Active hydrothermal vent ecosystems in the Indian Ocean are in need of protection
- 1Department of Ocean Systems (OCS), Royal Netherlands Institute for Sea Research (NIOZ), Texel, Netherlands
- 2Southern Marine Science and Engineering Guangdong Laboratory (Guangzhou), Guangzhou, China
- 3Department of Ocean Science, Hong Kong University of Science and Technology, Hong Kong, Hong Kong SAR, China
- 4Department of Science and Technology and International Cooperation, China Deep Ocean Affairs Administration, Beijing, China
Deep-sea hydrothermal vent fields are among the most pristine and remarkable ecosystems on Earth. They are fueled by microbial chemosynthesis, harbor unique life and can be sources of precipitated mineral deposits. As the global demand for mineral resources rises, vent fields have been investigated for polymetallic sulfides (PMS) and biological resources. The International Seabed Authority (ISA) has issued 7 contracts for PMS exploration, including 4 licenses for vent fields in the Indian Ocean. Here, we provide a summary of the available ecological knowledge of Indian vent communities and we assess their vulnerability, sensitivity, ecological and biological significance. We combine and apply scientific criteria for Vulnerable Marine Ecosystems (VMEs) by FAO, Particular Sensitive Sea Areas (PSSAs) by IMO, and Ecologically or Biologically Significant Areas (EBSAs) by CBD. Our scientific assessment shows that all active vent fields in the Indian Ocean appear to meet all scientific criteria for protection, and both the high degree of uniqueness and fragility of these ecosystems stand out.
1. Introduction
1.1. Ecology of hydrothermal vent ecosystems
Deep-sea hydrothermal vent ecosystems are exceptional habitats teeming with exotic life. Like oases in the desert, they are small but immensely productive hotspots on the otherwise barren seafloor. These dynamic ecosystems are located in geologically and tectonically active areas of the ocean, such as mid-ocean ridges, back-arc basins, and submarine volcanic arcs (Hannington et al., 2005; Beaulieu et al., 2013; Gollner et al., 2017). The release of geothermally heated fluid from the ocean crust supports chemosynthetic microbial communities that use reduced compounds from the vent fluids as energy to fix carbon and produce organic compounds that sustain high growth of fauna. A vent field usually covers multiple active or (temporarily) inactive vent sites with a shared subsurface circulation (Jamieson and Gartman, 2020). When hydrothermal fluids come into contact with the cool ocean water, polymetallic sulfide deposits (PMS) are formed as minerals from the fluid precipitate (von Damm, 1995; Hannington et al., 2005). Differences in geophysical settings, fluid chemistry, and fluxes in vent flow lead to high spatial and temporal variations of geochemical and physical nature in vent fields (Lutz and Kennish, 1993).
Hydrothermal activity creates a mosaic of microhabitats with distinct faunal zonations along the physicochemical gradient (Marsh et al., 2012; Watanabe and Beedessee, 2015; Copley et al., 2016; Galkin, 2016). Chemoautotrophic microbes form the basis of the food web, supporting dense aggregations of chemosynthetic macrofauna and primary and secondary consumers (Grassle, 1987). Vent species are physiologically well adapted to the unstable and extreme vent environment, such as to rapid and intense temperature shifts, a low pH, low oxygen concentrations, and high heavy metal exposure (Minic et al., 2006; Ramirez-Llodra et al., 2007). High growth rates, high biomass, and low faunal diversity are typical for vent assemblages (Grassle, 1985). The vast majority of taxa are vent-obligate (endemic), and communities drastically change when hydrothermal discharging ceases (van Dover, 2001; Gollner et al., 2020).
The ecosystem description of a “hydrothermal vent” can be as broad as the description “forest” (Thaler and Amon, 2019). Forests range from tropical to boreal, and vent systems are as diverse in their chemical, geological, and physical properties. Although every vent field is unique, distinct biogeographic provinces do exist, which are characterized by their dominant species and overall community structure (Mullineaux et al., 2018). The Indian Ocean has been considered to be one of these biogeographic provinces (Moalic et al., 2012).
The Indian Ocean has four mid-ocean ridges with intermediate to ultraslow spreading rates (Moalic et al., 2012; Rogers et al., 2012). Kairei on the Central Indian Ridge was the first vent field discovered in this province at the beginning of the 21st century (Hashimoto et al., 2001). Since then, another 12 active vent fields have been discovered in the region (Figure 1). The Indian Ocean has been hypothesized as an ecological corridor between Atlantic and Pacific vent populations, with a closer relation to the West Pacific metacommunity (van Dover, 2001; Watanabe and Beedessee, 2015). The mussel Bathymodiolus marisindicus and shrimp Rimicaris kairei are two dominant species that closely resemble their Atlantic counterparts (Hashimoto, 2001; Watabe and Hashimoto, 2002). Other common fauna are bythograeid crabs, neolepas barnacles, alviniconcha snails, and actinostlid anemones, all reminiscent of the West Pacific. However, several species are unique to the Indian Ocean communities such as most notably the scaly-foot snail Chrysomallon squamiferum (Warén et al., 2003; Chen et al., 2014).
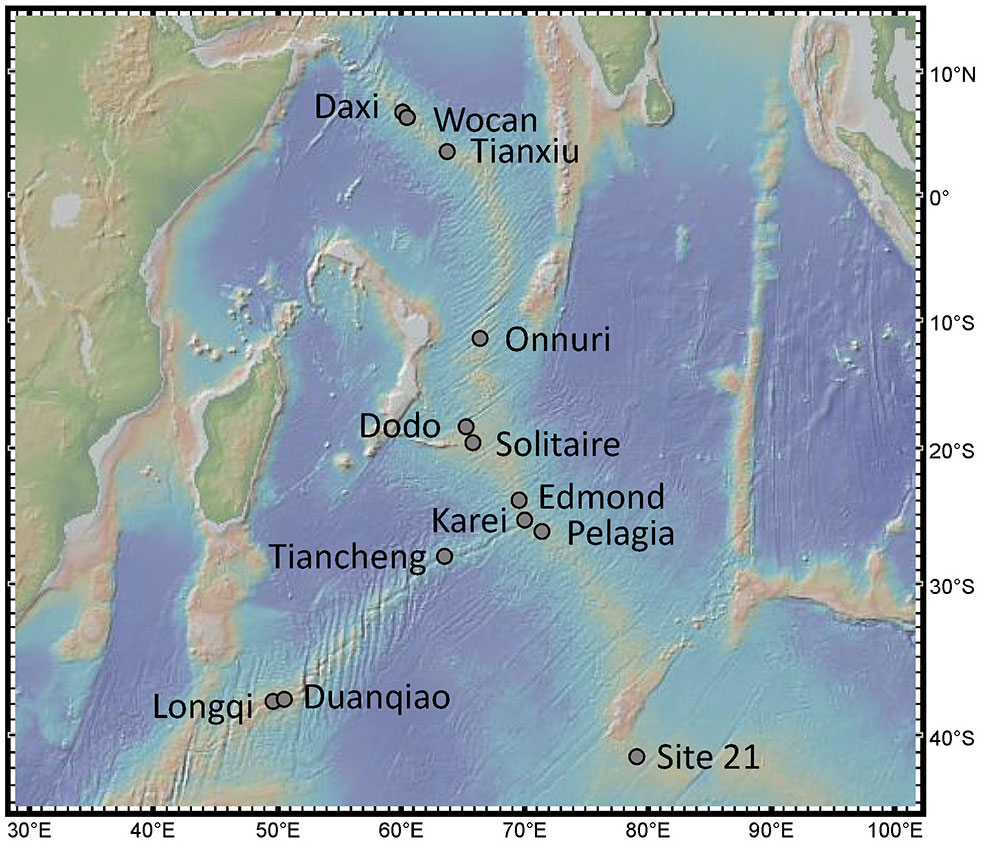
Figure 1 Location of the 13 active confirmed hydrothermal vent fields in the Indian ocean. Map created with GeoMapApp.
1.2. Exploration of polymetallic sulfide deposits and governance
Since the first discovery of a hydrothermal ecosystem in 1977, nearly 700 vents have been reported globally (Wang et al., 2021). The interest for deep-sea mining has accelerated accordingly due to an increased metal demand (Wedding et al., 2015). At vent fields, mineral resources are found in the form of polymetallic sulfide (PMS) deposits, which are also known as seafloor massive sulfides (SMS) (Levin et al., 2016b). The International Seabed Authority (ISA) is in charge of regulating all mining-related activities in the Areas Beyond National Jurisdiction (the Area) under the United Nations Convention on the Law of the Sea (UNCLOS)1. The ISA has the obligation to ensure that human activities in the international seabed are carried out for the benefit of humankind as a whole and to protect the marine environment from harmful impacts caused by resource extraction activities2. At present, the ISA has issued three licenses for PMS exploration on the northern Mid-Atlantic Ridge and four licenses on the Indian mid-ocean ridges3. The four contractors are the German Federal Institute for Geosciences and Natural Resources (BGR), the China Ocean Mineral Resources Research and Development Association (COMRA), the Ministry of Earth Sciences from the Government of India, and the Government of the Republic of Korea. Each contract area covers 10,000 km2 of which 75% will be relinquished. The contract areas of COMRA are on the South-West Indian Ridge, of the Government of India on the South-West Indian and the Central Indian Ridge, of BGR on the Central and South-East Indian ridge, and of The Government of the Republic of Korea on the Central Indian Ridge. Currently, 8 from the 13 confirmed active vent fields in the Indian Ocean (Longqi, Duanqiao, Tiancheng, Onnuri, Dodo, Kairei, Edmond, and Pelagia) are within those contract areas (Perez et al., 2021).
Mining activities may disturb vent ecosystems in an unprecedented manner on spatial and temporal scales (Gollner et al., 2017). Vent ecosystems on slow and ultraslow spreading ridges, such as those in the Indian Ocean, tend to be more stable and their biological communities are less exposed to natural disturbance events such as major volcanic eruptions than ones on fast spreading ridges (Gollner et al., 2017; van Dover et al., 2018). No disturbance experiments have been performed to mimic mining at vent ecosystems in the Area, but it is expected that mining would have severe consequences. Direct mining impacts may include removal of substrate and fauna, causing habitat loss, habitat fragmentation, and habitat modification, leading to loss of biodiversity at all levels: genetic, species, functional, and habitat (Levin et al., 2016b). Other direct mining impacts include light, sound, and electromagnetic exposure from mining equipment (Chen et al., 2021b) as well as changes in vent fluid flow (Kawagucci et al., 2013). Mining plumes can also create indirect mining impacts such as increased heavy metal load or high turbidities following sediment resuspension (Weaver et al., 2022). While it is expected that harm will be done to vent communities dependent on PMS as substrate, the ramifications extend beyond the local sphere (Levin et al., 2016a). Low recolonization of fauna in the vicinity of vents has been previously observed after a disturbance, likely due to lower resilience of those species (Gollner et al., 2015). In a wider region surrounding the vent, resuspension of potentially toxic sediment and returning plumes from the surface operational ship could affect both benthic and pelagic life.
The International Seabed Authority has been developing Regional Environmental Management Plans (REMP) for PMS at the northern Mid Atlantic Ridge (nMAR) and is planning to develop REMP for the Indian Ocean. A draft for the nMAR REMP has been released for stakeholder consultation on the 19th April 2022 (Lee, 2020), whilst a first workshop focusing on scientific issues related to REMP development in the Indian Ocean Region (IOR) has been co-organized by ISA, COMRA and Hong Kong University of Science and Technology.
In this study, we evaluate whether the confirmed active vent fields at the Indian mid-ocean ridges meet scientific criteria for protection from anthropogenic impacts, through a comprehensive review of the scientific literature, and a comparison of available baseline information of each vent field against criteria developed by the Food and Agricultural Organization (FAO) for Vulnerable Marine Ecosystems (VMEs), the Convention on Biological Diversity (CBD) for Ecologically or Biologically Significant Areas (EBSAs), and the International Maritime Organization (IMO) for Particularly Sensitive Sea Areas (PSSAs), following the same procedure as described in Gollner et al. (2021) for active vents fields under mineral exploration at the nMAR. All active vent fields of the IOR appear to be vulnerable and significant ecosystems.
2. Methods
In this study, we assessed 13 vent fields with confirmed activity from the Indian mid-ocean ridges from the Carlsberg Ridge (CR) to the Central Indian Ridge (CIR), the South-East Indian Ridge (SEIR), and the South-West Indian Ridge (SWIR): Daxi, Wocan, Tianxiu, Onnuri, Dodo, Solitaire, Edmond, Kairei, Pelagia, Tiancheng, Duanqiao, Longqi, and Site 21 (Figure 1).
The search engines Google Scholar and Scopus were used to collect scientific literature in November and December 2021, and June 2022, using English key words for our searches, which limited our search solely to publications in English. The following key words were used: “hydrothermal activity”, “hydrothermal field”, “vent fauna”, “vent-endemic biota”, “Indian Ocean”, “Indian mid-ocean ridge”, “deep-sea mining”, “polymetallic sulfide”, “mineral extraction”, “resilience”, the names of all Indian mid-ocean ridges, and individual vent fields. Additional literature used in this review was found through the references of initially selected articles. Furthermore, the search engine Ecosia was used to acquire general information from organizations such as the ISA, FAO, CBD, IMO, and IUCN, and to gain access to their online documents.
We first review the main ecological findings and shortly discuss i) the discovery and exploration of the vent field, ii) the geochemical setting, and then focus on iii) the biological composition. Regarding vent biodiversity, we focused on macro- and megafauna.
Scientific criteria for identifying hydrothermal ecosystems in the Indian Ocean in need of protection follow the determination as used in “Application of scientific criteria for identifying hydrothermal ecosystems in need of protection” for nMAR vents to evaluate ecosystem vulnerability and significance by Gollner et al. (2021) (Appendix 1). Criteria include 1) uniqueness and rarity, 2) functional significance, 3) fragility, 4) life-history traits of component species that make recovery difficult, 5) structural complexity, 6) biological diversity, 7) biological productivity, 8) naturalness, and 9) ecosystem services. The criteria have been derived from the guidelines for identifying VMEs from the FAO (FAO, 2009), EBSAs from the CBD (CBD, 2009), and PSSAs from the IMO (IMO, 2005). One PSSA criterion (social, cultural and economic criteria: Social or economic dependency, human dependency, cultural heritage) was not included in the scoring, but rather an Ecosystem Services criterion was proposed as a more relevant addition (Gollner et al., 2021). There is a precedent for applying ecosystem services in a deep-sea context, which entails supporting, provisioning, regulating, and cultural services (Earth Economics, 2015; Levin et al., 2016a; Le et al., 2017; van Dover et al., 2018; Turner et al., 2019; Orcutt et al., 2020).
Based on the literature review, both vent-specific and general information on 12 vents is used to determine if each vent field meets the scientific criteria for protection. For vent “Site 21”, there are only 2 references available – one on detection of plume anomaly and another on the collection of vent-endemic Neolepas barnacles with a dredge (Watanabe et al., 2018). Although these findings indicate that Site 21 shall be a confirmed active vent, we decided to exclude it in our assessment because of the lack of visual observation which complicates the scientific analyses of many criteria.
3. Results
3.1. Review of main ecological findings of IOR vent fields
An overview of different geochemical and biological characteristics for each vent field is listed in Table 1. The 13 active vents in the Indian Ocean occur across a depth range of ~2000 meters (from 1732 to 3690) along ~48° from the Northern to the Southern hemisphere, spanning four mid-ocean ridges.
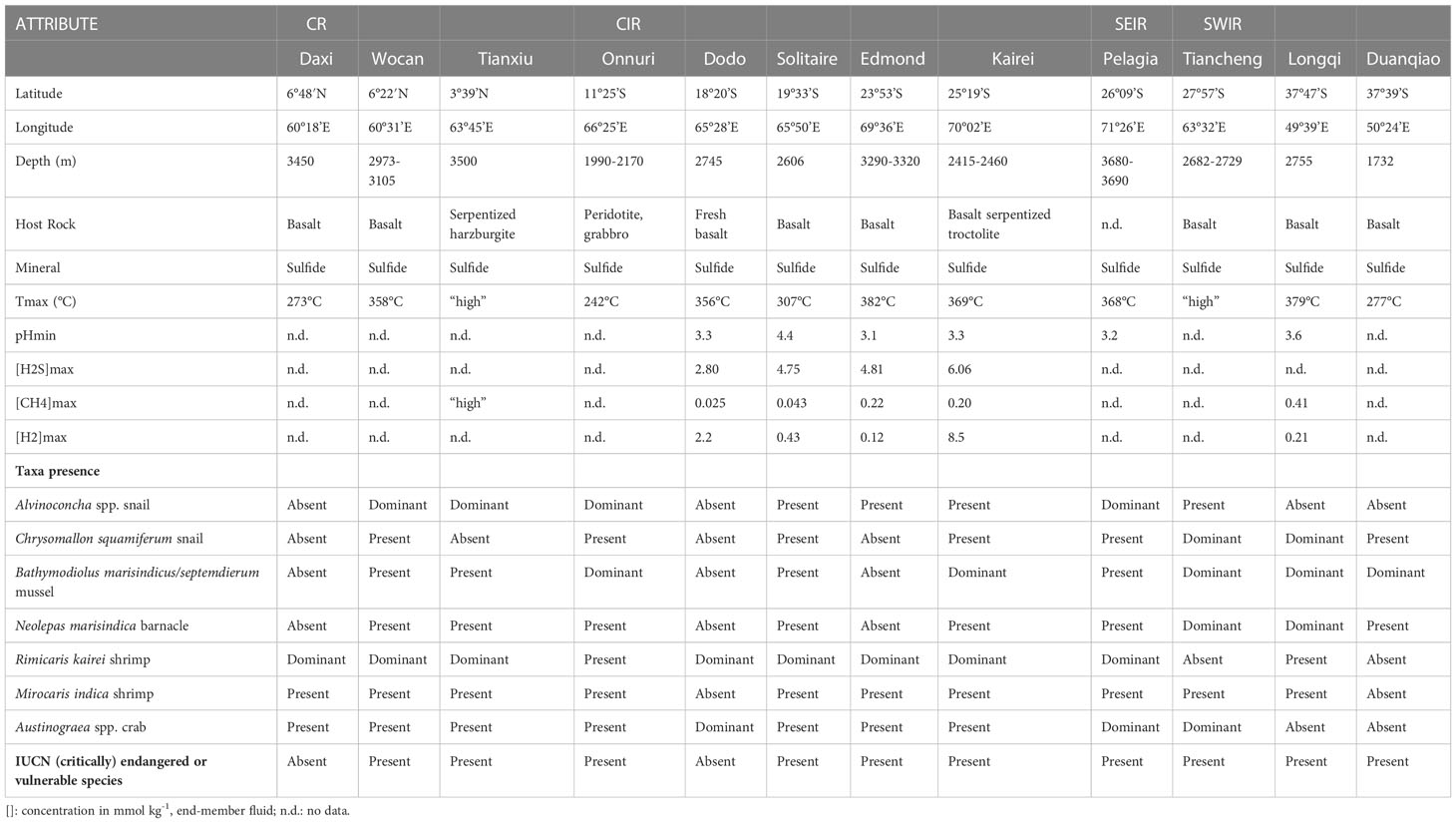
Table 1 Selected attributes of 12 active vent fields in the Indian Ocean, based on the literature used in this review.
3.1.1. Vent fields of the Carlsberg Ridge
The CR, which is also referred to as the North-West Indian Ridge or the northern section of the CIR, is a divergent tectonic plate boundary between the Somalic plate and the Indian plate. It borders the CIR in the south and the Owen Transform Fault in the north and has a slow spreading rate of 22 - 32 mm per year (Ray et al., 2012). Daxi, Wocan, and Tianxiu active vent fields were found on the CR over the last 10 years. In total, 34 species have been recorded in these three vent fields (Zhou et al., 2022). The benthic communities in Tianxiu and Daxi fields appear to be subsets of those in Wocan, with a high genetic connectivity among the three vents (Zhou et al., 2022). It was suggested that biological communities in the CR vents form a separate province from those in the CIR and SWIR, based on differences in faunal composition and clear genetic clustering of species among these ridges (Zhou et al., 2022). Although the CR shares some community characteristics with the CIR, the prominent presence of polychaetes sets the CR metacommunity apart (Zhou et al., 2022). Interestingly, 2 polychaete species found on the CR have been grouped into clades with their East Pacific congeners, elucidating a direct biogeographic linkage between the CR and distant East Pacific metapopulation (Zhou et al., 2019).
3.1.1.1. Daxi (6°48′N, 60°10’E, 3450m)
The basalt-hosted Daxi vent field was discovered during the Dayang (DY33) cruise of COMRA in 2015 (Wang et al., 2021). Three mounts are located in this vent field and at least one of them accommodates clusters of active black smokers at the top (Wang et al., 2021). Eight chimney complexes ranging from several to more than 10 meters were seen, some discharged their fluids vigorously and others slowly (Wang et al., 2021).
The vent community at Daxi consisted of 15 recognized mega-faunal species which are a subset of the community at Wocan (Zhou et al., 2022), and was dominated by alvinocaridid shrimps, polychaetes, and crabs (Wang et al., 2021). The shrimp Rimicaris kairei was very dominant nearby hydrothermal activity while actinostlid anemones were common at the periphery. The alvinellid worm Paralvinella mira was endemic in the Daxi and Wocan vent fields, with lower abundances at Daxi (Han et al., 2021). The alvinellid strongly resembles 2 species, P. hessleri and an unnamed species Paralvinella sp. ZMBN, from the West Pacific (Han et al., 2021). Austinograea rodriguezensis crabs, Munidopsis lobsters, and shrimps resembling Mirocaris indica were also present at Daxi, while other typical Indian vent species, such as Chrysomallon squamiferum scaly-foot snails, Alviniconcha marisindica hairy snails, Bathymodiolus marisindicus mussels, and Neolepas marisindica stalked barnacles, were absent (Wang et al., 2021). A recently described novel neolepetopsid limpet, Neolepetopsis prismatica and a potentially new Desbruyeresia species were found so far exclusively in this vent field (Chen et al., 2021c; Zhou et al., 2022).
3.1.1.2. Wocan (6°22′N, 60°31’E, 2973-3105m)
Wocan was the first vent field discovered on the CR during the COMRA’s DY28 cruise with the Research Vessel (RV) Zhukezhen in 2013 (Wang et al., 2017). TV-sled and TV-grab observations revealed the existence of two vent sites: Wocan-1 (~450 x 400 m) and ~1.7km to the northwest Wocan-2 (~200 x 400 m) (Qiu et al., 2021). During the COMRA’s DY38 cruise in 2017, a cluster of 17 active black smokers in Wocan-1 and a low-temperature diffuse flow area at Wocan-2 were confirmed (Qiu et al., 2021). Inactive (collapsed) chimneys, hydrothermal sediments, and sulfide talus were also found at Wocan-1 (Qiu et al., 2021).
Around the active vents, many vent-endemic species were discovered, including alvinocaridid shrimps, bythograeid crabs, actinostlid anemones, bathymodiolin mussels, and alviniconcha snails (Qiu et al., 2021). A potentially new shrimp species from the genus Rimicaris was recovered from Wocan (Zhou et al., 2022). The annelid Amphisamytha wocanensis was also found solely at Wocan, which surprisingly fell into a clade with species from the East Pacific (Zhou et al., 2019). A third polychaete observed at Wocan was the alvinellid worm Paralvinella mira, which was associated with Alviniconcha and C. squamiferum snails at at this vent field (Han et al., 2021). Strikingly, P. mira and Hesiolyra heteropoda were highly abundant and form an alvinellid-/hesionid-polychaete worm dominated assemblage unique to the Indian Ocean (Han et al., 2021).
3.1.1.3. Tianxiu (3°7’N, 63°8’E, 3500m)
The ultramafic-hosted Tianxiu was also discovered during the COMRA’s DY33 cruise in 2015 with a TV-sled and TV-grab (Chen et al., 2020). Black smoker chimney complexes, low-temperature diffuse vents, inactive massive sulfides, and hydrothermal sediments all occurred at Tianxiu (Zhou et al., 2022). A total of 12 megafaunal species were reported from the Tianxiu field, including Alviniconcha marisindica snails, Neolepas marisindica barnacles, Bathymodiolus marisindicus mussels, and a new crab species Austinograea sp. CR, and all of them were also found in the Wocan field (Zhou et al., 2022).
3.1.2. Vent fields of the Central Indian Ridge
Vent fields of the CIR are the most comprehensively studied among all the vents reported on the Indian mid-ocean ridges. The CIR borders the CR in the north and meets the SEIR and the SWIR at its most southern point in the Rodriguez Triple Junction (RTJ). The CIR has an average full spreading rate of 48 mm per year, which is moderate to slow spreading (Kumagai et al., 2008; Perez et al., 2021). To date, five active vent fields have been reported, including Onnuri, Dodo, Solitaire, Edmond, and Kairei (Hashimoto et al., 2001; van Dover et al., 2001; Tamaki, 2010; Nakamura et al., 2012; Ryu et al., 2019).
CIR vent communities show an immense diversity, including different morphotypes of Chrysomallon squamiferum. This scaly-foot snail hosts endosymbiotic sulphur-oxidizing bacteria (SOB) in its esophageal gland, which is rather unique among molluscs (Goffredi et al., 2004). Other characteristic CIR species are Rimicaris kairei, Mirocaris indica, and Bathymodiolus marisindicus, which are endemic to the Indian Ocean (Hashimoto et al., 2001; Watabe and Hashimoto, 2002; Komai et al., 2006). Furthermore, the vent-endemic polychaetes were discovered at Solitaire on the CIR (Nakamura et al., 2012). The copepod family Dirivultidae is the most species rich invertebrate family found in vent fields, yet members from this family have been hardly recorded at four Indian vent fields, three of which are located on the CIR (Gollner et al., 2010; Gollner et al., 2016; Lee et al., 2020; Sun et al., 2020). Onnuri, Solitaire, and Kairei clearly emerge as extraordinary ecosystems, considering the exceptional diversity reported so far in these vent fields. Edmonds community seems to be a subset of Kairei’s and may comprise source populations of both R. kairei and Alviniconcha sp. hairy snails on the CIR (Beedessee et al., 2013). Dodo is a relatively young vent field, which may explain its relatively low colonization by vent-endemic species (Nakamura et al., 2012). It was recently suggested that the CIR together with the northern SWIR (Tiancheng) forms a separate province from the CR and southern SWIR, with multiple vent sites that are of equal importance to the species pool (Zhou et al., 2022).
3.1.2.1. Onnuri (11°24’S, 66°25’E, 1990-2170m)
The Onnuri vent field was first explored with a TV-grab during a cruise on the RV ISABU from the Korean Institute of Ocean Science and Technology (KIOST) in 2018 (Kim and Lee, 2020). The field is ultramafic-hosted and was thought to have diffusive, clear, and low-temperature fluids from cracks in the seafloor (Kim and Lee, 2020; Kim et al., 2020). However, high temperatures were recorded during a re-visit to Onnuri (Suh et al., 2022).
During the first expedition scientists identified 21 macrofauna taxa and 65 nematodes, which were mainly distributed around cracks in the seafloor from which the hydrothermal fluids were emitted (Kim et al., 2020). Onnuri was visually dominated by three species of bathymodiolus mussels, Bathymodiolus marisindicus, Bathymodiolus sp.1, and Gigantidas vrijenhoeki as well as Neolepas marisindica barnacles (Jang et al., 2020; Kim et al., 2020). G. vrijenhoeki was associated with the scale worm Branchipolynoe onnuriensis, and both species were found exclusively at Onnuri (Jang et al., 2020). As the only member of the genus Gigantidas in the Indian Ocean, the Gigantidas mussel in Onnuri field is genetically closely related to two Gigantidas species from cold seeps in the Western Pacific (Jang et al., 2020; Ryu et al., 2021). This mussel species carries unique sulfur- and methane-oxidizing symbionts that differ in composition and are phylogenetically distinct from the symbionts of B. marisindicus at Onnuri (Jang et al., 2020). Besides B. onnuriensis and G. vrijenhoeki and their symbionts, many other organisms are only known from Onnuri. For example, Onnuri was the second Indian vent field from which copepods were retrieved so far, and the first with distinct species described alongside Solitaire (van Dover et al., 2001; Lee et al., 2020). This included a copepod species from the distant East Pacific, Aphotopontius limatulus, but also the novel species Stygopontius spinifer and Aphotopontius kiost which were found at Solitaire and Onnuri (Kim and Lee, 2020; Lee et al., 2020). Moreover, Munidopsis lauensis, Smacigastes pumila, Alvinocaris markensis, Paralepetopsis ferrugivora, Lepetodirilus sp. C, Nereis sp., and Branchipolynoe seepensis were absent at other Indian vent fields (Kim et al., 2020; Hwang et al., 2022). Three other taxa were known only from one other Indian vent field, Archinome sp. from Edmond (CIR), Branchipolynoe sp. from Kairei (CIR), and Hesiolyra cf. bergi from Longqi field (SWIR) (Kim et al., 2020). Besides the exceptional diversity of rare species, many taxa typical for Indian vents, including Alvinocaris shrimps, Mirocaris shrimps, Austinograea crabs, Munidopsis crabs, Chrysomallon snails, and Alviniconcha snails were present (Jang et al., 2020). Based on 16 retrieved taxa, seven trophic guilds, and four trophic levels have been recognized in the food web structure of Onnuri (Suh et al., 2022).
3.1.2.2. Dodo (18°20’S, 65°17’E, 2745m)
Dodo was visited during the cruise YK 09-13 on the RV Yokosuka of the Japan Agency for Marine-Earth Science and Technology (JAMSTEC) in 2009, following the detection of plume anomalies in 2006 (Kawagucci et al., 2008; Nakamura et al., 2012). The vent field is located amid the Dodo Great Lava Plain, which is a flat stretch of seafloor covered with sheet flow lava (Nakamura et al., 2012; Nakamura and Takai, 2015). Black smoker chimneys in a diameter of 15 meters were observed to emit hydrothermal fluids in 2009, but discharging was not observed in 2013 (Nakamura et al., 2012; Kawagucci et al., 2016). The system was hosted by fresh basalt and there were three main chimneys (Kawagucci et al., 2016).
Few vent megafauna species were found at Dodo, making this community emaciated in comparison to the communities of other CIR vents (Nakamura et al., 2012). However, sparsely distributed A. rodriguezensis crabs, patches of R. kairei shrimps on chimneys, some Marianactis sea anemones, lepetodrilid limpets and provannid snails were observed (Nakamura et al., 2012). The hydrothermal activity is suspected to be very young, which may explain the low biomass and faunal diversity (Nakamura et al., 2012). Microbial populations, however, were very abundant at Dodo (Kawagucci et al., 2016). The microbial density was several orders of magnitude higher than that at the neighboring Solitaire field and also significantly higher than those previously observed (Kawagucci et al., 2016).
3.1.2.3. Solitaire (19°33’S, 65°51’E, 2606m)
Like Dodo, the basalt-hosted Solitaire was explored during the JAMSTEC cruise YK 09-13 in 2009 after plume anomalies were detected in 2006 (Kawagucci et al., 2008; Nakamura et al., 2012). Hydrothermal fluids were emitted from a larger area (~50 x 50 m) than that of Dodo but the size was comparable to Kairei and Edmond (Nakamura et al., 2012). Three main chimneys of more than 5 m in height with mounds at their bases were present on top of talus, and extensive diffuse flows occurred from the permeable and thick talus throughout the field (Nakamura et al., 2012).
The lush vent community at Solitaire is rich in genetic, morphologic, and species diversity (Beedessee et al., 2013; Watanabe et al., 2018). For example, Solitaire was the first vent field outside of the Pacific where alvinellid polychaetes were found. Apart from Wocan, it remains the only field from which alvinellid worms have been described (Nakamura et al., 2012; Han et al., 2021). The alvinellid worms co-occurred with the C. squamiferum scaly-foot snail (Han et al., 2021). The white “Solitaire type” scaly-foot snail differed from the Kairei and Longqi morphotypes since they lack iron sulfide on their sclerites and shell, because Solitaire’s vent fluids were iron-depleted (Nakamura et al., 2012). The scaly-foot snails and alviniconcha hairy snails at Kairei live near low-temperature fluids, whereas they were present at chimneys emitting high-temperature diffuse fluids at Solitaire (Nakamura et al., 2012). Other typical endemic vent species of Solitaire community include R. kairei, M. indica, Bathymodiolus mussels with lepetodrillid limpets attached, phymorhynchus gastropods, undescribed scale worms, and Neolepas marisindica and Eochionellasmus sp. barnacles (Nakamura et al., 2012; Watanabe et al., 2018). Noteworthy were the two cohorts of different sized A. rodriguezensis crabs at Solitaire, as they could be the source population of these bythograeid species for the CIR (Beedessee et al., 2013). The presence of copepods was another special feature of this Indian vent community, as copepod species were previously described for only one other Indian vent field (Lee et al., 2020). The East Pacific Aphotopontius limatulus was shared by Solitaire and Onnuri, as well as the novel A. muricatus and Stygiopontius spinifer (Lee et al., 2020). However, the novel Benthoxynus constrictus, S. horridus and S. geminus were exclusively found at Solitaire (Lee et al., 2020). The first sessile barnacle of the family Chionelasmatidae outside of the Pacific was also found at Solitaire, and this novel species Eochionelasmus coreana has only been recorded here (Chan et al., 2020). Furthermore, the rare deep-sea snail Desbruyeresia marisindica was unique to Solitaire and Kairei (Watanabe and Beedessee, 2015). Other deep-sea taxa occasionally observed outside of the venting area included Macrourid fish, Marianactis anemones, Munidopsis squat lobsters and sea cucumbers (Nakamura et al., 2012).
3.1.2.4. Edmond (23°53’S, 69°36’E, 3290-3320m)
The basalt-hosted Edmond was the second active Indian vent discovered during the Voyage 162-13 with the RV Knorr and the Remotely Operated Vehicle (ROV) Jason in 2001 (van Dover et al., 2001). The vent field of 100 x 90 m is located in an area with cross-cutting ridge-crest structures reminiscent of the Trans-Atlantic Geotraverse (TAG) field on the nMAR (van Dover et al., 2001). Old, disaggregated sulfides, massive sulfide talus, and orange-brown iron-oxyhydroxide sediments are characteristic of Edmond, while chimney formations and venting styles varied greatly (van Dover et al., 2001).
Edmond supported a dense vent community with thousands of R. kairei shrimps swarming the black smokers and marianactis anemones dominating the vent periphery (van Dover et al., 2001). R. kairei populations between Edmond and Kairei sites showed no genetic differentiation and the former was suggested to provide the larval supply for other CIR vents since that population had the broadest size distribution (Beedessee et al., 2013). Edmond is also regarded as the source population of Alviniconcha sp. 3 snails (Beedessee et al., 2013). A. rodriguezensis crabs, Mirocaris indica shrimps, branchinotogluma scale worms, and phymorhynchus snails were also prevalent at Edmond (van Dover et al., 2001; Komai et al., 2006). The neolepetopsid limpet Eulepetopsis crystallina collected from the Edmond vent field was recently described (Chen et al., 2021c). Additionally, chemolithoautotrophic and heterotrophic bacteria not known to other vents have been isolated from Kairei and Edmond sulfides (van Dover et al., 2001).
3.1.2.5. Kairei (25°19’S, 70°02’E, 2415-2460m)
Kairei was the first active vent field discovered in the Indian Ocean during a JAMSTEC cruise with the RV Hakuho Maru and ROV Kaiko in 2000 and is one of the most visited vent fields (Hashimoto et al., 2001; van Dover et al., 2001; Komai et al., 2006). The hydrothermal activity covered an area of 40 x 80 m containing at least seven active vent sites with massive PMS deposits, including individual black smokers of more than10 m in height (Hashimoto et al., 2001; Han et al., 2018).
Kairei is characterized by a high biomass and high diversity with thousands of R. kairei shrimps living in the hydrothermally active zone and marianactis anemones occurring in the vent periphery, which is similar to those in Edmond. R. kairei shrimps partially feed on sulfide-oxidizing filamentous episymbionts in their branchial chambers, which were found to be the dominant primary producers in the Kairei community (van Dover et al., 2001; van Dover, 2002). Underneath the blanket of shrimps, bathymodiolus mussels, alviniconcha hairy snails and scaly-foot snails (C. squamiferum) formed clusters of hundreds of individuals (van Dover et al., 2001; van Dover, 2002). The scaly-foot snail was discovered in Kairei vent field and occurred only at Monju site (Nakamura et al., 2012). During the second excursion to Kairei, 36 invertebrate taxa were identified, with one-third of those species being found in the Pacific but many taxa belonged to new genera (van Dover et al., 2001). The recorded taxa include neolepas barnacles, actinostolid actinians, lepetodrilus limpets, bathymodiolus mussels, mirocaris shrimps, munidopsis lobsters, and many more (Hashimoto et al., 2001; van Dover et al., 2001; Watanabe and Beedessee, 2015). Further undescribed copepods, the new neolepetopsid limpet Eulepetopsis crystalline, and the new amphinomid polychaete Amphisamytha marisindica were collected at Kairei, all of which were found in a very limited number of Indian vent fields (van Dover et al., 2001; Zhou et al., 2019; Chen et al., 2021c). Furthermore, the lobster Munidopsis laticorpus and the snail Iphinopsis boucheti are unique to Kairei; and the snails Bruceiella wareni and Desbruyeresia marisindica are known only from Kairei, Wocan, and Solitaire (Cubelio et al., 2007; Watanabe and Beedessee, 2015; Zhou et al., 2022).
3.1.3. Vent fields of the South-East Indian Ridge
With a uniform spreading rate of 69 - 75 mm per year, the SEIR separates the Indo-Australian and Antarctic plates (Sempéré and Cochran, 1997) and meets the CIR at its most northwestern point in the RTJ and the Macquarie Triple Junction in the far east of the Pacific Ocean (Sempéré and Cochran, 1997). The SEIR is perhaps the less studied region of the Indian Ocean, with only two active vent fields reported so far.
3.1.3.1. Pelagia (26°1’S, 71°3’E, 3659m)
Pelagia was discovered during the INDEX 2014 cruise with the RV Pelagia by the German Federal Institute for Geosciences and Natural Resources (BGR) (BGR, 2015), and thereafter visited during the BGR INDEX 2016 cruise with the RV Pourquoi pas? and ROV VICTOR 6000 (Han et al., 2018).
Consistent with other vents, strong faunal zonation was recorded at Pelagia. One study examining merely a single chimney complex recorded 17 megafauna taxa, which occurred in 4 zones of proximity to the hydrothermal activity (Gerdes et al., 2019). Common Indian species found at Pelagia were the snail C. squamiferum, the shrimps M. indica and Alvinocaris solitaire, and the stalked barnacle N. marisindica (Gerdes et al., 2019). The hairy snails Alviniconcha marisindica and the crab A. rodriguezenis were highly abundant nearest to black smoker fluids, followed by the shrimp R. kairei and thereafter the mussel B. marisindicus (Gerdes et al., 2019). Note that the bathymodiolin species B. septemdierum and B. marisindicus cannot be distinguished based on the mCOI marker gene (Breusing et al., 2015). The community at Pelagia resembled those of other CIR vent fields, with newly described holothurian species (Chiridota hydrothermoca sp. inc) (Smirnov et al., 2000; Gerdes et al., 2021) and fish species (Pachycara angeloi) (Thiel et al., 2021), which were reported from other CIR vents as Chiridotidae and Zoarcidae (Gerdes et al., 2019). Additionally, rare microbial strains were found at Pelagia and Kairei that distinctly differed from other mid-ocean ridge samples (Han et al., 2018). They were sampled at both active venting zones and inactive areas, with much larger proportions of novel operational taxonomic units than at chimneys in most other vent ecosystems (Han et al., 2018). Such rare species are likely dormant until better conditions for growth arrive and may be responsible for large element turnovers that affect large-scale biogeochemical cycling (Han et al., 2018).
3.1.3.2. Site 21 (41°15’S, 79°06’E)
Plume anomalies were detected at Site 21 near the Amsterdam-St. Paul Plateau, and a vent-associated barnacle belonging to the genus Neolepas was retrieved by dredge (Scheirer et al., 1998). However, no further publications have confirmed the activity of Site 21.
3.1.4. Vent fields of the South-West Indian Ridge
The SWIR is the second most slow spreading ridge in the world with an ultraslow spreading rate of ~15 mm per year (Patriat et al., 1997). Meeting the other Indian Ridges in the RTJ, the SWIR is a tectonic plate boundary between the Antarctic and African plate (Patriat et al., 1997). Three active vent fields from the SWIR have so far been reported, namely Tiancheng, Longqi, and Duanqiao.
The SWIR comprises of two separate clusters, the south-western Longqi and Duanqiao and the north-eastern Tiancheng (Sun et al., 2020). This appears to be the case for both community composition and genetic divergence since the C. squamiferum population of Longqi shows low connectivity to the CIR populations (Sun et al., 2020; Zhou et al., 2022). Tiancheng however showed a striking similarity to CIR vent communities, and transform faults on the SWIR possibly form a powerful barrier to gene flow among SWIR vents (Sun et al., 2020). Geological or hydrodynamic barriers thus could be a more important determinant of connectivity between these vent communities than ridge characteristics such as spreading rate. The Longqi and Duanqiao populations share community components with the CIR, the MAR, and the East Scotia Ridge, and have been suggested to be a separate province (Zhou et al., 2018; Reid et al., 2020; Zhou et al., 2022). The northern SWIR vent Tiancheng would be part of the CIR-nSWIR province (Zhou et al., 2022). Only four species so far occur at all the three fields: the mussels B. marisindicus, the scale worms B. longqiensis, the barnacles N. marisindica, and the scaly-foot snail C. squamiferum (Zhou et al., 2018; Sun et al., 2020). An interesting feature of all SWIR vents is the absence of alvinocarid shrimp dominance, which is typical for most CIR vents. Instead, mussels were highly abundant throughout the SWIR, even though there is a large bathymetric range between these vents (1732 - 2760m) (Zhou et al., 2018).
3.1.4.1. Tiancheng (27°57’S, 63°32’E, 2682-2729m)
The basalt-hosted Tiancheng vent field lies in the most northeastern section of the SWIR on the slope of the Tiancheng seamount (Chen et al., 2018; Fang and Wang, 2021). Three vent sites have been found, of which two diffuse flow areas and one high-temperature black smoker area (Sun et al., 2020).
An initial report identified 11 taxa at Tiancheng early on when only the diffuse TC-1 vent site was known (Zhou et al., 2018), with dense aggregations of the mussel Bathymodiolus marisindicus in the center, actinostolid anemones in the periphery, and the presence of bythograeid crabs Phymorhynchus snails, Mirocaris shrimps, Phymorhynchus sp.“Tiancheng” snails, Neolepas marisindica barnacles, Polynoidae polychaetes, and Ophiidae fish (Zhou et al., 2018). However, a following visit to TC-2 and Tianchang recorded 12 additional taxa, including a distinct new morphotype of the snail C. squamiferum and the snail Desbruyeresia cf. marisindica (Sun et al., 2020). The “Tiancheng type” scaly-foot snail was dominant here and had reddish-brown zinc sulfide deposits on its dirty white scales and a brown shell (Sun et al., 2020). Some taxa that are uncommon in the Indian Ocean were recorded at Tiancheng, including undescribed copepods from the family Dirivultidae, the sea cucumber Chiridota, and the polychaete Ophryotrocha (Sun et al., 2020). Interestingly, Tiancheng’s vent community was more similar to the CIR vent fields and had low connectivity to other SWIR vent fields (Zhou et al., 2018; Sun et al., 2020). Species that were reported for CIR vents but not at the other SIWR vents include the limpet Eulepetopsis crystallina, crab A. rodriguezenis, and mussel B. marisindicus (Sun et al., 2020; Chen et al., 2021c).
3.1.4.2. Longqi (37°47’S, 49°39’E, 2755m)
Longqi was the first active vent field discovered on an ultraslow mid-ocean ridge worldwide, and is by far the most explored vent field on the SWIR (Tao et al., 2012; Zhou et al., 2018). The basalt-hosted field has three vent sites that extend laterally over ~1000 m (Tao et al., 2012; Zhou et al., 2018). The first vent site was identified during the COMRA DY19 cruise in 2007 and two other vent sites were explored during the COMRA DY20 cruise in 2008/2009 (Tao et al., 2012). Temperature of discharging fluids was over 300°C but diffuse venting of clear fluids was also observed (Copley et al., 2016).
Longqi harbors a stunning vent community of about ~35 identified taxa to date with a unique biodiversity and a complex zonation pattern (Zhou et al., 2018; Zhou et al., 2019; Chen et al., 2021a; Chen et al., 2021c), including the scaly-foot snail C. squamiferum, alvinocaridid shrimps R. kairei and M. indica, stalked barnacle N. marisindica, and mussel B. marisindica (Zhou et al., 2018). At Longqi, dense shrimp aggregations occurred only in small quantities (Zhou et al., 2018). A lack of suitable substrate might have prevented the dominance of R. kairei at Longqi, since high-temperature fluids were only released from chimney summits (Zhou et al., 2018). Dominant species were, in distance from the venting, the scaly-foot snails C. squamiferum, peltospirid snails Lepetodrilus sp. “SWIR” and Gigantopelta aegis, mussel B. marisindica, and barnacle N. marisindica (Copley et al., 2016). The black C. squamiferum Longqi population was highly connected to the nearby Duanqiao population but exhibited low connectivity to the CIR populations (Chen et al., 2015; Zhou et al., 2018; Sun et al., 2020). Three polychaetes of the same genus occurred at Longqi: Amphisamytha marisindica which likely has an ancestor in the East Pacific, and Amphisamytha collaris and Amphisamytha sp. Longqi which were closely related to a Southwest Pacific species (Zhou et al., 2019). To date, at least 12 species have been recorded only in this vent field: the peltospirid snails Dracogyra subfuscus, Lirapex politus and Lirapex felix, Phymorhynchus sp. “SWIR”, the lobster Munidopsis sp. “SWIR”, the limpet Neolepetopsis ardua, and the polychaetes Ophryotrocha jiaolongi, Amphisamytha collaris, Amphisamytha sp. Longqi, Polynoidae sp. 2, Polynoidae n. gen. n. sp. “655”, and Laonice sp. “Longqi” (Chen et al., 2017b; Zhang et al., 2017; Zhou et al., 2018; Zhou et al., 2019; Chen et al., 2021a; Chen et al., 2021c). The peltospirid snail Gigantopelta aegis, scale worm Peinaleopolynoe sp. “Dragon”, limpet Lepetodrilus sp. “SWIR”, and yeti crab Kiwa sp. “SWIR” occur only in one other vent field, the neighboring Duanqiao (Zhou et al., 2018). A unique feature of Longqi is the co-occurrence of five peltospirids snails, all within the same assemblage (Chen et al., 2021a).
3.1.4.3. Duanqiao (37°39’S, 50°24’E, 1732m)
The basalt-hosted Duanqiao vent field was discovered during the COMRA DY20 cruise (Tao et al., 2014). It was first believed to be an inactive field, until weak fluid emissions from a large sulfide edifice were observed with a TV-grab during the COMRA DY34 cruise in 2015 (Zhou et al., 2018). While only diffuse venting was observed from the manned submersible Shenhaiyongshi, high fluid temperatures were recorded during the same 2018/2019 cruise with the RV Tansuoyihao (Liao et al., 2019).
Duanqiao is less studied than the other two SWIR vents. However, 13 taxa were collected during the DY35 cruise (Zhou et al., 2018). Duanqiao shared ten of those taxa with Longqi, including the novel species Gigantopelta aegis snails, Lepetodrilus sp. “SWIR” limpets, and Peinaleopolynoe sp. “Dragon” scale worms that were so far not found at other vent fields (Zhou et al., 2018). B. marisindicus mussels and Neolepas marisindica barnacles were the most abundant species in both the Duanqiao and Longqi vent fields (Sun et al., 2020). At first glance, Duanqiao appeared to be a subset of the neighboring Lonqi, yet multiple species were recorded at Duanqiao but not Longqi, including the gastropod Desbruyeresia sp. “SWIR” and the sea spiders Sericosura bamberi, S. heteroscala, and S. duanqiaoensis, of which the latter was a newly discovered species (Wang et al., 2018; Zhou et al., 2018). The scaly-foot snail C. squamiferum population at Duanqiao also differed from those in other vent fields, with a black shell and white sclerites (Zhou et al., 2018). This “Duanqiao type” snail showed very low genetic divergence from Longqi, and the morphotype is the result of Duanqiao’s distinct chemical environment (Zhou et al., 2018).
3.2. Generic and field-by field characteristics of active vent fields on the Indian Ocean ridges applicable to VME, EBSA, and PSSA criteria (Appendix 3)
By their very nature, vent fields globally, including those on the Indian Ocean Ridges, share fundamental ecological characteristics relevant to each of the 9 criteria assessed (Gollner et al., 2021). Criteria that are met by all vent fields include 1) Uniqueness and rarity, 2) Functional significance, 3) Fragility, 4) Life history traits of component species that makes recovery difficult, 5) Structural complexity, 6) Biological productivity, 7) Biological diversity, 8) Naturalness, and 9) Ecosystem services (Appendix 1, Appendix 2). Our scientific assessment of 12 vents fields on the Indian Ocean Ridges shows that all vent fields meet all scientific criteria for protection (Table 2 and Figure 2). A detailed field-by field analyses is also given in Appendix 3.
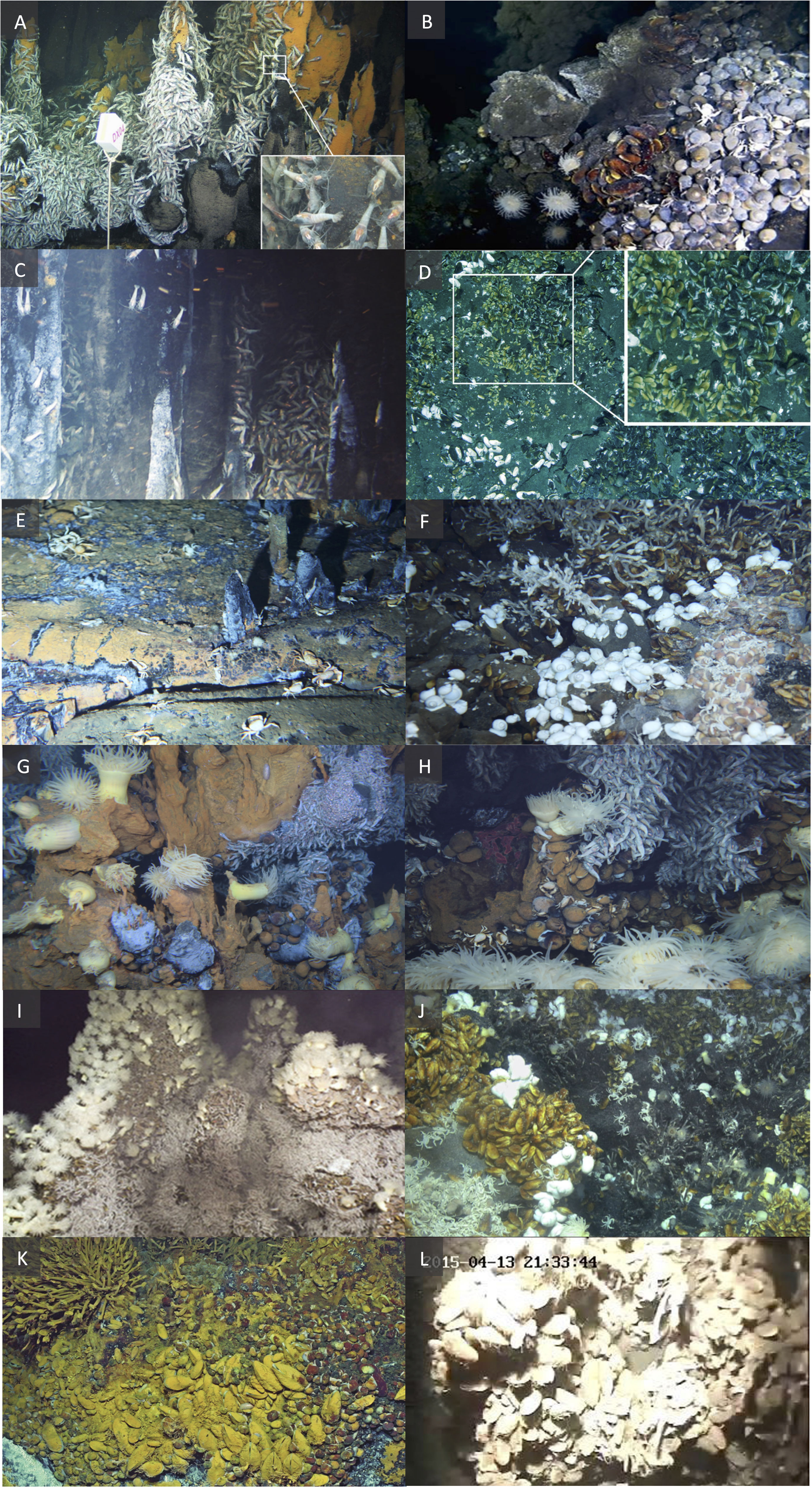
Figure 2 A selection of ecological features for each active Indian hydrothermal vent. (A) Extensive swarms of alvinocaridid shrimps at Daxi. (B) Snails, mussels, crabs, shrimps, and anemones near a black smoker at Wocan. (C) Alvinocaridid shrimp swarming on massive sulfide structures at Tianxiu. (D) Dominant mussel species Bathymodiolus marisindicus (yellow brown) and Gigantidas vrijenhoeki (dark brown) at Onnuri. (E) Sparsely distributed anemones and bythograeid crabs at Dodo. (F) Diffuse flow vent assemblage with mussels, different snails and barnacles at Solitaire. (G) Dense alvinocaridid shrimp aggregations surrounded by snails and anemones at Edmond. (H) High densities of shrimps, snails, crabs and anemones at Kairei. (I) Layered shrimp, mussel and anemone aggregations on a chimney structure at Pelagia. J) Mussel beds with patches of snails, crabs and shrimps at Tiancheng. (K) Tiamat chimney dominated by peltospirid snails, alongside dense mussel and barnacle colonies at Longqi. L) Mussel dominance at Duanqiao. Image credits; (A) Wang et al., 2021 rightslink # 5441960651432); (B) Qiu et al., 2021 (rightslink # 5441961199302); C) Zhou et al., 2022 (CC BY); (D) Jang et al., 2020 (rightslink # 5441970372620); (E, F, G, H) Beedessee et al., 2013 (CC BY); (I) Gerdes et al., 2019 (CC BY); (J) Sun et al., 2020 (CC BY); (K) Chen et al., 2021a. (rightslink # 5443111350852); (L) image from powerpoint presentation by Dr C.B. Soon at ISA workshop in Poland in 2018 (photo courtesy of COMRA).
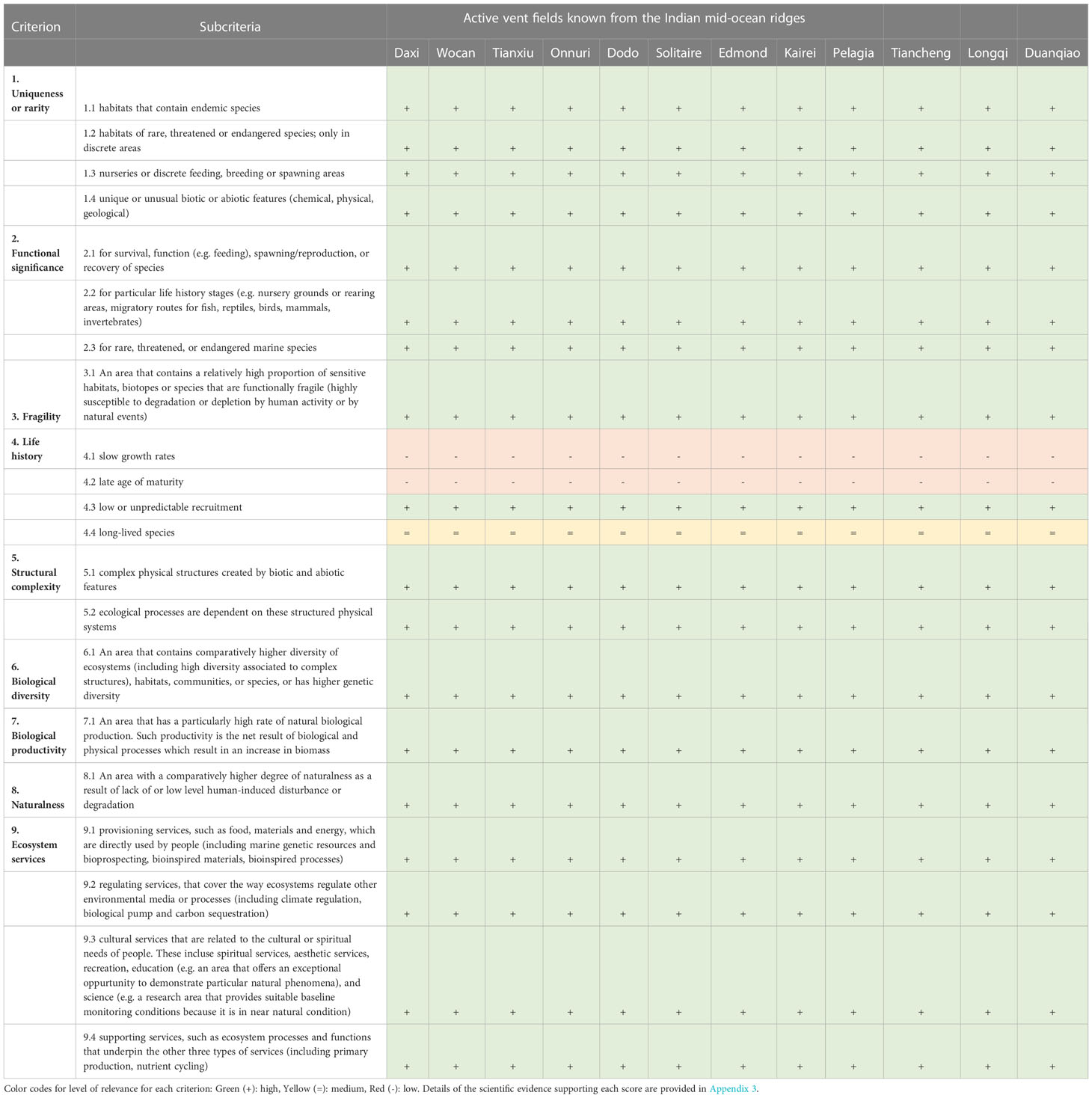
Table 2 Application of the scientific criteria for identifying hydrothermal ecosystems in need of protection at 12 Indian vent fields, based on the literature used in this review. Criteria definition follows Gollner et al. (2021) (see Appendix 1, 2).
The Indian Ocean vent fields are unique and rare as they host small, island-like vent ecosystems with distinctive biotic and abiotic features (Han et al., 2018; Wang et al., 2018; Kim et al., 2020; Chen et al., 2021a; Han et al., 2021) (Table 1). Autotrophic microorganisms use chemosynthesis to fix carbon, as animal symbionts (e.g. Alvinichona or Bathymodiolus foundation species), bacterial mats or free-living cells (Dick, 2019). All active vents are of functional significance, as they support primary production by microorganisms, serve as discrete feeding area, and are essential for the growth, survival, reproduction, and persistence of vent-endemic species (van Dover, 2001; Mullineaux et al., 2018). The juveniles and adults of vent-endemic (vent-obligate) taxa are adapted to the extreme chemical and physical conditions of the vent habitat and thrive in these specialized (unique) ecosystems. Many animal species and microbial strains found in the Indian Ocean are endemic to this specific region, or even restricted to a single vent field (Han et al., 2018; Dick, 2019). Structural habitat complexity and local chemical gradients create niches that expand biodiversity on a genetic, morphological, functional, and species level (van Dover, 2001; Dick, 2019)
Various Indian vent-endemic species so far have only been found in a single vent field, like the limpet Neolepetopsis prismatica, the sessile barnacle Eochionelasmus coreana, and the sea spider Sericosura duanqiaoensis (Wang et al., 2018; Chan et al., 2020; Chen et al., 2021c). Even when a species is present at multiple vents, local fluid composition may influence phenological expression (Minic et al., 2006; Ramirez-Llodra et al., 2007). For instance, there are at least 5 different morphotypes of the scaly-foot snail C. squamiferum due to the different chemical profiles of Solitaire, Kairei, Tiancheng, Longqi, and Duanqiao vent fluids. Furthermore, all Indian vents sustain rich microbial communities that are mostly shaped by local geochemical circumstances, often with a large variability between vent sites from a single field (Dick, 2019). Symbiotic species, such as Crysomallon squamiferum and the mussel Gigantidas vrijenhoeki, are associated with novel microbial strains (Goffredi et al., 2004; Jang et al., 2020). Novel chemolithoautotrophic and heterotrophic microbes were isolated from sulfides, sediments, and plumes of the Indian vent fields (van Dover et al., 2001; Ding et al., 2017; Hu et al., 2020).
Many benthic species rely on pelagic larval stages to maintain population and genetic connectivity (Mullineaux et al., 2018; Chapman et al., 2019). There are many uncertainties surrounding recruitment relating to reproduction, larval development, larval dispersal, and settlement (Perez et al., 2021). However, some Indian-endemic species are thought to have low dispersal abilities due to negatively buoyant larvae or eggs, including the peltospirid snails Chrysomallon squamiferum, Gigantopelta aegis, Dracogyra subfuscus, and Lirapex politus (CR) (Chen et al., 2014), or the crab Kiwa sp. “SWIR” (Reid et al., 2020). Habitat degradation and fragmentation due to anthropogenic disturbance may lead to local loss of biodiversity (Pardini et al., 2017; van Dover et al., 2017; Niner et al., 2018) and ecosystem services (Le et al., 2017).
Currently, 11 vent species found in the Indian Ocean are on red list of the IUCN4, including the vulnerable mussel Bathymodiolus marisindicus (VU), the endangered snails Alviniconcha marisindica, Chrysomallon squamiferum, Debruyeresia marisindica, and endangered mussel Bathymodiolus septemdierum (EN), as well as the critically endangered snails Gigantopelta aegis, Iphinopsis boucheti, Dracogyra subfuscus, Lirapex politus, Bruceiella wareni, and the mussel Gigantidas vrijenhoeki (CR). The first three species are characteristic members of the Indian vent metacommunities, as are the bythograeid crab Austinograea rodriguezensis, the stalked barnacle Neolepas marisindica, and the alvinocaridid shrimps Rimicaris kairei and Mirocaris indica. Although these species are common, they are absent at some vent fields (Table 1).
To date, vent fields in the Indian Ocean remain among the most pristine ecosystems on Earth as they have been visited by scientists only in recent years (Menini and van Dover, 2019; Gollner et al., 2021). Moreover, these ecosystems have an outstanding potential to contribute genetic resources and inspire biomedical, biotechnological and commercial applications (Harden-Davies, 2017; Adam et al., 2020; Orcutt et al., 2020). For instance, the ‘armor design’ of C. squamiferum may lead to innovations in military equipment, vehicles, construction machinery or astronautics (Yao et al., 2010). These ecosystems also harbor rich microbial communities that are sources of natural pharmaceuticals since some unique bacteria and archaea have so far been reported only from SWIR and CIR vents (Thornburg et al., 2010; Han et al., 2018; Dick, 2019; Dasgupta et al., 2021). More novel operational taxonomic units have been found at Edmond and Kairei than at most other vents (Han et al., 2018).
4. Discussion
Hydrothermal ecosystems in the Indian Ocean were discovered and explored much later than those in other regions, yet the uniqueness and vulnerability of each active Indian vent stands out. The application of scientific criteria for protection of these vents highlights the need for protection of active vent ecosystems in the Indian Ocean.
4.1. The ecology of Indian Ocean vent fields
The present review outlines the historic role of this region in supporting population connectivity among biogeographic provinces and reveals the similarities and differences between vents of the Indian ridges. The Indian Ocean was hypothesized to be a corridor between the Atlantic and Pacific biological communities in the past. Here, we recognize the importance of the Indian population as both a copious source of unique vent species and a central point on the global ocean floor, with widespread connections to the Atlantic, Antarctic, West-, and East-Pacific (van Dover et al., 2001; Rogers et al., 2012; Watanabe and Beedessee, 2015; Perez et al., 2021). The Indian meta-population is much like a patchwork, with clear subpopulations that may even belong to separate provinces, and are strongly linked to particular regions (Sun et al., 2020; Han et al., 2021; Perez et al., 2021).
For example, both the nSWIR-CIR and CR have a bias towards the West Pacific meta-community, with taxa like neolepas stalked barnacles, alviniconcha snails, and bythograeid crabs common in both communities (van Dover et al., 2001; Watanabe et al., 2018). The CR was also suggested to have a direct biogeographic linkage to the East Pacific because different Amphisamytha polychaetes from the CR are genetically closely related to their East Pacific counterparts (Zhou et al., 2019).
While nSWIR-CIR and CR vents were dominated by Rimicaris and Mirocaris shrimp assemblages reminiscent of the MAR, except Onnuri, sSWIR vents had high abundances of mussels and snails (Watanabe and Beedessee, 2015) (Table 1). Consistent with its relative geographical position, sSWIR vents seem to have strong ties to the East Scotia Ridge (ESR) in the Southern Ocean, characterized by high abundances of the stalked barnacle Neolepas spp., yeti crab Kiwa spp., and peltospirid snail Gigantopelta spp. (Chen et al., 2017a; Zhou et al., 2019). Similarity in community structure at species level to the ESR decreases over distance, as there is a clear continuum in community structure along the SWIR, CIR and CR (Sun et al., 2020). A proposed dispersal pathway from the Southern Pacific to the CIR via the SEIR was contradicted by molecular evidence on neolepas barnacles, indicating historic dispersal through the Southern Ocean was more likely (Watanabe et al., 2018). Still more research is needed, as the SEIR is the least studied Indian ridge and the only known active vent is merely 167 km away from Kairei, which may explain the high affinity between Pelagia and CIR vent communities.
The concept of “nearest vent, dearest friend” is further exemplified by the Tiancheng vent field, which is a nSWIR vent highly similar to CIR vents (Sun et al., 2020; Zhou et al., 2022). Besides the immediate proximity to the CIR vent fields and Kairei in particular, another important determinant of vent connectivity may be ridge continuity. Major transformation faults exist on the SWIR between Tiancheng and the other SWIR vents that presumably severely restrict larval dispersal (Sun et al., 2020). Previous genetic analyses in the East Pacific have also indicated animal dispersal is often constrained by geographical barriers common on mid-ocean ridges, such as trenches, transform faults, and microplates (Beedessee et al., 2013).
Consequently, future research should focus not only on biodiversity and functioning of single vent fields but also vent fields at a network scale. To date, only four studies have examined genetics across at least two Indian ridges and reported population connectivity of six different species (Chen et al., 2015; Watanabe et al., 2018; Sun et al., 2020; Zhou et al., 2022). However, recent work on population plasticity and genetic structure of the deep-sea mussel Bathymodiolus platifrons (Xu et al., 2017), the deep-sea limpet Bathyacmaea nipponica (Xu et al., 2021), and the deep-sea squat lobster Shinkaia crosnieri (Xiao et al., 2020) clearly showed that populations of these species from vent and cold-seep fields thousands of kilometers apart in the west Pacific Ocean could be well connected. Hydrodynamics plays a more important role than habitats or geographic distance in driving the gene flow and divergence for these populations (Xiao et al., 2020; Xu et al., 2021). These findings (and unpublished data on population genetics and connectivity from Qian Pei-Yuan’s laboratory) clearly demonstrate that population genomic surveys should be applied to future population connectivity studies of deep-sea animals.
Furthermore, there are many inferred active vent fields that have not been thoroughly studied yet. Based on the Interridge database, biological communities of 3 vents on the CR, 16 vents on the CIR, 50 vents on the SEIR and 22 vents on the SWIR wait to be described, and even more wait to be discovered. Disturbances at single vent fields may affect entire metacommunities due to the source-sink dynamics of vent species (Vrijenhoek, 2010; Mullineaux et al., 2018). Yet mining is likely to target vent fields with the largest PMS deposits, which may often be older vent fields with more developed community structures. Such vent fields can be the most diverse and likely serve as the sources of larval supplies for other vents. Before we decide on particular vent fields as representative management areas, characterization of individual locations must be done adequately while the paucity of knowledge on inter-vent connectivity within and between biogeographic provinces should also be addressed.
4.2. Governance
Previously, the International Guidelines of Deep-Sea Fisheries in the High Seas published by the FAO stated that vent fields and their associated communities “often display characteristics consistent with possible VMEs” (FAO, 2009). Lost City, Broken Spur, TAG, and Snake Pit are four active vent fields on the nMAR that have already been recognized as EBSAs by the CBD5 and in the same CBD report it was suggested other MAR vent fields should also be considered to be EBSAs. Moreover, an area of 135,688 km2 comprising 2 inferred active vents on the CIR has already been designated and implemented as a Benthic Protected Area (BPA) by the Southern Indian Ocean Fishery Agreement (SIODFA). The SIODFA BPA protects from deep-sea and mid-water trawling (Menini and van Dover, 2019) but does not prohibit other human activities. These measures are in line with the mandate to take precautionary action when managing deep-sea hydrothermal vent ecosystems (Levin et al., 2017b; van Dover et al., 2018).
Ten Indian deep-sea hydrothermal vent species are currently red-listed by the IUCN, with more than half being Critically Endangered.4 This number is likely to increase, as more deep-sea species are considered by the IUCN, due to the imperiled status of Indian vents compared to other vents globally (Thomas et al., 2021). Gollner et al. (2021) suggested that active nMAR vents are ecosystems in need of protection. In this review, we conclude that all active vent fields in Indian Ocean are also in need of protection, calling for a global effort to protect these unique ecosystems.
5. Conclusion
Hydrothermal vents are extraordinary ecosystems that may be at risk of serious harm due to future deep-sea mining or other anthropogenic impacts. This review provides an overview of the unique geochemical and biological attributes of each confirmed active vent in the Indian Ocean. The Indian meta-population was long hypothesized to be a mixture of Atlantic and Pacific communities yet is instead composed of taxa known from other biogeographic provinces and vent species unique to the Indian Ocean. Criteria previously used to classify vulnerable and significant marine ecosystems were used to assess vents at the Indian mid-ocean ridges. All active vent fields in the Indian Ocean meet the scientific criteria for protection, and both the high degree of uniqueness and fragility of these ecosystems stand out. This review and other scientific analyses underpin the important role of science in the ocean sector development. Future efforts should be focused on understanding population connectivity (gene flow), life-history, convergence, and divergence of deep-sea fauna in order to develop solid scientific evidence based regional environmental management plans for active hydrothermal ecosystems in the Indian Ocean.
Author contributions
NM drafted the article, P-YQ, YG, and SG contributed to the writing. All authors contributed to the article and approved the submitted version.
Funding
This work is supported by the UU-NIOZ project “Protecting deep seabed hydrothermal vent fields through area-based management tools” (to SG and Erik Molenaar), the grant from the Major Project of Basic and Applied Basic Research of Guangdong Province (2019B030302004), the Southern Marine Science and Engineering Guangdong Laboratory (Guangzhou) [2021HJ01, SMSEGL20SC01)], and GRF grant from the HKSAR government (16101822) to P-YQ.
Acknowledgments
The authors would like to thank Cindy L. van Dover and Terue Kihara for their valuable comments on a previous version of this manuscript.
Conflict of interest
The authors declare that the research was conducted in the absence of any commercial or financial relationships that could be construed as a potential conflict of interest.
Publisher’s note
All claims expressed in this article are solely those of the authors and do not necessarily represent those of their affiliated organizations, or those of the publisher, the editors and the reviewers. Any product that may be evaluated in this article, or claim that may be made by its manufacturer, is not guaranteed or endorsed by the publisher.
Supplementary material
The Supplementary Material for this article can be found online at: https://www.frontiersin.org/articles/10.3389/fmars.2022.1067912/full#supplementary-material
Footnotes
- ^ https://www.isa.org.jm/about-isa.
- ^ https://www.isa.org.jm/.
- ^ https://isa.org.jm/index.php/minerals/exploration-areas.
- ^ https://www.iucnredlist.org/search
- ^ https://chm.cbd.int/database/record?documentID=204107
References
Adam N., Schlicht S., Han Y., Bechelany M., Bachmann J., Perner M. (2020). Metagenomics meets electrochemistry: Utilizing the huge catalytic potential from the uncultured microbial majority for energy-storage. Front. bioengineering Biotechnol. 8, 567. doi: 10.3389/fbioe.2020.00567
Beaulieu S. E., Baker E. T., German C. R., Maffei A. (2013). An authoritative global database for active submarine hydrothermal vent fields. Geochemistry Geophysics Geosystems 14, 4892–4905. doi: 10.1002/2013GC004998
Beedessee G., Watanabe H., Ogura T., Nemoto S., Yahagi T., Nakagawa S., et al. (2013). High connectivity of animal populations in deep-sea hydrothermal vent fields in the central indian ridge relevant to its geological setting. PloS One 8, 81570. doi: 10.1371/journal.pone.0081570
BGR (2015) Report. Available at: https://www.bgr.bund.de/EN/Gemeinsames/Produkte/Downloads/Report2015_en.pdf?:blob=publicationFile&v=4 (Accessed September 2, 2022).
Breusing C., Johnson S. B., Tunnicliffe V., Vrijenhoek R. C. (2015). Population structure and connectivity in indo-pacific deep-sea mussels of the Bathymodiolus septemdierum complex. Conserv. Genet. 16, 1415–1430. doi: 10.1007/s10592-015-0750-0
CBD (2009) EBSA criteria. Available at: https://www.cbd.int/ebsa/resources.
Chan B. K. K., Ju S. J., Kim D. S., Kim S. J. (2020). First discovery of the sessile barnacle Eochionelasmus (Cirripedia: Balanomorpha) from a hydrothermal vent field in the Indian ocean. J. Mar. Biol. Assoc. United Kingdom 100, 585–593. doi: 10.1017/S0025315420000466
Chapman A. S. A., Beaulieu S. E., Colaço A., Gebruk A. v., Hilario A., Kihara T. C., et al. (2019). sFDvent: A global trait database for deep-sea hydrothermal-vent fauna. Global Ecol. Biogeography 28, 1538–1551. doi: 10.1111/geb.12975
Chen C., Copley J. T., Linse K., Rogers A. D. (2015). Low connectivity between ‘scaly-foot gastropod’ (Mollusca: Peltospiridae) populations at hydrothermal vents on the southwest Indian ridge and the central Indian ridge. Organisms Diversity Evol. 15, 663–670. doi: 10.1007/s13127-015-0224-8
Chen C., Han Y., Copley J. T., Zhou Y. (2021a). A new peltospirid snail (Gastropoda: Neomphalida) adds to the unique biodiversity of longqi vent field, southwest Indian ridge. J. Natural History 55, 851–866. doi: 10.1080/00222933.2021.1923851
Chen Y., Han X., Wang Y., Lu J. (2020). Precipitation of calcite veins in serpentinized harzburgite at tianxiu hydrothermal field on carlsberg ridge (3.67°N), Northwest Indian ocean: Implications for fluid circulation. J. Earth Sci. 31, 91–101. doi: 10.1007/s12583-020-0876-y
Chen C., Linse K., Copley J. T., Rogers A. D. (2014). The “scaly-foot gastropod”: A new genus and species of hydrothermal vent-endemic gastropod (Neomphalina: Peltospiridae) from the Indian ocean. J. Molluscan Stud. 81, 322–334. doi: 10.1093/mollus/eyv013
Chen C., Lin T. H., Watanabe H. K., Akamatsu T., Kawagucci S. (2021b). Baseline soundscapes of deep-sea habitats reveal heterogeneity among ecosystems and sensitivity to anthropogenic impacts. Limnology Oceanography 66, 3714–3727. doi: 10.1002/lno.11911
Chen J., Tao C., Liang J., Liao S., Dong C., Li H., et al. (2018). Newly discovered hydrothermal fields along the ultraslow-spreading southwest Indian ridge around 63°E. Acta Oceanologica Sin. 37, 61–67. doi: 10.1007/s13131-018-1333-y
Chen C., Uematsu K., Linse K., Sigwart J. D. (2017a). By more ways than one: Rapid convergence at hydrothermal vents shown by 3D anatomical reconstruction of Gigantopelta (Mollusca: Neomphalina). BMC Evolutionary Biol. 17, 62. doi: 10.1186/s12862-017-0917-z
Chen C., Zhou Y., Kayama Watanabe H., Zhang R., Wang C. (2021c). Neolepetopsid true limpets (Gastropoda: Patellogastropoda) from Indian ocean hot vents shed light on relationships among genera. Zoological J. Linn. Soc. 194, 276–296. doi: 10.1093/zoolinnean/zlab081/6408716
Chen C., Zhou Y., Wang C., Copley J. T. (2017b). Two new hot-vent peltospirid snails (gastropoda: Neomphalina) from longqi hydrothermal field, southwest Indian ridge. Front. Mar. Sci. 4. doi: 10.3389/fmars.2017.00392
Copley J. T., Marsh L., Glover A. G., Hühnerbach V., Nye V. E., Reid W. D. K., et al. (2016). Ecology and biogeography of megafauna and macrofauna at the first known deep-sea hydrothermal vents on the ultraslow-spreading southwest Indian ridge. Sci. Rep. 6, 39158. doi: 10.1038/srep39158
Cubelio S. S., Tsuchida S., Watanabe S. (2007). New species of Munidopsis (Decapoda: Anomura: Galatheidae) from hydrothermal vent areas of Indian and Pacific Oceans. Journal of the Marine Biological Association of the United Kingdom 88 (1), 111–117. doi: 10.1017/S0025315408000180
Dasgupta S., Peng X., Ta K. (2021). Interaction between microbes, minerals, and fluids in deep-sea hydrothermal systems. Minerals 11, 1324. doi: 10.3390/min11121324
Dick G. J. (2019). The microbiomes of deep-sea hydrothermal vents: distributed globally, shaped locally. Nat. Rev. Microbiol. 17, 271–283. doi: 10.1038/s41579-019-0160-2
Ding J., Zhang Y., Wang H., Jian H., Leng H., Xiao X. (2017). Microbial community structure of deep-sea hydrothermal vents on the ultraslow spreading southwest Indian ridge. Front. Microbiol. 8. doi: 10.3389/fmicb.2017.01012
Earth Economics (2015). Report: Environmental and social benchmarking analysis of nautilus minerals Inc. solwara 1 project.
Fang Z., Wang W. X. (2021). Size speciation of dissolved trace metals in hydrothermal plumes on the southwest Indian ridge. Sci. Total Environ. 771, 145367. doi: 10.1016/j.scitotenv.2021.145367
FAO (2009) VME criteria. Available at: http://www.fao.org/in-action/vulnerable-marine-ecosystems/criteria/en/.
Galkin S. v (2016). “Structure of hydrothermal vent communities,” in Trace metal biogeochemistry and ecology of deep-sea hydrothermal vent systems. Eds. Demina L. L., Galkin S. v (Cham: Springer International Publishing), 77–95. doi: 10.1007/698_2015_5018
Gerdes K., Arbizu P. M., Schwarz-Schampera U., Schwentner M., Kihara T. C. (2019). Detailed mapping of hydrothermal vent fauna: A 3d reconstruction approach based on video imagery. Front. Mar. Sci. 6. doi: 10.3389/fmars.2019.00096
Gerdes K., Kihara T. C., Arbizu P. M., Kuhn T., Schwarz-Schampera U., Mah C. L., et al. (2021). Megafauna of the German exploration licence area for seafloor massive sulphides along the central and south East Indian ridge (Indian ocean). Biodiversity Data J. 28 (9), e69955. doi: 10.3897/BDJ.9.e69955
Goffredi S. K., Warén A., Orphan V. J., van Dover C. L., Vrijenhoek R. C. (2004). Novel forms of structural integration between microbes and a hydrothermal vent gastropod from the Indian ocean. Appl. Environ. Microbiol. 70, 3082–3090. doi: 10.1128/AEM.70.5.3082-3090.2004
Gollner S., Colaço A., Gebruk A., Halpin P. N., Higgs N., Menini E., et al. (2021). Application of scientific criteria for identifying hydrothermal ecosystems in need of protection. Mar. Policy 132, 104641. doi: 10.1016/j.marpol.2021.104641
Gollner S., Govenar B., Arbizu P. M., Mills S., le Bris N., Weinbauer M., et al. (2015). Differences in recovery between deep-sea hydrothermal vent and vent-proximate communities after a volcanic eruption. Deep-Sea Res. Part I: Oceanographic Res. Papers 106, 167–182. doi: 10.1016/j.dsr.2015.10.008
Gollner S., Govenar B., Martinez Arbizu P., Mullineaux L. S., Mills S., le Bris N., et al. (2020). Animal community dynamics at senescent and active vents at the 9°N East pacific rise after a volcanic eruption. Front. Mar. Sci. 6. doi: 10.3389/fmars.2019.00832
Gollner S., Ivanenko V. N., Arbizu P. M., Bright M. (2010). Advances in taxonomy, ecology, and biogeography of dirivultidae (Copepoda) associated with chemosynthetic environments in the deep sea. PloS One 5, 9801.10. doi: 10.1371/journal.pone.0009801
Gollner S., Kaiser S., Menzel L., Jones D. O. B., Brown A., Mestre N. C., et al. (2017). Resilience of benthic deep-sea fauna to mining activities. Mar. Environ. Res. 129, 76–101. doi: 10.1016/j.marenvres.2017.04.010
Gollner S., Stuckas H., Kihara T. C., Laurent S., Kodami S., Arbizu P. M. (2016). Mitochondrial DNA analyses indicate high diversity, expansive population growth and high genetic connectivity of vent copepods (Dirivultidae) across different oceans. PloS One 11, 163776. doi: 10.1371/journal.pone.0163776
Grassle J. F. (1985). Hydrothermal vent animals: Distribution and biology. Sci. (1979) 229, 4715713–717. doi: 10.1126/science.229.4715.713
Grassle J. F. (1986). “The ecology of deep-sea hydrothermal vent communities,” in Advances in marine biology. Eds. Blaxter J. H. S., Southward A. J. (Academic Press), 301–362. doi: 10.1016/S0065-2881(08)60110-8
Han Y., Gonnella G., Adam N., Schippers A., Burkhardt L., Kurtz S., et al. (2018). Hydrothermal chimneys host habitat-specific microbial communities: Analogues for studying the possible impact of mining seafloor massive sulfide deposits. Sci. Rep. 8, 1–12. doi: 10.1038/s41598-018-28613-5
Hannington M. D., de Ronde C. E. J., Petersen S. (2005). “Sea-Floor tectonics and submarine hydrothermal systems,” in Economic geology 100th anniversary (Littleton, Colorado: Society of Economic Geologists, Inc), 111–141.
Han Y., Zhang D., Wang C., Zhou Y. (2021). Out of the pacific: A new alvinellid worm (Annelida: Terebellida) from the northern Indian ocean hydrothermal vents. Front. Mar. Sci. 8. doi: 10.3389/fmars.2021.669918
Harden-Davies H. (2017). Deep-sea genetic resources: New frontiers for science and stewardship in areas beyond national jurisdiction. Deep-Sea Res. Part II: Topical Stud. Oceanography 137, 504–513. doi: 10.1016/j.dsr2.2016.05.005
Hashimoto J. (2001). A new species of bathymodiolus (Bivalvia: Mytilidae) from hydrothermal vent communities in the Indian ocean. Venus (Journal Malacological Soc. Japan) 60 (3), 141–149. doi: 10.18941/venus.60.3_141
Hashimoto J., Ohta S., Gamo T., Chiba H., Yamaguchi T., Tsuchida S., et al. (2001). First hydrothermal vent communities from the Indian ocean discovered. Zoological Sci. 18, 717–721. doi: 10.2108/zsj.18.717
Hu B., Xu B., Yun J., Wang J., Xie B., Li C., et al. (2020). High-throughput single-cell cultivation reveals the underexplored rare biosphere in deep-sea sediments along the southwest Indian ridge. Lab. Chip 20, 363–372. doi: 10.1039/c9lc00761j
Hwang H. S., Cho B., Cho J., Park B., Kim T. (2022). New record of hydrothermal vent squat lobster (Munidopsis lauensis) provides evidence of a dispersal corridor between the pacific and Indian oceans. J. Mar. Sci. Eng. 10, 400. doi: 10.3390/jmse10030400
IMO (2005) PSSA criteria. Available at: https://www.imo.org/en/OurWork/Environment/Pages/PSSAs.aspx.
Jamieson J. W., Gartman A. (2020). Defining active, inactive, and extinct seafloor massive sulfide deposits. Mar. Policy 117, 103926. doi: 10.1016/j.marpol.2020.103926
Jang S. J., Ho P. T., Jun S. Y., Kim D., Won Y. J. (2020). A newly discovered Gigantidas bivalve mussel from the onnuri vent field in the northern central Indian ridge. Deep-Sea Res. Part I: Oceanographic Res. Papers 161, 103299. doi: 10.1016/j.dsr.2020.103299
Kawagucci S., Miyazaki J., Nakajima R., Nozaki T., Takaya Y., Kato Y., et al. (2013). Post-drilling changes in fluid discharge pattern, mineral deposition, and fluid chemistry in the iheya north hydrothermal field, Okinawa trough. Geochemistry Geophysics Geosystems 14, 4774–4790. doi: 10.1002/2013GC004895
Kawagucci S., Miyazaki J., Noguchi T., Okamura K., Shibuya T., Watsuji T., et al. (2016). Fluid chemistry in the solitaire and dodo hydrothermal fields of the central Indian ridge. Geofluids 16, 988–1005. doi: 10.1111/gfl.12201
Kawagucci S., Okamura K., Kiyota K., Tsunogai U., Sano Y., Tamaki K., et al. (2008). Methane, manganese, and helium-3 in newly discovered hydrothermal plumes over the central Indian ridge, 18°-20°S. Geochemistry Geophysics Geosystems 9, 2082. doi: 10.1029/2008GC002082
Kim J. G., Lee J. (2020). A new species of the genus Smacigastes ivanenko & defaye 2004 (Tegastidae, harpacticoida, copepoda) from the onnuri vent field in the Indian ocean. Zoosystematics Evol. 96, 699–714. doi: 10.3897/ZSE.96.54507
Kim J., Son S. K., Kim D., Pak S. J., Yu O. H., Walker S. L., et al. (2020). Discovery of active hydrothermal vent fields along the central Indian ridge, 8–12°S. Geochemistry Geophysics Geosystems 21, 9058. doi: 10.1029/2020GC009058
Komai T., Martin J. W., Zala K., Tsuchida S., Hashimoto J. (2006). A new species of Mirocaris (Crustacea: Decapoda: Caridea: Alvinocarididae) associated with hydrothermal vents on the central Indian ridge, Indian ocean. Scientia Marina 70, 109–119. doi: 10.3989/scimar.2006.70n1109
Kumagai H., Nakamura K., Toki T., Morishita T., Okino K., Ishibashi J. I., et al. (2008). Geological background of the kairei and edmond hydrothermal fields along the central Indian ridge: Implications of their vent fluids’distinct chemistry. in. Geofluids 8 (4), 239–251. doi: 10.1111/j.1468-8123.2008.00223.x
Lee J. (2020) Report of the workshop on the development of a regional environmental management plan for the area of the northern mid-atlantic ridge with a focus on polymetallic sulphide deposits. Available at: https://isa.org.jm/files/files/documents/ts22.pdf.
Lee J., Kim D., Kim I. H. (2020). Copepoda (Siphonostomatoida: Dirivultidae) from hydrothermal vent fields on the central indian ridge, Indian ocean. Zootaxa 4759, 301–337. doi: 10.11646/zootaxa.4759.3.1
Le J. T., Levin L. A., Carson R. T. (2017). Incorporating ecosystem services into environmental management of deep-seabed mining. Deep-Sea Res. Part II: Topical Stud. Oceanography 137, 486–503. doi: 10.1016/j.dsr2.2016.08.007
Levin L. A., Baco A. R., Bowden D. A., Colaco A., Cordes E. E., Cunha M. R., et al. (2016a). Hydrothermal vents and methane seeps: Rethinking the sphere of influence. Front. Mar. Sci. 3. doi: 10.3389/fmars.2016.00072
Levin L. A., Mengerink K., Gjerde K. M., Rowden A. A., van Dover C. L., Clark M. R., et al. (2016b). Defining “serious harm“ to the marine environment in the context of deep-seabed mining. Mar. Policy 74, 245–259. doi: 10.1016/j.marpol.2016.09.032
Liao S., Tao C., Dias Á.A., Su X., Yang Z., Ni J., et al. (2019). Surface sediment composition and distribution of hydrothermal derived elements at the duanqiao-1 hydrothermal field, southwest Indian ridge. Mar. Geology 416, 105975. doi: 10.1016/j.margeo.2019.105975
Lutz R. A., Kennish M. J. (1993). Ecology of deep-sea hydrothermal vent communities: A review. Rev. geophysics 31, 211–242. doi: 10.1029/93RG01280
Marsh L., Copley J. T., Huvenne V. A. I., Linse K., Reid W. D. K., Rogers A. D., et al. (2012). Microdistribution of faunal assemblages at deep-sea hydrothermal vents in the southern ocean. PloS One 7, 48348. doi: 10.1371/journal.pone.0048348
Menini E., van Dover C. L. (2019). An atlas of protected hydrothermal vents. Mar. Policy 108, 103654. doi: 10.1016/j.marpol.2019.103654
Minic Z., Serre V., Hervé G. (2006). Adaptation of organisms to extreme conditions of deep-sea hydrothermal vents. Comptes Rendus - Biologies 329, 527–540. doi: 10.1016/j.crvi.2006.02.001
Moalic Y., Desbruyères D., Duarte C. M., Rozenfeld A. F., Bachraty C., Arnaud-Haond S. (2012). Biogeography revisited with network theory: Retracing the history of hydrothermal vent communities. Systematic Biol. 61, 127–137. doi: 10.1093/sysbio/syr088
Mullineaux L. S., Metaxas A., Beaulieu S. E., Bright M., Gollner S., Grupe B. M., et al. (2018). Exploring the ecology of deep-sea hydrothermal vents in a metacommunity framework. Front. Mar. Sci. 4. doi: 10.3389/fmars.2018.00049
Nakamura K., Takai K. (2015). “Indian Ocean hydrothermal systems: Seafloor hydrothermal activities, physical and chemical characteristics of hydrothermal fluids, and vent-associated biological communities,” in Subseafloor biosphere linked to hydrothermal systems: TAIGA concept (Tokyo: Springer Japan), 147–161. doi: 10.1007/978-4-431-54865-2_12
Nakamura K., Watanabe H., Miyazaki J., Takai K., Kawagucci S., Noguchi T., et al. (2012). Discovery of new hydrothermal activity and chemosynthetic fauna on the central Indian ridge at 18°-20°S. PloS One 7, 32965. doi: 10.1371/journal.pone.0032965
Niner H. J., Ardron J. A., Escobar E. G., Gianni M., Jaeckel A., Jones D. O. B., et al. (2018). Deep-sea mining with no net loss of biodiversity-an impossible aim. Front. Mar. Sci. 5. doi: 10.3389/fmars.2018.00053
Orcutt B. N., Bradley J. A., Brazelton W. J., Estes E. R., Goordial J. M., Huber J. A., et al. (2020). Impacts of deep-sea mining on microbial ecosystem services. Limnology Oceanography 65, 1489–1510. doi: 10.1002/lno.11403
Pardini R., Nichols L., Püttker T. (2017). “Biodiversity response to habitat loss and fragmentation,” in Encyclopedia of the anthropocene 2017 (Oxford: Elsevier), 229–239. doi: 10.1016/b978-0-12-409548-9.09824-9
Patriat P., Sauter D., Munschy M., Parson L. (1997). A survey of the southwest Indian ridge axis between Atlantis II fracture zone and the Indian ocean triple junction: Regional setting and large scale segmentation. Mar. Geophysical Res. 19, 457–480. doi: 10.1023/A:1004312623534
Perez M., Sun J., Xu Q., Qian P. Y. (2021). Structure and connectivity of hydrothermal vent communities along the mid-ocean ridges in the west Indian ocean: A review. Front. Mar. Sci. 8. doi: 10.3389/fmars.2021.744874
Qiu Z., Han X., Li M., Wang Y., Chen X., Fan W., et al. (2021). The temporal variability of hydrothermal activity of wocan hydrothermal field, carlsberg ridge, northwest Indian ocean. Ore Geology Rev. 132, 103999. doi: 10.1016/j.oregeorev.2021.103999
Ramirez-Llodra E., Shank T. M., German C. R. (2007). Biodiversity and biogeography of hydrothermal vent species: Thirty years of discovery and investigations. Source: Oceanography 20, 30–41. doi: 10.2307/24859973
Ray D., Kamesh Raju K. A., Baker E. T., Srinivas Rao A., Mudholkar A., Lupton J. E., et al. (2012). Hydrothermal plumes over the carlsberg ridge, Indian ocean. Geochemistry Geophysics Geosystems 13, Q01009. doi: 10.1029/2011GC003888
Reid W. D. K., Wigham B. D., Marsh L., Weston J. N. J., Zhu Y., Copley J. T. (2020). Trophodynamics at the longqi hydrothermal vent field and comparison with the East Scotia and central Indian ridges. Mar. Biol. 167, 141. doi: 10.1007/s00227-020-03755-1
Rogers A. D., Tyler P. A., Connelly D. P., Copley J. T., James R., Larter R. D., et al. (2012). The discovery of new deep-sea hydrothermal vent communities in the southern ocean and implications for biogeography. PloS Biol. 10, 1001234. doi: 10.1371/journal.pbio.1001234
Ryu T., Kim J. G., Lee J., Yu O. H., Yum S., Kim D., et al. (2021). First transcriptome assembly of a newly discovered vent mussel, gigantidas vrijenhoeki, at onnuri vent field on the northern central Indian ridge. Mar. Genomics 57, 100819. doi: 10.1016/j.margen.2020.100819
Ryu T., Woo S., Lee N. (2019). The first reference transcriptome assembly of the stalked barnacle, neolepas marisindica, from the onnuri vent field on the central Indian ridge. Mar. Genomics 48, 100679. doi: 10.1016/j.margen.2019.04.004
Scheirer D. S., Baker E. T., Johnson K. T. M. (1998). Detection of hydrothermal plumes along the southeast Indian ridge near the Amsterdam-st. Paul plateau. Geophysical Res. Lett. 25, 97–100. doi: 10.1029/97GL03443
Sempéré J.-C., Cochran J. R. (1997). The southeast Indian ridge between 88°E and 118°E: Variations in crustal accretion at constant spreading rate. J. Geophysical Research: Solid Earth 102, 15489–15505. doi: 10.1029/97jb00171
Smirnov A. V., Gebruk A. V., Galkin S. V., Shank T. (2000). New species of holothurian (Echinodermata: Holothuroidea) from hydrothermal vent habitats. J. Mar. Biol. Assoc. United Kingdom 80, 321–328. doi: 10.1017/S0025315499001897
Suh Y. J., Kim M. S., Kim S. J., Kim D., Ju S. J. (2022). Carbon sources and trophic interactions of vent fauna in the onnuri vent field, Indian ocean, inferred from stable isotopes. Deep-Sea Res. Part I: Oceanographic Res. Papers 182, 103683. doi: 10.1016/j.dsr.2021.103683
Sun J., Zhou Y., Chen C., Kwan Y. H., Sun Y., Wang X., et al. (2020). Nearest vent, dearest friend: Biodiversity of tiancheng vent field reveals cross-ridge similarities in the Indian ocean. R. Soc. Open Sci. 7, 202110. doi: 10.1098/rsos.200110
Tamaki K. (2010). “Dodo field and solitaire field: Newly discovered hydrothermal fields at the central Indian ridge,” in AGU fall meeting abstracts (San Francisco, California: American Geophysical Union), OS21A–O1468.
Tao C., Li H., Jin X., Zhou J., Wu T., He Y., et al. (2014). Seafloor hydrothermal activity and polymetallic sulfide exploration on the southwest Indian ridge. Chin. Sci. Bull. 59, 2266–2276. doi: 10.1007/s11434-014-0182-0
Tao C., Lin J., Guo S., Chen Y. J., Wu G., Han X., et al. (2012). First active hydrothermal vents on an ultraslow-spreading center: Southwest Indian ridge. Geology 40, 47–50. doi: 10.1130/G32389.1
Thaler A. D., Amon D. (2019). 262 voyages beneath the Sea: A global assessment of macro- and megafaunal biodiversity and research effort at deep-sea hydrothermal vents. PeerJ 7, e7397. doi: 10.7717/peerj.7397
Thiel R., Knebelsberger T., Kihara T., Gerdes K. (2021). Description of a new eelpout pachycara angeloi sp. nov.(Perciformes: Zoarcidae) from deep-sea hydrothermal vent fields in the Indian ocean. Zootaxa 4980, 99112. doi: 10.11646/zootaxa.4980.1.6
Thomas E. A., Molloy A., Hanson N. B., Böhm M., Seddon M., Sigwart J. D. (2021). A global red list for hydrothermal vent molluscs. Front. Mar. Sci. 8. doi: 10.3389/fmars.2021.713022
Thornburg C. C., Mark Zabriskie T., McPhail K. L. (2010). Deep-sea hydrothermal vents: Potential hot spots for natural products discovery. J. Natural Products 73, 489–499. doi: 10.1021/np900662k
Turner P. J., Thaler A. D., Freitag A., Collins P. C. (2019). Deep-sea hydrothermal vent ecosystem principles: Identification of ecosystem processes, services and communication of value. Marine Policy 101, 118–124. doi: 10.1016/j.marpol.2019.01.003
van Dover C. L. (2001). The ecology of deep-Sea hydrothermal vents (Princeton University Press). doi: 10.1515/9780691239477
van Dover C. L. (2002). Trophic relationships among invertebrates at the kairei hydrothermal vent field (Central Indian ridge). Mar. Biol. 141, 761–772. doi: 10.1007/s00227-002-0865-y
van Dover C. L., Ardron J. A., Escobar E., Gianni M., Gjerde K. M., Jaeckel A., et al. (2017). Biodiversity loss from deep-sea mining. Nat. Geosci. 10, 464–465. doi: 10.2771/43949
van Dover C. L., Arnaud-Haond S., Gianni M., Helmreich S., Huber J. A., Jaeckel A. L., et al. (2018). Scientific rationale and international obligations for protection of active hydrothermal vent ecosystems from deep-sea mining. Mar. Policy 90, 20–28. doi: 10.1016/j.marpol.2018.01.020
van Dover C. L., Humphris S. E., Fornari D., Cavanaugh C. M., Collier R., Goffredi S. K., et al. (2001). Biogeography and ecological setting of Indian ocean hydrothermal vents. Science New Jersey 294, 818–823. doi: 10.1515/9780691239477
von Damm K. L. (1995). “Controls on the chemistry and temporal variability of seafloor hydrothermal fluids,” in Seafloor hydrothermal systems: Physical, chemical, biological, and geological interactions. Eds. Humphris S. E., Zierenberg R. A., Mullineaux L. S., Thomson R. E. (Washington DC: American Geophysical Union), 222–247.
Vrijenhoek R. C. (2010). Genetic diversity and connectivity of deep-sea hydrothermal vent metapopulations. Mol. Ecol. 19 (20), 4391–4411. doi: 10.1111/j.1365-294X.2010.04789.x
Wang Y., Han X., Petersen S., Frische M., Qiu Z., Li H., et al. (2017). Mineralogy and trace element geochemistry of sulfide minerals from the wocan hydrothermal field on the slow-spreading carlsberg ridge, Indian ocean. Ore Geology Rev. 84, 1–19. doi: 10.1016/j.oregeorev.2016.12.020
Wang Y., Han X., Zhou Y., Qiu Z., Yu X., Petersen S., et al. (2021). The daxi vent field: An active mafic-hosted hydrothermal system at a non-transform offset on the slow-spreading carlsberg ridge, 6°48′N. Ore Geology Rev. 129, 103888. doi: 10.1016/j.oregeorev.2020.103888
Wang J., Huang D., Shi X., Lin R., Niu W. (2018). First record and a new species of Sericosura fry hedgpeth1969 (Arthropoda: Pycnogonida: Ammotheidae) from a hydrothermal vent of southwestern Indian ridge. Zootaxa 4420, 131–138. doi: 10.11646/zootaxa.4420.1.8
Warén A., Bengtson S., Goffredi S. K., van Dover C. L. (2003). A hot-vent gastropod with iron sulfide dermal sclerites. Science 302, 1007. doi: 10.1126/science.1087696
Watabe H., Hashimoto J. (2002). A new species of the genus Rimicaris (Alvinocarididae: Caridea: Decapoda) from the active hydrothermal vent field, “Kairei field,” on the central Indian ridge, the Indian ocean. Zoological Sci. 19, 1167–1174. doi: 10.2108/zsj.19.1167
Watanabe H., Beedessee G. (2015). “Vent fauna on the central Indian ridge,” in Subseafloor biosphere linked to hydrothermal systems: TAIGA concept (Tokyo: Springer Japan), 205–212. doi: 10.1007/978-4-431-54865-2_16
Watanabe H. K., Chen C., Marie D. P., Takai K., Fujikura K., Chan B. K. K. (2018). Phylogeography of hydrothermal vent stalked barnacles: A new species fills a gap in the Indian ocean ‘dispersal corridor’ hypothesis. R. Soc. Open Sci. 5, 172408. doi: 10.1098/rsos.172408
Weaver P. P. E., Aguzzi J., Boschen-Rose R. E., Colaço A., de Stigter H., Gollner S., et al. (2022). Assessing plume impacts caused by polymetallic nodule mining vehicles. Mar. Policy 139, 105011. doi: 10.1016/j.marpol.2022.105011
Wedding L. M., Reiter S. M., Smith C. R., Gjerde K. M., Kittinger J. N., Friedlander A. M., et al. (2015). Managing mining of the deep seabed: Contracts are being granted, but protections are lagging. Science 349, 144–145. doi: 10.1126/science.aac6647
Xiao Y., Xu T., Sun J., Wang Y., Wong W. C., Kwan Y. H., et al. (2020). Population genetic structure and gene expression plasticity of the deep-sea vent seep squat lobster Shinkaia crosnieri. Frontier Mar. Sci. 7. doi: 10.3389/fmars.2020.587686
Xu T., Sun J., Watanabe H. K., Chen C., Nakamura M., Ji R., et al. (2017). Population genetic structure of the deep-sea mussel Bathymodiolus platifrons (Bivalvia: Mytilidae) in the Northwest pacific. Evolutionary Appl. 11, 1915–1930. doi: 10.1111/eva.12696
Xu T., Wang Y., Sun J., Cheng C., Watanabe H. K., Chen J. L., et al. (2021). Hidden historical habitat-linked population divergence and contemporary gene flow of a deep-se patellogastropod limpet. Mol. Biol. Evol. 38 (12), 5640–5654. doi: 10.1111/eva.12696
Yao H., Dao M., Imholt T., Huang J., Wheeler K., Bonilla A., et al. (2010). Protection mechanisms of the iron-plated armor of a deep-sea hydrothermal vent gastropod. Proc. Natl. Acad. Sci. United States America 107, 987–992. doi: 10.1073/pnas.0912988107
Zhang D. S., Zhou Y. D., Wang C. S., Rouse G. W. (2017). A new species of ophryotrocha (Annelida, eunicida, dorvilleidae) from hydrothermal vents on the southwest Indian ridge. Zookeys 687, 1–9. doi: 10.3897/zookeys.687.13046
Zhou Y., Chen C., Sun Y., Watanabe H. K., Zhang R., Wang C. (2019). Amphisamytha (Annelida: Ampharetidae) from Indian ocean hydrothermal vents: Biogeographic implications. Deep-Sea Res. Part I: Oceanographic Res. Papers 154, 103148. doi: 10.1016/j.dsr.2019.103148
Zhou Y., Chen C., Zhang D., Wang Y., Watanabe H. K., Sun J., et al. (2022). Delineating biogeographic regions in Indian ocean deep-sea vents and implications for conservation. Diversity Distributions. 28 (12), 1–13. doi: 10.1111/ddi.13535
Zhou Y., Zhang D., Zhang R., Liu Z., Tao C., Lu B., et al. (2018). Characterization of vent fauna at three hydrothermal vent fields on the southwest Indian ridge: Implications for biogeography and interannual dynamics on ultraslow-spreading ridges. Deep-Sea Res. Part I: Oceanographic Res. Papers 137, 1–12. doi: 10.1016/j.dsr.2018.05.001
Keywords: hydrothermal vent fields, Indian Ocean, deep-sea mining, vulnerable marine ecosystems (VMEs), ecologically or biologically significant areas (EBSAs), particularly sensitive sea areas (PSSAs), International Union for Conservation of Nature (IUCN)
Citation: van der Most N, Qian P-Y, Gao Y and Gollner S (2023) Active hydrothermal vent ecosystems in the Indian Ocean are in need of protection. Front. Mar. Sci. 9:1067912. doi: 10.3389/fmars.2022.1067912
Received: 12 October 2022; Accepted: 28 December 2022;
Published: 25 January 2023.
Edited by:
Maree E. Fudge, University of Tasmania, AustraliaReviewed by:
Brooks A. Kaiser, University of Southern Denmark, DenmarkMirjam Perner, Helmholtz Association of German Research Centres (HZ), Germany
Copyright © 2023 van der Most, Qian, Gao and Gollner. This is an open-access article distributed under the terms of the Creative Commons Attribution License (CC BY). The use, distribution or reproduction in other forums is permitted, provided the original author(s) and the copyright owner(s) are credited and that the original publication in this journal is cited, in accordance with accepted academic practice. No use, distribution or reproduction is permitted which does not comply with these terms.
*Correspondence: Naomi van der Most, nvdmost@protonmail.com; Pei-Yuan Qian, boqianpy@ust.hk