroccan coast. Comptes Rendus Biologies 328, 977–990. doi: 10.1016/j.crvi.2005.09.006
Behrens M. (2004) (18) (PDF) effects of marine reserves and urchin disease on southern Californian rocky reef communities. Available at: https://www.researchgate.net/publication/250218163_Effects_of_marine_reserves_and_urchin_disease_on_southern_Californian_rocky_reef_communities (Accessed 9.1.22).
Bianchi T. S., Allison M. A. (2009). Large-River delta-front estuaries as natural “recorders” of global environmental change. Proc. Natl. Acad. Sci. 106, 8085–8092. doi: 10.1073/pnas.0812878106
Borja A., Chust G., Muxika I. (2019). “Chapter three - forever young: the successful story of a marine biotic index,” in Advances in marine biology. Ed. Sheppard C. (Academic Press), 93–127. doi: 10.1016/bs.amb.2019.05.001
Bradley M. (2017) Hidden components in tropical seascapes: deep-estuary habitats support unique fish assemblages (Springer Link). Available at: https://link.springer.com/article/10.1007/s12237-016-0192-z (Accessed 8.31.22).
Broecke B. V., Mariën J., Sabuni C. A., Mnyone L., Massawe A. W., Matthysen E., et al. (2019). Relationship between population density and viral infection: a role for personality? Ecol. Evol. 9, 10213–10224. doi: 10.1002/ece3.5541
Burge C. A., Kim C. J. S., Lyles J. M., Harvell C. D. (2013). Special issue oceans and humans health: the ecology of marine opportunists. Microb. Ecol. 65, 869–879. doi: 10.1007/s00248-013-0190-7
Bushek D., Ford S. E., Burt I. (2012). Long-term patterns of an estuarine pathogen along a salinity gradient. J. Mar. Res. 70, 225–251. doi: 10.1357/002224012802851968
Byers J. E. (2020). Effects of climate change on parasites and disease in estuarine and nearshore environments. PloS Biol. 18, e3000743. doi: 10.1371/journal.pbio.3000743
Cai W.-J., Feely R. A., Testa J. M., Li M., Evans W., Alin S. R., et al. (2021). Natural and anthropogenic drivers of acidification in Large estuaries. Annu. Rev. Mar. Sci. 13, 23–55. doi: 10.1146/annurev-marine-010419-011004
Castello J., Leopold D., Smallidge P. (1995). Pathogens, patterns, and processes in forest ecosystems. Bioscience 45. doi: 10.2307/1312531
Chadwick (2021) Keystone species: how predators create abundance and stability – mother earth news. Available at: https://www.motherearthnews.com/sustainable-living/nature-and-environment/keystone-species-zm0z11zrog/ (Accessed 12.1.22).
Chesney E. J., Baltz D. M., Thomas R. G. (2000). Louisiana Estuarine and coastal fisheries and habitats: perspectives from a fish’s eye view. Ecol. Appl. 10, 350–366. doi: 10.1890/1051-0761(2000)010[0350:LEACFA]2.0.CO;2
Chevillot X., Tecchio S., Chaalali A., Lassalle G., Selleslagh J., Castelnaud G., et al. (2019). Global changes jeopardize the trophic carrying capacity and functioning of estuarine ecosystems. Ecosystems 22, 473–495. doi: 10.1007/s10021-018-0282-9
Chi Y., Zheng W., Shi H., Sun J., Fu Z. (2018). Spatial heterogeneity of estuarine wetland ecosystem health influenced by complex natural and anthropogenic factors. Sci. Total Environ. 634, 1445–1462. doi: 10.1016/j.scitotenv.2018.04.085
Chilton D., Hamilton D. P., Nagelkerken I., Cook P., Hipsey M. R., Reid R., et al. (2021). Environmental flow requirements of estuaries: providing resilience to current and future climate and direct anthropogenic changes. Front. Environ. Sci. 9. doi: 10.3389/fenvs.2021.764218
Cloern J. E., Abreu P. C., Carstensen J., Chauvaud L., Elmgren R., Grall J., et al. (2016). Human activities and climate variability drive fast-paced change across the world’s estuarine–coastal ecosystems. Global Change Biol. 22, 513–529. doi: 10.1111/gcb.13059
Cooper J. A. G. (2001). Geomorphological variability among microtidal estuaries from the wave-dominated south African coast. Geomorphology 40 (1–2), 99–122. doi: 10.1016/S0169-555X(01)00039-3
Costanza R., de Groot R., Sutton P., van der Ploeg S., Anderson S. J., Kubiszewski I., et al. (2014a). Changes in the global value of ecosystem services. Global Environ. Change 26, 152–158. doi: 10.1016/j.gloenvcha.2014.04.002
Costanza R., de Groot R., Sutton P., van der Ploeg S., Anderson S. J., Kubiszewski I., et al. (2014b). Changes in the global value of ecosystem services. Global Environ. Change 26, 152–158. doi: 10.1016/j.gloenvcha.2014.04.002
Costanza R. (1991). Ecological economics: the science and management of sustainability. (Columbia University Press)
Crosson L. M., Wight N., VanBlaricom G. R., Kiryu I., Moore J. D., Friedman C. S. (2014). Abalone withering syndrome: distribution, impacts, current diagnostic methods and new findings. Dis. Aquat. Organisms 108, 261–270. doi: 10.3354/dao02713
Cyrus D. P., Forbes A. T. (1996). Preliminary results on the role of KwaZulu-natal harbours as nursery grounds for juveniles of selected marine organisms which utilize estuaries. South Afr. J. Wildlife Res. - 24-month delayed Open Access 26, 26–33. doi: 10.10520/EJC116987
Danilović M., Maguire I., Füreder L. (2022). Overlooked keystone species in conservation plans of fluvial ecosystems in southeast Europe: a review of native freshwater crayfish species. Knowl. Manage. Aquat. Ecosyst. 21. doi: 10.1051/kmae/2022016
Deegan L. A., Finn J. T., Ayvazian S. G., Ryder-Kieffer C. A., Buonaccorsi J. (1997). Development and validation of an estuarine biotic integrity index. Estuaries 20, 601–617. doi: 10.2307/1352618
de Jesús Crespo R., Méndez Lázaro P., Yee S. H. (2019). Linking wetland ecosystem services to vector-borne disease: dengue fever in the San Juan bay estuary, Puerto Rico. Wetlands 39, 1281–1293. doi: 10.1007/s13157-017-0990-5
Elliott M., Whitfield A. K. (2011). Challenging paradigms in estuarine ecology and management. Estuarine Coast. Shelf Sci. 94, 306–314. doi: 10.1016/j.ecss.2011.06.016
Estes J. A., Palmisano J. F. (1974). Sea Otters: their role in structuring nearshore communities. Science 185, 1058–1060. doi: 10.1126/science.185.4156.1058
Eviner V. T., Hawkes C. V. (2008). Embracing variability in the application of plant-soil interactions to the restoration of communities and ecosystems. Restor. Ecol. 16, 713–729. doi: 10.1111/j.1526-100X.2008.00482.x
Fairweather P. G. (2009). Determining the ‘health’ of Estuaries: priorities for ecological research. Austral. Ecol. 24 (4), 441–451. doi: 10.1046/j.1442-9993.1999.00976.x
Fowles A. E., Edgar G. J., Stuart-Smith R. D., Kirkpatrick J. B., Hill N., Thomson R. J., et al. (2018). Effects of pollution from anthropogenic point sources on the recruitment of sessile estuarine reef biota. Front. Mar. Sci. 5. doi: 10.3389/fmars.2018.00417
Gillanders B. M., McMillan M. N., Reis-Santos P., Baumgartner L. J., Brown L. R., Conallin J., et al. (2022). “Climate change and fishes in estuaries,” in Fish and fisheries in estuaries (John Wiley & Sons, Ltd), 380–457. doi: 10.1002/9781119705345.ch7
Google Cloud (2023) Available at: https://arxiv.org/abs/2304.13738
Graham O. J., Aoki L. R., Stephens T., Stokes J., Dayal S., Rappazzo B., et al. (2021). Effects of seagrass wasting disease on eelgrass growth and belowground sugar in natural meadows. Front. Mar. Sci. 8. doi: 10.1098/rstb.2022.0016
Groner M. L., Eisenlord M. E., Yoshioka R. M., Fiorenza E. A., Dawkins P. D., Graham O. J., et al. (2021). Warming sea surface temperatures fuel summer epidemics of eelgrass wasting disease. Mar. Ecol. Prog. Ser. 679, 47–58. doi: 10.3354/meps13902
Groner M. L., Maynard J., Breyta R., Carnegie R. B., Dobson A., Friedman C. S., et al. (2016). Managing marine disease emergencies in an era of rapid change. Philos. Trans. R. Soc. B: Biol. Sci. 371, 20150364. doi: 10.1098/rstb.2015.0364
Hajishengallis G., Darveau R. P., Curtis M. A. (2012). The keystone-pathogen hypothesis. Nat. Rev. Microbiol. 10, 717–725. doi: 10.1038/nrmicro2873
Harvell C. D. (1999) Emerging marine diseases–climate links and anthropogenic factors. Available at: https://pubmed.ncbi.nlm.nih.gov/10498537/ (Accessed 10.4.22).
Harvell D. (2019) Disease epidemic and a marine heat wave are associated with the continental-scale collapse of a pivotal predator (Pycnopodia helianthoides) | science advances. Available at: https://www.science.org/doi/10.1126/sciadv.aau7042 (Accessed 9.1.22).
Harvell (2022) Available at: https://www.science.org/doi/full/10.1126/science.1063699?casa_token=A7sNJowvmOgAAAAA%3AeHsKx8g3bx6TBn2QD8lI0ZBLgCYRnkDzG8c-bCsU1_JGGB4M3oS0_94TBoiS7YpqdxlaHdMdPzhd_Q
He Q., Silliman B. R. (2019). Climate change, human impacts, and coastal ecosystems in the anthropocene. Curr. Biol. 29, R1021–R1035. doi: 10.1016/j.cub.2019.08.042
Hechinger R. F., Lafferty K. D., McLaughlin J. P., Fredensborg B. L., Huspeni T. C., Lorda J., et al. (2011). Food webs including parasites, biomass, body sizes, and life stages for three California/Baja California estuaries. Ecology 92, 791–791. doi: 10.1890/10-1383.1
Hoagland P., Anderson D. M., Kaoru Y., White A. W. (2002). The economic effects of harmful algal blooms in the united states: estimates, assessment issues, and information needs. Estuaries 25, 819–837. doi: 10.1007/BF02804908
Hoegh-Guldberg O., Bruno J. F. (2010). The impact of climate change on the world’s marine ecosystems. Science 328, 1523–1528. doi: 10.1126/science.1189930
Hoenig J. M., Groner M. L., Smith M. W., Vogelbein W. K., Taylor D. M., Landers D. F. Jr., et al. (2017). Impact of disease on the survival of three commercially fished species. Ecol. Appl. 27, 2116–2127. doi: 10.1002/eap.1595
Karr J. R. (1987). Biological monitoring and environmental assessment: a conceptual framework. Environ. Manage. 11 (2), 249–256. doi: 10.1007/BF01867203
Kennedy R., Arthur W., Keegan B. F. (2011). Long-term trends in benthic habitat quality as determined by multivariate AMBI and infaunal quality index in relation to natural variability: a case study in kinsale harbour, south coast of Ireland. Mar. Pollut. Bull. 62 (7), 1427–1436. doi: 10.1016/j.marpolbul.2011.04.030
Kennish M. J. (2002). Environmental threats and environmental future of estuaries. Environ. Conserv. 29, 78–107. doi: 10.1017/S0376892902000061
Kennish M. J. (2022). Management strategies to mitigate anthropogenic impacts in estuarine and coastal marine environments: a review. Open J. Ecol. 12, 667–688. doi: 10.4236/oje.2022.1210038
Kuris A. M., Hechinger R. F., Shaw J. C., Whitney K. L., Aguirre-Macedo L., Boch C. A., et al. (2008). Ecosystem energetic implications of parasite and free-living biomass in three estuaries. Nature 454, 515–518. doi: 10.1038/nature06970
Lafferty K. D. (2004) Are diseases increasing in the ocean? | annual review of ecology, evolution, and systematics. Available at: https://www.annualreviews.org/doi/abs/10.1146/annurev.ecolsys.35.021103.105704 (Accessed 10.4.22).
Lafferty K. D., Allesina S., Arim M., Briggs C. J., De Leo G., Dobson A. P., et al. (2008). Parasites in food webs: the ultimate missing links. Ecol. Lett. 11, 533–546. doi: 10.1111/j.1461-0248.2008.01174.x
Lafferty K. D., Mordecai E. A. (2016). The rise and fall of infectious disease in a warmer world. F1000Res. 5, F1000 Faculty Rev-2040. doi: 10.12688/f1000research.8766.1
Liu S., Haijing Z., He Y., Cheng X., Haofei Z., Qin Y., et al. (2021). Interdecadal variability in ecosystem health of changjiang (Yangtze) river estuary using estuarine biotic integrity index. J. Ocean. Limnol. 39, 1417–1429. doi: 10.1007/s00343-020-0188-1
Lonsdale J.-A., Leach C., Parsons D., Barkwith A., Manson S., Elliott M. (2022). Managing estuaries under a changing climate: a case study of the Humber estuary, UK. Environ. Sci. Policy 134, 75–84. doi: 10.1016/j.envsci.2022.04.001
Malham S. K., Rajko-Nenow P., Howlett E., E. Tuson K., L. Perkins T., W. Pallett D., et al. (2014). The interaction of human microbial pathogens, particulate material and nutrients in estuarine environments and their impacts on recreational and shellfish waters. Environ. Science: Processes Impacts 16, 2145–2155. doi: 10.1039/C4EM00031E
Malham K. S., Rajko-Nenow P., Howlett E., Tuson K. E., Perkins T. L., Pallett D. W., et al. (2014). The interaction of human microbial pathogens, particulate material and nutrients in estuarine environments and their impacts on recreational and shellfish waters. Environ. Science: Processes Impacts 16, 2145–2155. doi: 10.1039/C4EM00031E
Mastrocicco M., Busico G., Colombani N., Vigliotti M., Ruberti D. (2019). Modelling actual and future seawater intrusion in the variconi coastal wetland (Italy) due to climate and landscape changes. Water 11, 1502. doi: 10.3390/w11071502
McLaughlin J. P., Morton D. N., Lafferty K. D. (2020). “Parasites in marine food webs,” in Marine disease ecology. Eds. Behringer D. C., Silliman B. R., Lafferty K. D. (Oxford University Press), 0. doi: 10.1093/oso/9780198821632.003.0002
Medeiros J. (2012) Benthic condition in low salinity areas of the Mira estuary (Portugal): lessons learnt from freshwater and marine assessment tools. Available at: https://agris.fao.org/agris-search/search.do?recordID=US201500162905 (Accessed 8.31.22).
Miura O., Kuris A. M., Torchin M. E., Hechinger R. F., Chiba S. (2006). Parasites alter host phenotype and may create a new ecological niche for snail hosts. Proc. Biol. Sci. 273, 1323–1328. doi: 10.1098/rspb.2005.3451
Montagna P., Vaughan B., Ward G. (2012). “The importance of freshwater inflows to Texas estuaries,” in Water policy in Texas: responding to the rise of scarcity, 107–127. doi: 10.4324/9781936331888
Montecino-Latorre D., Eisenlord M. E., Turner M., Yoshioka R., Harvell C. D., Pattengill-Semmens C. V., et al. (2016). Devastating transboundary impacts of Sea star wasting disease on subtidal asteroids. PloS One 11, e0163190. doi: 10.1371/journal.pone.0163190
Moss R. H., Edmonds J. A., Hibbard K. A., Manning M. R., Rose S. K., van Vuuren D. P., et al. (2010). The next generation of scenarios for climate change research and assessment. Nature 463, 747–756. doi: 10.1038/nature08823
Natsiavas P., Maglaveras N., Koutkias V. (2017). A public health surveillance platform exploiting free-text sources via natural language processing and linked data: application in adverse drug reaction signal detection using pubmed and twitter. In: Riaño D., Lenz R., Reichert M. (eds) Knowledge representation for health care. ProHealth KR4HC 2016 2016. lecture notes in computer science(), vol. 10096. (Cham: Springer). doi: 10.1007/978-3-319-55014-5_4
Niemi G., Wardrop D., Brooks R., Anderson S., Brady V., Paerl H., et al. (2004). Rationale for a new generation of indicators for coastal waters. Environ. Health Perspect. 112, 979–986. doi: 10.1289/ehp.6903
NOAA (2022a) Estuary habitat | NOAA fisheries. Available at: https://www.fisheries.noaa.gov/national/habitat-conservation/estuary-habitat (Accessed 10.3.22).
NOAA (2022b) Human disturbances to estuaries. Available at: https://oceanservice.noaa.gov/education/tutorial_estuaries/est09_humandis.html (Accessed 3.13.23).
O’Brien A., Townsend K., Hale R., Sharley D., Pettigrove V. (2016). How is ecosystem health defined and measured? a critical review of freshwater and estuarine studies. Ecol. Indicators 69, 722–729. doi: 10.1016/j.ecolind.2016.05.004
O’Regan S. M., Archer S. K., Friesen S. K., Hunter K. L. (2021). A global assessment of climate change adaptation in marine protected area management plans. Front. Mar. Sci. 8. doi: 10.3389/fmars.2021.711085
Orth R. J., Carruthers T. J. B., Dennison W. C., Duarte C. M., Fourqurean J. W., Heck K. L., et al. (2006). A global crisis for seagrass ecosystems. BioScience 56, 987–996. doi: 10.1641/0006-3568(2006)56[987:AGCFSE]2.0.CO;2
Ostfeld R. S., Keesing F., Eviner V. T. (2008). Infectious disease ecology: effects of ecosystems on disease and of disease on ecosystems (Princeton University Press).
Ozturk O., Isik M. S., Sariturk B., Seker D. Z. (2022). Generation of Istanbul road data set using Google map API for deep learning-based segmentation. Int. J. Remote Sens. 43 (8), 2793–2812. doi: 10.1080/01431161.2022.2068989
Padilla D., Williams S. (2004). Beyond ballast water: aquarium and ornamental trades as sources of invasive species in aquatic ecosystems. Front. Ecol. Environ. - Front. Ecol. Environ. 2, 131–138. doi: 10.1890/1540-9295(2004)002[0131:BBWAAO]2.0.CO;2
Paerl H. W., Valdes L. M., Joyner A. R., Peierls B. L., Piehler M. F., Riggs S. R., et al. (2006). Ecological response to hurricane events in the pamlico sound system, north Carolina, and implications for assessment and management in a regime of increased frequency. Estuaries Coasts: J. ERF 29, 1033–1045. doi: 10.1007/BF02798666
Patz J. A., Graczyk T. K., Geller N., Vittor A. Y. (2000). Effects of environmental change on emerging parasitic diseases. Int. J. Parasitology Thematic Issue: Emerging Parasite Zoonoses 30, 1395–1405. doi: 10.1016/S0020-7519(00)00141-7
Peng W., Wang D., Cai Y. (2021). Assessing ecological vulnerability under climate change and anthropogenic influence in the Yangtze river estuarine island-chongming island, China. Int. J. Environ. Res. Public Health 18, 11642. doi: 10.3390/ijerph182111642
Precht W., Bruckner A., Aronson R., Bruckner R. (2002). Endangered acroporid corals of the Caribbean. Coral Reefs 21, 41–42. doi: 10.1007/s00338-001-0209-2
Preston D. L., Mischler J. A., Townsend A. R., Johnson P. T. J. (2016). Disease ecology meets ecosystem science. Ecosystems 19, 737–748. doi: 10.1007/s10021-016-9965-2
Rama H.-O., Roberts D., Tignor M., Poloczanska E. S., Mintenbeck K., Alegría A., et al. (2022). Climate change 2022: impacts, adaptation and vulnerability working group II contribution to the sixth assessment report of the intergovernmental panel on climate change. doi: 10.1017/9781009325844
Ranasinghe A., Salas F. (2012) Calibration and validation of the AZTI’s marine biotic index (AMBI) for southern California marine bays. Available at: https://www.academia.edu/26759491/Calibration_and_validation_of_the_AZTIs_Marine_Biotic_Index_AMBI_for_Southern_California_marine_bays (Accessed 8.31.22).
Rapport D. J., Gaudet C., Karr J. R., Baron J., Bohlen C., Jackson W. (1998). Evaluating landscape health: Integrating societal goals and biophysical process. J. Environ. Manage. doi. doi: 10.1006/jema.1998.0187
Robb C. K. (2014). Assessing the impact of human activities on British columbia’s estuaries. PloS One 9, e99578. doi: 10.1371/journal.pone.0099578
Röhrig B., du Prel J.-B., Wachtlin D., Blettner M. (2009). Types of study in medical research. Dtsch Arztebl Int. 106, 262–268. doi: 10.3238/arztebl.2009.0262
Rossiter W. (2013). Current opinions: zeros in host–parasite food webs: are they real? Int. J. Parasitol. Parasites Wildl 2, 228–234. doi: 10.1016/j.ijppaw.2013.08.001
Ruggiero P., Brown C. A., Komar P. D., Allan J. C., Reusser D. A., Lee Henry I. I. (2010). Impacts of climate change on oregon’s coasts and estuaries (Other government series), impacts of climate change on oregon’s coasts and estuaries (Oregon Climate Change Research Institute).
Ruiz G. M., Rawlings T. K., Dobbs F. C., Drake L. A., Mullady T., Huq A., et al. (2000). Global spread of microorganisms by ships. Nature 408, 49–50. doi: 10.1038/35040695
Scanes E., Scanes P. R., Ross P. M. (2020a). Climate change rapidly warms and acidifies Australian estuaries. Nat. Commun. 11, 1803. doi: 10.1038/s41467-020-15550-z
Scanes E., Scanes P. R., Ross P. M. (2020b). Climate change rapidly warms and acidifies Australian estuaries. Nat. Commun. 11, 1803. doi: 10.1038/s41467-020-15550-z
Schultz J. (2016) Evidence for a trophic cascade on rocky reefs following sea star mass mortality in British Columbia [PeerJ]. Available at: https://peerj.com/articles/1980/ (Accessed 9.1.22).
Seibel B. A., Childress J. J. (2013). The real limits to marine life: a further critique of the respiration index. Biogeosciences 10, 2815–2819. doi: 10.5194/bg-10-2815-2013
Smith R. W., Bergen M., Weisberg S. B., Cadien D., Dalkey A., Montagne D., et al. (2001). VelardeBenthic response index for assessing infaunal communities on the southern california mainland shelf. Ecol. Soc. Am. 11 (4), 1073–1084. doi: 10.1890/1051-0761(2001)011[1073:BRIFAI]2.0.CO;2Citations:100
Sobocinski K., Harvell C., Baloy N., Broadhurst G., Dethier M., Flower A., et al. (2022). Urban seas as hotspots of stress in the anthropocene ocean: the salish Sea example (Elementa: Science of the Anthropocene), 10. doi: 10.1525/elementa.2022.00055
Teixeira H., Weisberg S. B., Borja A., Ranasinghe J. A., Cadien D. B., Velarde R. G., et al. (2012). Calibration and validation of the AZTI’s marine biotic index (AMBI) for southern California marine bays. Ecol. Indicators Mar. Benthic Indic. 12, 84–95. doi: 10.1016/j.ecolind.2011.05.025
Thorhaug A. L., Poulos H. M., López-Portillo J., Barr J., Lara-Domínguez A. L., Ku T. C., et al. (2019). Gulf of Mexico estuarine blue carbon stock, extent and flux: mangroves, marshes, and seagrasses: a north American hotspot. Sci. Total Environ. 653, 1253–1261. doi: 10.1016/j.scitotenv.2018.10.011
Thorstad E. B., Bliss D., Breau C., Damon-Randall K., Sundt-Hansen L. E., Hatfield E. M. C., et al. (2021). Atlantic Salmon in a rapidly changing environment–facing the challenges of reduced marine survival and climate change. Aquat. Conservation: Mar. Freshw. Ecosyst. 31, 2654–2665. doi: 10.1002/aqc.3624
Thronson A., Quigg A. (2008). Fifty-five years of fish kills in coastal Texas. Estuaries Coasts 31, 802–813. doi: 10.1007/s12237-008-9056-5
Tracy A. (2019) Increases and decreases in marine disease reports in an era of global change | proceedings of the royal society b: biological sciences. Available at: https://royalsocietypublishing.org/doi/full/10.1098/rspb.2019.1718 (Accessed 10.6.22).
Trochta J. T., Groner M. L., Hershberger P. K., Branch T. A. (2022). A novel approach for directly incorporating disease into fish stock assessment: a case study with seroprevalence data. Can. J. Fish. Aquat. Sci. 79, 611–630. doi: 10.1139/cjfas-2021-0094
Tuohy E., Wade C., Weil E. (2020). Lack of recovery of the long-spined sea urchin diadema antillarum philippi in Puerto Rico 30 years after the Caribbean-wide mass mortality. PeerJ 8, e8428. doi: 10.7717/peerj.8428
Turner L., Tracey D., Tilden J., Dennison W. C. (2006). Where river meets Sea: exploring australia’s estuaries (Csiro Publishing).
Turpie J., Taljaard S., van Niekerk L., Adams J., Wooldridge T., Cyrus D., et al. (2013). The estuary health index: a standardised metric for use in estuary management and the determination of ecological water requirements. Available at: https://meridian.allenpress.com/jcr/article-abstract/29/5/1111/204332/Explaining-the-Spectral-Red-Edge-Features-of
Valença A. P. M. C., Santos P. J. P. (2012). Macrobenthic community for assessment of estuarine health in tropical areas (Northeast, brazil): review of macrofauna classification in ecological groups and application of AZTI marine biotic index. Mar. pollut. Bull. 64, 1809–1820. doi: 10.1016/j.marpolbul.2012.06.003
Ward J. R., Lafferty K. D. (2004). The elusive baseline of marine disease: are diseases in ocean ecosystems increasing? PloS Biol. 2, e120. doi: 10.1371/journal.pbio.0020120
Weber E. U. (2016). What shapes perceptions of climate change? new research since 2010. WIREs Climate Change 7, 125–134. doi: 10.1002/wcc.377
Wenqian C., Liu L., Meng W., Zheng B. (2012). The suitability of AMBI to benthic quality assessment on the intertidal zones of bohai Sea. Huanjing Kexue Xuebao/Acta Scientiae Circumstantiae 32, 992–1000. Available at: http://open.oriprobe.com/articles/29031173/The_suitability_of_AMBI_to_benthic_quality_assessm.htm
White E. Jr., Kaplan D. (2017). Restore or retreat? saltwater intrusion and water management in coastal wetlands. Ecosystem Health Sustainability 3, e01258. doi: 10.1002/ehs2.1258
Whitfield A. K., Elliott M. (2002). Fishes as indicators of environmental and ecological changes within estuaries: a review of progress and some suggestions for the future. J. Fish Biol. 61, 229–250. doi: 10.1111/j.1095-8649.2002.tb01773.x
Williams S. L., Grosholz E. D. (2008). The invasive species challenge in estuarine and coastal environments: marrying management and science. Estuaries Coasts: J. CERF 31, 3–20. doi: 10.1007/s12237-007-9031-6
Word J. Q. (1990). The infaunal trophic index, a functional approach to benthic community analyses. Dissertation or Thesis. University of Washington ProQuest Dissertations Publishing.
Yang X., Liu Z. (2005). Using satellite imagery and GIS for land-use and land-cover change mapping in an estuarine watershed. Int. J. Remote Sens. 26, 5275–5296. doi: 10.1080/01431160500219224
Keywords: aquatic disease, coastal health, marine diseases, estuary health, climate change, anthropogenic stressors
Citation: Tallam K and White E Jr (2023) The role of diseases in unifying the health of global estuaries. Front. Mar. Sci. 10:1185662. doi: 10.3389/fmars.2023.1185662
Received: 13 March 2023; Accepted: 30 May 2023;
Published: 22 June 2023.
Edited by:
Deepak R. Mishra, University of Georgia, United StatesReviewed by:
Michelle Jillian Devlin, Centre for Environment, Fisheries and Aquaculture Science (CEFAS), United KingdomDavid Bushek, Rutgers, The State University of New Jersey, United States
Copyright © 2023 Tallam and White. This is an open-access article distributed under the terms of the Creative Commons Attribution License (CC BY). The use, distribution or reproduction in other forums is permitted, provided the original author(s) and the copyright owner(s) are credited and that the original publication in this journal is cited, in accordance with accepted academic practice. No use, distribution or reproduction is permitted which does not comply with these terms.
*Correspondence: Krti Tallam, ktallam7@stanford.edu; krtital@gmail.com