- 1Section for Oral Biology and Immunopathology/Oral Medicine, Department of Odontology, Faculty of Health and Medical Sciences, University of Copenhagen, Copenhagen, Denmark
- 2Center for Rheumatology and Spine Diseases, University Hospital Rigshospitalet, Copenhagen, Denmark
Introduction: Sjögren’s syndrome (SS) is a systemic autoimmune disease, which affects the exocrine glands leading to glandular dysfunction and, particularly, symptoms of oral and ocular dryness. The aetiology of SS remains unclear, and the disease lacks distinctive clinical features. The current diagnostic work-up is complex, invasive and often time-consuming. Thus, there is an emerging need for identifying disease-specific and, ideally, non-invasive immunological and molecular biomarkers that can simplify the diagnostic process, allow stratification of patients, and assist in monitoring the disease course and outcome of therapeutic intervention in SS.
Methods: This systematic review addresses the use of proteomics and miRNA-expression profile analyses in this regard.
Results and discussion: Out of 272 papers that were identified and 108 reviewed, a total of 42 papers on proteomics and 23 papers on miRNA analyses in saliva, blood and salivary gland tissue were included in this review. Overall, the proteomic and miRNA studies revealed considerable variations with regard to candidate biomarker proteins and miRNAs, most likely due to variation in sample size, processing and analytical methods, but also reflecting the complexity of SS and patient heterogeneity. However, interesting novel knowledge has emerged and further validation is needed to confirm their potential role as biomarkers in SS.
1 Introduction
Sjögren’s syndrome (SS) is an autoimmune disease characterised by chronic lymphocytic infiltration of exocrine glands, particularly the salivary and lacrimal glands, leading to tissue destruction and, subsequently, glandular dysfunction. The predominant symptoms are oral and ocular dryness occurring due to hyposalivation and keratoconjunctivitis sicca, respectively, accompanied by arthralgia and fatigue (1, 2). The disease may occur alone and is then designated primary SS (pSS), or together with another autoimmune connective tissue disease, most often rheumatoid arthritis (RA), systemic lupus erythematosus (SLE) or systemic sclerosis (SSc), in which case it is designated secondary SS (sSS) (1, 2). SS can affect people of any age, but symptoms usually appear between the ages of 45 and 55. It affects ten times as many women as men (3).
The aetiology and pathogenesis of SS are not fully understood. It has been suggested that exposure to specific environmental factors (e.g. virus) in susceptible individuals results in dysregulation of the innate immune system involving the interferon (IFN) pathway (2, 4). Current evidence suggest that activated T cells are involved in the pathogenesis of SS by producing pro-inflammatory cytokines and by mediating B-cell-hyperactivity (5). The lymphocytic infiltrates observed in the salivary gland tissue mainly comprise CD4+ effector T cells, including IFN-γ-producing Th1 cells, IL-17-producing Th17 cells and IL-21-producing T follicular helper cells. Furthermore, CD4+ T-cell lymphopenia and increased number of circulating follicular helper T cells are often observed in the blood from patients with pSS (6). B-cell hyperactivity leads to autoantibody production, and in some cases, development of lymphoproliferative malignancy (2, 7). Anti-SSA/Ro and/or anti-SSB/La are found in about 70% of patients with pSS, often together with ANA (anti-nuclear antibodies) positivity. Hypergammaglobulinaemia is also prevalent as well as a positive rheumatoid factor (RF) (8). There is evidence to suggest that the salivary gland epithelial cells play a central role in regulation of the local autoimmune responses by inducing B-cell activation and survival of B cells within the target tissue (9, 10).
SS is associated with the human leukocyte antigen (HLA) class II types DR3 and DR2, particularly the DRB1*03/DQB1*02 and DRB1*15/DQB1*01 haplotypes, but only in patients having serum autoantibodies against SSA/Ro and SSB/La antigens (11–13). A recent, large study confirmed the absence of HLA association in patients with pSS negative for SSA and/SSB antibodies (14). On the basis of HLA association, presence of SSA and/or SSB autoantibodies, age of onset and clinical manifestations, it has been suggested that patients with pSS may be divided in two distinct subgroups (14).
Classification of pSS is currently based on the American College of Rheumatology-European League Against Rheumatism (ACR-EULAR) classification criteria utilizing the weighted sum of 5 objective aspects of pSS. These include anti-SSA/Ro antibody positivity, presence of focal lymphocytic sialadenitis with a focus score ≥1 in a labial salivary gland biopsy, an abnormal ocular staining score ≥5 (or van Bijsterveld score ≥4), a Schirmer’s test ≤5 mm/5 min and/or an unstimulated salivary flow rate ≤0.1 mL/min (15). None of the paraclinical findings, symptoms or disease manifestations are pathognomonic for SS, but may be observed in other autoimmune connective tissue diseases like RA and SLE (16). Furthermore, the highly variable symptomatology, autoantibody reactivity, histological features and gland functionality encompassed by the diagnosis of pSS suggest the existence of several different aetiopathogenic disease subtypes that may benefit from different treatment regimes. In order to characterize such subtypes, reliable biomarkers need to be identified. Moreover, due to the lack of distinctive clinical features and biomarkers, pSS may be diagnosed at a late stage where irreversible tissue damage has occurred. The diagnostic procedures currently in use are complicated, invasive and often time-consuming. This underlines the need for identification of new, ideally non-invasive, immunological and molecular biomarkers that can simplify the diagnostic work-up and stratification of patients and assist in monitoring the disease course and outcome of therapeutic interventions.
Recent and emerging advances within omics technologies offer opportunities to detect novel and reliable molecular diagnostic biomarkers, which can provide a higher sensitivity and specificity than the simple biomarkers and measures used today, and at the same time improve our insight into the aetiopathogenesis of SS and its subtypes. This systematic review provides an overview of current findings on proteomics and miRNA-expression profile analyses in SS, and presents the methods and biological materials used, such as saliva, blood and salivary gland tissue, for characterization of the proteomic and miRNA profiles.
2 Methods
A literature search was conducted through the PubMed, Medline and Embase databases up to January 2021 according to the standards of the Preferred Reporting Items for Systematic Reviews and Meta-Analyses (PRISMA) guidelines (Figure 1). Human studies that were published in English, without any restriction on publication date, and that examined proteomics and miRNAs in patients with SS (with and without comparison to healthy control subjects, or patients with other connective tissue diseases) using various methods and biological material (saliva, salivary gland tissue and blood) were included. Only primary publication types were included. Studies including less than 5 patients with SS were excluded. The following terms were used in combination to identify studies on proteomics in patients with SS: (“Sjögren’s syndrome”[MeSH]) AND “Proteomics”[MeSH]) OR Sjögren’s* proteomic* OR Sjögren’s proteomics OR primary Sjögren’s syndrome proteomic* OR primary Sjögren’s syndrome proteomics). The following terms were used in combination in the search of studies on miRNA expression-profile in patients with SS: “Sjögren’s syndrome”[MeSH]) AND “MicroRNAs” [MeSH]) OR primary Sjögren’s syndrome miRNA OR primary Sjögren’s syndrome microRNA.
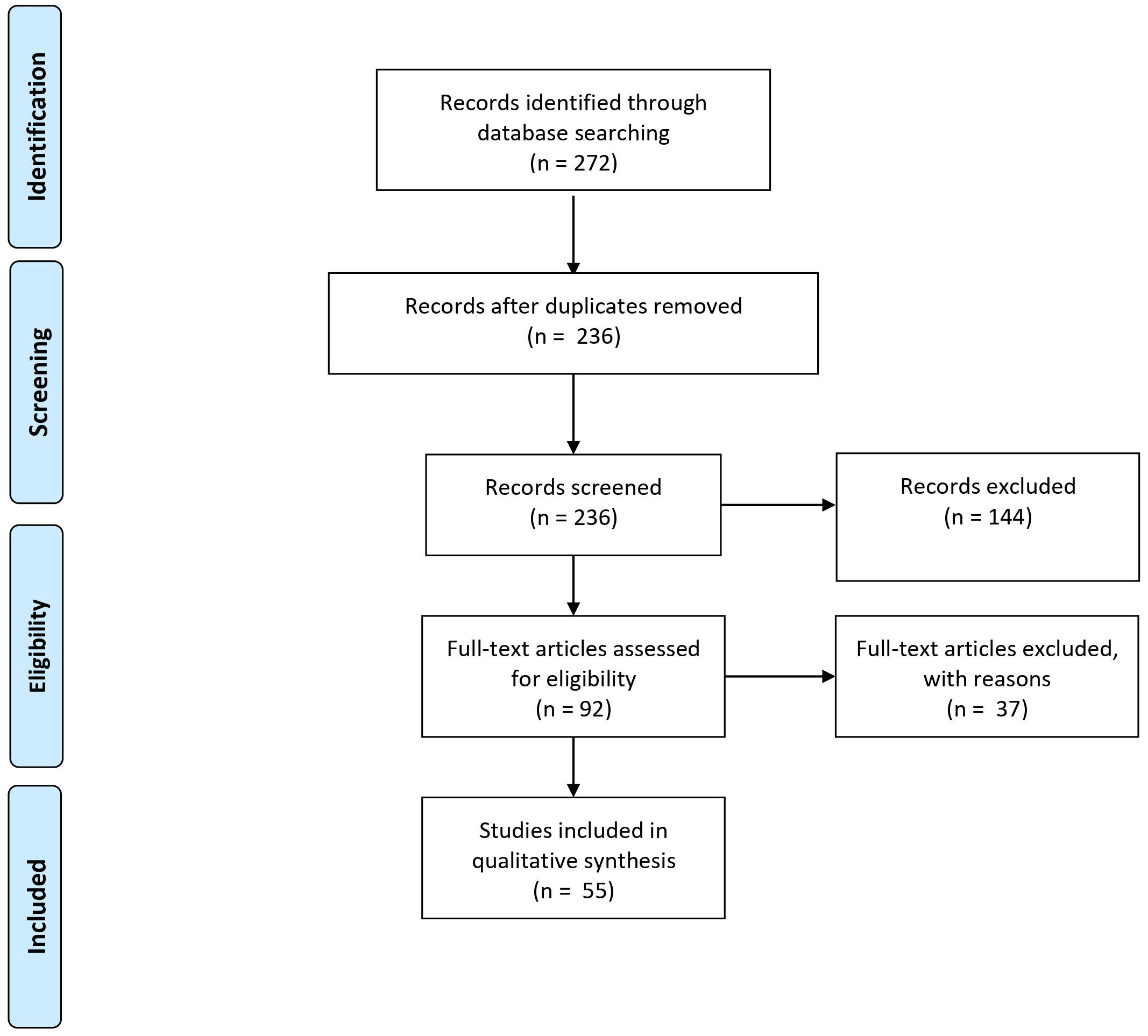
Figure 1 PRISMA diagram showing the number of records identified through database search, and the number of records screened and excluded.
Titles and abstracts were screened by two reviewers (SK and AMLP). If eligible based on the title and abstract, full-text articles were obtained when possible.
2.1 Saliva as a source of biomarkers
Saliva is an attractive source of biomarkers for diagnostic purposes, as salivary glands are the very organs affected by the disease, and saliva may reflect local and, possibly, systemic pathological changes of the disease (17–22). Furthermore, saliva samples can easily be obtained using a non-invasive, simple and safe procedure, and collection of saliva is thus ideal for monitoring of disease progression. Studies on the proteome in SS have mainly been performed on saliva, and stimulated whole saliva has proven more suitable for studying the salivary proteome than gland-specific saliva (23). There is also evidence suggesting that stimulated whole saliva contains a lower proteome variability than unstimulated whole saliva (24). In addition, the unstimulated whole saliva flow rate is often significantly reduced in patients with SS, which makes chewing-stimulated whole saliva more applicable. As the lacrimal glands are major target organs of SS, tear fluid represents another relevant biological material for identification of disease biomarkers. However, it may be challenging to obtain sufficient amount for analysis, and it is more complicated to collect than saliva (25).
2.2 Methods used for proteomic and miRNA profiling
Proteomic analysis provides insight to understand the function of proteins in health and disease. Most studies use mass spectrometry-based proteomics to obtain information on the proteome composition (e.g. shortgun gel-free proteomics) or protein interactions and structure by means of e.g. high-performance liquid chromatography-mass spectrometry (HPLC-MS), tandem mass-spectrometry (MS/MS) and matrix-assisted laser/ionization time-of-flight MS/MS (MALDI-TOF MS/MS). The methods most commonly applied for purification of proteins include absorbance colorimetry, liquid chromatography (LC), native and denaturing one-dimensional and two-dimensional gel-eletrophoresis (2-DGE), and Western blotting. LC separates proteins according to various properties and thus complements subsequent electrophoretic separation.
The method most commonly used for quantification of miRNA is real time (RT)-PCR.
3 Results and discussion
Figure 1 summarizes the number of records identified through database search, and after removal, as well as the number of records screened and excluded. A total of 42 and 23 articles on proteomic profile and miRNA expression profile, respectively, were eligible for inclusion. After screening, a total of 65 full-text articles could be included for further review. These articles were published between 2006 and 2020.
3.1 Proteomic analysis
All studies except 4 used a cross-sectional design, including two experimental studies (26, 27), one case-control study (28) and one longitudinal study (29).
In 22 studies, proteomic analysis was performed on whole saliva (unstimulated or stimulated) or saliva collected from individual glands (19, 21–23, 25, 26, 28, 30–43).
Four studies included proteomic analysis of salivary gland tissue (22, 39, 44, 45) and 19 studies included proteomic analyses of plasma, serum or peripheral mononuclear blood cells (PMBC) (22, 27, 29, 46–63). Two studies included proteomic analysis of both stimulated whole saliva and tear fluid and of extracellular vesicles of both saliva and tear fluid from patients with pSS, healthy controls and non-pSS, sicca controls (21, 25). One study simultaneously characterized the proteome in three different types of biological materials (whole saliva, plasma, and salivary gland tissue) from the same patients and non-pSS, sicca controls (22).
Based on their aim, study design and findings, the papers on proteomics could be divided into the following categories:
1. Comparative proteomic analysis of saliva, salivary gland tissue and/or blood from patients with pSS and healthy control subjects and/or non-pSS, sicca control subjects (21–23, 25, 30, 31, 33–35, 37, 38, 40, 43, 45, 52, 54, 57, 58, 60–62)
2. Comparative proteomic analysis of saliva, salivary gland tissue and/or blood from patients with pSS and patients with RA, SLE, systemic sclerosis (SSc), mixed connective tissue disease (MCTD), polymyositis and dermatomyositis (26, 28, 36, 39, 50, 53, 59, 63).
3. Proteomic analysis of saliva and/or serum specifically related to autoantibodies in patients with SS (pSS or sSS) and control subjects (27, 29, 32, 46–49, 51, 55, 56).
4. Proteomic analysis of saliva in SS patients with and without non-Hodgkin MALT (mucosa-associated lymphoid tissue) lymphoma (41, 42, 44).
3.2 Comparative proteomic analysis of saliva, salivary gland tissue and/or blood from patients with pSS, healthy control subjects and/or non-pSS, sicca control subjects
3.2.1 Proteomic analysis of saliva
Table 1 presents an overview of studies on the salivary proteome of patients with pSS compared to healthy controls and patients with non-pSS sicca. The number and type of differentially expressed salivary proteins identified vary between studies. This most likely reflects the heterogeneity of SS and the underlying complexity of the inflammatory immune responses leading to varying degrees of organ damage. Overall, the studies report upregulation of inflammatory mediators and downregulation of proteins of salivary acinar cell origin in SS patients compared to non-SS patients and healthy controls.
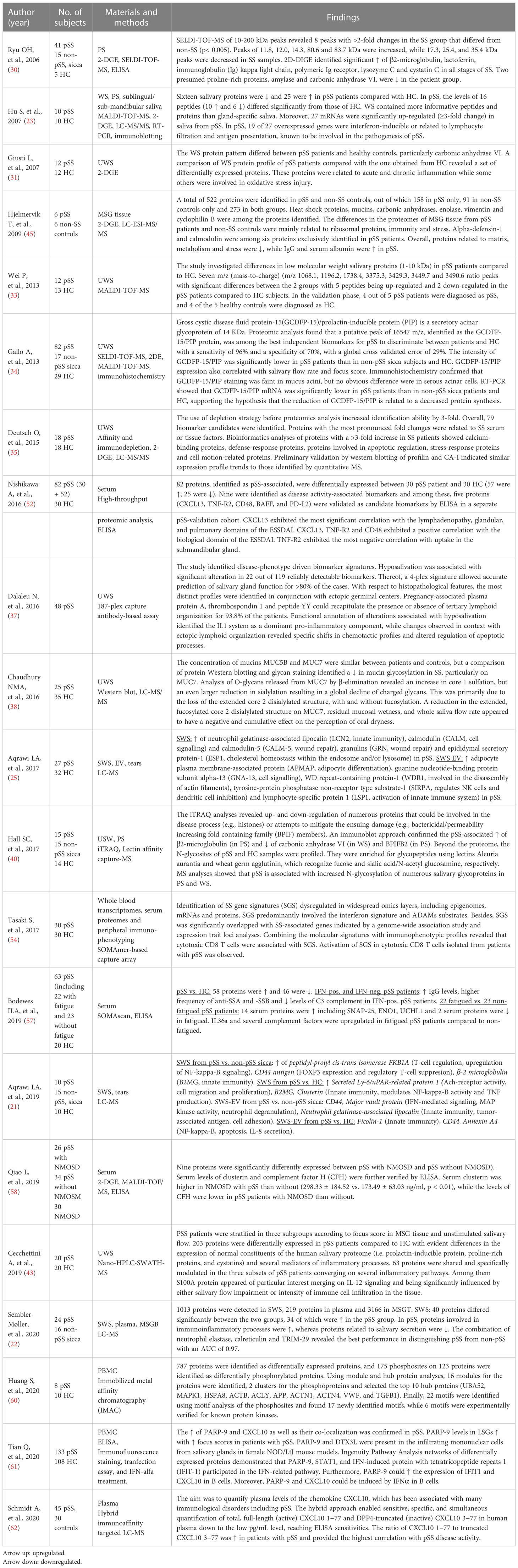
Table 1 Proteomic analysis of saliva, salivary gland tissue and/or blood from patients with pSS and healthy control (HC) subjects and/or non-pSS, sicca control subjects.
Hu et al. (23) compared different types of saliva from patients with pSS and healthy controls, including whole saliva, parotid saliva and saliva from the sublingual/submandibular glands by means of LC-MS/MS analysis. They found that whole saliva yielded more informative peptides and proteins than specific glandular saliva. On average, 53 MALDI peaks were detected in whole saliva from patients with pSS, in contrast to only 24 peaks and 26 peaks in parotid saliva and saliva from the submandibular/sublingual glands, respectively (23). Thus, stimulated whole saliva appears to be more suitable for detection of biomarkers than glandular specific secretions. In whole saliva, 25 proteins were upregulated while 16 proteins were downregulated in patients with pSS patients compared to healthy controls, all reflecting salivary gland damage and oral inflammation in pSS (23). Furthermore, 10 peptides were upregulated and six were downregulated in pSS, while 27 salivary mRNA transcripts were upregulated (≥3-fold) in pSS. Among the 27 genes that were highly overexpressed in pSS, 13 were validated by RT-quantitative PCR, substantiating upregulation of particularly IFN-inducible protein G1P2 in pSS patients. In addition, genes related to lymphocyte infiltration and antigen presentation were found overexpressed (23).
A number of studies report upregulation of β2-microglobulin in parotid saliva (23, 30, 40), unstimulated whole saliva (28, 44), stimulated whole saliva (21–23, 32) and sublingual/submandibular saliva (23) in patients with pSS versus non-pSS patients and/or healthy controls. This supports earlier findings based on different techniques, e.g. sandwich enzyme immunoassay (64). A study found that the combination of upregulated β2-microglobulin, cathepsin D and α-enolase yielded a receiver-operating characteristic (ROC) value of 0.99 in distinguishing patients with pSS from healthy subjects (65). However, this finding has not been further validated. β-2 microglobulin is a non-glycosylated protein, and constitutes the light-chain component of major histocompatibility (MHC) class I molecules. Interestingly, salivary levels of β2-microglobulin have been found associated with the extent of lymphocytic infiltration of the salivary glands in patients with pSS (40, 66) as well as with the presence of anti-SSA/Ro serum antibodies (28).
Alpha-enolase, also known as enolase 1 (ENO1), has also been found upregulated in patients with pSS compared to healthy controls and to patients with non-SS sicca in unstimulated whole saliva (28, 41). Of note, the levels of α-enolase are elevated in serum from pSS patients with fatigue compared to the levels in serum from non-fatigued pSS patients and healthy control subjects (57). Alpha-enolase is a glycolytic enzyme that is expressed in most tissues and has been identified as an autoantigen in Hashimoto encephalopathy (67) and cancer (68). It has been suggested that α-enolase is an autoantigen in pSS, as patients with pSS had higher levels of anti-citrullinated α-enolase IgG antibodies in serum than healthy controls and patients with RA (69). However, the presence of IgA and IgG antibodies against citrullinated and native α-enolase was not associated with any clinical manifestations (69).
A number of other salivary proteins have been found upregulated in pSS, including neutrophil gelatinase-associated lipocalin (NGAL/lipocalin-2) (21, 31), calgranulin A (S100A8) (35), calgranulin B (S100A9) (28), psoriasin (S100A7) (28, 35), epidermal fatty acid binding protein (E-FABP) (21, 23, 28), ACTB protein (actin) and b-Actin fragment (23, 31) as well as immunoglobulin kappa light chain (23, 28, 30, 35). Neutrophil gelatinase-associated lipocalin (NGAL), a glycoprotein belonging to the lipocalin family, is expressed in several normal tissues, where it provides protection against bacterial infection and modulates oxidative stress through anti-apoptotic effects. Levels of NGAL increase in response to inflammation, infection, intoxication, ischemia, acute kidney injury and neoplastic transformation (70). Interestingly, levels of acinar NGAL have been found elevated in minor salivary glands from patients with pSS compared to glands from non-SS patients (71). Calgranulin B (S100A9) and psoriasin (S100A7) are calcium-binding proteins involved in regulation of cellular growth. Both calgranulin-B and psoriasin have been found highly upregulated in ductal carcinoma in situ of the breast and in psoriasis (72). Cecchettini et al. (43) also demonstrated upregulation of S100A proteins in patients with pSS compared to healthy controls, especially in patients with a high focus score and low salivary flow rates, indicating that these proteins play a central role in the progression of glandular dysfunction. Jazaar et al. (42) found salivary S100A8/A9 associated with MALT lymphoma in pSS (see below 1.4.). Calgranulin A (S100A8) has been found upregulated in unstimulated whole saliva and parotid saliva from patients with pSS (35, 42). However, S100A8 was absent in whole saliva samples from patients with pSS in the study by Hu et al. (17). Sembler-Møller et al. (22) combined the analysis of stimulated whole saliva, plasma and salivary gland tissue. They found that salivary proteome of patients with pSS differed from that of non-pSS patients, but no significant difference in protein expression in plasma and labial salivary gland tissue was found between the groups. Several proteins were upregulated in stimulated whole saliva including neutrophil elastase, calreticulin, tripartite motif-containing protein 29 (TRIM29), clusterin and vitronectin. The combination of neutrophil elastase, calreticulin and TRIM-29 had the best performance in distinguishing pSS from non-pSS with an AUC of 0.97 (22).
Aqrawi et al. (25) identified additional upregulated proteins including calmodulin (CALM), calmodulin-like protein 5 (CALML5), granulins (GRN) and epididymal secretory protein-1 (ESP1) in whole saliva from patients with pSS. CALM and CALML5 are both calcium-binding proteins that play a role intracellular signalling and differentiation of keratinocytes, respectively. GRN are involved in wound repair and tissue remodelling, and ESP1 is involved in cholesterol homeostasis within the endosome and/or lysosome.
The most frequently identified downregulated proteins include prolactin-inducible protein (PIP) (21, 28, 34), carbon anhydrase VI (CA6) (23, 28, 30, 31, 40); salivary α-amylases (26, 28), most of the cystatins and a number of other secretory proteins (23, 28, 30, 31). PIP is a secretory protein, which is present in glycosylated and non-glycosylated forms in human saliva. Gross cystic disease fluid protein-15 (GCDFP-15)/PIP, which binds to aquaporin 5, a water channel critical to saliva formation, has been found downregulated in pSS, and the GCDFP-15/PIP expression correlated with both the salivary flow rate and focus score of minor salivary gland biopsies (34). Salivary PIP also binds to dental enamel (hydroxyapatite) and presumably participates in the formation of the enamel pellicle (73). It has been demonstrated that PIP-deficient mice (Pip −/− mice) develop impaired T-helper type 1 response and cell-mediated immunity (74). The role of PIP in the pathogenesis of SS requires further investigation.
CA6 is a zinc-containing metalloenzyme secreted in the salivary glands. CA6 catalyzes the conversion of salivary bicarbonate and hydrogen ions to carbon dioxide and water. The process is essential for the maintenance of salivary pH homeostasis and prevention of demineralization of the dental enamel (75). Reduced CA6 expression could imply impairment of the salivary bicarbonate buffer capacity and may explain the occurrence of increased caries activity in patients with SS.
Cystatins are cysteine protease inhibitors, which provide protecting against proteases by regulating uncontrolled proteolysis and tissue damages (76). The family of cystatins includes cystatin S, cystatin SN, cystatin C, and cystatin D. Cystatins may differ with respect to phosphorylation and/or glycosylation. Giusti et al. (31) found reduction of cystatin SN precursor in patients with pSS compared to control subjects, whereas the other cystatins were scarcely represented or totally absent. Ryu et al. (30) found an increase in salivary levels of cystatin C (about 1.3-fold) compared to the levels in saliva from controls. The diverging results may reflect variation in the levels of cystatins in the different types of saliva, i.e. whole saliva versus parotid saliva. Cystatin C levels have been reported increased in whole saliva of patients with periodontitis, and this may also contribute to explain the conflicting results. The reported decrease in salivary α-amylases (23, 28, 30, 31) may be a result of fragmentation caused by protease endogenous truncation or to the acinar cell damage.
Hall et al. (40) confirmed upregulation of β2-microglobulin in parotid saliva and downregulation of CA6 in whole saliva based on isobaric mass tagging (iTRAQ) and lectin affinity capture mass spectrometry (MS)-based approach, but also detected downregulation of parotid saliva BPIFB2 (bactericidal/permeability increasing fold containing family B2), and increased N-glycosylation of numerous salivary glycoproteins in both parotid and whole saliva from patients with pSS compared to control subjects (40).
Finally, Table 1 includes studies that have reported upregulated or downregulated MALDI peaks (m/z) (peptides or fragments derived from proteolytic activity in parotid or whole saliva from patients with pSS compared to healthy controls) (33).
3.2.2 Proteomic analysis of salivary gland tissue
In a study by Hjelmervik et al. (45), using LC-ESI-MS/MS and 2-D PAGE, six proteins were exclusively detected in labial salivary gland tissue from patients with pSS, including α-defensin-1 and calmodulin. Alpha-defensin-1 plays a central role in virus defence and upregulates the type I IFN response genes (77) and had previously been suggested as a salivary biomarker for oral inflammation in pSS (26). Calmodulin regulates inflammatory processes, and elevated levels of calmodulin-binding proteins in the salivary glands from pSS patients have also been reported (78).
3.2.3 Proteomic analysis of plasma, serum or PBMC
While Sembler-Møller et al. found no difference in the protein expression profile in plasma between patients with pSS and non-pSS sicca controls, other studies on serum, plasma and PBMC found multiple proteins to be upregulated and downregulated in patients with pSS compared to controls, which may reflect different control group designs (22). Bodewes et al. (57) identified a set of proteins that could not only discriminate pSS from healthy controls, but also distinguish fatigued-pSS patients from non-fatigued pSS patients. IFN-positive pSS patients had higher levels of IgG and anti-SSA and -SSB and lower levels of C3 complement than IFN-negative pSS patients. Several serum proteins, including neuroactive synaptosomal-associated protein 25 (SNAP-25), α-enolase, ubiquitin carboxyl-terminal hydrolase isozyme L1 (UCHL1) and IL36a as well as several complement factors were upregulated in fatigued pSS patients compared to non-fatigued pSS (57).
Another study by Qiao et al. (58) found upregulation of clusterin and complement factor H in pSS patients with neuromyelitis optica spectrum disorder (NMOSD) compared to pSS patients without this disorder. These findings suggest that serum clusterin and factor H could be potential biomarkers for pSS patients with NMOSD and play a role in the pathogenesis of the disease, but further verification is needed.
Nishikawa et al. (52) identified five proteins as disease activity-associated biomarkers, including CXCL13, TNF-R2, CD48, B-cell activating factor (BAFF), and PD-L2. CXCL13 significantly correlated to the lymphadenopathy, glandular, and pulmonary domains of the EULAR Sjögren’s syndrome disease activity index (ESSDAI). CXCL13, TNF-R2 and CD48 correlated positively with the biological domains of the ESSDAI, while TNF-R2 exhibited the most negative correlation with uptake in the submandibular gland.
In a study on PBMC (60), 787 proteins were identified as differentially expressed, and 175 phosphosites on 123 proteins were identified as differentially phosphorylated proteins. The study identified 16 modules for the proteins, two clusters for the phosphoproteins and selected the top 10 hub proteins (UBA52, MAPK1, HSPA8, ACTB, ACLY, APP, ACTN1, ACTN4, VWF, and TGFB1). Finally, 22 motifs were identified using motif analysis of the phosphosites, and the authors found 17 novel motifs, while 6 motifs were experimentally verified for known protein kinases. The differentially expressed proteins enabled discrimination of patients with pSS from healthy controls and were suggested as candidate biomarkers in the diagnosis of pSS.
3.3 Comparative proteomic analysis of saliva, salivary gland tissue and/or blood from patients with pSS and other inflammatory connective tissue diseases
Table 2 shows the proteomic analysis of saliva, salivary gland tissue and/or blood from patients with pSS and other inflammatory connective tissue diseases. An experimental study with peroral pilocarpine treatment on patients with pSS, sSS and healthy controls showed that the levels of almost all of the 60% salivary proteins, which was not present or present at low levels prior to treatment in pSS, reached similar levels in unstimulated whole saliva in all groups 30-60 minutes after treatment with pilocarpine. Patients with pSS also had β-defensin-2 in their saliva and higher levels of α-defensin-1 than patients with sSS and healthy controls, indicating that α-defensin-1 and β-defensin-2 may be used as biomarkers of oral inflammation in patients with pSS (26).
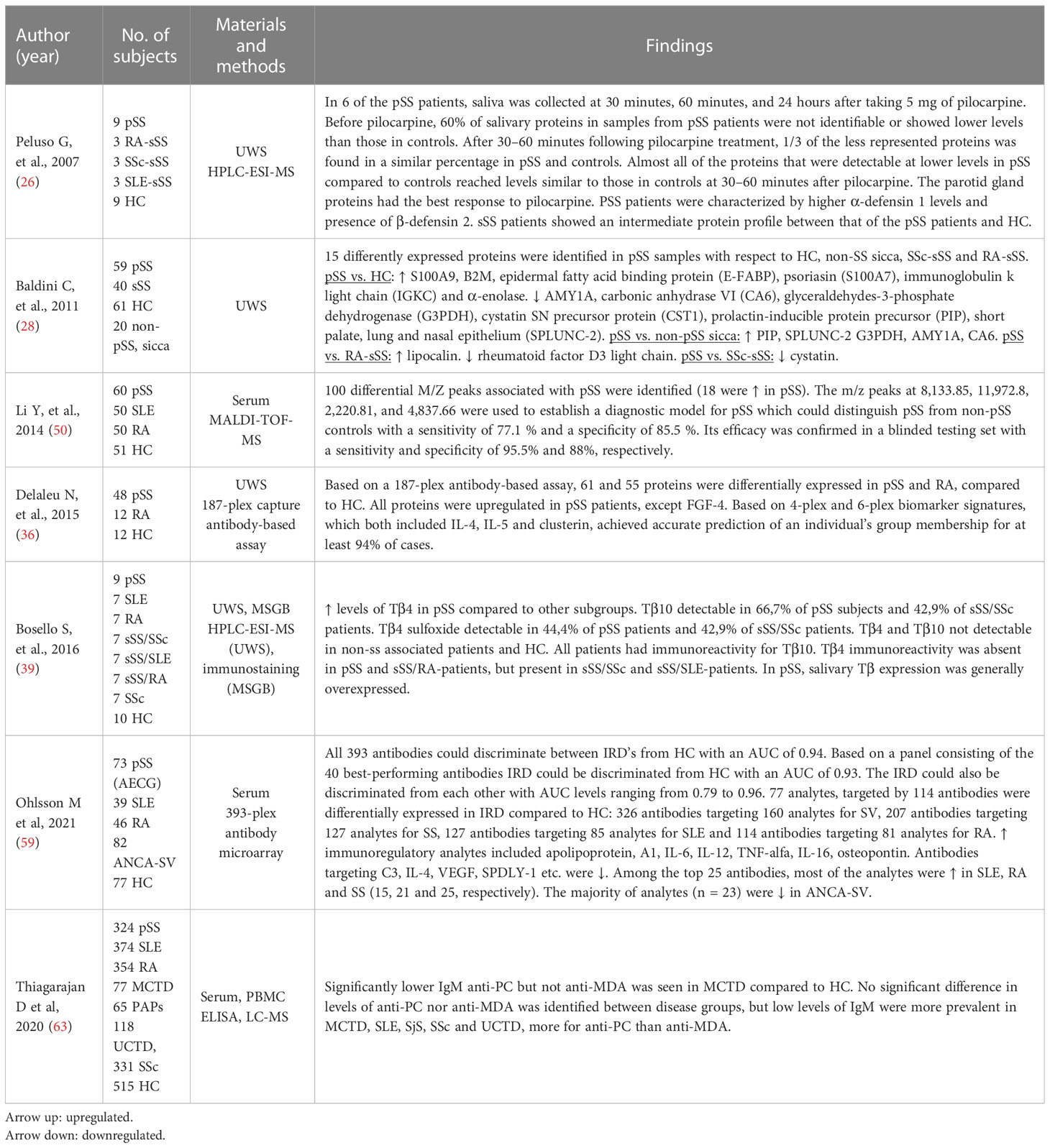
Table 2 Comparative proteomic analysis of saliva, salivary gland tissue and/or blood from patients with pSS patients with RA, SLE, systemic sclerosis, mixed connective tissue disease (MCTD) and poly- and dermatomyositis (1.2).
Baldini et al. (28) identified 15 differently expressed proteins in patients with pSS compared to healthy controls, non-pSS sicca, patients with RA-sSS and SSc-sSS. S100A9, β-2 microglobulin, epidermal fatty acid binding protein (E-FABP), psoriasin (S100A7), immunoglobulin k light chain (IGKC) and α-enolase were upregulated in patients with pSS compared to healthy controls, whereas AMY1A, carbonic anhydrase VI (CA6), glyceraldehydes-3-phosphate dehydrogenase (G3PDH), cystatin SN precursor protein (CST1), prolactin-inducible protein precursor (PIP), short palate, lung and nasal epithelium carcinoma associated protein 2 (SPLUNC-2) were downregulated in patients with pSS. PIP, SPLUNC-2 G3PDH, AMY1A, CA6 were upregulated in patients with pSS compared to non-pSS, sicca patients. Lipocalin was upregulated, while rheumatoid factor D5 light chain was downregulated in patients with pSS compared to patients with RA-sSS. Lastly, a comparison with patients with SSc-sSS revealed an upregulation of cystatin in patients with pSS (28).
One hundred differential m/z peaks associated with pSS were identified by Li Y al (13)., and the m/z (mass-to-charge) peaks at 8,133.85, 11,972.8, 2,220.81, and 4,837.66 were used to establish a diagnostic model for pSS. The proteomic model could distinguish pSS from non-pSS controls (SLE, RA and healthy controls) with a sensitivity of 77.1% and a specificity of 85.5%. The efficacy was confirmed by a blinded validation study.
Two studies detected differently expressed proteins using multiplexed antibody-assay in unstimulated whole saliva and serum (36, 59). Based on a 187-plex-antibody assay, 61 and 55 proteins were differently expressed in unstimulated whole saliva in pSS and RA, respectively, compared to healthy controls (36). A 4-plex and 6-plex biomarker signature was then developed, including IL-4, IL-5 and clusterin, which achieved accurate prediction of an individual group in at least 94% of the cases (36). Ohlsson et al., 2020 (59) used a 393-plex antibody microarray to test the discriminatory performance in distinguishing between different inflammatory rheumatic diseases (i.e., pSS, SLE, RA and antineutrophil cytoplasmic antibody-associated systemic vasculitis (ANCA-SV) from healthy controls in serum. An analysis including all 393 antibodies could discriminate the patient groups from the healthy controls (HC vs. SLE+RA+SS+SV) with an AUC of 0.94. Serum samples from patients with pSS could be distinguished from the other patient samples (SS vs. SLE+RA+SV) with an AUC of 0.80. A total of 207 antibodies targeting 127 analytes were found to be differentially expressed in patients with pSS compared to healthy controls.
Bosello S. et al. (39) investigated the salivary levels of Tβ4, Tβ10, and Tβ4 sulfoxide in patients with pSS, SLE, RA, SSc, sicca syndrome + SSc (ss/SSc), sicca syndrome + SLE (ss/SLE), sicca syndrome + RA (ss/RA) and healthy controls. Tβ10 and Tβ4 sulfoxide were not detectable in healthy controls and patients without sicca syndrome. Patients with pSS had higher levels of Tβ4 than the other patient groups. Tβ10 and Tβ4 sulfoxide were detectable in 66.7% and 44.4% of the patients with pSS, respectively. Overall, the patients with pSS displayed highest expression of salivary Tβ, whereas Tβ4 immunoreactivity in minor salivary glands was completely absent in patients with pSS.
3.4 Autoantibodies in patients with SS
Ten studies eligible for inclusion focused on proteomic analysis of serum or salivary autoantibodies in patients with SS (27, 29, 32, 46–51, 55, 56) (Table 3). Anti‐SSA and ‐SSB antibodies are clinically important antinuclear antibodies in patients with SS. However, these antibodies may not be present in all patients with SS, and they may also be found in other inflammatory rheumatic diseases, including SLE, polymyositis and SSc, as well as in patients with primary biliary cirrhosis (PBC) and hepatitis C viral infection (79). Anti-SSA/Ro autoantibodies are the most frequently identified antinuclear antibodies directed against Ro antigens, consisting of two different proteins, Ro60 and Ro52. The Ro 60 kDa autoantigen is an RNA-binding protein, associated with one of several human Y RNAs (small non-coding RNAs, required for DNA replication). Ro52/TRIM21 is an IFN-inducible protein, which is also induced by viral infection (80). Anti‐SSB/La also recognizes the Ro‐ribonucleoprotein. Anti-Ro52 and anti-Ro60 autoantibodies are closely linked but also display different clinical associations (81). Thus, anti-SSA/Ro52/TRIM21 antibodies show a wider spectrum of disease-associations than anti-SSA/Ro60 antibodies (82). Both Ro antigens have been found expressed in the surface of ductal epithelial salivary gland cells during apoptosis indicating that the initiation of anti-SSA/Ro60 and anti-Ro52/TRIM21 responses play a role of in the pathogenesis of SS (83). In addition, anti-SSA/Ro60- and anti-SSA/Ro52/TRIM21-specific B cells compatible with plasmablasts or plasma cells have been detected in salivary glands of patients with pSS (84). Autoantibodies may be present years before the clinical symptoms of SS, and anti-SSA and anti-SSB antibodies are therefore central biomarker targets that are useful in the diagnosis of SS (85).
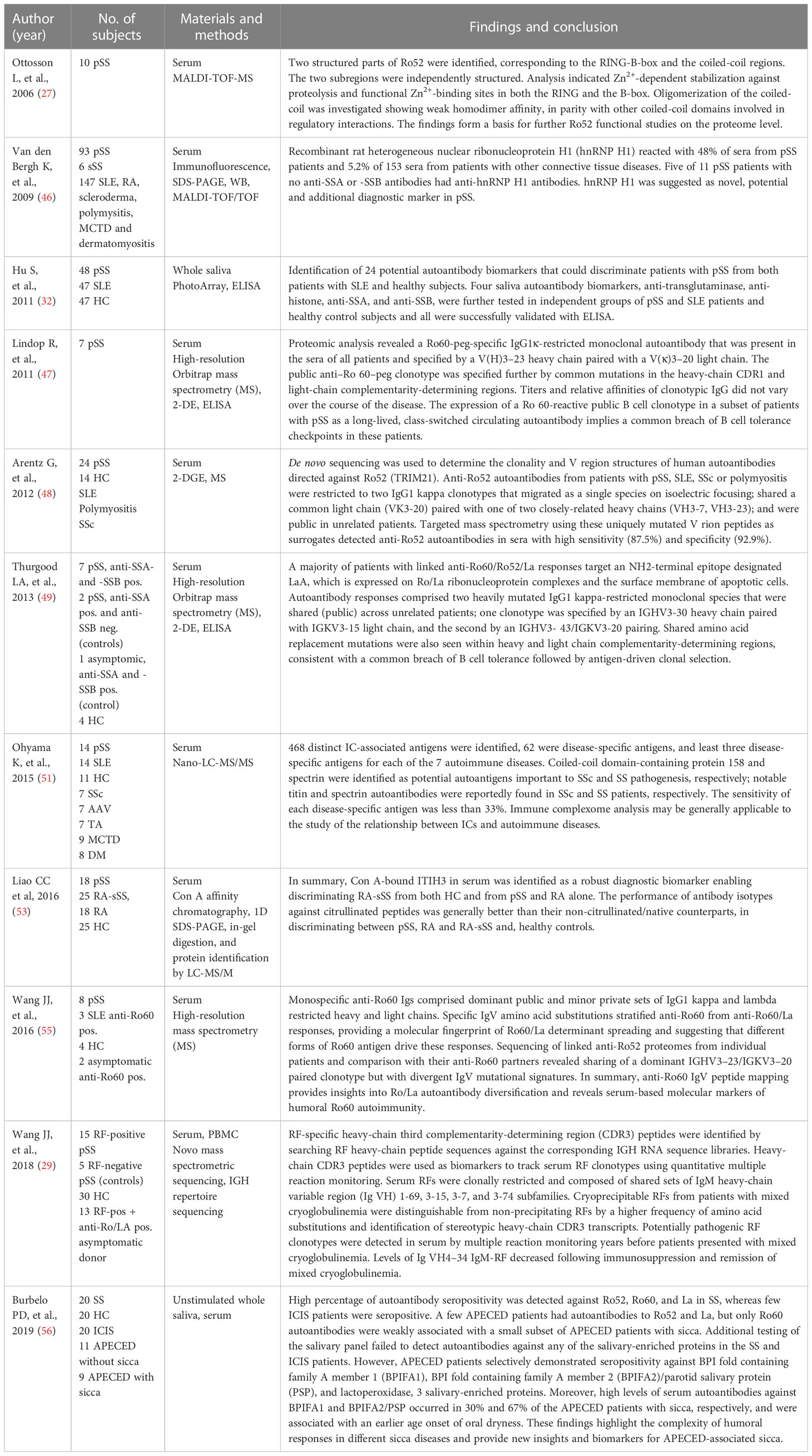
Table 3 Proteomic analysis of saliva and/or serum specifically related to autoantibodies in patients with SS and control subjects (1.3).
Patients having circulating serum autoantibodies often present more symptoms and clinical disease manifestations than patients without presence of circulating antibodies (14). It has been shown that anti-SSA/-SSB negative patients with SS have a lower prevalence of lymphoproliferative lesions and lower risk of developing lymphoma than those who are SSA and/or SSB autoantibody positive (86). Proteomic analyses of serum from patients with pSS have revealed expression of public clonotypic autoantibodies against Ro52/TRIM21 and Ro60 antigens as well as public clonotypic autoantibodies directed against an immunodominant epitope on La (47–49). The findings of shared amino acid replacement mutations within heavy and light chain complementarity-determining regions imply a common breach of B-cell tolerance checkpoints that is followed by an antigen-driven clonal selection (48, 49). Accordingly, the findings support that the humoral immune responses against protein-RNA complexes are mediated by public sets of autoreactive B-cell clonotypes leading to systemic autoimmunity. Moreover, anti-Ro52 serum autoantibodies from patients with pSS, SLE, systemic sclerosis and polymyositis were restricted to two IgG1/kappa clonotypes, and targeted mass spectrometry detected anti-SSA/Ro52 serum autoantibodies with high sensitivity and specificity compared with conventional ELISA-technique (48). Further analysis using high-resolution mass spectrometry to sequence precipitating anti-Ro60 proteomes revealed that specific immunoglobulin variable-region peptide signatures stratified anti-Ro60 from anti-Ro60/La responses, indicating that different forms of Ro60 antigen drive the humoral Ro60 immune responses (55).
An earlier study including an immunologic analysis of the stable protein regions in sera from patients with SS showed that immunodominant epitopes predominantly are localized in the structurally stable parts of Ro52 (27). Apparently, the findings have not been substantiated by further functional studies at the proteome level.
A more recent study reported a high percentage of autoantibodies against Ro52, Ro60, and La in SS, whereas only a few patients with immune checkpoint inhibitor-induced sicca (ICIS) presented with these autoantibodies. Further testing of the salivary panel did not reveal autoantibodies against any of the salivary-enriched proteins in patients with SS and ICIS (56).
Another recent study identified antibodies against heterogeneous nuclear ribonucleoprotein H1 (hnRNP H1) in serum from about 43% patients with SS (2 primary and 5 secondary) and in about 8% patients with various autoimmune connective tissues diseases, all being ANA-positive (titer >1:160). Interestingly, 45% (5/11) of the anti-SSA/anti-SSB sero-negative patients had anti-hnRNP H1 antibodies. Thus, anti-hnRNP H1 antibody represents a potential novel diagnostic marker that can be helpful in discriminating patients with pSS from patients with SLE (potentially with sSS) (46).
Hu et al. (32) identified 24 potential salivary autoantibody biomarkers that can discriminate patients with pSS from both patients with SLE and healthy subjects. Four of them, including anti-transglutaminase, anti-histone, anti-SSA and anti-SSB antibodies, were successfully validated by means of ELISA, and on independent groups of patients with pSS, SLE, and healthy control subjects (32).
A study on molecular profiling and clonal tracking of secreted rheumatoid factors (RF) in pSS found that cryoprecipitable RF clonotypes linked to vasculitis in pSS display different molecular profiles than non-precipitating RFs. Interestingly, potentially pathogenic RF clonotypes were detected in serum by multiple reaction monitoring years before patients presented with mixed cryoglobulinemia. Furthermore, levels of Ig VH4-34 IgM-RF diminished after immunosuppression and remission of cryoglobulinemia (29).
Liao et al. (53) found serum autoantibodies against the citrullinated-inter-alpha-trypsin inhibitor heavy chain (ITIH3)542-556 peptide in serum in patients with pSS, RA and RA-sSS. IHIT3 is one of five chains that comprises the family of inter-alpha-trypsin inhibitors (ITI), which have the ability to bind to hyaluronic acid (HA) to interact with inflammatory cells (87). Thus, the ITIH3-HA complex is potentially involved in inflammatory diseases such as RA and pSS (88). Previous studies have reported PTM (post-translational modification)-modified proteins in patients with pSS such as citrullinated Ro60 and histone 1 (89). Furthermore, salivary antibodies against cyclic citrullinated peptide (anti-CCP), a proxy for anti-citrullinated protein antibodies (ACPA), have been identified in patients with pSS (90), suggesting that citrullinated proteins can be a utilized as diagnostic biomarkers. Liao et al. (53) found that Concanavalin A (Con A)-bound ITIH3 in serum was a robust diagnostic biomarker enabling discriminating RA-sSS from both healthy controls and from pSS and RA alone. The performance of antibodies against citrullinated peptides was generally better than antibodies against their non-citrullinated/native counterparts in discriminating between pSS, RA and RA-sSS and healthy controls. In addition, we have previously found that anti-SSA alone can differentiate pSS from non-pSS with an AUC of 0.9, while combining the presence of anti-SSA positivity with the upregulation of TRIM29 protein marker raised the AUC to 0.995 with both sensitivity and specificity between 91%-100% (91).
3.5 Proteomic analysis of saliva and salivary gland tissue in SS patients with and without non-Hodgkin MALT lymphoma
Patients with SS, and particularly with pSS, have an increased risk of developing lymphoproliferative malignancy. About 5-10% of the patients develop non-Hodgkin lymphoma (NHL), most commonly the mucosa-associated lymphoid tissue (MALT) B-cell lymphoma type (92–94). Risk factors include swelling of the parotid gland, vasculitis, hypergammaglobulinemia, CD4+ T lymphocytopenia, low CD4+/CD8+ T-cell ratio, low complement protein levels, cryoglobulinemia and high focus score and ectopic germinal center formation in the salivary gland tissue (92, 94). The mechanisms underlying malignant transformation of a lymphoproliferative process remain elusive.
Three studies on proteomic analysis of saliva and salivary gland tissue were eligible for inclusion in this review (41, 42, 44) (Table 4). Hu et al. (44) aimed at clarifying differences in gene expression and proteomic profile in parotid gland tissue from patients with pSS, patients with pSS and MALT lymphoma and non-pSS control subjects (patients with oral or oro-pharyngeal squamous cell carcinoma). Six highly associated hub genes (i.e., genes involved in proteasome degradation, apoptosis, signal peptides, complement activation, cell growth and death and integrin-mediated cell adhesion, and major histocompatibility (MHC) genes) distinguished patients with pSS from non-pSS control subjects. Eight hub genes (i.e., genes involved in translation, ribosome, protease degradation, signal peptides (MHC) class I, G13 signaling pathway, complement activation, and integrin-mediated cell adhesion) distinguished pSS patients with MALT from pSS patients. A total of 115 proteins were upregulated in patients with pSS and pSS/MALT lymphoma. Twenty-five proteins were upregulated in both pSS groups compared to non-pSS control patients, 20 proteins were upregulated in pSS compared to pSS/MALT and non-pSS control patients, and 70 proteins were upregulated in pSS/MALT compared to pSS and non-pSS control patients. The identified disease hub genes represent promising targets for therapeutic intervention, diagnosis, and prognosis (44).
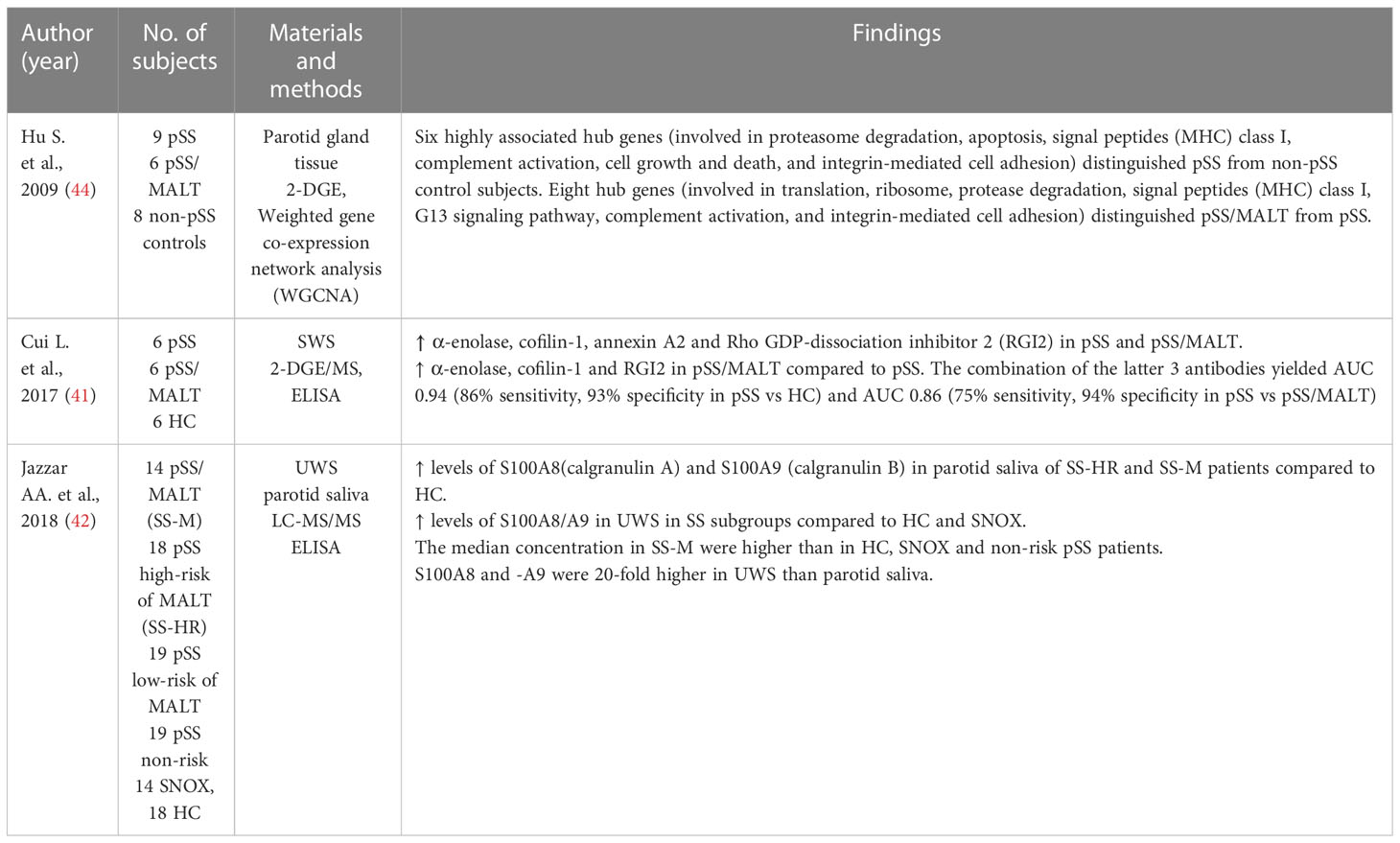
Table 4 Proteomic analysis of saliva and salivary gland tissue in SS patients with and without non-Hodgkin MALT lymphoma.
Cui et al. (41) found α-enolase, cofilin-1, annexin A2 and Rho GDP-dissociation inhibitor 2 (RGI2) overexpressed in parotid gland tissue from patients with pSS and in patients pSS and MALT (41). Moreover, the levels of anti-α-enolase, anti-coffilin-1, and anti-RGI2 antibodies were higher in patients with pSS/MALT than in patients with pSS and healthy subjects, and significantly higher in pSS patients than in healthy subjects (41). The combination of the three antibodies yielded an AUC of 0.94 with an 86% sensitivity and 93% specificity in distinguishing patients with pSS from healthy controls, an AUC of 0.99 with a 95% sensitivity and 94% specificity in distinguishing patients with pSS and MALT lymphoma from healthy controls, and an AUC of 0.86 with a 75% sensitivity and 94% specificity in distinguishing patients with pSS and MALT from patients with pSS (41) (Table 4). The combination of these autoantibodies may be helpful in the diagnosis of pSS and prediction of development into MALT lymphoma.
Another study investigated the potential of S100A8 (Calgranulin A) and S100A9 (Calgranulin B) as biomarkers to discriminate patients with pSS from healthy subjects and patients with pSS from patients with pSS and MALT lymphoma (42). The levels of S100A8 and S100A9 were upregulated in parotid saliva from patients with pSS compared to healthy controls. In addition, the levels of S100A8 and S100A9 were upregulated in unstimulated whole saliva of patients with pSS, pSS/MALT compared to healthy controls and disease controls (patients with non-specified sialadenitis). Furthermore, the levels were higher in pSS patients with MALT lymphoma and pSS patients with high lymphoma risk than in pSS patients with low risk of lymphoma, healthy subjects and disease controls. Interestingly, S100A8 and S100A9 levels were 20-fold higher in unstimulated whole saliva than in parotid saliva. These findings suggest that S100A8 and S100A9 can assist in distinguishing pSS patients from healthy subjects and pSS patients with MALT lymphoma from pSS without lymphoma (42) (Table 4). S100A8 and S100A9 are proinflammatory proteins that play a central role in the acute and chronic inflammation. They have been found associated with autoimmune diseases and upregulated in various human cancers (117, 118).
3.6 MiRNA analysis of blood, PBMC, saliva and salivary gland tissue
MicroRNAs (miRNAs) are short non-coding RNA molecules, which main function is to regulate gene expression posttranscriptionally by messenger (mRNA) degradation or translational repression (119). Previous findings indicate aberrant miRNA expression in PBMC (96, 98, 99, 102, 104, 109, 120) and salivary gland tissue of patients with pSS (95, 97, 121). However, the number of publications within the field of miRNA expression profiling is increasing, revealing novel biomarkers of interest.
All studies except one used a cross-sectional design, which was a longitudinal study (108) (Table 5). In 16 studies, the miRNA analysis was performed on plasma, serum or peripheral mononuclear blood cells (PMBC). Six studies included miRNA analysis on salivary gland tissue. We have previously performed combined miRNA analyses on whole saliva, plasma and minor salivary gland tissue (114), and Gourzi et al. (101) combined miRNA analyses on PBMC, minor salivary gland tissue and salivary gland epithelial cells.
As with the proteomics studies, the findings in the 23 studies were very heterogeneous in terms of number and type of differentially expressed miRNAs. Nevertheless, several studies identified similar miRNAs to be differentially expressed such as miR-146, miR-155 and miR-188.
A study by Zilahi et al. (96) found both miR-146a and miR-146b to be significantly overexpressed in patients with pSS compared to healthy controls inPBMC. Two additional studies (99, 108) supported these findings displaying a higher expression of miRNA-146a in patients with than in healthy controls in PBMC. Wang-Reanult et al. (109) found miR-146a to be differentially expressed in CD4+ T cells. Moreover, miR-146a has been found upregulated in saliva in patients with pSS and in addition, miR-146b levels correlated with ESSPRI scores, while being downregulated in patients with high salivary gland ultrasound scores (113).
MiR-155 is a central modulator of T-cell responses, and SOCS1 is its functional linked gene (100). Shi H et al. (99) reported a lower expression of miR-155 in patients with pSS. Furthermore, miR-155 correlated with VAS score for dry eyes. On the other hand, Chen et al. (100) reported higher expression of both miR-155 and SOCS1 gene in PBMC in patients with pSS. In another study, miR-155 was higher expressed in patients with SLE, but unchanged in patients with pSS (104). In a recent study, the expression levels of miR-155 and miR-125b-5p in CD4+ T cells were found associated with disease activity (116). These findings may provide insight in the pathogenesis and disease course of SS and suggest a novel target for treatment.
We identified a downregulation of several miR-17 family members (i.e. miR-17-5p, miR-106a, miR-106b, and miR-20b-5p) in patients with pSS compared to non-pSS subjects (114), which confirms the results of previous studies (109, 119, 121). The miR-17-family takes part in the miR-17-92 cluster, previously shown to be related to carcinogenesis and autoimmunity (122). In addition, we previously found upregulation of let-7i-5p in patients with pSS that was inversely correlated with salivary flow rates and positively correlated with higher focus score, suggesting that let-7i-5p canbe used as a marker for disease activity/advanced disease state (114).
Gourzi et al. (101) determined the levels of miR-181a among other miRNAs in minor salivary gland tissue and epithelial cells and PBMC in patients with pSS and non-pSS, sicca. The levels of miR-181a, let7b, miR-16 and miR-483-5p in minor salivary gland tissue correlated with Ro52/TRIM21-mRNA. On the contrary, riR181a and miR-200b-3p correlated negatively with Ro52/TRIM21 and Ro60/TROVE2-mRNA in salivary gland epithelial cells, respectively. Let7b, miR-200b-5p and miR-233 correlated with La/SSB-mRNA. In PMBCs, miR181a, miR-16 and let7b correlated with both Ro/52-TRIM21- and La/SSB-mRNA expression (101). It has previously been shown that miR-200b-5p correlate inversely with ESSDAI and focus score in labial salivary gland biopsies, and positively with serum C4 levels (107). Furthermore, Kaspsogeorgou et al. (107) found lower expression of miR-200b-5p in pSS patients with NHL or with a high risk of developing NHL compared to patients with pSS without lymphoma and patients with non-pSS sialadenitis. In fact, the levels of miR200b-5p were lower long before clinical onset of lymphoma and were proved to be strong independent predictor of patients who will develop Non-Hodgkin’s lymphoma (NHL). These findings suggest that levels of miR-200b-5p in minor salivary gland tissue may represent a predictive biomarker for development of SS-associated NHL.
Lopes et al. (106) investigated serum levels of 758 small non-coding RNAs (snRNA) including miRNAs in patients with pSS, non-pSS sicca and healthy controls. Three and nine sncRNAs were differentially expressed in the pSS-group and non-pSS sicca-group, respectively, compared to healthy controls. Two of the sncRNAs, including, U6-snRNA and miR-29c-3p, were differently expressed in both patient groups. Interestingly, the abundance of several of the differentially expressed snRNAs correlated with laboratory parameters and disease activity (i.e., low C3/C4, decreased leukocyte count, high focus score and anti-SSA/Ro and/or anti-SSB/La).
Furthermore, the specific pattern of snRNA expression could identify different disease phenotypes within the pSS group. Hierarchical clustering was performed to subgroup the pSS patients into 3 clusters based on their expression of the nine sncRNAs. One group (cluster 3) presented with an increased disease activity, including increased IgG levels, autoantibody positivity and IFN-score in their serum, decreased leukocyte count and furthermore, an overall decreased in the expression of all nine sncRNAs compared to the two other groups. It was concluded that no snRNAs could discriminate pSS from non-pSS sicca. However, the snRNA levels may reflect the disease activity and can be used to identify pSS patients with increased B cell hyperactivity. Hillen et al. (111) supported the findings of differentially expressed miR-29c as they reported miR-29a and miR-29c to be significantly downregulated in plasmacytoid dentritic cells (pDC) from patients with pSS compared to healthy controls.
Gallo et al. (112) found a higher expression of miR-18b, miR-20a, miR-106 and miR-146b in minor salivary gland tissue from patients with pSS than in those of healthy controls. These miRNAs correlated inversely with salivary flow rates. MiR-635 and miR-372 were downregulated and correlated positively with salivary flow rates. The most significant pathways that could be targeted by the 100 most regulated miRNA (>2 fold change) in minor salivary gland biopsies from the patients with pSS included the KEGG and mucin type O-glycan biosynthesis pathways. It is well-known that mucins are important for the rheological properties in saliva (123, 124). Interestingly, a glycosylation profiling analysis on salivary samples from pSS and healthy controls revealed deregulation of some glycosyltransferases and glycosidases suggesting that an alteration of miRNA-target genes in the mucin type O-glycan biosynthesis may influence the glycosylation of salivary mucins and salivary functions in pSS (112).
Two TRIM21-targeting miRNAs, miR-1207-5p and miR-4695-3p, have previously been identified (115), and their role in the development of pSS has been investigated using human submandibular gland cells. Transfection of miRNA mimics into human submandibular gland cells revealed downregulation of mRNA to 53% and 42% for miR-1207-5p and miR-4695-3p, respectively. The expression of TRIM21 was also downregulated to 18% and 33% for miR-1207-5p and miR-4695-3p, respectively. Additionally, upregulation of TRIM21 was seen after transfection of miRNA inhibitor. Findings indicate that miR-1207-5p and miR-4695-3p are anti-apoptotic in human submandibular glands cells and regulate the expression of TRIM21 and multiple pro-apoptotic genes in salivary glands (115). In pSS, an upregulated expression of TRIM21 due to decreased miR-1207-5p and miR-4695-3p may lead to upregulation of the CASP-8 and ultimately facilitates the minor salivary glands cells to undergo apoptosis (115).
Peng et al. (98) found 180 miRNAs upregulated and 202 miRNAs were downregulated in PBMC from patients with pSS. Eleven of these (miRNA-let-7b, miRNA-142-3p, MiRNA-142-5p, miRNA-146a, miRNA-148b, miRNA-155, miRNA-18b, miRNA-181a, miRNA-223, miRNA-23a and miRNA-574-3p) are involved immune regulatory mechanisms. MiR-181a was the most significant differentially expressed miRNA in patients with pSS compared to healthy controls. MiRNA-181a only correlated with ANA, suggesting that PBMC miRNA-181a is a potential biomarker to distinguish pSS from healthy controls, but cannot be used to discriminate between different disease phenotypes.
4 Conclusion
Overall, the proteomic and miRNA studies revealed considerable variations with regard to candidate biomarker proteins and miRNAs, most likely due to variation in collection method, sample size, processing and analytical methods, but also reflecting the complexity of SS and patient heterogeneity. Furthermore, the high rate of salivary posttranslational modifications such as glycosylation, phosphorylation, sulfation, transglutaminase and proteolytic cleavages adds structural and functional diversity to the proteome, which may challenge interpretation of the data. It should also be noted that while serum-derived proteins in saliva are mainly involved in cell cycle, signal transduction etc., salivary proteins and peptides are involved in antibacterial activity, lubrication, digestion, oral homeostasis and more likely express local changes and thus better reflect changes/diseases in the oral cavity.
Nevertheless, interesting novel knowledge has emerged and several studies support the findings of differential expression of certain proteins such as upregulation of cystatin A, β2M, α-enolase, actin, E-FABP, N-GAL, Ig-κ light-chain and C3 as well as downregulation of PRPs, CA6, α-amylase, histatins, PIP, cystatin S and cystatin SN. Another novel finding was the discriminatory performance of an anti-SSA/Ro combined with TRIM29, which showed a higher sensitivity than anti-SSA/Ro positivity alone. However, further validation with larger multicenter studies is needed to confirm their potential role as biomarkers in SS.
Data availability statement
The original contributions presented in the study are included in the article/supplementary material. Further inquiries can be directed to the corresponding author.
Author contributions
SK and AP: Contributed with conception of the work, data acquisition, prepared the figures and tables, and drafted the manuscript. SK, AP, MS-M, and CN critically reviewed and edited the final version of the manuscript. All authors contributed to the article and approved the submitted version.
Conflict of interest
The authors declare that the research was conducted in the absence of any commercial or financial relationships that could be construed as a potential conflict of interest.
Publisher’s note
All claims expressed in this article are solely those of the authors and do not necessarily represent those of their affiliated organizations, or those of the publisher, the editors and the reviewers. Any product that may be evaluated in this article, or claim that may be made by its manufacturer, is not guaranteed or endorsed by the publisher.
References
2. Brito-Zerón P, Baldini C, Bootsma H, Bowman SJ, Jonsson R, Mariette X, et al. Sjögren syndrome. Nat Rev Dis Primers. (2016) 2:16047. doi: 10.1038/nrdp.2016.47
3. Ramos-Casals M, Brito-Zerón P, Kostov B, Sisó-Almirall A, Bosch X, Buss D, et al. Google-Driven search for big data in autoimmune geoepidemiology: analysis of 394,827 patients with systemic autoimmune diseases. Autoimmun Rev (2015) 14(8):670–9. doi: 10.1016/j.autrev.2015.03.008
4. Shimizu T, Nakamura H, Kawakami A. Role of the innate immunity signaling pathway in the pathogenesis of sjögren’s syndrome. Int J Mol Sci (2021) 22(6):3090. doi: 10.3390/ijms22063090
5. Verstappen GM, Kroese FGM, Bootsma H. T Cells in primary sjögren’s syndrome: targets for early intervention. Rheumatol (Oxford) (2019) 60(7):3088–98.60(7):3088–3098. doi: 10.1093/rheumatology/kez004
6. Verstappen GM, Pringle S, Bootsma H, Kroese FGM. Epithelial–immune cell interplay in primary sjögren syndrome salivary gland pathogenesis. Nat Rev Rheumatol (2021) 17(6):333–48. doi: 10.1038/s41584-021-00605-2
7. Chivasso C, Sarrand J, Perret J, Delporte C, Soyfoo MS. The involvement of innate and adaptive immunity in the initiation and perpetuation of sjögren’s syndrome. Int J Mol Sci (2021) 22(2):658. doi: 10.3390/ijms22020658
8. Bowman SJ. Primary sjögren’s syndrome. Lupus (2018) 27(1_suppl):32–5. doi: 10.1177/0961203318801673
9. Goules AV, Kapsogeorgou EK, Tzioufas AG. Insight into pathogenesis of sjögren’s syndrome: dissection on autoimmune infiltrates and epithelial cells. Clin Immunol (2017) 182:30–40. doi: 10.1016/j.clim.2017.03.007
10. Rivière E, Pascaud J, Tchitchek N, Boudaoud S, Paoletti A, Ly B, et al. Salivary gland epithelial cells from patients with sjögren’s syndrome induce b-lymphocyte survival and activation. Ann Rheum Dis (2020) 79(11):1468–77. doi: 10.1136/annrheumdis-2019-216588
11. Gottenberg J-E, Busson M, Loiseau P, Cohen-Solal J, Lepage V, Charron D, et al. In primary sjögren’s syndrome, HLA class II is associated exclusively with autoantibody production and spreading of the autoimmune response: association of HLA and primary SS. Arthritis Rheumatol (2003) 48(8):2240–5. doi: 10.1002/art.11103
12. Lessard CJ, Li H, Adrianto I, Ice JA, Rasmussen A, Grundahl KM, et al. Variants at multiple loci implicated in both innate and adaptive immune responses are associated with sjögren’s syndrome. Nat Genet (2013) 45(11):1284–92. doi: 10.1038/ng.2792
13. Li Y, Zhang K, Chen H, Sun F, Xu J, Wu Z, et al. A genome-wide association study in han Chinese identifies a susceptibility locus for primary sjögren’s syndrome at 7q11. 23. Nat Genet (2013) 45(11):1361–5. doi: 10.1038/ng.2779
14. Thorlacius GE, Hultin-Rosenberg L, Sandling JK, Bianchi M, Imgenberg-Kreuz J, Pucholt P, et al. Genetic and clinical basis for two distinct subtypes of primary sjögren’s syndrome. Rheumatol (Oxford). (2021) 60(2):837–48. doi: 10.1093/rheumatology/keaa367
15. Shiboski CH, Shiboski SC, Seror R, Criswell LA, Labetoulle M, Lietman TM, et al. 2016 American College of rheumatology/European league against rheumatism classification criteria for primary sjögren’s syndrome: a consensus and data-driven methodology involving three international patient cohorts: Acr/Eular classification criteria for primary SS. Arthritis Rheumatol (2017) 69(1):35–45. doi: 10.1002/art.39859
16. Vitali C, Bombardieri S, Jonsson R, Moutsopoulos HM, Alexander EL, Carsons SE, et al. Classification criteria for sjögren’s syndrome: a revised version of the European criteria proposed by the American-European consensus group. Ann Rheum Dis (2002) 61(6):554–8. doi: 10.1136/ard.61.6.554
17. Hu S, Loo JA, Wong DT. Human saliva proteome analysis. Ann N Y Acad Sci (2007) 1098(1):323–9. doi: 10.1196/annals.1384.015
18. Baldini C, Giusti L, Bazzichi L, Lucacchini A, Bombardieri S. Proteomic analysis of the saliva: a clue for understanding primary from secondary sjögren’s syndrome? Autoimmun Rev (2008) 7(3):185–91. doi: 10.1016/j.autrev.2007.11.002
19. Fleissig Y, Deutsch O, Reichenberg E, Redlich M, Zaks B, Palmon A, et al. Different proteomic protein patterns in saliva of sjögren’s syndrome patients. Oral Dis (2009) 15(1):61–8. doi: 10.1111/j.1601-0825.2008.01465.x
20. Katsiougiannis S, Wong DTW. The proteomics of saliva in sjögren’s syndrome. Rheum Dis Clin North Am (2016) 42(3):449–56. doi: 10.1016/j.rdc.2016.03.004
21. Aqrawi LA, Galtung HK, Guerreiro EM, Øvstebø R, Thiede B, Utheim TP, et al. Proteomic and histopathological characterisation of sicca subjects and primary sjögren’s syndrome patients reveals promising tear, saliva and extracellular vesicle disease biomarkers. Arthritis Res Ther (2019) 21(1):181. doi: 10.1186/s13075-019-1961-4
22. Sembler-Møller ML, Belstrøm D, Locht H, Pedersen AML. Proteomics of saliva, plasma, and salivary gland tissue in sjögren’s syndrome and non-sjögren patients identify novel biomarker candidates. J Proteomics. (2020) 225(103877):103877. doi: 10.1016/j.jprot.2020.103877
23. Hu S, Wang J, Meijer J, Ieong S, Xie Y, Yu T, et al. Salivary proteomic and genomic biomarkers for primary sjögren’s syndrome. Arthritis Rheumatol (2007) 56(11):3588–600. doi: 10.1002/art.22954
24. Jasim H, Olausson P, Hedenberg-Magnusson B, Ernberg M, Ghafouri B. The proteomic profile of whole and glandular saliva in healthy pain-free subjects. Sci Rep (2016) 6(1):39073. doi: 10.1038/srep39073
25. Aqrawi LA, Galtung HK, Vestad B, Øvstebø R, Thiede B, Rusthen S, et al. Identification of potential saliva and tear biomarkers in primary sjögren’s syndrome, utilising the extraction of extracellular vesicles and proteomics analysis. Arthritis Res Ther (2017) 19(1):14. doi: 10.1186/s13075-017-1228-x
26. Peluso G, De Santis M, Inzitari R, Fanali C, Cabras T, Messana I, et al. Proteomic study of salivary peptides and proteins in patients with sjögren’s syndrome before and after pilocarpine treatment. Arthritis Rheumatol (2007) 56(7):2216–22. doi: 10.1002/art.22738
27. Ottosson L, Hennig J, Espinosa A, Brauner S, Wahren-Herlenius M, Sunnerhagen M. Structural, functional and immunologic characterization of folded subdomains in the Ro52 protein targeted in sjögren’s syndrome. Mol Immunol (2006) 43(6):588–98. doi: 10.1016/j.molimm.2005.04.013
28. Baldini C, Giusti L, Ciregia F, Da Valle Y, Giacomelli C, Donadio E, et al. Proteomic analysis of saliva: a unique tool to distinguish primary sjögren’s syndrome from secondary sjögren’s syndrome and other sicca syndromes. Arthritis Res Ther (2011) 13(6):R194. doi: 10.1186/ar3523
29. Wang JJ, Reed JH, Colella AD, Russell AJ, Murray-Brown W, Chataway TK, et al. Molecular profiling and clonal tracking of secreted rheumatoid factors in primary sjögren’s syndrome. Arthritis Rheumatol (2018) 70(10):1617–25. doi: 10.1002/art.40539
30. Ryu OH, Atkinson JC, Hoehn GT, Illei GG, Hart TC. Identification of parotid salivary biomarkers in sjögren’s syndrome by surface-enhanced laser desorption/ionization time-of-flight mass spectrometry and two-dimensional difference gel electrophoresis. Rheumatol (Oxford). (2006) 45(9):1077–86. doi: 10.1093/rheumatology/kei212
31. Giusti L, Baldini C, Bazzichi L, Ciregia F, Tonazzini I, Mascia G, et al. Proteome analysis of whole saliva: a new tool for rheumatic diseases – the example of sjögren’s syndrome. Proteomics (2007) 7(10):1634–43. doi: 10.1002/pmic.200600783
32. Hu S, Vissink A, Arellano M, Roozendaal C, Zhou H, Kallenberg CGM, et al. Identification of autoantibody biomarkers for primary sjögren’s syndrome using protein microarrays. Proteomics (2011) 11(8):1499–507. doi: 10.1002/pmic.201000206
33. Wei P, Kuo WP, Chen F, Hua H. Diagnostic model of saliva peptide finger print analysis of primary sjögren’s syndrome patients by using weak cation exchange magnetic beads. Biosci Rep (2013) 33(4):567–73. doi: 10.1042/BSR20130022
34. Gallo A. Gross cystic disease fluid protein-15(GCDFP-15)/prolactin-inducible protein (PIP) as functional salivary biomarker for primary sjögren’s syndrome. J Genet Syndr Gene Ther (2013) 04(04):10.4172/2157-7412. doi: 10.4172/2157-7412.1000140
35. Deutsch O, Krief G, Konttinen YT, Zaks B, Wong DT, Aframian DJ, et al. Identification of sjögren’s syndrome oral fluid biomarker candidates following high-abundance protein depletion. Rheumatol (Oxford). (2015) 54(5):884–90. doi: 10.1093/rheumatology/keu405
36. Delaleu N, Mydel P, Kwee I, Brun JG, Jonsson MV, Jonsson R. High fidelity between saliva proteomics and the biologic state of salivary glands defines biomarker signatures for primary sjögren’s syndrome: salivary proteomics closely mirrors the biologic state of salivary glands. Arthritis Rheumatol (2015) 67(4):1084–95. doi: 10.1002/art.39015
37. Delaleu N, Mydel P, Brun JG, Jonsson MV, Alimonti A, Jonsson R. Sjögren’s syndrome patients with ectopic germinal centers present with a distinct salivary proteome. Rheumatol (Oxford). (2016) 55(6):1127–37. doi: 10.1093/rheumatology/kew013
38. Chaudhury NMA, Proctor GB, Karlsson NG, Carpenter GH, Flowers SA. Reduced mucin-7 (Muc7) sialylation and altered saliva rheology in sjögren’s syndrome associated oral dryness. Mol Cell Proteomics. (2016) 15(3):1048–59. doi: 10.1074/mcp.M115.052993
39. Bosello S, Peluso G, Iavarone F, Tolusso B, Messana I, Faa G, et al. Thymosin β4 and β10 in sjögren’s syndrome: saliva proteomics and minor salivary glands expression. Arthritis Res Ther (2016) 18(1):229. doi: 10.1186/s13075-016-1134-7
40. Hall SC, Hassis ME, Williams KE, Albertolle ME, Prakobphol A, Dykstra AB, et al. Alterations in the salivary proteome and n-glycome of sjögren’s syndrome patients. J Proteome Res (2017) 16(4):1693–705. doi: 10.1021/acs.jproteome.6b01051
41. Cui L, Elzakra N, Xu S, Xiao GG, Yang Y, Hu S. Investigation of three potential autoantibodies in sjogren’s syndrome and associated MALT lymphoma. Oncotarget (2017) 8(18):30039–49. doi: 10.18632/oncotarget.15613
42. Jazzar AA, Shirlaw PJ, Carpenter GH, Challacombe SJ, Proctor GB. Salivary S100A8/A9 in sjögren’s syndrome accompanied by lymphoma. J Oral Pathol Med (2018) 47(9):900–6. doi: 10.1111/jop.12763
43. Cecchettini A, Finamore F, Ucciferri N, Donati V, Mattii L, Polizzi E, et al. Phenotyping multiple subsets in sjögren’s syndrome: a salivary proteomic SWATH-MS approach towards precision medicine. Clin Proteomics. (2019) 16(1):26. doi: 10.1186/s12014-019-9245-1
44. Hu S, Zhou M, Jiang J, Wang J, Elashoff D, Gorr S, et al. Systems biology analysis of sjögren’s syndrome and mucosa-associated lymphoid tissue lymphoma in parotid glands. Arthritis Rheumatol (2009) 60(1):81–92. doi: 10.1002/art.24150
45. Hjelmervik TOR, Jonsson R, Bolstad AI. The minor salivary gland proteome in sjögren’s syndrome. Oral Dis (2009) 15(5):342–53. doi: 10.1111/j.1601-0825.2009.01531.x
46. Van den Bergh K, Hooijkaas H, Blockmans D, Westhovens R, Op De Beéck K, Verschueren P, et al. Heterogeneous nuclear ribonucleoprotein h1, a novel nuclear autoantigen. Clin Chem (2009) 55(5):946–54. doi: 10.1373/clinchem.2008.115626
47. Lindop R, Arentz G, Chataway TK, Thurgood LA, Jackson MW, Reed JH, et al. Molecular signature of a public clonotypic autoantibody in primary sjögren’s syndrome: a “forbidden” clone in systemic autoimmunity. Arthritis Rheumatol (2011) 63(11):3477–86. doi: 10.1002/art.30566
48. Arentz G, Thurgood LA, Lindop R, Chataway TK, Gordon TP. Secreted human Ro52 autoantibody proteomes express a restricted set of public clonotypes. J Autoimmun (2012) 39(4):466–70. doi: 10.1016/j.jaut.2012.07.003
49. Thurgood LA, Arentz G, Lindop R, Jackson MW, Whyte AF, Colella AD, et al. An immunodominant La/SSB autoantibody proteome derives from public clonotypes: public La/SSB autoantibody clonotypes. Clin Exp Immunol (2013) 174(2):237–44. doi: 10.1111/cei.12171
50. Li Y, Sun X, Zhang X, Yang Y, Jia R, Liu X, et al. Establishment of a novel diagnostic model for sjögren’s syndrome by proteomic fingerprinting. Clin Rheumatol (2014) 33(12):1745–50. doi: 10.1007/s10067-014-2762-4
51. Ohyama K, Baba M, Tamai M, Aibara N, Ichinose K, Kishikawa N, et al. Proteomic profiling of antigens in circulating immune complexes associated with each of seven autoimmune diseases. Clin Biochem (2015) 48(3):181–5. doi: 10.1016/j.clinbiochem.2014.11.008
52. Nishikawa A, Suzuki K, Kassai Y, Gotou Y, Takiguchi M, Miyazaki T, et al. Identification of definitive serum biomarkers associated with disease activity in primary sjögren’s syndrome. Arthritis Res Ther (2016) 18(1):106. doi: 10.1186/s13075-016-1006-1
53. Liao C-C, Chou P-L, Cheng C-W, Chang Y-S, Chi W-M, Tsai K-L, et al. Comparative analysis of novel autoantibody isotypes against citrullinated-inter-alpha-trypsin inhibitor heavy chain 3 (ITIH3)542–556 peptide in serum from Taiwanese females with rheumatoid arthritis, primary sjögren’s syndrome and secondary sjögren’s syndrome in rheumatoid arthritis. J Proteomics. (2016) 141:1–11. doi: 10.1016/j.jprot.2016.03.031
54. Tasaki S, Suzuki K, Nishikawa A, Kassai Y, Takiguchi M, Kurisu R, et al. Multiomic disease signatures converge to cytotoxic CD8 T cells in primary sjögren’s syndrome. Ann Rheum Dis (2017) 76(8):1458–66. doi: 10.1136/annrheumdis-2016-210788
55. Wang JJ, Al Kindi MA, Colella AD, Dykes L, Jackson MW, Chataway TK, et al. IgV peptide mapping of native Ro60 autoantibody proteomes in primary sjögren’s syndrome reveals molecular markers of Ro/La diversification. Clin Immunol (2016) 173:57–63. doi: 10.1016/j.clim.2016.09.001
56. Burbelo PD, Ferré EMN, Chaturvedi A, Chiorini JA, Alevizos I, Lionakis MS, et al. Profiling autoantibodies against salivary proteins in sicca conditions. J Dent Res (2019) 98(7):772–8. doi: 10.1177/0022034519850564
57. Bodewes ILA, van der Spek PJ, Leon LG, Wijkhuijs AJM, van Helden-Meeuwsen CG, Tas L, et al. Fatigue in sjögren’s syndrome: a search for biomarkers and treatment targets. Front Immunol (2019) 10:312. doi: 10.3389/fimmu.2019.00312
58. Qiao L, Deng C, Wang Q, Zhang W, Fei Y, Xu Y, et al. Serum clusterin and complement factor h may be biomarkers differentiate primary sjögren’s syndrome with and without neuromyelitis optica spectrum disorder. Front Immunol (2019) 10:2527. doi: 10.3389/fimmu.2019.02527
59. Ohlsson M, Hellmark T, Bengtsson AA, Theander E, Turesson C, Klint C, et al. Proteomic data analysis for differential profiling of the autoimmune diseases SLE, RA, SS, and ANCA-associated vasculitis. J Proteome Res (2021) 20(2):1252–60. doi: 10.1021/acs.jproteome.0c00657
60. Huang S, Zheng F, Liu L, Meng S, Cai W, Zhang C, et al. Integrated proteome and phosphoproteome analyses of peripheral blood mononuclear cells in primary sjögren syndrome patients. Aging (Albany NY). (2020) 13(1):1071–95. doi: 10.18632/aging.202233
61. Tian Q, Zhao H, Ling H, Sun L, Xiao C, Yin G, et al. Poly(ADP -ribose) polymerase enhances infiltration of mononuclear cells in primary sjögren’s syndrome through interferon-induced protein with tetratricopeptide repeats 1–mediated up-regulation of CXCL 10. Arthritis Rheumatol (2020) 72(6):1003–12. doi: 10.1002/art.41195
62. Schmidt A, Farine H, Keller MP, Sebastian A, Kozera L, Welford RWD, et al. Immunoaffinity targeted mass spectrometry analysis of human plasma samples reveals an imbalance of active and inactive CXCL10 in primary sjögren’s syndrome disease patients. J Proteome Res (2020) 19(10):4196–209. doi: 10.1021/acs.jproteome.0c00494
63. Thiagarajan D, Oparina N, Lundström S, Zubarev R, Sun J. PRECISESADS clinical consortium, et al. IgM antibodies against malondialdehyde and phosphorylcholine in different systemic rheumatic diseases. Sci Rep (2020) 10(1):11010. doi: 10.1038/s41598-020-66981-z
64. Mogi M, Kage T, Chino T, Yoshitake K, Harada M. Increased beta 2-microglobulin in both parotid and submandibular/sublingual saliva from patients with sjögren’s syndrome. Arch Oral Biol (1994) 39(10):913–5. doi: 10.1016/0003-9969(94)90024-8
65. Hu S, Gao K, Pollard R, Arellano-Garcia M, Zhou H, Zhang L, et al. Preclinical validation of salivary biomarkers for primary sjögren’s syndrome. Arthritis Care Res (Hoboken). (2010) 62(11):1633–8. doi: 10.1002/acr.20289
66. Michalski JP, Daniels TE, Talal N, Grey HM. Beta2Microglobulin and lymphocytic infiltration in sjögren’s syndrome. N Engl J Med (1975) 293(24):1228–31. doi: 10.1056/NEJM197512112932404
67. Yoneda M, Fujii A, Ito A, Yokoyama H, Nakagawa H, Kuriyama M. High prevalence of serum autoantibodies against the amino terminal of alpha-enolase in hashimoto’s encephalopathy. J Neuroimmunol (2007) 185(1–2):195–200. doi: 10.1016/j.jneuroim.2007.01.018
68. He P, Naka T, Serada S, Fujimoto M, Tanaka T, Hashimoto S, et al. Proteomics-based identification of alpha-enolase as a tumor antigen in non-small lung cancer. Cancer Sci (2007) 98(8):1234–40. doi: 10.1111/j.1349-7006.2007.00509.x
69. Olivares-Martínez E, Hernández-Ramírez DF, Núñez-Álvarez CA, Llorente L, Hernandez-Molina G. α-enolase is an antigenic target in primary sjögren’s syndrome. Clin Exp Rheumatol (2019) 37 Suppl 118(3):29–35.
70. Singer E, Markó L, Paragas N, Barasch J, Dragun D, Müller DN, et al. Neutrophil gelatinase-associated lipocalin: pathophysiology and clinical applications. Acta Physiol (Oxf). (2013) 207(4):663–72. doi: 10.1111/apha.12054
71. Aqrawi LA, Jensen JL, Fromreide S, Galtung HK, Skarstein K. Expression of NGAL-specific cells and mRNA levels correlate with inflammation in the salivary gland, and its overexpression in the saliva, of patients with primary sjögren’s syndrome. Autoimmunity (2020) 53(6):333–43. doi: 10.1080/08916934.2020.1795140
72. Carlsson H, Yhr M, Petersson S, Collins N, Polyak K, Enerbäck C. Psoriasin (S100A7) and calgranulin-b (S100A9) induction is dependent on reactive oxygen species and is downregulated by bcl-2 and antioxidants. Cancer Biol Ther (2005) 4(9):998–1005. doi: 10.4161/cbt.4.9.1969
73. Dorkhan M, Svensäter G, Davies JR. Salivary pellicles on titanium and their effect on metabolic activity in streptococcus oralis. BMC Oral Health (2013) 13(1):32. doi: 10.1186/1472-6831-13-32
74. Edechi CA, Nasr MR, Karim A, Blanchard AA, Ellison CA, Qui H, et al. The prolactin inducible protein/gross cystic disease fluid protein-15 deficient mice develop anomalies in lymphoid organs. Immunobiology (2019) 224(6):811–6. doi: 10.1016/j.imbio.2019.08.005
75. Bardow A, Moe D, Nyvad B, Nauntofte B. The buffer capacity and buffer systems of human whole saliva measured without loss of CO2. Arch Oral Biol (2000) 45(1):1–12. doi: 10.1016/s0003-9969(99)00119-3
76. Baron A, DeCarlo A, Featherstone J. Functional aspects of the human salivary cystatins in the oral environment. Oral Dis (1999) 5(3):234–40. doi: 10.1111/j.1601-0825.1999.tb00307.x
77. Falco A, Mas V, Tafalla C, Perez L, Coll JM, Estepa A. Dual antiviral activity of human alpha-defensin-1 against viral haemorrhagic septicaemia rhabdovirus (VHSV): inactivation of virus particles and induction of a type I interferon-related response. Antiviral Res (2007) 76(2):111–23. doi: 10.1016/j.antiviral.2007.06.006
78. Deming FP, Al-Hashimi I, Haghighat N, Hallmon WW, Kerns DG, Abraham C, et al. Comparison of salivary calmodulin binding proteins in sjögren’s syndrome and healthy individuals: calmodulin binding proteins in sjögren’s syndrome. J Oral Pathol Med (2007) 36(3):132–5. doi: 10.1111/j.1600-0714.2006.00494.x
79. Franceschini F, Cavazzana I. Anti-Ro/SSA and La/SSB antibodies. Autoimmunity (2005) 38(1):55–63. doi: 10.1080/08916930400022954
80. Strandberg L, Ambrosi A, Espinosa A, Ottosson L, Eloranta M-L, Zhou W, et al. Interferon-alpha induces up-regulation and nuclear translocation of the Ro52 autoantigen as detected by a panel of novel Ro52-specific monoclonal antibodies. J Clin Immunol (2008) 28(3):220–31. doi: 10.1007/s10875-007-9157-0
81. Schulte-Pelkum J, Fritzler M, Mahler M. Latest update on the Ro/SS-a autoantibody system. Autoimmun Rev (2009) 8(7):632–7. doi: 10.1016/j.autrev.2009.02.010
82. Menéndez A, Gómez J, Escanlar E, Caminal-Montero L, Mozo L. Clinical associations of anti-SSA/Ro60 and anti-Ro52/TRIM21 antibodies: diagnostic utility of their separate detection. Autoimmunity (2013) 46(1):32–9. doi: 10.3109/08916934.2012.732131
83. Ohlsson M, Jonsson R, Brokstad KA. Subcellular redistribution and surface exposure of the Ro52, Ro60 and La48 autoantigens during apoptosis in human ductal epithelial cells: a possible mechanism in the pathogenesis of sjögren’s syndrome: SS autoantigens and apoptosis. Scand J Immunol (2002) 56(5):456–69. doi: 10.1046/j.1365-3083.2002.01072_79.x
84. Aqrawi LA, Skarstein K, Øijordsbakken G, Brokstad KA. Ro52- and Ro60-specific b cell pattern in the salivary glands of patients with primary sjögren’s syndrome: SSA-specific b cells in SG of pSS patients. Clin Exp Immunol (2013) 172(2):228–37. doi: 10.1111/cei.12058
85. Theander E, Jonsson R, Sjöström B, Brokstad K, Olsson P, Henriksson G. Prediction of sjögren’s syndrome years before diagnosis and identification of patients with early onset and severe disease course by autoantibody profiling: prediagnostic autoantibody profiling in primary SS. Arthritis Rheumatol (2015) 67(9):2427–36. doi: 10.1002/art.39214
86. Quartuccio L, Baldini C, Bartoloni E, Priori R, Carubbi F, Corazza L, et al. Anti-SSA/SSB-negative sjögren’s syndrome shows a lower prevalence of lymphoproliferative manifestations, and a lower risk of lymphoma evolution. Autoimmun Rev (2015) 14(11):1019–22. doi: 10.1016/j.autrev.2015.07.002
87. Ebana Y, Ozaki K, Inoue K, Sato H, Iida A, Lwin H, et al. A functional SNP in ITIH3 is associated with susceptibility to myocardial infarction. J Hum Genet (2007) 52(3):220–9. doi: 10.1007/s10038-006-0102-5
88. Zhuo L, Hascall VC, Kimata K. Inter-alpha-trypsin inhibitor, a covalent protein-glycosaminoglycan-protein complex. J Biol Chem (2004) 279(37):38079–82. doi: 10.1074/jbc.R300039200
89. Terzoglou AG, Routsias JG, Moutsopoulos HM, Tzioufas AG. Post-translational modifications of the major linear epitope 169-190aa of Ro60 kDa autoantigen alter the autoantibody binding. Clin Exp Immunol (2006) 146(1):60–5. doi: 10.1111/j.1365-2249.2006.03192.x
90. Herrera-Esparza R, Rodríguez-Rodríguez M, Pérez-Pérez ME, Badillo-Soto MA, Torres-Del-Muro F, Bollain-Y-Goytia JJ, et al. Posttranslational protein modification in the salivary glands of sjögren’s syndrome patients. Autoimmune Dis (2013) 2013:548064. doi: 10.1155/2013/548064
91. Sembler-Møller ML, Belstrøm D, Locht H, Pedersen AML. Combined serum anti-SSA/Ro and salivary TRIM29 reveals promising high diagnostic accuracy in patients with primary sjögren’s syndrome. PloS One (2021) 16(10):e0258428. doi: 10.1371/journal.pone.0258428
92. Theander E. Lymphoma and other malignancies in primary sjogren’s syndrome: a cohort study on cancer incidence and lymphoma predictors. Ann Rheum Dis (2006) 65(6):796–803. doi: 10.1136/ard.2005.041186
93. Liang Y, Yang Z, Qin B, Zhong R. Primary sjögren’s syndrome and malignancy risk: a systematic review and meta-analysis. Ann Rheum Dis (2014) 73(6):1151–6. doi: 10.1136/annrheumdis-2013-203305
94. Kapsogeorgou EK, Voulgarelis M, Tzioufas AG. Predictive markers of lymphomagenesis in sjögren’s syndrome: from clinical data to molecular stratification. J Autoimmun (2019) 104(102316):102316. doi: 10.1016/j.jaut.2019.102316
95. Alevizos I, Alexander S, Turner RJ, Illei GG. MicroRNA expression profiles as biomarkers of minor salivary gland inflammation and dysfunction in sjögren’s syndrome. Arthritis Rheumatol (2011) 63(2):535–44. doi: 10.1002/art.30131
96. Zilahi E, Tarr T, Papp G, Griger Z, Sipka S, Zeher M. Increased microRNA-146a/b, TRAF6 gene and decreased IRAK1 gene expressions in the peripheral mononuclear cells of patients with sjögren’s syndrome. Immunol Lett (2012) 141(2):165–8. doi: 10.1016/j.imlet.2011.09.006
97. Tandon M, Gallo A, Jang S-I, Illei GG, Alevizos I. Deep sequencing of short RNAs reveals novel microRNAs in minor salivary glands of patients with sjögren’s syndrome: novel miRNA discovery in sjögren’s syndrome. Oral Dis (2012) 18(2):127–31. doi: 10.1111/j.1601-0825.2011.01849.x
98. Peng L, Ma W, Yi F, Yang Y-J, Lin W, Chen H, et al. MicroRNA profiling in Chinese patients with primary sjögren syndrome reveals elevated miRNA-181a in peripheral blood mononuclear cells. J Rheumatol (2014) 41(11):2208–13. doi: 10.3899/jrheum.131154
99. Shi H, Zheng L-Y, Zhang P, Yu C-Q. miR-146a and miR-155 expression in PBMCs from patients with sjögren’s syndrome. J Oral Pathol Med (2014) 43(10):792–7. doi: 10.1111/jop.12187
100. Chen J-Q, Zilahi E, Papp G, Sipka S, Zeher M. Simultaneously increased expression of microRNA-155 and suppressor of cytokine signaling 1 (SOCS1) gene in the peripheral blood mononuclear cells of patients with primary sjögren’s syndrome. Int J Rheum Dis (2017) 20(5):609–13. doi: 10.1111/1756-185x.12804
101. Gourzi VC, Kapsogeorgou EK, Kyriakidis NC, Tzioufas AG. Study of microRNAs (miRNAs) that are predicted to target the autoantigens Ro/SSA and La/SSB in primary sjögren’s syndrome: MiRNAs targeting Ro/SSA and La/SSB autoantigens in sjögren’s syndrome. Clin Exp Immunol (2015) 182(1):14–22. doi: 10.1111/cei.12664
102. Williams AEG, Choi K, Chan AL, Lee YJ, Reeves WH, Bubb MR, et al. Sjögren’s syndrome-associated microRNAs in CD14(+) monocytes unveils targeted TGFβ signaling. Arthritis Res Ther (2016) 18(1):95. doi: 10.1186/s13075-016-0987-0
103. Yan T, Shen J, Chen J, Zhao M, Guo H, Wang Y. Differential expression of miR-17-92 cluster among varying histological stages of minor salivary gland in patients with primary sjögren’s syndrome. Clin Exp Rheumatol (2019) 37 Suppl 118(3):49–54.
104. Chen JQ, Papp G, Póliska S, Szabó K, Tarr T, Bálint BL, et al. MicroRNA expression profiles identify disease-specific alterations in systemic lupus erythematosus and primary sjögren’s syndrome. PloS One (2017) 12(3):e0174585. doi: 10.1371/journal.pone.0174585
105. Jiang Z, Tao J-H, Zuo T, Li X-M, Wang G-S, Fang X, et al. The correlation between miR-200c and the severity of interstitial lung disease associated with different connective tissue diseases. Scand J Rheumatol (2017) 46(2):122–9. doi: 10.3109/03009742.2016.1167950
106. Lopes AP, Hillen MR, Chouri E, Blokland SLM, Bekker CPJ, Kruize AA, et al. Circulating small non-coding RNAs reflect IFN status and b cell hyperactivity in patients with primary sjögren’s syndrome. PloS One (2018) 13(2):e0193157. doi: 10.1371/journal.pone.0193157
107. Kapsogeorgou EK, Papageorgiou A, Protogerou AD, Voulgarelis M, Tzioufas AG. Low miR200b-5p levels in minor salivary glands: a novel molecular marker predicting lymphoma development in patients with sjögren’s syndrome. Ann Rheum Dis (2018) 77(8):1200–7. doi: 10.1136/annrheumdis-2017-212639
108. Jiang C-R, Li H-L. The value of MiR-146a and MiR-4484 expressions in the diagnosis of anti-SSA antibody positive sjogren syndrome and the correlations with prognosis. Eur Rev Med Pharmacol Sci (2018) 22(15):4800–5. doi: 10.26355/eurrev_201808_15614
109. Wang-Renault S-F, Boudaoud S, Nocturne G, Roche E, Sigrist N, Daviaud C, et al. Deregulation of microRNA expression in purified T and b lymphocytes from patients with primary sjögren’s syndrome. Ann Rheum Dis (2018) 77(1):133–40. doi: 10.1136/annrheumdis-2017-211417
110. Wang J, Wang X, Wang L, Sun C, Xie C, Li Z. MiR-let-7d-3p regulates IL-17 expression through targeting AKT1/mTOR signaling in CD4+ T cells. In Vitro Cell Dev Biol Anim. (2020) 56(1):67–74. doi: 10.1007/s11626-019-00409-5
111. Hillen MR, Chouri E, Wang M, Blokland SLM, Hartgring SAY, Concepcion AN, et al. Dysregulated miRNome of plasmacytoid dendritic cells from patients with sjögren’s syndrome is associated with processes at the centre of their function. Rheumatol (Oxford). (2019) 58(12):2305–14. doi: 10.1093/rheumatology/kez195
112. Gallo A, Vella S, Tuzzolino F, Cuscino N, Cecchettini A, Ferro F, et al. MicroRNA-mediated regulation of mucin-type O-glycosylation pathway: a putative mechanism of salivary gland dysfunction in sjögren syndrome. J Rheumatol (2019) 46(11):1485–94. doi: 10.3899/jrheum.180549
113. Talotta R, Mercurio V, Bongiovanni S, Vittori C, Boccassini L, Rigamonti F, et al. Evaluation of salivary and plasma microRNA expression in patients with sjögren’s syndrome, and correlations with clinical and ultrasonographic outcomes. Clin Exp Rheumatol (2019) 37 Suppl 118(3):70–7.
114. Sembler-Møller ML, Belstrøm D, Locht H, Pedersen AML. Distinct microRNA expression profiles in saliva and salivary gland tissue differentiate patients with primary sjögren’s syndrome from non-sjögren’s sicca patients. J Oral Pathol Med (2020) 49(10):1044–52. doi: 10.1111/jop.13099
115. Yang Y, Hou Y, Li J, Zhang F, Du Q. Characterization of antiapoptoticmicroRNAsin primary sjögren’s syndrome. Cell Biochem Funct (2020) 38(8):1111–8. doi: 10.1002/cbf.3569
116. Gong B, Zheng L, Lu Z, Huang J, Pu J, Pan S, et al. Mesenchymal stem cells negatively regulate CD4+ T cell activation in patients with primary sjögren syndrome through the miRNA−125b and miRNA−155 TCR pathway. Mol Med Rep (2021) 23(1):1. doi: 10.3892/mmr.2020.1168
117. Salama I, Malone PS, Mihaimeed F, Jones JL. A review of the S100 proteins in cancer. Eur J Surg Oncol (2008) 34(4):357–64. doi: 10.1016/j.ejso.2007.04.009
118. Bresnick AR, Weber DJ, Zimmer DB. S100 proteins in cancer. Nat Rev Cancer. (2015) 15(2):96–109. doi: 10.1038/nrc3893
119. Alevizos I, Illei GG. MicroRNAs in sjögren’s syndrome as a prototypic autoimmune disease. Autoimmun Rev (2010) 9(9):618–21. doi: 10.1016/j.autrev.2010.05.009
120. Pauley KM, Stewart CM, Gauna AE, Dupre LC, Kuklani R, Chan AL, et al. Altered miR-146a expression in sjögren’s syndrome and its functional role in innate immunity: innate immunity. Eur J Immunol (2011) 41(7):2029–39. doi: 10.1002/eji.201040757
121. Wang Y, Zhang G, Zhang L, Zhao M, Huang H. Decreased microRNA-181a and -16 expression levels in the labial salivary glands of sjögren syndrome patients. Exp Ther Med (2018) 15(1):426–32. doi: 10.3892/etm.2017.5407
122. Mogilyansky E, Rigoutsos I. The miR-17/92 cluster: a comprehensive update on its genomics, genetics, functions and increasingly important and numerous roles in health and disease. Cell Death Differ (2013) 20(12):1603–14. doi: 10.1038/cdd.2013.125
123. Veerman EC, Valentijn-Benz M, Amerongen N. Viscosity of human salivary mucins: effect of pH and ionic-strength and role of sialic-acid. J Biol Buccale. (1989) 17(4):297–306.
Keywords: Sjögren’s syndrome, proteomics, miRNA - microRNA, salivary glands, saliva
Citation: Kamounah S, Sembler-Møller ML, Nielsen CH and Pedersen AML (2023) Sjögren’s syndrome: novel insights from proteomics and miRNA expression analysis. Front. Immunol. 14:1183195. doi: 10.3389/fimmu.2023.1183195
Received: 09 March 2023; Accepted: 09 May 2023;
Published: 18 May 2023.
Edited by:
Alexandre Wagner Silva De Souza, Federal University of São Paulo, BrazilReviewed by:
Liting Jiang, Shanghai Jiao Tong University, ChinaJason Dale Turner, University of Birmingham, United Kingdom
Copyright © 2023 Kamounah, Sembler-Møller, Nielsen and Pedersen. This is an open-access article distributed under the terms of the Creative Commons Attribution License (CC BY). The use, distribution or reproduction in other forums is permitted, provided the original author(s) and the copyright owner(s) are credited and that the original publication in this journal is cited, in accordance with accepted academic practice. No use, distribution or reproduction is permitted which does not comply with these terms.
*Correspondence: Anne Marie Lynge Pedersen, amlp@sund.ku.dk