Gastropod chemoreception behaviors—Mechanisms underlying the perception and location of targets and implications for shellfish fishery development in aquatic environments
- 1Research and Development Center for Efficient Utilization of Coastal Bioresources, Yantai Institute of Coastal Zone Research, Chinese Academy of Sciences, Yantai, China
- 2CAS Key Laboratory of Marine Ecology and Environmental Sciences, Institute of Oceanology, Chinese Academy of Sciences, Qingdao, China
- 3Laboratory for Marine Ecology and Environmental Science, Qingdao National Laboratory for Marine Science and Technology, Qingdao, China
- 4Center for Ocean Mega-Science, Chinese Academy of Sciences, Qingdao, China
- 5CAS Engineering Laboratory for Marine Ranching, Institute of Oceanology, Chinese Academy of Sciences, Qingdao, China
- 6Shandong Province Key Laboratory of Experimental Marine Biology, Qingdao, China
Chemoreception is one of the most important senses and it plays a key role in the survival and adaptation of animals to their environments. Many gastropods, such as Rapana venosa and Neptunea cumingii, have emerged as economically important shellfish. Meanwhile, invasive gastropods have destroyed commercial shellfisheries in many countries. Given the importance of chemoreception in the behavior of aquatic gastropods, the mechanism of their chemical perception has recently garnered attention. To provide a foundation for understanding the chemoreception of aquatic gastropods, we reviewed recent studies and summarized the chemoreception behaviors and mechanisms that gastropods use to perceive and locate targets in aquatic environments, along with relevant molecular and genetic insights. We highlight several of the implications of these studies for chemoreception-related research and shellfish fishery development. This review should aid in the rational development and utilization of gastropod resources as well as in controlling the populations of invasive species.
1. Introduction
Chemical molecules are known to influence intraspecific and interspecific interactions, thereby shaping ecosystem structures. Unsurprisingly, chemical communication is one of the most important languages in nature (Kirsch, 2022). Animals recognize and distinguish different chemical cues in the environment through olfaction or gustation, thus obtaining information that affects their behavior and is ultimately necessary for survival. Marine and freshwater environments are particularly suitable for molecular transmission and chemical molecules are important sources of information for aquatic organisms. Many of the behaviors of these organisms can be regulated through the perception of chemical molecules (Zimmer and Butman, 2000).
Gastropods typically receive limited visual information, due to environmental and/or physiological conditions, but they have evolved to detect chemical signals. Therefore, whether herbivorous or carnivorous, gastropods must select useful chemical cues from surrounding chemical cues to adapt to complex and changing environments within their sensory range. Deciding whether to approach or avoid the sources of different chemical cues may be one of the most important strategies in gastropod survival. Chemical signals mediate many of the key life processes of aquatic organisms, such as predation, aggregation, settlement, and metamorphosis. For example, gastropods can use chemical signals transmitted by the water to find food (Weissburg, 2000; Webster and Weissburg, 2009) and chemical molecules can mediate the recognition or localization of potential mates among gastropods (Moomjian et al., 2003). Gastropod larvae also use chemical cues to settle and metamorphose (Bornancin et al., 2017). All of these chemically mediated chemoreception behaviors directly affect individuals and indirectly affect populations, communities, and ecosystems.
Researchers have made great progress in understanding gastropod chemoreception at the behavioral and morphological levels. For instance, studies have been conducted on the influence of water flow on chemoreception predation (Ferner and Weissburg, 2005; Yu et al., 2019b), and Kirsch (2022) described intra- and interspecific chemical communication in freshwater gastropods. However, only a few studies at the molecular and genetic levels exist (Cummins et al., 2009a; Cummins et al., 2009b; Yu et al., 2022) and systematic work on chemoreception behavior and the mechanisms by which targets (including prey and conspecifics) are perceived and located in aquatic environments remain lacking. Most research has primarily focused on developing a basic understanding of gastropod chemical perception and has lacked relevant suggestions on the development and management of gastropod fisheries. In this review, we summarize the mechanisms of gastropod chemoreception behavior, perception, and localization in aquatic environments by assessing what is known from the molecular and genetic levels. Our aim is to provide a reference for future research on the development of gastropod fisheries, invasive species control, and gastropod behavior, ecology, and physiology.
2. Gastropod chemoreception behavior
Chemical cues mediate many of the key life processes of aquatic gastropods, such as predation, aggregation, settlement and metamorphosis. Predation plays a key role in structuring ecological communities. Many of the activities of both predator and prey species, such as their distribution, and population dynamics, are driven by predation (Hu et al., 2016). Aggregation is an important group behavior that benefits individuals and populations by reducing water and energy loss (Yoder et al., 2002; Gilbert et al., 2007; Kotze et al., 2008), deterring predation (Hatle and Salazar, 2001), and enhancing fecundity (Wells et al., 1990). Many organisms, including mammals, insects, and aquatic organisms, exhibit aggregation behaviors, which are effective for resisting adversity and improving survivorship (Scheibling and Lauzon-Guay, 2007; Kobak et al., 2009; Zhang et al., 2018b). Settlement and metamorphosis are key processes in the ontogenetic development of many gastropods from larvae to juvenile snails (Yang et al., 2023). Larval settlement and metamorphosis often result in larval death, which is the key factor leading to the failure of artificial breeding of gastropods. Therefore, the correct understanding of gastropod chemoreception behavior impacts the population dynamics, distribution and resource utilization of gastropods fishery and is of ecological importance.
2.1. Predation
Understanding how predators perceive their prey is crucial to the study of predator–prey interactions. Predatory behaviors that are mediated by chemical information in the water play an important role in the food selection of gastropods.Gastropods have a precise chemoreception mechanism and can perceive and locate food through chemical information. Chemoreception has been studied in many gastropods, including scavenging, carnivorous, and herbivorous taxa (Ferner and Weissburg, 2005; Bornancin et al., 2017). For example, the marine gastropod Buccinum undam can be attracted to dead fish (Lapointe and Sainte-Marie, 1992), while Busycon carica has been shown to effectively prey on bivalves and barnacles from a distance of 1–2 m by olfaction (Ferner and Weissburg, 2005), Similarly, Littorina irroratus exhibits efficient sensory responses to the odors of its plant prey (Duval et al., 1994).
The lack of understanding of how predators perceive and locate prey often leads to important problems in gastropod artificial breeding and resource management, such as food waste, water pollution and reduction of gastropod resources. In a recent study, Yu et al. (2019b) proposed that unreasonable feeding method is one of the reasons for the high mortality rate (30% - 50%) of the juvenile snails (shell height 10 – 40 mm) of Rapana venosa. Therefore, it is of great significance to understand predation behavior mediated by chemical cues for gastropod aquaculture and resource management.
2.2. Aggregation
Inter- and intraspecies communication in gastropods via chemical cues often triggers aggregation behavior (Kirsch, 2022). Different species exhibit one or more of the three main aggregation behaviors due to their lifestyles and reproductive habits. The first of these behaviors is reproductive aggregation (Figure 1A). During the summer breeding season, R. venosa obviously aggregates when mating and spawning (Song, 2015), which may improve their reproductive capabilities. Meanwhile, the freshwater gastropod Physa fontinalis only aggregates when seeking a mate (Townsend and McCarthy, 1980). Reproductive aggregation in gastropods is related to their intraspecific chemical perception of pheromones and gastropods commonly seek the pheromones of conspecifics (Wyeth, 2019). For example, the Ilyanassa obsoleta has sex-specific responses to at least three different pheromones involved in mating and spawn aggregation (Moomjian et al., 2003). The second primary type of aggregation behavior observed in gastropods is adaptive aggregation (Figure 1B), which is an effective strategy for adapt to environmental conditions. For example, Yu et al. (2020) found that juvenile Neptunea cumingii can adapt to unsuitable environments by showing increased aggregation behavior at low (4°C) or high (22°C) temperatures. Similarly, Nerita atramentosa exhibits aggregation behavior in winter in intertidal habitat, with the body temperatures of aggregated snails being 2°C higher than those of non-aggregated individuals (Chapperon and Seuront, 2012). The final common form of gastropod aggregation behavior is predator–prey aggregation (Figure 1C). Predatory aggregation is a common behavior among gastropods (Lapointe and Sainte-Marie, 1992; Yu et al., 2020). While reproductive aggregation and adaptive aggregation are aimed at resisting adversity and enhancing reproductive capacity, predator–prey aggregation is driven by individual preferences. As its driving mechanism is different, it often results in intraspecific competition.
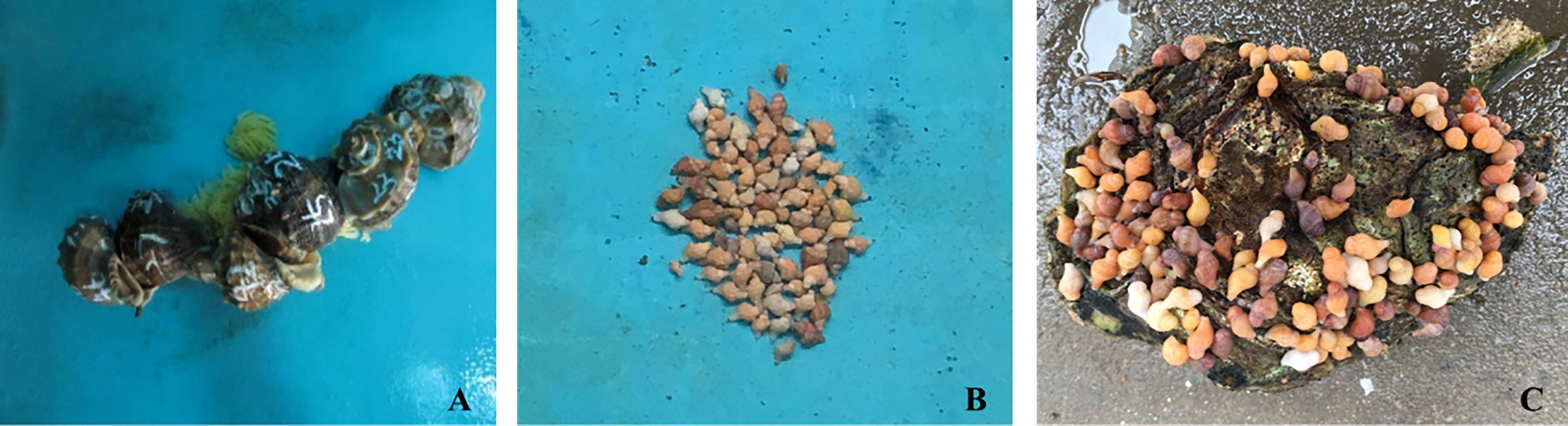
Figure 1 Gastropod aggregation behavior: (A) reproductive aggregation of Rapana venosa (Shell height 6 ± 1 cm) and (B) adaptive and (C) predator–prey aggregation of Neptunea cumingii (Shell height 1 ± 0.3 cm).
2.3. Larval settlement and metamorphosis
As highly effective chemoreception organisms, gastropods can effectively sense polysaccharides, amino acids, nucleotides, fatty acids, and volatile organic compounds in their prey (Emery, 1992; Kamio and Derby, 2017). Chemoreception plays a key role in the settlement and metamorphosis of gastropod larvae. Zhang et al. (2020) found that the presence of bivalves can induce the settlement and metamorphosis of the larvae of R. venosa. Yu et al. (2022) found that the larvae of R. venosa can sense bivalves and that only the competent larvae (shell length: 1000–1500 μm) show active settlement and metamorphosis in the presence of bivalve odors. Additionally, Ilyanassa obsoleta is dependent on its olfactory ability to locate prey, and its larvae can also detect the odors generated by prey species and trigger metamorphosis (Leise et al., 2009).
The clues that induce larvae to settle and metamorphose usually include three main biological sources of chemical cues: conspecifics, biofilms, and prey. Conspecifics may include larvae and/or adults; for example, the settlement of Crepidula fornicata, an invasive snail, is triggered by the chemical signals released by conspecifics (Taris et al., 2010). Biofilms may impact settlement and metamorphosis; for example, natural biofilms can induce the metamorphosis of Crepidula onyx larvae (Zhao and Qian, 2002). Finally, the presence of prey can induce metamorphosis. For example, the nudibranch Adalaria proxima will metamorphose in the presence of the chemical signals released by their bryozoan prey Electra pilosa; the inducer may be a peptide with a low molecular weight (<500 kda) (Bornancin et al., 2017).
3. Mechanisms of chemoreception
The chemical molecules released by targets are transported through aquatic environments and combine with the chemical receptors on the chemoreception organs of gastropods to stimulate intracellular electrical signals. These signals are then transmitted to the central nervous system to form olfaction or gustation (Krieger and Breer, 1999; Ache and Young, 2005).
3.1. Olfaction and gustation in aquatic environment
Usually, smell is regarded as a sense of distance, while taste is regarded as a sense of contact. Biomolecules less than 300 Da, which can be transported through air and bind to odor receptors, are generally considered odor molecules. Instead, taste sensing molecules must be in solution and in contact with receptors. However, when considering the aquatic environment, we should reconsider our so-called “smell” and “taste”, because species live in water, and remote chemical perception of hydrophilic substances is a simple thing (Mollo et al., 2017), and gustation can also act as a distance sense in aquatic environment. In the aquatic environment, the chemical cues transmitted by water is considered as the counterpart of the air transmitted signal and is perceived by the sense of smell. However, some typical taste molecules are thought to be perceived by the olfactory system in the aquatic environment. For example, sugar and glutamic acid cannot be regarded as olfactory molecules just because they can be perceived in dissolved form in water, because the existence of sweet and umami taste receptors has been supported by evidence (Mollo et al., 2014). Therefore, we should prefer to use the more general term “chemical perception” rather than “olfaction/gustation ”.
3.2. Chemical molecules
For gastropods, the available chemical molecules are mainly divided into two categories: inter- and intraspecific. The chemical molecules from other species (Whittaker and Feeny, 1971) drive gastropods to locate prey, avoid predators, metamorphose, and grow. In contrast, the chemical molecules — specifically pheromones — of conspecifics (Shorey, 1976) have an important impact on the reproductive and aggregation behavior of gastropods.
In addition to their function in transmitting diverse information, chemical molecules are very complex and they differ markedly in their compositions and structures. Previous studies have shown that chemical molecules, such as animal extracts, amino acids, nucleotides, fatty acids, and sulfur- and nitrogen-containing compounds can induce predatory behavior in aquatic organisms (Brönmark and Hansson, 2012; Kamio and Derby, 2017). Table 1 shows some of the chemical molecules that induce chemoreception behaviors of gastropods. The chemical molecules released by organisms are often mixtures of many different molecules. Though a single chemical molecule can cause physiological and behavioral responses in aquatic organisms, complete behaviors often require the stimulation of complex mixtures of odor molecules.
The chemical molecules that can be perceived by gastropods listed in Table 1 are water-soluble. However, Mollo et al. (2017) indicated that marine benthos can perceive insoluble chemical molecules through “touch” smell. This allows slow moving benthos to track or avoid chemical traces attached to the substrate by recognizing the concentration gradient, while professional nudibranchs can use insoluble but volatile chemical molecules to find food and mates.
3.3. Chemical receptors
The sense of smell and taste of gastropods is realized through the contact between chemical molecules and chemical receptors (Mollo et al., 2017). Aquatic invertebrates have many types of chemical receptor proteins (Derby, 2020). Olfactory receptors (ORs) are the physiological key to olfactory perception and are closely related to the ability of animals to distinguish different odors (Krieger and Breer, 1999). ORs belong to the G protein-coupled receptor (GPCR) family, and their structural characteristics are as follows: one C-terminal in the membrane, a 7-α helical transmembrane structural region, three intracellular loops, three extracellular loops, and one N-terminal outside the membrane (Tacher et al., 2005). The acceptance of water borne but non-volatile compounds that lead to the perception of salty, sweet, bitter, sour and delicious is mediated by typical taste receptors (TRs). Gustatory Receptor-Like proteins are seven-transmembrane ionic receptors. The representativeness of these receptor proteins has been identified in most animal branches, including molluscs, but disappeared in vertebrates (Derby, 2020).
The ORs of aquatic gastropods have been studied to some extent, but there is a lack of relevant research on the function of TRs. Cummins et al. (2009a) found that rhodopsin G-protein-coupled receptor-like gene subfamilies were expressed in the sensory epithelial cells of sea rabbits, which encode functional chemoreceptors. Yu et al. (2022) has identified the OR genes of R. venosa and studied the role of chemical perception in its settlement and metamorphosis. ORs have evolved in marine invertebrates at least 550 million years ago, and in cnidarian Nematostella vectensis, we can see the antiquity of ORs from their orthologs (Churcher and Taylor, 2011). From sea to land, the OR gene family changes gradually with the evolution of species. Therefore, there are significant interspecific differences between vertebrates and invertebrates in the structure of olfactory receptor proteins and the genes encoding them.
In addition, ionic receptors (IRs) evolved from ionic glutamate receptors and diversified. IRs has an ancient evolutionary function in odor detection, which may play a general role in initiating chemical sensing signals. Studies have shown that the gastropod Aplysia expresses IRs in chemoreceptors (Croset et al., 2010). Liang et al. (2016) revealed two types of receptors, including IR, in Biomphalaria glabrata and found that there were seven putative IR, some of which were expressed in its chemoreceptor organs. Since GPCR has been identified as a key receptor in olfaction, Liang et al. (2016) proposed an interesting possibility, that is, IR may also cooperate with GPCR in B. glabrata, and both of these two pathways may contribute to the output of gastropod olfactory sensory neurons.
3.4. Chemosensory organs
Behavioral studies have shown that many marine invertebrates have chemosensory organs that impact their chemoreception behavior (Kamio and Derby, 2017). Gastropods also have sensitive chemosensory organs (Table 2). Previous studies have shown that chemosensory cells are widely distributed across the body surfaces of gastropods, including the foot, siphon, osphradium, and antennae (Lindberg and Sigwart, 2015; Derby, 2020). One or two pairs of symmetrical antennae on both sides of the head, the osphradium near the mantle, and the siphon near the foot are considered to be the main chemosensory organs of gastropods, but they vary among species (Kamio and Derby, 2017; Wyeth, 2019; Derby, 2020).
Gastropod tentacles obviously lengthen and swing left and right when they are looking for food, indicating their olfactory function (Gan, 2017).The rhinophores and tentacles are the main chemoreceptive organs of gastropods (e.g., Aplysia californica) (Audesirk and Audesirk, 1977), in which the epidermal cells in the tentacles sensory area are often columnar, with abundant ciliated cells. Li et al. (2006) found that there are cilia at the tip of the tentacles of Haliotis diversicolor and the epithelial cells are composed of sertoli cells — sensory and glandular cells that have tactile and olfactory functions. The osphradium is another important chemoreceptor that sits in or near the mantle cavity of many gastropods (Lindberg and Sigwart, 2015). Chitramvong et al. (2002) determined that the osphradium has two functions: chemoreception (participating in the locating of food or detecting of odor molecules) and tactile sensation (responsible for assessing the amount of sediment entering the mantle cavity or for regulating respiration by detecting changes in seawater pH). The osphradium of neogastropods is larger and its main role is olfactory perception (Lindberg and Ponder, 2001). However, Wyeth (2019) suggested that scent detector is mainly used to regulate the behavior and physiology of organisms according to the presence (or concentration) of environmental odors but cannot control the movement of gastropods toward the source of an odor. The siphon is an important sensory organ in gastropods. Ferner and Weissburg (2005) found that when successful predators of the species B. carica moved toward the source of an odor, their siphons moved from side to side, likely to search for the odor released by their prey.
3.5. Transduction and nervous systems
The combination of stimulated chemical molecules with receptor proteins will induce the electrical reaction of chemical receptor cells. The G protein mediated transduction cascade (including G protein, second messenger such as cAMP or IP3, protein kinase) has been confirmed in many aquatic invertebrates, including gastropods (Krieger and Breer, 1999; Cummins et al., 2009a; Cummins et al., 2009b). The chemical perception of gastropods requires the involvement of the central nervous system and the peripheral nervous system. Research shows that dopamine, histamine, glutamate and oligopeptide FMRFa exist in sensory neurons of different peripheral regions of gastropods, including tentacles, lip, foot and mantle (Wyeth and Croll, 2011; Vallejo et al., 2014). Horváth et al. (2020) characterized the sensory system of the main sensory areas (tentacles and lips) and the anterior foot of aquatic gastropod Lymnaea stagnalis, paying special attention to the neurotransmitter content of sensory neurons and their relationship with the external elements of the central nervous system, and the results showed that a wider range of signal molecules participated in the peripheral sensory process of L. stagnalis, thus affecting its response to environmental cues. During development, gastropods will change their perception of environmental chemical molecules by regulating the neuroendocrine system. Yang et al. (2022) observed significant changes in the key genes in the neuroendocrine system induced by oysters in the competent larvae of R. venosa, and proposed a hypothetical model for oyster induced metamorphosis of the snails, that is, the chemical molecules released by oysters may interact with the larvae through taste or smell receptors, thus triggering changes in the neuroendocrine system, including 5-HT and 5-HT receptors, as well as NO and NOS.
4. Chemoreception targets location
Aquatic animals that rely on chemical molecules for long-distance perception are greatly affected by the fluid dynamics of their habitats. The fluid environment not only directly affects the spatial and temporal distributions of chemical molecules, but also determines the ability of organisms to orient toward the perceived chemical molecules (Vickers, 2000; Weissburg, 2000).
4.1. Potential navigation strategies for locating targets
Chemoreception navigation in aquatic environments can be achieved via three main strategies: kinesis, chemotaxis, and odor-gated rheotaxis (Wyeth, 2019). Kinesis, which is commonly found in microorganisms, is a stimulus-induced motility processes in which the direction of movement is independent of existing concentration gradients. In this case, organisms continue moving in a straight line, even when increasing concentrations are detected, and turn more frequently to move in random directions when decreasing concentrations are detected (Webster and Weissburg, 2009). Only the presence of chemical molecules is detected through kinesis, so only one sensor is required. Chemotaxis involves the movement of an organism in the direction of an increasing concentration gradient of chemical molecules. In this case, movement is mostly directly toward the source of the chemical molecules. Chemotaxis usually requires two sensors or one sensor that can move to establish the direction of a chemical gradient. Odor-gated rheotaxis involves the upstream movement of aquatic organisms in the direction of the source of an odor. Odor-gated rheotaxis requires at least one chemical sensor, with which organisms can sense the presence of water flow. In this case, organisms can usually search for and locate their prey faster than via kinesis or chemotaxis. According to the fluid velocity and flow state, chemical molecules are transmitted in the water through diffusion, laminar advection, or turbulence, and then are received by gastropods (corresponding navigation strategies), triggering the behavioral and physiological reactions of gastropods (Webster and Weissburg, 2009).
4.2. Still water
In still-water environments, the primary mode of scent transmission is molecular diffusion. The structures of scents are characterized by slow diffusion speeds and smooth concentration gradients (i.e., the concentration of an odor at the source is high and gradually declines into its surroundings). In such environments, the only signal available to aquatic organisms is the chemical molecules itself and its spatiotemporal gradient; thus, the ability to detect chemical gradients can be used to move toward or away from chemical molecules sources. Wyeth (2019) suggested that there is not enough evidence that gastropods use kinesis to locate food in still water because there are only a few studies in environments with little or no water flow, but rather gastropods may use chemotaxis to determine the direction of their prey.
4.3. Laminar flow
Laminar flow occurs at low Reynolds numbers. In still water, the speed of molecular diffusion affects the speed at which odor molecules move, so transmission is very slow. However, under laminar flow, the flow of water will cause chemical molecules to spread quickly; even if the movement is slow, it will be much faster than under diffusion alone. Under laminar flow, the structures of scents are characterized by small concentration gradients over space and time, though these are affected by variations in flow (Webster and Weissburg, 2009). Many aquatic organisms communicate with chemical molecules transported by laminar advection, including gastropods.
4.4. Turbulence
At large Reynolds numbers, turbulence is caused by large fluctuations in water flow. Under these conditions, chemical molecules are distributed chaotically in space and time, with intermittent and unpredictable characteristics. Over fast time-scales, measurements have shown that odor plumes are highly intermittent and high concentrations are interspersed with zones of low or zero concentration. As the distance from the odor source increases, the odor plume tends to spread horizontally and vertically. In turbulent environments, aquatic organisms may use the cues of intermittent or time-averaged odor plumes to locate the direction of odor sources (Vickers, 2000; Weissburg, 2000).
Many aquatic organisms, such as crayfish (Moore and Grills, 1999; Moore et al., 2015), blue crabs (Weissburg and Zimmer-Faust, 1994; Powers and Kittinger, 2002), and gastropods (Ferner and Weissburg, 2005; Yu et al., 2019b), can sense and locate their prey through odor plumes under turbulent conditions. However, different organisms use different olfactory strategies, which are affected by their sizes and speeds. Generally, large and fast-moving organisms (e.g., blue crabs) adopt spatially dependent perception strategies, while small and slow-moving creatures (e.g., gastropods) adopt time-dependent perception strategies (Kamio and Derby, 2017). For example, blue crabs can use chemical sensors to compare odor signals in space and move toward odor plumes by comparing the instantaneous concentrations obtained by different sensors. Under turbulent conditions, with increasing flow velocities, changes in the intermittent and instantaneous concentrations of odor plumes are accelerated. Due to the rapid speed of blue crabs, the perception time required to evaluate such changes is too long, making them unable to perceive trends in odor plumes, thereby reducing their ability to sense and locate prey. As slow-moving organisms, gastropods experience the rapid coincidence of odor molecules at a single location. Gastropods can average odor concentrations to accurately estimate the position of an odor plume and then move toward the odor source. This time-dependent perception strategy allows gastropods to forage in turbulent environments — a feat that remains challenging to fast-moving predators, such as blue crabs (Powers and Kittinger, 2002; Ferner and Weissburg, 2005; Moore et al., 2015; Kamio and Derby, 2017).
Gastropods may use two strategies to sense and locate prey — chemotaxis or odor-gated rheotaxis. In still water, there is a stable concentration gradient of odors and gastropods can use chemotaxis to sense the direction of a concentration gradient. Meanwhile, under laminar or turbulent flow conditions, gastropods use time-dependent perception strategies to sense and locate prey through chemotaxis or odor-gated rheotaxis.
5. Future prospects and conclusions
5.1. Future research considerations
5.1.1. Efficient trapping in gastropod fisheries
Gastropods are one of the most important economic shellfish in the world. In terms of large-scale fisheries, aquaculture techniques are well developed for the three most important gastropods — abalone (Haliotis species), trochus (Trochus niloticus), and queen conch (Strombus gigas) (Castell, 2003; Stoner and Appeldoorn, 2022). Additionally, the potential of some other gastropod fishery species has received increasing attention, including that of R. venosa (Zhang et al., 2020), N. cumingii (Zhang et al., 2018a), and B. undatum (Morrissey et al., 2022). However, due to the bottleneck of artificial seedling technology for some species (e.g., R. venosa), current resources are mainly derived from manual harvesting by divers or as by-products when recycling ground cage nets. This results in low harvesting efficiency and high costs without meeting market demands. Invasive gastropod species also present a problem in some countries, including R. venosa in the southern Black Sea (Mutlu et al., 2022) and Pomacea canaliculate in many Asian countries (Yusa et al., 2006). Thus, efficiently trapping gastropods has attracted increasing attention. The use of pheromones or species-specific baits based on chemoreception has been remarkably effective in controlling insects (Guo et al., 2020). Similarly, the use of pheromones, species-specific baits, and other substances to induce gastropod aggregation behaviors may provide new means of efficiently capturing gastropods. Although some chemical molecules have been confirmed to induce certain behaviors among gastropods (Table 1), the complex chemical molecules that induce the complete chemoreception behaviors still warrant systematic evaluation.
5.1.2. Prevention and control of gastropod predators of economic shellfish
Carnivorous gastropods prey on economic bivalves, such as clams and oysters, causing heavy economic losses (Hu et al., 2016). For example, the invasive species R. venosa is an important cause of the dramatic decline in the abundance of bivalve species in the Black Sea (Snigirov et al., 2013). Purpura gradata damages oyster farms and its predation on oyster seedlings accounts for at least 50% of all harvested seedlings (Lou, 1963). Based on the summary provided here, we know that gastropods can successfully sense and locate prey by chemoreception. In fact, measures that might affect the emission of chemical cues by prey, as well as the transportation, perception, or response of gastropods to chemical cues, can successfully prevent the destruction of shellfisheries. Controlling the direction of water flow may also assist in protection shellfisheries, as gastropods cannot catch bivalves downstream (Yu et al., 2019b). Nevertheless, research on blocking gastropod chemoreception is still needed in natural sea areas.
5.1.3. Induction of larval settlement and metamorphosis in gastropod aquaculture
Although artificial breeding technologies have been established for some gastropods, there remain many economic gastropods for which such technologies are limited. For example, extremely low rates of metamorphosis limit the development of R. venosa aquaculture. This species is a typical carnivorous snail with planktonic larvae that change their feeding habits during metamorphosis (i.e., from herbivorous to carnivorous) (Zhang et al., 2020). Although oysters can induce larvae to attach and metamorphose, the cost of oysters is high, resulting in low benefits when using this method. Yu et al. (2019a) found that oyster polysaccharides may induce metamorphosis, but the rate of metamorphosis is significantly lower than that of oyster. Therefore, identifying effective odor molecules that can improve the efficiency of larval settlement and metamorphosis is urgently needed to effectively improve the economic benefits of the artificial breeding of R. venosa.
5.1.4. Molecular mechanisms of olfactory perception in gastropods
At present, OR genes have been extensively studied in fish (Lv et al., 2019); however, few studies have been conducted on OR or TR and their related genes in gastropods. Yu et al. (2022) was only able to reveal the OR genes of R. venosa from the transcriptome level and the identification of functional genes and related functions requires further study. Additionally, the identification of gastropod ORs or TRs and the molecular mechanisms through which they recognize different odors also warrant further examination. Understanding the molecular mechanisms of gastropod chemoreception can provide a theoretical basis for efficient gastropod trapping, bivalve pest control, and larval settlement and metamorphosis.
6. Conclusions
This review introduces the chemoreception behavior of gastropods and the mechanisms underlying the perception and location of targets in the aquatic environment. Prey or conspecifics release chemical molecules into their aquatic environments and chemical molecules are transported by diffusion or water flow. Chemical molecules combine with Chemical receptors on the chemosensory organs of gastropods to form chemoreception. Gastropods use different strategies to locate food or conspecifics in different aquatic environments (i.e., chemotaxis in still water, chemotaxis or odor-gated rheotaxis in laminar and turbulent flow environments). These chemoreception processes mediate gastropod predation, aggregation, settlement, and metamorphosis behaviors (Figure 2). Based on this, we put forward the prospect of gastropod culture and fishery management in the future, hoping to provide suggestions for the rational utilization of gastropod resources and biological invasion.
Author contributions
TZ and X-TY conceived and designed the review. Z-LY conducted the review, analyzed the data, and wrote the manuscript. M-JY and HS contributed references, data, and analytical tools. All authors contributed to the article and approved the submitted version.
Funding
This study was supported by the National Natural Science Foundation of China (Grant numbers 32002374, 31972814, and 32002409), China Postdoctoral Science Foundation (Grant No. 2021M703248), the earmarked fund for CARS (CARS-49). This study was supported by the Creative Team Project of the Laboratory for Marine Ecology and Environmental Science, Qingdao National Laboratory for Marine Science and Technology (number LMEES-CTSP-2018-1).
Acknowledgments
The authors wish to thank all of their many collaborators who participated in research and discussions leading up to this review.
Conflict of interest
The authors declare that the research was conducted in the absence of any commercial or financial relationships that could be construed as a potential conflict of interest.
Publisher’s note
All claims expressed in this article are solely those of the authors and do not necessarily represent those of their affiliated organizations, or those of the publisher, the editors and the reviewers. Any product that may be evaluated in this article, or claim that may be made by its manufacturer, is not guaranteed or endorsed by the publisher.
References
Ache B. W., Young J. M. (2005). Olfaction: diverse species, conserved principles. Neuron 48 (3), 417–430. doi: 10.1016/j.neuron.2005.10.022
Audesirk T. E., Audesirk G. J. (1977). Chemoreception in Aplysia californica–II. electrophysiological evidence for detection of the odor of conspecifics. Comp. Biochem. Physiol. Part A: Physiol. 56 (3), 267–270. doi: 10.1016/0300-9629(77)90234-1
Bornancin L., Bonnard I., Mills S. C., Banaigs B. (2017). Chemical mediation as a structuring element in marine gastropod predator-prey interactions. Natural Product Rep. 34 (6), 644–676. doi: 10.1039/c6np00097e
Brönmark C., Hansson L.-A. (2012). Chemical ecology in aquatic systems (New York: Oxford University Press).
Castell L. (2003). “Marine gastropods,” in Aquaculture: farming aquatic animals and plants. Eds. Lucas J. S., Southgate P. C., Fishing New Book, 467–487.
Chapperon C., Seuront L. (2012). Keeping warm in the cold: On the thermal benefits of aggregation behaviour in an intertidal ectotherm. J. Thermal Biol. 37 (8), 640–647. doi: 10.1016/j.jtherbio.2012.08.001
Chitramvong Y., Kruatrchue M., Upatham E. S., Singhakaew S., Parkpoomkamol K. (2002). The pallial organs of Haliotis asinina linnaeus (Gastropoda : Haliotidae). ScienceAsia 28 (1), 17–23. doi: 10.2306/scienceasia1513-1874.2002.28.017
Churcher A. M., Taylor J. S. (2011). The antiquity of chordate odorant receptors is revealed by the discovery of orthologs in the cnidarian Nematostella vectensis. Genome Biol. Evol. 3, 36–43. doi: 10.1093/gbe/evq079
Croset V., Rytz R., Cummins S. F., Budd A., Brawand D., Kaessmann H., et al. (2010). Ancient protostome origin of chemosensory ionotropic glutamate receptors and the evolution of insect taste and olfaction. PloS Genet. 6 (8), e1001064. doi: 10.1371/journal.pgen.1001064
Cummins S. F., Erpenbeck D., Zou Z., Claudianos C., Moroz L. L., Nagle G. T., et al. (2009a). Candidate chemoreceptor subfamilies differentially expressed in the chemosensory organs of the mollusc Aplysia. BMC Biol. 7, 28. doi: 10.1186/1741-7007-7-28
Cummins S. F., Leblanc L., Degnan B. M., Nagle G. T. (2009b). Molecular identification of candidate chemoreceptor genes and signal transduction components in the sensory epithelium of aplysia. J. Exp. Biol. 212 (13), 2037–2044. doi: 10.1242/jeb.026427
Derby C. D. (2020). “3.04 - chemoreception in aquatic invertebrates,” in The senses: A comprehensive reference (Second edition). Ed. Fritzsch B. (Oxford: Elsevier), 65–84.
Duval M. A., Calzetta A. M., Rittschof D. (1994). Behavioral responses of Littoraria irrorata (SAY) to water-borne odors. J. Chem. Ecol. 20 (12), 3321–3334. doi: 10.1007/BF02033729
Emery D. G. (1992). Fine structure of olfactory epithelia of gastropod molluscs. Microscopy Res. Technique 22 (4), 307–324. doi: 10.1002/jemt.1070220402
Ferner M. C., Weissburg M. J. (2005). Slow-moving predatory gastropods track prey odors in fast and turbulent flow. J. Exp. Biol. 208 (5), 809–819. doi: 10.1242/jeb.01438
Fink P., Von Elert E., Jüttner F. (2006). Oxylipins from freshwater diatoms act as attractants for a benthic herbivore. Archiv für Hydrobiol. 167 (1-2), 561–574. doi: 10.1127/0003-9136/2006/0167-0561
Gan Y. (2017). Olfaction and its response under ocean acidification of Babylonia areolata (Xiamen: Xiamen University).
Göbbeler K., Klussmann-Kolb A. (2007). A comparative ultrastructural investigation of the cephalic sensory organs in opisthobranchia (Mollusca, Gastropoda). Tissue Cell 39 (6), 399–414. doi: 10.1016/j.tice.2007.07.002
Guo X. J., Yu Q. Q., Chen D. F., Wei J. N., Yang P. C., Yu J., et al. (2020). 4-vinylanisole is an aggregation pheromone in locusts. Nature 584 (7822), 584–588. doi: 10.1038/s41586-020-2610-4
Hatle J. D., Salazar B. A. (2001). Aposematic coloration of gregarious insects can delay predation by an ambush predator. Environ. Entomol. 30 (1), 51–54. doi: 10.1603/0046-225X-30.1.51
Horváth R., Battonyai I., Maász G., Schmidt J., Fekete Z. N., Elekes K. (2020). Chemical-neuroanatomical organization of peripheral sensory-efferent systems in the pond snail (Lymnaea stagnalis). Brain Structure Funct. 225 (8), 2563–2575. doi: 10.1007/s00429-020-02145-z
Humphrey J. A. C., Mellon D. J. (2007). Analytical and numerical investigation of the flow past the lateral antennular flagellum of the crayfish Procambarus clarkii. J. Exp. Biol. 210 (17), 2969–2978. doi: 10.1242/jeb.005942
Hu N., Wang F., Zhang T., Song H., Yu Z. L., Liu D. P. (2016). Prey selection and foraging behavior of the whelk Rapana venosa. Mar. Biol. 163 (11), 233. doi: 10.1007/s00227-016-3006-8
Jager J. C. (1971). A quantitative study of a chemoresponse to sugars in Lymnaea stagnalis (L.). Netherlands J. Zool. 21 (1), 1–59. doi: 10.1163/002829671X00014
Kamio M., Derby C. D. (2017). Finding food: how marine invertebrates use chemical cues to track and select food. Natural product Rep. 34 (5), 514–528. doi: 10.1039/C6NP00121A
Katie T., Mark A. F., Irene Y., Michelle M. D. (2019). Histology atlas and systematic approach to postmortem examination of the queen conch Lobatus gigas. J. Shellfish Res. 38 (1), 131–148. doi: 10.2983/035.038.0113
Kicklighter C. E., Germann M., Kamio M., Derby C. D. (2007). Molecular identification of alarm cues in the defensive secretions of the sea hare Aplysia californica. Anim. Behav. 74 (5), 1481–1492. doi: 10.1016/j.anbehav.2007.02.015
Kirsch D. R. (2022). Freshwater gastropods as an important group for studying the impact of inter- and intra-specific chemical communication on aquatic community dynamics. Aquat. Ecol. 56 (2), 361–375. doi: 10.1007/s10452-022-09961-x
Kobak J., Poznańska M., Kakareko T. (2009). Effect of attachment status and aggregation on the behaviour of the zebra mussel Dreissena polymorpha. J. Molluscan Stud. 75 (2), 119–126. doi: 10.1093/mollus/eyn046
Kotze J., Bennett N. C., Scantlebury M. (2008). The energetics of huddling in two species of mole-rat (Rodentia: Bathyergidae). Physiol. Behav. 93 (1-2), 215–221. doi: 10.1016/j.physbeh.2007.08.016
Krieger J., Breer H. (1999). Olfactory reception in invertebrates. Science 286 (5440), 720–723. doi: 10.1126/science.286.5440.720
Lapointe V., Sainte-Marie B. (1992). Currents, predators, and the aggregation of the gastropod Buccinum undatum around bait. Mar. Ecol. Prog. Ser. 85, 245–257. doi: 10.3354/meps085245
Leise E. M., Froggett S. J., Nearhoof J. E., Cahoon L. B. (2009). Diatom cultures exhibit differential effects on larval metamorphosis in the marine gastropod Ilyanassa obsoleta (Say). J. Exp. Mar. Biol. Ecol. 379 (1), 51–59. doi: 10.1016/j.jembe.2009.08.007
Liang D., Wang T., Rotgans B. A., McManus D. P., Cummins S. F. (2016). Ionotropic receptors identified within the tentacle of the freshwater snail Biomphalaria glabrata, an intermediate host of Schistosoma mansoni. PloS One 11 (6), e0156380. doi: 10.1371/journal.pone.0156380
Lindberg D. R., Ponder W. F. (2001). The influence of classification on the evolutionary interpretation of structure a re-evaluation of the evolution of the pallial cavity of gastropod molluscs. Organisms Diversity Evol. 1 (4), 273–299. doi: 10.1078/1439-6092-00025
Lindberg D. R., Sigwart J. D. (2015). What is the molluscan osphradium? a reconsideration of homology. Zool. Anzeiger - A J. Comp. Zool. 256, 14–21. doi: 10.1016/j.jcz.2015.04.001
Li N., Shi H. R., Li H. Y., Ke H. (2006). Microstructure and ultrastructure of the cephalic tentacle of Haliotis diversicolor. Acta Zool. Sin. 52 (4), 755–764. doi: 10.3321/j.issn:1000-3207.2007.04.011
Lisova E. D., Vortsepneva E. V. (2022). New data on nudibranchs rhinophore morphology and their spicule complex in Onchidoris muricata (Doridina, Gastropoda). Zool. Anzeiger 296, 58–70. doi: 10.1016/j.jcz.2021.11.003
Lou T. K. (1963). An observation on the habit of the natural enemy of oysters–—Purpura gradata Jonas. Oceanol. Et Limnol. Sin. 5 (1), 56–70.
Lv L. Y., Liang X. F., He S. (2019). Genome-wide identification and characterization of olfactory receptor genes in Chinese perch, Siniperca chuatsi. Genes 10 (2), 178. doi: 10.3390/genes10020178
Mollo E., Fontana A., Roussis V., Polese G., Amodeo P., Ghiselin M. T. (2014). Sensing marine biomolecules: smell, taste, and the evolutionary transition from aquatic to terrestrial life. Front. Chem. 2. doi: 10.3389/fchem.2014.00092
Mollo E., Garson M. J., Polese G., Amodeo P., Ghiselin M. T. (2017). Taste and smell in aquatic and terrestrial environments. Natural Product Rep. 34 (5), 496–513. doi: 10.1039/c7np00008a
Moomjian L., Nystrom S., Rittschof D. (2003). Behavioral responses of sexually active mud snails: kariomones and pheromones. J. Chem. Ecol. 29 (2), 497–501. doi: 10.1023/A:1022603728239
Moore P. A., Ferrante P. A., Bergner J. L. (2015). Chemical orientation strategies of the crayfish are influenced by the hydrodynamics of their native environment. Am. Midland Nat. 173, 17–30. doi: 10.1674/0003-0031-173.1.17
Moore P. A., Grills J. L. (1999). Chemical orientation to food by the crayfish orconectes rusticus: influence of hydrodynamics. Anim. Behav. 58 (5), 953–963. doi: 10.1006/anbe.1999.1230
Morrissey D., Goodall J., Castilho R., Cameron T. C., Taylor M. L. (2022). Population genomics reveals a single semi-continuous population of a commercially exploited marine gastropod. Fisheries Res. 254, 106418. doi: 10.1016/j.fishres.2022.106418
Mutlu E., Kideys A. E., Şahin F., Erik G., Aksu H., Erdem E., et al. (2022). Population dynamics and ecology of the invasive veined rapa whelk, Rapana venosa in the southern black Sea. Estuarine Coast. Shelf Sci. 268, 107807. doi: 10.1016/j.ecss.2022.107807
Powers S. P., Kittinger J. N. (2002). Hydrodynamic mediation of predator–prey interactions: differential patterns of prey susceptibility and predator success explained by variation in water flow. J. Exp. Mar. Biol. Ecol. 273 (2), 171–187. doi: 10.1016/S0022-0981(02)00162-4
Scheibling R. E., Lauzon-Guay J.-S. (2007). Feeding aggregations of sea stars (Asterias spp. and Henricia sanguinolenta) associated with sea urchin (Strongylocentrotus droebachiensis) grazing fronts in Nova Scotia. Mar. Biol. 151 (3), 1175–1183. doi: 10.1007/s00227-006-0562-3
Snigirov S., Medinets V., Chichkin V., Sylantyev S. (2013). Rapa whelk controls demersal community structure off zmiinyi island, black Sea. Aquat. Invasions 8 (3), 289–297. doi: 10.3391/ai.2013.8.3.05
Song J. (2015). The reproduction ethogram of rapana venosa and food preference of juveniles (Qingdao: Qingdao Agricultural University).
Stoner A. W., Appeldoorn R. S. (2022). Synthesis of research on the reproductive biology of queen conch (Aliger gigas): Toward the goals of sustainable fisheries and species conservation. Rev. Fisheries Sci. Aquaculture 30 (3), 346–390. doi: 10.1080/23308249.2021.1968789
Tacher S., Quignon P., Rimbault M., Dreano S., Andre C., Galibert F. (2005). Olfactory receptor sequence polymorphism within and between breeds of dogs. J. Hered 96 (7), 812–816. doi: 10.1093/jhered/esi113
Taris N., Comtet T., Stolba R., Lasbleiz R., Pechenik J. A., Viard F. (2010). Experimental induction of larval metamorphosis by a naturally-produced halogenated compound (dibromomethane) in the invasive mollusc Crepidula fornicata (L.). J. Exp. Mar. Biol. Ecol. 393 (1), 71–77. doi: 10.1016/j.jembe.2010.07.001
Townsend C. R., McCarthy T. K. (1980). On the defence strategy of Physa fontinalis (L.), a freshwater pulmonate snail. Oecologia 46 (1), 75–79. doi: 10.1007/BF00346969
Uhazy L. S., Tanaka R. D., MacInnis A. J. (1978). Schistosoma mansoni: identification of chemicals that attract or trap its snail vector, Biomphalaria glabrata. Science 201 (4359), 924–926. doi: 10.1126/science.684418
Vallejo D., Habib M. R., Delgado N., Vaasjo L. O., Croll R. P., Miller M. W. (2014). Localization of tyrosine hydroxylase-like immunoreactivity in the nervous systems of Biomphalaria glabrata and Biomphalaria alexandrina, intermediate hosts for schistosomiasis. J. Comp. Neurol. 522 (11), 2532–2552. doi: 10.1002/cne.23548
Vickers N. J. (2000). Mechanisms of animal navigation in odor plumes. Biol. Bull. 198 (2), 203–212. doi: 10.2307/1542524
Webster D. R., Weissburg M. J. (2009). The hydrodynamics of chemical cues among aquatic organisms. Annu. Rev. Fluid Mechanics 41 (1), 73–90. doi: 10.1146/annurev.fluid.010908.165240
Weissburg M. J. (2000). The fluid dynamical context of chemosensory behavior. Biol. Bull. 198, 188–202. doi: 10.2307/1542523
Weissburg M. J., Zimmer-Faust R. K. (1994). Odor plumes and how blue crabs use them in finding prey. J. Exp. Biol. 197, 349–375. doi: 10.1242/jeb.197.1.349
Wells H., Wells P. H., Cook P. (1990). The importance of overwinter aggregation for reproductive success of monarch butterflies (Danaus plexippus l.). J. Theor. Biol. 147 (1), 115–131. doi: 10.1016/S0022-5193(05)80255-3
Whittaker R. H., Feeny P. P. (1971). Allelochemics: chemical interactions between species. Science 171 (3973), 757–770. doi: 10.1126/science.171.3973.757
Wyeth R. C. (2019). Olfactory navigation in aquatic gastropods. J. Exp. Biol. 222 (Suppl 1), jeb185843. doi: 10.1242/jeb.185843
Wyeth R. C., Croll R. P. (2011). Peripheral sensory cells in the cephalic sensory organs of Lymnaea stagnalis. J. Comp. Neurol. 519 (10), 1894–1913. doi: 10.1002/cne.22607
Yang M.-J., Song H., Feng J., Yu Z.-L., Shi P., Liang J., et al. (2022). Symbiotic microbiome and metabolism profiles reveal the effects of induction by oysters on the metamorphosis of the carnivorous gastropod rapana venosa. Comput. Struct. Biotechnol. J. 20, 1–14. doi: 10.1016/j.csbj.2021.11.041
Yang M. J., Song H., Shi H., Liang J., Zhou C., et al. (2023). Integrated mRNA and miRNA transcriptomic analysis reveals the response of Rapana venosa to the metamorphic inducer (juvenile oysters). Computational and Structural Biotechnology Journal 21, 702–715. doi: 10.1016/j.csbj.2022.12.047
Yoder J., Hobbs H. H. III, Hazelton M. C. (2002). Aggregate protection against dehydration in adult females of the cave cricket, Hadenoecus cumberlandicus (Orthoptera, rhaphidophoridae). J. cave karst Stud. 64 (2), 140–144.
Yoshihito N. (2009). On the origin and evolution of vertebrate olfactory receptor genes: Comparative genome analysis among 23 chordate species. Genome Biol. Evol. 1, 34–44. doi: 10.1093/gbe/evp003
Yu Z. L., Feng J., Song H., Yang M. J., Hu Z., Zhou C., et al. (2022). Identification and characterization of olfactory receptor genes and olfactory perception in rapa whelk rapana venosa (Valenciennes 1846) during larval settlement and metamorphosis. Gene 825, 146403. doi: 10.1016/j.gene.2022.146403
Yu Z. L., Hu Z., Song H., Xu T., Yang M. J., Zhou C., et al. (2020). Aggregation behavior of juvenile neptunea cumingii and effects on seed production. J. Oceanol. Limnol. 38 (5), 1590–1598. doi: 10.1007/s00343-020-0042-5
Yu Z. L., Hu N., Yang M. J., Song H., Hu Z., Wang X.-L., et al. (2019b). Environmental water flow can boost foraging success of the juvenile rapa whelk Rapana venosa (Muricidae) in aquaculture tanks with still or flowing water: Indication of chemosensory foraging. Aquaculture 513, 734392. doi: 10.1016/j.aquaculture.2019.734392
Yusa Y., Sugiura N., Wada T. (2006). Predatory potential of freshwater animals on an invasive agricultural pest, the apple snail Pomacea canaliculata (Gastropoda: Ampullariidae), in southern Japan. Biol. Invasions 8 (2), 137–147. doi: 10.1007/s10530-004-1790-4
Yu Z., Yang M., Song H., Hu Z., Zhou C., Wang X., et al. (2019a). Settlement and metamorphosis of Rapana venosa (Gastropoda: Muricidae) with implications for artificial culture. J. Oceanol. Limnol. 38 (1), 249–259. doi: 10.1007/s00343-019-9107-8
Zhang S. S., Han L. W., Shi Y. P., Li X., Zhang X. M., Hou H., et al. (2018a). Two novel multi-functional peptides from meat and visceral mass of marine snail Neptunea arthritica cumingii and their activities in vitro and in vivo. Mar. Drugs 16 (12), 473. doi: 10.3390/md16120473
Zhang T., Song H., Xue D. X., Yang M. J., Yu Z. L. (2020). Biology and aquacultural techniques of veined rapa whelk (Rapana venosa) (Beijing: Science Press).
Zhang X. Y., Sukhchuluun G., Bo T. B., Chi Q. S., Yang J. J., Chen B., et al. (2018b). Huddling remodels gut microbiota to reduce energy requirements in a small mammal species during cold exposure. Microbiome 6 (1), 103. doi: 10.1186/s40168-018-0473-9
Zhao B., Qian P. Y. (2002). Larval settlement and metamorphosis in the slipper limpet Crepidula onyx (Sowerby) in response to conspecific cues and the cues from biofilm. J. Exp. Mar. Biol. Ecol. 269 (1), 39–51. doi: 10.1016/S0022-0981(01)00391-4
Keywords: chemoreception behavior, chemical perception, chemical localization, gastropod, management of shellfish fishery
Citation: Yu Z-L, Yang M-J, Song H, Zhang T and Yuan X-T (2023) Gastropod chemoreception behaviors—Mechanisms underlying the perception and location of targets and implications for shellfish fishery development in aquatic environments. Front. Mar. Sci. 9:1042962. doi: 10.3389/fmars.2022.1042962
Received: 13 September 2022; Accepted: 30 December 2022;
Published: 16 January 2023.
Edited by:
Bin Xia, Qingdao Agricultural University, ChinaReviewed by:
Dongxue Xu, Qingdao Agricultural University, ChinaGe Hongxing, Huaihai Institute of Technology, China
Ji Liu, Qingdao Agricultural University, China
Copyright © 2023 Yu, Yang, Song, Zhang and Yuan. This is an open-access article distributed under the terms of the Creative Commons Attribution License (CC BY). The use, distribution or reproduction in other forums is permitted, provided the original author(s) and the copyright owner(s) are credited and that the original publication in this journal is cited, in accordance with accepted academic practice. No use, distribution or reproduction is permitted which does not comply with these terms.
*Correspondence: Tao Zhang, tzhang@qdio.ac.cn; Xiu-Tang Yuan, xtyuan@yic.ac.cn