- 1State Key Laboratory of Animal Nutrition, College of Animal Science and Technology, China Agricultural University, Beijing, China
- 2Animal Production Systems Group, Wageningen University & Research, Wageningen, Netherlands
- 3School of Agriculture, Ningxia University, Yinchuan, China
The transition period is the stage of the high incidence of metabolic and infectious diseases in dairy cows. Improving transition dairy cows’ health is crucial for the industry. This study aimed to determine the effects of dietary supplementation medium-chain fatty acids (MCFAs) on immune function, metabolic status, performance of transition dairy cows. Twenty multiparous Holstein cows randomly assigned to two treatments at 35 d before calving. 1) CON (fed the basal 2) MCFA treatment (basal diet was supplemented at an additional 20 g MCFAs mixture every day) until 70 d after calving. The results showed that the serum amyloid A myeloperoxidase concentrations in the blood of cows in MCFA treatment significantly decreased during the early lactation (from 1 d to 28 d after calving) 0.03, 0.04, respectively) compared with the CON, while the tumor necrosis factor concentration was significantly decreased at 56 d after calving (P = 0.02). In addition, the concentration of insulin in the pre-calving (from 21 d before calving to calving) blood of cows in MCFA treatment was significantly decreased (P = 0.04), and concentration of triglyceride also showed a downward trend at 28 d after calving 0.07). Meanwhile, MCFAs supplementation significantly decreased the concentrations of lithocholic acid, hyodeoxycholic acid, and hyocholic acid in the blood at 1 d calving (P = 0.02, < 0.01, < 0.01, respectively), and the level of hyocholic acid taurocholic acid concentrations (P < 0.01, = 0.01, respectively) decreased dramatically at 14 d after calving. However, compared with the CON, the pre-calving dry matter intake and the early lactation milk yield in MCFA treatment were significantly decreased (P = 0.05, 0.02, respectively). In conclusion, MCFAs supplementation transition diet could improve the immune function and metabolic status of dairy cows, and the health of transition cows might be beneficial from the endocrine status.
Introduction
The transition period is widely regarded as the most challenging period o the lactation cycle of dairy cows (1). To cope with various challenges, it is necessary to use the integrated adaptation mechanism coordinated by the endocrine system and the immune system (2, 3). However, adaptive ability varies among individual dairy cows (4), and different degrees of immunosuppression might occur, further resulting in infectious and metabolic diseases (5).
The lack of energy in transition (6, 7) results in the mobilization of body fat (8). Non-esterified fatty acid (NEFA) (9), triglyceride (TG), and ketone bodies are produced in this process (10), which affects liver metabolism, resulting in complex and difficult-to-regulate systemic responses (11). Meanwhile, the increase in the feed energy density of post-partum cows may lead to (12) a decrease in dry matter intake (DMI) and feed efficiency (13). Studies have shown that decreased DMI and increased negative energy balance (NEB) could trigger competition for essential nutrients between the immune system and milk generation. The lack of energy will impact immune response and immune cell function, resulting in immune suppression and increased disease susceptibility (14). Meanwhile, transition immunosuppression and excessive inflammatory responses are predisposing factors for metabolic disorders (15). Therefore, the disturbance of nutrient metabolism and immune homeostasis in dairy cows may cause harmful feedback loops, which affect the health and performance of dairy cows (2).
MCFAs (Medium chain fatty acids) are fatty acids containing 6-12 carbon atoms and are found mainly in coconut oil and palm kernel oil (16). After ingestion, MCFAs are absorbed by the gastrointestinal epithelial cells, some of which can be absorbed by the epithelial cells to enhance intestinal integrity (17), and some can enter the liver through the portal vein and directly metabolize. Besides, MCFAs can regulate the metabolism of carbohydrates and lipids in the liver (18) and promote the formation of liver glycogen (19) and the synthesis and excretion of hepatic bile acids (20, 21). In addition, MCFAs can regulate the release of inflammatory cytokines, such as IL-6 and IL-8, preventing inflammation and decreasing the damage caused by inflammation (22).
Currently, the application of MCFAs in animal production is mainly carried out on monogastric animals. It was reported that MCFA supplementation could improve DMI, feed efficiency, and the average daily gain of nursery pigs (23). The application values of MCFAs in the monogastric animal are attributed to their antipathogen activity and immune-improving reaction (24, 25). However, the application values of MCFAs in ruminants are still controversial. Relevant studies showed that MCFA supplementation could improve fat digestibility (26) and promote milk fat synthesis in lactating dairy cattle (27, 28). Nevertheless, other studies on dairy cows and swamp buffalo have reported that MCFAs decrease the digestibility of fiber, which leads to a decline in DMI (29, 30). Besides, several cell studies have shown that MCFAs have antipathogen (31) and anti-inflammatory activities (32). It carries out the relevant functions by stimulating cellular immunity and supporting transition cows to face various challenges (33), such as reducing the incidence rate of mastitis (34, 35). Moreover, there are few studies on MCFAs in transition dairy cows (36), so it is necessary for us to explore the application prospects of MCFAs in peripartum cows.
Overall, our hypothesis focuses on whether MCFAs could improve the immune function and metabolic status of dairy cows in the transition period and whether MCFA supplementation could modify dairy cows’ rumen fermentation and performance.
Materials and methods
Animals involved in this experiment were fed according to the guidelines from the committee of animal welfare and animal experimental ethical inspection of China Agricultural University. The committee reviewed and approved the experiment and all procedures involving animals (Protocol number: CAU20201024-2).
Cows, housing, feeding management,and treatment
Twenty healthy multiparity Holstein dairy cows with similar body condition scores (parity = 2.50 ± 1.42; body condition score = 3.28 ± 0.23; mean ± SD) were randomly assigned to two treatments, with 10 cows in each treatment. The control group (CON; parity = 2.67 ± 1.32; body condition score = 3.33 ± 0.28) was fed a basal diet, while the transition diet was followed in the pre-calving stage (21 d before calving to calving) and the lactation diet was followed in the early lactation (1 d to 28 d after calving) and peak lactation diet (29 d to 70 d after calving) (Table 1). The MCFA treatment (MCFA; parity = 2.33 ± 1.58; body condition score = 3.22 ± 0.15) was supplemented with 20 g of MCFA mixture consisting of 10% octanoic acid, 7% decanoic acid, 17% lauric acid, and 66% carrier (consisting of silica, wheat flour, and barley flour).
The experiment was carried out in a barn equipped with automatic feeding bins (RIC, Roughage Intake Control, Insentec B.V. Marknesse). The barn was divided into two parts before the experiment (Barn 1 and Barn 2) to separate the transition and lactating cows. Cows were transferred to Barn 1 35 d before calving and 14 d for the adaptive phase and remained there until calving. After calving, they were transferred to Barn 2 until the end of the experiment. Both Barn 1 and Barn 2 were equipped with adequate feeding bins, cubicles, and water bins, providing cows with a comfortable environment, guaranteeing the experiment’s success and the animals’ welfare. No additional MCFA mixture was added to the cows’ diet during these two days as the cows needed to be taken care of in the calving room after calving, which took 1-2 d.
Animal performance
The feed intake of dairy cows was recorded by the automatic RIC system. TMR samples were collected weekly before calving and every two weeks during the lactation period. After the feed samples were collected and weighed, they were dried in a forced-air-drying oven at 55 degrees for 48 hours (Senxin experimental instrument series DGG-9003, Shanghai, China). The dried samples were then equilibrated at ambient temperature and humidity before reweighing. Each cow wore a collar that recorded its rumination behavior after calving. An electronic monitoring system (HR-TAG-LD, SCR Engineers Ltd) recorded data every two hours to monitor the cow’s daily rumination time.
At the 6th, 12th, 18th, and 24th hours of the last 3 d (68 d - 70 d after calving), 300 - 500 g feces were collected from the rectum, and the fecal samples were mixed with 10% tartaric acid and dried in a forced-air-drying oven at 55 degrees for 48 h (37). The dried TMR and feces samples were ground using a high-speed vertical grinder (RT-34, Kunjie Yucheng Machinery Equipment, Beijing, China) and then passed through a 1 mm mesh screen. Sieved TMR and feces samples were collected and tested for crude protein (CP) (Dumas combustion, Rapid MAX N Exceed, Elementar Analysensysteme GmbH, Hanau, Germany), ether extract (EE) (ANKOM XT15Ii, Extractor, ANKOM Technology, Macedon, America), neutral detergent fiber (NDF), and acid detergent fiber (ADF) (38) (ANKOM 2000Ii, Automated Fiber Analyzer, ANKOM Technology, Macedonia, USA), and the digestibility of each nutrient was calculated (39).
Daily milk production was recorded using an automatic milking system (ALPROTM, DeLaval, Sweden Tumba) for 70 d after calving. On days 14, 28, 42, 56, and 70 after calving, 50 mL milk was collected at the ratio of morning: afternoon: night (4:3:3). After mixing, 50mL milk was immediately sent to the lab to determine the content of milk fat, milk protein, and lactose, as well as the levels of urea nitrogen and the somatic cell count (SCC) (near-infrared reflectance spectroscopy, Series300 combi-foss; Foss Electric, Schiller ød, Denmark). Energy-corrected milk (ECM = 0.327× kg/d of milk+12.95× kg/d of milk fat yield+7.2× kg/d of milk protein yield) and fat-corrected milk (FCM = 0.4× kg/d of milk yield+15×milk fat %× kg/d of milk yield) were calculated according to milk composition. The feed efficiency of lactation was calculated by FCM/DMI.
Rumen fluid collection and analysis
On days 21 and 7 before calving and 1 d, 14 d, 28 d, 42 d, 56 d, and 70 d after calving before morning feeding, 50 mL rumen fluid was extracted by oral intubation, filtered by four layers of gauze, and its pH value was determined immediately (SEVEN2Go portable pH meter, METTLER TOLEDO, Switzerland). The filtered rumen fluid was centrifuged at 5400 g/min for 10 min, 1 mL of supernatant was taken, and 0.2 mL 25% metaphosphoric acid solution containing standard internal 2 ethylbutanoic acid (2-EB) was added and mixed. Gas chromatography (6890N; Agilent Technologies, Avondale, PA, USA) and capillary column (HP-Innowax 19091N-213, Agilent) for determination of acetate, propionate, iso-butyrate, butyrate, iso-valerate and valerate acid in rumen fluid. Ammonia nitrogen (NH3-N) content was determined by the phenol-sodium hypochlorite colorimetric method (40)
Blood sampling and analysis
Blood samples were taken from the tail vein of six randomly selected dairy cows 21 d and 7 d before calving and 1 d, 28 d, 56 d, and 70 d after calving. Before morning feeding, 10 mL of blood was collected from blood vessels containing anticoagulant EDTA. After centrifugation at 300 g for 15 min, upper plasma was collected and stored at -20 degrees until analysis. Insulin (INS) was determined by radioimmunoassay (BFM-96, multi-tube radioimmunocounter, Zhongcheng Electromechanical Technology). Plasma TG was determined by an automatic biochemical analyzer (CLS880, Jiangsu Zecheng Biotechnology). NEFA, β -hydroxybutyric acid (BHBA), immunoglobulin G, A, and M (IgG, IgA, IgM), interleukin (IL-2, IL-6, IL-8, IL-10), tumor necrosis factor (TNF-α), myeloperoxidase (MPO), and serum amyloid A (SAA) were detected using the ELISA method (fully automated ELISA machine, THERMO Multiskan Ascent) (41, 42).
The bile acids in the blood samples were extracted and purified by liquid chromatography-tandem mass spectrometry with internal isotope standard and then separated by BEHC18 ultra-high liquid chromatographic column. Gradient elution was performed with 0.1% formic acid-water and 0.1% formic acid-acetonitrile as mobile phases, and mass spectrometry was performed with negative ion mode. At the same time, a variety of isotope-labeled bile acids were used as an internal standard to correct errors in sample pretreatment and mass spectrometry analysis (43).
Phagocytic ability of neutrophils
The tail vein blood of five dairy cows in the two treatments was collected using an EDTA tube 14 d, 28 d, 42 d, 56 d, and 70 d after calving. Neutrophils were isolated from the EDTA anticoagulated tube within 3 hours using the bovine peripheral blood neutrophil isolation kit (P9400, Solarbio, China). The isolated neutrophils were then added to 500 μL cell culture medium (RPMI-1640). After mixing well, viable cell concentrations were recorded with a cell counter (TC20TM, Bio-Red, America). Approximately 1×106 neutrophils were absorbed according to the concentration, and the equivalent fluorescent microspheres (F8821, ThermoFisher, China) were added, mixed, and cultivated for 30 min at 37°C. Then, 200 μL of paraformaldehyde was added (blown and mixed) fixed for 30 min, and centrifuged at 1200 g for 8 min. The supernatant was discarded, and 350 μL of FACS (1 μL fetal bovine serum + 49 μL phosphate buffer saline) was added and transferred to a flow cytometry tube after passing through a 70-μm pore size cell filter, the flow cytometry was then used to analyze the samples (early lactation, BD FACS Calibur, BD Company, America) (peak lactation, BD FACS Verse, BD Company, America). Data were analyzed with FlowJo software and presented with histograms, using the fluorescent channel, PE as the abscissa. The parameter PE provided an accurate measurement of the brightness of the stained cells. Subsequent statistical analysis was performed according to the percentage of neutrophils that phagocytosed the fluorescent microspheres (44, 45).
Statistical analysis
SAS Studio (SAS institute, Carry, NC, USA) software was used for statistical analysis. Before analysis, SAS was used to test the normal distribution of the data, and all the data were in line with normal distribution except SCC. The SCC results were then converted into SCC scores by log. Due to the great physiological changes of cows during the transition period, the experiment was divided into three stages for separate analysis: pre-calving (21 d before calving to calving), early lactation (1 d to 28 d after calving), and peak lactation (29 d to 70 d after calving). One-way ANOVA was used to analyze the nutrient digestibility of feed in the peak lactation stage of dairy cows. All other data were analyzed using the MIXED model of SAS, as follows:
Where Yijk is the dependent variable, μ is the overall mean, αi is the treatment effect (i=1, 2), βj is the effect of sampling time for the pre-calving cows, the early lactation cows, or the peak lactation cows, (αβ)ij is the interaction effect of treatment and sampling time, and eijk is the residual error.
By status, “week” was treated as a repeated measure and “cow” as the subject of the repeated statement. Significance was declared at P ≤ 0.05, and tendencies were reported if 0.05< P≤ 0.10. If the P-value of an interaction term was ≤ 0.05, it was retained; otherwise, interaction terms were removed from the model.
Results
Animal performance
Dietary supplements of MCFAs significantly reduced the DMI in the pre-calving cows (P = 0.05). And in early lactation, milk yield in MCFA treatment was significantly lower than that in CON (P = 0.02), and ECM also had a decreasing trend (P = 0.06) (Table 2).
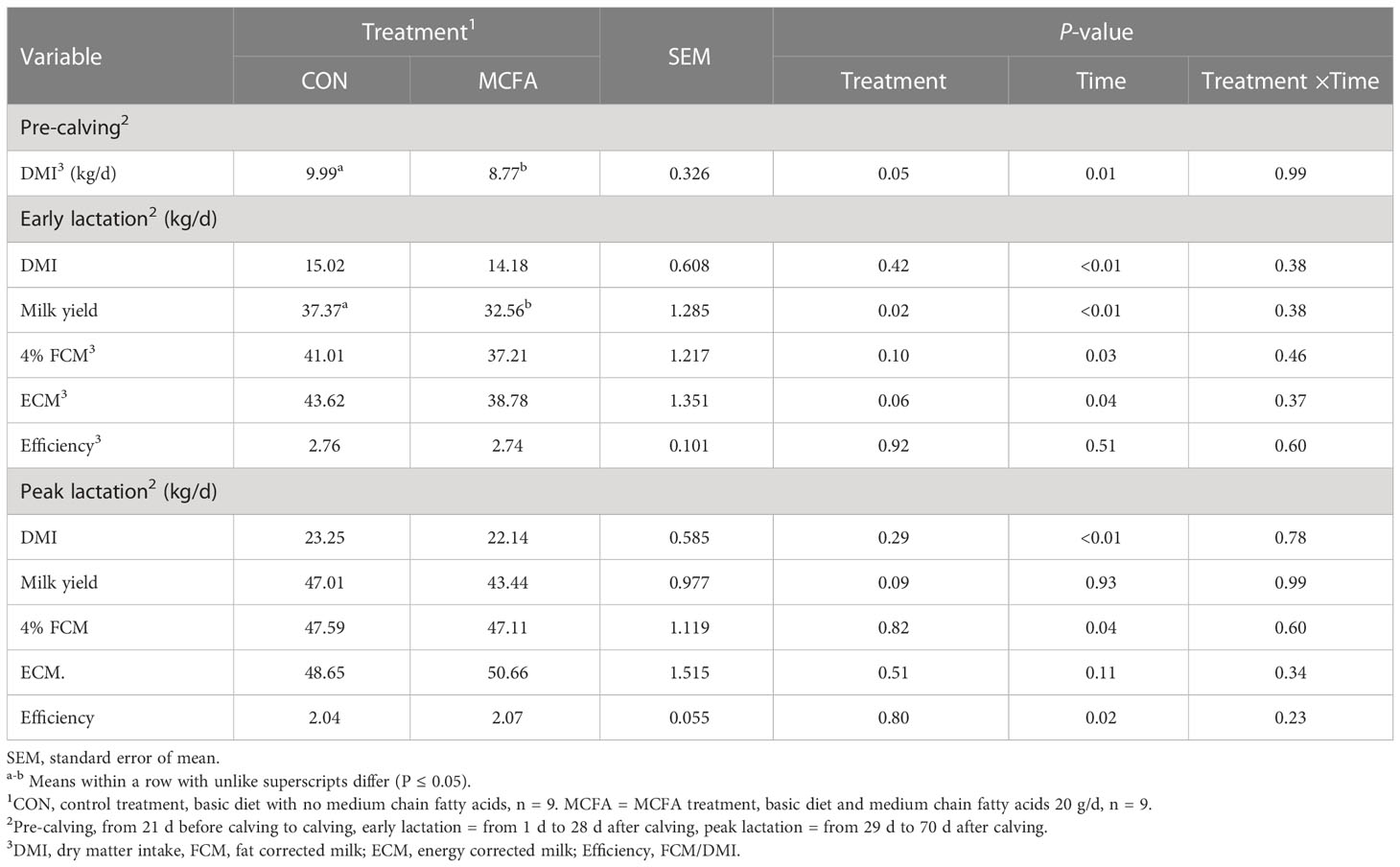
Table 2 Effects of feeding medium chain fatty acid on the performance of pre-calving, early lactation, and peak lactation cows2.
Milk composition analysis showed that milk fat has an increasing trend in early lactation (P = 0.09), while milk protein yield and lactose yield decreased significantly (P< 0.01, = 0.03, respectively) (Table 3). In peak lactation, milk lactose and milk fat percentage saw an interaction of time and treatment (P = 0.01, 0.07, respectively). MCFA supplementation significantly increased lactose percentage 42 d (P = 0.04) but significantly inhibited it 56 d after calving (P = 0.03). Milk fat percentage showed an increasing trend 56 d after calving (P = 0.09) (Table 3). The addition of MCFAs did not significantly influence the digestibility of each nutrient (Table 4).
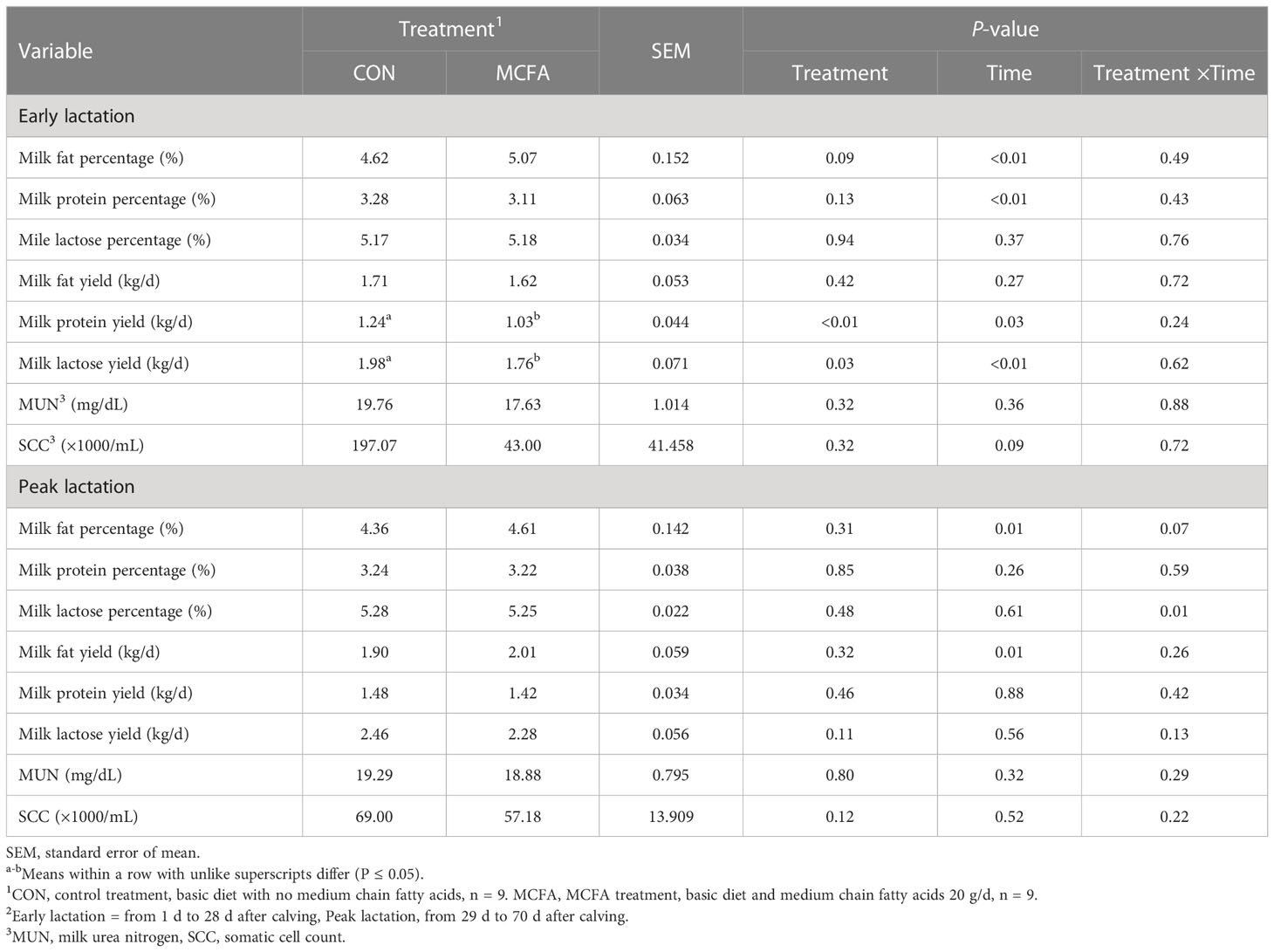
Table 3 Effects of feeding medium-chain fatty acid on milk composition of early lactation and peak lactation cows2.
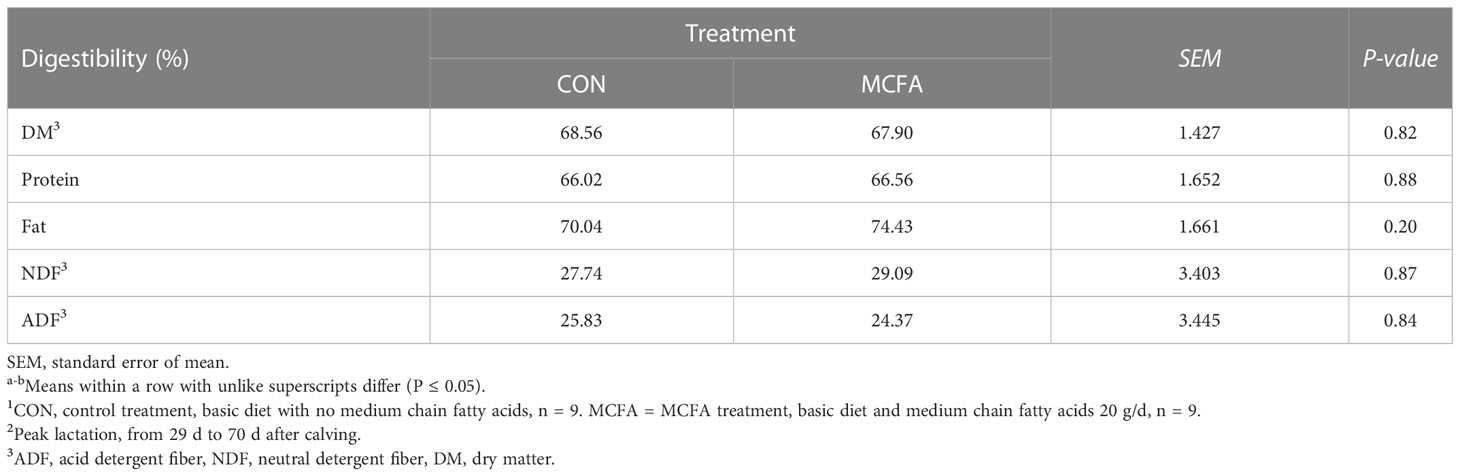
Table 4 Effects of feeding medium-chain fatty acid on nutrient digestibility of the peak lactation cows.
Inflammatory marker, immune globulin, and phagocytic ability in blood
In the pre-calving period, there was a significant time × treatment interaction for γ-IFN (P = 0.04). However, there was no difference in γ-IFN at each time point before calving (Table 5). In early lactation, compared with CON, the concentrations of MPO and SAA in the serum of cows undergoing MCFA treatment were significantly decreased (all P< 0.05, Table 5). There was also an interaction trend of IL-6 (P = 0.06). Compared with the CON, the concentration of IL-6 in the blood of cows under MCFA treatment was significantly increased 1 d after calving (P = 0.04, Table 5). In peak lactation, TNF-α saw a significant interaction between time and treatment (P = 0.01) and decreased significantly 56 d after calving (P = 0.02) (Table 5).
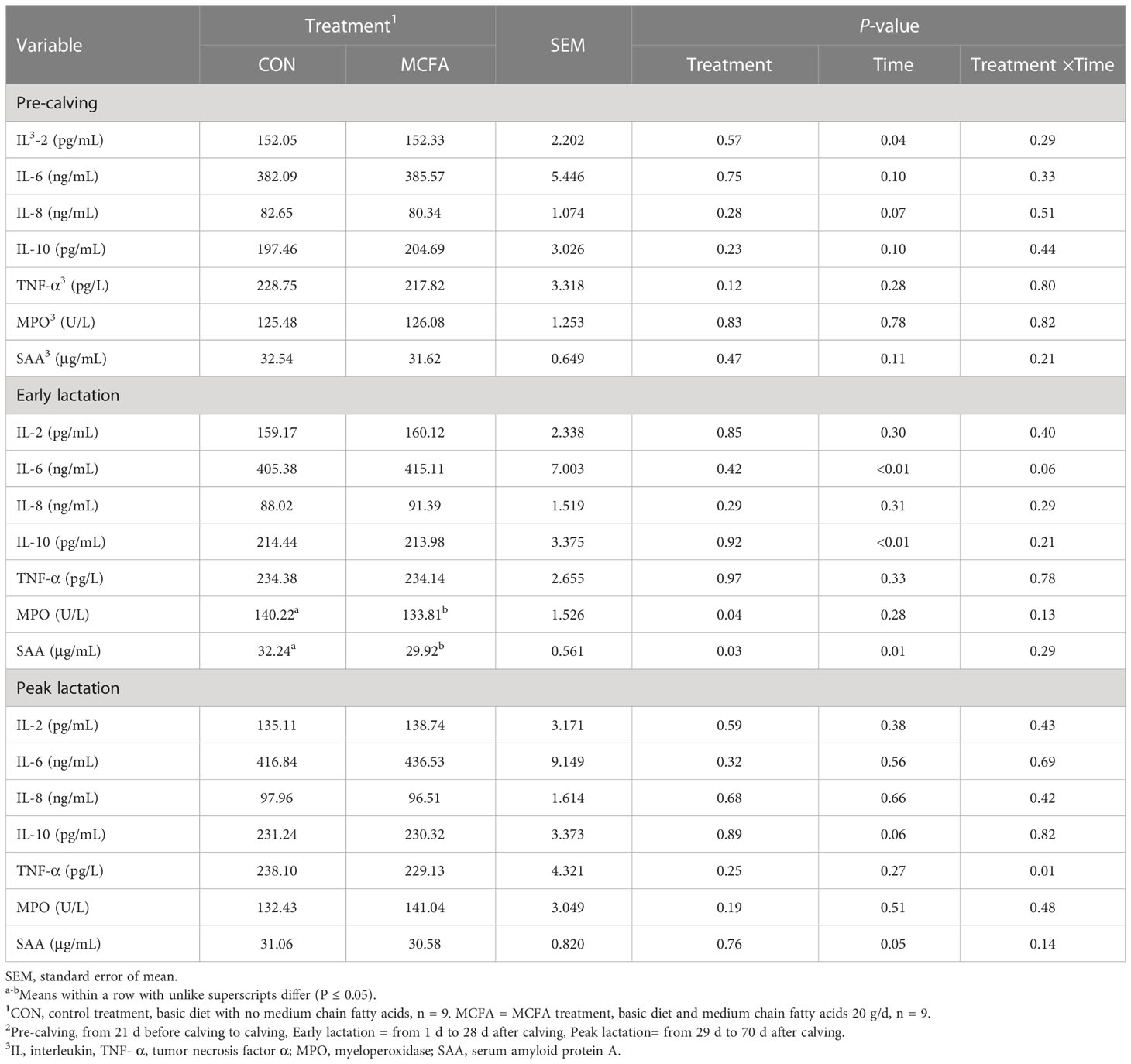
Table 5 Effects of feeding medium chain fatty acid on blood neutrophils phagocytosis of early lactation cows and peak lactation cows2.
There was an interaction trend for IgM (P = 0.09) in the pre-calving stage. IgM was significantly decreased 21 d before calving compared with the CON treatment (P = 0.02, Table 6). In early lactation, there was a significant interaction between time and treatment of IgM and IgA (P= 0.03,0.03, respectively). IgA concentration was also significantly decreased 28 d after calving (P = 0.01, Table 6), but IgM concentration was significantly increased 14 d after calving (P = 0.02, Table 6).
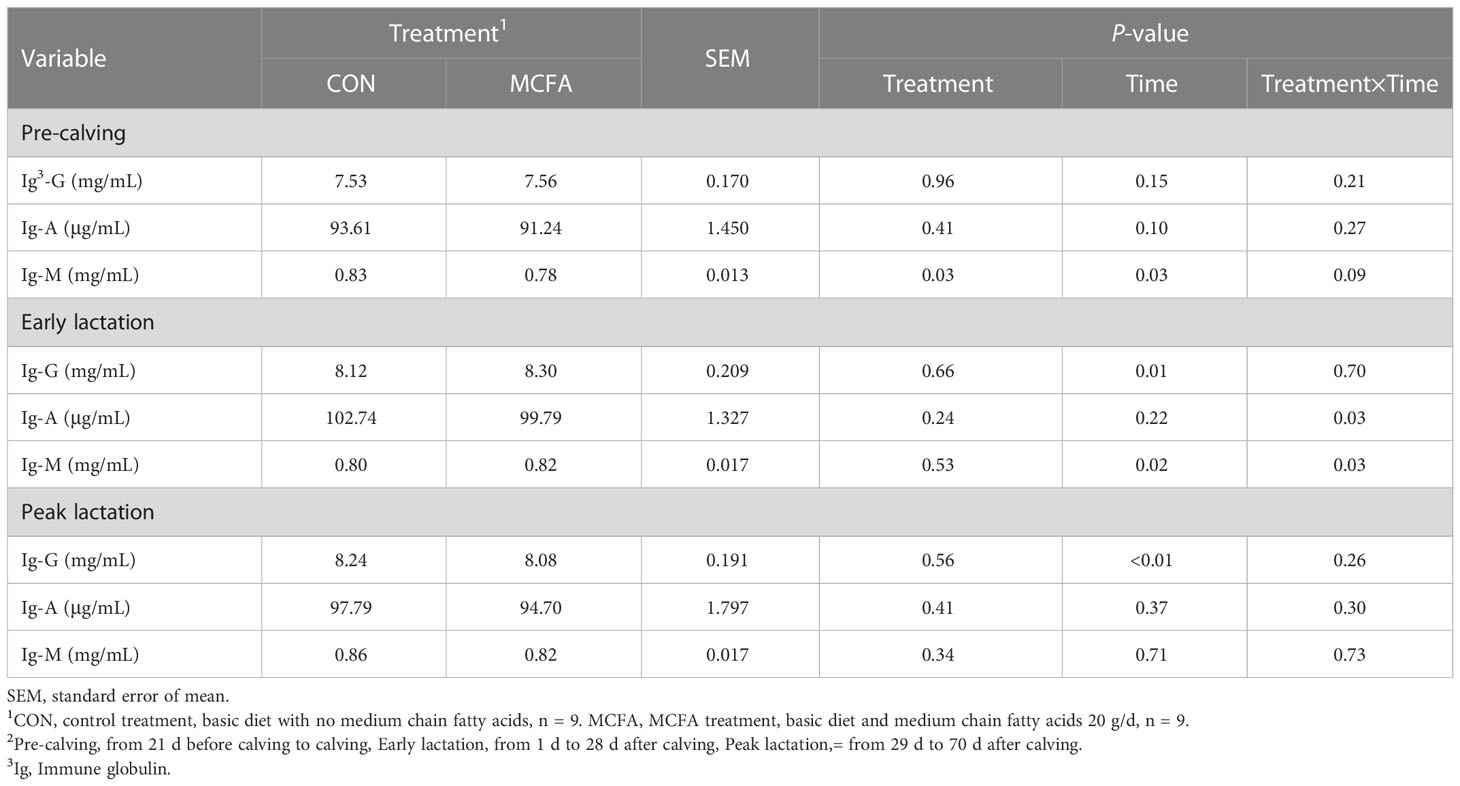
Table 6 Effects of feeding medium chain fatty acid on blood immune globulin of the pre-calving, early lactation, and peak lactation cows2.
Furthermore, there was no significant difference in neutrophils phagocytosis during early lactation and peak lactation (Figures 1A, B).
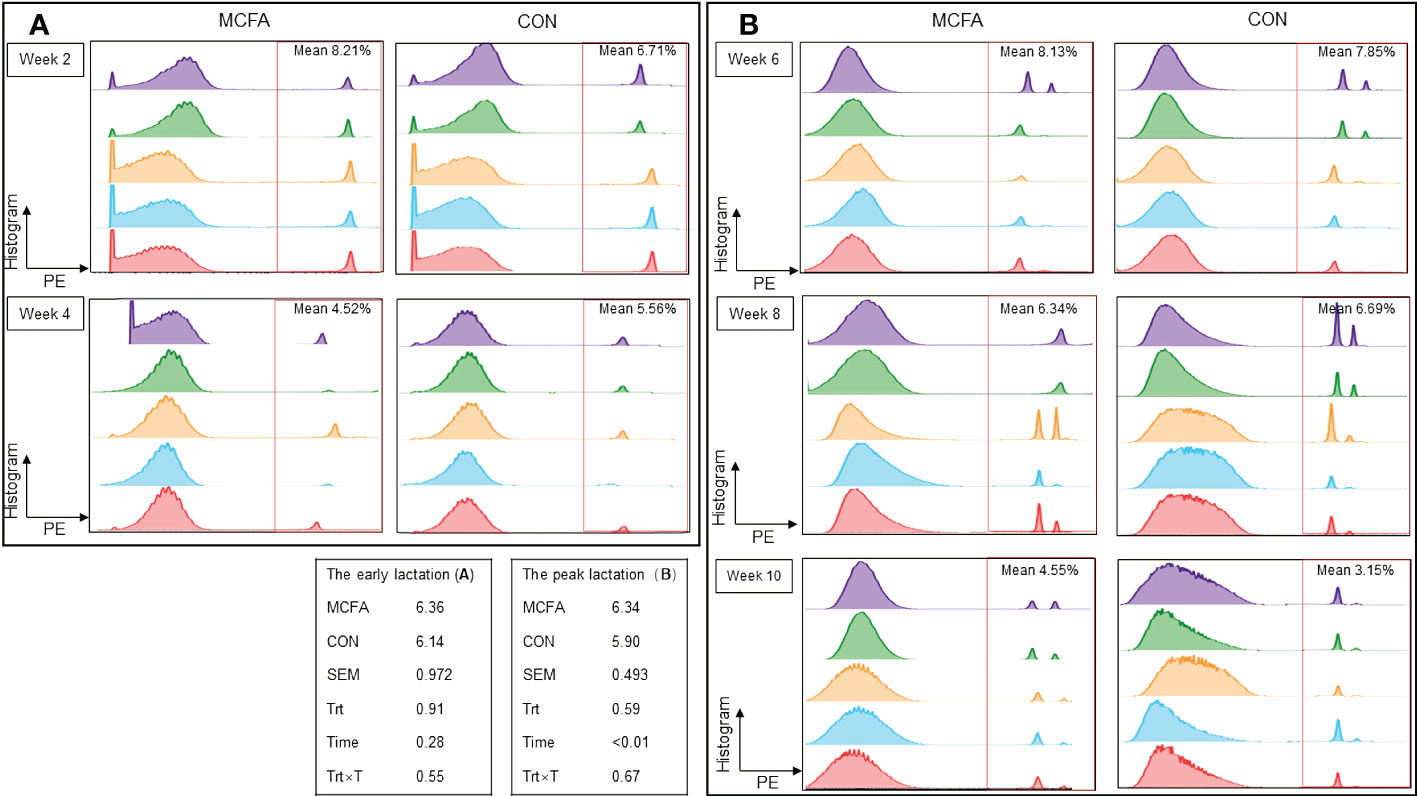
Figure 1 Effects of feeding medium chain fatty acid on blood neutrophils phagocytosis of the early lactation cows and the peak lactation cows. (A) The blood neutrophils phagocytosis of the early lactation dairy cows; (B) the blood neutrophils phagocytosis of the peak lactation dairy cows. CON, control treatment, n = 9; MCFA: MCFA treatment (basic diet and medium chain fatty acids 20 g/d), n = 9. SEM, standard error of mean. Trt, treatment effect; Time, the calving time effect; Trt × T, the interaction effect between treatment effect and time effect. The early lactation: from 1d to 28d after calving; the peak lactation: from 29 d to 70 d after calving.
Serum energy, insulin, and bile acid metabolism
The results of related metabolites and hormones in blood during the pre-calving period show that INS concentration was significantly reduced (P = 0.04; Table 7). TG showed a trend of interaction (P = 0.08) during early lactation, which had a decreasing trend compared with CON 28 d after calving (P = 0.07, Table 7).

Table 7 Effects of feeding medium-chain fatty acid on blood metabolites and hormones of pre-calving, early lactation, and peak lactation cows2.
The interaction between treatment and time-affected lithocholic acid (LCA), hyodeoxycholic acid (HDCA), hyocholic acid (HCA), taurocholic acid (TCA), chenodeoxycholic acid (CDCA), and ursodeoxycholic acid (UDCA) (P< 0.01,< 0.01,< 0.01, = 0.03, = 0.03, = 0.06, respectively). Specifically, the addition of MCFAs downregulated the serum concentrations of LCA, HDCA, HCA, and UDCA in MCFA treatment cows 1 d post-calving (P = 0.02,< 0.01,< 0.01, = 0.09, respectively, Table 8), as well as the serum concentrations of HCA and TCA in cows 14 d post-calving (P< 0.01, = 0.01, respectively, Table 8). However, CDCA concentration increased significantly 14 d after calving (P = 0.02, Table 8). LCA also had an upward trend (P = 0.08, Table 8).
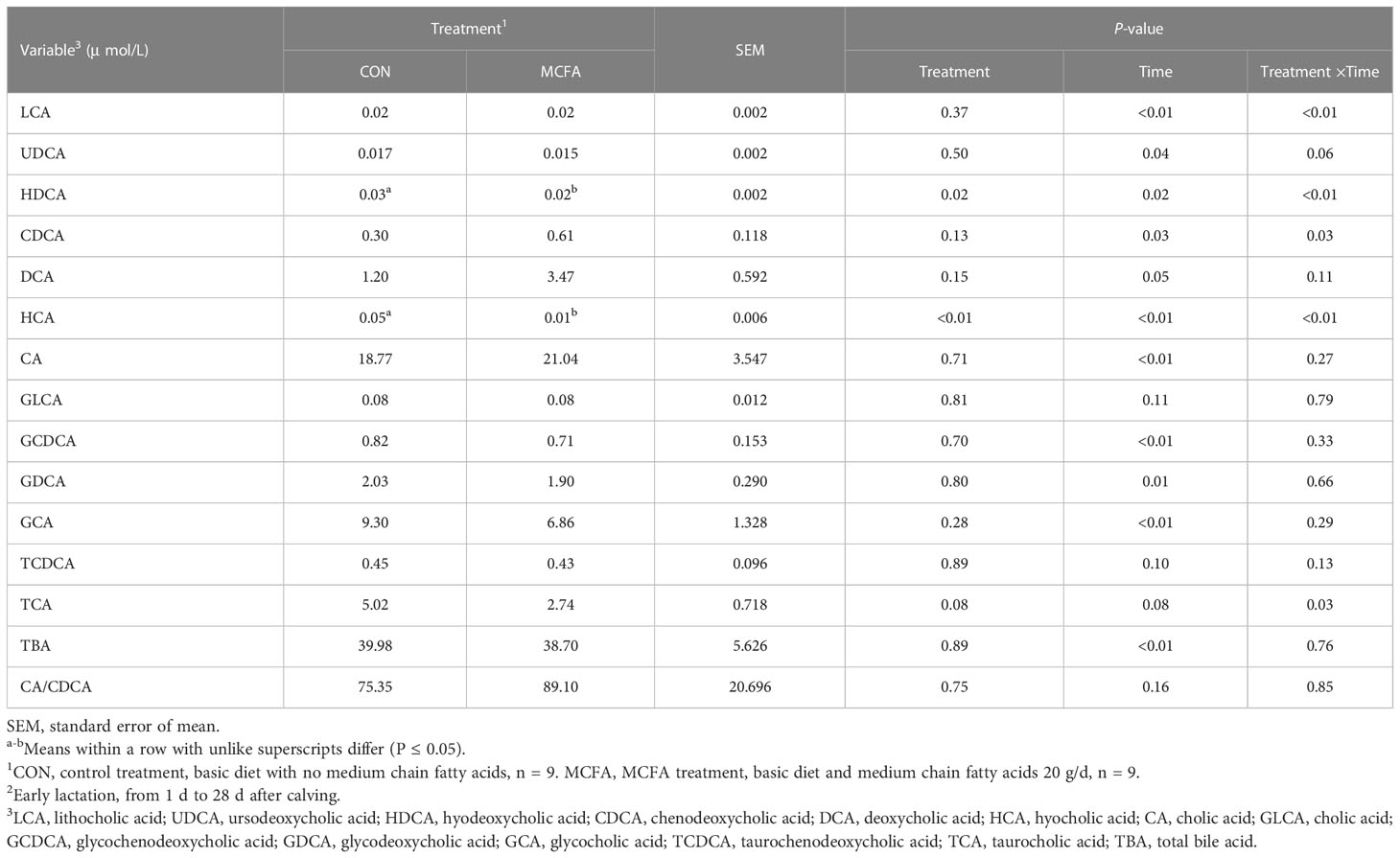
Table 8 Effects of feeding medium-chain fatty acid on blood bile acid metabolism of early lactation cows2.
Rumen fermentation
After analysis of rumen fermentation parameters, the concentrations of iso-butyrate and isovalerate in the rumen fluid of dairy cows during the pre-calving period were significantly decreased (P< 0.01, 0.01, respectively, Table 9), but the total volatile acid content was not affected. Besides, the acetate/propionate had a decreasing trend in early lactation (P = 0.08, Table 9),
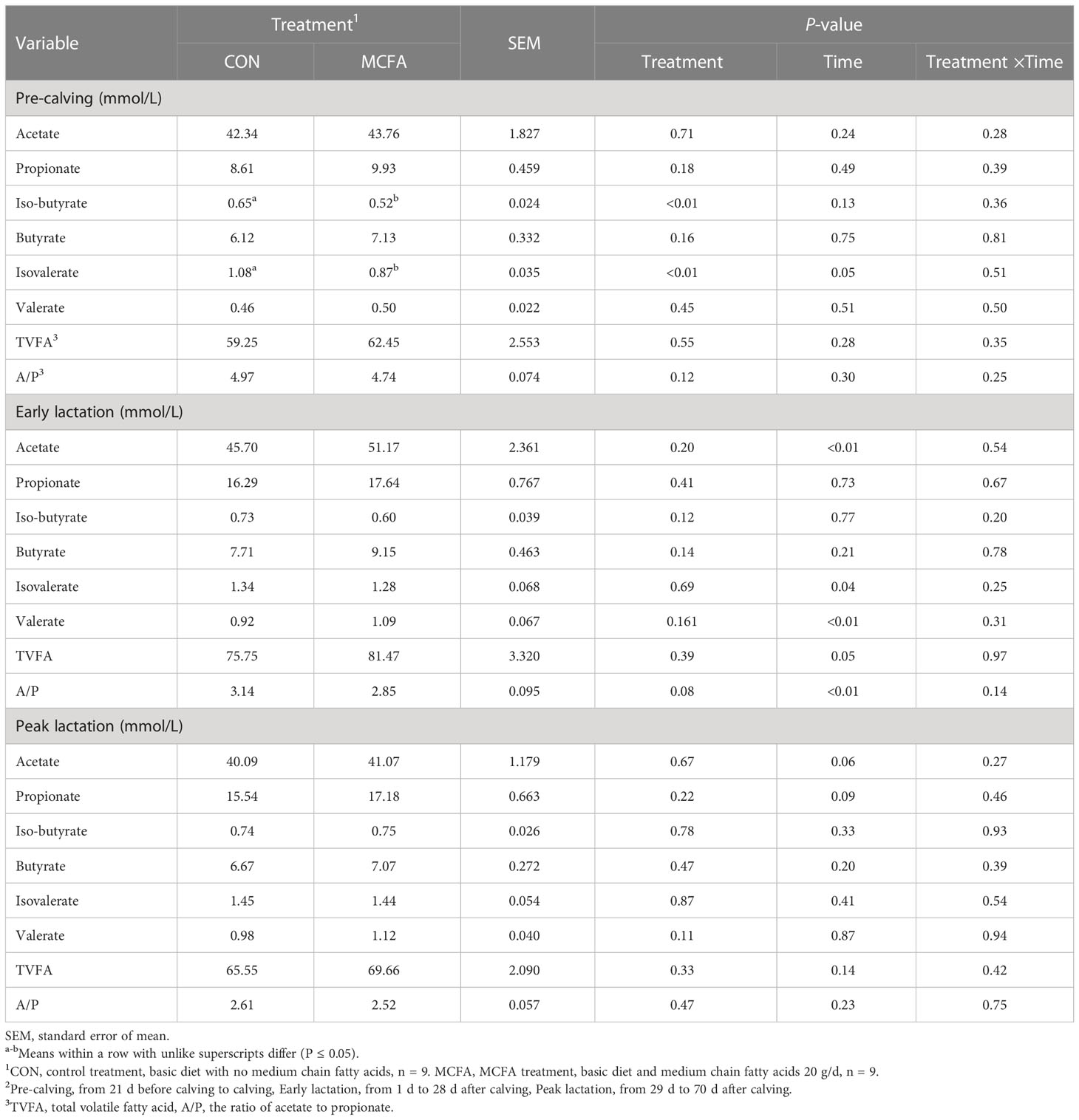
Table 9 Effects of feeding medium-chain fatty acid on rumen fermentation of pre-calving, early lactation, and peak lactation cows2.
The NH3-N had an increasing trend in this period (P = 0.09). Furthermore, different treatments had significant interaction with NH3-N in rumen fluid in terms of time in early lactation (P = 0.02), which shows that the concentration of NH3-N in MCFA treatment was significantly downregulated 14 d after calving (P = 0.04) (Table 10). In addition, the pH decreased significantly during early lactation (P = 0.01, Table 10).
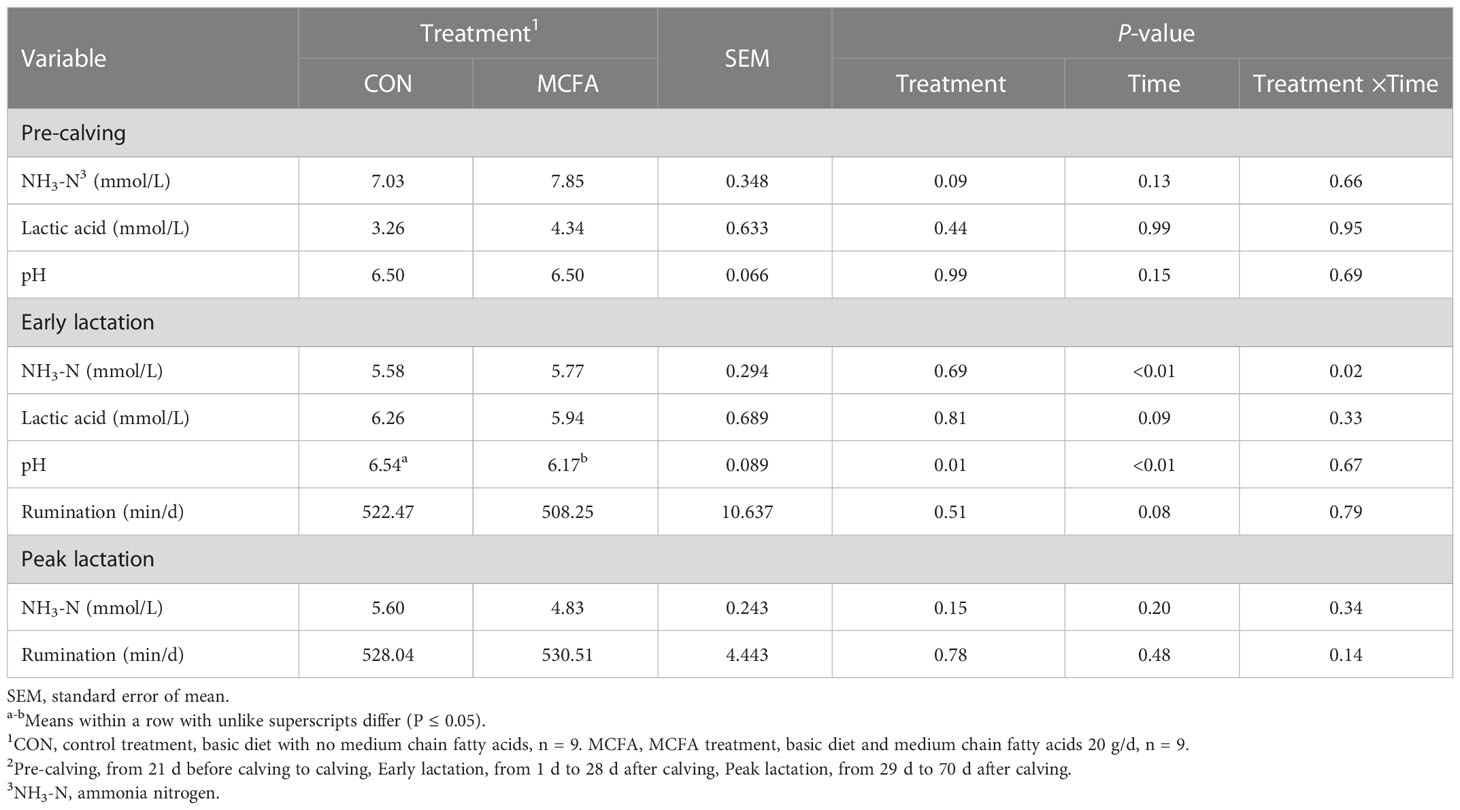
Table 10 Effects of feeding medium-chain fatty acid on rumen fermentation and ruminant behavior of pre-calving, early lactation, and peak lactation cows2.
Discussion
The nutritional metabolism and immune function of dairy cows are interdependent (46). During the transition period, decreased DMI cannot meet cows’ energy needs, leading to the occurrence of NEB and stimulating the mobilization of body fat. Relevant metabolites such as NEFA and BHBA may also affect the function of immune cells, leading to immune dysfunction in dairy cows (47). Also, transition cows go through huge changes in physiological status and various stress factors before and after calving, resulting in the occurrence of excessive inflammatory reactions, which may further affect the metabolic status of animals (48).
In general, the occurrence of inflammatory response is often accompanied by an increase in the concentration of inflammatory cytokines and positive acute phase protein (APP) in the blood. SAA is a signature positive APP in inflammation, and its concentration in the blood is low under healthy conditions, but it increases significantly during the onset of the inflammation (49). Similarly, as an important marker of the inflammatory response, MPO is considered an important tool for diagnosing bacterial infections (50). Compared with CON, the significant decrease of SAA and MPO in dairy cows under MCFA treatment during early lactation may indicate less inflammation in the body. In addition, MCFAs can mediate inflammatory responses by activating GPR84 and TLR-2 (51), promoting proinflammatory cytokine IL-6 production of the immune cell (52), and facilitating the rapid initiation of immune responses to clear pathogens, which may play an important role in alleviating the high stress and infection risk faced by cows during calving (53). Therefore, MCFAs may improve immune cell function and rapidly clear invading pathogens, prevent the occurrence of an excessive inflammatory response, and reduce the incidence of infectious diseases.
The liver function of transition cows often experiences a dramatic change as well. Liver function impairment is considered one of the important reasons for NEB and metabolic disorders in dairy cows. Bile acids are synthesized in the liver and, through interaction with farnesoid X receptor (FXR), regulate digestion, fat metabolism (54), and intestinal inflammation (55). In general, liver reuptake of blood bile acids is reduced, resulting in higher concentrations of bile acids in the blood, which may indicate impaired liver function. Meanwhile, the increasing level of serum bile acid is considered the main characteristic of bile acid siltation. Relevant reports have pointed out that bile acid siltation in animals during pregnancy affects glucose homeostasis. Cholic acid (CA), glycocholic acid (GCA), and taurocholic acid (TCA) are the three bile acids with the highest levels in the blood of dairy cows (56). Our results show that the level of TCA decreased significantly 14 d postpartum and the levels of HDCA, HCA, and LCA decreased significantly at different time points in early lactation. Thus, MCFAs may promote bile acid metabolism and improve the liver function of dairy cows, which plays a vital role in alleviating NEB.
Previous studies have pointed out that MCFAs could improve insulin resistance (57). The increasing level of INS in the prepartum blood of dairy cows may represent reduced sensitivity to insulin receptors in various organs and tissues in the body, thereby reducing insulin sensitivity and triggering insulin resistance (58, 59). The occurrence of insulin resistance reflects that the cows face a certain degree of NEB, which further leads to increased mobilization of body tissues, making cows face greater metabolic pressure and increasing the risk of metabolic diseases. Compared with the cows in the CON group, the concentration of INS in the prepartum blood of cows under MCFA treatment was significantly lower, which may imply that MCFA supplementation can improve the prepartum insulin sensitivity of cows. In addition, the concentrations of BHBA and NEFA in the blood are also numerically down-regulated, and prepartum NEFA and BHBA in the blood are correlated with the occurrence of postpartum metabolic and infectious diseases in dairy cows (60). Furthermore, increased TG concentration in the blood may lead to the deposition of TG in the liver, resulting in the occurrence of fatty liver (61, 62) and adversely affecting the function of the immune system (63). The decreasing trend of the blood TG in our experiment might be linked with the improvement of metabolism. Meanwhile, although the dose of MCFAs applied in this study was small, we cannot exclude the beneficial effect of MCFAs as a fast and efficient energy metabolite on the metabolism regulation of transition cows (64, 65).
The beneficial effects of MCFAs on the immunity and metabolism of dairy cows do not seem to be reflected in productive performance. It has been reported that MCFAs are rapidly absorbed into the liver for metabolism to generate energy and send signals to the brain through the vagus nerve to form satiety signals, thus affecting animal feeding (66). Our results show that MCFA application significantly decreased DMI prepartum, but the DMI in early and peak lactation cows was not affected dramatically. Meanwhile, the prepartum diet consists of higher roughage, which was degraded relatively slowly. So, dairy cows might not adapt to the supplement of MCFAs in the pre-calving period. With regard to the significant decrease in milk production in early lactation, we believe that MCFA supplementation may change the energy allocation of the animal body, and the reduced milk production will be used to reduce the metabolic stress and improve the immune function of cows, thus enhancing the health of cows in the transition period (31). This discrepancy between health and performance needs to be demonstrated in subsequent studies. Furthermore, milk fat percentage increased in both early and peak lactation periods. This is because MCFAs could improve the digestibility of whole digestive tract fat in dairy cows (26).
Although the DMI decreased in the pre-calving period, we observed that the TVFA in rumen fluid saw a numerical increase under MCFA treatment. In other words, MCFA supplementation may improve the rumen function of transition dairy cows, and this effect is more likely based on the beneficial regulation of rumen microbiota and intestinal health. Relevant studies have shown that MCFAs can inhibit intestinal pathogenic microorganisms, enhance intestinal mucosal barrier function, and mediate intestinal immunity (67, 68). IgA plays an important role in defending the enteric pathogenic microorganism passage through the intestinal mucosa, and the decrease in blood Ig A under the MCFA treatment may suggest that the intestinal mucosa is less affected by pathogens (69). The intestinal tract is the largest immune system and plays an important role in resisting the infection of pathogenic microorganisms from feed. MCFAs may improve intestinal health and the microorganism community, which can promote rumen fermentation and prevent systemic inflammation.
Conclusion
MCFA supplementation can improve the immune function of transition dairy cows to some extent. MCFAs can regulate the level of SAA, MPO, IL-6, TNF-α, and other related immune markers, which will be of benefit in preventing the occurrence of inflammatory diseases. Furthermore, MCFAs might improve the metabolic status of dairy cows in the transition period by modifying liver function and INS sensitivity.
Data availability statement
The original contributions presented in the study are included in the article/supplementary material. Further inquiries can be directed to the corresponding author.
Ethics statement
The animal study was reviewed and approved by committee of animal welfare and animal experimental ethical inspection of China Agricultural University. Written informed consent was obtained from the owners for the participation of their animals in this study.
Author contributions
Conceptualization: WW; methodology: WW and ZC; software: ZW; validation:WW; formal analysis: ZW; investigation: ZW, QW, JY, DL, TX, YZ, ZY, and CG; resources: CL and XS; data curation: ZW; writing (original draft preparation): ZW; writing (review and editing): ZW and WW; visualization: ZW; supervision: WW; project administration: WW; and funding acquisition: WW. All authors have read and agreed to the published version of the manuscript.
Funding
The National Key R&D Program of China (No.2022YFD1301400).
Acknowledgments
The authors thank Zhongdi Animal Husbandry Technology Company for providing the experimental animals.
Conflict of interest
The authors declare that the research was conducted in the absence of any commercial or financial relationships that could be construed as a potential conflict of interest.
Publisher’s note
All claims expressed in this article are solely those of the authors and do not necessarily represent those of their affiliated organizations, or those of the publisher, the editors and the reviewers. Any product that may be evaluated in this article, or claim that may be made by its manufacturer, is not guaranteed or endorsed by the publisher.
References
1. Mills KE, Weary DM, von Keyserlingk MAG. Identifying barriers to successful dairy cow transition management. J Dairy Sci (2020) 103:1749–58. doi: 10.3168/jds.2018-16231
2. Sordillo LM. Nutritional strategies to optimize dairy cattle immunity. J Dairy Sci (2016) 99:4967–82. doi: 10.3168/jds.2015-10354
3. Ceciliani F, Lecchi C, Urh C, Sauerwein H. Proteomics and metabolomics characterizing the pathophysiology of adaptive reactions to the metabolic challenges during the transition from late pregnancy to early lactation in dairy cows. J Proteomics (2018) 178:92–106. doi: 10.1016/j.jprot.2017.10.010
4. Minuti A, Jahan N, Lopreiato V, Piccioli-Cappelli F, Bomba L, Capomaccio S, et al. Evaluation of circulating leukocyte transcriptome and its relationship with immune function and blood markers in dairy cows during the transition period. Funct Integr Genomics (2020) 20:293–305. doi: 10.1007/s10142-019-00720-0
5. Gao ST, Girma DD, Bionaz M, Ma L, Bu DP. Hepatic transcriptomic adaptation from prepartum to postpartum in dairy cows. J Dairy Sci (2021) 104:1053–72. doi: 10.3168/jds.2020-19101
6. Bertics SJ, Grummer RR, Cadorniga-Valino C, Stoddard EE. Effect of prepartum dry matter intake on liver triglyceride concentration and early lactation. J Dairy Sci (1992) 75:1914–22. doi: 10.3168/jds.S0022-0302(92)77951-X
7. Havekes CD, Duffield TF, Carpenter AJ, DeVries TJ. Effects of molasses-based liquid feed supplementation to a high-straw dry cow diet on feed intake, health, and performance of dairy cows across the transition period. J Dairy Sci (2020) 103:5070–89. doi: 10.3168/jds.2019-18085
8. White HM. ADSA foundation scholar award: Influencing hepatic metabolism: Can nutrient partitioning be modulated to optimize metabolic health in the transition dairy cow? J Dairy Sci (2020) 103:6741–50. doi: 10.3168/jds.2019-18119
9. Brzozowska A, Lukaszewicz M, Oprzadek J. Energy-protein supplementation and lactation affect fatty acid profile of liver and adipose tissue of dairy cows. Molecules (2018) 23:618. doi: 10.3390/molecules23030618
10. White H. The role of TCA cycle anaplerosis in ketosis and fatty liver in periparturient dairy cows. Anim (Basel) (2015) 5:793–802. doi: 10.3390/ani5030384
11. Spaans OK, Kuhn-Sherlock B, Hickey A, Crookenden MA, Heiser A, Burke CR, et al. Temporal profiles describing markers of inflammation and metabolism during the transition period of pasture-based, seasonal-calving dairy cows. J Dairy Sci (2022) 105:2669–98. doi: 10.3168/jds.2021-20883
12. Cruywagen CW, Taylor S, Beya MM, Calitz T. The effect of buffering dairy cow diets with limestone, calcareous marine algae, or sodium bicarbonate on ruminal pH profiles, production responses, and rumen fermentation. J Dairy Sci (2015) 98:5506–14. doi: 10.3168/jds.2014-8875
13. Cardoso FC, Kalscheur KF, Drackley JK. Symposium review: Nutrition strategies for improved health, production, and fertility during the transition period. J Dairy Sci (2020) 103:5684–93. doi: 10.3168/jds.2019-17271
14. Esposito G, Irons PC, Webb EC, Chapwanya A. Interactions between negative energy balance, metabolic diseases, uterine health and immune response in transition dairy cows. Anim Reprod Sci (2014) 144:60–71. doi: 10.1016/j.anireprosci.2013.11.007
15. Mezzetti M, Cattaneo L, Passamonti MM, Lopreiato V, Minuti A, Trevisi E. The transition period updated: A review of the new insights into the adaptation of dairy cows to the new lactation. Dairy (2021) 2:617–36. doi: 10.3390/dairy2040048
16. Wallace TC. Health effects of coconut oil-a narrative review of current evidence. J Am Coll Nutr (2019) 38:97–107. doi: 10.1080/07315724.2018.1497562
17. Guillot E, Vaugelade P, Lemarchali P, Re Rat A. Intestinal absorption and liver uptake of medium-chain fatty acids in non-anaesthetized pigs. Br J Nutr (1993) 69:431–42. doi: 10.1079/BJN19930045
18. Schönfeld P, Wojtczak L. Short- and medium-chain fatty acids in energy metabolism: the cellular perspective. J Lipid Res (2016) 57:943–54. doi: 10.1194/jlr.R067629
19. Geelen MJ. Medium-chain fatty acids as short-term regulators of hepatic lipogenesis. Biochem J (1994) 302(Pt 1):141–46. doi: 10.1042/bj3020141
20. Xu Q, Xue C, Zhang Y. Medium-chain fatty acids enhanced the excretion of fecal cholesterol and cholic acid in C57BL/6J mice fed a cholesterol-rich diet. Biosci Biotechnol Biochem (2013) 77:1390–1396. doi: 10.1271/bbb.120999
21. Boemeke L, Marcadenti A, Busnello FM, Gottschall CBA. Effects of coconut oil on human health. Open J Endocrine Metab Dis (2015) 05:84–7. doi: 10.4236/ojemd.2015.57011
22. Papada E, Kaliora AC, Gioxari A, Papalois A, Forbes A. Anti-inflammatory effect of elemental diets with different fat composition in experimental colitis. Br J Nutr (2014) 111:1213–20. doi: 10.1017/S0007114513003632
23. Thomas LL, Woodworth JC, Tokach MD, Dritz SS, DeRouchey JM, Goodband RD, et al. Evaluation of different blends of medium-chain fatty acids, lactic acid, and monolaurin on nursery pig growth performance12. Transl Anim Sci (2020) 4:548–57. doi: 10.1093/tas/txaa024
24. Zhang JY, Baek DH, Kim IH. Effect of dietary supplemental medium chain fatty acids instead of antibiotics on the growth performance, digestibility and blood profiles in growing pigs. J Anim Physiol Anim Nutr (Berl) (2019) 103:1946–51. doi: 10.1111/jpn.13175
25. Mohana Devi S, Kim IH. Effect of medium chain fatty acids (MCFA) and probiotic (Enterococcus faecium) supplementation on the growth performance, digestibility and blood profiles in weanling pigs. Vet Med (2014) 59:527–35. doi: 10.17221/7817-VETMED
26. van Zijderveld SM, Dijkstra J, Perdok HB, Newbold JR, Gerrits WJJ. Dietary inclusion of diallyl disulfide, yucca powder, calcium fumarate, an extruded linseed product, or medium-chain fatty acids does not affect methane production in lactating dairy cows. J Dairy Sci (2011) 94:3094–104. doi: 10.3168/jds.2010-4042
27. Vyas D, Teter BB, Erdman RA. Milk fat responses to dietary supplementation of short- and medium-chain fatty acids in lactating dairy cows. J Dairy Sci (2012) 95:5194–202. doi: 10.3168/jds.2011-5277
28. Sun Y, Bu DP, Wang JQ, Cui H, Zhao XW, Xu XY, et al. Supplementing different ratios of short- and medium-chain fatty acids to long-chain fatty acids in dairy cows: Changes of milk fat production and milk fatty acids composition. J Dairy Sci (2013) 96:2366–73. doi: 10.3168/jds.2012-5356
29. Kongmun P, Wanapat M, Pakdee P, Navanukraw C, Yu Z. Manipulation of rumen fermentation and ecology of swamp buffalo by coconut oil and garlic powder supplementation. Livest Sci (2011) 135:84–92. doi: 10.1016/j.livsci.2010.06.131
30. Reveneau C, Karnati SKR, Oelker ER, Firkins JL. Interaction of unsaturated fat or coconut oil with monensin in lactating dairy cows fed 12 times daily. i. protozoal abundance, nutrient digestibility, and microbial protein flow to the omasum. J Dairy Sci (2012) 95:2046–60. doi: 10.3168/jds.2011-4887
31. Deen A, Visvanathan R, Wickramarachchi D. Chemical composition and health benefits of coconut oil: an overview. J Sci Food Agric (2021) 6:2182–93. doi: 10.1002/jsfa.1087x
32. Alva-Murillo N, Ochoa-Zarzosa A, López-Meza JE. Effects of sodium octanoate on innate immune response of mammary epithelial cells duringStaphylococcus aureus internalization. BioMed Res Int (2013) 2013:1–08. doi: 10.1155/2013/927643
33. Piepers S, De Vliegher S. Oral supplementation of medium-chain fatty acids during the dry period supports the neutrophil viability of peripartum dairy cows. J Dairy Res (2013) 80:309–18. doi: 10.1017/S0022029913000228
34. Nair MK, Joy J, Vasudevan P, Hinckley L, Hoagland TA, Venkitanarayanan KS. Antibacterial effect of caprylic acid and monocaprylin on major bacterial mastitis pathogens. J Dairy Sci (2005) 88:3488–95. doi: 10.3168/jds.S0022-0302(05)73033-2
35. Alva-Murillo N, Ochoa-Zarzosa A, López-Meza JE. Sodium octanoate modulates the innate immune response of bovine mammary epithelial cells through the TLR2/P38/JNK/ERK1/2 pathway: Implications during staphylococcus aureus internalization. Front Cell Infect Microbiol (2017) 7:78. doi: 10.3389/fcimb.2017.00078
36. Jackman JA, Boyd RD, Elrod CC. Medium-chain fatty acids and monoglycerides as feed additives for pig production: towards gut health improvement and feed pathogen mitigation. J Anim Sci Biotechnol (2020) 11:1–15. doi: 10.1186/s40104-020-00446-1
37. Wang C, Han L, Zhang GW, Du HS, Wu ZZ, Liu Q, et al. Effects of copper sulphate and coated copper sulphate addition on lactation performance, nutrient digestibility, ruminal fermentation and blood metabolites in dairy cows. Br J Nutr (2021) 125:251–59. doi: 10.1017/S0007114520002986
38. Van Soest PJ, Robertson JB, Lewis BA. Symposium: carbohydrate methodology, metabolism, and nutritional implications in dairy cattle. J Dairy Sci (1991) 10:3583–97.
39. Zhong RZ, Li JG, Gao YX, Tan ZL, Ren GP. Effects of substitution of different levels of steam-flaked corn for finely ground corn on lactation and digestion in early lactation dairy cows. J Dairy Sci (2008) 91:3931–37. doi: 10.3168/jds.2007-0957
40. Hao Y, Guo C, Gong Y, Sun X, Wang W, Wang Y, et al. Rumen fermentation, digestive enzyme activity, and bacteria composition between pre-weaning and post-weaning dairy calves. Anim (Basel) (2021) 11:2527. doi: 10.3390/ani11092527
41. Cardoso FF, Donkin SS, Pereira MN, Pereira RAN, Peconick AP, Santos JP, et al. Effect of protein level and methionine supplementation on dairy cows during the transition period. J Dairy Sci (2021) 104:5467–78. doi: 10.3168/jds.2020-19181
42. Zachut M, Honig H, Striem S, Zick Y, Boura-Halfon S, Moallem U. Periparturient dairy cows do not exhibit hepatic insulin resistance, yet adipose-specific insulin resistance occurs in cows prone to high weight loss. J Dairy Sci (2013) 96:5656–69. doi: 10.3168/jds.2012-6142
43. Gao J, Xu B, Zhang X, Cui Y, Deng L, Shi Z, et al. Association between serum bile acid profiles and gestational diabetes mellitus: A targeted metabolomics study. Clin Chim Acta (2016) 459:63–72. doi: 10.1016/j.cca.2016.05.026
44. Wu ZH, Yu Y, Alugongo GM, Xiao JX, Li JH, Li YX, et al. Short communication: Effects of an immunomodulatory feed additive on phagocytic capacity of neutrophils and relative gene expression in circulating white blood cells of transition Holstein cows. J Dairy Sci (2017) 100:7549–55. doi: 10.3168/jds.2016-12528
45. Couto SR, Morrison EI, Bogado PO, DeVries TJ, Duffield TF, LeBlanc SJ. The effect of prepartum negative dietary cation-anion difference and serum calcium concentration on blood neutrophil function in the transition period of healthy dairy cows. J Dairy Sci (2020) 103:6200–08. doi: 10.3168/jds.2019-18012
46. Sordillo LM, Raphael W. Significance of metabolic stress, lipid mobilization, and inflammation on transition cow disorders. Vet Clin North Am Food Anim Pract (2013) 29:267–78. doi: 10.1016/j.cvfa.2013.03.002
47. Mezzetti M, Bionaz M, Trevisi E. Interaction between inflammation and metabolism in periparturient dairy cows. J Anim Sci (2020) 98:S155–74. doi: 10.1093/jas/skaa134
48. Sun B, Cao Y, Cai C, Yu C, Li S, Yao J. Temporal dynamics of nutrient balance, plasma biochemical and immune traits, and liver function in transition dairy cows. J Integr Agric (2020) 19:820–37. doi: 10.1016/S2095-3119(20)63153-7
49. Ceciliani F, Ceron JJ, Eckersall PD, Sauerwein H. Acute phase proteins in ruminants. J Proteomics (2012) 75:4207–31. doi: 10.1016/j.jprot.2012.04.004
50. Depreester E, Meyer E, Demeyere K, Van Eetvelde M, Hostens M, Opsomer G. Flow cytometric assessment of myeloperoxidase in bovine blood neutrophils and monocytes. J Dairy Sci (2017) 100:7638–47. doi: 10.3168/jds.2016-12186
51. Huang Q, Feng D, Liu K, Wang P, Xiao H, Wang Y, et al. A medium-chain fatty acid receptor Gpr84 in zebrafish: Expression pattern and roles in immune regulation. Dev Comp Immunol (2014) 45:252–58. doi: 10.1016/j.dci.2014.03.017
52. Sam QH, Ling H, Yew WS, Tan Z, Ravikumar S, Chang MW, et al. The divergent immunomodulatory effects of short chain fatty acids and medium chain fatty acids. Int J Mol Sci (2021) 22:6453. doi: 10.3390/ijms22126453
53. Masmeijer C, Rogge T, van Leenen K, De Cremer L, Deprez P, Cox E, et al. Effects of glycerol-esters of saturated short- and medium chain fatty acids on immune, health and growth variables in veal calves. Prev Vet Med (2020) 178:104983. doi: 10.1016/j.prevetmed.2020.104983
54. Prawitt J, Caron S, Staels B. Bile acid metabolism and the pathogenesis of type 2 diabetes. Curr Diabetes Rep (2011) 11:160–66. doi: 10.1007/s11892-011-0187-x
55. Jia W, Xie G, Jia W. Bile acid–microbiota crosstalk in gastrointestinal inflammation and carcinogenesis. Nat Rev Gastroenterol Hepatol (2018) 15:111–28. doi: 10.1038/nrgastro.2017.119
56. Washizu T, Tomoda I, Kaneko J. Serum bile acid composition of the dog, cow, horse and human. J Vet Med Sci (1991) 1:81–6. doi: 10.1292/jvms.53.81
57. Roopashree PG, Shetty SS, Suchetha Kumari N. Effect of medium chain fatty acid in human health and disease. J Funct Foods (2021) 87:104724. doi: 10.1016/j.jff.2021.104724
58. De Koster J, Hostens M, Van Eetvelde M, Hermans K, Moerman S, Bogaert H, et al. Insulin response of the glucose and fatty acid metabolism in dry dairy cows across a range of body condition scores. J Dairy Sci (2015) 98:4580–92. doi: 10.3168/jds.2015-9341
59. Nagao K, Yanagita T. Medium-chain fatty acids: Functional lipids for the prevention and treatment of the metabolic syndrome. Pharmacol Res (2010) 61:208–12. doi: 10.1016/j.phrs.2009.11.007
60. Lin X, Li K, Ju L, Hao X, Jiang Y, Hou Q, et al. Effects of supplementation with <i<Saccharomyces cerevisiae</i< and its fermentation products on production performance and its mechanism in perinatal dairy cows. Adv Biosci Biotechnol (2021) 12:193–212. doi: 10.4236/abb.2021.127013
61. Rabelo E, Rezende RL, Bertics SJ, Grummer RR. Effects of pre- and postfresh transition diets varying in dietary energy density on metabolic status of periparturient dairy cows. J Dairy Sci (2005) 88:4375–83. doi: 10.3168/jds.S0022-0302(05)73124-6
62. McFadden JW. Review: Lipid biology in the periparturient dairy cow: contemporary perspectives. Animal (2020) 14:s165–75. doi: 10.1017/S1751731119003185
63. Drackley JK, Dann HM, Douglas N, Guretzky NAJ, Litherland NB, Underwood JP, et al. Physiological and pathological adaptations in dairy cows that may increase susceptibility to periparturient diseases and disorders. Ital J Anim Sci (2005) 4:323–44. doi: 10.4081/ijas.2005.323
64. Zentek J, Buchheit-Renko S, Ferrara F, Vahjen W, Van Kessel AG, Pieper R. Nutritional and physiological role of medium-chain triglycerides and medium-chain fatty acids in piglets. Anim Health Res Rev (2011) 12:83–93. doi: 10.1017/S1466252311000089
65. Aoyama T, Nosaka N, Kasai M. Research on the nutritional characteristics of medium-chain fatty acids. J Med Invest (2007) 54:385–88. doi: 10.2152/jmi.54.385
66. Allen MS, Bradford BJ, Oba M. BOARD-INVITED REVIEW: The hepatic oxidation theory of the control of feed intake and its application to ruminants1. J Anim Sci (2009) 87:3317. doi: 10.2527/jas.2009-1779
67. Jia M, Zhang Y, Gao Y, Ma X. Effects of medium chain fatty acids on intestinal health of monogastric animals. Curr Protein Pept Sci (2020) 21:777–84. doi: 10.2174/1389203721666191231145901
68. Lopez-Colom P, Castillejos L, Rodriguez-Sorrento A, Puyalto M, Mallo JJ, Martin-Orue SM. Efficacy of medium-chain fatty acid salts distilled from coconut oil against two enteric pathogen challenges in weanling piglets. J Anim Sci Biotechnol (2019) 10:89. doi: 10.1186/s40104-019-0393-y
69. De Keyser K, Dierick N, Kanto U, Hongsapak T, Buyens G, Kuterna L, et al. Medium-chain glycerides affect gut morphology, immune- and goblet cells in post-weaning piglets: In vitro fatty acid screening with escherichia coli and in vivo consolidation with LPS challenge. J Anim Physiol Anim Nutr (Berl) (2019) 103:221–30. doi: 10.1111/jpn.12998
Keywords: transition, dairy cows, medium chain fatty acids, immunity, metabolism
Citation: Wang Z, Wang Q, Tang C, Yuan J, Luo C, Li D, Xie T, Sun X, Zhang Y, Yang Z, Guo C, Cao Z, Li S and Wang W (2023) Medium chain fatty acid supplementation improves animal metabolic and immune status during the transition period: A study on dairy cattle. Front. Immunol. 14:1018867. doi: 10.3389/fimmu.2023.1018867
Received: 14 August 2022; Accepted: 13 January 2023;
Published: 27 January 2023.
Edited by:
Ziwei Zhang, Northeast Agricultural University, ChinaReviewed by:
Linshu Jiang, Beijing University of Agriculture, ChinaJuncai Chen, Southwest University, China
Michael Edward Van Amburgh, Cornell University, United States
Copyright © 2023 Wang, Wang, Tang, Yuan, Luo, Li, Xie, Sun, Zhang, Yang, Guo, Cao, Li and Wang. This is an open-access article distributed under the terms of the Creative Commons Attribution License (CC BY). The use, distribution or reproduction in other forums is permitted, provided the original author(s) and the copyright owner(s) are credited and that the original publication in this journal is cited, in accordance with accepted academic practice. No use, distribution or reproduction is permitted which does not comply with these terms.
*Correspondence: Wei Wang, wei.wang@cau.edu.cn