- 1Shanghai Municipal Hospital of Traditional Chinese Medicine, Shanghai University of Traditional Chinese Medicine, Shanghai, China
- 2Shaanxi University of Chinese Medicine, Xianyang, Shaanxi, China
Background: Emerging evidence suggests a correlation between the lymphocyte-monocyte ratio (LMR) and the prognosis in patients with gastric cancer (GC) undergoing immune checkpoint inhibitor (ICI) therapy. Nevertheless, the existing findings remain contentious.
Methods: A comprehensive search of literature was conducted in databases including PubMed, Embase, Web of Science, and the Cochrane Library, spanning from the inception of each database to August 30, 2023 to collect studies exploring the interplay between LMR and clinical outcomes. Eligible studies were selected following predefined inclusion and exclusion criteria. Primary outcomes encompassed progression-free survival (PFS) and overall survival (OS), which were estimated using hazard ratios (HR) and corresponding 95% confidence intervals (CI).
Results: Our analysis incorporated eight cohort studies, involving 815 patients. Aggregate data revealed associations between an elevated LMR at baseline and prolonged PFS (HR=0.58; 95% CI: 0.47–0.71, p<0.00001) and improved OS (HR=0.51, 95% CI: 0.33–0.79; p=0.003). Furthermore, LMR exhibited a favorable association with PFS after treatment (HR=0.48; 95% CI: 0.29–0.79; p= 0.004), while such a correlation was not evident in the OS analysis. Importantly, a high level of LMR was associated with prolonged PFS across varying sample sizes, follow-up duration, treatment combinations, line of therapy, and cut-off values.
Conclusion: A high pre-treatment LMR is associated with improved OS and PFS in GC patients treated with ICIs. LMR emerges as a potent biomarker for prognostic assessment in these patients, offering valuable insights for informed treatment decisions within the domain of GC immunotherapy.
Systematic review registration: PROSPERO, identifier CRD42021228512
1 Introduction
Despite the decline in the incidence of gastric cancer (GC) in recent decades, it continues to pose a substantial global health challenge, standing as the fifth most prevalent malignancy globally and the third foremost cause of mortality related to cancer, especially in East Asia (1). Recent years have witnessed a transformative shift in cancer treatment with the introduction of immune checkpoint inhibitors (ICIs), such as monoclonal antibodies targeting programmed cell death ligand-1 (PD-L1) and programmed cell death-1 (PD-1). These ICIs suppress and harness the immune checkpoint pathway to combat cancer cells (2–4). Noteworthy among these advancements is pembrolizumab, a PD-1 inhibitor, which has shown substantial efficacy as a standalone treatment for advanced gastric cancer patients who have undergone at least two prior therapeutic approaches, achieving an objective response rate (ORR) of 11.6% (95% confidence interval [CI]: 8.0%–16.1%) (5). Furthermore, a study exhibited a notable improvement in survival rates when comparing nivolumab, another PD-1 inhibitor, to a placebo (HR=0.63, 95% CI: 0.51–0.78) among patients with advanced gastric cancer who had received two or more lines of therapy (6). However, it is crucial to recognize that although ICIs offer enduring anti-tumor effects, they also come with the potential for severe toxicity and substantial treatment costs. Therefore, the identification of the patients most likely to benefit from ICI therapy holds paramount importance (7). Nevertheless, the pursuit of effective biomarkers capable of predicting immunotherapy outcomes remains a challenge in contemporary clinical practice.
It is increasingly acknowledged that tumor development is influenced not only by tumor-specific factors but also by the host’s immune status, as the systemic inflammatory response of the host is essential for processes like tumor development, angiogenesis, and disease progression (8–10). Systemic inflammation can be evaluated by examining changes in the cellular composition of the peripheral blood, including lymphocytes, monocytes, neutrophils, and platelets. through metrics such as the systemic immunoinflammatory index (SII), the platelet-lymphocyte ratio (PLR), and the lymphocyte-monocyte ratio (LMR) (11). Numerous studies have established significant correlations between these biomarkers and survival in various malignant tumors. For instance, elevated PLR, along with reduced LMR, have consistently been associated with poorer prognosis in conditions like lung cancer, colorectal cancer, and melanoma (12–14). Additionally, several investigations have confirmed the significant prognostic role of LMR in predicting adverse survival outcomes in GC patients who have undergone radical resection or chemotherapy (15, 16). Nonetheless, the utilization of LMRs in the context of GC immunotherapy remains an area that requires further exploration.
While meta-analyses have been published examining the impact of LMR on the prognosis of GC patients who have undergone curative resection (17), no comprehensive reviews have been conducted to assess the prognostic impact of LMR in GC patients who received ICI treatment to date. Consequently, this meta-analysis was undertaken to assess the prognostic impact of LMR.
2 Materials and methods
2.1 Literature search
The reporting of this study followed the guidelines outlined in the Preferred Reporting Items for Systematic Reviews and Meta-Analyses (PRISMA2020) statement (18), and the research protocol was registered on the International Prospective Systematic Evaluation Registry (PROSPERO: CRD42021228512). Two investigators, MPP and ZYR, were responsible for crafting the search strategy. They independently developed subject terms and keywords for the search of multiple databases including PubMed, Embase, Web of Science, and the Cochrane Library, covering the period from the inception of the databases to August 30, 2023. The search used a wide range of terms such as “gastric cancer,” “gastric carcinoma,” “gastric tumor,” “stomach neoplasms,” “stomach tumor,” “Immune Checkpoint Blockade,” “Immune Checkpoint Inhibitor,” “PD-L1 Inhibitors,” “PD 1 Inhibitors,” “Programmed Death-Ligand 1 Inhibitors,” “CTLA-4 Inhibitors,” “Cytotoxic T-Lymphocyte-Associated Protein 4 Inhibitors,” “pembrolizumab,” “nivolumab,” “tremelimumab,” “avelumab,” “sintilimab,” “ipilimumab,” “Lymphocytes,” “Monocytes,” “monocyte lymphocyte ratio(MLR),” and “lymphocyte monocyte ratio(LMR).” Supplementary Table S1 presents the literature search strategy.
2.2 Study selection
Studies eligible for inclusion in our analysis should meet the following criteria: (1) Patients were diagnosed with GC through pathologic observation; (2) ICIs were administered either as a monotherapy or in combination; (3) Studies focused on the assessment of the prognostic impact of LMR on PFS or OS; (4) Studies provided data on the risk ratio (HR) with a 95% confidence interval (CI), which could be either extracted directly from studies or computed based on available data; (5) Patients were categorized into high-LMR and low-LMR groups according to specified cut-off values; (6) Studies have been fully published. In contrast, the exclusion criteria are as follows: (1) Reviews, comments, meeting abstracts case reports, and letters were excluded; (2) Literature lacking sufficient information to compute HR and 95% CI were not considered; (3) Studies not providing survival data were removed; (4) Studies with data that were duplicated or overlapping were excluded.
Two researchers (MPP and ZYR) independently reviewed the titles and abstracts of studies retrieved from databases, downloaded full-text articles, and evaluated them to obtain eligible studies. Any disagreements during the study selection process were resolved through consensus.
2.3 Data extraction
The extraction of data was carried out by two researchers, MPP and ZYR, independently. Any disagreements were settled through consensus among all co-authors. Extracted information included the name of the first author, publication year, country (study location), study type, sample size, patient age, study duration, treatment method, specific immune checkpoint inhibitors used, timing of detection, cut-off value, follow-up duration, and HRs (95% CIs) for OS and PFS. It should be noted that, in terms of studies that reported MLR data (19, 20), we took the reciprocal of related HR values and corresponding confidence intervals, and exchanged the upper and lower confidence limits to convert MLR into LMR values, so as to facilitate our statistical analysis.
2.4 Quality assessment
We utilized the Newcastle-Ottawa Quality Assessment Scale (NOS) to assess studies incorporated into our meta-analysis, where they were evaluated based on three parameters: selection, comparability, and outcomes, with a maximum score of nine points awarded to a study (21). Studies scoring between 7 and 9 were classified as having high quality (22).
2.5 statistical analysis
The pooled HRs with associated 95% CIs were calculated to evaluate the prognostic value of LMR in GC patients treated with ICIs, where MLR correlation results were converted into LMR format as necessary. Cochran’s Q test and Higgins I2 statistic were utilized for measuring heterogeneity (23). Subsequently, a random-effects model was employed for data analysis when I2 >50%; otherwise, a fixed-effects model was used. Subgroup and sensitivity analyses were conducted to validate the robustness of results related to OS and PFS. To assess the presence of publication bias, we employed funnel plots and conducted Egger’s and Begg’s tests. P<0.05 was set as the threshold for statistical significance. All statistical analyses were carried out using STATA 15.0 and Review Manager 5.4 software.
3 Results
3.1 Study characteristics
A total of 209 articles were obtained from the initial search of databases. Among them, 29 articles were removed for duplicate publication. Upon reviewing the titles and abstracts of the remaining studies, we excluded 171 studies. The full texts of nine studies were then assessed. Among these, three studies were excluded primarily due to insufficient relevant data required for survival analysis. Ultimately, this meta-analysis included six studies, encompassing a total of 815 patients (19, 20, 24–27) into (Figure 1).
Among the eligible six studies, one study was conducted in Japan, while the remaining five were conducted in China. Notably, two of the eligible articles (19, 20) included two cohort studies respectively, resulting in a total of eight cohort studies. Among them, seven cohort studies were retrospective (19, 20, 24, 26, 27), whereas the remaining one was prospective (25). All cohort studies were published in English and released between 2021 and 2022. All studies employed PD-1/PD-L1 antibodies and incorporated two groups for analysis: high-LMR and low-LMR groups. Regarding the measurement of LMR, six studies measured LMR at baseline, one evaluated LMR after treatment, and one examined both baseline and post-treatment LMR. In light of LMR evaluation, eight studies probed into the prognostic implications of LMR on OS, while seven studies delved into its prognostic significance on PFS. Table 1 presents the included studies’ characteristics.
3.2 Study quality
All eight studies scored between 7 and 8 on the NOS scale, indicating high quality (Supplementary Table S2).
3.3 Meta-analysis results
3.3.1 LMR and OS
We investigated the relationship between LMR and OS, involving eight cohort studies comprising 815 participants. Among these studies, six provided baseline LMR values exclusively, one offered post-treatment LMR data only, and one provided both baseline and post-treatment LMR values. Given the substantial heterogeneity amongst related studies (I2 = 67%, p=0.002), a random-effects model was adopted (Figure 2A). The results revealed a significant and favorable correlation of high LMR values with prolonged OS in GC patients receiving ICI therapy (HR=0.61, 95% CI: 0.40–0.92; p=0.02, Figure 2A). Subgroup analysis was conducted based on the timing of LMR measurement. The analysis results demonstrated an association between high baseline LMR values and improved OS (HR=0.51, 95% CI: 0.33–0.79; p=0.003, Figure 2A), and there was significant heterogeneity (I2 = 55%, p=0.04). No significant association was found between LMR and OS after treatment (HR=1.03, 95% CI: 0.26–4.19; p=0.96, Figure 2A).
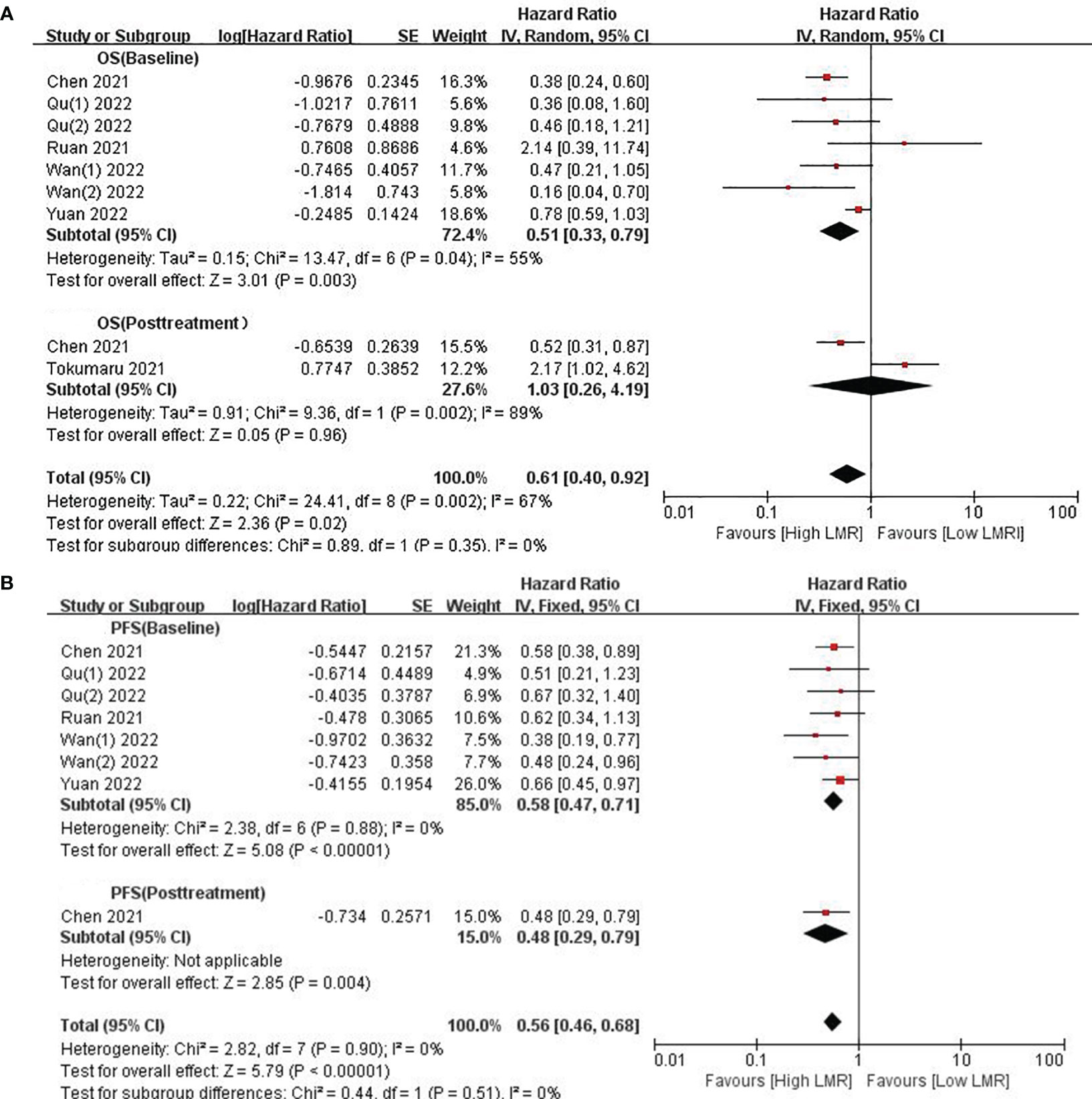
Figure 2 (A) Forest plots for the association between LMR and OS; (B) Forest plots for the association between LMR and PFS.
Given that only two studies provided post-treatment LMR data, limiting the feasibility of subgroup analysis, we proceeded with stratified analysis based on baseline LMR. To detect potential heterogeneity, we conducted subgroup analyses by sample size, follow-up time, drug combination, line of therapy, and cut-off values. Table 2 showcases the outcomes of these analyses. Firstly, in studies with a sample size < 100 (HR: 0.46; 95% CI: 0.25–0.84; p=0.01), the high-LMR group exhibited a significantly improved OS. Conversely, in studies with a sample size ≥100, no significant prognostic effect of LMR was found (HR=0.56, 95% CI: 0.28–1.13; p=0.1). Secondly, subgroup analysis based on follow-up time revealed a significantly better OS in the high-LMR group among patients followed for over 16 months compared to those receiving shorter duration of follow-up (HR=0.40, 95% CI: 0.27–0.59; p<0.00001). Thirdly, the subgroup analysis based on types of immunotherapy unveiled no significant prognostic effect of either monotherapy (P=0.38) or the combination of different ICIs (P=0.1). However, significantly improved OS was observed in participants with high LMR and who received combination therapy (HR: 0.40; 95% CI: 0.23–0.67; p=0.0006). In addition, a high LMR in GC patients receiving first-line treatment (HR=0.37; 95% CI: 0.20–0.69; p=0.002) was an important prognostic factor for favorable OS compared to GC patients receiving second-line treatment (P=0.07). Furthermore, a high LMR cut-off (≥3.0) was predictive of increased OS in GC patients (HR=0.39, 95% CI: 0.26–0.58; p<0.00001).
3.3.2 LMR and PFS
Eight studies contributed data on LMR and PFS, encompassing six articles providing baseline LMR values and one study providing baseline and post-treatment LMR. Echoing the findings from our analysis of OS, elevated LMR was found to be correlated with prolonged PFS in GC patients on ICIs (HR=0.56, 95% Cl: 0.46–0.68;p<0.00001, Figure 2B), with no substantial heterogeneity observed (I2 = 0%, p=0.90). Similarly, a higher baseline LMR was linked to enhanced PFS (HR=0.58; 95% CI: 0.47–0.71; p<0.00001, Figure 2B), with no significant heterogeneity observed (I2 = 0%, p=0.88). Only one study reported on patients with higher LMR after treatment (HR=0.48; 95% CI: 0.29–0.79; p=0.004, Figure 2B), and the results indicated that higher LMR after treatment was correlated with prolonged PFS.
Therefore, our subgroup analyses concentrated solely on baseline LMR. These analyses revealed the association between high levels of baseline LMR and improved PFS, but no significant prognostic effect was observed with monotherapy (P=0.12). Across various parameters, including sample size, follow-up time, combination of drugs, line of treatment, and cut-off value, elevated LMR was consistently correlated with improved PFS (p<0.05; Table 2), and there was no significant heterogeneity (I2 = 0%).
3.4 Sensitivity analysis
A sensitivity analysis was conducted to examine the robustness of our analysis results associated with the clinical significance of baseline LMR, revealing that the effect size remained consistent within the original range after each study was sequentially removed. This indicated that no single study disproportionately influenced the outcomes for both OS (Figure 3A) and PFS (Figure 3B), confirming the reliability of analysis results.
3.5 Publication bias
Publication bias was assessed using a funnel plot and Egger’s test. The symmetrical funnel plot suggested the absence of substantial publication bias in the meta-analysis concerning OS (Egger: p=0.36) (Figure 4A). The Egger’s test results also indicated no significant publication bias in the meta-analysis for PFS (Egger: p=0.19) (Figure 4B). However, we were unable to conduct a publication bias analysis for the remaining studies due to the limited number of studies (<3 studies).
4 Discussion
Throughout the various stages of tumor development, systemic inflammation emerges as a central player, influencing genetic mutations, genomic instability, epigenetic alterations, tumor metastasis, and the proliferation of cancer cells (28, 29). Blood-derived parameters offer a readily accessible and reproducible means of assessing systemic inflammation, serving as objective biomarkers to predict patient prognosis (30, 31). However, the evidence linking a high LMR with improved survival outcomes in these cancers has been somewhat limited (32). Initially conceived as a prognostic indicator for hematologic malignancies, the pre-treatment LMR has been demonstrated in numerous studies to reflect systemic inflammation and positively correlate with the prognoses of various solid neoplasms, such as melanoma, breast cancer, and GC (19, 20, 24–27, 33–35). Nevertheless, the precise mechanism underlying the prognostic impact of LMR on GC remains elusive. LMR hinges on the levels of lymphocytes and monocytes, which serve as indicators of anti-tumor immunity and tumor burden (36). Low lymphocyte counts can result in an impaired immune response against cancer cells, particularly tumor-infiltrating lymphocytes (TILs), which are pivotal for the cell-mediated anti-tumor immune response (37). Furthermore, increased TILs are associated with improved outcomes in cancer patients (38). The infiltration of CD4 T cells triggers the activation of CD8 T cells, instigating apoptosis and cytotoxic activity against cancer cells (39, 40). Consequently, diminished lymphocyte counts may contribute to reduced survival rates across various cancers (41, 42). In addition to lymphocytes, blood monocytes hold the capacity to differentiate into tumor-associated macrophages (TAMs) that play a crucial role in tumorigenesis. TAMs directly affect regulatory T-cells, impeding tumor immunity while accelerating angiogenesis and the degeneration of the extracellular matrix. These actions promote the development and progression of tumors (43, 44). As a result, TAMs can be considered an indicator of a high cancer burden. Thus, LMR is regarded as a reflection of the immune state and holds the potential to function as a prognostic indicator for the effectiveness of ICIs.
This meta-analysis involved 815 patients and aimed to evaluate the prognostic significance of LMR in GC patients on ICIs. Significant positive associations were observed between baseline LMR and OS, as well as between OS and PFS. It’s worth noting that only one study in our analysis reported an improvement in PFS for patients with a high LMR after treatment. However, the correlation between OS and post-treatment LMR was not as evident. This discrepancy could be attributed to the scarcity of studies providing data on post-treatment LMR and the variability in the timing of LMR assessments, spanning from 2 to 6 weeks after the initial dosage. Evidence has shown the “true” mobilization time of activated white blood cells into the bloodstream typically requires at least 4 weeks (45). This observation may help clarify the inconsistent conclusions observed in articles reporting on post-treatment LMR. Therefore, future research could examine whether variations in the timing of post-treatment LMR assessment indeed impact clinical outcomes and whether changes in LMR before and after ICI administration correlate with patient prognosis. In our efforts to provide a more nuanced analysis, we conducted subgroup assessments of various treatments to explore the relationship between a high LMR and both OS and PFS. Our analysis results were deemed reliable following the publication bias test. To the best of our knowledge, this meta-analysis is the initial endeavor to examine the predictive significance of LMR in GC patients who received ICI treatment. Our findings bear significant implications for the clinical management of GC patients undergoing PD-1/PD-L1 antibody treatment. Specifically, our analysis results suggest that GC patients with a low LMR before treatment may face an elevated risk of cancer progression or relapse following initial chemotherapy. This underscores the importance of close monitoring and follow-up for these patients. Notably, in the study by Ruan et al. (25), the univariate analysis revealed that similar predictive performance of LMR for OS compared to other studies (HR=0.27, 95% CI: 0.12-0.61), whereas the multivariate analysis unraveled opposite results on OS(HR=2.14, 95%CI: 0.39-11.69).
Meta-analyses of various studies have consistently highlighted the promising prognostic potential of LMR in specific cancer patient populations undergoing ICI treatment. For instance, a recent meta-analysis that encompassed 21 studies illuminated the potential of a high pre-treatment LMR as a robust prognostic biomarker for advanced cancer patients receiving immunotherapy (46). Our meta-analysis, wherein we amalgamated data from 815 patients, affirmed the significant prognostic significance of LMR in GC patients on ICIs. This finding aligns with previous prior research on the prognostic significance of LMR in diverse tumor types.
Our meta-analysis, albeit informative, does come with some limitations that warrant consideration. Firstly, all eligible studies in our analysis were conducted in Asia, specifically China and Japan. Consequently, our conclusions should be interpreted within this geographical context, and prudence is warranted when extrapolating our findings to patients residing in Europe, Africa, the Americas, and other regions. Indeed, additional research is necessary to validate the prognostic relevance of LMR in non-Asian GC patients undergoing ICI treatment. Secondly, the majority of the studies included in our analysis adopted a retrospective design rather than a prospective one. This retrospective nature introduces the potential for confounding factors that may influence the reliability of our results. Furthermore, the variability in LMR cut-off values employed across the included studies is another limitation. These cut-off values ranged from 2.8 to 5, potentially introducing inherent heterogeneity into our meta-analysis due to data inconsistencies. To foster greater reliability and comparability in future studies, it is essential that researchers establish a standardized cut-off value for LMR.
In summary, our meta-analysis reveals that high pre-treatment LMR is significantly correlated with favorable outcomes, such as prolonged OS and PFS, in GC patients treated with ICIs. This indicates that LMR could serve as an independent and informative prognostic biomarker for GC, thereby aiding in making informed treatment decisions concerning immunotherapy for GC. However, given the limitations inherent in the studies incorporated into our analysis, additional prospective trials are required to substantiate our findings across diverse ethnicities and geographical regions.
Data availability statement
The original contributions presented in the study are included in the article/Supplementary Material. Further inquiries can be directed to the corresponding authors.
Author contributions
PM: Conceptualization, Data curation, Formal analysis, Methodology, Software, Writing – original draft. WF: Conceptualization, Investigation, Supervision, Writing – review & editing. YZ: Data curation, Methodology, Supervision, Writing – review & editing. XG: Funding acquisition, Project administration, Resources, Supervision, Writing – review & editing.
Funding
The author(s) declare financial support was received for the research, authorship, and/or publication of this article. This study was funded by the National Natural Science Foundation of China (81873308 and 82305230); Shanghai University of Traditional Chinese Medicine Industrial Development Center Combined Medical and Nursing Science and Technology Innovation Project (YYKC-2021-01-14); Future Plan for Traditional Chinese Medicine development of Science and Technology of Shanghai Municipal Hospital of Traditional Chinese Medicine (WL-HBQN-2122013K); Future Plan for Traditional Chinese Medicine Inheritance and Development of Shanghai Municipal Hospital of Traditional Chinese Medicine (WLJH2021ZY-MZY024 and WLJH2021ZY-GZS003); Shanghai Municipal Health Commission Project (ZY 2021-2023-0204-01).
Conflict of interest
The authors declare that the research was conducted in the absence of any commercial or financial relationships that could be construed as a potential conflict of interest.
Publisher’s note
All claims expressed in this article are solely those of the authors and do not necessarily represent those of their affiliated organizations, or those of the publisher, the editors and the reviewers. Any product that may be evaluated in this article, or claim that may be made by its manufacturer, is not guaranteed or endorsed by the publisher.
Supplementary material
The Supplementary Material for this article can be found online at: https://www.frontiersin.org/articles/10.3389/fimmu.2023.1321584/full#supplementary-material
References
1. Sung H, Ferlay J, Siegel RL, Laversanne M, Soerjomataram I, Jemal A, et al. Global cancer statistics 2020: GLOBOCAN estimates of incidence and mortality worldwide for 36 cancers in 185 countries. CA Cancer J Clin (2021) 71(3):209–49. doi: 10.3322/caac.21660
2. Kotecha R, Miller JA, Venur VA, Mohammadi AM, Chao ST, Suh JH, et al. Melanoma brain metastasis: the impact of stereotactic radiosurgery, BRAF mutational status, and targeted and/or immune-based therapies on treatment outcome. J Neurosurg (2018) 129(1):50–9. doi: 10.3171/2017.1.JNS162797
3. Sun Q, Hong Z, Zhang C, Wang L, Han Z, Ma D. Immune checkpoint therapy for solid tumours: clinical dilemmas and future trends. Signal Transduct Target Ther (2023) 8(1):320. doi: 10.1038/s41392-023-01522-4
4. Muro K, Chung HC, Shankaran V, Geva R, Catenacci D, Gupta S, et al. Pembrolizumab for patients with PD-L1-positive advanced gastric cancer (KEYNOTE-012): a multicentre, open-label, phase 1b trial. Lancet Oncol (2016) 17(6):717–26. doi: 10.1016/S1470-2045(16)00175-3
5. Fuchs CS, Doi T, Jang RW, Muro K, Satoh T, MaChado M, et al. Safety and efficacy of pembrolizumab monotherapy in patients with previously treated advanced gastric and gastroesophageal junction cancer: phase 2 clinical KEYNOTE-059 trial. JAMA Oncol (2018) 4(5):e180013. doi: 10.1001/jamaoncol.2018.0013
6. Kang YK, Boku N, Satoh T, Ryu MH, Chao Y, Kato K, et al. Nivolumab in patients with advanced gastric or gastro-oesophageal junction cancer refractory to, or intolerant of, at least two previous chemotherapy regimens (ONO-4538-12, ATTRACTION-2): a randomised, double-blind, placebo-controlled, phase 3 trial. Lancet (2017) 390(10111):2461–71. doi: 10.1016/S0140-6736(17)31827-5
7. Bhardwaj PV, Abdou YG. The evolving landscape of immune checkpoint inhibitors and antibody drug conjugates in the treatment of early-stage breast cancer. Oncologist (2023) 28(10):832–44. doi: 10.1093/oncolo/oyad233
8. Chen DS, Mellman I. Elements of cancer immunity and the cancer-immune set point. Nature (2017) 541(7637):321–30. doi: 10.1038/nature21349
9. Leone P, Buonavoglia A, Fasano R, Solimando AG, De Re V, Cicco S, et al. Insights into the regulation of tumor angiogenesis by micro-RNAs. J Clin Med (2019) 8(12):2030. doi: 10.3390/jcm8122030
10. Zhi X, Jiang K, Shen Y, Su X, Wang K, Ma Y, et al. Peripheral blood cell count ratios are predictive biomarkers of clinical response and prognosis for non-surgical esophageal squamous cell carcinoma patients treated with radiotherapy. J Clin Lab Anal (2020) 34(10):e23468. doi: 10.1002/jcla.23468
11. Wang H, Ding Y, Li N, Wu L, Gao Y, Xiao C, et al. Prognostic value of neutrophil-lymphocyte ratio, platelet-lymphocyte ratio, and combined neutrophil-lymphocyte ratio and platelet-lymphocyte ratio in stage IV advanced gastric cancer. Front Oncol (2020) 10:841. doi: 10.3389/fonc.2020.00841
12. Guthrie GJ, Charles KA, Roxburgh CS, Horgan PG, McMillan DC, Clarke SJ. The systemic inflammation-based neutrophil-lymphocyte ratio: experience in patients with cancer. Crit Rev Oncol Hematol (2013) 88(1):218–30. doi: 10.1016/j.critrevonc.2013.03.010
13. Suh KJ, Kim SH, Kim YJ, Kim M, Keam B, Kim TM, et al. Post-treatment neutrophil-to-lymphocyte ratio at week 6 is prognostic in patients with advanced non-small cell lung cancers treated with anti-PD-1 antibody. Cancer Immunol Immunother (2018) 67(3):459–70. doi: 10.1007/s00262-017-2092-x
14. Urakawa S, Yamasaki M, Goto K, Haruna M, Hirata M, Morimoto-Okazawa A, et al. Peri-operative monocyte count is a marker of poor prognosis in gastric cancer: increased monocytes are a characteristic of myeloid-derived suppressor cells. Cancer Immunol Immunother (2019) 68(8):1341–50. doi: 10.1007/s00262-019-02366-0
15. Hsu JT, Wang CC, Le PH, Chen TH, Kuo CJ, Lin CJ, et al. Lymphocyte-to-monocyte ratios predict gastric cancer surgical outcomes. J Surg Res (2016) 202(2):284–90. doi: 10.1016/j.jss.2016.01.005
16. Lin JP, Lin JX, Cao LL, Zheng CH, Li P, Xie JW, et al. Preoperative lymphocyte-to-monocyte ratio as a strong predictor of survival and recurrence for gastric cancer after radical-intent surgery. Oncotarget (2017) 8(45):79234–47. doi: 10.18632/oncotarget.17058
17. Ma JY, Liu Q. Clinicopathological and prognostic significance of lymphocyte to monocyte ratio in patients with gastric cancer: A meta-analysis. Int J Surg (2018) 50:67–71. doi: 10.1016/j.ijsu.2018.01.002
18. Page MJ, McKenzie JE, Bossuyt PM, Boutron I, Hoffmann TC, Mulrow CD, et al. The PRISMA 2020 statement: an updated guideline for reporting systematic reviews. Bmj (2021) 372:n71. doi: 10.1136/bmj.n71
19. Qu Z, Wang Q, Wang H, Jiao Y, Li M, Wei W, et al. The effect of inflammatory markers on the survival of advanced gastric cancer patients who underwent anti-programmed death 1 therapy. Front Oncol (2022) 12:783197. doi: 10.3389/fonc.2022.783197
20. Wan M, Ding Y, Mao C, Ma X, Li N, Xiao C, et al. Association of inflammatory markers with survival in patients with advanced gastric cancer treated with immune checkpoint inhibitors combined with chemotherapy as first line treatment. Front Oncol (2022) 12:1029960. doi: 10.3389/fonc.2022.1029960
21. Wells G, Shea B, O’connell D, Peterson J, Welch V, Losos M, et al. The Newcastle–Ottawa Scale (NOS) for assessing the quality of nonrandomised studies in meta-analyses (2014). Ottawa (ON: Ottawa Health Research Institute (Accessed December 11, 2013).
22. Kim SR, Kim K, Lee SA, Kwon SO, Lee JK, Keum N, et al. Effect of red, processed, and white meat consumption on the risk of gastric cancer: an overall and dose-Response meta-analysis. Nutrients (2019) 11(4):826. doi: 10.3390/nu11040826
23. Higgins JP, Thompson SG, Deeks JJ, Altman DG. Measuring inconsistency in meta-analyses. Bmj (2003) 327(7414):557–60. doi: 10.1136/bmj.327.7414.557
24. Chen Y, Zhang C, Peng Z, Qi C, Gong J, Zhang X, et al. Association of lymphocyte-to-monocyte ratio with survival in advanced gastric cancer patients treated with immune checkpoint inhibitor. Front Oncol (2021) 11:589022. doi: 10.3389/fonc.2021.589022
25. Ruan DY, Chen YX, Wei XL, Wang YN, Wang ZX, Wu HX, et al. Elevated peripheral blood neutrophil-to-lymphocyte ratio is associated with an immunosuppressive tumour microenvironment and decreased benefit of PD-1 antibody in advanced gastric cancer. Gastroenterol Rep (Oxf) (2021) 9(6):560–70. doi: 10.1093/gastro/goab032
26. Tokumaru S, Koizumi T, Sekino Y, Takeuchi N, Nakata S, Miyagawa Y, et al. Lymphocyte-to-monocyte ratio is a predictive biomarker of response to treatment with nivolumab for gastric cancer. Oncology (2021) 99(10):632–40. doi: 10.1159/000517344
27. Yuan J, Zhao X, Li Y, Yao Q, Jiang L, Feng X, et al. The association between blood indexes and immune cell concentrations in the primary tumor microenvironment predicting survival of immunotherapy in gastric cancer. Cancers (Basel) (2022) 14(15):3608. doi: 10.3390/cancers14153608
28. Sparmann A, Bar-Sagi D. Ras-induced interleukin-8 expression plays a critical role in tumor growth and angiogenesis. Cancer Cell (2004) 6(5):447–58. doi: 10.1016/j.ccr.2004.09.028
29. Rosenberg SA. Progress in human tumour immunology and immunotherapy. Nature (2001) 411(6835):380–4. doi: 10.1038/35077246
30. Huang R, Zheng Y, Zou W, Liu C, Liu J, Yue J. Blood biomarkers predict survival outcomes in patients with hepatitis B virus-induced hepatocellular carcinoma treated with PD-1 inhibitors. J Immunol Res (2022) 2022:3781109. doi: 10.1155/2022/3781109
31. Trinh H, Dzul SP, Hyder J, Jang H, Kim S, Flowers J, et al. Prognostic value of changes in neutrophil-to-lymphocyte ratio (NLR), platelet-to-lymphocyte ratio (PLR) and lymphocyte-to-monocyte ratio (LMR) for patients with cervical cancer undergoing definitive chemoradiotherapy (dCRT). Clin Chim Acta (2020) 510:711–6. doi: 10.1016/j.cca.2020.09.008
32. Kocarnik JM, Shiovitz S, Phipps AI. Molecular phenotypes of colorectal cancer and potential clinical applications. Gastroenterol Rep (Oxf) (2015) 3(4):269–76. doi: 10.1093/gastro/gov046
33. Nishijima TF, Muss HB, Shachar SS, Tamura K, Takamatsu Y. Prognostic value of lymphocyte-to-monocyte ratio in patients with solid tumors: A systematic review and meta-analysis. Cancer Treat Rev (2015) 41(10):971–8. doi: 10.1016/j.ctrv.2015.10.003
34. Li ZM, Huang JJ, Xia Y, Sun J, Huang Y, Wang Y, et al. Blood lymphocyte-to-monocyte ratio identifies high-risk patients in diffuse large B-cell lymphoma treated with R-CHOP. PloS One (2012) 7(7):e41658. doi: 10.1371/journal.pone.0041658
35. Porrata LF, Ristow K, Colgan JP, Habermann TM, Witzig TE, Inwards DJ, et al. Peripheral blood lymphocyte/monocyte ratio at diagnosis and survival in classical Hodgkin's lymphoma. Haematologica (2012) 97(2):262–9. doi: 10.3324/haematol.2011.050138
36. Goto W, Kashiwagi S, Asano Y, Takada K, Takahashi K, Hatano T, et al. Predictive value of lymphocyte-to-monocyte ratio in the preoperative setting for progression of patients with breast cancer. BMC Cancer (2018) 18(1):1137. doi: 10.1186/s12885-018-5051-9
37. Oh SY, Kim YB, Suh KW. Prognostic significance of systemic inflammatory response in stage II colorectal cancer. J Surg Res (2017) 208:158–65. doi: 10.1016/j.jss.2016.08.100
38. Grimm M, Feyen O, Hofmann H, Teriete P, Biegner T, Munz A, et al. Immunophenotyping of patients with oral squamous cell carcinoma in peripheral blood and associated tumor tissue. Tumour Biol (2016) 37(3):3807–16. doi: 10.1007/s13277-015-4224-2
39. Chan JC, Chan DL, Diakos CI, Engel A, Pavlakis N, Gill A, et al. The lymphocyte-to-monocyte ratio is a superior predictor of overall survival in comparison to established biomarkers of resectable colorectal cancer. Ann Surg (2017) 265(3):539–46. doi: 10.1097/SLA.0000000000001743
40. Lin GN, Liu PP, Liu DY, Peng JW, Xiao JJ, Xia ZJ. Prognostic significance of the pre-chemotherapy lymphocyte-to-monocyte ratio in patients with previously untreated metastatic colorectal cancer receiving FOLFOX chemotherapy. Chin J Cancer (2016) 35:5. doi: 10.1186/s40880-015-0063-1
41. Li Y, Jia H, Yu W, Xu Y, Li X, Li Q, et al. Nomograms for predicting prognostic value of inflammatory biomarkers in colorectal cancer patients after radical resection. Int J Cancer (2016) 139(1):220–31. doi: 10.1002/ijc.30071
42. Facciorusso A, Del Prete V, Crucinio N, Serviddio G, Vendemiale G, Muscatiello N. Lymphocyte-to-monocyte ratio predicts survival after radiofrequency ablation for colorectal liver metastases. World J Gastroenterol (2016) 22(16):4211–8. doi: 10.3748/wjg.v22.i16.4211
43. Schirizzi A, De Leonardis G, Lorusso V, Donghia R, Rizzo A, Vallarelli S, et al. Targeting angiogenesis in the era of biliary tract cancer immunotherapy: biological rationale, clinical implications, and future research avenues. Cancers (Basel) (2023) 15(8):2376. doi: 10.3390/cancers15082376
44. Song A, Eo W, Lee S. Comparison of selected inflammation-based prognostic markers in relapsed or refractory metastatic colorectal cancer patients. World J Gastroenterol (2015) 21(43):12410–20. doi: 10.3748/wjg.v21.i43.12410
45. Rui R, Zhou L, He S. Cancer immunotherapies: advances and bottlenecks. Front Immunol (2023) 14:1212476. doi: 10.3389/fimmu.2023.1212476
Keywords: lymphocyte-to-monocyte ratio, gastric cancer, immune checkpoint inhibitors, prognostic value of survival, meta-analysis
Citation: Mei P, Feng W, Zhan Y and Guo X (2023) Prognostic value of lymphocyte-to-monocyte ratio in gastric cancer patients treated with immune checkpoint inhibitors: a systematic review and meta-analysis. Front. Immunol. 14:1321584. doi: 10.3389/fimmu.2023.1321584
Received: 14 October 2023; Accepted: 13 November 2023;
Published: 27 November 2023.
Edited by:
Alberto Bongiovanni, IRCCS Istituto Romagnolo per lo Studio dei Tumori (IRST) “Dino Amadori”, ItalyReviewed by:
Zhiren Li, Capital Medical University, ChinaHasan Cagri Yildirim, Hacettepe University, Türkiye
Copyright © 2023 Mei, Feng, Zhan and Guo. This is an open-access article distributed under the terms of the Creative Commons Attribution License (CC BY). The use, distribution or reproduction in other forums is permitted, provided the original author(s) and the copyright owner(s) are credited and that the original publication in this journal is cited, in accordance with accepted academic practice. No use, distribution or reproduction is permitted which does not comply with these terms.
*Correspondence: Pingping Mei, MPP1125069522@163.com; Xiutian Guo, 11210752@stu.lzjtu.edu.cn