- 1School of Public Health, Shandong First Medical University and Shandong Academy of Medical Sciences, Taian, China
- 2Centre for Precision Health, School of Medical and Health Sciences, Edith Cowan University, Perth, WA, Australia
- 3Beijing Key Laboratory of Clinical Epidemiology, School of Public Health, Capital Medical University, Beijing, China
Misunderstanding temporal coincidence of adverse events during mass vaccination and invalid assessment of possible safety concerns have negative effects on immunization programs, leading to low immunization coverage. We conducted this systematic review and meta-analysis to identify the incidence rates of GBS that are temporally associated with viral vaccine administration but might not be attributable to the vaccines. By literature search in Embase and PubMed, we included 48 publications and 2,110,441,600 participants. The pooled incidence rate of GBS was 3.09 per million persons (95% confidence interval [CI]: 2.67 to 3.51) within six weeks of vaccination, equally 2.47 per 100,000 person-year (95%CI: 2.14 to 2.81). Subgroup analyses illustrated that the pooled rates were 2.77 per million persons (95%CI: 2.47 to 3.07) for individuals who received the influenza vaccine and 2.44 per million persons (95%CI: 0.97 to 3.91) for human papillomavirus (HPV) vaccines, respectively. Our findings evidence the GBS-associated safety of virus vaccines. We present a reference for the evaluation of post-vaccination GBS rates in mass immunization campaigns, including the SARS-CoV-2 vaccine.
Introduction
The coronavirus disease-2019 (COVID-19), induced by the severe acute respiratory syndrome coronavirus-2 (SARS-CoV-2), has been challenging all over the world since December, 2019 (1). As of December 29, 2021, the total number of confirmed cases is over 281 million worldwide, including more than five million deaths (2). SARS-CoV-2 infection is commonly characterized by fever, cough, shortness of breath, headache fatigue, pneumonia and congestion (3, 4). In severe cases, especially among individuals over 60 years old and those with underlying chronic comorbidities, the infection leads to acute respiratory distress syndrome (ARDS), renal failure, meningoencephalitis, cerebrovascular accidents, sepsis and even death (5). Compared with its predecessors (i.e., SARS-CoV and MERS-CoV), SARS-CoV-2 transmits much more efficiently from person to person (6).
Currently, mass vaccine immunization is believed essential to control the pandemic (7). The SARS-CoV-2 vaccine has been expedited through preclinical and clinical investigations (8). As of December 29, 2021, a total of 8,687,201,202 vaccine doses have been administered worldwide (2). Efforts have been made to promote mass vaccination programs against SARS-CoV-2. The unprecedented campaign of mass immunization will pose many challenges to the assessment of vaccine safety. Potential adverse events following immunization (AEFI), induced by vaccination of SARS-CoV-2, will foreseeably raise potential concerns under the pandemic. The public needs frequent reassurance of vaccine safety when adverse events occur in temporally coincident association with SARS-CoV-2 vaccination, even when the events are not caused by the vaccines. Awareness of possible adverse events is essential for the assessment of vaccine safety and may help to separate AEFI from events that are temporally associated with but might not be attributed to vaccination (9).
Viral vaccines were considered to be related to AEFIs including vomiting, diarrhea, nausea or abdominal pain, acute otitis media, vaccine-related paralytic poliomyelitis (VAPP), Guillain-Barré syndrome (GBS), anaphylactic shock, epilepsy and meningitis (10), among which GBS is considered one of the most severe conditions (11). GBS is featured by immune mediators damaging to peripheral nerves and associated with muscle weakness or paralysis (12). The initial symptoms of GBS are severe nerve pain in the neck, shoulder and waist, followed by acute progressive acute paralysis of limbs and subjective sensory disturbance (13). Reported incidence rates of GBS for all ages combined range from 0.2 to 3.0 per 100,000 person-years (14).
An 11- to 18-fold increase of incidence rate of GBS within three weeks after influenza vaccination and a 4- to 9-fold increase within six weeks have been released previously (12). Consideration about GBS that was in the wake of post-vaccination appeared for the first time in the influenza vaccine season from 1976 to 1977 (15). Mass human papillomavirus (HPV) immunization has also been suggested to be related to GBS (16). At present, HPV vaccines are recommended by World Health Organization (WHO) for girls between 9 and 13 years old (17, 18).
Recently, an 82-year-old female developed GBS two weeks after receipt of the first dose of Pfizer COVID-19 vaccine (19). Thereby, rational interpretation of GBS occurrence temporally associated with vaccination is needed to the public. A valid interpretation of the coincidental adverse events may prevent from misunderstanding such reports, and contribute to the acceptance of vaccination campaigns.
In order to identify the incidence rate of GBS in populations that received viral vaccines during mass immunization campaigns, we conducted this systematic review and meta-analysis. We expected to provide the reference for the public to assess post-vaccination GBS validly, and engage in averting potential spurious association between the vaccine and coincidental adverse events during the mass vaccination against SARS-CoV-2.
Materials and Methods
This study was conducted by reference to the Preferred Reporting Items for Systematic Reviews and Meta-Analyses (PRISMA) guideline (20), which is provided in Table S1.
Literature Search Strategy
We performed a systematic literature search on Embase and PubMed databases to identify all relevant studies published up to December 31, 2020. The search strategy was based on the combination of the following terms: “Guillain-Barré syndrome”, “Guillain-Barre syndrome”, “acute infectious polyneuritis”, “acute inflammatory demyelinating polyneuropathy”, “Landry-Kussmaul syndrome”, “Landry-Guillain-Barré syndrome”, “Landry′s syndrome”, “Kussmaul-Landry syndrome”, “Landry′s paralysis”, “vaccine”, “vaccination”, “inoculation”, “immunize”, “vaccinum”, “bacterin”, “immunization”, “immunise”, “immune”, “vaccines”. References cited in the included articles were also screened to find additional studies.
Literature Screening and Selection
Firstly, the titles and abstracts of the publications were reviewed by two authors (FW and DW) independently. Secondly, the full text and online supplementary data were read to determine the eligibility of the publications. Any uncertainties and discrepancies were resolved by discussion with the third author (YW). The inclusion criteria were: 1) studies that reported temporal coincidence of GBS in mass immunizations; 2) participants received viral vaccines, including but not limited to influenza vaccine, HPV vaccine, polio vaccine, hepatitis vaccine, measles-rubella vaccine, rubella vaccine, or measles-mumps-rubella (MMR); 3) the following data were available or can be calculated: number of GBS patients, number of vaccinated populations, or background rate of GBS after vaccination. The quality of the literature was assessed by two authors (CL and YW) independently (Table S2).
Studies matching the following items were excluded: 1) reviews, case report studies, letters and conference abstracts; 2) animal studies; 3) clinical studies evaluating the safety of vaccines; 4) studies did not provide the information of vaccines in detail; 5) studies with the duplicate publication or overlapping data.
Data Extraction
Two authors collected the following data independently: 1) first author’s name; 2) publication year; 3) characteristic of patients (e.g., ethnicity, region, gender, age-range); 4) information of vaccines (e.g., the target viruses of vaccines, type of vaccine, follow-up duration after vaccination, the valence of vaccines and adjuvants of vaccines); 5) the number of study participants; 6) background rate and/or number of coincident cases of GBS during vaccination; 7) sources of vaccination. If there were duplicate data, the studies with larger sample size or newly published ones were involved.
Statistical Analysis
The meta-analysis, a statistical procedure for the combination of the results from multiple independent studies, was performed using STATA version 16.0 (STATA Corp, College Station, TX, USA) and/or R version 4.0.4 (Foundation for Statistical Computing, Vienna, Austria), by which the pooled background rate and its 95% confidence interval (CI) were calculated. Cochran’s Q-test and I2 statistics were applied to measure the significance of heterogeneity across eligible studies. Heterogeneity was assumed insignificant if P > 0.05 and I2 < 50%, then a fixed-effect model meta-analysis was carried out, otherwise, heterogeneity was considered statistically significant, then the Der-Simonian Laird’s random-effect model was implemented (21). Moreover, subgroup analyses were conducted on the basis of gender, age range, ethnicity, target virus, type of vaccines, follow-up duration after vaccination, the valence of vaccines and adjuvants of vaccines. To estimate the stability of the pooled results and distinguish the potential influence of individual studies, a sensitivity analysis was conducted by sequential removal of every single study one at a time. In addition, the publication bias was modeled by the funnel plots and analyzed by the Egger’s test. Furthermore, the trim-fill method was used to adjust for publication bias when it is significant. A P < 0.05 was considered significant if not mentioned specifically.
Results
Literature Search
A total of 2,201 publications (1,081 from PubMed and 1,120 from Embase) were retrieved, among which 943 duplicate records were excluded. After reviewed by titles and abstracts of the remained studies 1,124 publications were excluded for the following reasons: 188 studies were reviewed, case report studies, letters and conference abstracts; 102 were no-human-based researches; 179 were clinical trials; 226 were not studies on the incidence of GBS in vaccinees; 305 were not studies on virus vaccines; and 124 were researches on the mechanism of GBS. Among the 134 articles evaluated by full-text, 64 were excluded due to no details of vaccines or doses; 17 were excluded due to no number of coincident cases of GBS during vaccination; 5 were excluded due to duplicate data.
We checked the database of original studies on GBS after vaccination, and excluded one study of seasonal influenza vaccine in the U.S (22), one H1N1 vaccine study in the U.S (23), one HPV vaccine study in the U.S (24), and two studies of H1N1 vaccine in China due to duplicate data (25, 26). Finally, 48 publications with 58 independent studies were included in our meta-analysis (12, 27–74). The flow chart of literature screening process is shown in Figure 1.
Characteristics of Included Studies
Of the 58 studies, 45 reported the incidence of GBS for influenza vaccines, seven reported HPV vaccines, one reported polio vaccine, one reported hepatitis vaccine, two reported Measles-Rubella vaccines, one reported Rubella vaccine, one reported measles-mumps-rubella vaccine (MMR) vaccine. There are 20 studies on inactivated virus vaccines, seven on live-attenuated vaccines, one on the recombinant vaccine and one on the split-virion vaccine. With regard to the duration of follow-up, 32 studies reported the background rate of GBS within six weeks after vaccination. The details are listed in Table 1.
Pooled Results of Post-Vaccination GBS Rate
As shown in Figure 2, the pooled GBS rate, synthesized by a random-effects model, was 5.29 per million (95% CI: 3.66 to 6.93 per million) after immunization of viral vaccines. The heterogeneity test showed significant heterogeneity between studies (I2 = 98%, P < 0.01). Since the time period between 0 and 6 weeks is considered as the risk window after vaccination, we evaluated the GBS rate in this period. As result, the pooled rate was 3.09 per million persons (95% CI: 2.67 to 3.51 per million) for the 42-day window, equally 2.47 per 100,000 person-year (95%CI: 2.14 to 2.81 per 100,000 person-year). In contrast, as shown in Table S3, the previous studies that estimated incidence rates of GBS within general populations showed a range from 0.42 to 2.42 per 100,000 per person-year, meaning that there was no significant increase in GBS among population received viral vaccines.
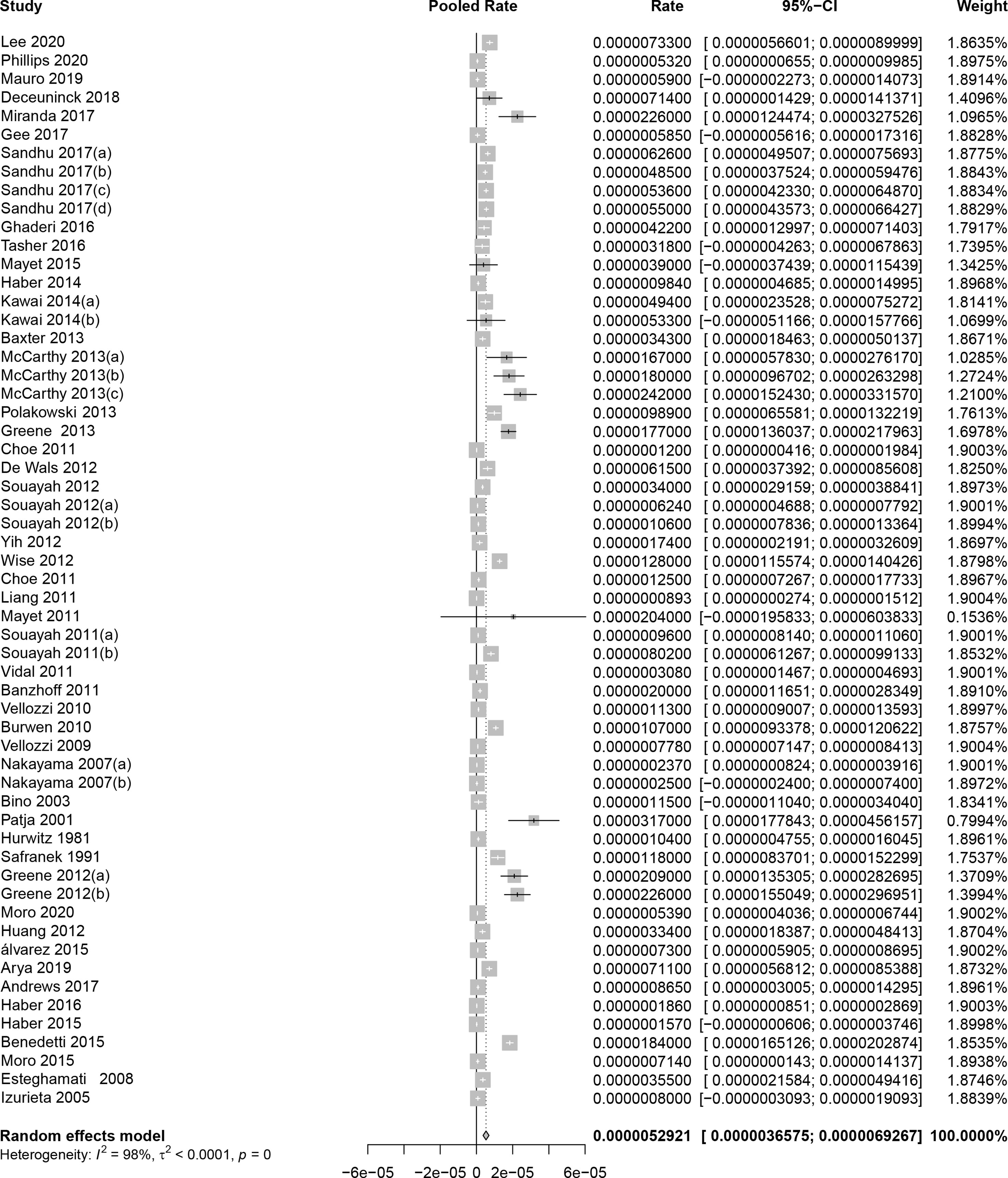
Figure 2 Pooled background rates of Guillain-Barré syndrome during mass immunization. CI, confidence interval.
Subgroup Analyses
Subgroup analyses were performed on the basis of gender, age, ethnicity, target virus, type of vaccines, the valence of vaccines and adjuvants of vaccines. As shown in Table 2, the pooled incidence rates of GBS were 7.26 per million (95%CI: 3.11 to 11.41 per million) among people aged <18 years, 0.99 per million (95%CI: 0.24 to 1.73 per million) among people aged 18 to 59 years, and 6.06 per million (95%CI: 2.51 to 9.61 per million) among people aged ≥ 60 years of age. The pooled rates were 6.31 per million (95%CI: 0.81 to 11.82 per million) among men, and 6.41 per million (95%CI: 2.53 to 10.30 per million) among women. The pooled GBS rates were 5.89 per million (95%CI: 4.05 to 7.72 per million) among Caucasian vaccinees, and 0.61 per million (95%CI: 0.32 to 0.91 per million) among Asian vaccinees.
Based on 29 original studies reported vaccines types, the pooled GBS rates were 5.01 per million (95%CI: 2.29 to 7.73 per million) for inactivated viral vaccine, 0.68 per million (95%CI: 0.17 to 1.20 per million) for the live-attenuated vaccine, respectively. The pooled background rates of GBS were 2.77 per million (95%CI: 2.47 to 3.07 per million) for individuals received influenza vaccine, and 2.44 per million (95%CI: 0.97 to 3.91 per million) for those received HPV vaccine. In addition, the pooled background rates were 3.98 per million (95% CI: 2.65 to 5.32 per million) for monovalent vaccines of influenza vaccine, 1.94 per million (95% CI: 1.46 to 2.41 per million) for trivalent vaccines of influenza, and 0.18 per million (95% CI: 0.09 to 0.27 per million) for quadrivalent vaccines of influenza, respectively.
There were four studies reported the details of vaccine adjuvants. Among them three used AS03 adjuvant, and one had MF59 adjuvant. The pooled background rate of GBS was 5.40 per million (95%CI: 3.54 to 7.26 per million) for vaccine with AS03 adjuvant.
Publication Bias and Sensitivity Analyses
Funnel plot analysis and Egger’s test were used to examine the significance of publication bias underlying our study, by which a statistical significance was identified (Table 2 and Figure 3). In order to control publication bias the trim-fill method was further performed, by which the pooled GBS rate was 1.71 per million (95% CI: 0 to 3.96 per million) after immunization of vaccine against virus, and 1.89 per million (95% CI: 1.48 to 2.30 per million) in 6 weeks follow-up after immunization.
To examine the strength of the pooled results, we performed a sensitivity analysis by omitting one study at a time. Consequently, the pooled result was not dominantly affected by any of the individual studies (Figure S1), indicating high stability of our results.
Discussion
To the best of our knowledge, this study is the first to comprehensively summarize the incidence rates of GBS following mass immunizations of viral vaccines. Our meta-analyses, involving 58 original studies and 2,110,441,600 participants, identified a pooled rate 5.29 per million (95% CI:3.66 to 6.93 per million) among people received viral vaccines, and a pooled rate 3.09 per million (95% CI:2.67 to 3.51 per million) in 6 weeks of vaccination, equally 2.47 per 100,000 person-year (95%CI: 2.14 to 2.81 per 100,000 person-year). There was no significant increase in GBS incidence among population received viral vaccines compared to general population without prior vaccination. Subgroup analyses released the pooled rates of 2.77 per million (95%CI: 2.47 to 3.07 per million) for individuals received influenza vaccine and 2.44 per million (95%CI: 0.97 to 3.91 per million) for HPV vaccinees, respectively.
GBS is a demyelinating transient neurological disorder characterized by lack of paralysis and sensory impairment. GBS is an immune-related disorder, in which the immune response generates antibodies that cross-react with gangliosides (i.e., GM1, GD1a, GT1b and GQ1b) at nerve membranes (75). This autoimmune response results in nerve damage or functional blockade of nerve conduction (76). Aberrant active immunization induced by artificial vaccines, hypothetically, is able to stimulate the immune system to produce specific antibodies, which contribute to cross reaction with epitopes on myelin or axons, leading to nerve damage (77). Vaccines might, as understood, damage the peripheral nerves directly (78). However, the causal associations between vaccines and GBS have not been substantially proved, i.e., the association might not be causally established.
The mass immunization against COVID-19 has started unprecedentedly on a global scale. Recently, coincident GBS case was observed after administrated with COVID-19 vaccine (19). New considerations about vaccine safety will undoubtedly arise. Toward the public, it is critical to distinguish events that are temporally associated with vaccination from those directly caused by vaccines. Misinterpretation of GBS incidence that is only temporally coincident with but not caused by vaccination will not only obstruct the success of mass vaccination, but also hinder the development of newer vaccines (9).
During the 1976-77 A/H1N1 influenza immunization campaign, an increase of GBS was reported after vaccine administration (15), which suspended the immunization program temporarily, and initiated vaccine safety concerns. In the 1993-1994 influenza seasons, public concern of vaccine-related GBS arose again due to the increment of GBS (79). The 2009 H1N1 influenza pandemic motivated H1N1 vaccine campaigns in North America and Europe, where post-vaccination GBS concern was raised consequently (29). However, in 2009-2010, a surveillance of H1N1 influenza vaccine in 45 million persons showed a lower excess risk for GBS during the immunization campaign compared to earlier vaccination (73). In France, a study did not support the causation between GBS and H1N1 vaccination (80). Our pooled results show that the temporal coincidence of GBS in influenza vaccinees is not higher than that among general populations unvaccinated.
With regard to HPV, the debate on vaccine safety still exists, which remains one of the barriers to achievement of intensive global vaccination coverage. The Vaccine Adverse Event Reporting System (VAERS) in the United States reported a GBS rate of 0.2 per 100,000 dosages coincided with HPV vaccination from 2006 to 2008 (24). In a school-based HPV study in Canada, the overall background rate was 0.73/100,000 person-year for adolescents aged 7-17 years (81). Similarly, our study did not observe an increase in background rate of GBS after HPV vaccine administration.
The adjuvants of vaccines could affect the magnitude and quality of immune response. The AS03 adjuvant contains α-tocopherol, which might promote immune system activation in the nonregional lymph nodes (82), whereas MF59 might modulate cellular immune response at the injection site or regional lymph nodes (83). Moreover, an in vitro study demonstrated that α-tocopherol can raise the expression of hypocretin, leading to antigen presentation via human leukocyte antigens (84), which results in an autoimmune response, and damages hypocretin-producing neurons. In this current study, both vaccines adjuvanted with AS03 and those with MF59 have lower background rates of GBS than that of general populations, even though the background rate among individuals received vaccines with AS03 adjuvant is higher than that with MF59 adjuvant.
Our study has potential limitations that usually exist in observational studies and systematic reviews. Firstly, we aimed to summary data from studies that reported background rate of GBS in mass immunizations, which reflected the temporally coincidence of GBS in “real world”. In accordance with the predefined protocol, we did not include clinical trials that evaluate the safety of vaccines. Secondly, there was heterogeneity across original studies, which might limit the consolidation of the findings. Thirdly, most of original studies involved Caucasian participants, limiting the representability of our findings for other ethnic groups. Fourthly, as all the original studies are based on vaccine surveillance data, the methodological quality was not able to be evaluated. Fifthly, the increase in GBS reported in vaccination campaigns might result from the higher detection rate among vaccinees and increased reporting levels of GBS cases following receipt of vaccines.
In conclusion, our findings evidenced a mild increase in coincidental GBS during virus vaccination. We presented a reference for evaluation of the coincidental occurrence of GBS in mass vaccination campaigns, including SARS-CoV-2 vaccine.
Data Availability Statement
The original contributions presented in the study are included in the article/Supplementary Material. Further inquiries can be directed to the corresponding authors.
Author Contributions
HH, YXW, and WW designed this study. FW, DW, YJW, and CL contributed to literature search, review, data extraction. YLZ, ZG, PL, and YCZ conducted statistical analyses. FW, DW, YJW, and CL wrote the manuscript. HH, YXW, and WW contributed to manuscript revision. All authors have reviewed and approved the final version of this manuscript.
Conflict of Interest
The authors declare that the research was conducted in the absence of any commercial or financial relationships that could be construed as a potential conflict of interest.
Publisher’s Note
All claims expressed in this article are solely those of the authors and do not necessarily represent those of their affiliated organizations, or those of the publisher, the editors and the reviewers. Any product that may be evaluated in this article, or claim that may be made by its manufacturer, is not guaranteed or endorsed by the publisher.
Acknowledgments
We thank all the authors of the included studies of this meta-analysis.
Supplementary Material
The Supplementary Material for this article can be found online at: https://www.frontiersin.org/articles/10.3389/fimmu.2022.782198/full#supplementary-material.
Supplementary Figure 1 | Forest plot of sensitivity analysis.CI, confidence interval.
References
1. Khalifa M, Zakaria F, Ragab Y, Saad A, Bamaga A, Emad Y, et al. Guillain-Barré Syndrome Associated With Severe Acute Respiratory Syndrome Coronavirus 2 Detection and Coronavirus Disease 2019 in a Child. J Pediatr Infect Dis Soc (2020) 9(4):510–3. doi: 10.1093/jpids/piaa086
2. WHO. Coronavirus (COVID-19) Dashboard. (2021). Available at: https://covid19.who.int/.
3. Iltaf S Sr, Fatima M, Salman S Sr, Salam JU, Abbas S. Frequency of Neurological Presentations of Coronavirus Disease in Patients Presenting to a Tertiary Care Hospital During the 2019 Coronavirus Disease Pandemic. Cureus (2020) 12(8):e9846. doi: 10.7759/cureus.9846
4. Alramthan A, Aldaraji W. Two Cases of COVID-19 Presenting With a Clinical Picture Resembling Chilblains: First Report From the Middle East. Clin Exp Dermatol (2020) 45(6):746–8. doi: 10.1111/ced.14243
5. Mostel Z, Ayat P, Capric V, Trimmingham A, McFarlane SI. Guillain-Barré Syndrome in a COVID-19 Patient: A Case Report and Review of Management Strategies. Am J Med Case Rep (2021) 9(3):198–200. doi: 10.12691/ajmcr-9-3-16
6. Corbett KS, Edwards DK, Leist SR, Abiona OM, Boyoglu-Barnum S, Gillespie RA, et al. SARS-CoV-2 mRNA Vaccine Design Enabled by Prototype Pathogen Preparedness. Nature (2020) 586(7830):567–71. doi: 10.1038/s41586-020-2622-0
7. Hodgson SH, Mansatta K, Mallett G, Harris V, Emary KRW, Pollard AJ. What Defines an Efficacious COVID-19 Vaccine? A Review of the Challenges Assessing the Clinical Efficacy of Vaccines Against SARS-CoV-2. Lancet Infect Dis (2021) 21(2):e26–35. doi: 10.1016/s1473-3099(20)30773-8
8. Lee WS, Wheatley AK, Kent SJ, DeKosky BJ. Antibody-Dependent Enhancement and SARS-CoV-2 Vaccines and Therapies. Nat Microbiol (2020) 5(10):1185–91. doi: 10.1038/s41564-020-00789-5
9. Black S, Eskola J, Siegrist CA, Halsey N, MacDonald N, Law B, et al. Importance of Background Rates of Disease in Assessment of Vaccine Safety During Mass Immunisation With Pandemic H1N1 Influenza Vaccines. Lancet (Lond Engl) (2009) 374(9707):2115–22. doi: 10.1016/s0140-6736(09)61877-8
10. Milligan R, Paul M, Richardson M, Neuberger A. Vaccines for Preventing Typhoid Fever. Cochrane Database Syst Rev (2018) 5(5):Cd001261. doi: 10.1002/14651858.CD001261.pub4
11. Sriwastava S, Kataria S, Tandon M, Patel J, Patel R, Jowkar A, et al. Guillain Barré Syndrome and its Variants as a Manifestation of COVID-19: A Systemic Review of Case Report and Case Series. J Neurol Sci (2021) 420:117263. doi: 10.1016/j.jns.2020.117263
12. Burwen DR, Ball R, Bryan WW, Izurieta HS, Voie LL, Gibbs NA, et al. Evaluation of Guillain-Barré Syndrome Among Recipients of Influenza Vaccine in 2000 and 2001. Am J Prev Med (2010) 39(4):296–304. doi: 10.1016/j.amepre.2010.05.022
13. Malek E, Salameh J. Guillain-Barre Syndrome. Semin Neurol (2019) 39(5):589–95. doi: 10.1055/s-0039-1693005
14. Delannoy A, Rudant J, Chaignot C, Bolgert F, Mikaeloff Y, Weill A. Guillain-Barré Syndrome in France: A Nationwide Epidemiological Analysis Based on Hospital Discharge Data (2008-2013). J Peripher Nerv Syst JPNS (2017) 22(1):51–8. doi: 10.1111/jns.12202
15. Schonberger LB, Bregman DJ, Sullivan-Bolyai JZ, Keenlyside RA, Ziegler DW, Retailliau HF, et al. Guillain-Barre Syndrome Following Vaccination in the National Influenza Immunization Program, United States, 1976–1977. Am J Epidemiol (1979) 110(2):105–23. doi: 10.1093/oxfordjournals.aje.a112795
16. Mouchet J, Salvo F, Raschi E, Poluzzi E, Antonazzo IC, De Ponti F, et al. Human Papillomavirus Vaccine and Demyelinating Diseases-A Systematic Review and Meta-Analysis. Pharmacol Res (2018) 132:108–18. doi: 10.1016/j.phrs.2018.04.007
17. Mahumud RA, Alam K, Keramat SA, Ormsby GM, Dunn J, Gow J. Cost-Effectiveness Evaluations of the 9-Valent Human Papillomavirus (HPV) Vaccine: Evidence From a Systematic Review. PloS One (2020) 15(6):e0233499. doi: 10.1371/journal.pone.0233499
18. Wang A, Liu C, Wang Y, Yin A, Wu J, Zhang C, et al. Pregnancy Outcomes After Human Papillomavirus Vaccination in Periconceptional Period or During Pregnancy: A Systematic Review and Meta-Analysis. Hum Vaccines Immunotherapeut (2020) 16(3):581–9. doi: 10.1080/21645515.2019.1662363
19. Waheed S, Bayas A, Hindi F, Rizvi Z, Espinosa PS. Neurological Complications of COVID-19: Guillain-Barre Syndrome Following Pfizer COVID-19 Vaccine. Cureus (2021) 13(2):e13426. doi: 10.7759/cureus.13426
20. Hou H, Zhao Y, Yu W, Dong H, Xue X, Ding J, et al. Association of Obstructive Sleep Apnea With Hypertension: A Systematic Review and Meta-Analysis. J Glob Health (2018) 8(1):10405. doi: 10.7189/jogh.08.010405
21. Higgins JP, Thompson SG. Quantifying Heterogeneity in a Meta-Analysis. Stat Med (2002) 21(11):1539–58. doi: 10.1002/sim.1186
22. Geier MR, Geier DA, Zahalsky AC. Influenza Vaccination and Guillain Barre Syndrome. Clin Immunol (2003) 107: (2):116–21. doi: 10.1016/S1521-6616(03)00046-9
23. Vellozzi C, Iqbal S, Stewart B, Tokars J, DeStefano F. Cumulative Risk of Guillain-Barré Syndrome Among Vaccinated and Unvaccinated Populations During the 2009 H1N1 Influenza Pandemic. Am J Public Health (2014) 104(4):696–701. doi: 10.2105/AJPH.2013.301651
24. Slade BA, Leidel L, Vellozzi C, Woo EJ, Hua W, Sutherland A, et al. Postlicensure Safety Surveillance for Quadrivalent Human Papillomavirus Recombinant Vaccine. JAMA J Am Med Assoc (2009) 302(7):750–7. doi: 10.1001/jama.2009.1201
25. Wu J, Xu F, Lu L, Lu M, Miao L, Gao T, et al. Safety and Effectiveness of a 2009 H1N1 Vaccine in Beijing. N Engl J Med (2010) 363(25):2416–23. doi: 10.1056/NEJMoa1006736
26. Huang WT, Yang HW, Liao TL, Wu WJ, Yang SE, Chih YC, et al. Safety of Pandemic (H1N1) 2009 Monovalent Vaccines in Taiwan: A Self-Controlled Case Series Study. PloS One (2013) 8(3):e58827. doi: 10.1371/journal.pone.0058827
27. Andrews N, Stowe J, Miller E. No Increased Risk of Guillain-Barré Syndrome After Human Papilloma Virus Vaccine: A Self-Controlled Case-Series Study in England. Vaccine (2017) 35(13):1729–32. doi: 10.1016/j.vaccine.2017.01.076
28. Arya DP, Said MA, Izurieta HS, Perez-Vilar S, Zinderman C, Wernecke M, et al. Surveillance for Guillain-Barré Syndrome After 2015–2016 and 2016–2017 Influenza Vaccination of Medicare Beneficiaries. Vaccine (2019) 37(43):6543–9. doi: 10.1016/j.vaccine.2019.08.045
29. Banzhoff A, Haertel S, Praus M. Passive Surveillance of Adverse Events of an MF59-Adjuvanted H1N1v Vaccine During the Pandemic Mass Vaccinations. Hum Vaccines (2011) 7(5):539–48. doi: 10.4161/hv.7.5.14821
30. Baxter R, Bakshi N, Fireman B, Lewis E, Ray P, Vellozzi C, et al. Lack of Association of Guillain-Barré Syndrome With Vaccinations. Clin Infect Dis (2013) 57(2):197–204. doi: 10.1093/cid/cit222
31. Benedetti MD, Pugliatti M, Dalessandro R, Beghi E, Chiò A, Logroscino G, et al. A Multicentric Prospective Incidence Study of Guillain-Barre Syndrome in Italy. The ITANG Study. Neuroepidemiology (2015) 45(2):90–9. doi: 10.1159/000438752
32. Bino S, Kakarriqi E, Xibinaku M, Ion-Nedelcu N, Bukli M, Emiroglu N, et al. Measles-Rubella Mass Immunization Campaign in Albania, November 2000. J Infect Dis (2003) 187(SUPPL. 1):S223–9. doi: 10.1086/368055
33. Chang S, O'Connor PM, Slade BA, Woo EJ. U.S. Postlicensure Safety Surveillance for Adolescent and Adult Tetanus, Diphtheria and Acellular Pertussis Vaccines: 2005-2007. Vaccine (2013) 31(10):1447–52. doi: 10.1016/j.vaccine.2012.10.097
34. Choe YJ, Cho H, Kim SN, Bae GR, Lee JK. Serious Adverse Events Following Receipt of Trivalent Inactivated Influenza Vaccine in Korea, 2003-2010. Vaccine (2011) 29(44):7727–32. doi: 10.1016/j.vaccine.2011.07.129
35. De Wals P, Deceuninck G, Toth E, Boulianne N, Brunet D, Boucher RM, et al. Risk of Guillain-Barré Syndrome Following H1N1 Influenza Vaccination in Quebec. JAMA J Am Med Assoc (2012) 308(2):175–81. doi: 10.1001/jama.2012.7342
36. Deceuninck G, Sauvageau C, Gilca V, Boulianne N, De Serres G. Absence of Association Between Guillain-Barré Syndrome Hospitalizations and HPV-Vaccine. Expert Rev Vaccines (2018) 17(1):99–102. doi: 10.1080/14760584.2018.1388168
37. Esteghamati A, Gouya MM, Keshtkar AA, Mahoney F. Relationship Between Occurrence of Guillain-Barre Syndrome and Mass Campaign of Measles and Rubella Immunization in Iranian 5-14 Years Old Children. Vaccine (2008) 26(39):5058–61. doi: 10.1016/j.vaccine.2008.07.014
38. Choe YJ, Cho H, Bae GR, Lee JK. Guillain-Barré Syndrome Following Receipt of Influenza A (H1N1) 2009 Monovalent Vaccine in Korea With an Emphasis on Brighton Collaboration Case Definition. Vaccine (2011) 29(11):2066–70. doi: 10.1016/j.vaccine.2011.01.007
39. Gee J, Sukumaran L, Weintraub E. Risk of Guillain-Barré Syndrome Following Quadrivalent Human Papillomavirus Vaccine in the Vaccine Safety Datalink. Vaccine (2017) 35(43):5756–8. doi: 10.1016/j.vaccine.2017.09.009
40. Ghaderi S, Gunnes N, Bakken IJ, Magnus P, Trogstad L, Håberg SE. Risk of Guillain-Barré Syndrome After Exposure to Pandemic Influenza A(H1N1)pdm09 Vaccination or Infection: A Norwegian Population-Based Cohort Study. Eur J Epidemiol (2016) 31(1):67–72. doi: 10.1007/s10654-015-0047-0
41. Greene SK, Rett M, Weintraub ES, Li L, Yin R, Amato AA, et al. Risk of Confirmed Guillain-Barre Syndrome Following Receipt of Monovalent Inactivated Influenza A (H1N1) and Seasonal Influenza Vaccines in the Vaccine Safety Datalink Project, 2009-2010. Am J Epidemiol (2012) 175(11):1100–9. doi: 10.1093/aje/kws195
42. Greene SK, Rett MD, Vellozzi C, Li L, Kulldorff M, Marcy SM, et al. Guillain-Barré Syndrome, Influenza Vaccination, and Antecedent Respiratory and Gastrointestinal Infections: A Case-Centered Analysis in the Vaccine Safety Datalink, 2009-2011. PloS One (2013) 8(6):e67185. doi: 10.1371/journal.pone.0067185
43. Haber P, Moro PL, Cano M, Lewis P, Stewart B, Shimabukuro TT. Post-Licensure Surveillance of Quadrivalent Live Attenuated Influenza Vaccine United States, Vaccine Adverse Event Reporting System (VAERS), July 2013-June 2014. Vaccine (2015) 33(16):1987–92. doi: 10.1016/j.vaccine.2015.01.080
44. Haber P, Moro PL, Lewis P, Woo EJ, Jankosky C, Cano M. Post-Licensure Surveillance of Quadrivalent Inactivated Influenza (IIV4) Vaccine in the United States, Vaccine Adverse Event Reporting System (VAERS), July 1, 2013-May 31, 2015. Vaccine (2016) 34(22):2507–12. doi: 10.1016/j.vaccine.2016.03.048
45. Haber P, Moro PL, McNeil MM, Lewis P, Woo EJ, Hughes H, et al. Post-Licensure Surveillance of Trivalent Live Attenuated Influenza Vaccine in Adults, United States, Vaccine Adverse Event Reporting System (VAERS), July 2005-June 2013. Vaccine (2014) 32(48):6499–504. doi: 10.1016/j.vaccine.2014.09.018
46. Huang WT, Huang WI, Huang YW, Hsu CW, Chuang JH. The Reporting Completeness of a Passive Safety Surveillance System for Pandemic (H1N1) 2009 Vaccines: A Capture-Recapture Analysis. Vaccine (2012) 30(12):2168–72. doi: 10.1016/j.vaccine.2012.01.013
47. Hurwitz ES, Schonberger LB, Nelson DB, Holman RC. Guillain-Barré Syndrome and the 1978-1979 Influenza Vaccine. N Engl J Med (1981) 304(26):1557–61. doi: 10.1056/nejm198106253042601
48. Izurieta HS, Haber P, Wise RP, Iskander J, Pratt D, Mink C, et al. Adverse Events Reported Following Live, Cold-Adapted, Intranasal Influenza Vaccine. J Am Med Assoc (2005) 294(21):2720–5. doi: 10.1001/jama.294.21.2720
49. Kawai AT, Li L, Kulldorff M, Vellozzi C, Weintraub E, Baxter R, et al. Absence of Associations Between Influenza Vaccines and Increased Risks of Seizures, Guillain-Barré Syndrome, Encephalitis, or Anaphylaxis in the 2012-2013 Season. Pharmacoepidemiol Drug Saf (2014) 23(5):548–53. doi: 10.1002/pds.3575
50. Lee H, Kang HY, Jung SY, Lee YM. Incidence of Guillain-Barré Syndrome Is Not Associated With Influenza Vaccination in the Elderly. Vaccines (2020) 8(3):1–13. doi: 10.3390/vaccines8030431
51. Liang XF, Li L, Liu DW, Li KL, Wu WD, Zhu BP, et al. Safety of Influenza A (H1N1) Vaccine in Postmarketing Surveillance in China. N Engl J Med (2011) 364(7):638–47. doi: 10.1056/NEJMoa1008553
52. Mauro AB, Fernandes EG, Miyaji KT, Arantes BA, Valente MG, Sato HK, et al. Adverse Events Following Quadrivalent HPV Vaccination Reported in Sao Paulo State, Brazil, in the First Three Years After Introducing the Vaccine for Routine Immunization (March 2014 to December 2016). Rev Do Inst Med Trop Sao Paulo (2019) 61:e43. doi: 10.1590/s1678-9946201961043
53. Mayet A, Duron S, Meynard JB, Koeck JL, Deparis X, Migliani R. Surveillance of Adverse Events Following Vaccination in the French Armed Forces, 2011-2012. Public Health (2015) 129(6):763–8. doi: 10.1016/j.puhe.2015.03.003
54. Mayet A, Ligier C, Gache K, Manet G, Nivoix P, Dia A, et al. Adverse Events Following Pandemic Influenza Vaccine Pandemrix® Reported in the French Military Forces-2009-2010. Vaccine (2011) 29(14):2576–81. doi: 10.1016/j.vaccine.2011.01.056
55. McCarthy NL, Gee J, Lin ND, Thyagarajan V, Pan Y, Su S, et al. Evaluating the Safety of Influenza Vaccine Using a Claims-Based Health System. Vaccine (2013) 31(50):5975–82. doi: 10.1016/j.vaccine.2013.10.031
56. Miranda S, Chaignot C, Collin C, Dray-Spira R, Weill A, Zureik M. Human Papillomavirus Vaccination and Risk of Autoimmune Diseases: A Large Cohort Study of Over 2 Million Young Girls in France. Vaccine (2017) 35(36):4761–8. doi: 10.1016/j.vaccine.2017.06.030
57. Moro PL, Winiecki S, Lewis P, Shimabukuro TT, Cano M. Surveillance of Adverse Events After the First Trivalent Inactivated Influenza Vaccine Produced in Mammalian Cell Culture (Flucelvax®) Reported to the Vaccine Adverse Event Reporting System (VAERS), United States, 2013-2015. Vaccine (2015) 33(48):6684–8. doi: 10.1016/j.vaccine.2015.10.084
58. Moro PL, Woo EJ, Marquez P, Cano M. Monitoring the Safety of High-Dose, Trivalent Inactivated Influenza Vaccine in the Vaccine Adverse Event Reporting System (VAERS), 2011 – 2019. Vaccine (2020) 38(37):5923–6. doi: 10.1016/j.vaccine.2020.07.007
59. Nakayama T, Onoda K. Vaccine Adverse Events Reported in Post-Marketing Study of the Kitasato Institute From 1994 to 2004. Vaccine (2007) 25(3):570–6. doi: 10.1016/j.vaccine.2006.05.130
60. Patja A, Paunio M, Kinnunen E, Junttila O, Hovi T, Peltola H. Risk of Guillain-Barré Syndrome After Measles-Mumps-Rubella Vaccination. J Pediatr (2001) 138(2):250–4. doi: 10.1067/mpd.2001.111165
61. Phillips A, Hickie M, Totterdell J, Brotherton J, Dey A, Hill R, et al. Adverse Events Following HPV Vaccination: 11 Years of Surveillance in Australia. Vaccine (2020) 38(38):6038–46. doi: 10.1016/j.vaccine.2020.06.039
62. Polakowski LL, Sandhu SK, Martin DB, Ball R, Macurdy TE, Franks RL, et al. Chart-Confirmed Guillain-Barre Syndrome After 2009 H1N1 Influenza Vaccination Among the Medicare Population, 2009-2010. Am J Epidemiol (2013) 178(6):962–73. doi: 10.1093/aje/kwt051
63. Ropero-Álvarez AM, Whittembury A, Bravo-Alcántara P, Kurtis HJ, Danovaro-Holliday MC, Velandia-González M. Events Supposedly Attributable to Vaccination or Immunization During Pandemic Influenza A (H1N1) Vaccination Campaigns in Latin America and the Caribbean. Vaccine (2015) 33(1):187–92. doi: 10.1016/j.vaccine.2014.10.070
64. Safranek TJ, Lawrence DN, Kurland LT, Culver DH, Wiederholt WC, Hayner NS, et al. Reassessment of the Association Between Guillain-Barré Syndrome and Receipt of Swine Influenza Vaccine in 1976-1977: Results of a Two-State Study. Expert Neurology Group. Am J Epidemiol (1991) 133(9):940–51. doi: 10.1093/oxfordjournals.aje.a115973
65. Sandhu SK, Hua W, MaCurdy TE, Franks RL, Avagyan A, Kelman J, et al. Near Real-Time Surveillance for Guillain-Barré Syndrome After Influenza Vaccination Among the Medicare Population, 2010/11 to 2013/14. Vaccine (2017) 35(22):2986–92. doi: 10.1016/j.vaccine.2017.03.087
66. Souayah N, Michas-Martin PA, Nasar A, Krivitskaya N, Yacoub HA, Khan H, et al. Guillain-Barré Syndrome After Gardasil Vaccination: Data From Vaccine Adverse Event Reporting System 2006-2009. Vaccine (2011) 29(5):886–9. doi: 10.1016/j.vaccine.2010.09.020
67. Souayah N, Yacoub HA, Khan HMR, Farhad K, Maybodi L, Qureshi AI, et al. Analysis of Data From the CDC/FDA Vaccine Adverse Event Reporting System (1990-2009) on Guillain-Barre Syndrome After Hepatitis Vaccination in the USA. J Clin Neurosci (2012) 19(8):1089–92. doi: 10.1016/j.jocn.2011.11.022
68. Souayah N, Yacoub HA, Khan HMR, Michas-Martin PA, Menkes DL, Maybodi L, et al. Guillain-Barré Syndrome After H1N1 Vaccination in the United States: A Report Using the CDC/FDA Vaccine Adverse Event Reporting System (2009). Neuroepidemiology (2012) 38(4):227–32. doi: 10.1159/000336113
69. Tasher D, Kopel E, Anis E, Grossman Z, Somekh E. Causality Assessment of Serious Neurologic Adverse Events Following the bOPV National Vaccination Campaign in Israel. Israel Med Assoc J (2016) 18(10):590–3.
70. Vellozzi C, Broder KR, Haber P, Guh A, Nguyen M, Cano M, et al. Adverse Events Following Influenza A (H1N1) 2009 Monovalent Vaccines Reported to the Vaccine Adverse Event Reporting System, United States, October 1, 2009-January 31, 2010. Vaccine (2010) 28(45):7248–55. doi: 10.1016/j.vaccine.2010.09.021
71. Vellozzi C, Burwen DR, Dobardzic A, Ball R, Walton K, Haber P. Safety of Trivalent Inactivated Influenza Vaccines in Adults: Background for Pandemic Influenza Vaccine Safety Monitoring. Vaccine (2009) 27(15):2114–20. doi: 10.1016/j.vaccine.2009.01.125
72. Vidal P, Reyna J, Saldaña P, Richardson VL. Events Temporarily Associated With Anti-Influenza A (H1N1) Vaccination in Mexico. Arch Med Res (2011) 42(7):627–32. doi: 10.1016/j.arcmed.2011.10.007
73. Wise ME, Viray M, Sejvar JJ, Lewis P, Baughman AL, Connor W, et al. Guillain-Barre Syndrome During the 2009-2010 H1N1 Influenza Vaccination Campaign: Population-Based Surveillance Among 45 Million Americans. Am J Epidemiol (2012) 175(11):1110–9. doi: 10.1093/aje/kws196
74. Yih WK, Lee GM, Lieu TA, Ball R, Kulldorff M, Rett M, et al. Surveillance for Adverse Events Following Receipt of Pandemic 2009 H1N1 Vaccine in the Post-Licensure Rapid Immunization Safety Monitoring (PRISM) System, 2009-2010. Am J Epidemiol (2012) 175(11):1120–8. doi: 10.1093/aje/kws197
75. Kaida K, Ariga T, Yu RK. Antiganglioside Antibodies and Their Pathophysiological Effects on Guillain-Barré Syndrome and Related Disorders–a Review. Glycobiology (2009) 19(7):676–92. doi: 10.1093/glycob/cwp027
76. van den Berg B, Walgaard C, Drenthen J, Fokke C, Jacobs BC, van Doorn PA. Guillain-Barré Syndrome: Pathogenesis, Diagnosis, Treatment and Prognosis. Nat Rev Neurol (2014) 10(8):469–82. doi: 10.1038/nrneurol.2014.121
77. Haber P, Sejvar J, Mikaeloff Y, DeStefano F. Vaccines and Guillain-Barré Syndrome. Drug Saf (2009) 32(4):309–23. doi: 10.2165/00002018-200932040-00005
78. Martín Arias LH, Sanz R, Sáinz M, Treceño C, Carvajal A. Guillain-Barré Syndrome and Influenza Vaccines: A Meta-Analysis. Vaccine (2015) 33(31):3773–8. doi: 10.1016/j.vaccine.2015.05.013
79. Lasky T, Terracciano GJ, Magder L, Koski CL, Ballesteros M, Nash D, et al. The Guillain-Barré Syndrome and the 1992-1993 and 1993-1994 Influenza Vaccines. New Engl J Med (1998) 339(25):1797–802. doi: 10.1056/nejm199812173392501
80. Grimaldi-Bensouda L, Alpérovitch A, Besson G, Vial C, Cuisset JM, Papeix C, et al. Guillain-Barre Syndrome, Influenzalike Illnesses, and Influenza Vaccination During Seasons With and Without Circulating A/H1N1 Viruses. Am J Epidemiol (2011) 174(3):326–35. doi: 10.1093/aje/kwr072
81. Deceuninck G, Boucher RM, de Wals P, Ouakki M. Epidemiology of Guillain-Barré Syndrome in the Province of Quebec. Can J Neurol Sci (2008) 35(4):472–5. doi: 10.1017/S0317167100009136
82. Arango MT, Kivity S, Shoenfeld Y. Is Narcolepsy a Classical Autoimmune Disease? Pharmacol Res (2015) 92:6–12. doi: 10.1016/j.phrs.2014.10.005
83. Calabro S, Tortoli M, Baudner BC, Pacitto A, Cortese M, O'Hagan DT, et al. Vaccine Adjuvants Alum and MF59 Induce Rapid Recruitment of Neutrophils and Monocytes That Participate in Antigen Transport to Draining Lymph Nodes. Vaccine (2011) 29(9):1812–23. doi: 10.1016/j.vaccine.2010.12.090
Keywords: Guillain-Barré syndrome, virus, vaccine, mass immunization, systematic review, meta-analysis
Citation: Wang F, Wang D, Wang Y, Li C, Zheng Y, Guo Z, Liu P, Zhang Y, Wang W, Wang Y and Hou H (2022) Population-Based Incidence of Guillain-Barré Syndrome During Mass Immunization With Viral Vaccines: A Pooled Analysis. Front. Immunol. 13:782198. doi: 10.3389/fimmu.2022.782198
Received: 24 September 2021; Accepted: 14 January 2022;
Published: 03 February 2022.
Edited by:
Marc H. V. Van Regenmortel, Centre National de la Recherche Scientifique (CNRS), FranceReviewed by:
Harald Hegen, Innsbruck Medical University, AustriaElizabeth Miller, University of London, United Kingdom
Copyright © 2022 Wang, Wang, Wang, Li, Zheng, Guo, Liu, Zhang, Wang, Wang and Hou. This is an open-access article distributed under the terms of the Creative Commons Attribution License (CC BY). The use, distribution or reproduction in other forums is permitted, provided the original author(s) and the copyright owner(s) are credited and that the original publication in this journal is cited, in accordance with accepted academic practice. No use, distribution or reproduction is permitted which does not comply with these terms.
*Correspondence: Wei Wang, wei.wang@ecu.edu.au; Youxin Wang, wangy@ccmu.edu.cn; Haifeng Hou, hfhou@163.com
†These authors have contributed equally to this work