Selection on female reproductive schedules in the marula fly, Ceratitis cosyra (Diptera: Tephritidae) affects dietary optima for female reproductive traits but not lifespan
- 1Department of Zoology and Entomology, University of Pretoria, Hatfield, South Africa
- 2Institute for Evolutionary Ecology and Conservation Genomics, University of Ulm, Ulm, Germany
- 3School of Science, Western Sydney University, Penrith, NSW, Australia
Introduction: A changing environment can select on life-history traits and trade-offs in a myriad of ways. For example, global warming may shift phenology and thus the availability of host-plants. This may alter selection on survival and fertility schedules in herbivorous insects. If selection on life-histories changes, this may in turn select for altered nutrient intake, because the blend of nutrients organisms consume helps determine the expression of life-history traits. However, we lack empirical work testing whether shifts in the timing of oviposition alter nutrient intake and life-history strategies.
Methods: We tested in the marula fruit fly, Ceratitis cosyra, how upward-selection on the age of female oviposition, in comparison with laboratory adapted control flies, affects the sex-specific relationship between protein and carbohydrate intake and life-history traits including lifespan, female lifetime egg production and daily egg production. We then determined the macronutrient ratio consumed when flies from each selection line and sex were allowed to self-regulate their intake.
Results: Lifespan, lifetime egg production and daily egg production were optimised at similar protein to carbohydrate (P:C) ratios in flies from both selection lines. Likewise, females and males of both lines actively defended similar nutrient intake ratios (control =1:3.6 P:C; upward-selected = 1:3.2 P:C).
Discussion: Our results are comparable to those in non-selected C. cosyra, where the optima for each trait and the self-selected protein to carbohydrate ratio observed were nearly identical. The nutrient blend that needs to be ingested for optimal expression of a given trait appeared to be well conserved across laboratory adapted and experimentally selected populations. These results suggest that in C. cosyra, nutritional requirements do not respond to a temporal change in oviposition substrate availability.
Introduction
Seasonality is an important evolutionary driver because annual environmental fluctuations may select on life-history traits and trade-offs between them. In climatic regions where the year is usually characterised by favourable and unfavourable seasons (e.g., hot and cold or dry and wet), organisms have evolved strategies to cope with fluctuating environments and prioritise investment in some life-history traits over others, depending on the time of the year (1). In tropical and sub-tropical environments for example, where temperatures never drop to levels that may prompt diapause, insects shift their relative investment in reproduction versus survival as the seasons change (2–4). In such environments, the factor triggering this shift appears to be host plant availability. Hence, in Anaea butterflies (2) and Bactrocera fruit flies (3), populations prioritise survival over reproduction during the dry season, while populations invest in reproduction at the expense of life expectancy in the wet season. Furthermore, Clarke et al. (4) suggested that Bactrocera species undergo a reproductive arrest in the unfavourable season when host plants are unavailable or scarce. This differential seasonal investment in reproduction or survival that appears to be linked to host availability could be associated with a change in nutrient availability, or nutrient regulation, between seasons. Food sources used by adult tephritid fruit flies in nature are not well known, but consumption of fruit juice, nectar, faeces and honeydew is reported (5, 6). Supporting the idea of a differential seasonal investment linked to nutrition, individual nutrient intake affects ageing and reproduction in tephritid flies. Without a source of protein, which is essential to sexual maturation, flies survive in a “waiting mode” (7, 8). Accordingly, seasonal variation in nutrient availability could shift individual investment in reproduction versus survival. Similarly, organisms could adjust their nutrient regulation strategies to adjust expression of either trait. Understanding how variation in host availability affects nutrient regulation and life-history, is particularly important in a world where the phenology of herbivorous insects and their hosts is changing (9, 10).
The nutritional geometric framework (NGF) is a dietary mapping technique that allows us to determine how the intake of two or more nutrients, both singly and in combination, affects the expression of traits of interest (11). Typically, the NGF has been used to test how protein (P) and carbohydrate (C) affect phenotypes in herbivorous species, and P and lipid (L) in carnivorous species (12). The NGF is a powerful tool to study how these nutrients interact to shape life-history traits and the trade-offs between them. Using this approach, it was shown that expression of traits including lifespan (13–15), fertility (16) and immune function (17) depend critically on the ratio of nutrients that organisms consume.
Meta-analyses show that the negative impact of high P:C ratios on survival is widespread across Orthoptera, Hymenoptera, Diptera and Lepidoptera (18, 19). While high protein typically reduces lifespan, protein is often necessary for female fertility (20). This means that the nutrient blends that optimise female lifespan and fertility are often different. This necessitates a resource-based trade-off because females cannot eat a nutrient blend that maximises expression of both traits at the same time. Our understanding of dietary optima for male reproduction is far less developed than our understanding of female dietary requirements. Nevertheless, in species for which we have data in both sexes, typically females experience a diet-mediated trade-off between lifespan and fertility, while males do not (14, 21).
When the optimal nutrient blends for lifespan and fertility differ such that individuals cannot optimise both traits simultaneously, individuals given a choice of foods can regulate their intake to maximise expression of either trait or self-select an intermediate nutrient blend that allows moderate expression of both traits. In Ceratitis cosyra, females offered a choice of diets choose a nutrient blend (1:3 P:C) closer to the optimum for daily egg production (1:2.5 P:C) than lifespan (0:1 P:C) (15). However, in field crickets, females appear to choose a nutrient blend (1:2.2 P:C) that is intermediate between the dietary optima for lifespan (1:8 P:C) and daily fecundity (1:1 P:C) (16). Hence, flexible dietary intake allows individuals to prioritise specific life-history traits and adjust their life-history strategies. While different species appear to resolve trade-offs in different ways, it is unclear how often strategies of nutrient intake differ within species, between the sexes or among different populations.
In nature, life-history strategies vary enormously between populations. For example, lifespan can differ within species along latitudinal or altitudinal gradients (22) or in relation to the number of predators that occur in sympatry (23). It is not clear if this affects how individuals regulate their nutrition in species that experience dietary mediated trade-offs between lifespan and fertility. In populations under selection for long lifespan, individuals might regulate their nutrient intake towards nutrient blends that promote lifespan. Similarly, if populations are under selection for a shorter lifespan, it could be expected that individuals regulate their intake towards nutrient blends that maximise short-term reproductive investment. To date, these ideas have not been tested empirically. Nevertheless, a modelling approach combining the NGF with an agent-based model predicts that intake of nutrient blends that favour reproduction at the expense of lifespan should evolve in conditions of increased mortality risk in adults (24).
To understand how the variation in host availability shapes the relationship between food, sex and death and strategies of nutrient regulation, we selected upwards on age of female reproduction in replicate lines of C. cosyra. After 20 generations of selection, these upward-selected lines showed delayed reproductive effort illustrating successful selection but, against expectations, lived shorter lives and males transferred fewer sperm at mating (25). In this species, females and males have divergent dietary optima for lifespan (females: 0:1 P:C; males: 1:10 P:C), and the best nutrient blend for fertility and survival differs in females (fertility: 1:2.5 P:C; lifespan: 0:1 P:C), but both sexes regulate their intake towards a 1:3 P:C ratio (15). The fitness consequences of this are unknown in males because dietary optima for reproductive success have not been determined, but in females, this nutrient regulation strategy improves daily egg production at the expense of reduced lifespan. Given the effects of the selection regime on the phenotype of the flies (i.e. upward-selected flies), we predict that the dietary optima for lifespan and reproductive traits changed in upward-selected lines but not self-regulated intake. As the selection regime also reduced male lifespan in a similar way, we predict that males will target a nutrient blend that does not differ from that of the females, and that female and male lifespan are optimised on very similar P:C ratios.
Materials and methods
Fly populations and husbandry
Infested mangoes from across the Mpumalanga province, South Africa, were collected and pupae of C. cosyra retrieved. The wild flies emerging from these pupae were used to establish a culture. The procedure followed to establish a culture of C. cosyra was identical to the one used in Malod et al. (25). In brief, the culture was maintained at ~ 23°C in a climate room with a 14:10 light:dark photoperiod. Adults were kept in groups of ca. 200 flies in 5 L plastic cages with food (hydrolysed yeast and sugar in separate dishes) and water (water-soaked cotton wool) ad libitum.
Selection regime
From the culture, we established four replicate populations of two selection lines. In control (CT) lines, oviposition substrate was provided when flies were 15 days old, which is the average age when eggs are typically collected from this species in laboratory conditions (e.g., 5, 26). In upward-selected lines (US), oviposition substrate was provided when flies were 25 days old, meaning that only females that survived to this age to oviposit would contribute to the next generation. Because female C. cosyra do not store sperm for long periods of time (important decline in sperm storage observed at 14 days post-mating) (27), the effect of this selection regime on male fertility is likely to be similar to what is observed in females. We maintained the selection regime for over 35 generations. Experimental flies used in Experiment I were collected from the 35th generation and flies tested in Experiment II were from the 37th generation. Because flies were selected on age of oviposition, each selection regime was inevitably assayed at different time points. Therefore, selection lines differ in their assay date. However, given that results are similar across selection regimes and that the patterns of expression for lifespan and reproduction correspond with what was observed in previous generations (25, 28), it is unlikely that results were affected by a temporal blocking effect.
Experimental diets and consumption
Fifteen liquid experimental diets (Table S1) were created that varied in their P:C ratios (0:1; 1:8; 1:4; 1:2; 1:1 P:C) and total concentration of protein and carbohydrate (45, 180 and 360 g/L). This diet range is similar to the one previously used for C. cosyra (15), except that we discarded the 2:1 P:C ratios due to the short lives of the flies on this diet (about 20 days on average), which indicates a pathological effect. A blend of 18 amino acids was used as a source of protein and sucrose as a source of carbohydrate (Table S2). All diets were prepared with equal concentrations of micronutrients (Table S2) and 1.3 mL/L of blue food dye (Robertsons, Johannesburg, South Africa) was added to facilitate reading of the liquid volume. Either one (Experiment I, no-choice experiment) or two (Experiment II, choice experiment) diets and water were provided to individual flies on their day of emergence in 200 µL pipette tips (ROLL s.a.s, Italy), capped loosely with putty-like adhesive (Bostik, South Africa).
The volume of food consumed was determined by measuring pipette tips containing liquid diets with 1 mm scale graph paper (Canson, France). We replaced pipette tips for food and water every 5 days (no-choice experiment), 4 days (choice experiment) or earlier if depleted. Food was measured before and after replacing the pipette tips. Consumption was calculated using the difference between the initial length (for 100 µL of liquid) and the remaining length of diet in the pipette tip. Linear measurements of consumption were converted into volumes with a mathematical function that was obtained from a standard curve (Figure S1). In the no-choice experiment, each diet had three pipette tips used as controls (i.e., placed in the climate room, but in containers without flies) to assess evaporation in the climate room. In the choice experiment, two containers with two pipette tips per diet were maintained in the climate room to assess evaporative loss. For both experiments, the volume of evaporated diet was measured at the same time as the volume of diet consumed by the flies. The amount of diet volume lost for each ratio and concentration was then used to correct consumption for evaporation.
Experiment I: effects of five protein to carbohydrate ratios at three concentrations
For each line and replicate, we analysed the effect of protein and carbohydrate on lifespan (LS) and reproduction by providing one of the 15 experimental diets to each of 5 females and 5 males (n = 15 diets × 2 selection lines × 4 replicates × 2 sexes × 5 flies = 1200 flies). Virgin females and males were placed individually in plastic transparent containers (125 mL) within 24 h of emergence. Each fly was supplied with two 200 µL pipette tips, one containing filtered water and one containing 100 µL of experimental diet. Mortality, diet and water levels were checked daily. Reproductive effort was measured by recording female fecundity. To do this, at the base of the females’ containers we placed a black screw-top lid (ø 32 mm) as an oviposition dish, filled with 2.5 mL of 10% orange essence solution (Robertsons, Johannesburg, South Africa). The dish was covered with a double layer of laboratory film that was pierced several times. In this species, females are able to lay eggs even when they are virgin (26). The dish was placed for both selected lines from the beginning of the experiments, and eggs were counted every six days when dishes were replaced. These data allowed us to estimate daily egg production (eggs/day, DEP) for an individual, and lifetime egg production (i.e., giving the number of eggs laid throughout the entire lifespan, LEP). The average temperature and relative humidity (RH) in the climate room during the no-choice experiment was 24.2 ± 1.4°C and 55 ± 9% for the CT line and 22.9 ± 1.6°C and 58 ± 12% for the US line.
Experiment II: nutrient intake under dietary choice
To assess how individuals regulate their nutrient intake when they have a choice of diets, we recorded consumption of protein and carbohydrate when flies were given a dietary choice using established methods and combinations of P:C ratios (14, 15, 21). Flies were maintained as in the no-choice experiment, the only difference being that instead of one diet, they were given a pair of diets. Flies were randomly assigned to one of the following dietary pairs (Figure S2): Pair 1: 1:1 (180 g/L) vs 0:1 (180 g/L); Pair 2: 1:1 (180 g/L) vs 0:1 (360 g/L); Pair 3: 1:1 (360 g/L) vs 0:1 (180 g/L); Pair 4: 1:1 (360 g/L) vs 0:1 (360 g/L). For each diet in the pair, 100 µL was provided in a different pipette tip. The diet pairs were tested for each selected line and replicate on five flies of each sex (n total= 280 flies). Due to one replicate line collapsing, the US line was tested on only three replicates in Experiment II. Diet consumption was recorded every four days, starting within 24 h after emergence over a period of 16 days. No flies died or escaped before the end of the experiment. The average temperature and RH during the assay for the CT line was 22.6 ± 2.1°C and 65 ± 7%, and during the US line assay 21.7 ± 1.2°C and 55 ± 4%.
Statistical analyses
Experiment I
In the first experiment, as longevity among the diet groups was highly variable, we divided total consumption by days lived to express male and female consumption in mg per day so that consumption by individuals was more comparable. Moreover, to standardize the response variables (LS, LEP and DEP) and nutrient intake, a Z-transformation to a mean of zero and standard deviation of one was used. Data from flies escaping during the experiment or dying from non-natural death (trapped in a drop of liquid diet) were removed. Then, the statistical procedure described in detail in Rapkin et al. (29) and Bunning et al. (30) was followed. To summarise, a multivariate response-surface approach was used to estimate the linear and non-linear (interactions between P × P, C × C and P × C) effects of protein and carbohydrate on response variables (LS, LEP, DEP) for each selected line and sex. First, a model containing only protein and carbohydrate was built to assess if intake of each nutrient significantly affected each response variable. Then, a second model including the linear and quadratic and correlational effects of protein and carbohydrate was built to determine the non-linear and interaction effects on the response variable. Replicate was added to the models as a random factor (four levels) to account for replication. A sequential approach was then used to compare nutritional landscapes across selected lines in females and males, between sexes for LS, and across the different traits in females (LS, LEP and DEP). For these comparisons it was necessary to create a dummy variable “trait type” (i.e., a column in the dataset with the two compared traits). The landscape comparisons were also performed using a sequential model building approach. The first model contained the linear effects only and was compared using partial F-tests to a second model with the linear effects plus their interaction with the dummy variable. In the third model, the quadratic effects (P × P and C × C) were added, and the model was compared to a fourth model including the interactions with the dummy variable. Finally, the correlational effect (P × C) was added to the fifth model and compared to the final model including the interaction between the correlational effect and the dummy variable. When an overall significant difference between models was detected using the sequential approach, a univariate analysis was used to determine which nutrient contributed to the effect. Generalised linear mixed effects models were built using the glmmTMB function from the glmmTMB package (31). To visualize the data, nutritional landscapes were constructed with the function Tps from the package FIELDS (32) in R v 4.2.1 statistical environment (R core team, 2022, Vienna, Austria); untransformed values (raw data) for the response variables and nutrient intake were used to construct the surface responses. To determine the nutritional optima (i.e., the exact amount of protein and carbohydrate needed to optimise the trait) on these landscapes we used the function OptRegionTps from the OptimaRegion package (33). To test for any difference between lines in overall food consumption, we calculated the total consumption (P+C). A generalised linear mixed effects model was used, with total consumption (square-root transformation to normalise the residuals of the model) as the response variable, selection, sex and their interaction as fixed factors, concentration and P:C ratio as covariates and replicate as a random factor. The model was built using the glmmTMB function.
Experiment II
In the choice experiment, the intake of nutrients expected had individuals fed at random from each diet was calculated for each fly (i.e., the volume of nutrients consumed if flies ate equal amounts of each food in the diet pair). A random intake would indicate that flies were not regulating their nutrient consumption. Expected intakes were subtracted from the observed intake values and the difference was compared with zero (one sample t test). Having determined if flies eat at random or not on the diet pairs, we characterised nutrient regulation strategies (i.e., if there was a preference for protein or carbohydrate) (see supplementary material Tables S4 and S5). For each selection regime, sex and diet pair we calculated a cumulative intake for protein and carbohydrate, then a regulated intake point as the mean total intake of protein and carbohydrate across all diet pairs. To determine how the regulated intake point differed between selection regimes and between sexes within each selection regime, we ran generalised linear mixed models. In each model, carbohydrate intake was added as response variable, protein intake as a covariate, selection regime or sex as fixed effects, as well as their interaction with the covariate, and replicate was added as a random factor. A significant interaction between the protein intake and the fixed factor would indicate that the regulated intake point differs between selection regimes or sexes. Protein and carbohydrate intake was expressed in mg rather than mg per day as the dietary choice experiment was performed over the same duration of 16 days for all individuals.
Results
Experiment I: effects of five protein to carbohydrate ratios at three concentrations
In both selection lines, female lifespan (Figures 1A, B) peaked in the low protein, high carbohydrate region of the nutritional landscapes. However, the optimum for lifespan was more protein biased for US (Protein = 2.8 mg/day; Carbohydrate = 24.9 mg/day) than for CT females (Protein = 0.21 mg/day; Carbohydrate = 24.2 mg/day). These positive effects of carbohydrate on lifespan were evident in both selection lines as significant linear effects of carbohydrate (Table 1). There were also positive linear effects of protein intake on lifespan. However, in both cases lifespan increased more steeply with carbohydrate intake than protein intake (see gradients in Table 1). Further, in females of both selection lines, the significant negative quadratic effect of carbohydrate on lifespan indicated a peak in expression in flies fed a high carbohydrate diet (between 15 and 24 mg/day for CT and 23 and 25 mg/day for US). In addition, a significant positive quadratic effect of protein was detected in CT females, indicating a trough (1:30 to 1:10 P:C) ranging from low to high protein intake on the surface response (Figure 1A). Because the contribution of carbohydrate intake to lifespan was much greater than the contribution of protein, there was also a significant negative correlational effect, meaning that there was negative covariance between protein and carbohydrate that increased lifespan. No significant quadratic effect of protein or a correlational effect were detected in US females.
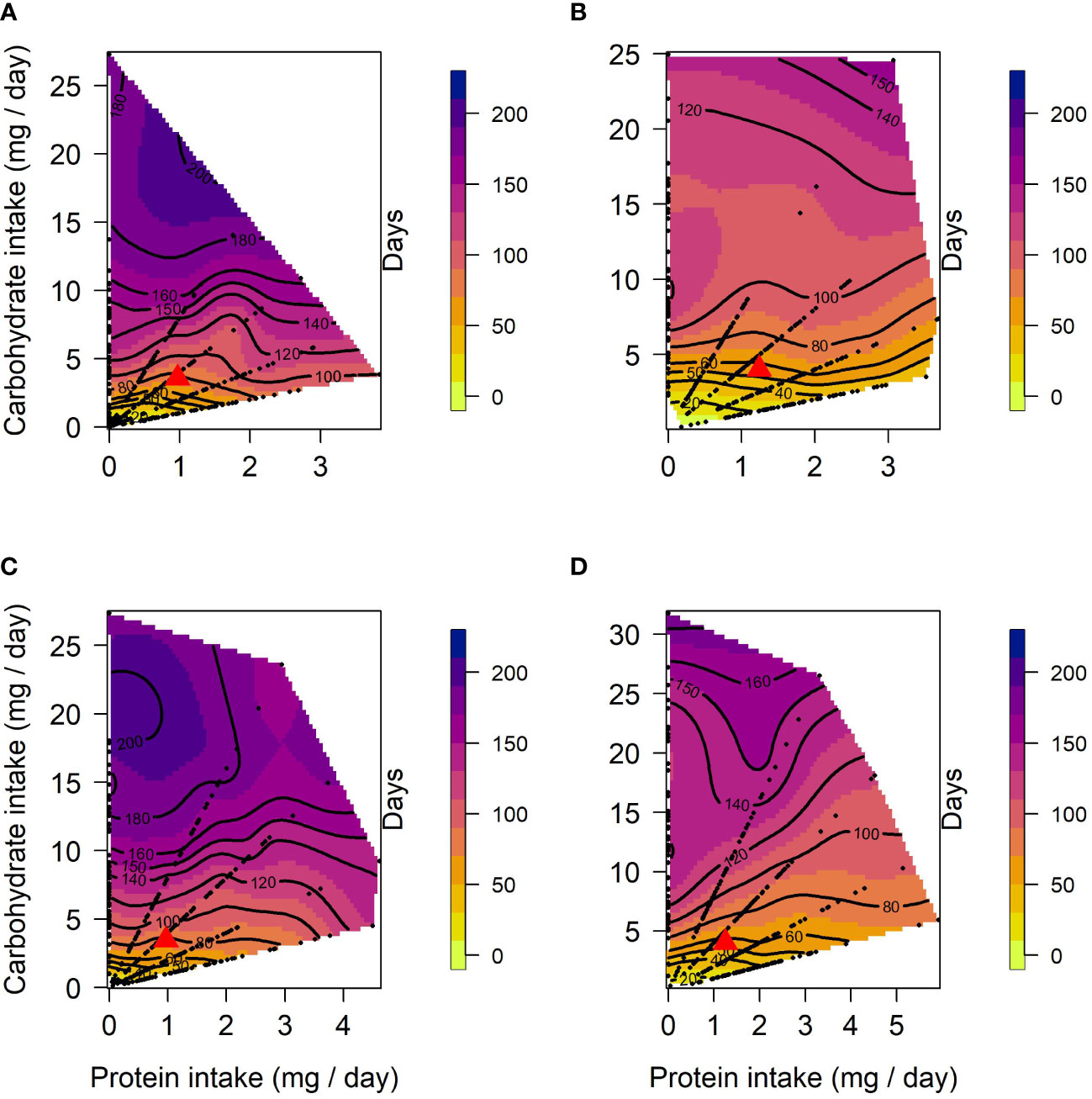
Figure 1 Nutritional landscapes for female (A, B) and male (C, D) lifespan in CT (A, C) and US (B, D) lines of C. cosyra selected on age of female oviposition. The colour gradient indicates how individuals perform for lifespan on a specific P:C intake. Red triangles indicate the regulated intake point. Each nutritional landscape represents 300 individuals.
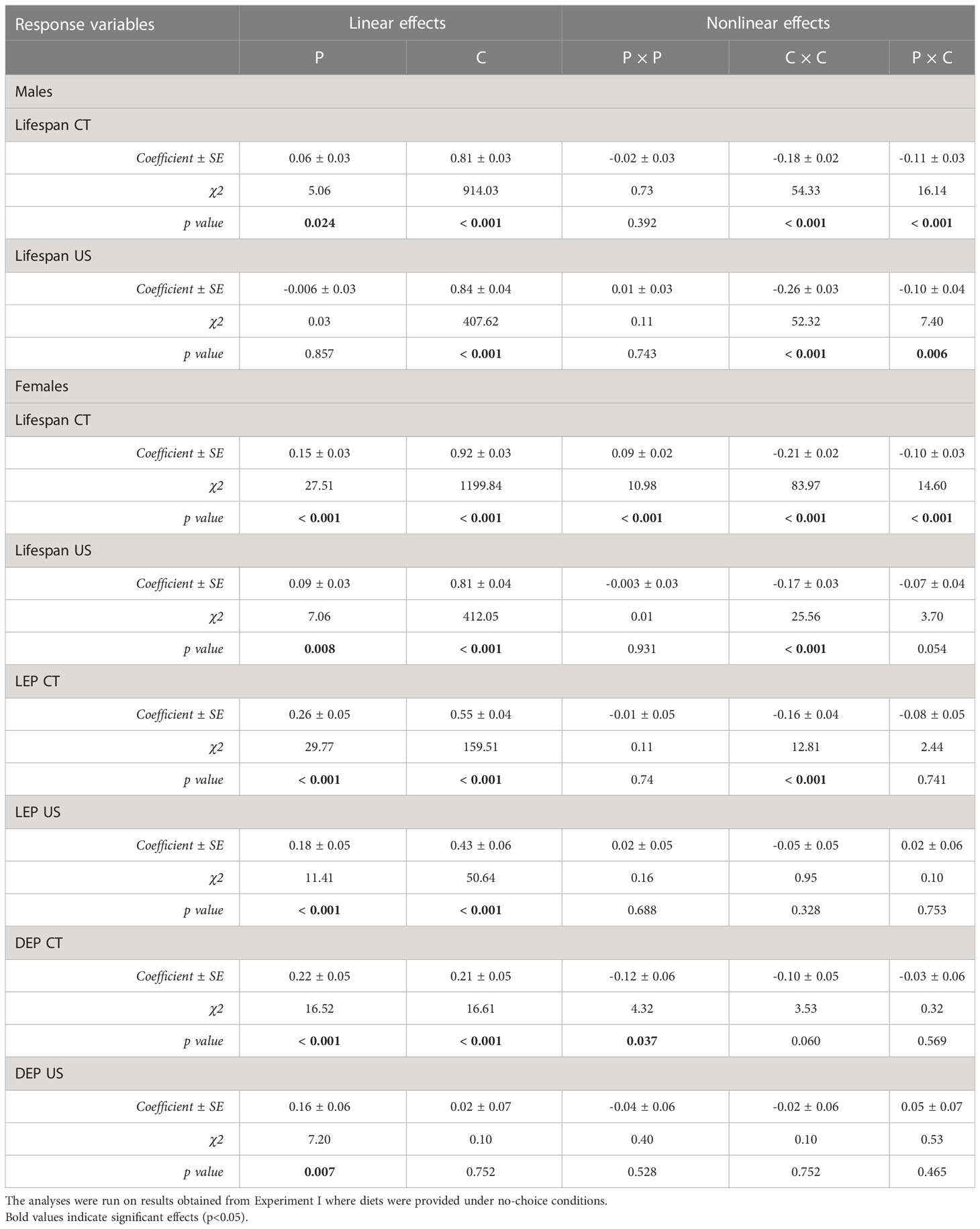
Table 1 Effects of five protein to carbohydrate ratios at three concentrations on lifespan (LS) and female reproductive traits (LEP and DEP) in C. cosyra in control lines (CT) and lines selected upwards on age of female oviposition for 35 generations.
In males, lifespan increased with intake of carbohydrate in both lines (Figures 1C, D), as indicated by a significant linear effect of carbohydrate (Table 1). A positive significant linear effect of protein was also found in CT males, indicating that lifespan also increased with the intake of protein, but to a lesser extent than carbohydrate (Table 1). As in females, there was a negative quadratic effect of carbohydrate in both lines (Table 1), indicating a peak in lifespan when carbohydrate intake was around 15 to 25 mg/day and 29 to 32 mg/day for CT and US respectively. The surface responses indicated that lifespan was optimised at low P:C ratios (Figures 1C, D). A negative correlational effect was detected in males of both lines, once again indicating a negative covariance between both nutrients that affect lifespan. For both CT and US males, lifespan was optimised at low P:C, the calculated nutritional optima were: Protein = 0.07 mg/day, Carbohydrate = 27.3 mg/day for CT, and Protein = 0.09 mg/day, Carbohydrate = 31.7 mg/day for US.
The effects of protein and carbohydrate on female lifetime egg production were similar to those on lifespan. In both lines, there was a significant positive linear effect of protein and carbohydrate, with lifetime egg production increasing with the intake of both, but to a lesser degree with protein intake (Table 1). A significant negative quadratic effect for carbohydrate was only found in CT females, indicating a peak in the expression of lifetime egg production with carbohydrate intake around 24 to 27 mg/day. Optimal expression of lifetime egg production in CT females was at a higher carbohydrate intake relative to protein, the peak for lifetime egg production was determined to be at a protein intake of 0.01 mg/day and carbohydrate intake of 25.8 mg/day (Figure 2A). In contrast, there was no clear peak detected in US females for lifetime egg production (Figure 2B). No correlational effects were found in either line. Daily egg production for CT and US lines responded differently to dietary manipulations. In CT females, both protein and carbohydrate positively contributed to daily egg production expression to a similar extent (Table 1), and a significant negative quadratic effect of protein indicated a peak. Calculation of the nutritional optima indicated that the peak in daily egg production among CT females was at a protein intake of 1.6 mg/day and carbohydrate intake of 16.7 mg/day (Figure 2C). In contrast, only a significant linear effect of protein was detected in US females, indicating that an increase in protein intake contributed to higher daily egg production expression without any optimal value being reached in our study (Table 1) (Figure 2D).
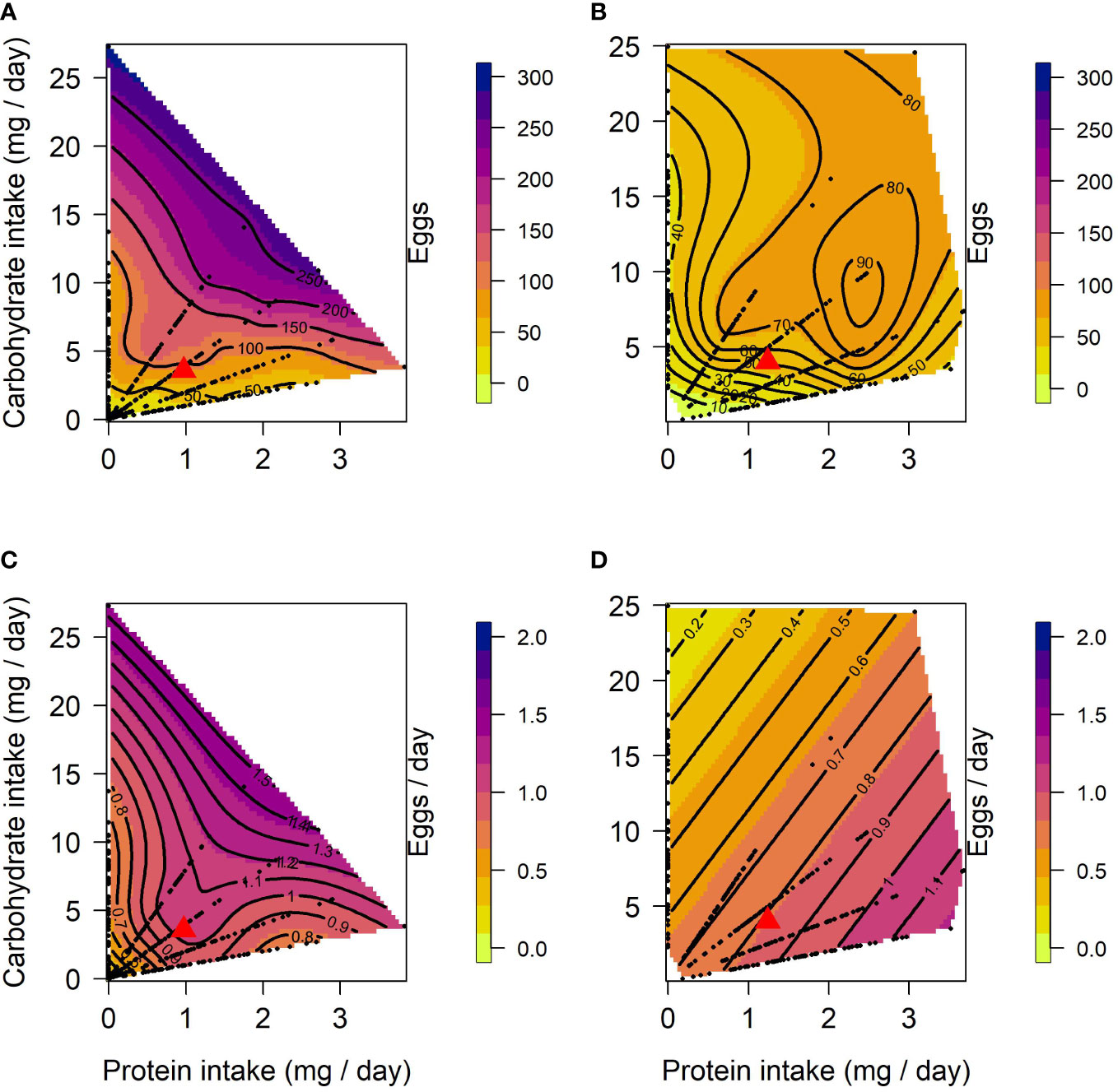
Figure 2 Nutritional landscapes for female lifetime egg production (A, B) and daily egg production (C, D) in CT (A, C) and US (B, D) lines of C. cosyra selected on age of female oviposition. The colour gradient indicates how individuals perform for a trait on a specific P:C intake. Each nutritional landscape represents 300 individuals.
Using a sequential building approach to compare nutritional landscapes for lifespan between selection lines, we found no difference in linear, quadratic or correlational effects of protein or carbohydrate between CT and US males (Table 2). However, in females there was a significant difference in the linear effects of protein and carbohydrate on lifespan across the selection regimes (Table 2). This suggests that the effects of both nutrients were stronger in CT females (i.e., the linear gradients were steeper for both protein and carbohydrate, meaning that lifespan increased more with each additional unit of macronutrient consumed).
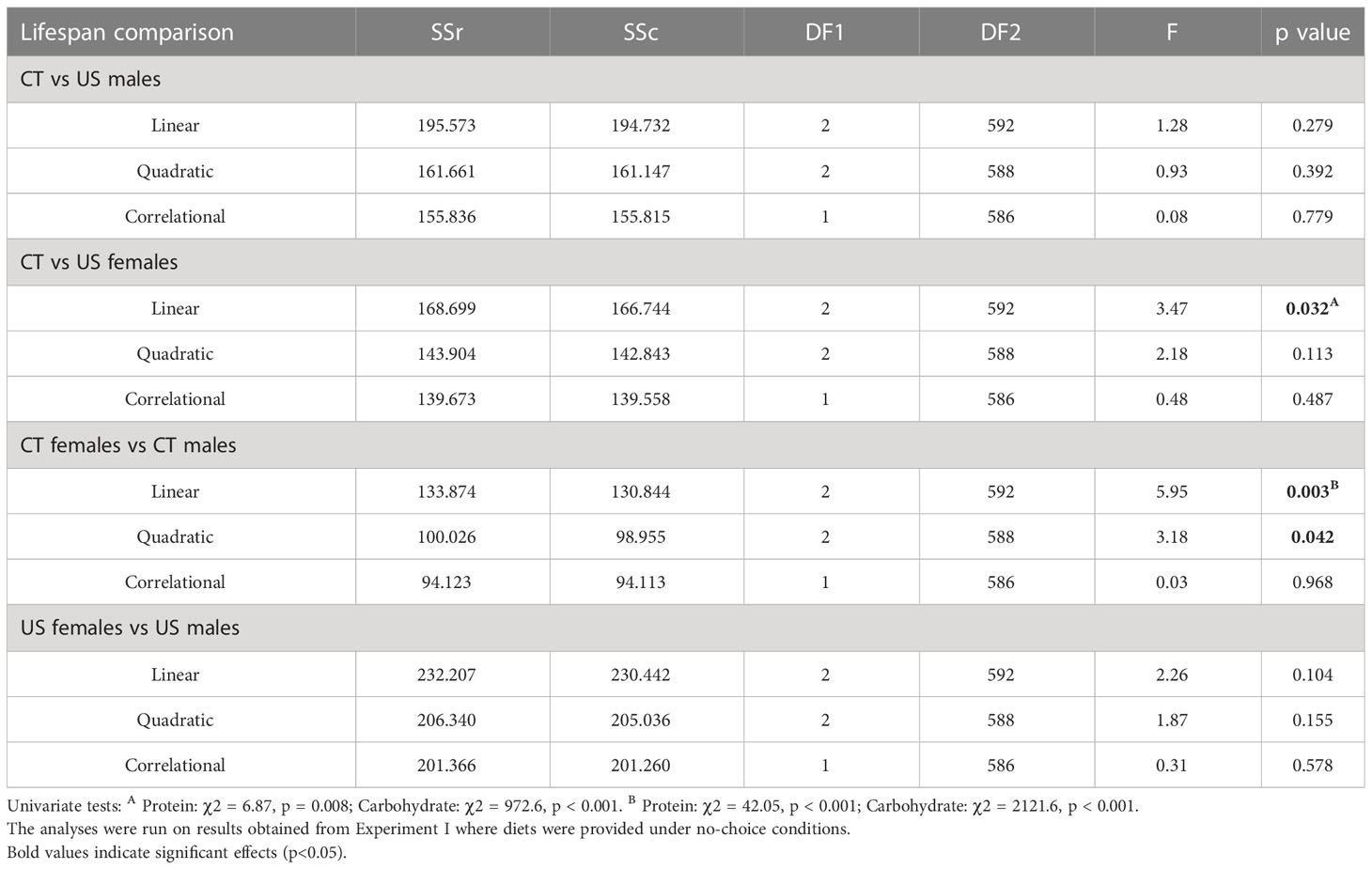
Table 2 Comparison of nutritional landscapes for lifespan between sexes and lines of C. cosyra selected on age of female oviposition.
Having compared nutrient landscapes for lifespan between selection lines within each sex, we then compared landscapes between females and males belonging to the same selection lines. We did not find differences in the linear, quadratic or correlational effects of protein and carbohydrate across the sexes in US lines (Table 2). However, CT females and males differed in their linear and quadratic effects for protein and carbohydrate (Table 2). The difference in linear effects is due to protein and carbohydrate contributing more to lifespan expression in CT females than males, and the difference in the quadratic effect is due to a significant quadratic effect of protein in females but not in males.
When comparing lifespan to reproductive traits in CT females we found differences in the linear effects for all comparisons and in quadratic effects when lifespan was compared to daily egg production (Table 3). The linear differences between lifespan and lifetime egg production in CT females arose because protein and, to a greater extent, carbohydrate made a greater contribution to the expression of lifespan than lifetime egg production (Table 1). The significant difference in the linear effects between daily egg production and lifespan in CT females was due to a difference in the magnitude of the contribution of carbohydrate to the expression of these traits, and to a lesser extent to the difference in protein contribution (Table 1). The significant difference in the quadratic effects reflects the absence of a quadratic effect of carbohydrate in daily egg production but not lifespan, and a difference in the direction of the quadratic effect of protein (peak for daily egg production, through for lifespan) (Table 1). In US females we detected significant differences in the linear effects of nutrients when comparing lifespan to lifetime egg production and daily egg production (Table 3), which were caused only by a stronger contribution of carbohydrate to lifespan.
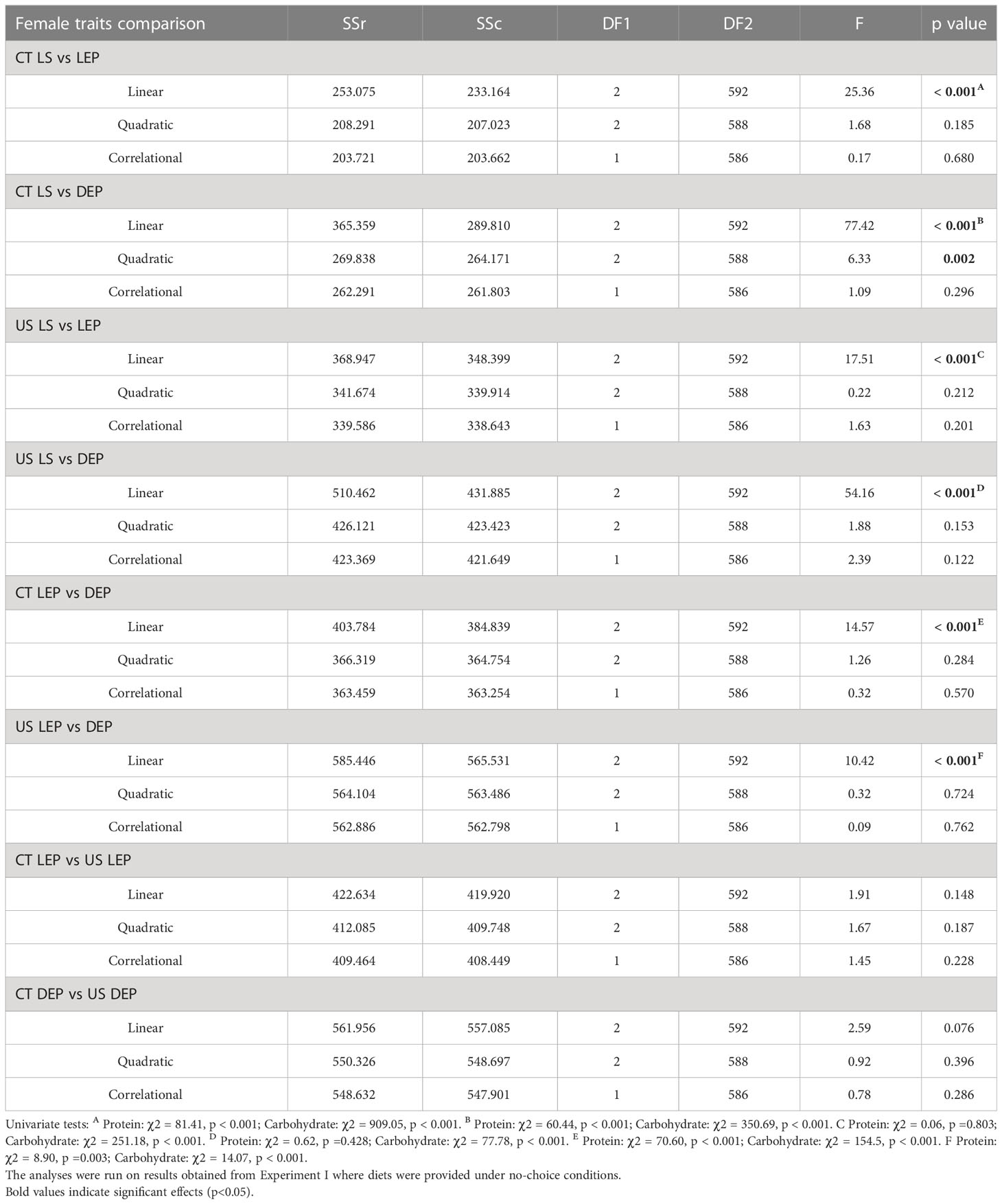
Table 3 Comparison of nutritional landscapes between lifespan (LS) and female reproductive traits in C. cosyra lines selected on age of oviposition.
Surface responses for lifetime egg production and daily egg production differed in their linear effects for both CT and US lines (Table 3). Both nutrients were responsible for this significant difference (Table 3), and this is the result of the larger contribution of carbohydrate towards the expression of lifetime egg production than daily egg production (Table 1). When comparing surface responses for reproductive traits across selection lines we did not detect any significant differences (Table 3).
Total consumption (protein and carbohydrate together) was significantly affected by selection regime (χ2 = 51.09, p < 0.001) and sex (χ2 = 35.53, p < 0.001). Overall, CT flies consumed significantly less diet than US flies (estimate = -0.56, p < 0.001) (Figure 3), and males significantly more than females (estimate = 0.36, p < 0.001). There was also a significant effect of the concentration and the P:C ratio (Concentration: χ2 = 6.86, p = 0.008; P:C ratio: χ2 = 347.28, p < 0.001). Consumption decreased as the concentration (coefficient= 0.004, p = 0.008) and P:C ratio (coefficient = -1.14, p < 0.001) increased.
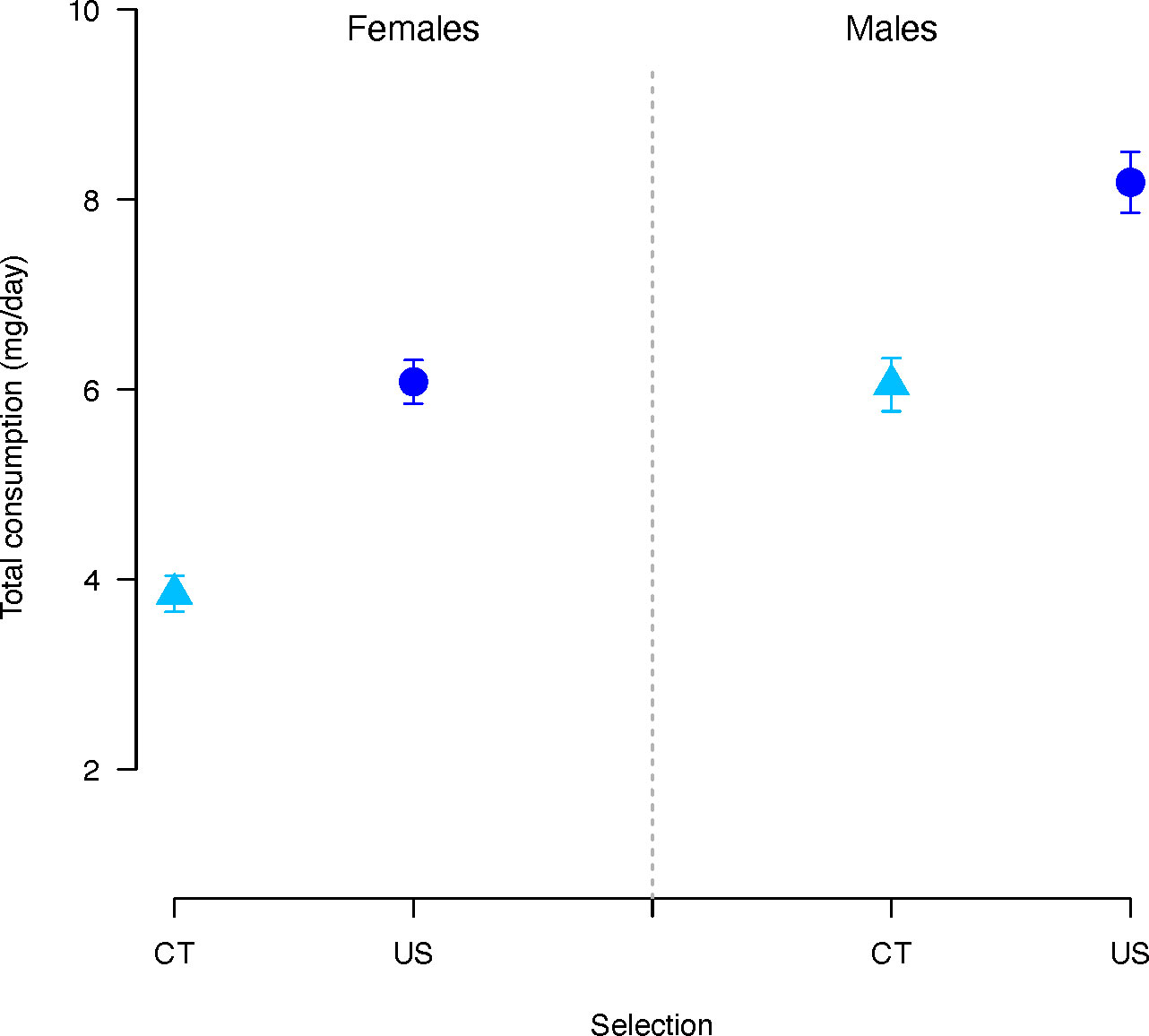
Figure 3 Total nutrient consumption (P+C) in females and males C. cosyra of control (light blue triangles) and upward-selected (blue circles) lines on age of female oviposition. Error bars indicate the standard error of the mean. Each bar represents 300 individuals.
Experiment II: nutrient intake under dietary choice
The total amount of nutrients consumed by females and males was similar within selected lines but differed between lines (CT: female: Protein = 15.65 mg, Carbohydrate = 57.33 mg; male: Protein = 15.46 mg, Carbohydrate = 55.45 mg; US: female: Protein = 19.93 mg, Carbohydrate = 64.39 mg; male: Protein = 20.06 mg, Carbohydrate = 64.55 mg). Despite the difference between selected lines in the amount of nutrients consumed, the regulated intake points for the CT and US lines were in the same region of the nutrient space between the nutritional rails representing the P:C ratios 1:4 and 1:3 (CT: 1:3.6; US: 1:3.2) and were the same between sexes (Figure 4).
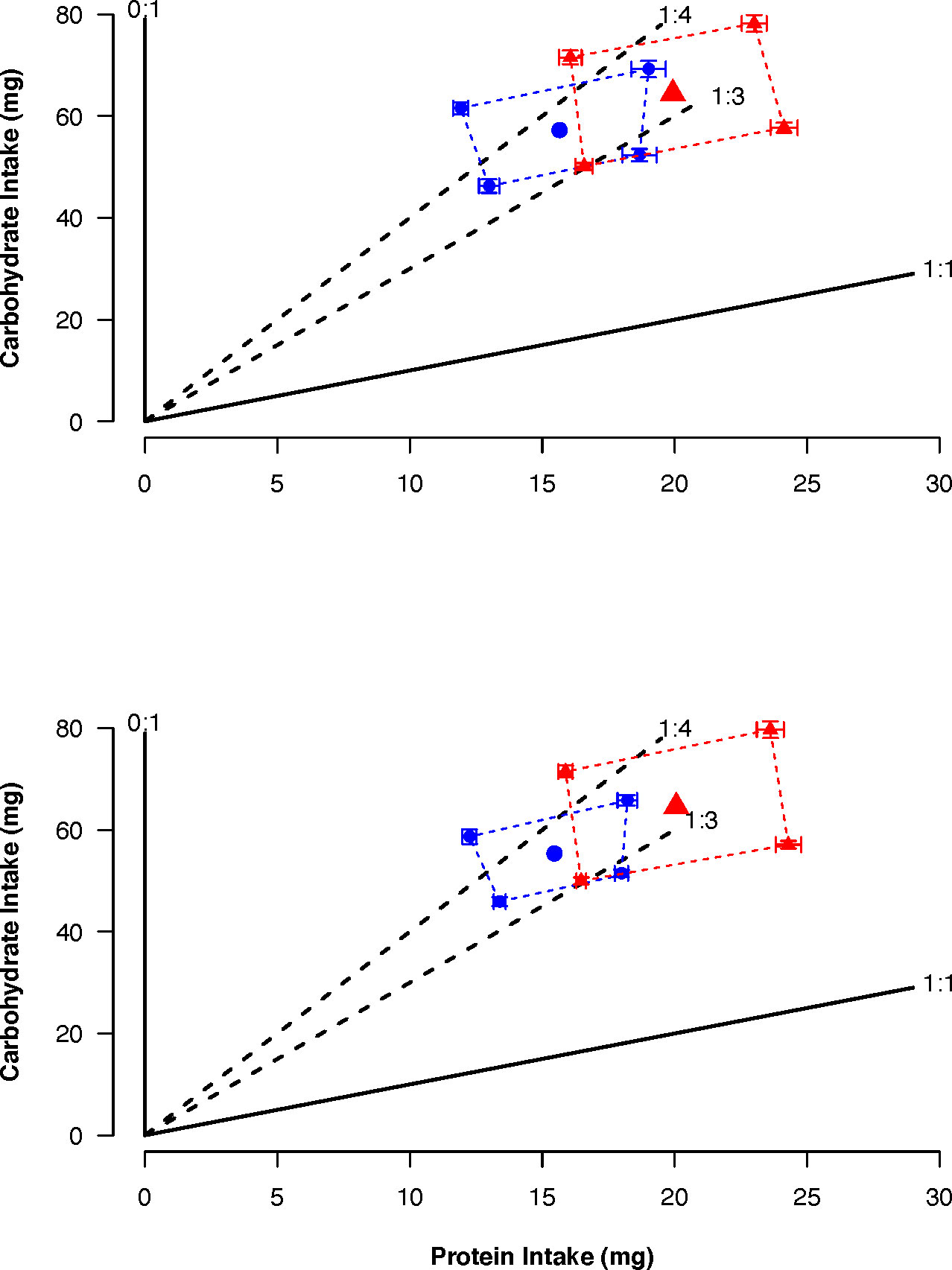
Figure 4 Average total intake ( ± SE) of protein and carbohydrate when females (top) and males (bottom) of control (blue) and upward-selected (red) lines were given the choice between two diets over a 16 day feeding period. The regulated intake points are represented by the large blue circles and red triangles. Diet pairs were: Pair 1: 1:1 (180 g/L) vs 0:1 (180 g/L); Pair 2: 1:1 (180 g/L) vs 0:1 (360 g/L); Pair 3: 1:1 (360 g/L) vs 0:1 (180 g/L); Pair 4: 1:1 (360 g/L) vs 0:1 (360 g/L). For each sex and pair, blue circles and triangles represent 20 individuals and red circles and triangles 15 individuals.
We found that flies only ate at random when feeding on 1:1 (180 g/L) vs 0:1 (360 g/L) (Pair 2) for CT flies and 1:1 (180 g/L) vs 0:1 (180 g/L) (Pair 1) for US flies, and flies were not eating at random from each other diet pair (Table S4). The intake of carbohydrate differed between selected lines (Table 4). This was because CT flies had a lower intake than US ones (coefficient = -0.20, p = 0.041) (Figure 4). In addition, there was a significant effect of the protein intake (Table 4), as intake of carbohydrate increased with the intake of protein (coefficient = 0.01, p < 0.001). The absence of a significant interaction between protein intake and selection regime indicated that the regulated intake point (i.e., self-selected P:C ratio) did not differ between selected lines. We found no difference between strategies of nutrient regulation across the sexes within the same selected lines (Table 4). In both selected lines, there was a significant effect of the protein intake on the carbohydrate intake (Table 4). The intake of carbohydrate increased with the intake of protein (CT: coefficient = 0.02, p < 0.001; US: coefficient = 0.01, p = 0.016).
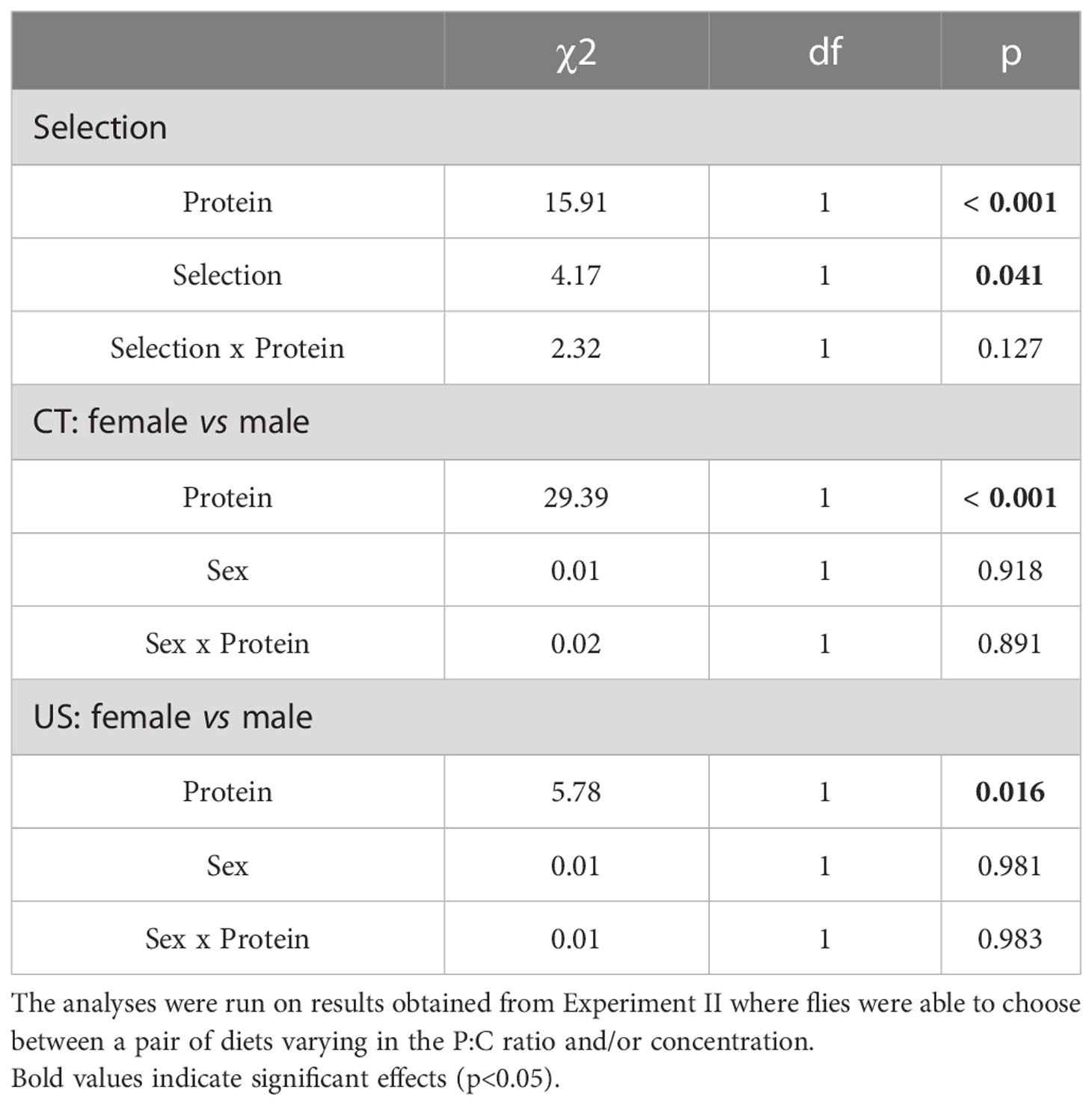
Table 4 Comparison of the regulated intake of carbohydrate by C. cosyra between lines selected on age of female reproduction and sexes.
Discussion
In insects, the trade-off between lifespan and reproduction is often mediated by diet in one or both sexes (13, 16, 21, 24, 34, 35). These studies have also reported that dietary optima for reproductive traits and lifespan are sex specific and that females often have different dietary optima for lifespan and reproduction. Given that optimal foraging theory predicts that individuals regulate intake to optimize fitness (36), this means that optimizing fitness via nutrient regulation may involve a compromise between consuming a diet that is optimal for lifespan and one that promotes reproduction. In keeping with this idea, female insects tend to regulate towards an intake that is not optimal for either lifespan or daily reproductive effort, but rather lies between the two divergent optima (13, 21, 37). Therefore, in our upward-selected flies where oviposition substrate was available at a later age, which induced a drop in reproductive effort and lifespan (25), we expected the amount or blend of nutrients ingested to differ from those of the control flies, towards a nutrient blend that promoted survival until the reproduction substrate became available.
The selection regime had a minor impact on how both nutrients affected lifespan. Both nutrients contributed slightly more to the expression of lifespan in control than in upward-selected lines. Nevertheless, in both lines and both sexes, carbohydrate was the most important nutrient for lifespan, with the trait peaking at low protein to high carbohydrate ratios, and high intake of diet. This aligns with previous studies on other insect species and C. cosyra (15, 20). In contrast to lifespan, the effects of nutrition on reproductive traits differed between control and upward-selected flies. Lifetime egg production increased with the intake of both nutrients, but the contribution of protein and carbohydrate was greater in control than upward-selected flies. Differences in how nutrition affected phenotype were most pronounced for daily egg production, with an absence of peak in upward-selected lines but a peak at high P:C ratios in control flies. While both carbohydrate and protein contributed equally to the expression of daily egg production in control lines, only protein contributed to daily egg production in upward-selected ones. Thus, both laboratory adapted lines differed from non-laboratory adapted flies (assayed in a previous study) in which both macronutrients contributed to optimal expression of female reproductive traits (15).
In the control lines, the magnitude of the nutrients’ contribution to the phenotype differed between sexes, but both macronutrients were required to optimize lifespan expression. This was not the case in upward-selected lines. Carbohydrate intake alone modulated lifespan expression in upward-selected males, but in females, lifespan was positively correlated with the intake of both nutrients (although the contribution of carbohydrate was tenfold greater than the contribution of protein). These differences are in contrast with a previous study using non laboratory adapted C. cosyra (15), where protein was detrimental to lifespan, and the magnitude of this deleterious effect was greater in males than females. Here, those differences occurred regardless of the selection regime, and therefore the differences between Malod et al. (15) and the current study might have been caused by laboratory adaptation of the fly colonies. In addition, while the proportion of protein and carbohydrate ingested by the laboratory adapted lines from this study were similar to those of wild flies (15), there was a noticeable change in nutrient preference across the two studies. Wild marula flies show a clear preference for protein over carbohydrate (15), whereas this pattern has been lost in both lines of the current study. Instead, control flies displayed a preference for carbohydrate over protein, and upward-selected flies rather preferred carbohydrate or had no preference. The increase in lifespan and total number of eggs laid by control females in comparison with wild females from Malod et al. (15) also suggests that there was laboratory adaptation. This is similar to observations in Bactrocera tryoni, where females from a long-term laboratory strain produced more eggs than wild females (38). Fruit flies rely on amino acids as a source of nitrogen (39), and given that in the wild such resources are difficult to acquire for fruit flies, it was suggested in Malod et al. (15) that the preference for protein in wild flies is an adaptation to resource scarcity. In an environment where protein is not limited, the selection pressure for protein preference may have been relaxed in favour of carbohydrate. This aligns with observations in Blatella germanica, where preference for protein in wild cockroaches is higher than in laboratory cultured ones (40).
Nutrient regulation did not differ between selected lines. Both lines regulated their nutrient intake similarly, at 1:3.6 P:C and 1:3.2 P:C for control and upward-selected flies respectively. This regulated intake point is nearly identical (1:3 P:C) to that found for non-laboratory adapted marula flies (15), and another species of the same family, Bactrocera tryoni (37). While this ratio allows C. cosyra or B. tryoni to maximise female reproductive effort, or at least get close to the optimum, with a mild to moderate cost to lifespan, this is not the case in our laboratory-selected lines. Although this ratio also allows the control flies to perform well, they do not require consumption of such a protein-biased ratio to optimise reproductive effort, which was as good on lower P:C ratios. Reproductive traits in control females can be optimised on several P:C ratios if nutrient intake is high. However, this is not the case for lifespan as it peaks at low P:C ratios with high nutrient intake. Eating towards a ratio between 1:4 and 1:3 P:C has a cost to lifespan but does not bring additional gains in reproductive effort for a control female. In the upward-selected flies, the situation is different as expression of their reproductive traits was poor and there was no peak for reproductive traits. Both selected lines ate towards a P:C ratio that has no benefit for reproductive trait expression and shortens lifespan. This may show that, despite changes in life-history strategies induced by a change in the environment (i.e., time of oviposition substrate availability), the proportion of protein and carbohydrate that an individual will target is well conserved at species level. This is even if it is no longer the best compromise in terms of lifespan or reproductive effort. This may mean that if dietary optima shift due to environmental change, organisms are not good at tracking their changing nutritional needs, as it was previously observed in African honey bees (41). An alternative explanation is that that other traits, which have not been considered in this study but are critical to fitness, benefit from this P:C ratio. That is, experimental insects were eating towards a nutrient blend that had a positive impact on other traits. One candidate is immunity, as there is a trade-off between reproduction and traits associated with immune functions (e.g. encapsulation ability in insects), and this trade-off appears to be mediated by diet (17).
Flies from upward-selected lines consistently ingested more nutrients than the control flies, whether they were in a no-choice or dietary choice situation. This is surprising, as analysis showed that trait expression increased with nutrient intake. Yet, despite consuming more nutrients, the fitness of the upward-selected flies was lower. Because both lines were fed the same diets, the increased diet ingestion observed in upward-selected flies cannot be attributed to compensatory feeding behaviours usually observed when insects are fed lower food quality (13, 37, 42). On the nutritional landscapes it appears that, while they ate more than the control flies, they never consumed enough nutrients to reach similar trait expression. It could be that the selection regime impaired nutrient assimilation in the upward-selected flies. Assimilation and nutrient allocation are essential to a good expression of life-history traits, and physiological dysfunctions in nutrient assimilation can lead to early death (43). Gut bacteria are essential to fruit fly nutrition and laboratory adaptation is known to alter gut microbiome diversity with potential deleterious consequences on fitness and life-history traits such as lifespan and reproduction (44–47). Therefore, it might be that selecting upwards on age of female reproduction negatively affected the gut microbiome. This demonstrates a more general theme, that to really understand the relationship between nutrition and phenotype requires also considering what happens after nutrient ingestion. For example, it was shown that egg resorption may be one means of salvaging nutrients when individuals consume a suboptimal nutrient blend (48). Although it is not known if C. cosyra can resorb their eggs, apoptosis has been observed in a closely related species, C. capitata (49), meaning that C. cosyra is potentially equipped for egg resorption. Furthermore, females from the Bactrocera genus reabsorb their follicles when the environment is unsuitable (e.g., temperature or absence of host), as a possible means of nutrient reallocation towards survival (50, 51). This may be one means by which female C. cosyra buffer the impacts of consuming nutritionally imbalanced or inadequate foods.
These data demonstrate how a change in reproductive scheduling induced by environmental variation in resources acts on nutrient requirements and regulation. The environment we created, with a substrate for reproduction available at different ages between selected lines, did not affect nutritional requirements. In a world where environmental conditions are changing and host plant availability may shift, this suggests that nutritional requirements for herbivorous insects, such as C. cosyra, do not necessarily need to evolve. Furthermore, the amount of nutrient ingested was higher in upward-selected flies, although they had an overall fitness lower than flies that were not selected upwards. We suggest that this higher food intake might be a behavioural response to physiological dysfunctions caused by laboratory adaptation, but it might also be that the higher nutrient intake is necessary to meet nutrient requirements in other life-history traits that we have not measured. Further investigation is needed to understand how life-history strategies may affect nutrition. For example, downward-selection for age of female reproduction (i.e., an environment with an oviposition substrate more frequently available) in the marula fly reduces lifespan and improves early reproductive effort (25). Therefore, investigations using other types of selection pressure while measuring more life-history traits may bring valuable information on how well conserved the relationship between ingested nutrients and trait expression is and how it connects to temporal fluctuations of the environment.
Data availability statement
The raw data supporting the conclusions of this article will be made available by the authors, without undue reservation.
Author contributions
CA, CW and SN conceived the ideas and designed methodology. KM and JH conducted data analysis. KM collected laboratory data. KM led the writing of the manuscript. All authors contributed to the article and approved the submitted version.
Funding
KM was supported by a National Research Foundation Competitive Programme for Rated Researchers grant awarded to CW, CA and SN (No:93686). JH was funded by a grant from the Australian Research Council (DP180101708).
Acknowledgments
We thank Dr. James Rapkin who provided rapid help with the determination of the nutritional optima. We thank Dr. Aruna Manrakhan, Rooikie Beck and Dr. Charmaine Theron who collected fruit to establish the laboratory culture and provided larval diet. We also thank Eileen Engelbrecht and Claudia Loucas for assistance with maintenance of the experiment.
Conflict of interest
The authors CW, RA and KV declared that they were an editorial board member of Frontiers, at the time of submission. This had no impact on the peer review process and the final decision.
The remaining authors declare that the research was conducted in the absence of any commercial or financial relationships that could be constructed as a potential conflict of interest.
Publisher’s note
All claims expressed in this article are solely those of the authors and do not necessarily represent those of their affiliated organizations, or those of the publisher, the editors and the reviewers. Any product that may be evaluated in this article, or claim that may be made by its manufacturer, is not guaranteed or endorsed by the publisher.
Supplementary material
The Supplementary Material for this article can be found online at: https://www.frontiersin.org/articles/10.3389/finsc.2023.1166753/full#supplementary-material
References
1. Varpe Ø. Life history adaptations to seasonality. Integr Comp Biol (2017) 57(5):943–60. doi: 10.1093/icb/icx123
2. McElderry RM. Seasonal life history trade-offs in two leafwing butterflies: delaying reproductive development increases life expectancy. J Insect Physiol (2016) 87:30–4. doi: 10.1016/j.jinsphys.2016.02.006
3. Tasnin MS, Bode M, Merkel K, Clarke AR. A polyphagous, tropical insect herbivore shows strong seasonality in age-structure and longevity independent of temperature and host availability. Sci Rep (2021) 11(1):11410. doi: 10.1038/s41598-021-90960-7
4. Clarke AR, Leach P, Measham PF. The fallacy of year-round breeding in polyphagous tropical fruit flies (Diptera: tephritidae): evidence for a seasonal reproductive arrestment in Bactrocera species. Insects (2022) 13(10):882. doi: 10.3390/insects13100882
5. Manrakhan A, Lux SA. Contribution of natural food sources to reproductive behaviour, fecundity and longevity of Ceratitis cosyra, C. fasciventris and C. capitata (Diptera: tephritidae). Bull entomological Res (2006) 96(3):259–68. doi: 10.1079/BER2006421
6. Taylor PW, Pérez-Staples D, Weldon CW, Collins SR, Fanson BG, Yap S, et al. Post-teneral nutrition as an influence on reproductive development, sexual performance and longevity of Queensland fruit flies. J Appl Entomology (2013) 137(s1):113–25. doi: 10.1111/j.1439-0418.2011.01644.x
7. Carey JR, Liedo P, Müller H-G, Wang J-L, Vaupel JW. Dual modes of aging in Mediterranean fruit fly females. Science (1998) 281(5379):996–8. doi: 10.1126/science.281.5379.996
8. Yap S, Fanson BG, Taylor PW. Mating reverses actuarial aging in female Queensland fruit flies. PloS One (2015) 10(7):e0132486. doi: 10.1371/journal.pone.0132486
9. Piao S, Liu Q, Chen A, Janssens IA, Fu Y, Dai J, et al. Plant phenology and global climate change: current progresses and challenges. Global Change Biol (2019) 25(6):1922–40. doi: 10.1111/gcb.14619
10. Freimuth J, Bossdorf O, Scheepens JF, Willems FM. Climate warming changes synchrony of plants and pollinators. Proc R Soc B: Biol Sci (2022) 289(1971):20212142. doi: 10.1098/rspb.2021.2142
11. Simpson SJ, Raubenheimer D. A multi-level analysis of feeding behaviour: the geometry of nutritional decisions. Philos Trans R Soc London B Biol Sci (1993) 342:381–402. doi: 10.1098/rstb.1993.0166
12. Jensen K, Mayntz D, Toft S, Clissold F, Hunt J, Raubenheimer D, et al. Optimal foraging for specific nutrients in predatory beetles. Proc R Soc Biol Sci Ser B (2012) 279:2212–8. doi: 10.1098/rspb.2011.2410
13. Lee KP, Simpson SJ, Clissold FJ, Brooks R, Ballard JWO, Taylor PW, et al. Lifespan and reproduction in Drosophila: new insights from nutritional geometry. Proc Natl Acad Sci United States America Biol Sci (2008) 105(7):2498–503. doi: 10.1073/pnas.0710787105
14. Maklakov AA, Simpson SJ, Zajitschek F, Hall MD, Dessmann J, Clissold F, et al. Sex-specific fitness effects of nutrient intake on reproduction and lifespan. Curr Biol (2008) 18(14):1062–6. doi: 10.1016/j.cub.2008.06.059
15. Malod K, Archer CR, Hunt J, Nicolson SW, Weldon CW. Effects of macronutrient intake on the lifespan and fecundity of the marula fruit fly, Ceratitis cosyra (Tephritidae): extreme lifespan in a host specialist. Ecol Evol (2017) 7(22):9808–17. doi: 10.1002/ece3.3543
16. Rapkin J, Archer CR, Grant CE, Jensen K, House CM, Wilson AJ, et al. Little evidence for intralocus sexual conflict over the optimal intake of nutrients for life span and reproduction in the black field cricket Teleogryllus commodus. Evolution (2017) 71(9):2159–77. doi: 10.1111/evo.13299
17. Rapkin J, Jensen K, Archer CR, House CM, Sakaluk SK, del Castillo E, et al. The geometry of nutrient space–based life-history trade-offs: sex-specific effects of macronutrient intake on the trade-off between encapsulation ability and reproductive effort in decorated crickets. Am Nat (2018) 191(4):452–74. doi: 10.1086/696147
18. Nakagawa S, Lagisz M, Hector KL, Spencer HG. Comparative and meta-analytic insights into life extension via dietary restriction. Aging Cell (2012) 11(3):401–9. doi: 10.1111/j.1474-9726.2012.00798.x
19. Simpson SJ, Le Couteur DG, Raubenheimer D, Solon-Biet S, Cooney GJ, Cogger VC, et al. Dietary protein, aging and nutritional geometry. Ageing Res Rev (2017) 39:78–86. doi: 10.1016/j.arr.2017.03.001
20. Le Couteur DG, Solon-Biet S, Cogger VC, Mitchell SJ, Senior A, de Cabo R, et al. The impact of low-protein high-carbohydrate diets on aging and lifespan. Cell Mol Life Sci (2016) 73(6):1237–52. doi: 10.1007/s00018-015-2120-y
21. Jensen K, Mcclure C, Priest N, Hunt J. Sex-specific effects of protein and carbohydrate intake on reproduction but not lifespan in Drosophila melanogaster. Aging Cell (2015) 14(4):605–15. doi: 10.1111/acel.12333
22. Laiolo P, Obeso JR. Life-history responses to the altitudinal gradient. In: Catalan J, Ninot JM, Aniz MM, editors. High mountain conservation in a changing world. Cham: Springer International Publishing (2017). p. 253–83.
23. Valcu M, Dale J, Griesser M, Nakagawa S, Kempenaers B. Global gradients of avian longevity support the classic evolutionary theory of ageing. Ecography (2014) 37(10):930–8. doi: 10.1111/ecog.00929
24. Hosking CJ, Raubenheimer D, Charleston MA, Simpson SJ, Senior AM. Macronutrient intakes and the lifespan-fecundity trade-off: a geometric framework agent-based model. J R Soc Interface (2019) 16(151):20180733. doi: 10.1098/rsif.2018.0733
25. Malod K, Roets PD, Bosua H, Archer CR, Weldon CW. Selecting on age of female reproduction affects lifespan in both sexes and age-dependent reproductive effort in female (but not male) Ceratitis cosyra. Behav Ecol Sociobiology (2021) 75(8):123. doi: 10.1007/s00265-021-03063-8
26. Roets PD, Bosua H, Archer CR, Weldon CW. Life history and demographic traits of the marula fruit fly, Ceratitis cosyra (Walker) (Diptera: tephritidae): potential consequences of host specialisation. Physiol Entomology (2018) 43(4):259–67. doi: 10.1111/phen.12255
27. Pogue T, Malod K, Weldon CW. Patterns of remating behaviour in Ceratitis (Diptera: tephritidae) species of varying lifespan. Front Physiol (2022) 13:824768. doi: 10.3389/fphys.2022.824768
28. Malod K, Roets PD, Oosthuizen C, Blount JD, Archer CR, Weldon CW. Selection on age of female reproduction in the marula fruit fly, Ceratitis cosyra (Walker) (Diptera: tephritidae), decreases total antioxidant capacity and lipid peroxidation. J Insect Physiol (2020) 104084. doi: 10.1016/j.jinsphys.2020.104084
29. Rapkin J, Jensen K, Lane SM, House CM, Sakaluk SK, Hunt J. Macronutrient intake regulates sexual conflict in decorated crickets. J Evolutionary Biol (2015) 29(2):395–406. doi: 10.1111/jeb.12794
30. Bunning H, Bassett L, Clowser C, Rapkin J, Jensen K, House CM, et al. Dietary choice for a balanced nutrient intake increases the mean and reduces the variance in the reproductive performance of male and female cockroaches. Ecol Evol (2016) 6(14):4711–30. doi: 10.1002/ece3.2243
31. Brooks ME, Kristensen K, van Benthem KJ, Magnusson A, Berg CW, Nielsen A, et al. glmmTMB balances speed and flexibility among packages for zero-inflated generalized linear mixed modeling. R J (2017) 9(2):378–400. doi: 10.32614/RJ-2017-066
32. Nychka D, Furrer R, Sain S. Fields: tools for spatial data. R Package version 8.2-1. (2015). doi: 10.5065/D6W957CT
33. del Castillo E, Chen P, Meyers A, Hunt J, Rapkin J. Confidence regions for the location of response surface optima: the r package OptimaRegion. Commun Stat - Simulation Comput (2022) 51(12):7074–94. doi: 10.1080/03610918.2020.1823412
34. Ng SH, Simpson SJ, Simmons LW. Macronutrients and micronutrients drive trade-offs between male pre- and postmating sexual traits. Funct Ecol (2018) 32(10):2380–94. doi: 10.1111/1365-2435.13190
35. Carey MR, Archer CR, Rapkin J, Castledine M, Jensen K, House CM, et al. Mapping sex differences in the effects of protein and carbohydrates on lifespan and reproduction in Drosophila melanogaster: is measuring nutrient intake essential? Biogerontology (2022) 23(1):129–44. doi: 10.1007/s10522-022-09953-2
36. Pyke GH. Optimal foraging theory: an introduction. In: Choe JC, editor. Encyclopedia of animal behavior (Second edition). Oxford: Academic Press (2019). p. 111–7.
37. Fanson BG, Weldon CW, Perez-Staples D, Simpson SJ, Taylor PW. Nutrients, not caloric restriction, extend lifespan in Queensland fruit flies (Bactrocera tryoni). Aging Cell (2009) 8(5):514–23. doi: 10.1111/j.1474-9726.2009.00497.x
38. Meats A, Holmes HM, Kelly GL. Laboratory adaptation of Bactrocera tryoni (Diptera: tephritidae) decreases mating age and increases protein consumption and number of eggs produced per milligram of protein. Bull Entomological Res (2004) 94(6):517–24. doi: 10.1079/BER2004332
39. Hendrichs J, Lauzon CR, Cooley SS, Prokopy RJ. Contribution of natural food sources to adult longevity and fecundity of Rhagoletis pomonella (Diptera: tephritidae). Ann Entomological Soc America (1993) 86(3):250–64. doi: 10.1093/aesa/86.3.250
40. McPherson S, Wada-Katsumata A, Hatano E, Silverman J, Schal C. Comparison of diet preferences of laboratory-reared and apartment-collected German cockroaches. J Economic Entomology (2021) 114(5):2189–97. doi: 10.1093/jee/toab139
41. Archer CR, Pirk CWW, Wright GA, Nicolson SW. Nutrition affects survival in African honeybees exposed to interacting stressors. Funct Ecol (2014) 28(4):913–23. doi: 10.1111/1365-2435.12226
42. Fanson BG, Taylor PW. Protein:carbohydrate ratios explain life span patterns found in Queensland fruit fly on diets varying in yeast:sugar ratios. AGE (2012) 34(6):1361–8. doi: 10.1007/s11357-011-9308-3
43. Huang J-H, Jing X, Douglas AE. The multi-tasking gut epithelium of insects. Insect Biochem Mol Biol (2015) 67:15–20. doi: 10.1016/j.ibmb.2015.05.004
44. Akami M, Ren X-M, Qi X, Mansour A, Gao B, Cao S, et al. Symbiotic bacteria motivate the foraging decision and promote fecundity and survival of Bactrocera dorsalis (Diptera: tephritidae). BMC Microbiol (2019) 19(1):229. doi: 10.1186/s12866-019-1607-3
45. Woruba DN, Morrow JL, Reynolds OL, Chapman TA, Collins DP, Riegler M. Diet and irradiation effects on the bacterial community composition and structure in the gut of domesticated teneral and mature Queensland fruit fly, Bactrocera tryoni (Diptera: tephritidae). BMC Microbiol (2019) 19(1):281. doi: 10.1186/s12866-019-1649-6
46. Majumder R, Sutcliffe B, Adnan SM, Mainali B, Dominiak BC, Taylor PW, et al. Artificial larval diet mediates the microbiome of Queensland fruit fly. Front Microbiol (2020) 11:576156. doi: 10.3389/fmicb.2020.576156
47. Roque-Romero L, Guillén-Navarro K, Zarza E, Montoya P, Liedo P. Bacterial diversity associated with Anastrepha obliqua males change under mass-rearing conditions and with irradiation. Curr Microbiol (2022) 80(1):26. doi: 10.1007/s00284-022-03111-0
48. Ruth Archer C, Bunning H, Rapkin J, Jensen K, Moore PJ, House CM, et al. Ovarian apoptosis is regulated by carbohydrate intake but not by protein intake in speckled cockroaches. J Insect Physiol (2022) 143:104452. doi: 10.1016/j.jinsphys.2022.104452
49. Velentzas A, Nezis I, Stravopodis D, Papassideri I, Margaritis L. Stage-specific regulation of programmed cell death during oogenesis of the medfly Ceratitis capitata (Diptera, tephritidae). Int J Dev Biol (2007) 51(1):57–66. doi: 10.1387/ijdb.062164av
50. Fletcher BS, Pappas S, Kapatos E. Changes in the ovaries of olive flies (Dacus oleae (Gmelin)) during the summer, and their relationship to temperature, humidity and fruit availability. Ecol Entomology (1978) 3(2):99–107. doi: 10.1111/j.1365-2311.1978.tb00908.x
Keywords: nutritional geometry, Tephritidae, life-history strategy, trade-off, lifespan, experimental selection
Citation: Malod K, Archer CR, Hunt J, Nicolson SW and Weldon CW (2023) Selection on female reproductive schedules in the marula fly, Ceratitis cosyra (Diptera: Tephritidae) affects dietary optima for female reproductive traits but not lifespan. Front. Insect Sci. 3:1166753. doi: 10.3389/finsc.2023.1166753
Received: 15 February 2023; Accepted: 28 April 2023;
Published: 17 May 2023.
Edited by:
Anita Giglio, University of Calabria, ItalyReviewed by:
Frank Chidawanyika, International Centre of Insect Physiology and Ecology (ICIPE), KenyaLarissa Guillen, Instituto de Ecología (INECOL), Mexico
Copyright © 2023 Malod, Archer, Hunt, Nicolson and Weldon. This is an open-access article distributed under the terms of the Creative Commons Attribution License (CC BY). The use, distribution or reproduction in other forums is permitted, provided the original author(s) and the copyright owner(s) are credited and that the original publication in this journal is cited, in accordance with accepted academic practice. No use, distribution or reproduction is permitted which does not comply with these terms.
*Correspondence: Christopher W. Weldon, cwweldon@zoology.up.ac.za
†Present address: Kevin Malod, Department of Conservation Ecology and Entomology, Faculty of AgriSciences, Stellenboch University, Stellenbosch, South Africa
‡Deceased