- 1School of Veterinary Medicine, University of Surrey, Guildford, United Kingdom
- 2Institute of Biodiversity, Animal Health and Comparative Medicine, University of Glasgow, Glasgow, United Kingdom
- 3Wellcome Centre for Integrative Parasitology, University of Glasgow, Glasgow, United Kingdom
Schistosomiasis is a parasitic disease acquired through contact with contaminated freshwater. The definitive hosts are terrestrial mammals, including humans, with some Schistosoma species crossing the animal-human boundary through zoonotic transmission. An estimated 12 million people live at risk of zoonotic schistosomiasis caused by Schistosoma japonicum and Schistosoma mekongi, largely in the World Health Organization’s Western Pacific Region and in Indonesia. Mathematical models have played a vital role in our understanding of the biology, transmission, and impact of intervention strategies, however, these have mostly focused on non-zoonotic Schistosoma species. Whilst these non-zoonotic-based models capture some aspects of zoonotic schistosomiasis transmission dynamics, the commonly-used frameworks are yet to adequately capture the complex epi-ecology of multi-host zoonotic transmission. However, overcoming these knowledge gaps goes beyond transmission dynamics modelling. To improve model utility and enhance zoonotic schistosomiasis control programmes, we highlight three pillars that we believe are vital to sustainable interventions at the implementation (community) and policy-level, and discuss the pillars in the context of a One-Health approach, recognising the interconnection between humans, animals and their shared environment. These pillars are: (1) human and animal epi-ecological understanding; (2) economic considerations (such as treatment costs and animal losses); and (3) sociological understanding, including inter- and intra-human and animal interactions. These pillars must be built on a strong foundation of trust, support and commitment of stakeholders and involved institutions.
Introduction
Neglected Tropical Diseases (NTDs) predominantly affect communities in low- and middle-income countries and impose a significant human, economic and social burden, thus perpetuating a cycle of poverty. Schistosomiasis is caused by infection with parasitic worms of the genus Schistosoma. An estimated 240 million people are infected1 and the disease is classified by the World Health Organization (WHO) as an NTD. The main Schistosoma species responsible for human disease are Schistosoma haematobium, causing urogenital disease, and S. mansoni, S. japonicum, and S. mekongi causing intestinal disease. They differ in geographical distribution and host species they infect. Schistosoma haematobium and S. mansoni mainly infect humans as the definitive host. Schistosoma japonicum (found in the Philippines, China and Indonesia), and S. mekongi (Cambodia and Laos) use human and non-human mammals as definitive hosts, driving zoonotic transmission. Over 12 million people are estimated at risk of zoonotic infection in Asia with three million requiring treatment. Though vital to transmission, the number of animals at risk is generally not reported2 (1). The life-cycle of zoonotic schistosomes is maintained by human and animal contact with contaminated freshwater sources, where the intermediate hosts (species-specific freshwater snails) are present, and where the access to safe water and sanitation is limited (Figure 1). Infections can occur through recreational, habitual and employment activities of humans, and watering or grazing of animals. Human intestinal schistosomiasis symptoms range from abdominal pain, diarrhoea, blood in the stool, to liver and spleen enlargement, cancers and death (2). There is a dearth of data regarding the clinical impact of S. japonicum and S. mekongi in animals.
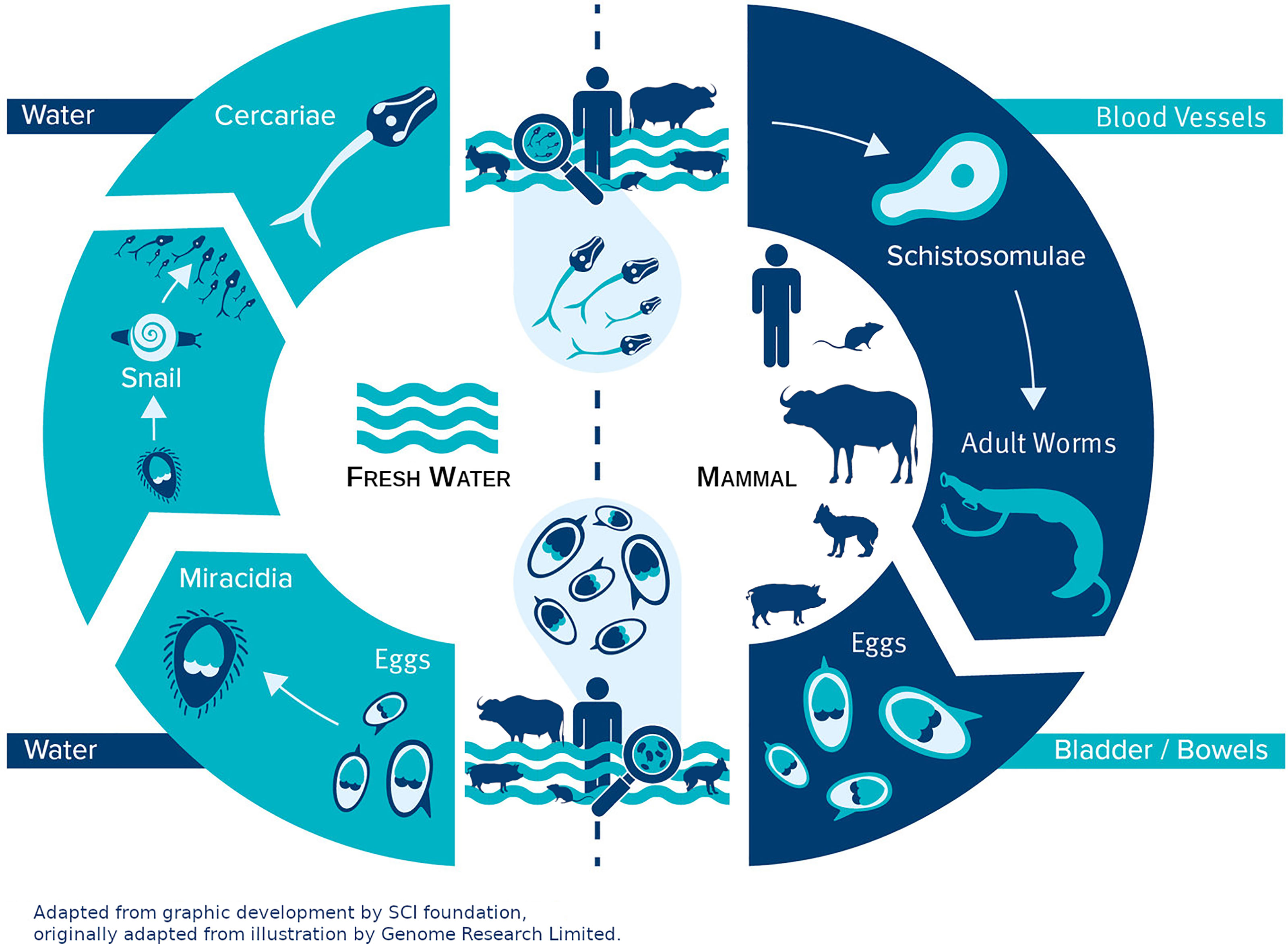
Figure 1 Life cycle of S. japonicum and S. mekongi. An infected definitive host, a mammal, passes schistosome eggs through the faeces into freshwater. The eggs may hatch into free-swimming larval miracidia that infect intermediate hosts, freshwater snails. After multiplication and development, snails shed free-swimming cercariae daily. The cercariae penetrate the skin of a mammal which comes in contact with water. Within the mammal, the cercariae shed their forked tail to form schistosomulae which mature and become worms. Paired male and female adult worms copulate and migrate to the mesenteric venules of the bowel and/or rectum where they lay thousands of eggs a day. Some of the eggs get back into the water through faeces and start the life cycle again, while some eggs get trapped in the organs causing disease symptoms.
In January 2021, schistosomiasis was targeted for elimination as a public-health problem (EPHP) globally in the WHO’s Road map for neglected tropical diseases 2021-2030 (3). This is achieved when the proportion of heavy intensity infections (over 400 eggs per gram of stool by Kato-Katz diagnostic) is reduced below 1% (3). The cornerstone of schistosomiasis control in endemic areas is preventive chemotherapy with the anthelmintic praziquantel. However, drug treatment alone is likely insufficient to reach elimination goals since only a few infected individuals can maintain the transmission cycle (4). Significant gains towards S. japonicum elimination have been achieved with integrated intervention approaches (including preventive and selective chemotherapy, mollusciciding, health-education, sanitation and environmental improvement) in countries such as China. Although transmission was interrupted in some provinces for over 10 years, other provinces remained endemic, and re-emergence has recently been observed (1, 5–7). Progress towards elimination seems to be slowing, with transmission still ongoing in many regions, and zoonotic schistosomiasis remains a public-health problem, particularly in the Philippines (8).
Efforts have been largely concentrated on the more common non-zoonotic schistosomiasis. Data needs have been reviewed for various NTDs (9) and models have been successfully used to inform control strategies (10, 11). Nevertheless, zoonotic schistosomes pose a unique challenge to achieving EPHP as multiple definitive host species contribute to transmission dynamics (12). The complexity of zoonotic schistosomiasis must be captured by these models if they are to continue playing a major role in public-health policy. Data on animal reservoirs need to be collected along with data on humans to adequately calibrate the models. Historical and current modelling approaches to capture the dynamics of schistosomes and zoonotic transmission are reviewed elsewhere (8). Here we discuss the challenges of collecting and analysing data and developing models to inform control programmes, and how a One-Health approach, recognising the interconnection between people, animals, and their shared environment (13), can improve them. Our vision builds on three cross-cutting pillars that are vital to sustainable public-health policy: (1) understanding epidemiology; (2) economic considerations; and (3) accounting for sociological aspects. All pillars must stand on a strong foundation of trust and support, requiring involvement and commitment of stakeholders and other involved institutions (14). We illustrate the three pillars framework for building a sustainable control programme in the context of zoonotic schistosomiasis EPHP, where animal reservoirs’ contribution to transmission accompanies all pillars. Nevertheless, this framework can be applied to improve public-health policy across diseases.
Pillar I: Epidemiology
This first pillar encompasses our understanding of the disease in its geographical context, including environmental drivers, host heterogeneity, parasite interactions and intervention efficacy, derived from a combination of epidemiological data (parasitological, surveys, etc.) and analyses. Prior to data collection, mathematical modelling can inform on optimal study sample sizes, sampling frequencies, and target participant groups necessary to accurately estimate parameters (15). Next, in collaboration with stakeholders, optimal and accepted epidemiological and biological data collection can be undertaken (16–18). To complete the understanding of disease transmission dynamics and to predict impacts of interventions, mathematical models calibrated to, and informed by, available data provide quantitative evidence, capable of informing both clinical trials and the final implementation of large-scale interventions, thus making these critical aspects of disease control more ethical and effective in the long-term. Model parameters and assumptions are based on field data, guided by stakeholder knowledge, in turn determining model quality (9).
Mathematical models have informed intervention programmes and played a vital role in informing the WHO’s roadmap for NTDs 2021-2030 (11, 19). Model development and the coordination of necessary data collection should be conducted in consultation with stakeholders, who are ideally placed to understand and advise on local situations, expectations of modelled outputs, and optimal methods for data collection (14). Ensuring that modelling results are interpreted correctly, and that strengths and limitations of the models are recognised, requires communication at several structural levels. Introductory and follow-up meetings with institutions involved in policy decision-making, and discussions with stakeholders at community, district and national level (14) will result in the strongest interventions. Analyses focused exclusively on this first pillar can identify the most effective strategies to achieve a target health outcome and determine timelines for achieving them (20–23).
It is widely acknowledged that to provide relevant insights, data quantity and quality needs to be improved (24). Specific data needs for some NTDs, including schistosomiasis, are reviewed elsewhere (9). The need for community-wide data is recognised, in particular, age-infection intensity profiles from adults – hindered because most studies focus on school-aged children. Low sensitivity and questionable specificities of available diagnostic tools (e.g. Kato-Katz and antigen-based diagnostics) also pose a challenge to data quality (25, 26). This becomes a greater problem as infection intensities are reduced after decades of preventive chemotherapy, further reducing the proportion of infections detected when egg-based diagnostics are the norm. Regarding interventions, if not applied yet, the lack of data can be overcome by making suggestions either based on data from different locations, or based on current interventions and expertise assumptions from stakeholders increasing uncertainty of modelled results. Many infection and population characteristics which influence disease transmission, such as present prevalence, age-intensity distributions, community- and age-contact rates and transmission rates, vary spatially. Therefore, studies mapping the spatial distribution in endemic areas need to be performed (27–32). Furthermore, some biological aspects of infection and hosts cannot be, or are difficult to be, measured. For example, it is still not known what the dominant mechanism is behind age-intensity distribution – age-dependent water contact rate, acquired immunity, worm density dependence, concomitant immunity or (likely) some combination. These unknown aspects must be further explored (33–37).
Zoonotic transmission makes data collection and analyses more complicated. When human and animal data are collected independently, it becomes harder to consolidate and unify, hindering calibration and evaluation of multiple-host models. As highlighted by the WHO, providing centralised data access will facilitate understanding, expediting analyses and consequently the decision-making process (3). An example of this is the Pan-African Rabies Control Network, which established a platform for centralised rabies data collection and analysis, with an option for open data sharing (38, 39). We see opportunity in extending such a framework to other zoonotic diseases across a wider geographic area. Mapping the spatial distribution can help to identify locations and groups where efforts should be concentrated to prevent infection, improve interventions and increase coordinated communication across veterinary and human health teams (40).
In communities where zoonotic schistosomiasis is endemic, livestock can be a major asset, residing closely with owners. However, this also fosters an environment where domestic animals can be responsible for a considerable amount of transmission to humans (4, 41). Infection dynamics in these systems with multiple hosts differ significantly from single-host systems, and are more complex to model with greater data requirements. Transmission rates vary across definitive host species, which can be due to differing behaviour like water contact, driving diversity in exposure and contamination rates. Similarly, each definitive host species will have different epidemiological characteristics in terms of recovery, birth, and mortalityrates. Capturing definitive host heterogeneities in disease dynamic processes translates to more accurately predicting higher prevalences and intensities of infection (42, 43). Additionally, estimating the contribution of each definitive host species to parasite transmission is vital (13, 19).
The best combination of interventions is expected to vary spatially according to animal host species’ densities. For example, in China, attention had initially been exclusively on bovines, because historically, most of the research was conducted in lake areas where bovines were ubiquitous (41, 44–46). It is known today that rodent species are the main animal hosts of S. japonicum in mountainous provinces, whilst bovines drive infection around the lakes (4, 47–49). To understand how to manage multi-host zoonotic systems, it is crucial to determine prevalence in animals and identify location-specific dominant animal reservoirs (50). Quantifying each host’s contribution to transmission enables identification of maintenance and essential hosts, and the predicted impacts of control strategies targeting these hosts (51–55). Human-only treatment for schistosomaisis is insufficient to achieve EPHP in some settings because transmission is maintained by untreated reservoirs. Alternatively, interventions focused on the main reservoir predict success in reducing transmission to humans (4, 46, 49). Other work has explored the possible impact of a range of animal- and environment-focused controls (46, 55, 56). Different modelling approaches for the dynamics of intestinal helminths, including schistosomes and zoonotic transmission are reviewed elsewhere (8).
Pillar II: Economics
The second pillar accounts for the economic implications of, in this case, different interventions to achieve EPHP. Primarily, a centralised data collection and analysis platform similar to that described above could reduce costs, as similar data needs across NTDs facilitate cross-cutting data collection and treatment activities (9). Furthermore, for an effective intervention strategy to be feasible, it must be affordable to individuals, governments and/or donors in addition to biologically effective. This includes the generally high upfront start-up investment, as well as recurrent maintenance costs. A useful economic evaluation approach is cost-effectiveness analysis, where costs and non-monetary health effects of different control interventions can be compared (57). Results are expressed as additional costs per unit of improved health outcome, such as reduction in transmission rate, prevalence/incidence, or deaths (58). The communication of results to policy-makers is key, as sometimes the most effective intervention for reducing disease might not be cost-effective (14). Cost-effective analysis has already been used for numerous NTDs and can be integrated into detailed infection transmission models. In the context of zoonotic diseases such as S. japonicum and S. mekongi, it should be leveraged to explore the costs and effectiveness of animal-based and other combined interventions (59). The costs to be considered include expected resources used for implementing and eventually maintaining an intervention, including the net savings to patients and healthcare providers due to reducing the disease burden (57). Savings comprise out-of-pocket and health system expenses, travel costs of care-seeking and opportunity costs of ill health, such as reduced patient productivity or school and work attendance, which are unfortunately often overlooked. In zoonotic schistosomiasis, additional costs are incurred due to animal death or illness, which adds time and cost to replace animals, as well as reduced livestock productivity (60).
One challenge remains regarding the appropriate metric to use for health outcomes that is generalizable. Disability-adjusted life-years (DALYs) are widely used to measure disease burden, with one DALY representing the loss of one life-year lived in optimal health, thereby translating both disease mortality (the years of life lost, YLL), and morbidity (the years lived with disability, YLD) into a single metric (61, 62). This enables comparison across studies, settings, and interventions targeting the same or different diseases (58). However, DALYs face some limitations, as they frequently disregard the infection-associated mental health burden or the need to adjust for co-morbidities. To estimate morbidity, DALYs rely on general estimates of disability weights – most of them estimated by an expert medical panel, instead of a preference-based valuation method, raising universality concerns (63). This leads to the health impacts of infection being underestimated. Lastly, DALYs are unsuitable when evaluating zoonotic diseases because they disregard effectiveness resulting from improving animals’ and owners’ quality of life and well-being due to averted animal morbidity/mortality. Efforts have been made to quantify the zoonosis burden on humans and animals simultaneously – zoonosis disability-adjusted life-years (zDALYs) (64). These include an additional component called animal loss equivalents, which converts expected livestock production and local per capital income losses to the equivalent number of human YLD. Nevertheless, this metric has been rarely used in cost-effectiveness analyses of zoonotic diseases (65, 66), and only once for schistosomiasis (67).
Most economic evaluations of schistosomiasis interventions have focused on chemotherapy with praziquantel (68–70). The WHO NTD roadmap has highlighted the benefits, including financial, of cross-cutting interventions (3). However, the use of different effectiveness measures (i.e. health outcomes) for evaluation of new control interventions hampers comparison within and between NTDs (71, 72). Standardising the use of a common metric across economic analyses will enable cost-effectiveness comparisons across multiple NTDs and beyond. Such metrics should be extendable, as appropriate to zoonotic diseases. Programmes that consider the first and second pillars together are more sustainable, as knowledge of the most effective interventions from an epidemiological perspective can be supplemented by evaluations of their economic impact and the time horizon for cost benefits.
Pillar III: Sociology
The third pillar acknowledges the impact of human behaviour on intervention outcomes. This is most commonly considered in the context of adherence, referring to how individuals interact with a given intervention. For example, when offered medication, whether a person will ingest it or not, in the context of animal treatment, whether an owner gives the drug to the animal – an important differentiation between treatment coverage and treatment compliance (36, 73). If enough individuals do not adhere to control measures, effectiveness at population levels can be lower than predicted. This can lead to failure in meeting targets like EPHP and/or increases in intervention costs, which could change the cost-effectiveness of the programme. Indeed, numerous studies across diseases (not just NTDs) suggest that it is not realistic to expect full compliance with any control measure (74–78). Intervention strategies can be better informed when models explicitly account for inconsistent adherers (36, 79).
Assessing the impact of novel interventions in a given location can be challenging when the social determinants of participation are unknown. The WHO has suggested that Water, Sanitation, and Hygiene (WaSH) methods should be incorporated into NTD control programmes (3). This will be crucial in the context of zoonotic schistosomiasis where efficacy of WaSH interventions can be strongly affected by non-compliance because very few individuals (human or animal) are required to maintain transmission. Modelling the impacts of WaSH interventions is not novel for non-NTDs (80–82) or NTDs (83). Existing studies focused on WaSH interventions to control schistosomiasis have been reviewed (84), and new studies have been performed (85) or are currently ongoing (86). Interventions that include participatory processes are more likely to be successful as people are more interested and perceive responsibility/ownership for the outcomes of the control programmes (3, 87, 88). Pre-intervention consultations with communities may help identify challenges in intervention design that can be addressed. For example, lack of clarity of ownership and maintenance obligations were reported as reasons to limit or halt usage of unmaintained boreholes (89). Quantification of preferences for different WaSH interventions can be obtained using discrete choice experiments (DCE) which enable the comparison of preferences for WaSH and health-education interventions, considering monetary and non-monetary costs (90). Health-education interventions can help people understand the transmission cycle, including animal contribution, and increase their perception of the disease threat, thus improving the adherence to WaSH and other interventions (90). Interventions informed by DCEs in areas endemic for zoonotic schistosomiasis will need to include preferences for animal-related interventions. The outcome of a strategy built on these pillars will provide more robust, sustainable policy recommendations.
Discussion
Sustainability has been discussed in many fields, and always encompasses three pillars, environmental, economic, and social. Here, we have linked these three pillars in the context of NTDs, for the purpose of informing sustainable global-health policy. This is of particular importance for zoonotic NTDs (e.g. schistosomiasis caused by S. japonicum and S. mekongi), where the interconnection between people, animals, and their shared environment should be recognised – emphasising the importance of a One-Health approach. For the first pillar, epidemiology, we focus on definitive host heterogeneity and quantifying their contribution to overall transmission, enabling us to inform optimal intervention strategies and timelines to programmatic targets. Epidemiological understanding will be strengthened through access to improved cross-disease data. The second pillar, economics, can inform interventions by accounting for costs to all stakeholders involved and the time horizon for cost benefits. Challenges remain in establishing a standardised metric for cost-effectiveness evaluation that is appropriate for zoonotic diseases. The third, and last, pillar, sociology, considers the impact of human behaviour – particularly adherence – on programmatic success. A strong foundation of stakeholder engagement and commitment is needed from scientists across disciplines, politicians, and public-health experts. Only by combining these three pillars and commitment of all involved, can the best strategies to achieve the desired target health outcomes be developed, whilst considering costs, that will be championed by relevant stakeholders approved by local communities. The modelling community may use these recommendations to make the model predictions more accurate, assisting decision-makers to design sustainable control programmes to reach the WHO’s ambitious 2030 goal.
Author Contributions
EJ and JP conceived the study. EJ, JC, and OK wrote the original draft. All authors critically reviewed and edited the manuscript. JP provided overall supervision of the project.
Funding
This work was supported by the European Research Council (starting grant SCHISTO_PERSIST_680088 awarded to PL, supporting JC); Wellcome Trust (grant 204820/Z/16/Z awarded to PL, supporting JC); the Engineering and Physical Sciences Research Council (grant EP/T003618/1 to PL, JP, supporting EJ and SA.); the Drugs for Neglected Diseases Initiative (grant awarded to PL, supporting JC); the Medical Research Council (grant MR/P025447/1 to PL, supporting JC) and the Newton Fund awarded through the Medical Research Council (MRC; grant number MR/R025592/1 to MB, JP, supporting OK).
Conflict of Interest
The authors declare that the research was conducted in the absence of any commercial or financial relationships that could be construed as a potential conflict of interest.
Publisher’s Note
All claims expressed in this article are solely those of the authors and do not necessarily represent those of their affiliated organizations, or those of the publisher, the editors and the reviewers. Any product that may be evaluated in this article, or claim that may be made by its manufacturer, is not guaranteed or endorsed by the publisher.
Footnotes
- ^ Who.int. 2021. Schistosomiasis. [online] Available at: https://www.who.int/news-room/fact-sheets/detail/schistosomiasis [Accessed 27 November 2021].
- ^ Who.int. 2021. Preventive chemotherapy (PC) data portal [online] Available at: https://www.who.int/ Global Health Observatory [Accessed 27 November 2021].
References
1. Gordon CA, Kurscheid J, Williams GM, Clements ACA, Li Y, Zhou XN, et al. Asian Schistosomiasis: Current Status and Prospects for Control Leading to Elimination. Trop Med Infect Dis (2019) 4:40. doi: 10.3390/tropicalmed4010040
2. Gray DJ, Ross AG, Li YS, McManus DP. Diagnosis and Management of Schistosomiasis. BMJ (2011) 342:d2651. doi: 10.1136/bmj.d2651
3. WHO. Ending the Neglect to Attain the Sustainable Development Goals: A Road Map for Neglected Tropical Diseases. Geneva: World Health Organization (2020). pp. 2021–30.
4. Rudge JW, Webster JP, Lu DB, Wang TP, Fang GR, Basáñez MG. Identifying Host Species Driving Transmission of Schistosomiasis Japonica, a Multihost Parasite System, in China. PNAS (2013) 110:11457–62. doi: 10.1073/pnas.1221509110
5. Xiang J, Chen H, Ishikawa H. A Mathematical Model for the Transmission of Schistosoma Japonicum in Consideration of Seasonal Water Level Fluctuations of Poyang Lake in Jiangxi, China. Parasitol Int (2013) 62:118–26. doi: 10.1016/j.parint.2012.10.004
6. Zhu HR, Liu L, Zhou XN, Yang GJ. Ecological Model to Predict Potential Habitats of Oncomelania Hupensis, the Intermediate Host of Schistosoma Japonicum in the Mountainous Regions, China. PloS Negl Trop Dis (2015) 9:e0004028. doi: 10.1371/journal.pntd.0004028
7. World Health Organization and others. Expert Consultation to Accelerate Elimination of Asian Schistosomiasis, Shanghai, China, 22-23 May 2017: Meeting Report. Tech. Rep. Manila:WHO Regional Office for the Western Pacific (2017).
8. Betson M, Alonte AJI, Ancog RC, Aquino AMO, Belizario VY, Bordado AMD, et al. Chapter Two - Zoonotic Transmission of Intestinal Helminths in Southeast Asia: Implications for Control and Elimination. In: Rollinson D, Stothard R, editors. Advances in Parasitology, vol. 108. London: Academic Press (2020). p. 47–131. doi: 10.1016/bs.apar.2020.01.036
9. Toor J, Hamley JID, Fronterre C, Castaño MS, Chapman LAC, Coffeng LE, et al. Strengthening Data Collection for Neglected Tropical Diseases: What Data Are Needed for Models to Better Inform Tailored Intervention Programmes? PloS Negl Trop Dis (2021) 15:e0009351. doi: 10.1371/journal.pntd.0009351
10. Consortium NM, of Control of Neglected Tropical Diseases WD. Achieving NTD Control, Elimination and Eradication Targets Post-2020 Modelling Perspectives and Priorities. Gates Open Res (2019) 3:1664. doi: 10.21955/gatesopenres.1116563.1
11. Clark J, Stolk WA, Basáñez MG, Coffeng LE, Cucunubá ZM, Dixon MA, et al. How Modelling can Help Steer the Course Set by the World Health Organization 2021-2030 Roadmap on Neglected Tropical Diseases. Gates Open Res (2021) 5:112. doi: 10.12688/gatesopenres.13327.1
12. Webster JP, Borlase A, Rudge JW. Who Acquires Infection From Whom and How? Disentangling Multi-Host and Multi-Mode Transmission Dynamics in the ‘Elimination’ Era. Philos Trans R Soc B: Biol Sci (2017) 372:20160091. doi: 10.1098/rstb.2016.0091
13. Laing G, Vigilato MAN, Cleaveland S, Thumbi SM, Blumberg L, Salahuddin N, et al. One Health for Neglected Tropical Diseases. Trans R Soc Trop Med Hyg (2021) 115:182–4. doi: 10.1093/trstmh/traa117
14. Behrend MR, Basáñez MG, Hamley JID, Porco TC, Stolk WA, Walker M, et al. Modelling for Policy: The Five Principles of the Neglected Tropical Diseases Modelling Consortium. PloS Negl Trop Dis (2020) 14:e0008033. doi: 10.1371/journal.pntd.0008033
15. Pinsent A, Hollingsworth TD. Optimising Sampling Regimes and Data Collection to Inform Surveillance for Trachoma Control. PloS Negl Trop Dis (2018) 12:e0006531. doi: 10.1371/journal.pntd.0006531
16. Yang J, Zhou J, Jin J, Sun Q. The Stakeholders’ Views on Planting Trees to Control Schistosomiasis in China. Int J Environ Res Public Health (2020) 17:939. doi: 10.3390/ijerph17030939
17. Musuva RM, Odiere MR, Mwinzi PNM, Omondi IO, Rawago FO, Matendechero SH, et al. Unprotected Water Sources and Low Latrine Coverage Are Contributing Factors to Persistent Hotspots for Schistosomiasis in Western Kenya. PloS One (2021) 16:e0253115. doi: 10.1371/journal.pone.0253115
18. Dalisay SNM, Belizario VY, Joe JAS, Lumangaya CR, Cruz RD. Critical Medical Ecology and Intersectionality Perspectives in Schistosomiasis Prevention and Control in Selected Communities in Mindanao, the Philippines. J Biosoc Sci (2022) 1–20. doi: 10.1017/S0021932021000766
19. NTD Modelling Consortium Schistosomiasis Group. Insights From Quantitative and Mathematical Modelling on the Proposed WHO 2030 Goal for Schistosomiasis. Gates Open Res (2019) 3:1517. doi: 10.12688/gatesopenres.13052.2
20. Farrell SH, Coffeng LE, Truscott JE, Werkman M, Toor J, de Vlas SJ, et al. Investigating the Effectiveness of Current and Modified World Health Organization Guidelines for the Control of Soil-Transmitted Helminth Infections. Clin Infect Dis (2018) 66:S253–9. doi: 10.1093/cid/ciy002
21. Toor J, Alsallaq R, Truscott JE, Turner HC, Werkman M, Gurarie D, et al. Are We on Our Way to Achieving the 2020 Goals for Schistosomiasis Morbidity Control Using Current World Health Organization Guidelines? Clin Infect Dis (2018) 66:S245–52. doi: 10.1093/cid/ciy001
22. Kura K, Ayabina D, Toor J, Hollingsworth TD, Anderson RM. Disruptions to Schistosomiasis Programmes Due to COVID-19: An Analysis of Potential Impact and Mitigation Strategies. Trans R Soc Trop Med Hyg (2021) 115:236–44. doi: 10.1093/trstmh/traa202
23. Toor J, Adams ER, Aliee M, Amoah B, Anderson RM, Ayabina D, et al. Predicted Impact of COVID-19 on Neglected Tropical Disease Programs and the Opportunity for Innovation. Clin Infect Dis (2021) 72:1463–6. doi: 10.1093/cid/ciaa933
24. Hollingsworth TD. Counting Down the 2020 Goals for 9 Neglected Tropical Diseases: What Have We Learned From Quantitative Analysis and Transmission Modeling? Clin Infect Dis (2018) 66:S237–44. doi: 10.1093/cid/ciy284
25. Lamberton PHL, Kabatereine NB, Oguttu DW, Fenwick A, Webster JP. Sensitivity and Specificity of Multiple Kato-Katz Thick Smears and a Circulating Cathodic Antigen Test for Schistosoma Mansoni Diagnosis Pre- and Post-Repeated-Praziquantel Treatment. PloS Negl Trop Dis (2014) 8:e3139. doi: 10.1371/journal.pntd.0003139
26. Clark J, Moses A, Nankasi A, Faust CL, Moses A, Ajambo D, et al. Reconciling Egg- and Antigen-Based Estimates of Schistosoma Mansoni Clearance and Reinfection: A Modeling Study. Clin Infect Dis (2021) ciab679:1–7. doi: 10.1093/cid/ciab679
27. Clements ACA, Lwambo NJS, Blair L, Nyandindi U, Kaatano G, Kinung’hi S, et al. Bayesian Spatial Analysis and Disease Mapping: Tools to Enhance Planning and Implementation of a Schistosomiasis Control Programme in Tanzania. Trop Med Int Health (2006) 11:490–503. doi: 10.1111/j.1365-3156.2006.01594.x
28. Gomes de Amorim Santos I, Santos Ramos RE, Soares Gomes D, Pereira Bezerra L, Oliveira Silva L, Martins Cirilo T, et al. Analysis and Spatial Distribution of Schistosomiasis Mansoni in a Historically Endemic Area of Northeastern Brazil. Trop Med Int Health (2020) 25:1085–92. doi: 10.1111/tmi.13458
29. Adekeye TA, Onile OS, Adebayo AS, Osundiran AJ, Anumudu CI, Awobode HO. Spatial Distribution and Ecology of Soil Parasites and Schistosoma Haematobium Infection in Eggua, Nigeria. Environ Adv (2021) 5:100080. doi: 10.1016/j.envadv.2021.100080
30. Amoah B, Fronterre C, Johnson O, Dejene M, Seife F, Negussu N, et al. Model-Based Geostatistics Enables More Precise Estimates of Neglected Tropical-Disease Prevalence in Elimination Settings: Mapping Trachoma Prevalence in Ethiopia. Int J Epidemiol (2021) dyab227:1–11. doi: 10.1093/ije/dyab227
31. Giorgi E, Fronterrè C, Macharia PM, Alegana VA, Snow RW, Diggle PJ. Model Building and Assessment of the Impact of Covariates for Disease Prevalence Mapping in Low-Resource Settings: To Explain and to Predict. J R Soc Interface (2021) 18:20210104. doi: 10.1098/rsif.2021.0104
32. Johnson O, Fronterre C, Amoah B, Montresor A, Giorgi E, Midzi N, et al. Model-Based Geostatistical Methods Enable Efficient Design and Analysis of Prevalence Surveys for Soil-Transmitted Helminth Infection and Other Neglected Tropical Diseases. Clin Infect Dis (2021) 72:S172–9. doi: 10.1093/cid/ciab192
33. Warren KS. Regulation of the Prevalence and Intensity of Schistosomiasis in Man: Immunology or Ecology? J Infect Dis (1973) 127:595–609. doi: 10.1093/infdis/127.5.595
34. Chan MS, Guyatt HL, Bundy D, Booth M, Fulford AJC, Medley GF. The Development of an Age Structured Model for Schistosomiasis Transmission Dynamics and Control and Its Validation for Schistosoma Mansoni. Epidemiol Infect (1995) 115:325–44. doi: 10.1017/S0950268800058453
35. Buck JC, De Leo GA, Sokolow SH. Concomitant Immunity and Worm Senescence may Drive Schistosomiasis Epidemiological Patterns: An Eco-Evolutionary Perspective. Front Immunol (2020) 11:160. doi: 10.3389/fimmu.2020.00160
36. Graham M, Ayabina D, Lucas TC, Collyer BS, Medley GF, Hollingsworth TD, et al. SCHISTOX: An Individual Based Model for the Epidemiology and Control of Schistosomiasis. Infect Dis Modell (2021) 6:438–47. doi: 10.1016/j.idm.2021.01.010
37. Kura K, Hardwick RJ, Truscott JE, Anderson RM. What Is the Impact of Acquired Immunity on the Transmission of Schistosomiasis and the Efficacy of Current and Planned Mass Drug Administration Programmes? PloS Negl Trop Dis (2021) 15:e0009946. doi: 10.1371/journal.pntd.0009946
38. Scott TP, Coetzer A, de Balogh K, Wright N, Nel LH. The Pan-African Rabies Control Network (PARACON): A Unified Approach to Eliminating Canine Rabies in Africa. Antiviral Res (2015) 124:93–100. doi: 10.1016/j.antiviral.2015.10.002
39. Haselbeck AH, Rietmann S, Tadesse BT, Kling K, Kaschubat-Dieudonné ME, Marks F, et al. Challenges to the Fight Against Rabies—The Landscape of Policy and Prevention Strategies in Africa. Int J Environ Res Public Health (2021) 18:1736. doi: 10.3390/ijerph18041736
40. Menghistu HT, Mersha TT, Shumuye NA, Woldie BM, Redda YT, Hadush B, et al. Neglected Tropical Zoonotic Diseases in Tigray Region, Northern Ethiopia: Spatial Distribution and Trend Analysis of Rabies, Tuberculosis, Schistosomiasis, and Visceral Leishmaniasis in Humans. Zoonoses Public Health (2021) 68:823–33. doi: 10.1111/zph.12874
41. Guo J, Li Y, Gray D, Ning A, Hu G, Chen H, et al. A Drug-Based Intervention Study On The Importance Of Buffaloes For Human Schistosoma Japonicum Infection Around Poyang Lake, People’s Republic Of China. Am J Trop Med Hyg (2006) 74:335–41. doi: 10.4269/ajtmh.2006.74.335
42. Barbour AD. Modelling the Transmission of Schistosomiasis: An Introductory View. Am J Trop Med Hyg (1996) 55:135–43. doi: 10.4269/ajtmh.1996.55.135
43. Mari L, Ciddio M, Casagrandi R, Perez-Saez J, Bertuzzo E, Rinaldo A, et al. Heterogeneity in Schistosomiasis Transmission Dynamics. J Theor Biol (2017) 432:87–99. doi: 10.1016/j.jtbi.2017.08.015
44. Williams GM, Sleigh AC, Li Y, Feng Z, Davis GM, Chen H, et al. Mathematical Modelling of Schistosomiasis Japonica: Comparison of Control Strategies in the People’s Republic of China. Acta Trop (2002) 82:253–62. doi: 10.1016/S0001-706X(02)00017-7
45. Gao SJ, Cao HH, He YY, Liu YJ, Zhang XY, Yang GJ, et al. The Basic Reproductive Ratio of Barbour’s Two-Host Schistosomiasis Model With Seasonal Fluctuations. Parasites Vectors (2017) 10:42. doi: 10.1186/s13071-017-1983-1
46. Williams GM, Li YS, Gray DJ, Zhao Z-Y, Harn DA, Shollenberger LM, et al. Field Testing Integrated Interventions for Schistosomiasis Elimination in the People’s Republic of China: Outcomes of a Multifactorial Cluster-Randomized Controlled Trial. Front Immunol (2019) 10:645. doi: 10.3389/fimmu.2019.00645
47. Seto EYW, Remais JV, Carlton EJ, Wang S, Liang S, Brindley PJ, et al. Toward Sustainable and Comprehensive Control of Schistosomiasis in China: Lessons From Sichuan. PLOS Negl Trop Dis (2011) 5:e1372. doi: 10.1371/journal.pntd.0001372
48. Webster JP, Gower CM, Knowles SCL, Molyneux DH, Fenton A. One Health – An Ecological and Evolutionary Framework for Tackling Neglected Zoonotic Diseases. Evol Appl (2016) 9:313–33. doi: 10.1111/eva.12341
49. Zou HY, Yu QF, Qiu C, Webster JP, Lu DB. Meta-Analyses of Schistosoma Japonicum Infections in Wild Rodents Across China Over Time Indicates a Potential Challenge to the 2030 Elimination Targets. PloS Negl Trop Dis (2020) 14:e0008652. doi: 10.1371/journal.pntd.0008652
50. Budiono NG, Murtini S, Satrija F, Ridwan Y, Handharyani E. Humoral Responses to Schistosoma Japonicum Soluble Egg Antigens in Domestic Animals in Lindu Subdistrict, Central Sulawesi Province, Indonesia. Int J One Health (2020) 6:99–108. doi: 10.14202/IJOH.2020.99-108
51. Streicker DG, Fenton A, Pedersen AB. Differential Sources of Host Species Heterogeneity Influence the Transmission and Control of Multihost Parasites. Ecol Lett (2013) 16:975–84. doi: 10.1111/ele.12122
52. Fenton A, Streicker DG, Petchey OL, Pedersen AB. Are All Hosts Created Equal? Partitioning Host Species Contributions to Parasite Persistence in Multihost Communities. Am Nat (2015) 186:610–22. doi: 10.1086/683173
53. Bielby J, Price SJ, Monsalve-CarcaÑo C, Bosch J. Host Contribution to Parasite Persistence Is Consistent Between Parasites and Over Time, But Varies Spatially. Ecol Appl (2021) 31:e02256. doi: 10.1002/eap.2256
54. Erazo D, Pedersen AB, Gallagher K, Fenton A. Who Acquires Infection From Whom? Estimating Herpesvirus Transmission Rates Between Wild Rodent Host Groups. Epidemics (2021) 35:100451. doi: 10.1016/j.epidem.2021.100451
55. Borlase A, Rudge JW, Léger E, Diouf ND, Fall CB, Diop SD, et al. Spillover, Hybridization, and Persistence in Schistosome Transmission Dynamics at the Human–Animal Interface. PNAS (2021) 118:e2110711118. doi: 10.1073/pnas.2110711118
56. Dong Y, Du CH, Zhang Y, Wang LF, Song J, Wu MS, et al. Role of Ecological Approaches to Eliminating Schistosomiasis in Eryuan County Evaluated by System Modelling. Infect Dis Poverty (2018) 7:129. doi: 10.1186/s40249-018-0511-7
57. Drummond MF, Sculpher MJ, Claxton K, Stoddart GL, Torrance GW. Methods for the Economic Evaluation of Health Care Programmes. Oxford:Oxford University Press (2015). Google-Books-ID: yzZSCwAAQBAJ.
58. Turner HC, French MD, Montresor A, King CH, Rollinson D, Toor J. Economic Evaluations of Human Schistosomiasis Interventions: A Systematic Review and Identification of Associated Research Needs. Wellcome Open Res (2020) 5:45. doi: 10.12688/wellcomeopenres.15754.2
59. Adeyemo P, Léger E, Hollenberg E, Diouf N, Sene M, Webster JP, et al. Estimating the Financial Impact of Livestock Schistosomiasis on Traditional Subsistence and Transhumance Farmers Keeping Cattle, Sheep and Goats in Northern Senegal. Res Sq (2021). doi: 10.21203/rs.3.rs-757540/v1. preprint, In Review.
60. Narrod C, Zinsstag J, Tiongco M. A One Health Framework for Estimating the Economic Costs of Zoonotic Diseases on Society. Ecohealth (2012) 9:150–62. doi: 10.1007/s10393-012-0747-9
61. Murray CJ, Lopez AD, Jamison DT. The Global Burden of Disease in 1990: Summary Results, Sensitivity Analysis and Future Directions. Bull World Health Organ (1994) 72:495–509 Available at: https://pubmed.ncbi.nlm.nih.gov/8062404/.
62. Ung L, Stothard JR, Phalkey R, Azman AS, Chodosh J, Hanage WP, et al. Towards Global Control of Parasitic Diseases in the Covid-19 Era: One Health and the Future of Multisectoral Global Health Governance. Adv Parasitol (2021) 114:1–26. doi: 10.1016/bs.apar.2021.08.007
63. Chen A, Jacobsen KH, Deshmukh AA, Cantor SB. The Evolution of the Disability-Adjusted Life Year (DALY). Socio-Econ Plann Sci (2015) 49:10–5. doi: 10.1016/j.seps.2014.12.002
64. Torgerson PR, Rüegg S, Devleesschauwer B, Abela-Ridder B, Havelaar AH, Shaw APM, et al. zDALY: An Adjusted Indicator to Estimate the Burden of Zoonotic Diseases. One Health (2018) 5:40–5. doi: 10.1016/j.onehlt.2017.11.003
65. Okello WO, Okello AL, Inthavong P, Tiemann T, Phengsivalouk A, Devleesschauwer B, et al. Improved Methods to Capture the Total Societal Benefits of Zoonotic Disease Control: Demonstrating the Cost-Effectiveness of an Integrated Control Programme for Taenia Solium, Soil Transmitted Helminths and Classical Swine Fever in Northern Lao PDR. PloS Negl Trop Dis (2018) 12:e0006782. doi: 10.1371/journal.pntd.0006782
66. Saadi A, Amarir F, Filali H, Thys S, Rhalem A, Kirschvink N, et al. The Socio-Economic Burden of Cystic Echinococcosis in Morocco: A Combination of Estimation Method. PloS Negl Trop Dis (2020) 14:e0008410. doi: 10.1371/journal.pntd.0008410
67. Zayas LPN, Rüegg S, Torgerson P. The Burden of Zoonoses in Paraguay: A Systematic Review. PloS Negl Trop Dis (2021) 15:e0009909. doi: 10.1371/journal.pntd.0009909
68. Tan D, Yu D, Li Y, Xie MS, Wen HM, Cai WB, et al. Analysis of Cost-Effectiveness on Two Chemotherapy Schemes to Schistosomiasis in Hyperendemic Villages of Hunan Province. Pract Prevent Med (2001) 8: 165–8 Available at: https://caod.oriprobe.com/articles/3461930/Analysis_of_Cost__Effectivenaess_on_Two_Chemothera.htm, https://en.cnki.com.cn/Article_en/CJFDTotal-SYYY200103002.html.
69. Yu D, Sarol JN Jr, Hutton G, Tan D, Tanner M. Cost Effectiveness Analysis of the Impacts on Infection and Morbidity Attributable to Three Chemotherapy Schemes Against Schistosoma Japonicum Hyperendemic Areas of the Dongting Lake Region, China. Southeast Asian J Trop Med Public Health (2002) 33:441–57.
70. Croce D, Porazzi E, Foglia E, Restelli U, Sinuon M, Socheat D, et al. Cost-Effectiveness of a Successful Schistosomiasis Control Programme in Cambodia (1995–2006). Acta Trop (2010) 113:279–84. doi: 10.1016/j.actatropica.2009.11.011
71. Zhang HM, Yu Q, Zhang X, Coa CL, Li SZ, Zhu H. Cost-Effectiveness Evaluation on Comprehensive Control Measures Carrying Out in Schistosomiasis Endemic Areas With Regard to Different Layers of Administrative Villages Stratified by Infection Situation of Human and Domestic Animals. I. Cost-Effectiveness Study in Inner Embankment of Marshland and Lake Regions From 2006 to 2010. Zhongguo Xue Xi Chong Bing Fang Zhi Za Zhi (2014) 26:254–9.
72. Yang Y, Li J-B, Liu H, Li S-G, He Z, Cai B, et al. Cost-Effectiveness of Comprehensive Schistosomiasis Control Strategy With Focus on Cattle and Sheep Removal in Junshan District, Yueyang City. Zhongguo Xue Xi Chong Bing Fang Zhi Za Zhi (2017) 30:14–7. doi: 10.16250/j.32.1374.2017136
73. Adriko M, Faust CL, Carruthers LV, Moses A, Tukahebwa EM, Lamberton PHL. Low Praziquantel Treatment Coverage for Schistosoma Mansoni in Mayuge District, Uganda, Due to the Absence of Treatment Opportunities, Rather Than Systematic Non-Compliance. Trop Med Infect Dis (2018) 3:111. doi: 10.3390/tropicalmed3040111
74. Babu BV, Babu GR. Coverage of, and Compliance With, Mass Drug Administration Under the Programme to Eliminate Lymphatic Filariasis in India: A Systematic Review. Trans R Soc Trop Med Hyg (2014) 108:538–49. doi: 10.1093/trstmh/tru057
75. Chami GF, Kontoleon AA, Bulte E, Fenwick A, Kabatereine NB, Tukahebwa EM, et al. Community-Directed Mass Drug Administration Is Undermined by Status Seeking in Friendship Networks and Inadequate Trust in Health Advice Networks. Soc Sci Med (2017) 183:37–47. doi: 10.1016/j.socscimed.2017.04.009
76. Inobaya MT, Chau TN, Ng SK, MacDougall C, Olveda RM, Tallo VL, et al. Mass Drug Administration and the Sustainable Control of Schistosomiasis: An Evaluation of Treatment Compliance in the Rural Philippines. Parasites Vectors (2018) 11:1–11. doi: 10.1186/s13071-018-3022-2
77. Chami GF, Bundy DAP. More Medicines Alone Cannot Ensure the Treatment of Neglected Tropical Diseases. Lancet Infect Dis (2019) 19:e330–6. doi: 10.1016/S1473-3099(19)30160-4
78. Cahapay MB. To Get or Not to Get: Examining the Intentions of Philippine Teachers to Vaccinate Against COVID-19. J Hum Behav Soc Environ (2021) 0:1–11. doi: 10.1080/10911359.2021.1896409
79. Dyson L, Stolk WA, Farrell SH, Hollingsworth TD. Measuring and Modelling the Effects of Systematic Non-Adherence to Mass Drug Administration. Epidemics (2017) 18:56–66. doi: 10.1016/j.epidem.2017.02.002
80. Chun-Hai Fung I, Fitter DL, Borse RH, Meltzer MI, Tappero JW. Modeling the Effect of Water, Sanitation, and Hygiene and Oral Cholera Vaccine Implementation in Haiti. Am J Trop Med Hyg (2013) 89:633–40. doi: 10.4269/ajtmh.13-0201
81. Mellor J, Abebe L, Ehdaie B, Dillingham R, Smith J. Modeling the Sustainability of a Ceramic Water Filter Intervention. Water Res (2014) 49:286–99. doi: 10.1016/j.watres.2013.11.035
82. Patrick R, McElroy S, Schwarz L, Kayser G, Benmarhnia T. Modeling the Impact of Population Intervention Strategies on Reducing Health Disparities: Water, Sanitation, and Hygiene Interventions and Childhood Diarrheal Disease in Peru. Am J Trop Med Hyg (2021) 104:338–45. doi: 10.4269/ajtmh.19-0775
83. Coffeng LE, Nery SV, Gray DJ, Bakker R, Vlas S, Clements ACA. Predicted Short and Long-Term Impact of Deworming and Water, Hygiene, and Sanitation on Transmission of Soil-Transmitted Helminths. PloS Negl Trop Dis (2018) 12:e0006758. doi: 10.1371/journal.pntd.0006758
84. Grimes JE, Croll D, Harrison WE, Utzinger J, Freeman MC, Templeton MR. The Roles of Water, Sanitation and Hygiene in Reducing Schistosomiasis: A Review. Parasites Vectors (2015) 8:156. doi: 10.1186/s13071-015-0766-9
85. Mari L, Gatto M, Ciddio M, Dia ED, Sokolow SH, De Leo GA, et al. Big-Data-Driven Modeling Unveils Country-Wide Drivers of Endemic Schistosomiasis. Sci Rep (2017) 7:489. doi: 10.1038/s41598-017-00493-1
86. Mekete K, Ower A, Dunn J, Sime H, Tadesse G, Abate E, et al. The Geshiyaro Project: A Study Protocol for Developing a Scalable Model of Interventions for Moving Towards the Interruption of the Transmission of Soil-Transmitted Helminths and Schistosome Infections in the Wolaita Zone of Ethiopia. Parasites Vectors (2019) 12:503. doi: 10.1186/s13071-019-3757-4
87. Wood S, Sawyer R, Simpson-Hébert M. Participatory Hygiene and Sanitation Transformation Initiative (PHAST) Step by Step Guide: A Participatory Approach for the Control of Diarrhoeal Disease. Geneva: World Health Organization (1998).
88. Mulopo C, Chimbari MJ. Water, Sanitation, and Hygiene for Schistosomiasis Prevention: A Qualitative Analysis of Experiences of Stakeholders in Rural KwaZulu-Natal. J Water Sanit Hyg Dev (2021) 11:255–70. doi: 10.2166/washdev.2021.182
89. Kosinski KC, Kulinkina AV, Abrah AFA, Adjei MN, Breen KM, Chaudhry HM, et al. A Mixed-Methods Approach to Understanding Water Use and Water Infrastructure in a Schistosomiasis-Endemic Community: Case Study of Asamama, Ghana. BMC Public Health (2016) 16:322. doi: 10.1186/s12889-016-2976-2
Keywords: Schistosoma japonicum, Schistosoma mekongi, NTD, epidemiology, economics, sociology, mathematical modelling, zoonotic transmission
Citation: Janoušková E, Clark J, Kajero O, Alonso S, Lamberton PHL, Betson M and Prada JM (2022) Public Health Policy Pillars for the Sustainable Elimination of Zoonotic Schistosomiasis. Front. Trop. Dis 3:826501. doi: 10.3389/fitd.2022.826501
Received: 30 November 2021; Accepted: 07 February 2022;
Published: 16 March 2022.
Edited by:
Luc E. Coffeng, Erasmus Medical Center, NetherlandsReviewed by:
Rico Ancog, University of the Philippines Los Baños, PhilippinesJames Truscott, Imperial College London, United Kingdom
Copyright © 2022 Janoušková, Clark, Kajero, Alonso, Lamberton, Betson and Prada. This is an open-access article distributed under the terms of the Creative Commons Attribution License (CC BY). The use, distribution or reproduction in other forums is permitted, provided the original author(s) and the copyright owner(s) are credited and that the original publication in this journal is cited, in accordance with accepted academic practice. No use, distribution or reproduction is permitted which does not comply with these terms.
*Correspondence: Eva Janoušková, e.janouskova@surrey.ac.uk