- 1School of Pharmacy, Lanzhou University, Lanzhou, China
- 2Macao Centre for Research and Development in Chinese Medicine, Institute of Chinese Medical Sciences, University of Macau, Macao, Macao SAR, China
Matrix metalloproteinases (MMPs) are an important class of enzymes in the body that function through the extracellular matrix (ECM). They are involved in diverse pathophysiological processes, such as tumor invasion and metastasis, cardiovascular diseases, arthritis, periodontal disease, osteogenesis imperfecta, and diseases of the central nervous system. MMPs participate in the occurrence and development of numerous cancers and are closely related to immunity. In the present study, we review the immunomodulatory role of MMPs in colitis-associated cancer (CAC) and discuss relevant clinical applications. We analyze more than 300 pharmacological studies retrieved from PubMed and the Web of Science, related to MMPs, cancer, colitis, CAC, and immunomodulation. Key MMPs that interfere with pathological processes in CAC such as MMP-2, MMP-3, MMP-7, MMP-9, MMP-10, MMP-12, and MMP-13, as well as their corresponding mechanisms are elaborated. MMPs are involved in cell proliferation, cell differentiation, angiogenesis, ECM remodeling, and the inflammatory response in CAC. They also affect the immune system by modulating differentiation and immune activity of immune cells, recruitment of macrophages, and recruitment of neutrophils. Herein we describe the immunomodulatory role of MMPs in CAC to facilitate treatment of this special type of colon cancer, which is preceded by detectable inflammatory bowel disease in clinical populations.
1 Introduction
1.1 Classification and structural characteristics of matrix metalloproteinases
MMPs are a kind of calcium-and zinc-dependent proteolytic enzyme (1), that exist in invertebrates, vertebrates and plants (2). They are produced by multiple cells and tissues, with neutrophils and dermal fibroblasts being the main sources (3). Connective tissue, pro-inflammatory and uteroplacental cells, including endothelial cells, osteoblasts, cytotrophoblasts, lymphocytes, macrophages, and vascular smooth muscle are also capable of secreting MMPs (4). Degrading the ECM is the main function of MMPs (5). The ECM plays an important role in the proliferation, growth, organization, differentiation, migration of cells, and in the exchange among information cells; it also acts as a physical barrier for microorganisms (6, 7). To date, 28 types of MMPs have been found. The homologous domains of these MMPs include the signal peptide domain, propeptide domain, catalytic domain and hinge region or linker peptide along with a hemopexin domain (4, 8). The hinge region connects the catalytic domain to the hemopexin domain (7, 9, 10). MMPs are divided into collagenases (e.g., MMP-1, MMP-8, MMP-13, and MMP-18) (11, 12), gelatinases (e.g., MMP-2 and MMP-9) (13, 14), stromelysins (e.g. MMP-3, MMP-10, and MMP-11) (15, 16), matrilysins (e.g. MMP-7 and MMP-26) (17), membrane-type MMPs (MT-MMPs) (MMP-14, MMP-15, MMP-16, MMP-17, MMP-24, and MMP-25), and others (MMP-12, MMP-19, MMP-21, and MMP-28) based on structural features and substrates (18–24). In particular, gelatinases have a special additional exosome insert in the catalytic domain called the collagen binding domain; matrilysins lack a C-terminal hemopexin-like domain linked by a hinge or linker region, and MT-MMPs have a C-terminal transmembrane domain with a short cytoplasmic tail (9, 25). The classifications and structures of MMPs are shown in Figure 1.
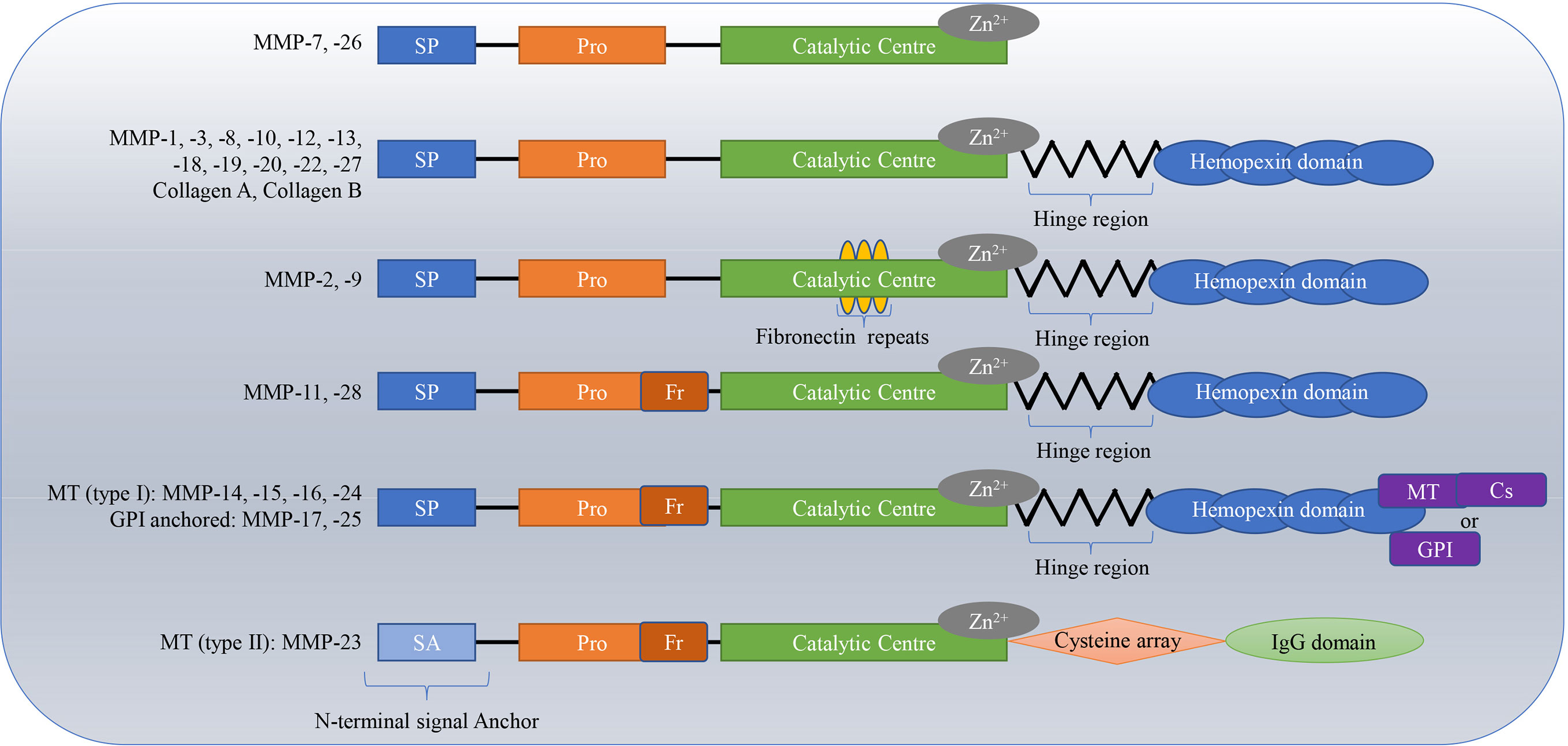
Figure 1 Classifications and structures of matrix metalloproteinases (MMPs). The homologous domains of these MMPs include the signal peptide domain (SP), propeptide domain (Pro), catalytic domain, and hinge region or linker peptide along with a hemopexin domain. The hinge region connects the catalytic domain to the hemopexin domain. Matrilysins lack a C-terminal hemopexin-like domain linked by a hinge or linker region; Gelatinases have special additional fibronectin repeats in the catalytic domain; MT-MMPs have a C-terminal transmembrane domain with a short cytoplasmic tail. MT, membrane-type; Cs, cytosolic; GPI, glycosylphosphatidylinositol.
1.2 Regulation of MMPs at multiple levels
MMP activity is regulated in three different ways, through transcriptional regulation, inhibition by specific inhibitors, or activation by the proenzyme (26). At the transcriptional level, the activator protein (AP) -1 and -2 sites, the NF-κB site, the signal transducer and activator of transcription site, the polyomavirus enhancer-A binding protein-3 site, and others are key transcription binding sites for regulation of the MMP gene (8, 27–33). The AP-1 site is located close to the most proximal promoter of the typical TATA box and is the major mediator in MMP gene regulation. In many MMP promoters that contain AP-1 site, the juxtaposed transcription factor binding sites bind multiple erythroblastosis twenty-six factors, which determine the peculiarities among different genes and affect gene expression (8). The polyomavirus enhancer-A binding protein-3 site, can combine with members of the erythroblastosis twenty-six factors family of oncoproteins and work in synergy with the AP-1 site nearby to promote the production of MMPs among cancer cells for migration and invasiveness (28). MicroRNA is a non-coding single-stranded RNA. In liver cancer cells, mRNA expression of MMP-2 and MMP-9 is up-regulated as a result of the inhibition of miR-21, which stimulates the invasion and migration of tumor cells. miR-224 is associated with gene expression of MMP-1, which enables breast tumors to metastasize to the bone (34). NEMO-binding domain, a synthetic peptide corresponding to the S100A4-binding domain of methionine aminopeptidase 2 [MetAP2], blocks interaction between the metastasis−enhancing calcium−binding protein (S100A4) and its effector protein (MetAP2). And this blockage inhibits specificity protein 1 (Sp1) and ultimately leads to the downregulation of MMP-14 gene expression (35). MMP-7 antisense oligonucleotides inhibit gene expression of MMP-7 and inhibit the metastasis of gastric and colon cancer by interfering with protein translation or promoting mRNA degradation (36). The modulation of gene expression differs in various physiological and pathophysiological events, such as Ets1 enhancing gene expression of MMP-1 through c-Jun (29, 30). Note that modulation of the MMP gene can be affected by several stimuli. Some factors, such as phorbol esters and ultraviolet B radiation, activate expression of the MMP gene, whereas others (37–39), such as transforming growth factor β (TGF-β), glucocorticoids and retinoic acid (40, 41), suppress it. In addition, the MMP gene may be induced indirectly by several signaling pathways. Inflammatory cytokines, for example, interleukin (IL)-1 and tumor necrosis factor, indirectly influence MMP gene expression and activate the ceramide signaling pathway. Three distinct MAP kinase pathways, p38, ERK1/2 and c-Jun N-terminal kinase (JNK) affect ceramide-dependent MMP-1 gene expression in human skin fibroblasts (42–45). MMPs genes are not generally upregulated by gene amplification or mutation like classical oncogenes. Usually transcriptional changes and/or epigenetic modifications result in an upregulation of MMP gene expression in colorectal cancer (CRC) (46, 47). Besides transcriptional regulation, MMP activity is related to proMMPs, which are secreted as inactive zymogens. Extracellular activation of proMMPs involves two steps. First, the N-terminal sequence of the propeptide domain is cleaved and releases the Zn2+-binding site stemming from catalytic domain exposition. Second, propeptide cleavage resulting in an active form of enzyme (21, 48, 49). For example, proMMP-9 was activated and then generated MMP-9, thus catalyzing angiogenesis via the FGF-2/FGFR-2 pathway (50). In addition, the activity of MMPs is regulated by α2-macroglobulin and tissue inhibitors of MMPs (TIMs), the two main endogenous inhibitors (26, 51). Thiol-modifying reagents, sodium dodecyl sulfate, and oxygen radicals also induce activation of MMPs in vitro(52).Variation in temperature or a decrease pH in the physicochemical environment serves the same purpose (25).
1.3 Immunological function of MMPs
The immune system, human beings’ main defense against disease, is indispensable. It eliminates foreign invaders through the immune response in a sophisticated and scientific way. Components that participate in immune regulation include innate immune cells, which act as early-responders, and adaptive immune cells, which enhance the response and generate immunological memory and molecules. Cytokines and chemokines control the immunoreaction in time and space. They take part in cell migration to the site of inflammation, proliferation, intercellular communication, and cell death (53, 54). The immune response does not necessarily lead to an inflammatory response, but inflammation accompanies the immune response in most cases (55). Inflammation commonly occurs after infection and damage (56). When antigens enter the body, macrophages or epithelial cells secrete chemokines, causing an increase in the vascular epithelial cell gap and vascular expansion; a large number of neutrophils, mast cells, basophils, and eosinophils infiltrate from the blood vessels into tissue fluid, resulting in localized febrile response, redness, swelling, and pain the cardinal signs of inflammation. An excessive immune response can lead to inflammation, such as pathogenic microbial infection, tumor, autoimmune disease, and tissue damage induced by physical or chemical elements. Immune cells and cytokines play an important role in the occurrence and resolution of inflammation. Numerous immune cells (e.g., macrophages, neutrophils) infiltrate the inflamed area and activated immune cells to release inflammatory factors (e.g., TNF-α, IL-6 and IL-1β), which worsens the inflammation (56, 57). Resolving this inflammatory response requires the release of anti-inflammatory cytokines (e.g., IL-10) by immune cells (58–61).
The composition of the tumor microenvironment is very complex. The tumor stroma is composed of the ECM, immune cells, fibroblasts, endothelial cells and other non‐neoplastic cells (62). MMPs produced in immune cells take part in innate and acquired immunity (63). Many immune cells express low levels of MMPs in the resting state. In mouse splenic CD4+ T cells, membrane-anchored disintegrin metalloproteinase-10 (ADAM-10) and ADAM-17 mRNAs are expressed highly, whereas the mRNA expressions of MMPs, such as MMP-2, MMP-9 and MMP-14 are low (64). Under normal conditions, when the expression of inflammatory cytokines and chemokines increases, MMPs of immune cells are secreted and activated. When stimulated by IL-8, TNF, or chemo-attractive formyl-Met-Leu-Phe peptide, MMP-9 in neutrophils is immediately released from gelatinase granules (also called tertiary granules) (65). In addition, TIMP1 protein is not expressed in neutrophils (66). However, B cells, T cells, and unstimulated human peripheral blood monocytes are able to express TIMPs in the steady state. The transcript levels of TIMP1, TIMP2 and TIMP4 are expressed more highly in monocytes than in B cells or T cells. In contrast, TIMP3 mRNA is highly expressed in B cells (67). ADAM17 and MMP-8 cleave the lymphotoxin (LT)-α1β2 heterotrimer, causing the release of heterotrimers from polarized T helper 1 (TH1) and TH17 cells (68). The combination of LTα1β2 heterotrimers and LTβ receptor activates primary synovial fibroblasts, eventually leading to synovial inflammation (68). The OX40 (a member of the TNF superfamily)–OX40 ligand axis is involved in numerous inflammatory diseases, anti-tumor immune responses and metabolic syndromes (69). MMP-2-specific CD4(+) T cells exist in tumor-infiltrating lymphocytes from melanoma patients, and they have an inflammatory T(H)2 (Type 2 helper T cells) profile. Dendritic cells (DCs) with MMP-2 initiates TH2 responses against several melanoma-associated antigens. As a reaction to exogenous melanoma antigens, active MMP-2 promotes TH2 cell differentiation, degrades the IF-α/β receptor in immature DCs and increases the protein expression of OX40 ligand in mature DCs. Therefore, researchers speculate that the mechanisms by which activated MMP-2 promotes tumor development is as follows: MMP-2 polarizes tumor-infiltrating lymphocytes toward a TH2 cell phenotype, which restrains the tumoricidal TH1-type response. Moreover, MMP-2 inhibits the powerful promoter of TH1 cell polarization-IL-12 subunit p35 (IL-12α) (70).
Important factors in building an immune response are efficient migration of neutrophils along a chemotactic gradient and extravasation through blood vessels and tissues to sites of infection. MMPs play a role in these processes by modifying chemotactic agents. A cleavage mediated by MMP-7 releases the heparan sulfate proteoglycan syndecan 1, and its associated CXC-chemokine ligand 3 (CXCL3), which attracts neutrophils to the site of infection (71). MMP‐8 which is mainly produced by neutrophils can be detected in the inflammatory response and some malignant tumors. In one study, there was a persistent inflammatory response after MMP-8-deficient mice were injected with methylcholanthrene. The incidence of skin tumors in male mice of this type increased significantly; female mice that were treated with tamoxifen or had their ovaries removed were more likely than wild-type mice to develop tumors. These results indicate that MMP-8 has a tumor suppressor function to some extent (72). This function is also supported by the finding that MMP-8 inhibits melanoma growth in vitro and in vivo (73). MMP-8 is necessary for recruiting chemokine CXCL6 to activate neutrophils; neutrophils are not able to migrate to sites of LPS administration without MMP-8 (74). IL-8 is a prototype chemokine that activates neutrophils. There is positive feedback between MMP-9 and IL-8. Stimulated by IL-8, neutrophils secrete gelatinase granules whose main component is MMP-9 (65). MMP-9 truncates an amino-terminal fragment of IL-8 for large increases in IL-8 potency (75). MMP‐2 cooperates with MMP‐9 to promote neutrophil infiltration (76, 77). MMP-2 and MMP-9 have synergistic effects on cleaving CXCL5 to increase neutrophil migration to the peritoneum during IL-1β-induced peritonitis (78). Meprins are members of the metzincin superfamily of zinc metalloproteinases, the cleaved substrate involved in many pathological processes, such as inflammation, cancer and fibrosis. Meprins participate in activating MMP-9 in the immune response. MMP-3 is an efficient activator of proMMP-9. The cleavage mediated by meprins improves the activation kinetics of proMMP-9 by MMP-3 (79). In contrast, MMP-2 may suppress the inflammatory response inactivating monocyte chemotactic protein 3 or Chemokine (C-C motif) ligand 7 (80).
Monocyte precursors are capable of differentiating into local macrophages in tissues (81). In different microenvironments, the cell surface phenotypes and functions of macrophage populations are heterogeneous (82). Macrophages play a prominent role in anti-infection and, anti-tumor activity and immune regulation (83). Similar to what happens in neutrophils, macrophages move directionally along the concentration gradient of certain chemicals and accumulate at the site of the lesion, where these substances are released (56). Metalloproteinases are able to affect macrophage recruitment (84–86). In mice with TIMP3-null mammary glands, the inflammatory response is exacerbated, the number of CD3+ T-cells increases, and macrophage infiltration is more pronounced than in wild-type mice glands (87). A classic means of activating macrophages (classically activated macrophages or M1 macrophages) is through Toll-like receptor ligands and pro-inflammatory mediators, such as TNF-α, interferon-γ (IFN-γ), and IL-1. Additionally, alternatively-activated macrophages, or M2 macrophages, can also be alternatively activated by distinct mediators, like IL-4 and IL-13 (88, 89). In inflammation, M1 macrophages effectively dispose of infectious organisms, and orchestrate angiogenesis and the ingress of connective tissue cells to form a granuloma. The function of MMPs ECM remodeling is vital in that process (90). In healing, M2 macrophages may promote connective tissue cells to remodel the ECM (88, 89). In vitro-differentiated M1 macrophages, mRNA expression of MMP-1, MMP-3, MMP-7, MMP-10, MMP-14 and MMP-25 are increased, and mRNA expression of TIMP-3 is decreased. mRNA expression of MMP-11, MMP-12, MMP-25 and TIMP-3 are upregulated, whereas MMP-2, MMP-8 and MMP -19 were reduced in M2 macrophages (91). Researchers speculate that the function of macrophages is related to the profile of MMP expression profile. The upregulation of MMP-12 in M2 macrophages is a major influence on the formation of aneurysms (92). Higher levels of MMP-1 collagenase may might be linked to higher collagenolytic activity of M1 macrophages (91). Macrophages also participate in specific immune response by releasing either pro- or anti-inflammatory cytokines (93, 94). MMP-14 (MT1-MMP) can control inflammatory gene responses. MMP-14-deficient macrophages produce excessive chemokine and cytokine responses to immune stimulation both in vitro and in vivo: they increase the gene and protein expression levels of the pro-inflammatory IL-12p40 (also called IL-12β) and IL-6, along with decrease the gene and protein levels of the anti-inflammatory IL-10. Phosphoinositide 3-kinase δ (PI3Kδ), a key regulator of macrophage immune responses, is the downstream transcriptional target of MMP-14 (95–97). Protein expression of MMP-14-dependent PI3Kδ evokes the expression and activation of a PI3Kδ/Akt/GSK3β signaling axis, thus mediating the immunoregulatory Mi-2/nucleosome remodeling and nucleosome remodeling and deacetylase to limit the expression of proinflammatory mediators in macrophage (97–100). MMP-12 originating from macrophages participated in abrogating the acute immune response. In MMP-12-deficient mice, leukocytes accumulated at the site of infection. MMP-12 cleaves and inactivates numerous CXC-chemokines and CC-chemokines which are implicated in the influx of leukocytes at the site of inflammation (101).
MMP-7 is involved in the immune activity of macrophages and neutrophils. One immunological function of MMP-7 is proteolytically activating α-defensins (cryptdins), which are a group of six cationic anti-bacterial peptides that work by disrupting bacterial membranes (102, 103). When stimulated by bacterial products, such as LPS and lipoteichoic acid, α-defensins are secreted from neutrophils, monocyte/macrophages and Paneth cells at the base of the crypts in the small intestine (104). α-defensins also act as the chemo-attractants for monocytes, T-cells and DCs to connect innate immunity to adaptive immunity (104). α-defensins are also mitogenic for epithelial cells and fibroblasts to aid in wound healing (105).
The cDNA sequence of MMP-25 from Japanese sea bass (Lateolabrax japonicus) (LjMMP25) regulates the production of inflammatory cytokines and promotes phagocytosis and bactericidal activity in monocytes/macrophages. Moreover, LjMMP25 regulates the inflammatory response by modulating NF-κB activity during innate immunity (106). Macrophages have a negative impact on cancer treatment (107–109). They create an inflammatory environment to promote tumorigenesis and tumor progression, such as angiogenesis, migration and invasion, and immunosuppression (109). For example, the penetration of cancer cells and leukocytes into the cerebral vessels is a complex multi-step process. The activity of macrophage-derived MMP-2 and MMP-9 is pivotal to leukocyte’s ability to penetrate the parenchymal basement membrane in mice with the ability autoimmune encephalomyelitis. These MMPs can be inhibited to protect the brain parenchyma from damage by preventing the infiltration of leukocytes (110). Moreover, the activation of TNF‐α by MMPs contributes to tumor progression (63, 111). The membrane-bound precursor, proTNF‐α, is mainly expressed in macrophages. ADAM17 (a TNF-converting enzyme) and MMPs, such as MMP-1, MMP-2, MMP-3, MMP-9, MMP-12, MMP-14, MMP-15 and MMP-17, convert proTNF-α into TNF‐α (112).
2 MMPs in pathological processes
2.1 Multifaceted role of MMPs in biological and pathological processes
Under normal physiological conditions, the activities of MMPs are controlled by various stimuli at multiple levels. However, under pathological conditions, this dynamic balance is broken. Over-degradation of the ECM due to overactivation of MMPs, is associated with a great many diseases, such as cardiovascular disease (4, 113–115), arthritis (116), periodontal diseases (117–119), osteogenesis imperfecta (120), disorder of the central nervous system (121), tumor invasion and metastasis (34, 122), age-related macular degeneration (123) and many other pathological states (7). Moreover, a decrease in MMPs can give rise to hypertensive pregnancy, preeclampsia (124), and inflammatory damage (125, 126).
As mentioned above, there are several main types of MMPs including collagenases, gelatinases, stromelysins, matrilysins, and MT-MMPs. Different MMPs have different three-dimensional structures, along with corresponding specific inhibitors or drugs. A typical MMPs consists of a prodomain, a propeptide, a catalytic domain and a hemophosphate domain. Approximately 80 amino acids make up the propeptide domain, and about 170 amino acids make up the catalytic metalloproteinase domain. The polypeptide folding of the MMP catalytic domain is basically superposition. The domain consists of a five-stranded β fold sheet, three α helices, and connecting rings. It contains two zinc ions and up to three stable calcium ions. The joint peptide contained in MMPs consists of a hinge region of variable length and a hemophosphate domain of about 200 amino acids. Exceptions include MMP-7, MMP-26, and MMP-23, which lack the hinge region and heme domain; MMP-23 has unique cysteine-rich domains and immunoglobulin domains (127–129). MMP-1, MMP-8, MMP-13, and MMP-18 are collagenases, and their key feature is their ability to cleave interstitial collagen I, II, and III at specific sites three-fourths away from the N-terminus. MMP-2 and MMP-9 are gelatin enzymes, which have three type II fiber-fiber-domain repeats in the catalytic domain and can bind to gelatin, collagen and laminin to digest denatured collagen. MMP-3 and MMP-10 are matrix lysins that digest ECM components (21). The classification of common MMPs implied in pathological processes is shown in Table 1. Collagenases recognize the substrate via a hemopexin-like domain, degrade fibrillar collagen and affect the ECM environment (19). Collagenases are closely related to the occurrence and development of diseases characterized by the degradation or change in the ECM are closely related to collagenase, including heart failure, atherosclerosis, cancer, arthritis, abdominal aortic aneurysm (130–132, 136, 137, 167, 168). In addition, they play a protective role in some diseases, for instance hypertrophic cardiomyopathy (134). Furthermore, collagenases improve liver fibrosis (133, 138). MMP-1 inhibits the development of atherosclerosis (130). MMP-8 plays a protective role in arthritis (135). Another category of MMPs is gelatinase. These MMPs act as digestive agents for components of the ECM, such as type I and IV collagen (19). They are induced or inhibited by a diverse range of resolvable factors, including growth factors, cytokines and hormones, and are acted on by cellular contacts through specific signaling pathways (169). MMP-9 has pro-inflammatory properties, whereas MMP-2 has pro-homeostatic properties (169, 170). Gelatinases have a profound influence in inflammatory process and tumor progression and have therefore long been considered one of the most significant anti-tumor targets (139, 140, 171). In terms of non-neoplasticity, gelatinases are mainly involved in cardiovascular pathology and auto-immune diseases (20). Moreover, MMP-9 is associated with many respiratory diseases (143). A reduction in vascular MMP-2 and MMP-9 gives rise to hypertensive pregnancy and preeclampsia (124). Stromelysins, another class of MMPs, have a structural domain arranged similarly to that of collagenases. However, these MMPs do not cleave fibrillar collagen type I (19). An important physiological function of stromelysins is to activate other members of the MMP family (21, 129). The most widely described pathological role of stromelysins is in cancer progression (144, 147–149). In addition, they function in the progression of cardiovascular, degenerative, and auto-immune diseases (145, 146, 172). Matrilysins, yet another category of MMPs, do not contain a hemopexin-like domain and are able to decompose collagen type IV but not type I (19). Matrilysins are associated with a number of pathological conditions in humans, mainly cancer and, respiratory, cardiovascular, and neurological diseases (20, 143, 151, 153, 154). A large number of studies have demonstrated that MMP-7 acts in the development and migration of cancer (151, 173). Moreover, MMP-7 also has a critical role in pathogenesis of tonsillitis and permanent hearing loss (150, 152). A study confirmed the early role of MMP-26 in the invasion and angiogenesis of malignant tumors (139). The final member of the MMPs family discussed here is MT-MMPs, which are an important mediator of infiltration. The influence of MT-MMPs on pathological process is mainly reflected in their promotion of tumor invasion (140, 155–161, 165, 166). The ability to activate MMP-2 is one of the reasons why most MT-MMPs play these roles (173). A different example is MMP-17, which has no regulatory effect on MMP-2 although it still affects tumor invasion (174). In addition, studies have demonstrated that MT-MMPs are also implicated in the pathological process of osteoarthritis, atherosclerosis and Alzheimer’s disease (85, 132, 162–164). MMPs are medicinal targets highly relevant to the treatment of a variety of diseases. As understanding of the role of MMPs in biology and pathology increases, greater understanding of the structural similarities and differences among MMP families makes it possible to discover highly selective MMP inhibitors.
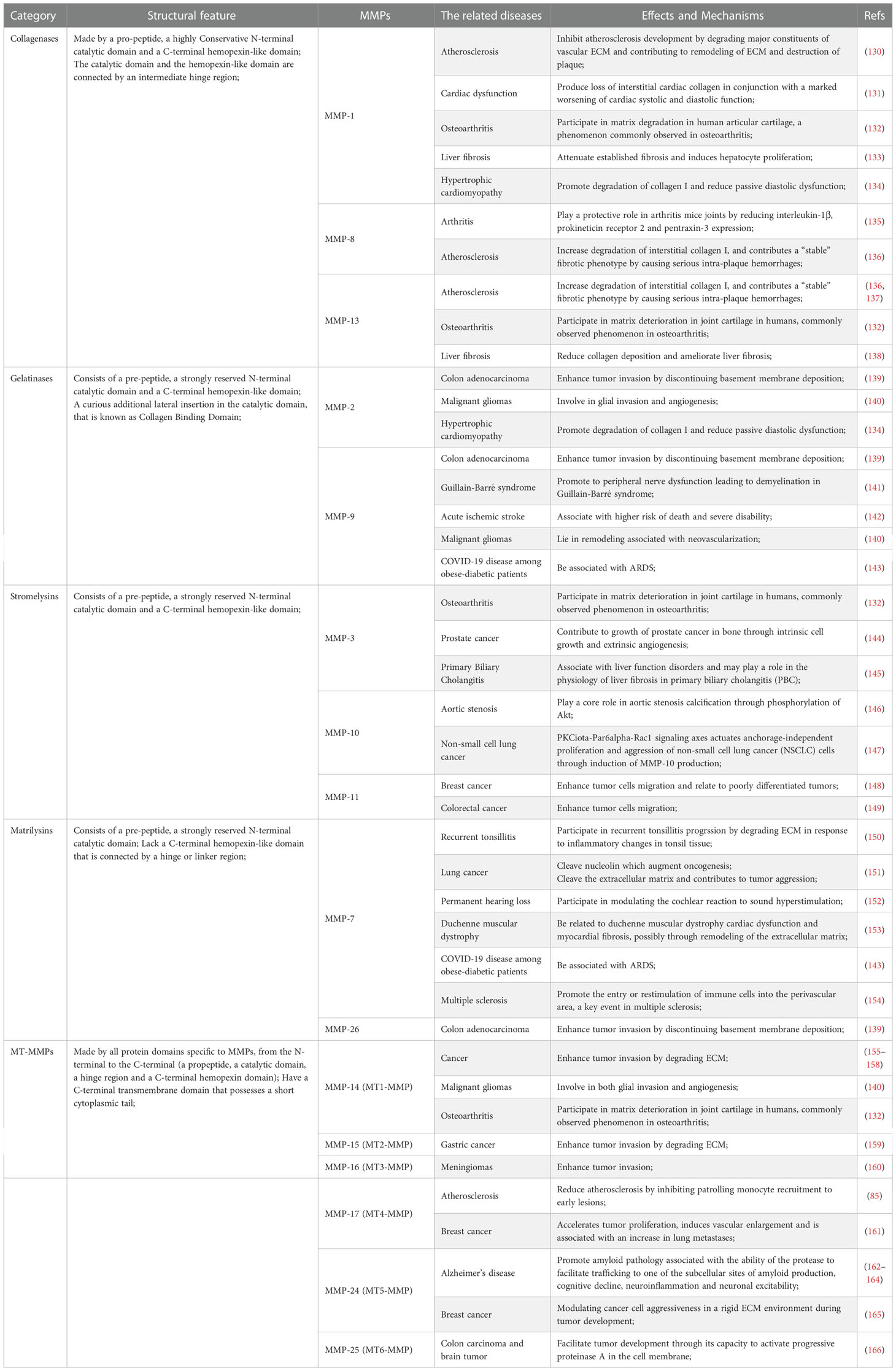
Table 1 The classification of common matrix metalloproteinases (MMPs) implied in pathological processes.
In the tumor microenvironment, the activity of a variety of MMPs, including MMP-1, MMP-2, MMP-3, MMP-7, MMP-8, MMP-9, MMP-10, MMP-11 and MMP-14, are up-regulated. These MMPs participate in tumor proliferation, survival, and angiogenesis, enabling replication immortality, invasion/migration, immunity evasion and other processes (175, 176). These MMPs control tumor cell growth by the releasing of ectodomains of growth factor, regulating the bioavailability of growth factors and regulating signaling pathways related to cell proliferation (177). MMP-3 and MMP-7 expression in tumor cells may contribute to an apoptosis resistant phenotype (178, 179). In addition, the MMP family is necessary for tumor angiogenesis via a two-way action, that is promoting or inhibiting angiogenesis. MMP-1, MMP-2, MMP-7, MMP-9 and MMP-14 regulate this process, and the first three of them play critical roles (180–182). Another key process in which MMPs are the migration of tumor cells. MMP-14 is among the key contributors to cancer invasion and promotes cancer development by activating proMMP-2 and degrading the ECM to promote cancer migration (176). MMP-7 acts in tumor cell metastasis by activating the ERK 1/2 and JNK 1/2 signaling pathways (183). MMP-1, MMP-2, MMP-8, MMP-11, and MMP-13 are implicated in the regulation of tumor cell migration (184–188). Finally, MMPs, such as MMP-14, also participate in tumor immune monitoring (176, 189, 190). Increasing attention has been paid to the role of MMPs in tumor immune regulation, such as their effects on inflammatory and immune responses, the tumor immune microenvironment and their diagnostic or prognostic potential (191–196).
2.2 Relationship between MMPs and immune-related diseases
MMPs affect the process of colitis. MMP-2 is causative for inflammatory bowel disease (IBD), which is derived from weak mRNA expression of pro-inflammatory cytokines including IFN-γ and TNF-α, and weak protein expression of IL-6 and less overgrowth of the colonic lumen by potentially pro-inflammatory enterobacteria from the commensal gut microbiota (197). MMP-9 plays a potentially key role in the progress of ulcerative colitis (UC) by regulating the immune system (198). MMP-19 coordinates the appropriate innate immune response in colitis, which is critical to balancing the host response to colon pathogens (126). MMP-9 is a member of MMPs closely related to cancer. MMP-9 is related to immune infiltration in pan-cancer and can be used as a biomarker of cancer prognosis and metastasis (199). It is overexpressed in peripheral blood NK cells of prostate cancer (86). And MMP-9 also effectively reduces the tumor killing-effect of T cells via cutting the MHC class I molecule, cell surface antigen-presenting complex molecules expressed in melanoma cells (200). In addition, high expression of MMP-11 is associated with worse survival rate in breast cancer, which is related to a low immune response, such as the reduction in the number of CD8+T cells, CD4+T cells, B cells and activated DCs (201).
2.3 Relationship between MMPs and inflammatory diseases
Inflammation is a fundamental pathological process that occurs when biological tissue is stimulated by certain kinds of injury, such as trauma and infection. Topical presentations of inflammation include redness, swelling, heat, pain and functional impairment. Systemic reactions include fever and changes in peripheral blood levels. MMPs are vital elements implicated in the manifold regulation of inflammation (202, 203). In one study, levels of some MMPs, such as MMP-1, MMP-2, MMP-3, MMP-7, MMP-9, MMP-10, MMP-12, and MMP-13, were significantly elevated in ulcer biopsies from patients with inflammatory disease (204–208). MMPs have not only a negative influence (125), but they also have an impact on vascular permeability (209–211), ECM remodeling, epithelial proliferation, and angiogenesis in different stages of inflammation (4, 173). In a model of colonic injury induced by sodium dextran sulfate, MMP-10 had a positive effect on disease (172). Because the progression of damage due to lack of MMP-10 is accelerated with viciousness-potential, enhancing expression of MMP-10 is helpful (125). A similar observation can be found for MMP-19 (126).
3 Key MMPs in CAC and their immunomodulatory aspects
3.1 Important role of MMPs in colitis
Chronic inflammatory disease is often associated with the occurrence and development of various cancers. A classic example is the increased risk for CAC in patients with IBD. In chronic environments marked by chronic inflammation, the ECM is a major factor in maintaining and promoting tumor growth, and MMPs are the major protease involved in the pathogenesis of IBD. Although both sporadic CRC and CAC are malignancies of the colon, CAC differs from sporadic colon cancer in several respects. CRC is produced through three main pathways: the adenomato-carcinoma sequence, the serrated pathway, and the inflammatory pathway. In contrast, the development of CAC is associated with the inflammatory-dysplasia-carcinoma pathway. MMPs counteract ECM proteins expressed in the gastrointestinal tract during inflammation (212, 213). Therefore, this study was conducted to evaluate the role of MMPs in CAC and its related mechanisms (214). The essential role of these enzymes in the remodeling and destruction of tissue in IBD has been well documented (205, 215–220). Pathological results of IBD, progressive mucosal disintegration (e.g., ulcers and fistulas) and fibrosis due to excessive deposition of collagen (the main component of ECM), is related to a disruption in the balance between composition and breakdown of the ECM (221). As an important molecule in mucosa and submucosa, ECM is the substrate of MMPs, which is why MMPs play such an essential role in the development of IBD. In Crohn’s disease (CD), TNF-α and activated T cells stimulate mesenchymal cells to increase the secretion of MMPs, and then MMPs causes tissue damage by degrading the lamina propria matrix (222, 223). MMP-3 and MMP-9 participate in the formation of fistula in CD by degrading the ECM (224). Moreover, MMPs are the key element in wound healing in the late stage of IBD through their effects on degradation of the ECM (225). MMP-1, MMP-7, and MMP-10 are expressed in migratory enterocytes in this process (226), which is important for epithelial regeneration and wound granulation (225, 227, 228). Furthermore, MMP-3 is crucial in scar contraction and ECM remodeling (229–231).
Regarding the ECM, MMPs have roles in a diverse array of substrates (232), including cytokines (90, 233), chemokines (234–237), TNF-α (238), α1-antitrypsin/α1-antichymotrypsin (239), IL-1β (240, 241), stromal cell-derived factor-1 (234), growth factors (239) and so forth. Some factors, such as TNF-α and IL-1β, in turn, stimulate the production of MMPs (242–244). Injury to the intestinal barrier is also responsible for IBD. When the intestinal barrier is disrupted, gene and protein expression of MMP-1, MMP-2, MMP-3, MMP-7, MMP-9, MMP-10 and MMP-13 increase, and leukocytes are summoned to inflamed areas (219, 245–247). MMP-8 and MMP-9 are released from neutrophils to regulate proinflammatory cytokines and chemokines to increase the number of leukocytes and eliminate bacteria (204, 224, 245). Macrophages phagocytose bacteria, along with MMP-9 released externally and MMP-12 entering into the phagosome (248). MMP-12 has a direct bactericidal effect. Briefly, when bacterial pathogens invade, MMP-12 is mobilized to macrophage phagolysosomes and adhere to bacterial cell walls, destroying cell membranes and causing bacterial death (249). MMP-7 plays an indirect bactericidal role by activating and releasing bactericidal alpha defensins into the gut lumen (250, 251). MMP-10 from infiltrating myeloid cells participates in the recovery of DSS-induced damage to the colon (125). Research has also shown that the susceptibility to colitis, including significant disease progression, increased mortality, severe tissue destruction, increases level of pro-inflammatory regulators in the colon and plasma, and a significant delay in neutrophil infiltration and persistent inflammation, increased markedly in MMP-19-null mice. In IBD, MMP-14 in endothelial cells promotes angiogenesis, which is achieved by combining the C-terminal fragment of MMP-14 substrate thrombospondin-1 with CD47/αvβ3 integrin to produce nitric oxide (252). Moreover, the migration of macrophages that lack MMP-19 is reduced in vivo and in vitro and the mucosal barrier is damaged (126). Chemokine fractalkine (CX3CL1), a substrate of MMP-19, is an essential component of the response to DSS in acute colitis. Because CX3CL1 receptors exist on innate immune cells (e.g., macrophages, neutrophils), impaired immune cell trafficking may be associated with a lack of the soluble CX3CL1 in MMP-19-deficient mice. Mice without the receptor CX3CR1 have more serious symptoms of DSS-induced colitis (126, 253–255). The application value of MMPs as biomarkers in IBD has also been recognized. A number of studies have demonstrated the high sensitivity of MMP-9 in evaluation of active UC (256–258). In addition, through an analysis of emerging BiomARKers (EMBARK), the researcher not only proposed that the combination of fecal calprotectin and serum MMP-9 can be used as a biomarker of UC, but also confirmed the value of MMP-9 as a biomarker of CD, indicating the combination of fecal calprotectin, serum MMP-9 and serum IL-22 can be used as a biomarker of CD (259).
In conclusion, MMPs participate in the host immune defense, would healing, and epithelial regeneration and they have bidirectional effects in IBD. On the one hand, they are involved in the development of IBD through the process of inflammation. MMPs are indirectly associated with progressive organ damage, ulceration or over accumulation of collagen, the persistence of inflammation, and fibrosis because of their substrate ECM. On the other hand, some members of MMP family have an inhibitory effect on inflammation (215, 260).
3.2 Key MMPs in CAC
CAC is a very common fatal complication of IBD (261–264). The pathogenesis of CAC is multifactorial, although a key driver of colitis is neoplastic progression (265–267). The lifetime risk for CAC in IBD patients is 15-40%, and CAC accounts for about 15% of mortality in these patients (268). Chronic inflammation generates oxidative stress that induces DNA damage that might activate some oncogenes and inactivate some anti-oncogenes (267). Related mechanisms include oxidative base lesions, replication stress, DNA crosslinking, and strand breaks, which eventually lead to genomic destabilization and tumorigenesis (269). MMPs play a roles in both promoting and inhibiting regulation of CAC development and progression, as shown in Table 2. The main role of MMPs in colitis and CAC is shown in Figure 2, and the network of MMPs that interfere with CAC is shown in Figure 3. A list of all genes mentioned here could be found in Table S1.
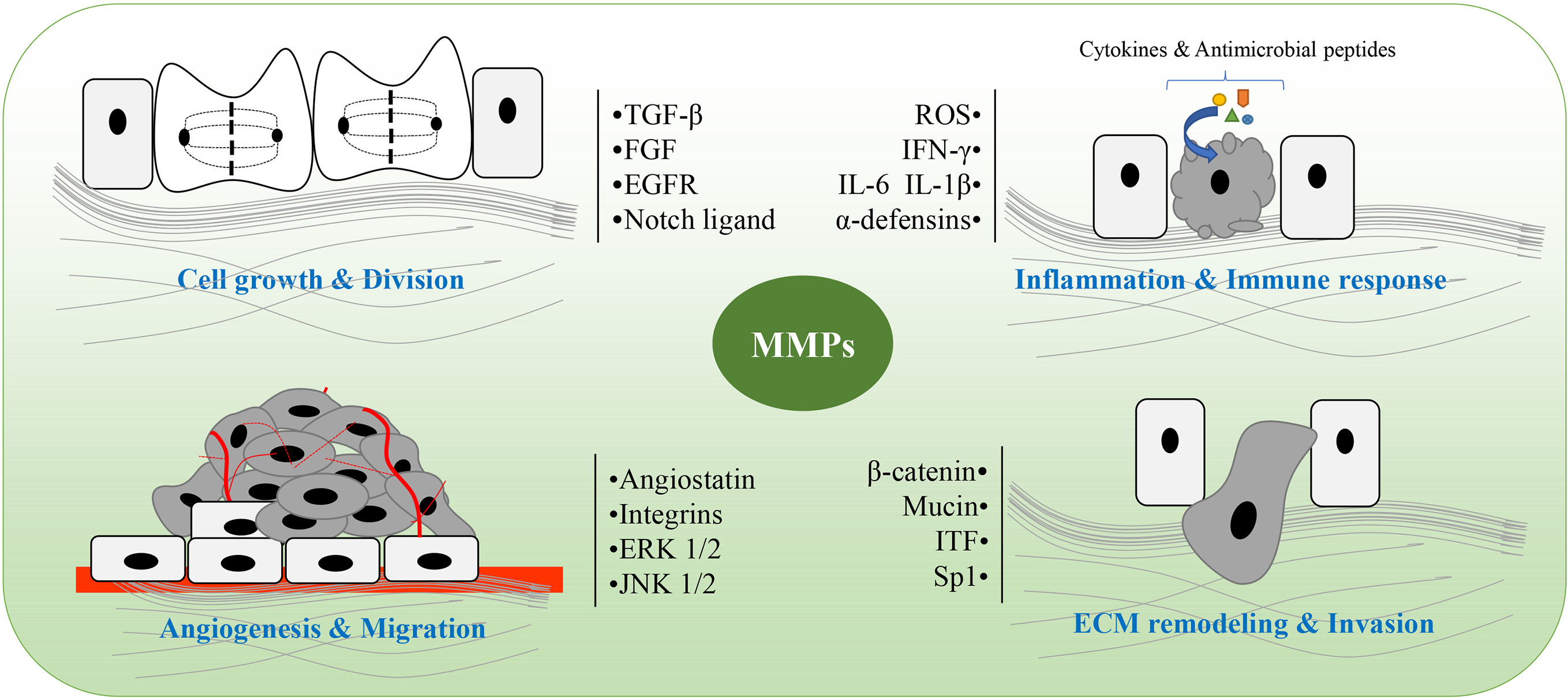
Figure 2 The main role of matrix metalloproteinases (MMPs) in colitis and colitis-associated cancers (CAC). MMPs are involved in pathological processes of colitis and CAC, including cell growth and division, angiogenesis and migration, ECM remodeling and invasion, as well as inflammation and immune response. ROS, reactive oxygen species; ITF, intestinal trefoil factor; Sp1, specificity protein 1; ECM, extracellular matrix.
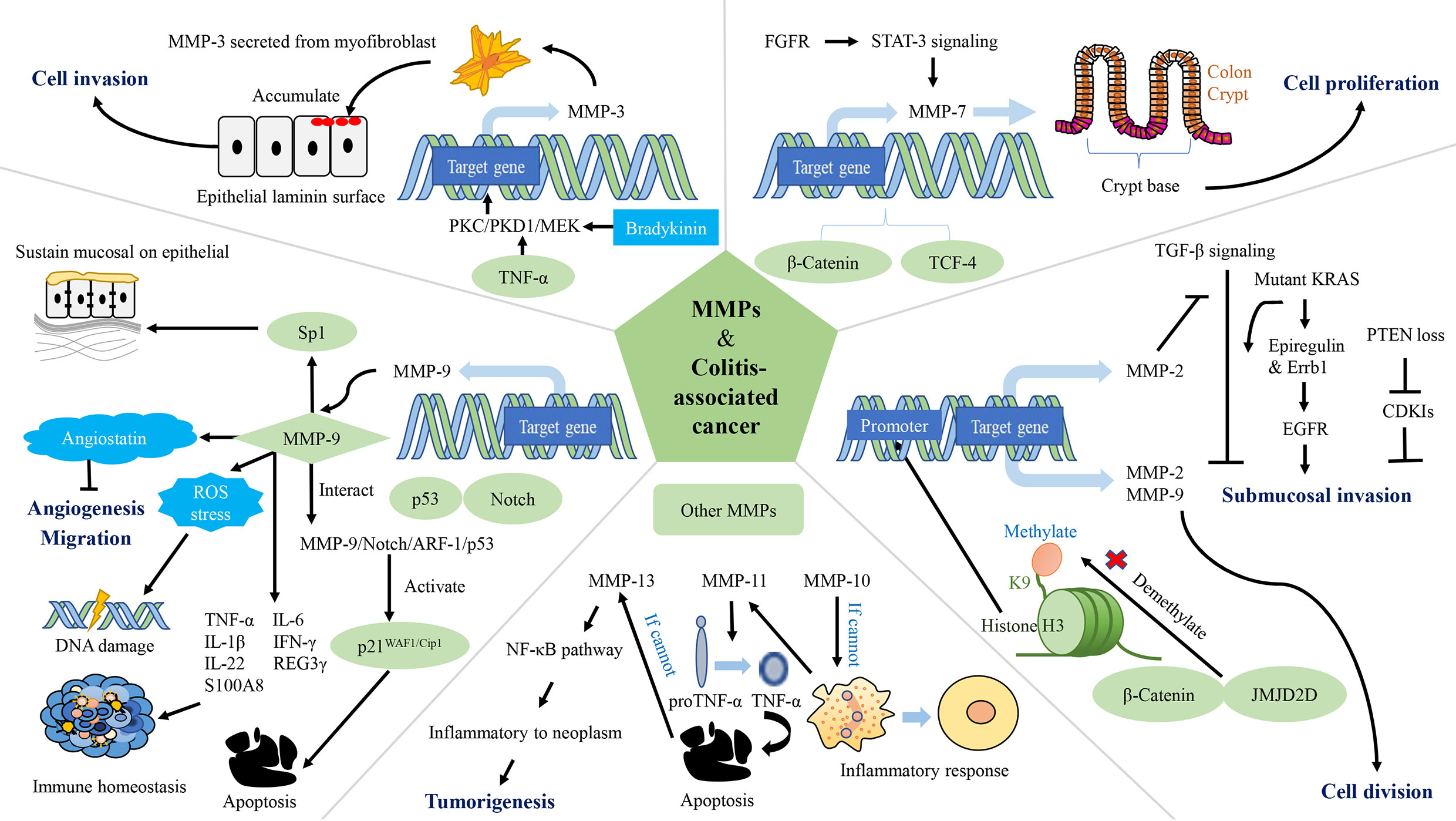
Figure 3 The network of matrix metalloproteinases (MMPs) interfering with colitis-associated cancers (CAC). MMP-2, MMP-3, MMP-7, MMP-9, MMP-10, MMP-11, and MMP-13 are involved in the regulation of CAC. MMP-2 and MMP-9 promote CRC cell proliferation. MMP-2 and MMP-3 contribute to tumor invasion. Expression of MMP-9 leads to apoptosis, reduced accumulation of ROS, DNA damage and inhibition of tumor vascularization, while maintaining epithelial mucosa, microbiota homeostasis and immune homeostasis. MMP-10 plays a role in inflammation regression. MMP-11 reduces apoptosis in cancer cells. PKC/PKD1/MEK: protein kinase C/protein kinase D1/mitogen-activated protein; TCF-4: T-cell factor-4; PTEN: phosphatase and tensin homolog deleted on chromosome 10; EGFR, epidermal growth factor receptor; FGFR, fibroblast growth factor receptors; CDK, cyclin dependent kinase; Sp1, specificity protein 1; ROS, reactive oxygen species.
MMPs degrade the protein components of the ECM and basement membranes, which provides a channel for cancer cells to invade to the vascular and lymphatic systems as well as promotes metastasis (276). In the process of tumor growth, MMPs are up-regulated, which strengthens the permeability of vascular endothelial cells, thereby increasing cell proliferation, migration and angiogenesis (287). Histone demethylase (JMJD2D) and β-catenin interacts physically (JMJD2D demethylates H3K9me3 on the promoter of β-catenin target genes), which increases the promoter activity of target genes (including MMP-2 and MMP-9) of β-catenin; activates transcription of MMP-2, MMP-9, and others; and ultimately cause CRC cells to proliferate, migrate and invade, and form colorectal tumors in mice (270). In a mouse model of tumor invasion, macrophages infiltrate and express MT1-MMP, resulting in activation of MMP-2 and consequent inhibition of TGF-β. This process leads to submucosal invasion of epithelial cells when it occurs in conjunction with KRAS or phosphatase and tensin homolog deleted on chromosome 10 (271). Specifically, when the inhibition of TGF-β is accompanied by the expression of KRAS, activation of the epidermal growth factor receptor (EGFR) signaling pathway is increased as a result of increased protein expression of epiregulin and mRNA expression of Errb1. When the inhibition of TGF-β is accompanied by phosphatase and tensin homolog deleted on chromosome 10 deletion, mRNA expressions of cyclin-dependent kinase (CDK) inhibitors (Cdkn2b/p15Ink4b, Cdkn1a/p21Cip1 and Cdkn1b/p27Kip1) is down-regulated (288, 289). The level of MMP-3 secreted from myofibroblasts is up-regulated in IBD and tumorigenesis (272, 273). TNF-α and bradykinin enhance expression of MMP-3 at a transcriptional level through the protein kinase C/protein kinase D1/mitogen-activated protein signaling pathway, and thus mediate CAC (272). This mediating effect is related to the promotion of tumor invasion by MMP-3 (274). MMP-7 is connected to the occurrence and progression of CAC, and is expressed intensely at crypt bases of epithelial cells and in dysplastic CAC biopsy, as observed in CRC (275). Because of the lack of adenomatosis polyposis coli, the WNT signaling pathway is deregulated and β-catenin and T-cell factor-4 accumulate. Hence, the expression of MMP-7 up-regulated (276, 277). In addition, fibroblast growth factor receptors in cancer-related fibroblasts activate Stat-3 signaling, thereby inducing MMP-7 expression (278). In contrast, the highly expressed MMP-9 in CAC inhibits the tumor by affecting the Notch signaling pathway. Specifically, MMP-9 activates p21WAF1/Cip1 by regulating notch activity, a key transcription factor in epithelial cell lineage, resulting in β-catenin inhibition and cell cycle arrest (213). MMP-9 from the colonic epithelium also acts as a tumor suppressor by activating the MMP-9-Notch1-ARF-p53 axis, which leads to apoptosis and DNA damage (279). Previous study claimed that epithelium-derived MMP-9 is beneficial for chronic inflammation, regardless of tissue origin, in contrast to neutrophil-derived MMP-9. They also proposed that MMP-9 (stemming from epithelium or neutrophils) is a pivotal regulator of acute IBD and sporadic cancers (279). MMP-9 reduces reactive oxygen species and DNA destruction in CAC as well (212). Some researchers have also found that the hemopexin domain of MMP-9 has an inhibitory effect on the metastasis and adhesion of CRC (280). The decrease in MMP-9 in plasma causes down-regulation of angiostatin synthesis, which results in tumor growth and vascularization (280, 281). MMP-9 expressed in the colonic epithelium maintains the microbiota balance. Antimicrobial peptides including REG3 and S100A families, are effective agents of the innate immune system (290–292). In transgenic mice constitutively expressing MMP-9 in the colonic epithelium mRNA levels of TNF-α, IL-6, IL-1β and IFN-γ increased, but mRNA levels of IL-22, REG3γ and S100A8 decreased. MMP-9 maintains epithelial and mucosal integrity by increasing mucin and intestinal trefoil factor protein levels and down-regulating the STAT3 pathway in vivo. Moreover, MMP-9 acts on the EGFR-nuclear transcription factor-Sp1 signaling pathway to sustain epithelial mucosa and functioning as well as immune homeostasis (282).
MMP-9 and MMP-10 are only significantly expressed in inflamed tissue, not normal colon tissue, and they start to peter out when healing begins (276). MMP-10 is mostly expressed by macrophages. In UC, it is found in enterocytes at the margins of ulcers and in the cells of granulation tissue (276). Researchers believe that MMP-10 from infiltrating bone marrow cells plays a role in resolving the inflammation. With a lack of MMP-10, susceptibility to DSS-induced colitis increases, and prolonged IBD may eventually lead to dysplasia (276). In miR-148/152-deficient mice, expressions of MMP-10 and MMP-13 increases, thus activating pro-TNF-α turning into TNF-α and promotes activation of the NF-κB signaling pathway. Damaged functioning of the intestinal barrier accelerates colitis and CAC (283). Similarly, MMP-11 is virtually absent in regular tissues (276). The mRNA level of MMP-11 is related to CAC in some way (284, 285). The mRNA level of MMP-11 is up-regulated in CAC, and is associated with the increase in the number of β-catenin accumulated crypts (284). The proton pump inhibitor-omerprazole and TNF-α blocker-infliximab reduce the mRNA level of MMP-11 and induces cells apoptosis in CAC (285). MMP-13 is highly increased in CAC colonic tissues, but do not change as the CAC progression (286). Compared to other MMPs, MMP-14 (MT1-MMP) does not increase markedly in CAC. Researchers have also found that Omerprazole and Infliximab were able to down-regulate the mRNA levels of MMP-14 (284). In a mouse model of CAC, miR-128, miR-134 and miR-330 are influenced by Dicer1. These microRNAs inhibit tumor growth in vitro and in vivo and modulate expression of MMP-3, MMP-10, and MMP-13 (285, 293).
3.3 Mechanisms underlying of typical MMPs in CAC
In CAC, typical MMPs affecting the organism’s immune function and their expressions are regulated by the immune system, as shown in Figure 4 (10). MMP-7 decreases the sensitivity of mice to intestinal bacteria. Specifically, MMP-7 knockout mice do not activate pro-a-defensins in the gut to their mature active forms, with the result that these mice are highly susceptible to intestinal bacterial infection (250). MMP-8 affects the immune response to tumor and helps to resolve necrosis, which is positively related to the degree of primary tumor necrosis and blood neutrophil count, as well as negatively correlated with destructive inflammatory infiltration and Crohn’s-like lymphoid reaction (294). MMP-8, which is involved in resolving acute and chronic inflammation and helps to recruit neutrophils during acute inflammation, is mainly produced by neutrophils (295, 296). It plays a role in the recruitment of neutrophils to necrotic areas and in tissue remodeling, including collagen breakdown (294). MMP-9 is associated with the onset of lymphadenitis in patients with CAC, and is significantly up-regulated before the onset of lymphadenitis in these patients (297). In addition, MMP-9 maintains the integrity of epithelial mucosa and acts as a tumor suppressor in CAC, which is inseparable from its function of mediating the level of proinflammatory cytokines (282). The linings of gastrointestinal epithelial mucosa act as an external physical barrier and a functional immune barrier for an immune monitoring system (298). The imbalance in immune cells is crucial to the development of cancer (299). The inflammatory cytokines released by immune cells function in immune defense, and promote the development of cancer in specific circumstances (300, 301). MMP-9 increases mRNA levels of IL-6, IL-1β, TNF-α and IFN-γ, but decreases the mRNA level of IL-22 (282). Regarding the regulation of MMPs by the immune system, elevated MMP-8 is associated with systemic inflammation and increased secretion of various cytokines, including IL-1ra, IL-7 and IL-8, and is negatively associated with the number of tumors infiltrating mast cells (302). In a mouse model of colitis-associated CRC, the NF-κB mediated inflammatory reaction promotes protein expression of cyclin D1, phosphorylated ribosomal protein S6 and MMP-9 in the colon tissues of these mice, which plays a beneficial role in CRC progression (303). In the mouse model of CAC, MMP-9 expression is associated with excessive angiogenesis and cell proliferation, which is related to CXCL2 and neutrophil recruitment (304). CXCR2 is present in neutrophils and interacts with CXCL2 (305). This interaction promotes the recruitment of neutrophil and the synthesis of MMP-8 and MMP-9 (304, 306, 307). The proinflammatory factors IL-17 and IL-21 increase the MMPs secreted by human intestinal fibroblasts, including MMP-1, MMP-2, MMP-3 and MMP-9 (308–310). Among them, the inducing effect of IL-17 on MMP-1 and MMP-3 depends on the rapid activation of mitogen-activated protein kinase (308, 311). The regulation of MMPs by IL-21 does not occur at the level of transcription and translation and stimulating fibroblast with IL-21 does not increase the intracellular level of MMP RNA transcripts and proteins. The up-regulation of MMPs by IL-21 may depend on preferentially increasing the secretion of preconstituted or newly synthesized MMPs (309). In addition, expression of MMPs is regulated by TNF-α and IFN-γ (312).
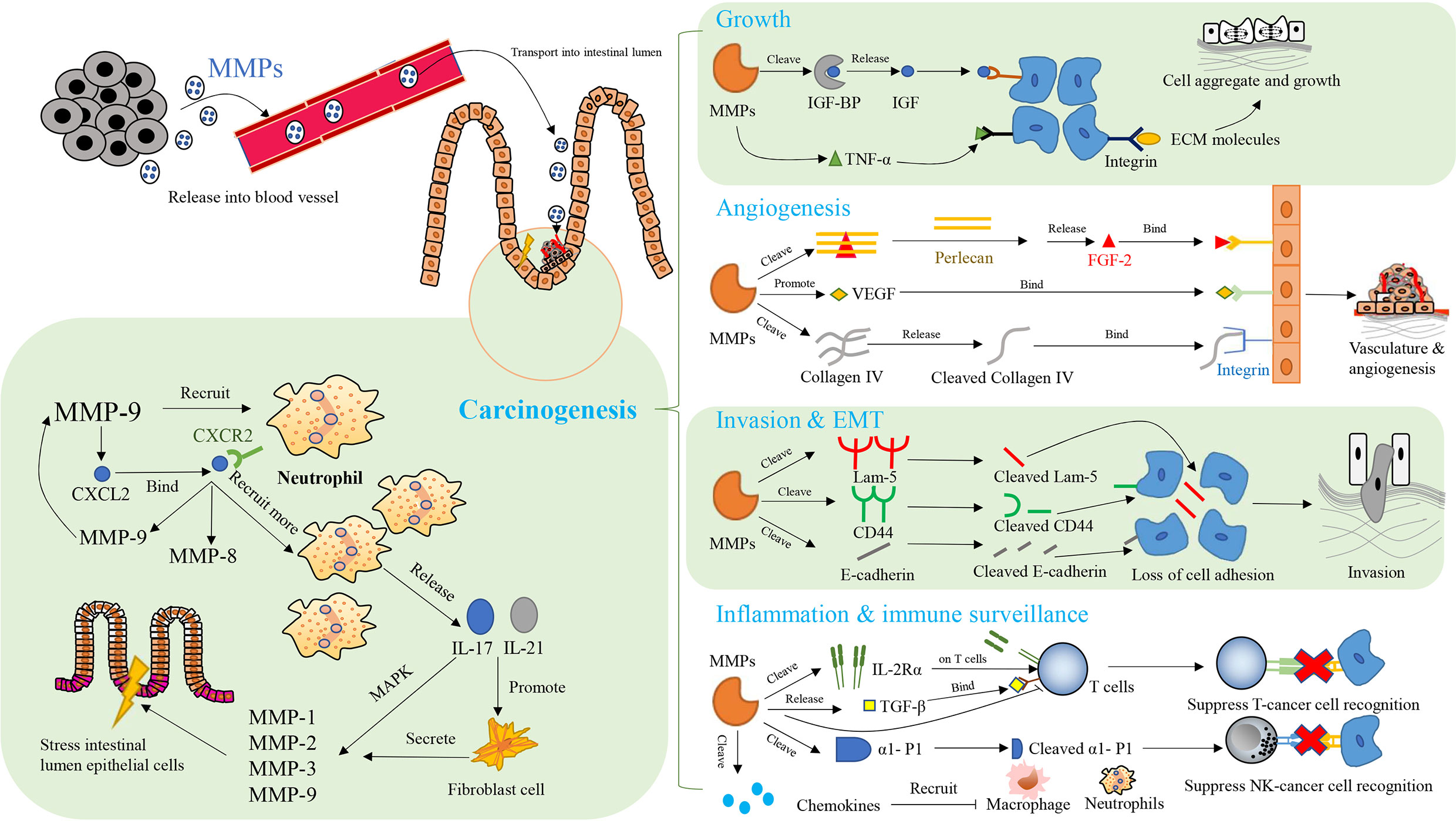
Figure 4 The network of MMPs and therapeutic targets in colitis-associated cancers (CAC). Key aspects of carcinogenesis are mediated by MMPs, including cell growth, angiogenesis, invasion, epithelial-mesenchymal transition (EMT), inflammation, and immune surveillance in immunomodulatory manner. CXCL2: CXC-chemokine ligand 2; CXCR 2: CXC chemokine receptor 2; MAPK, mitogen-activated protein kinases; FGF-2, fibroblast growth factor 2; IGF, insulin-like growth factors; IGF-BP, insulin-like growth factors binding protein; TNF-β, tumor necrosis factor-β; TNF-α, tumor necrosis factor-α; ECM, extracellular matrix; Lam-5, laminin-5; CD44, cluster of differentiation-44; VEGF, vascular endothelial-derived growth factor; α1-P1, alpha 1-proteinase inhibitor;IL-17, interleukin-17; IL-21, interleukin-21; IL-2Rα, interleukin-2 receptor alpha.
3.4 Potential clinical applications of MMP inhibitors
Clinical trials of MMPs mainly focus on three factors. They are respectively the changes in clinical levels of MMPs in different disease states, the clinical use of MMP inhibitors in colitis and colorectal cancer, and combining MMPs and some regulatory factors with other drugs to control inflammation and tumors. MMP-2, MMP-3, MMP-7 and MMP-9 are the key MMPS in this process. However, current clinical trials have shown that inhibiting MMPs has no obvious effect on tumor responses, although it has a certain role in stabilizing the condition of diseases.
Many MMPs are upregulated in IBD. These MMPs remodel tissue and release several small protein fragments. In a clinical trial with 164 volunteers, these protein fragments could be used to distinguish between CD and UC. For example, measuring segments of vimentin (MMP-2 and MMP-8 decomposed and citrullinated-vimentin [VICM]) and type III (MMP-9 decomposed collagen type III [C3M]) can distinguish between CD and UC (313). A total of 138 participants took part in an IBD-related study, including different types of disease. Fecal MMP-9 can be used to diagnose and differentiate between UC and pouchitis, because it is strongly associated with clinical, histological, and endoscopic activities of different forms of IBD (257). A clinical trial evaluated the relationship between MMP and prognosis in CRC. This study enrolled 198 consecutive patients who had undergone operation for CRC, (85 females and 113 males). Of the patient, 67% were older than 65 years old, and their Tumor-Node-Metastasis classification ranged from 1 to 4. Expression of MMPs was higher in tumor tissue than in normal mucosa. This result indicates that high expression of MMP-2 and MMP-9 in the mucosa of CRC patients is related to poorer 5-year survival rates (314). MMP-7 is implicated in multiple processes of tumor development. To estimate the contribution of serum MMP-7 to the prognosis of resected CRC, researchers have conducted several clinical trials. In a study with 303 CRC patients (87 healthy controls, 96 nonmetastatic patients and 120 advanced patients), high serum MMP-7 was associated with a higher risk of death in terminal CRC patients (315). Included in another study were 175 curatively resected CRC patients. In two Cox proportional hazard models (overall survival and disease-free survival), higher MMP-7 was associated with higher recurrence and faster progression (316). Given the role of MMP-3 in cancer progression and metastasis, a study with 73 CRC patients who underwent minimally invasive colorectal resection investigated the relationship between increased plasma MMP-3 and residual metastases after surgery. Minimally invasive surgery directly up-regulated MMP-3 levels owing to surgery or subsequent wound healing or indirectly up-regulated MMP-3 by increasing TNF-α and IL-1 in the acute inflammatory response after surgery (317).
BAY 12-9566 inhibits MMP-2, MMP-3, and MMP-9. A phase I clinical enrolled 13 patients with colorectal, renal, gastroesophageal junction, duodenum, lung, and sarcoma cancer. Subjects were given BAY 12-9566 at four dosages. No tumor responses were found, but two patients had stable disease after 1.1 and 1.5 years of treatment (318). In another phase I clinical trial, 27 patients with advanced solid tumors took BAY 12-9566 100 to 1,600 mg/day. These patients had colorectal, lung, breast, ovarian and cervical cancers. The condition of 48% patients was stable. BAY 12-9566 did not reduce the size of the tumor, but slowed their growth (319). BMS-275291 is another wide-spectrum inhibitor of MMPs. In an open-label, phase I trial, 40 late-stage or metastatic cancer patients were given BMS-275291, most of them had CRC or non-small cell lung cancer. Although the researchers found no objective tumor responses, the condition of some patients stabilized (320).
Two clinical trials have been conducted on drug combinations. In a randomized, double-blind, clinical trial of rectal cancer, 34 patients receiving chemoradiotherapy were divided into a placebo group and a conjugated linoleic acid group. Supplementing conjugated linoleic acid decreased the levels of TNF-α, IL-1β, hsCRP, MMP-2 and MMP-9, which are biomarkers of tumor aggression and angiogenesis (321). A trial that included 37 patients with CRC lasted for 7 weeks. These patients who underwent chemotherapy, were separated into two groups: a fisetin group (n=18) and a placebo group (n=19). Flavonoid fisetin reduced levels of MMP-7, and significantly lowered levels of high-sensitivity C-reactive protein and IL-8 by the end of the study (322).
Despite the important role of MMPs in many human diseases, no broad-spectrum synthetic MMP inhibitor has successfully passed the clinical trial stage because of the bilateral pro-tumor and anti-tumorigenic effects of MMPs in cancer (323). A variety of MMPs, including MMP-2, MMP-9 and MMP-14, can degrade the basal layer of capillaries and promote exosmosis of tumor cells. MMP-9 also down-regulates the IL receptor on the surface of T cells, further inhibiting immunity and promoting cancer tolerance (324–326). By eliminating cell apoptosis, MMP-7 reduces the effect of chemotherapy even promoting tumor growth. However, MMP-8 may directly inhibit tumor metastasis in tumor cells. One of the side effects of broad-spectrum MMP inhibitors is that they interfere with the tumor-inhibiting function of MMP-8 (76). With more specific MMPs inhibitors now available, MMPs targeting can be reconsidered for cancer therapy (326).
4 Conclusion
Given their role in degrading the ECM, MMPs are associated with the occurrence and development of many diseases, especially inflammatory diseases. Most MMPs, such as MMP-2, MMP-3, MMP-7, MMP-9, MMP-10, MMP-12, and MMP-13 are increased in colitis and CAC. Therefore, reducing levels of these MMPs could effectively prevent the development of inflammation and CAC, as well as the progression of colitis-the eventual cause of CAC- from acute inflammation to chronic. However, the effect of some MMPs, like MMP-9, on CAC is bidirectional, which means they are involved in the pathogenesis of IBD and promote the metastasis and spread of malignant tumors, but also play a role in tumor suppression as well. Therefore, how to balance the bidirectional role of MMPs in clinical applications is a vital question. In specific diseases, it might be advisable to clarify the therapeutic target, especially the definitive role and efficacy of a certain MMP. Given their multifaceted role in colitis and CAC, more in-depth research is needed. In addition, MMPs participate in the host immune defense, wound healing, and epithelial regeneration. Normally MMPs are secreted and activated in immune cells when the expression of inflammatory cytokines and chemokines increase. MMPs modulate immune system activity by interfering with the differentiation and immune activity of immune cells, recruitment of macrophages, and migration of neutrophils. In clinical trials, the condition of CRC patients could be stabilized to a certain extent by inhibiting levels of MMPs. Therefore, levels of MMPs could be used to predict the condition and development of inflammatory diseases and CAC. Furthermore, MMPs have very broad prospects in the treatment of CAC through immunoregulation, which is also a promising direction in future research.
Author contributions
LH and QK wrote and revised this manuscript. KC constructed figures in manuscript and helped to revise it. YZ helped to revise the manuscript and provided valuable feedback to this conception. ZZ revised the entire manuscript and edited the language for scientific presentation. The corresponding authors, ZZ and WT conceived and organized this study. All authors contributed to the article and approved the submitted version.
Funding
This study was supported by the Young Scientists Fund of the National Natural Science Fund (81903844), the Gansu Province Science Fund for Distinguished Young Scholars (20JR10RA650), and the Outstanding Youth of the Fundamental Research Funds for the Central Universities (lzujbky-2021-ey21). This study was also supported by the Macao Science and Technology Development Fund (FDCT 007/2020/ALC and 0006/2020/AKP), Natural Science Foundation of Guangdong Province, China (2020A1515010922), Traditional Chinese Medicine Bureau of Guangdong Province, China (20201183), Shenzhen–Hong Kong-Macau S&T Program (Category C) (SGDX2020110309420200), and Research Fund of the University of Macau (CPG2023-00028-ICMS).
Conflict of interest
The authors declare that the research was conducted in the absence of any commercial or financial relationships that could be construed as a potential conflict of interest.
Publisher’s note
All claims expressed in this article are solely those of the authors and do not necessarily represent those of their affiliated organizations, or those of the publisher, the editors and the reviewers. Any product that may be evaluated in this article, or claim that may be made by its manufacturer, is not guaranteed or endorsed by the publisher.
Supplementary material
The Supplementary Material for this article can be found online at: https://www.frontiersin.org/articles/10.3389/fimmu.2022.1093990/full#supplementary-material
References
1. Gross J, Lapiere CM. Collagenolytic activity in amphibian tissues: A tissue culture assay. Proc Natl Acad Sci U.S.A. (1962) 48(6):1014–22. doi: 10.1073/pnas.48.6.1014
2. Obiso RJ, Azghani AO, Wilkins TD. The bacteroides fragilis toxin fragilysin disrupts the paracellular barrier of epithelial cells. Infect Immun (1997) 65(4):1431–9. doi: 10.1128/iai.65.4.1431-1439.1997
3. Saito S, Trovato MJ, You R, Lal BK, Fasehun F, Padberg FT, et al. Role of matrix metalloproteinases 1, 2, and 9 and tissue inhibitor of matrix metalloproteinase-1 in chronic venous insufficiency. J Vasc Surg (2001) 34(5):930–8. doi: 10.1067/mva.2001.119503
4. Wang X, Khalil RA. Matrix metalloproteinases, vascular remodeling, and vascular disease. Adv Pharmacol (2018) 81:241–330. doi: 10.1016/bs.apha.2017.08.002
5. Madzharova E, Kastl P, Sabino F, Auf dem Keller U. Post-translational modification-dependent activity of matrix metalloproteinases. Int J Mol Sci (2019) 20(12):3077. doi: 10.3390/ijms20123077
6. Mott JD, Werb Z. Regulation of matrix biology by matrix metalloproteinases. Curr Opin Cell Biol (2004) 16(5):558–64. doi: 10.1016/j.ceb.2004.07.010
7. Vargová V, Pytliak M, Mechírová V. Matrix metalloproteinases. Exp Suppl (2012) 103:1–33. doi: 10.1007/978-3-0348-0364-9_1
8. Clark IM, Swingler TE, Sampieri CL, Edwards DR. The regulation of matrix metalloproteinases and their inhibitors. Int J Biochem Cell Biol (2008) 40(6-7):1362–78. doi: 10.1016/j.biocel.2007.12.006
9. Sternlicht MD, Werb Z. How matrix metalloproteinases regulate cell behavior. Annu Rev Cell Dev Biol (2001) 17:463–516. doi: 10.1146/annurev.cellbio.17.1.463
10. Egeblad M, Werb Z. New functions for the matrix metalloproteinases in cancer progression. Nat Rev Cancer (2002) 2(3):161–74. doi: 10.1038/nrc745
11. Mallya SK, Mookhtiar KA, Gao Y, Brew K, Dioszegi M, Birkedal-Hansen H, et al. Characterization of 58-kilodalton human neutrophil collagenase: Comparison with human fibroblast collagenase. Biochemistry (1990) 29(47):10628–34. doi: 10.1021/bi00499a008
12. Lovejoy B, Welch AR, Carr S, Luong C, Broka C, Hendricks RT, et al. Crystal structures of MMP-1 and -13 reveal the structural basis for selectivity of collagenase inhibitors. Nat Struct Biol (1999) 6(3):217–21. doi: 10.1038/6657
13. Fridman R, Fuerst TR, Bird RE, Hoyhtya M, Oelkuct M, Kraus S, et al. Domain structure of human 72-kDa gelatinase/type IV collagenase. Characterization of proteolytic activity and identification of the tissue inhibitor of metalloproteinase-2 (TIMP-2) binding regions. J Biol Chem (1992) 267(22):15398–405. doi: 10.1016/S0022-5193(05)80617-4
14. Kaur K, Zhu K, Whittemore MS, Petersen RL, Lichte A, Tschesche H, et al. Identification of the active site of gelatinase B as the structural element sufficient for converting a protein to a metalloprotease. Biochemistry (2002) 41(15):4789–97. doi: 10.1021/bi015930p
15. Becker JW, Marcy AI, Rokosz LL, Axel MG, Burbaum JJ, Fitzgerald PM, et al. Stromelysin-1: Three-dimensional structure of the inhibited catalytic domain and of the c-truncated proenzyme. Protein Sci (1995) 4(10):1966–76. doi: 10.1002/pro.5560041002
16. Pavlovsky AG, Williams MG, Ye QZ, Ortwine DF, Purchase CF, White AD, et al. X-Ray structure of human stromelysin catalytic domain complexed with nonpeptide inhibitors: Implications for inhibitor selectivity. Protein Sci (1999) 8(7):1455–62. doi: 10.1110/ps.8.7.1455
17. Ganea E, Trifan M, Laslo AC, Putina G, Cristescu C. Matrix metalloproteinases: Useful and deleterious. Biochem Soc Trans (2007) 35(Pt 4):689–91. doi: 10.1042/BST0350689
18. Fernandez-Catalan C, Bode W, Huber R, Turk D, Calvete JJ, Lichte A, et al. Crystal structure of the complex formed by the membrane type 1-matrix metalloproteinase with the tissue inhibitor of metalloproteinases-2, the soluble progelatinase a receptor. EMBO J (1998) 17(17):5238–48. doi: 10.1093/emboj/17.17.5238
19. PittaYAPruek P, Meephansan J, Prapapan O, Komine M, Ohtsuki M. Role of matrix metalloproteinases in photoaging and photocarcinogenesis. Int J Mol Sci (2016) 17(6):868. doi: 10.3390/ijms17060868
20. Sbardella D, Fasciglione GF, Gioia M, Ciaccio C, Tundo GR, Marini S, et al. Human matrix metalloproteinases: An ubiquitarian class of enzymes involved in several pathological processes. Mol Aspects Med (2012) 33(2):119–208. doi: 10.1016/j.mam.2011.10.015
21. Visse R, Nagase H. Matrix metalloproteinases and tissue inhibitors of metalloproteinases: Structure, function, and biochemistry. Circ Res (2003) 92(8):827–39. doi: 10.1161/01.RES.0000070112.80711.3D
22. Marchenko GN, Strongin AY. MMP-28, a new human matrix metalloproteinase with an unusual cysteine-switch sequence is widely expressed in tumors. Gene (2001) 265(1-2):87–93. doi: 10.1016/s0378-1119(01)00360-2
23. Brinckerhoff CE, Matrisian LM. Matrix metalloproteinases: A tail of a frog that became a prince. Nat Rev Mol Cell Biol (2002) 3(3):207–14. doi: 10.1038/nrm763
24. Fischer T, Riedl R. Challenges with matrix metalloproteinase inhibition and future drug discovery avenues. Expert Opin Drug Discovery (2021) 16(1):75–88. doi: 10.1080/17460441.2020.1819235
25. Nagase H, Woessner JF. Matrix metalloproteinases. J Biol Chem (1999) 274(31):21491–4. doi: 10.1074/jbc.274.31.21491
26. Gresele P, Falcinelli E, Sebastiano M, Momi S. Matrix metalloproteinases and platelet function. Prog Mol Biol Transl Sci (2017) 147:133–65. doi: 10.1016/bs.pmbts.2017.01.002
27. Vincenti MP, Brinckerhoff CE. Signal transduction and cell-type specific regulation of matrix metalloproteinase gene expression: Can MMPs be good for you? J Cell Physiol (2007) 213(2):355–64. doi: 10.1002/jcp.21208
28. Yan J, Erdem H, Li R, Cai Y, Ayala G, Ittmann M, et al. Steroid receptor coactivator-3/AIB1 promotes cell migration and invasiveness through focal adhesion turnover and matrix metalloproteinase expression. Cancer Res (2008) 68(13):5460–8. doi: 10.1158/0008-5472.CAN-08-0955
29. Tallant C, Marrero A, Gomis-Rüth FX. Matrix metalloproteinases: Fold and function of their catalytic domains. Biochim Biophys Acta (2010) 1803(1):20–8. doi: 10.1016/j.bbamcr.2009.04.003
30. Westermarck J, Seth A, Kähäri VM. Differential regulation of interstitial collagenase (MMP-1) gene expression by ETS transcription factors. Oncogene (1997) 14(22):2651–60. doi: 10.1038/sj.onc.1201111
31. Vincenti MP, Brinckerhoff CE. Transcriptional regulation of collagenase (MMP-1, MMP-13) genes in arthritis: Integration of complex signaling pathways for the recruitment of gene-specific transcription factors. Arthritis Res (2002) 4(3):157–64. doi: 10.1186/ar401
32. Hnia K, Gayraud J, Hugon G, Ramonatxo M, de la Porte S, Matecki S, et al. L-arginine decreases inflammation and modulates the nuclear factor-κB/Matrix metalloproteinase cascade in Mdx muscle fibers. Am J Pathol (2008) 172(6):1509–19. doi: 10.2353/ajpath.2008.071009
33. Wang Z, Kong D, Banerjee S, Li Y, Adsay NV, Abbruzzese J, et al. Down-regulation of platelet-derived growth factor-D inhibits cell growth and angiogenesis through inactivation of notch-1 and nuclear factor-κB signaling. Cancer Res (2007) 67(23):11377–85. doi: 10.1158/0008-5472.CAN-07-2803
34. Alaseem A, Alhazzani K, Dondapati P, Alobid S, Bishayee A, Rathinavelu A. Matrix metalloproteinases: A challenging paradigm of cancer management. Semin Cancer Biol (2019) 56:100–15. doi: 10.1016/j.semcancer.2017.11.008
35. Takenaga K, Ochiya T, Endo H. Inhibition of the invasion and metastasis of mammary carcinoma cells by NBD peptide targeting S100A4 via the suppression of the Sp1/MMP-14 axis. Int J Oncol (2021) 58(3):397–408. doi: 10.3892/ijo.2021.5173
36. Yan C, Boyd DD. Regulation of matrix metalloproteinase gene expression. J Cell Physiol (2007) 211(1):19–26. doi: 10.1002/jcp.20948
37. Auble DT, Brinckerhoff CE. The AP-1 sequence is necessary but not sufficient for phorbol induction of collagenase in fibroblasts. Biochemistry (1991) 30(18):4629–35. doi: 10.1021/bi00232a039
38. Brenneisen P, Wenk J, Klotz LO, Wlaschek M, Briviba K, Krieg T, et al. Central role of Ferrous/Ferric iron in the ultraviolet B irradiation-mediated signaling pathway leading to increased interstitial collagenase (Matrix-degrading metalloprotease (MMP)-1) and stromelysin-1 (MMP-3) mRNA levels in cultured human dermal fibroblasts. J Biol Chem (1998) 273(9):5279–87. doi: 10.1074/jbc.273.9.5279
39. Fisher GJ, Datta SC, Talwar HS, Wang ZQ, Varani J, Kang S, et al. Molecular basis of sun-induced premature skin ageing and retinoid antagonism. Nature (1996) 379(6563):335–9. doi: 10.1038/379335a0
40. Duivenvoorden WC, Hirte HW, Singh G. Transforming growth factor Beta1 acts as an inducer of matrix metalloproteinase expression and activity in human bone-metastasizing cancer cells. Clin Exp Metastasis (1999) 17(1):27–34. doi: 10.1023/a:1026404227624
41. Wada K, Sato H, Kinoh H, Kajita M, Yamamoto H, Seiki M. Cloning of three caenorhabditis elegans genes potentially encoding novel matrix metalloproteinases. Gene (1998) 211(1):57–62. doi: 10.1016/s0378-1119(98)00076-6
42. Korzus E, Nagase H, Rydell R, Travis J. The mitogen-activated protein kinase and JAK-STAT signaling pathways are required for an oncostatin M-responsive element-mediated activation of matrix metalloproteinase 1 gene expression. J Biol Chem (1997) 272(2):1188–96. doi: 10.1074/jbc.272.2.1188
43. Reunanen N, Westermarck J, Häkkinen L, Holmström TH, Elo I, Eriksson JE, et al. Enhancement of fibroblast collagenase (Matrix metalloproteinase-1) gene expression by ceramide is mediated by extracellular signal-regulated and stress-activated protein kinase pathways. J Biol Chem (1998) 273(9):5137–45. doi: 10.1074/jbc.273.9.5137
44. Spiegel S, Foster D, Kolesnick R. Signal transduction through lipid second messengers. Curr Opin Cell Biol (1996) 8(2):159–67. doi: 10.1016/s0955-0674(96)80061-5
45. Zitka O, Kukacka J, Krizkova S, Huska D, Adam V, Masarik M, et al. Matrix metalloproteinases. Curr Med Chem (2010) 17(31):3751–68. doi: 10.2174/092986710793213724
46. Sizemore ST, Sizemore GM, Booth CN, Thompson CL, Silverman P, Bebek G, et al. Hypomethylation of the MMP-7 promoter and increased expression of MMP-7 distinguishes the basal-like breast cancer subtype from other triple-negative tumors. Breast Cancer Res Treat (2014) 146(1):25–40. doi: 10.1007/s10549-014-2989-4
47. Chicoine E, Estève P-O, Robledo O, Van Themsche C, Potworowski EF, St-Pierre Y. Evidence for the role of promoter methylation in the regulation of MMP-9 gene expression. Biochem Biophys Res Commun (2002) 297(4):765–72. doi: 10.1016/S0006-291X(02)02283-0
48. Springman EB, Angleton EL, Birkedal-Hansen H, Van Wart HE. Multiple modes of activation of latent human fibroblast collagenase: Evidence for the role of a Cys73 active-site zinc complex in latency and a "Cysteine switch" mechanism for activation. Proc Natl Acad Sci U.S.A. (1990) 87(1):364–8. doi: 10.1073/pnas.87.1.364
49. Van Wart HE, Birkedal-Hansen H. The cysteine switch: A principle of regulation of metalloproteinase activity with potential applicability to the entire matrix metalloproteinase gene family. Proc Natl Acad Sci U.S.A. (1990) 87(14):5578–82. doi: 10.1073/pnas.87.14.5578
50. Ardi VC, Van den Steen PE, Opdenakker G, Schweighofer B, Deryugina EI, Quigley JP. Neutrophil MMP-9 proenzyme, unencumbered by TIMP-1, undergoes efficient activation in vivo and catalytically induces angiogenesis via a basic fibroblast growth factor (FGF-2)/FGFR-2 pathway. J Biol Chem (2009) 284(38):25854–66. doi: 10.1074/jbc.M109.033472
51. Stetler-Stevenson WG. Tissue inhibitors of metalloproteinases in cell signaling: Metalloproteinase-independent biological activities. Sci Signal (2008) 1(27):re6. doi: 10.1126/scisignal.127re6
52. Lijnen HR, Lupu F, Moons L, Carmeliet P, Goulding D, Collen D. Temporal and topographic matrix metalloproteinase expression after vascular injury in mice. Thromb Haemost (1999) 81(5):799–807. doi: 10.1055/s-0037-1614573
53. Khokha R, Murthy A, Weiss A. Metalloproteinases and their natural inhibitors in inflammation and immunity. Nat Rev Immunol (2013) 13(9):649–65. doi: 10.1038/nri3499
55. Kaur BP, Secord E. Innate immunity. Pediatr Clin North Am (2019) 66(5):905–11. doi: 10.1016/j.pcl.2019.06.011
56. Sun L, Wang X, Saredy J, Yuan Z, Yang X, Wang H. Innate-adaptive immunity interplay and redox regulation in immune response. Redox Biol (2020) 37:101759. doi: 10.1016/j.redox.2020.101759
57. Arabpour M, Saghazadeh A, Rezaei N. Anti-inflammatory and M2 macrophage polarization-promoting effect of mesenchymal stem cell-derived exosomes. Int Immunopharmacol (2021) 97:107823. doi: 10.1016/j.intimp.2021.107823
58. Tomar N, De RK. A brief outline of the immune system. Methods Mol Biol (2014) 1184:3–12. doi: 10.1007/978-1-4939-1115-8_1
59. Saraiva M, O'Garra A. The regulation of IL-10 production by immune cells. Nat Rev Immunol (2010) 10(3):170–81. doi: 10.1038/nri2711
60. Liew PX, Kubes P. The neutrophil's role during health and disease. Physiol Rev (2019) 99(2):1223–48. doi: 10.1152/physrev.00012.2018
61. Shaw DM, Merien F, Braakhuis A, Dulson D. T-Cells and their cytokine production: The anti-inflammatory and immunosuppressive effects of strenuous exercise. Cytokine (2018) 104:136–42. doi: 10.1016/j.cyto.2017.10.001
62. Karta J, Bossicard Y, Kotzamanis K, Dolznig H, Letellier E. Mapping the metabolic networks of tumor cells and cancer-associated fibroblasts. Cells (2021) 10(2):304. doi: 10.3390/cells10020304
63. Shimoda M, Ohtsuka T, Okada Y, Kanai Y. Stromal metalloproteinases: Crucial contributors to the tumor microenvironment. Pathol Int (2021) 71(1):1–14. doi: 10.1111/pin.13033
64. Murthy A, Shao YW, Defamie V, Wedeles C, Smookler D, Khokha R. Stromal TIMP3 regulates liver lymphocyte populations and provides protection against Th1 T cell-driven autoimmune hepatitis. J Immunol (2012) 188(6):2876–83. doi: 10.4049/jimmunol.1102199
65. Masure S, Proost P, Van Damme J, Opdenakker G. Purification and identification of 91-kDa neutrophil gelatinase. release by the activating peptide interleukin-8. Eur J Biochem (1991) 198(2):391–8. doi: 10.1111/j.1432-1033.1991.tb16027.x
66. Opdenakker G, Van den Steen PE, Dubois B, Nelissen I, Van Coillie E, Masure S, et al. Gelatinase B functions as regulator and effector in leukocyte biology. J Leukoc Biol (2001) 69(6):851–9. doi: 10.1189/jlb.69.6.851
67. Bar-Or A, Nuttall RK, Duddy M, Alter A, Kim HJ, Ifergan I, et al. Analyses of all matrix metalloproteinase members in leukocytes emphasize monocytes as major inflammatory mediators in multiple sclerosis. Brain (2003) 126(Pt 12):2738–49. doi: 10.1093/brain/awg285
68. Young J, Yu X, Wolslegel K, Nguyen A, Kung C, Chiang E, et al. Lymphotoxin-alphabeta heterotrimers are cleaved by metalloproteinases and contribute to synovitis in rheumatoid arthritis. Cytokine (2010) 51(1):78–86. doi: 10.1016/j.cyto.2010.03.003
69. Summers deLuca L, Gommerman JL. Fine-tuning of dendritic cell biology by the TNF superfamily. Nat Rev Immunol (2012) 12(5):339–51. doi: 10.1038/nri3193
70. Godefroy E, Manches O, Dréno B, Hochman T, Rolnitzky L, Labarrière N, et al. Matrix metalloproteinase-2 conditions human dendritic cells to prime inflammatory T(H)2 cells via an IL-12- and OX40L-dependent pathway. Cancer Cell (2011) 19(3):333–46. doi: 10.1016/j.ccr.2011.01.037
71. Li Q, Park PW, Wilson CL, Parks WC. Matrilysin shedding of syndecan-1 regulates chemokine mobilization and transepithelial efflux of neutrophils in acute lung injury. Cell (2002) 111(5):635–46. doi: 10.1016/s0092-8674(02)01079-6
72. Balbín M, Fueyo A, Tester AM, Pendás AM, Pitiot AS, Astudillo A, et al. Loss of collagenase-2 confers increased skin tumor susceptibility to Male mice. Nat Genet (2003) 35(3):252–7. doi: 10.1038/ng1249
73. Palavalli LH, Prickett TD, Wunderlich JR, Wei X, Burrell AS, Porter-Gill P, et al. Analysis of the matrix metalloproteinase family reveals that MMP8 is often mutated in melanoma. Nat Genet (2009) 41(5):518–20. doi: 10.1038/ng.340
74. Tester AM, Cox JH, Connor AR, Starr AE, Dean RA, Puente XS, et al. Lps responsiveness and neutrophil chemotaxis in vivo require PMN MMP-8 activity. PLos One (2007) 2(3):e312. doi: 10.1371/journal.pone.0000312
75. Van den Steen PE, Proost P, Wuyts A, Van Damme J, Opdenakker G. Neutrophil gelatinase B potentiates interleukin-8 tenfold by aminoterminal processing, whereas it degrades CTAP-III, PF-4, and GRO-alpha and leaves RANTES and MCP-2 intact. Blood (2000) 96(8):2673–81. doi: 10.1182/blood.V96.8.2673
76. Kessenbrock K, Plaks V, Werb Z. Matrix metalloproteinases: Regulators of the tumor microenvironment. Cell (2010) 141(1):52–67. doi: 10.1016/j.cell.2010.03.015
77. Chou J, Chan MF, Werb Z. Metalloproteinases: A functional pathway for myeloid cells. Microbiol Spectr (2016) 4(2):MCHD-0002-2015. doi: 10.1128/microbiolspec.MCHD-0002-2015
78. Song J, Wu C, Zhang X, Sorokin LM. In vivo processing of CXCL5 (LIX) by matrix metalloproteinase (MMP)-2 and MMP-9 promotes early neutrophil recruitment in IL-1β-induced peritonitis. J Immunol (2013) 190(1):401–10. doi: 10.4049/jimmunol.1202286
79. Geurts N, Becker-Pauly C, Martens E, Proost P, Van den Steen PE, Stöcker W, et al. Meprins process matrix metalloproteinase-9 (MMP-9)/gelatinase B and enhance the activation kinetics by MMP-3. FEBS Lett (2012) 586(24):4264–9. doi: 10.1016/j.febslet.2012.10.033
80. McQuibban GA, Gong JH, Tam EM, McCulloch CA, Clark-Lewis I, Overall CM. Inflammation dampened by gelatinase a cleavage of monocyte chemoattractant protein-3. Science (2000) 289(5482):1202–6. doi: 10.1126/science.289.5482.1202
81. Zheng MH, Fan Y, Smith A, Wysocki S, Papadimitriou JM, Wood DJ. Gene expression of monocyte chemoattractant protein-1 in giant cell tumors of bone osteoclastoma: Possible involvement in CD68+ macrophage-like cell migration. J Cell Biochem (1998) 70(1):121–9. doi: 10.1002/(SICI)1097-4644(19980701)70:1<121::AID-JCB12>3.0.CO;2-S
82. Eshghjoo S, Kim DM, Jayaraman A, Sun Y, Alaniz RC. Macrophage polarization in atherosclerosis. Genes (2022) 13(5):756. doi: 10.3390/genes13050756
83. Yu Q, Wang Y, Dong L, He Y, Liu R, Yang Q, et al. Regulations of glycolytic activities on macrophages functions in tumor and infectious inflammation. Front Cell Infection Microbiol (2020) 10:287. doi: 10.3389/fcimb.2020.00287
84. Hingorani DV, Lippert CN, Crisp JL, Savariar EN, Hasselmann JPC, Kuo C, et al. Impact of MMP-2 and MMP-9 enzyme activity on wound healing, tumor growth and RACPP cleavage. PLos One (2018) 13(9):e0198464. doi: 10.1371/journal.pone.0198464
85. Clemente C, Rius C, Alonso-Herranz L, Martín-Alonso M, Pollán Á, Camafeita E, et al. MT4-MMP deficiency increases patrolling monocyte recruitment to early lesions and accelerates atherosclerosis. Nat Commun (2018) 9(1):910. doi: 10.1038/s41467-018-03351-4
86. Gallazzi M, Baci D, Mortara L, Bosi A, Buono G, Naselli A, et al. Prostate cancer peripheral blood NK cells show enhanced CD9, CD49a, CXCR4, CXCL8, MMP-9 production and secrete monocyte-recruiting and polarizing factors. Front Immunol (2020) 11:586126. doi: 10.3389/fimmu.2020.586126
87. Hojilla CV, Jackson HW, Khokha R. TIMP3 regulates mammary epithelial apoptosis with immune cell recruitment through differential TNF dependence. PLos One (2011) 6(10):e26718. doi: 10.1371/journal.pone.0026718
88. Gordon S, Taylor PR. Monocyte and macrophage heterogeneity. Nat Rev Immunol (2005) 5(12):953–64. doi: 10.1038/nri1733
89. Martinez FO, Helming L, Gordon S. Alternative activation of macrophages: An immunologic functional perspective. Annu Rev Immunol (2009) 27:451–83. doi: 10.1146/annurev.immunol.021908.132532
90. Parks WC, Wilson CL, López-Boado YS. Matrix metalloproteinases as modulators of inflammation and innate immunity. Nat Rev Immunol (2004) 4(8):617–29. doi: 10.1038/nri1418
91. Huang W-C, Sala-Newby GB, Susana A, Johnson JL, Newby AC. Classical macrophage activation up-regulates several matrix metalloproteinases through mitogen activated protein kinases and nuclear factor-κB. PLos One (2012) 7(8):e42507. doi: 10.1371/journal.pone.0042507
92. Shimizu K, Shichiri M, Libby P, Lee RT, Mitchell RN. Th2-predominant inflammation and blockade of IFN-gamma signaling induce aneurysms in allografted aortas. J Clin Invest (2004) 114(2):300–8. doi: 10.1172/JCI19855
93. Mosser DM, Edwards JP. Exploring the full spectrum of macrophage activation. Nat Rev Immunol (2008) 8(12):958–69. doi: 10.1038/nri2448
94. Nathan C, Ding A. Nonresolving inflammation. Cell (2010) 140(6):871–82. doi: 10.1016/j.cell.2010.02.029
95. Fukao T, Koyasu S. PI3K and negative regulation of TLR signaling. Trends Immunol (2003) 24(7):358–63. doi: 10.1016/s1471-4906(03)00139-x
96. Beurel E, Michalek SM, Jope RS. Innate and adaptive immune responses regulated by glycogen synthase kinase-3 (GSK3). Trends Immunol (2010) 31(1):24–31. doi: 10.1016/j.it.2009.09.007
97. Shimizu-Hirota R, Xiong W, Baxter BT, Kunkel SL, Maillard I, Chen X-W, et al. MT1-MMP regulates the PI3Kδ·Mi-2/NuRD-dependent control of macrophage immune function. Genes Dev (2012) 26(4):395–413. doi: 10.1101/gad.178749.111
98. Ramirez-Carrozzi VR, Nazarian AA, Li CC, Gore SL, Sridharan R, Imbalzano AN, et al. Selective and antagonistic functions of SWI/SNF and Mi-2beta nucleosome remodeling complexes during an inflammatory response. Genes Dev (2006) 20(3):282–96. doi: 10.1101/gad.1383206
99. Lai D, Wan M, Wu J, Preston-Hurlburt P, Kushwaha R, Grundström T, et al. Induction of TLR4-target genes entails calcium/calmodulin-dependent regulation of chromatin remodeling. Proc Natl Acad Sci U.S.A. (2009) 106(4):1169–74. doi: 10.1073/pnas.0811274106
100. Zhou D, Collins CA, Wu P, Brown EJ. Protein tyrosine phosphatase shp-1 positively regulates TLR-induced IL-12p40 production in macrophages through inhibition of phosphatidylinositol 3-kinase. J Leukoc Biol (2010) 87(5):845–55. doi: 10.1189/jlb.0409289
101. Dean RA, Cox JH, Bellac CL, Doucet A, Starr AE, Overall CM. Macrophage-specific metalloelastase (MMP-12) truncates and inactivates ELR+ CXC chemokines and generates CCL2, -7, -8, and -13 antagonists: Potential role of the macrophage in terminating polymorphonuclear leukocyte influx. Blood (2008) 112(8):3455–64. doi: 10.1182/blood-2007-12-129080
102. Ganz T. Defensins and host defense. Science (1999) 286(5439):420–1. doi: 10.1126/science.286.5439.420
103. Yang D, Chertov O, Oppenheim JJ. Participation of mammalian defensins and cathelicidins in anti-microbial immunity: Receptors and activities of human defensins and cathelicidin (LL-37). J Leukoc Biol (2001) 69(5):691–7. doi: 10.1189/jlb.69.5.691
104. Ayabe T, Satchell DP, Wilson CL, Parks WC, Selsted ME, Ouellette AJ. Secretion of microbicidal alpha-defensins by intestinal paneth cells in response to bacteria. Nat Immunol (2000) 1(2):113–8. doi: 10.1038/77783
105. Murphy CJ, Foster BA, Mannis MJ, Selsted ME, Reid TW. Defensins are mitogenic for epithelial cells and fibroblasts. J Cell Physiol (1993) 155(2):408–13. doi: 10.1002/jcp.1041550223
106. Chen S-Y, Zhan X-L, Jiang R, Dai Y-W, Lu J-F, Yang G-J, et al. Matrix metalloproteinase-25 from Japanese Sea bass (Lateolabrax japonicus) is involved in pro-inflammatory responses. Dev Comp Immunol (2022) 129:104348. doi: 10.1016/j.dci.2022.104348
107. Bingle L, Brown NJ, Lewis CE. The role of tumour-associated macrophages in tumour progression: Implications for new anticancer therapies. J Pathol (2002) 196(3):254–65. doi: 10.1002/path.1027
108. Shima T, Shimoda M, Shigenobu T, Ohtsuka T, Nishimura T, Emoto K, et al. Infiltration of tumor-associated macrophages is involved in tumor programmed death-ligand 1 expression in early lung adenocarcinoma. Cancer Sci (2020) 111(2):727–38. doi: 10.1111/cas.14272
109. Qian B-Z, Pollard JW. Macrophage diversity enhances tumor progression and metastasis. Cell (2010) 141(1):39–51. doi: 10.1016/j.cell.2010.03.014
110. Agrawal S, Anderson P, Durbeej M, van Rooijen N, Ivars F, Opdenakker G, et al. Dystroglycan is selectively cleaved at the parenchymal basement membrane at sites of leukocyte extravasation in experimental autoimmune encephalomyelitis. J Exp Med (2006) 203(4):1007–19. doi: 10.1084/jem.20051342
111. Balkwill F. TNF-alpha in promotion and progression of cancer. Cancer Metastasis Rev (2006) 25(3):409–16. doi: 10.1007/s10555-006-9005-3
112. Manicone AM, McGuire JK. Matrix metalloproteinases as modulators of inflammation. Semin Cell Dev Biol (2008) 19(1):34–41. doi: 10.1016/j.semcdb.2007.07.003
113. Lenti M, Falcinelli E, Pompili M, de Rango P, Conti V, Guglielmini G, et al. Matrix metalloproteinase-2 of human carotid atherosclerotic plaques promotes platelet activation. correlation with ischaemic events. Thromb Haemost (2014) 111(6):1089–101. doi: 10.1160/TH13-07-0588
114. Schmidt R, Bültmann A, Ungerer M, Joghetaei N, Bülbül O, Thieme S, et al. Extracellular matrix metalloproteinase inducer regulates matrix metalloproteinase activity in cardiovascular cells: Implications in acute myocardial infarction. Circulation (2006) 113(6):834–41. doi: 10.1161/CIRCULATIONAHA.105.568162
115. Major TC, Liang L, Lu X, Rosebury W, Bocan TMA. Extracellular matrix metalloproteinase inducer (EMMPRIN) is induced upon monocyte differentiation and is expressed in human atheroma. Arterioscler Thromb Vasc Biol (2002) 22(7):1200–7. doi: 10.1161/01.atv.0000021411.53577.1c
117. Overall CM, Wiebkin OW, Thonard JC. Demonstration of tissue collagenase activity in vivo and its relationship to inflammation severity in human gingiva. J Periodontal Res (1987) 22(2):81–8. doi: 10.1111/j.1600-0765.1987.tb01544.x
118. Checchi V, Maravic T, Bellini P, Generali L, Consolo U, Breschi L, et al. The role of matrix metalloproteinases in periodontal disease. Int J Environ Res Public Health (2020) 17(14):4923. doi: 10.3390/ijerph17144923
119. Malemud CJ. Matrix metalloproteinases and synovial joint pathology. Prog Mol Biol Transl Sci (2017) 148:305–25. doi: 10.1016/bs.pmbts.2017.03.003
120. Arai Y, Choi B, Kim BJ, Park S, Park H, Moon JJ, et al. Cryptic ligand on collagen matrix unveiled by MMP13 accelerates bone tissue regeneration via MMP13/Integrin α3/RUNX2 feedback loop. Acta Biomater (2021) 125:219–30. doi: 10.1016/j.actbio.2021.02.042
121. Zipfel P, Rochais C, Baranger K, Rivera S, Dallemagne P. Matrix metalloproteinases as new targets in Alzheimer’s disease: opportunities and challenges. J Med Chem (2020) 63(19):10705–25. doi: 10.1021/acs.jmedchem.0c00352
122. Isaacson KJ, Martin Jensen M, Subrahmanyam NB, Ghandehari H. Matrix-metalloproteinases as targets for controlled delivery in cancer: An analysis of upregulation and expression. J Control Release (2017) 259:62–75. doi: 10.1016/j.jconrel.2017.01.034
123. García-Onrubia L, Valentín-Bravo FJ, Coco-Martin RM, González-Sarmiento R, Pastor JC, Usategui-Martín R, et al. Matrix metalloproteinases in age-related macular degeneration (AMD). Int J Mol Sci (2020) 21(16):5934. doi: 10.3390/ijms21165934
124. Chen J, Khalil RA. Matrix metalloproteinases in normal pregnancy and preeclampsia. Prog Mol Biol Transl Sci (2017) 148:87–165. doi: 10.1016/bs.pmbts.2017.04.001
125. Koller FL, Dozier EA, Nam KT, Swee M, Birkland TP, Parks WC, et al. Lack of MMP10 exacerbates experimental colitis and promotes development of inflammation-associated colonic dysplasia. Lab Invest (2012) 92(12):1749–59. doi: 10.1038/labinvest.2012.141
126. Brauer R, Tureckova J, Kanchev I, Khoylou M, Skarda J, Prochazka J, et al. MMP-19 deficiency causes aggravation of colitis due to defects in innate immune cell function. Mucosal Immunol (2016) 9(4):974–85. doi: 10.1038/mi.2015.117
127. Jabłońska-Trypuć A, Matejczyk M, Rosochacki S. Matrix metalloproteinases (MMPs), the main extracellular matrix (ECM) enzymes in collagen degradation, as a target for anticancer drugs. J Enzyme Inhibition Medicinal Chem (2016) 31(sup1):177–83. doi: 10.3109/14756366.2016.1161620
128. Fischer T, Senn N, Riedl R. Design and structural evolution of matrix metalloproteinase inhibitors. Chemistry (2019) 25(34):7960–80. doi: 10.1002/chem.201805361
129. Nagase H, Visse R, Murphy G. Structure and function of matrix metalloproteinases and TIMPs. Cardiovasc Res (2006) 69(3):562–73. doi: 10.1016/j.cardiores.2005.12.002
130. Galis ZS, Sukhova GK, Kranzhöfer R, Clark S, Libby P. Macrophage foam cells from experimental atheroma constitutively produce matrix-degrading proteinases. Proc Natl Acad Sci U.S.A. (1995) 92(2):402–6. doi: 10.1073/pnas.92.2.402
131. Kim HE, Dalal SS, Young E, Legato MJ, Weisfeldt ML, D'Armiento J. Disruption of the myocardial extracellular matrix leads to cardiac dysfunction. J Clin Invest (2000) 106(7):857–66. doi: 10.1172/jci8040
132. Klatt AR, Paul-Klausch B, Klinger G, Kühn G, Renno JH, Banerjee M, et al. A critical role for collagen II in cartilage matrix degradation: Collagen II induces pro-inflammatory cytokines and MMPs in primary human chondrocytes. J Orthopaedic Res (2009) 27(1):65–70. doi: 10.1002/jor.20716
133. Iimuro Y, Nishio T, Morimoto T, Nitta T, Stefanovic B, Choi SK, et al. Delivery of matrix metalloproteinase-1 attenuates established liver fibrosis in the rat. Gastroenterology (2003) 124(2):445–58. doi: 10.1053/gast.2003.50063
134. Lombardi R, Betocchi S, Losi MA, Tocchetti CG, Aversa M, Miranda M, et al. Myocardial collagen turnover in hypertrophic cardiomyopathy. Circulation (2003) 108(12):1455–60. doi: 10.1161/01.Cir.0000090687.97972.10
135. García S, Forteza J, López-Otin C, Gómez-Reino JJ, González A, Conde C. Matrix metalloproteinase-8 deficiency increases joint inflammation and bone erosion in the K/BxN serum-transfer arthritis model. Arthritis Res Ther (2010) 12(6):R224. doi: 10.1186/ar3211
136. Cheng C, Tempel D, van Haperen R, van Damme L, Algür M, Krams R, et al. Activation of MMP8 and MMP13 by angiotensin II correlates to severe intra-plaque hemorrhages and collagen breakdown in atherosclerotic lesions with a vulnerable phenotype. Atherosclerosis (2009) 204(1):26–33. doi: 10.1016/j.atherosclerosis.2009.01.025
137. Quillard T, Tesmenitsky Y, Croce K, Travers R, Shvartz E, Koskinas KC, et al. Selective inhibition of matrix metalloproteinase-13 increases collagen content of established mouse atherosclerosis. Arterioscler Thromb Vasc Biol (2011) 31(11):2464–72. doi: 10.1161/atvbaha.111.231563
138. Kim EJ, Cho HJ, Park D, Kim JY, Kim YB, Park TG, et al. Antifibrotic effect of MMP13-encoding plasmid DNA delivered using polyethylenimine shielded with hyaluronic acid. Mol Ther (2011) 19(2):355–61. doi: 10.1038/mt.2010.262
139. Gutschalk CM, Yanamandra AK, Linde N, Meides A, Depner S, Mueller MM. GM-CSF enhances tumor invasion by elevated MMP-2, -9, and -26 expression. Cancer Med (2013) 2(2):117–29. doi: 10.1002/cam4.20
140. Forsyth PA, Wong H, Laing TD, Rewcastle NB, Morris DG, Muzik H, et al. Gelatinase-a (MMP-2), gelatinase-b (MMP-9) and membrane type matrix metalloproteinase-1 (MT1-MMP) are involved in different aspects of the pathophysiology of malignant gliomas. Br J Cancer (1999) 79(11-12):1828–35. doi: 10.1038/sj.bjc.6690291
141. Sharshar T, Durand MC, Lefaucheur JP, Lofaso F, Raphaël JC, Gherardi RK, et al. MMP-9 correlates with electrophysiologic abnormalities in Guillain-Barré syndrome. Neurology (2002) 59(10):1649–51. doi: 10.1212/01.wnl.0000033799.58866.7a
142. Zhong C, Yang J, Xu T, Xu T, Peng Y, Wang A, et al. Serum matrix metalloproteinase-9 levels and prognosis of acute ischemic stroke. Neurology (2017) 89(8):805–12. doi: 10.1212/WNL.0000000000004257
143. Nasr El-Din A, Ata KAE, Abdel-Gawad AR, Fahmy NF. Impact of high serum levels of MMP-7, MMP-9, TGF-β and PDGF macrophage activation markers on severity of COVID-19 in obese-diabetic patients. Infection Drug Resistance (2021) 14:4015–25. doi: 10.2147/idr.S329004
144. Frieling JS, Li T, Tauro M, Lynch CC. Prostate cancer-derived MMP-3 controls intrinsic cell growth and extrinsic angiogenesis. Neoplasia (2020) 22(10):511–21. doi: 10.1016/j.neo.2020.08.004
145. Bauer A, Habior A. Concentration of serum matrix metalloproteinase-3 in patients with primary biliary cholangitis. Front Immunol (2022) 13:885229. doi: 10.3389/fimmu.2022.885229
146. Matilla L, Roncal C, Ibarrola J, Arrieta V, García-Peña A, Fernández-Celis A, et al. A role for MMP-10 (Matrix metalloproteinase-10) in calcific aortic valve stenosis. Arterioscler Thromb Vasc Biol (2020) 40(5):1370–82. doi: 10.1161/atvbaha.120.314143
147. Frederick LA, Matthews JA, Jamieson L, Justilien V, Thompson EA, Radisky DC, et al. Matrix metalloproteinase-10 is a critical effector of protein kinase Ciota-Par6alpha-Mediated lung cancer. Oncogene (2008) 27(35):4841–53. doi: 10.1038/onc.2008.119
148. Cheng CW, Yu JC, Wang HW, Huang CS, Shieh JC, Fu YP, et al. The clinical implications of MMP-11 and CK-20 expression in human breast cancer. Clinica Chimica Acta (2010) 411(3-4):234–41. doi: 10.1016/j.cca.2009.11.009
149. Tian X, Ye C, Yang Y, Guan X, Dong B, Zhao M, et al. Expression of CD147 and matrix metalloproteinase-11 in colorectal cancer and their relationship to clinicopathological features. J Trans Med (2015) 13:337. doi: 10.1186/s12967-015-0702-y
150. Acioglu E, Yigit O, Alkan Z, Server EA, Uzun H, Gelisgen R. The role of matrix metalloproteinases in recurrent tonsillitis. Int J Pediatr Otorhinolaryngol (2010) 74(5):535–9. doi: 10.1016/j.ijporl.2010.02.016
151. Hsu TI, Lin SC, Lu PS, Chang WC, Hung CY, Yeh YM, et al. MMP-7-mediated cleavage of nucleolin at ASP255 induces MMP9 expression to promote tumor malignancy. Oncogene (2015) 34(7):826–37. doi: 10.1038/onc.2014.22
152. Hu BH, Cai Q, Hu Z, Patel M, Bard J, Jamison J, et al. Metalloproteinases and their associated genes contribute to the functional integrity and noise-induced damage in the cochlear sensory epithelium. J Neurosci (2012) 32(43):14927–41. doi: 10.1523/jneurosci.1588-12.2012
153. Soslow JH, Xu M, Slaughter JC, Crum K, Chew JD, Burnette WB, et al. The role of matrix metalloproteinases and tissue inhibitors of metalloproteinases in duchenne muscular dystrophy cardiomyopathy. J Cardiac Failure (2019) 25(4):259–67. doi: 10.1016/j.cardfail.2019.02.006
154. Buhler LA, Samara R, Guzman E, Wilson CL, Krizanac-Bengez L, Janigro D, et al. Matrix metalloproteinase-7 facilitates immune access to the CNS in experimental autoimmune encephalomyelitis. BMC Neurosci (2009) 10:17. doi: 10.1186/1471-2202-10-17
155. Harada T, Arii S, Mise M, Imamura T, Higashitsuji H, Furutani M, et al. Membrane-type matrix metalloproteinase-1(MT1-MTP) gene is overexpressed in highly invasive hepatocellular carcinomas. J Hepatol (1998) 28(2):231–9. doi: 10.1016/0168-8278(88)80010-2
156. Li T, Xie J, Shen C, Cheng D, Shi Y, Wu Z, et al. miR-150-5p inhibits hepatoma cell migration and invasion by targeting MMP14. PLos One (2014) 9(12):e115577. doi: 10.1371/journal.pone.0115577
157. Kurahara S, Shinohara M, Ikebe T, Nakamura S, Beppu M, Hiraki A, et al. Expression of MMPs, MT-MMP, and TIMPs in squamous cell carcinoma of the oral cavity: Correlations with tumor invasion and metastasis. Head Neck (1999) 21(7):627–38. doi: 10.1002/(SICI)1097-0347(199910)21:7<627::AID-HED7>3.0.CO;2-2
158. Mimori K, Fukagawa T, Kosaka Y, Ishikawa K, Iwatsuki M, Yokobori T, et al. A Large-scale study of MT1-MMP as a marker for isolated tumor cells in peripheral blood and bone marrow in gastric cancer cases. Ann Surg Oncol (2008) 15(10):2934–42. doi: 10.1245/s10434-008-9916-z
159. Xu X, Chen L, Xu B, Xie Q, Sun M, Deng X, et al. Increased MT2-MMP expression in gastric cancer patients is associated with poor prognosis. Int J Clin Exp Pathol (2015) 8(2):1985–90.
160. Jalali S, Singh S, Agnihotri S, Wataya T, Salehi F, Alkins R, et al. A role for matrix remodelling proteins in invasive and malignant meningiomas. Neuropathol Appl Neurobiol (2015) 41(2):e16–28. doi: 10.1111/nan.12166
161. Chabottaux V, Sounni NE, Pennington CJ, English WR, van den Brûle F, Blacher S, et al. Membrane-type 4 matrix metalloproteinase promotes breast cancer growth and metastases. Cancer Res (2006) 66(10):5165–72. doi: 10.1158/0008-5472.Can-05-3012
162. Baranger K, Bonnet AE, Girard SD, Paumier JM, García-González L, Elmanaa W, et al. MT5-MMP promotes Alzheimer’s pathogenesis in the frontal cortex of 5xFAD mice and app trafficking in vitro. Front Mol Neurosci (2016) 9:163. doi: 10.3389/fnmol.2016.00163
163. Baranger K, Marchalant Y, Bonnet AE, Crouzin N, Carrete A, Paumier JM, et al. MT5-MMP is a new pro-amyloidogenic proteinase that promotes amyloid pathology and cognitive decline in a transgenic mouse model of Alzheimer’s disease. Cell Mol Life Sci (2016) 73(1):217–36. doi: 10.1007/s00018-015-1992-1
164. Pilat D, Paumier JM, García-González L, Louis L, Stephan D, Manrique C, et al. MT5-MMP promotes neuroinflammation, neuronal excitability and Aβ production in primary neuron/astrocyte cultures from the 5xFAD mouse model of Alzheimer’s disease. J Neuroinflamm (2022) 19(1):65. doi: 10.1186/s12974-022-02407-z
165. Sugimoto W, Itoh K, Hirata H, Abe Y, Torii T, Mitsui Y, et al. MMP24 as a target of YAP is a potential prognostic factor in cancer patients. Bioengineering (2020) 7(1):18. doi: 10.3390/bioengineering7010018
166. Velasco G, Cal S, Merlos-Suárez A, Ferrando AA, Alvarez S, Nakano A, et al. Human MT6-matrix metalloproteinase: Identification, progelatinase a activation, and expression in brain tumors. Cancer Res (2000) 60(4):877–82.
167. López B, González A, Díez J. Circulating biomarkers of collagen metabolism in cardiac diseases. Circulation (2010) 121(14):1645–54. doi: 10.1161/circulationaha.109.912774
168. Choke E, Cockerill G, Wilson WR, Sayed S, Dawson J, Loftus I, et al. A review of biological factors implicated in abdominal aortic aneurysm rupture. Eur J Vasc Endovascular Surg (2005) 30(3):227–44. doi: 10.1016/j.ejvs.2005.03.009
169. Van den Steen PE, Dubois B, Nelissen I, Rudd PM, Dwek RA, Opdenakker G. Biochemistry and molecular biology of gelatinase B or matrix metalloproteinase-9 (MMP-9). Crit Rev Biochem Mol Biol (2002) 37(6):375–536. doi: 10.1080/10409230290771546
170. Ram M, Sherer Y, Shoenfeld Y. Matrix metalloproteinase-9 and autoimmune diseases. J Clin Immunol (2006) 26(4):299–307. doi: 10.1007/s10875-006-9022-6
171. Overall CM, López-Otín C. Strategies for MMP inhibition in cancer: Innovations for the post-trial era. Nat Rev Cancer (2002) 2(9):657–72. doi: 10.1038/nrc884
172. Ahmed AS, Li J, Erlandsson-Harris H, Stark A, Bakalkin G, Ahmed M. Suppression of pain and joint destruction by inhibition of the proteasome system in experimental osteoarthritis. Pain (2012) 153(1):18–26. doi: 10.1016/j.pain.2011.08.001
173. Mittal R, Patel AP, Debs LH, Nguyen D, Patel K, Mh G, et al. Intricate functions of matrix metalloproteinases in physiological and pathological conditions. J Cell Physiol (2016) 231(12):2599–621. doi: 10.1002/jcp.25430
174. Puente XS, Pendás AM, Llano E, Velasco G, López-Otín C. Molecular cloning of a novel membrane-type matrix metalloproteinase from a human breast carcinoma. Cancer Res (1996) 56(5):944–9.
175. Cabral-Pacheco GA, Garza-Veloz I, Castruita-De la Rosa C, Ramirez-Acuña JM, Perez-Romero BA, Guerrero-Rodriguez JF, et al. The roles of matrix metalloproteinases and their inhibitors in human diseases. Int J Mol Sci (2020) 21(24):9739. doi: 10.3390/ijms21249739
176. Raeeszadeh-Sarmazdeh M, Do LD, Hritz BG. Metalloproteinases and their inhibitors: Potential for the development of new therapeutics. Cells (2020) 9(5):1313. doi: 10.3390/cells9051313
177. Peschon JJ, Slack JL, Reddy P, Stocking KL, Sunnarborg SW, Lee DC, et al. An essential role for ectodomain shedding in mammalian development. Science (1998) 282(5392):1281–4. doi: 10.1126/science.282.5392.1281
178. Strand S, Vollmer P, van den Abeelen L, Gottfried D, Alla V, Heid H, et al. Cleavage of CD95 by matrix metalloproteinase-7 induces apoptosis resistance in tumour cells. Oncogene (2004) 23(20):3732–6. doi: 10.1038/sj.onc.1207387
179. Ahonen M, Poukkula M, Baker AH, Kashiwagi M, Nagase H, Eriksson JE, et al. Tissue inhibitor of metalloproteinases-3 induces apoptosis in melanoma cells by stabilization of death receptors. Oncogene (2003) 22(14):2121–34. doi: 10.1038/sj.onc.1206292
180. Chun TH, Sabeh F, Ota I, Murphy H, McDonagh KT, Holmbeck K, et al. MT1-MMP-dependent neovessel formation within the confines of the three-dimensional extracellular matrix. J Cell Biol (2004) 167(4):757–67. doi: 10.1083/jcb.200405001
181. Stratman AN, Saunders WB, Sacharidou A, Koh W, Fisher KE, Zawieja DC, et al. Endothelial cell lumen and vascular guidance tunnel formation requires MT1-MMP-dependent proteolysis in 3-dimensional collagen matrices. Blood (2009) 114(2):237–47. doi: 10.1182/blood-2008-12-196451
182. Rundhaug JE. Matrix metalloproteinases, angiogenesis, and cancer: Commentary re: A. c. Lockhart et al., reduction of wound angiogenesis in patients treated with BMS-275291, a broad spectrum matrix metalloproteinase inhibitor. Clin Cancer Res (2003) 9(2):551–4. doi: 10.1093/carcin/24.2.343
183. Ghasemi A, Hashemy SI, Aghaei M, Panjehpour M. Leptin induces matrix metalloproteinase 7 expression to promote ovarian cancer cell invasion by activating ERK and JNK pathways. J Cell Biochem (2018) 119(2):2333–44. doi: 10.1002/jcb.26396
184. Bosch R, Philips N, Suárez-Pérez JA, Juarranz A, Devmurari A, Chalensouk-Khaosaat J, et al. Mechanisms of photoaging and cutaneous photocarcinogenesis, and photoprotective strategies with phytochemicals. Antioxidants (2015) 4(2):248–68. doi: 10.3390/antiox4020248
185. Cui Q, Wang B, Li K, Sun H, Hai T, Zhang Y, et al. Upregulating MMP-1 in carcinoma-associated fibroblasts reduces the efficacy of Taxotere on breast cancer synergized by Collagen IV. Oncol Lett (2018) 16(3):3537–44. doi: 10.3892/ol.2018.9092
186. Wang QM, Lv L, Tang Y, Zhang L, Wang LF. MMP-1 is overexpressed in triple-negative breast cancer tissues and the knockdown of MMP-1 expression inhibits tumor cell malignant behaviors in vitro. Oncol Lett (2019) 17(2):1732–40. doi: 10.3892/ol.2018.9779
187. Yamada T, Oshima T, Yoshihara K, Tamura S, Kanazawa A, Inagaki D, et al. Overexpression of MMP-13 gene in colorectal cancer with liver metastasis. Anticancer Res (2010) 30(7):2693–9. doi: 10.1097/CAD.0b013e32833ab7a0
188. Zhang X, Huang S, Guo J, Zhou L, You L, Zhang T, et al. Insights into the distinct roles of MMP-11 in tumor biology and future therapeutics (Review). Int J Oncol (2016) 48(5):1783–93. doi: 10.3892/ijo.2016.3400
189. Knapinska AM, Fields GB. The expanding role of MT1-MMP in cancer progression. Pharmaceuticals (2019) 12(2):77. doi: 10.3390/ph12020077
190. Gialeli C, Theocharis AD, Karamanos NK. Roles of matrix metalloproteinases in cancer progression and their pharmacological targeting. FEBS J (2011) 278(1):16–27. doi: 10.1111/j.1742-4658.2010.07919.x
191. Ye Y, Kuang X, Xie Z, Liang L, Zhang Z, Zhang Y, et al. Small-molecule MMP2/MMP9 inhibitor SB-3CT modulates tumor immune surveillance by regulating PD-L1. Genome Med (2020) 12(1):83. doi: 10.1186/s13073-020-00780-z
192. Wells JM, Gaggar A, Blalock JE. MMP generated matrikines. Matrix Biol (2015) 44-46:122–9. doi: 10.1016/j.matbio.2015.01.016
193. Niland S, Riscanevo AX, Eble JA. Matrix metalloproteinases shape the tumor microenvironment in cancer progression. Int J Mol Sci (2021) 23(1):146. doi: 10.3390/ijms23010146
194. Gobin E, Bagwell K, Wagner J, Mysona D, Sandirasegarane S, Smith N, et al. A pan-cancer perspective of matrix metalloproteases (MMP) gene expression profile and their diagnostic/prognostic potential. BMC Cancer (2019) 19(1):581. doi: 10.1186/s12885-019-5768-0
195. Buttacavoli M, Di Cara G, Roz E, Pucci-Minafra I, Feo S, Cancemi P. Integrated multi-omics investigations of metalloproteinases in colon cancer: Focus on MMP2 and MMP9. Int J Mol Sci (2021) 22(22):12389. doi: 10.3390/ijms222212389
196. Leifler KS, Svensson S, Abrahamsson A, Bendrik C, Robertson J, Gauldie J, et al. Inflammation induced by MMP-9 enhances tumor regression of experimental breast cancer. J Immunol (2013) 190(8):4420–30. doi: 10.4049/jimmunol.1202610
197. Heimesaat MM, Dunay IR, Fuchs D, Trautmann D, Fischer A, Kühl AA, et al. The distinct roles of MMP-2 and MMP-9 in acute DSS colitis. Eur J Microbiol Immunol (2011) 1(4):302–10. doi: 10.1556/EuJMI.1.2011.4.6
198. Lin X, Li J, Zhao Q, Feng JR, Gao Q, Nie JY. WGCNA reveals key roles of IL8 and MMP-9 in progression of involvement area in colon of patients with ulcerative colitis. Curr Med Sci (2018) 38(2):252–8. doi: 10.1007/s11596-018-1873-6
199. Zeng Y, Gao M, Lin D, Du G, Cai Y. Prognostic and immunological roles of MMP-9 in pan-cancer. BioMed Res Int (2022) 2022:2592962. doi: 10.1155/2022/2592962
200. Wu J, Chen Z, Wickström SL, Gao J, He X, Jing X, et al. Interleukin-33 is a novel immunosuppressor that protects cancer cells from TIL killing by a macrophage-mediated shedding mechanism. Advanced Sci (2021) 8(21):e2101029. doi: 10.1002/advs.202101029
201. Kim HS, Kim MG, Min KW, Jung US, Kim DH. High MMP-11 expression associated with low CD8+ T cells decreases the survival rate in patients with breast cancer. PLos One (2021) 16(5):e0252052. doi: 10.1371/journal.pone.0252052
202. Fingleton B. Matrix metalloproteinases as regulators of inflammatory processes. Biochim Biophys Acta Mol Cell Res (2017) 1864(11 Pt A):2036–42. doi: 10.1016/j.bbamcr.2017.05.010
203. McMahan RS, Birkland TP, Smigiel KS, Vandivort TC, Rohani MG, Manicone AM, et al. Stromelysin-2 (MMP10) moderates inflammation by controlling macrophage activation. J Immunol (2016) 197(3):899–909. doi: 10.4049/jimmunol.1600502
204. Baugh MD, Perry MJ, Hollander AP, Davies DR, Cross SS, Lobo AJ, et al. Matrix metalloproteinase levels are elevated in inflammatory bowel disease. Gastroenterology (1999) 117(4):814–22. doi: 10.1016/s0016-5085(99)70339-2
205. von Lampe B, Barthel B, Coupland SE, Riecken EO, Rosewicz S. Differential expression of matrix metalloproteinases and their tissue inhibitors in colon mucosa of patients with inflammatory bowel disease. Gut (2000) 47(1):63–73. doi: 10.1136/gut.47.1.63
206. Matsuno K, Adachi Y, Yamamoto H, Goto A, Arimura Y, Endo T, et al. The expression of matrix metalloproteinase matrilysin indicates the degree of inflammation in ulcerative colitis. J Gastroenterol (2003) 38(4):348–54. doi: 10.1007/s005350300062
207. Saarialho-Kere UK, Vaalamo M, Puolakkainen P, Airola K, Parks WC, Karjalainen-Lindsberg ML. Enhanced expression of matrilysin, collagenase, and stromelysin-1 in gastrointestinal ulcers. Am J Pathol (1996) 148(2):519–26. doi: 10.1111/j.1399-0039.1996.tb02552.x
208. Sánchez K, Maguire-Zeiss K. MMP13 expression is increased following mutant α-synuclein exposure and promotes inflammatory responses in microglia. Front Neurosci (2020) 14:585544. doi: 10.3389/fnins.2020.585544
209. Le NTV, Xue M, Castelnoble LA, Jackson CJ. The dual personalities of matrix metalloproteinases in inflammation. Front Biosci (2007) 12:1475–87. doi: 10.2741/2161
210. Vanlaere I, Libert C. Matrix metalloproteinases as drug targets in infections caused by gram-negative bacteria and in septic shock. Clin Microbiol Rev (2009) 22(2):224–39. doi: 10.1128/CMR.00047-08
211. Tressel SL, Kaneider NC, Kasuda S, Foley C, Koukos G, Austin K, et al. A matrix metalloprotease-PAR1 system regulates vascular integrity, systemic inflammation and death in sepsis. EMBO Mol Med (2011) 3(7):370–84. doi: 10.1002/emmm.201100145
212. Walter L, Canup B, Pujada A, Bui TA, Arbasi B, Laroui H, et al. Matrix metalloproteinase 9 (MMP9) limits reactive oxygen species (ROS) accumulation and DNA damage in colitis-associated cancer. Cell Death Dis (2020) 11(9):767. doi: 10.1038/s41419-020-02959-z
213. Garg P, Sarma D, Jeppsson S, Patel NR, Gewirtz AT, Merlin D, et al. Matrix metalloproteinase-9 functions as a tumor suppressor in colitis-associated cancer. Cancer Res (2010) 70(2):792–801. doi: 10.1158/0008-5472.CAN-09-3166
214. Goel GA, Kandiel A, Achkar JP, Lashner B. Molecular pathways underlying IBD-associated colorectal neoplasia: Therapeutic implications. Am J Gastroenterol (2011) 106(4):719–30. doi: 10.1038/ajg.2011.51
215. OʼShea NR, Smith AM. Matrix metalloproteases role in bowel inflammation and inflammatory bowel disease: An up to date review. Inflammation Bowel Dis (2014) 20(12):2379–93. doi: 10.1097/MIB.0000000000000163
216. Medina C, Videla S, Radomski A, Radomski MW, Antolín M, Guarner F, et al. Increased activity and expression of matrix metalloproteinase-9 in a rat model of distal colitis. Am J Physiol Gastrointestinal Liver Physiol (2003) 284(1):G116–22. doi: 10.1152/ajpheart.00036.2002
217. Ravi A, Garg P, Sitaraman SV. Matrix metalloproteinases in inflammatory bowel disease: Boon or a bane? Inflamm Bowel Dis (2007) 13(1):97–107. doi: 10.1002/ibd.20011
218. Gao Q, Meijer MJW, Kubben FJGM, Sier CFM, Kruidenier L, van Duijn W, et al. Expression of matrix metalloproteinases-2 and -9 in intestinal tissue of patients with inflammatory bowel diseases. Digestive Liver Dis (2005) 37(8):584–92. doi: 10.1016/j.dld.2005.02.011
219. Medina C, Santana A, Paz-Cabrera MC, Parra-Blanco A, Nicolás D, Gimeno-Garcia AZ, et al. Increased activity and expression of gelatinases in ischemic colitis. Dig Dis Sci (2006) 51(12):2393–9. doi: 10.1007/s10620-006-9255-5
220. Marshall DC, Lyman SK, McCauley S, Kovalenko M, Spangler R, Liu C, et al. Selective allosteric inhibition of MMP9 is efficacious in preclinical models of ulcerative colitis and colorectal cancer. PLos One (2015) 10(5):e0127063. doi: 10.1371/journal.pone.0127063
221. Kusugami K, Nobata K, Tsuzuki T, Ando T, Ina K. Mucosal expression of matrix metalloproteinases and their tissue inhibitors in ulcerative colitis patients. J Gastroenterol (2003) 38(4):412–3. doi: 10.1007/s005350300075
222. Pender SL, Tickle SP, Docherty AJ, Howie D, Wathen NC, MacDonald TT. A major role for matrix metalloproteinases in T cell injury in the gut. J Immunol (1997) 158(4):1582–90.
223. Pender SL, Fell JM, Chamow SM, Ashkenazi A, MacDonald TT. A P55 TNF receptor immunoadhesin prevents T cell-mediated intestinal injury by inhibiting matrix metalloproteinase production. J Immunol (1998) 160(8):4098–103. doi: 10.4049/jimmunol.160.8.4098
224. Kirkegaard T, Hansen A, Bruun E, Brynskov J. Expression and localisation of matrix metalloproteinases and their natural inhibitors in fistulae of patients with Crohn's disease. Gut (2004) 53(5):701–9. doi: 10.1136/gut.2003.017442
225. Pilcher BK, Dumin JA, Sudbeck BD, Krane SM, Welgus HG, Parks WC. The activity of collagenase-1 is required for keratinocyte migration on a type I collagen matrix. J Cell Biol (1997) 137(6):1445–57. doi: 10.1083/jcb.137.6.1445
226. Salmela MT, Pender SLF, Karjalainen-Lindsberg ML, Puolakkainen P, Macdonald TT, Saarialho-Kere U. Collagenase-1 (MMP-1), matrilysin-1 (MMP-7), and stromelysin-2 (MMP-10) are expressed by migrating enterocytes during intestinal wound healing. Scand J Gastroenterol (2004) 39(11):1095–104. doi: 10.1080/00365520410003470
227. Gill SE, Parks WC. Metalloproteinases and their inhibitors: Regulators of wound healing. Int J Biochem Cell Biol (2008) 40(6-7):1334–47. doi: 10.1016/j.biocel.2007.10.024
228. Hayden DM, Forsyth C, Keshavarzian A. The role of matrix metalloproteinases in intestinal epithelial wound healing during normal and inflammatory states. J Surg Res (2011) 168(2):315–24. doi: 10.1016/j.jss.2010.03.002
229. Bamba S, Andoh A, Yasui H, Araki Y, Bamba T, Fujiyama Y. Matrix metalloproteinase-3 secretion from human colonic subepithelial myofibroblasts: Role of interleukin-17. J Gastroenterol (2003) 38(6):548–54. doi: 10.1007/s00535-002-1101-8
230. Bullard KM, Mudgett J, Scheuenstuhl H, Hunt TK, Banda MJ. Stromelysin-1-deficient fibroblasts display impaired contraction in vitro. J Surg Res (1999) 84(1):31–4. doi: 10.1006/jsre.1999.5599
231. Bullard KM, Lund L, Mudgett JS, Mellin TN, Hunt TK, Murphy B, et al. Impaired wound contraction in stromelysin-1-deficient mice. Ann Surg (1999) 230(2):260–5. doi: 10.1097/00000658-199908000-00017
232. Nissinen L, Kähäri V-M. Matrix metalloproteinases in inflammation. Biochim Biophys Acta (2014) 1840(8):2571–80. doi: 10.1016/j.bbagen.2014.03.007
233. Butler GS, Overall CM. Matrix metalloproteinase processing of signaling molecules to regulate inflammation. Periodontol 2000 (2013) 63(1):123–48. doi: 10.1111/prd.12035
234. McQuibban GA, Butler GS, Gong JH, Bendall L, Power C, Clark-Lewis I, et al. Matrix metalloproteinase activity inactivates the CXC chemokine stromal cell-derived factor-1. J Biol Chem (2001) 276(47):43503–8. doi: 10.1074/jbc.M107736200
235. McQuibban GA, Gong J-H, Wong JP, Wallace JL, Clark-Lewis I, Overall CM. Matrix metalloproteinase processing of monocyte chemoattractant proteins generates CC chemokine receptor antagonists with anti-inflammatory properties in vivo. Blood (2002) 100(4):1160–7. doi: 10.1182/blood.V100.4.1160.h81602001160_1160_1167
236. Van Den Steen PE, Wuyts A, Husson SJ, Proost P, Van Damme J, Opdenakker G. Gelatinase B/MMP-9 and neutrophil collagenase/MMP-8 process the chemokines human GCP-2/CXCl6, ENA-78/CXCl5 and mouse GCP-2/LIX and modulate their physiological activities. Eur J Biochem (2003) 270(18):3739–49. doi: 10.1046/j.1432-1033.2003.03760.x
237. Starr AE, Dufour A, Maier J, Overall CM. Biochemical analysis of matrix metalloproteinase activation of chemokines CCL15 and CCL23 and increased glycosaminoglycan binding of CCL16. J Biol Chem (2012) 287(8):5848–60. doi: 10.1074/jbc.M111.314609
238. Haro H, Crawford HC, Fingleton B, Shinomiya K, Spengler DM, Matrisian LM. Matrix metalloproteinase-7-dependent release of tumor necrosis factor-alpha in a model of herniated disc resorption. J Clin Invest (2000) 105(2):143–50. doi: 10.1172/JCI7091
239. McCawley LJ, Matrisian LM. Matrix metalloproteinases: They're not just for matrix anymore! Curr Opin Cell Biol (2001) 13(5):534–40. doi: 10.1016/s0955-0674(00)00248-9
240. Schönbeck U, Mach F, Libby P. Generation of biologically active IL-1 beta by matrix metalloproteinases: A novel caspase-1-dependent pathway of IL-1 beta processing. J Immunol (1998) 161(7):3340–6. doi: 10.4049/jimmunol.161.7.3340
241. Ito A, Mukaiyama A, Itoh Y, Nagase H, Thogersen IB, Enghild JJ, et al. Degradation of interleukin 1beta by matrix metalloproteinases. J Biol Chem (1996) 271(25):14657–60. doi: 10.1074/jbc.271.25.14657
242. Hosokawa Y, Hosokawa I, Ozaki K, Matsuo T. Sudachitin inhibits matrix metalloproteinase-1 and -3 production in tumor necrosis factor-α-stimulated human periodontal ligament cells. Inflammation (2019) 42(4):1456–62. doi: 10.1007/s10753-019-01007-z
243. Ahn S-J, Rhim E-M, Kim J-Y, Kim K-H, Lee H-W, Kim E-C, et al. Tumor necrosis factor-α induces matrix metalloproteinases-3, -10, and -13 in human periodontal ligament cells. J Periodontol (2014) 85(3):490–7. doi: 10.1902/jop.2013.130063
244. Du G, Liu C, Li X, Chen W, He R, Wang X, et al. Induction of matrix metalloproteinase-1 by tumor necrosis factor-α is mediated by interleukin-6 in cultured fibroblasts of keratoconus. Exp Biol Med (2016) 241(18):2033–41. doi: 10.1177/1535370216650940
245. Castaneda FE, Walia B, Vijay-Kumar M, Patel NR, Roser S, Kolachala VL, et al. Targeted deletion of metalloproteinase 9 attenuates experimental colitis in mice: Central role of epithelial-derived MMP. Gastroenterology (2005) 129(6):1991–2008. doi: 10.1053/j.gastro.2005.09.017
246. Mimura T, Bateman AC, Lee RL, Johnson PA, McDonald PJ, Talbot IC, et al. Up-regulation of collagen and tissue inhibitors of matrix metalloproteinase in colonic diverticular disease. Dis Colon Rectum (2004) 47(3):371–8. doi: 10.1007/s10350-003-0050-5
247. Bister V, Salmela MT, Heikkilä P, Anttila A, Rintala R, Isaka K, et al. Matrilysins-1 and -2 (MMP-7 and -26) and metalloelastase (MMP-12), unlike MMP-19, are up-regulated in necrotizing enterocolitis. J Pediatr Gastroenterol Nutr (2005) 40(1):60–6. doi: 10.1097/00005176-200501000-00011
248. Hasty KA, Pourmotabbed TF, Goldberg GI, Thompson JP, Spinella DG, Stevens RM, et al. Human neutrophil collagenase. a distinct gene product with homology to other matrix metalloproteinases. J Biol Chem (1990) 265(20):11421–4. doi: 10.4049/jimmunol.161.7.3340
249. Houghton AM, Hartzell WO, Robbins CS, Gomis-Rüth FX, Shapiro SD. Macrophage elastase kills bacteria within murine macrophages. Nature (2009) 460(7255):637–41. doi: 10.1038/nature08181
250. Wilson CL, Ouellette AJ, Satchell DP, Ayabe T, López-Boado YS, Stratman JL, et al. Regulation of intestinal alpha-defensin activation by the metalloproteinase matrilysin in innate host defense. Science (1999) 286(5437):113–7. doi: 10.1126/science.286.5437.113
251. Pal S, Schmidt AP, Peterson EM, Wilson CL, de la Maza LM. Role of matrix metalloproteinase-7 in the modulation of a chlamydia trachomatis infection. Immunology (2006) 117(2):213–9. doi: 10.1111/j.1365-2567.2005.02281.x
252. Esteban S, Clemente C, Koziol A, Gonzalo P, Rius C, Martínez F, et al. Endothelial MT1-MMP targeting limits intussusceptive angiogenesis and colitis via TSP1/Nitric oxide axis. EMBO Mol Med (2020) 12(2):e10862. doi: 10.15252/emmm.201910862
253. Marelli G, Erreni M, Anselmo A, Taverniti V, Guglielmetti S, Mantovani A, et al. Heme-Oxygenase-1 production by intestinal CX3CR1 macrophages helps to resolve inflammation and prevents carcinogenesis. Cancer Res (2017) 77(16):4472–85. doi: 10.1158/0008-5472.CAN-16-2501
254. Medina-Contreras O, Geem D, Laur O, Williams IR, Lira SA, Nusrat A, et al. CX3CR1 regulates intestinal macrophage homeostasis, bacterial translocation, and colitogenic Th17 responses in mice. J Clin Invest (2011) 121(12):4787–95. doi: 10.1172/JCI59150
255. Zhuang Q, Ou J, Zhang S, Ming Y. Crosstalk between the CX3CL1/CX3CR1 axis and inflammatory signaling pathways in tissue injury. Curr Protein Pept Sci (2019) 20(8):844–54. doi: 10.2174/1389203720666190305165722
256. de Bruyn M, Arijs I, Wollants WJ, Machiels K, Van Steen K, Van Assche G, et al. Neutrophil gelatinase B-associated lipocalin and matrix metalloproteinase-9 complex as a surrogate serum marker of mucosal healing in ulcerative colitis. Inflamm Bowel Dis (2014) 20(7):1198–207. doi: 10.1097/mib.0000000000000068
257. Farkas K, Saródi Z, Bálint A, Földesi I, Tiszlavicz L, Szűcs M, et al. The diagnostic value of a new fecal marker, matrix metalloprotease-9, in different types of inflammatory bowel diseases. J Crohns Colitis (2015) 9(3):231–7. doi: 10.1093/ecco-jcc/jjv005
258. Annaházi A, Molnár T, Farkas K, Rosztóczy A, Izbéki F, Gecse K, et al. Fecal MMP-9: A new noninvasive differential diagnostic and activity marker in ulcerative colitis. Inflamm Bowel Dis (2013) 19(2):316–20. doi: 10.1002/ibd.22996
259. Faubion WA Jr., Fletcher JG, O'Byrne S, Feagan BG, de Villiers WJ, Salzberg B, et al. Emerging BiomARKers in inflammatory bowel disease (EMBARK) study identifies fecal calprotectin, serum MMP9, and serum IL-22 as a novel combination of biomarkers for Crohn's disease activity: Role of cross-sectional imaging. Am J Gastroenterol (2013) 108(12):1891–900. doi: 10.1038/ajg.2013.354
260. Naito Y, Yoshikawa T. Role of matrix metalloproteinases in inflammatory bowel disease. Mol Aspects Med (2005) 26(4-5):379–90. doi: 10.1016/j.mam.2005.07.009
261. Langholz E, Munkholm P, Davidsen M, Binder V. Colorectal cancer risk and mortality in patients with ulcerative colitis. Gastroenterology (1992) 103(5):1444–51. doi: 10.1016/0016-5085(92)91163-x
262. Prior P, Fielding JF, Waterhouse JA, Cooke WT. Mortality in Crohn's disease. Lancet (1970) 1(7657):1135–7. doi: 10.1016/s0140-6736(70)91213-4
263. Xue M, Shi L, Wang W, Chen S, Wang L. An overview of molecular profiles in ulcerative colitis-related cancer. Inflammation Bowel Dis (2018) 24(9):1883–94. doi: 10.1093/ibd/izy221
264. Baek S-J, Kim S-H. Colitis-associated colorectal cancer in patients with inflammatory bowel disease. Minerva Chir (2017) 72(6):520–9. doi: 10.23736/S0026-4733.17.07437-5
265. Shah SC, Itzkowitz SH. Colorectal cancer in inflammatory bowel disease: Mechanisms and management. Gastroenterology (2022) 162(3):715–30.e3. doi: 10.1053/j.gastro.2021.10.035
266. Itzkowitz SH, Yio X. Inflammation and Cancer IV. Colorectal cancer in inflammatory bowel disease: the role of inflammation. Am J Physiol Gastrointest Liver Physiol (2004) 287(1):G7–17. doi: 10.1152/ajpgi.00079.2004
267. Short SP, Pilat JM, Barrett CW, Reddy VK, Haberman Y, Hendren JR, et al. Colonic epithelial-derived Selenoprotein P is the source for antioxidant-mediated protection in colitis-associated cancer. Gastroenterology (2021) 160(5):1694–708.e3. doi: 10.1053/j.gastro.2020.12.059
268. Garg P, Jeppsson S, Dalmasso G, Ghaleb AM, McConnell BB, Yang VW, et al. Notch1 regulates the effects of matrix metalloproteinase-9 on colitis-associated cancer in mice. Gastroenterology (2011) 141(4):1381–92. doi: 10.1053/j.gastro.2011.06.056
269. Cadet J, Wagner JR. DNA base damage by reactive oxygen species, oxidizing agents, and UV radiation. Cold Spring Harb Perspect Biol (2013) 5(2):a012559. doi: 10.1101/cshperspect.a012559
270. Peng K, Kou L, Yu L, Bai C, Li M, Mo P, et al. Histone demethylase JMJD2D interacts with β-catenin to induce transcription and activate colorectal cancer cell proliferation and tumor growth in mice. Gastroenterology (2019) 156(4):1112–26. doi: 10.1053/j.gastro.2018.11.036
271. Oshima H, Nakayama M, Han TS, Naoi K, Ju X, Maeda Y, et al. Suppressing TGFβ signaling in regenerating epithelia in an inflammatory microenvironment is sufficient to cause invasive intestinal cancer. Cancer Res (2015) 75(4):766–76. doi: 10.1158/0008-5472.Can-14-2036
272. Yoo J, Rodriguez Perez CE, Nie W, Sinnett-Smith J, Rozengurt E. Protein kinase D1 mediates synergistic MMP-3 expression induced by TNF-α and bradykinin in human colonic myofibroblasts. Biochem Biophys Res Commun (2011) 413(1):30–5. doi: 10.1016/j.bbrc.2011.08.029
273. Adegboyega PA, Ololade O, Saada J, Mifflin R, Di Mari JF, Powell DW. Subepithelial myofibroblasts express cyclooxygenase-2 in colorectal tubular adenomas. Clin Cancer Res (2004) 10(17):5870–9. doi: 10.1158/1078-0432.CCR-0431-03
274. Cheng SM, Xing B, Li JC, Cheung BK, Lau AS. Interferon-gamma regulation of TNF alpha-induced matrix metalloproteinase 3 expression and migration of human glioma T98G cells. Int J Cancer (2007) 121(6):1190–6. doi: 10.1002/ijc.22729
275. Newell KJ, Matrisian LM, Driman DK. Matrilysin (Matrix metalloproteinase-7) expression in ulcerative colitis-related tumorigenesis. Mol Carcinog (2002) 34(2):59–63. doi: 10.1002/mc.10049
276. Walter L, Harper C, Garg P. Role of matrix metalloproteinases in Inflammation/Colitis-associated colon cancer. ImmunoGastroenterology (2013) 2(2):22–2822. doi: 10.7178/ig.29
277. Ou Y-r, Jing G-y, Wu Q, Gao S, Cheng Z-n, Dong X-q. Expression of Tcf-4, MMP-7 and survivin in colorectal cancer and its clinical significance. J Sichuan Univ Med Sci Edition (2015) 46(4):554–9.
278. Bai YP, Shang K, Chen H, Ding F, Wang Z, Liang C, et al. FGF-1/-3/FGFR4 signaling in cancer-associated fibroblasts promotes tumor progression in colon cancer through ERK and MMP-7. Cancer Sci (2015) 106(10):1278–87. doi: 10.1111/cas.12745
279. Walter L, Pujada A, Bhatnagar N, Bialkowska AB, Yang VW, Laroui H, et al. Epithelial derived-matrix metalloproteinase (MMP9) exhibits a novel defensive role of tumor suppressor in colitis associated cancer by activating MMP9-Notch1-ARF-P53 axis. Oncotarget (2017) 8(1):364–78. doi: 10.18632/oncotarget.13406
280. Pozzi A, LeVine WF, Gardner HA. Low plasma levels of matrix metalloproteinase 9 permit increased tumor angiogenesis. Oncogene (2002) 21(2):272–81. doi: 10.1038/sj.onc.1205045
281. Hald A, Rønø B, Melander MC, Ding M, Holck S, Lund LR. MMP9 is protective against lethal inflammatory mass lesions in the mouse colon. Dis Model Mech (2011) 4(2):212–27. doi: 10.1242/dmm.005801
282. Pujada A, Walter L, Patel A, Bui TA, Zhang Z, Zhang Y, et al. Matrix metalloproteinase MMP9 maintains epithelial barrier function and preserves mucosal lining in colitis associated cancer. Oncotarget (2017) 8(55):94650–65. doi: 10.18632/oncotarget.21841
283. Tang K, Wu Z, Sun M, Huang X, Sun J, Shi J, et al. Elevated MMP10/13 mediated barrier disruption and NF-κB activation aggravate colitis and colon tumorigenesis in both individual or full miR-148/152 family knockout mice. Cancer Lett (2022) 529:53–69. doi: 10.1016/j.canlet.2021.12.033
284. Kim YJ, Lee JS, Hong KS, Chung JW, Kim JH, Hahm KB. Novel application of proton pump inhibitor for the prevention of colitis-induced colorectal carcinogenesis beyond acid suppression. Cancer Prev Res (2010) 3(8):963–74. doi: 10.1158/1940-6207.CAPR-10-0033
285. Kim YJ, Hong KS, Chung JW, Kim JH, Hahm KB. Prevention of colitis-associated carcinogenesis with infliximab. Cancer Prev Res (2010) 3(10):1314–33. doi: 10.1158/1940-6207.CAPR-09-0272
286. Yoon SM, Myung S-J, Ye BD, Kim I-W, Lee NG, Ryu YM, et al. Near-infrared fluorescence imaging using a protease-specific probe for the detection of colon tumors. Gut Liver (2010) 4(4):488–97. doi: 10.5009/gnl.2010.4.4.488
287. Setia S, Nehru B, Sanyal SN. The PI3K/Akt pathway in colitis associated colon cancer and its chemoprevention with celecoxib, a Cox-2 selective inhibitor. BioMed Pharmacother (2014) 68(6):721–7. doi: 10.1016/j.biopha.2014.07.006
288. Yu M, Trobridge P, Wang Y, Kanngurn S, Morris SM, Knoblaugh S, et al. Inactivation of TGF-β signaling and loss of PTEN cooperate to induce colon cancer in vivo. Oncogene (2014) 33(12):1538–47. doi: 10.1038/onc.2013.102
289. Trobridge P, Knoblaugh S, Washington MK, Munoz NM, Tsuchiya KD, Rojas A, et al. TGF-β receptor inactivation and mutant kras induce intestinal neoplasms in mice via a β-catenin-independent pathway. Gastroenterology (2009) 136(5):1680–8.e7. doi: 10.1053/j.gastro.2009.01.066
290. Ostaff MJ, Stange EF, Wehkamp J. Antimicrobial peptides and gut microbiota in homeostasis and pathology. EMBO Mol Med (2013) 5(10):1465–83. doi: 10.1002/emmm.201201773
291. Liévin-Le Moal V, Servin AL. The front line of enteric host defense against unwelcome intrusion of harmful microorganisms: Mucins, antimicrobial peptides, and microbiota. Clin Microbiol Rev (2006) 19(2):315–37. doi: 10.1128/CMR.19.2.315-337.2006
292. Gallo RL, Hooper LV. Epithelial antimicrobial defence of the skin and intestine. Nat Rev Immunol (2012) 12(7):503–16. doi: 10.1038/nri3228
293. Ai F, Zhang X, Li X, Qin Z, Ye Q, Tian L, et al. Up-regulation of matrix metalloproteinases in a mouse model of chemically induced colitis-associated cancer: The role of micrornas. Oncotarget (2015) 6(7):5412–25. doi: 10.18632/oncotarget.3027
294. Väyrynen JP, Vornanen J, Tervahartiala T, Sorsa T, Bloigu R, Salo T, et al. Serum MMP-8 levels increase in colorectal cancer and correlate with disease course and inflammatory properties of primary tumors. Int J Cancer (2012) 131(4):E463–74. doi: 10.1002/ijc.26435
295. Van Lint P, Libert C. Matrix metalloproteinase-8: Cleavage can be decisive. Cytokine Growth Factor Rev (2006) 17(4):217–23. doi: 10.1016/j.cytogfr.2006.04.001
296. Van Lint P, Wielockx B, Puimège L, Noël A, López-Otin C, Libert C. Resistance of collagenase-2 (Matrix metalloproteinase-8)-Deficient mice to TNF-induced lethal hepatitis. J Immunol (2005) 175(11):7642–9. doi: 10.4049/jimmunol.175.11.7642
297. Tyan K, Abu-Shawer O, Baginska J, Severgnini M, Manos M, Vaitkevicius H, et al. Multiple high-grade and rare immune-related adverse events in a colon cancer patient with genomic and cytokine profiling. Immunotherapy (2022) 14(11):843–50. doi: 10.2217/imt-2021-0298
298. Bischoff SC, Barbara G, Buurman W, Ockhuizen T, Schulzke JD, Serino M, et al. Intestinal permeability–a new target for disease prevention and therapy. BMC Gastroenterol (2014) 14:189. doi: 10.1186/s12876-014-0189-7
299. Ignacio A, Morales CI, Câmara NO, Almeida RR. Innate sensing of the gut microbiota: Modulation of inflammatory and autoimmune diseases. Front Immunol (2016) 7:54. doi: 10.3389/fimmu.2016.00054
300. Landskron G, de la Fuente M, Thuwajit P, Thuwajit C, Hermoso MA. Chronic inflammation and cytokines in the tumor microenvironment. J Immunol Res (2014) 2014:149185. doi: 10.1155/2014/149185
301. West NR, McCuaig S, Franchini F, Powrie F. Emerging cytokine networks in colorectal cancer. Nat Rev Immunol (2015) 15(10):615–29. doi: 10.1038/nri3896
302. Sirniö P, Tuomisto A, Tervahartiala T, Sorsa T, Klintrup K, Karhu T, et al. High-serum MMP-8 levels are associated with decreased survival and systemic inflammation in colorectal cancer. Br J Cancer (2018) 119(2):213–9. doi: 10.1038/s41416-018-0136-4
303. Mattaveewong T, Wongkrasant P, Chanchai S, Pichyangkura R, Chatsudthipong V, Muanprasat C. Chitosan oligosaccharide suppresses tumor progression in a mouse model of colitis-associated colorectal cancer through AMPK activation and suppression of NF-κB and mTOR signaling. Carbohydr Polymers (2016) 145:30–6. doi: 10.1016/j.carbpol.2016.02.077
304. Shang K, Bai YP, Wang C, Wang Z, Gu HY, Du X, et al. Crucial involvement of tumor-associated neutrophils in the regulation of chronic colitis-associated carcinogenesis in mice. PLos One (2012) 7(12):e51848. doi: 10.1371/journal.pone.0051848
305. Baggiolini M. Chemokines and leukocyte traffic. Nature (1998) 392(6676):565–8. doi: 10.1038/33340
306. Pham CT. Neutrophil serine proteases: Specific regulators of inflammation. Nat Rev Immunol (2006) 6(7):541–50. doi: 10.1038/nri1841
307. Strieter RM, Polverini PJ, Kunkel SL, Arenberg DA, Burdick MD, Kasper J, et al. The functional role of the elr motif in CXC chemokine-mediated angiogenesis. J Biol Chem (1995) 270(45):27348–57. doi: 10.1074/jbc.270.45.27348
308. Yagi Y, Andoh A, Inatomi O, Tsujikawa T, Fujiyama Y. Inflammatory responses induced by interleukin-17 family members in human colonic subepithelial myofibroblasts. J Gastroenterol (2007) 42(9):746–53. doi: 10.1007/s00535-007-2091-3
309. Monteleone G, Caruso R, Fina D, Peluso I, Gioia V, Stolfi C, et al. Control of matrix metalloproteinase production in human intestinal fibroblasts by interleukin 21. Gut (2006) 55(12):1774–80. doi: 10.1136/gut.2006.093187
310. Xie Z, Qu Y, Leng Y, Sun W, Ma S, Wei J, et al. Human colon carcinogenesis is associated with increased interleukin-17-Driven inflammatory responses. Drug design Dev Ther (2015) 9:1679–89. doi: 10.2147/dddt.S79431
311. Hata K, Andoh A, Shimada M, Fujino S, Bamba S, Araki Y, et al. Il-17 stimulates inflammatory responses via nf-κB and MAP kinase pathways in human colonic myofibroblasts. Am J Physiol Gastrointestinal Liver Physiol (2002) 282(6):G1035–44. doi: 10.1152/ajpgi.00494.2001
312. Pedersen G. Development, validation and implementation of an in vitro model for the study of metabolic and immune function in normal and inflamed human colonic epithelium. Danish Med J (2015) 62(1):B4973.
313. Mortensen JH, Godskesen LE, Jensen MD, Van Haaften WT, Klinge LG, Olinga P, et al. Fragments of citrullinated and MMP-degraded vimentin and MMP-degraded type iii collagen are novel serological biomarkers to differentiate Crohn's disease from ulcerative colitis. J Crohns Colitis (2015) 9(10):863–72. doi: 10.1093/ecco-jcc/jjv123
314. Langers AMJ, Verspaget HW, Hawinkels LJAC, Kubben FJGM, van Duijn W, van der Reijden JJ, et al. MMP-2 and MMP-9 in normal mucosa are independently associated with outcome of colorectal cancer patients. Br J Cancer (2012) 106(9):1495–8. doi: 10.1038/bjc.2012.80
315. Maurel J, Nadal C, Garcia-Albeniz X, Gallego R, Carcereny E, Almendro V, et al. Serum matrix metalloproteinase 7 levels identifies poor prognosis advanced colorectal cancer patients. Int J Cancer (2007) 121(5):1066–71. doi: 10.1002/ijc.22799
316. Martínez-Fernandez A, García-Albeniz X, Pineda E, Visa L, Gallego R, Codony-Servat J, et al. Serum matrilysin levels predict outcome in curatively resected colorectal cancer patients. Ann Surg Oncol (2009) 16(5):1412–20. doi: 10.1245/s10434-009-0405-9
317. Shantha Kumara HMC, Gaita DJ, Miyagaki H, Yan X, Herath SAC, Cekic V, et al. Minimally invasive colorectal resection is associated with significantly elevated levels of plasma matrix metalloproteinase 3 (MMP-3) during the first month after surgery which may promote the growth of residual metastases. Surg Endosc (2014) 28(12):3322–8. doi: 10.1007/s00464-014-3612-9
318. Erlichman C, Adjei AA, Alberts SR, Sloan JA, Goldberg RM, Pitot HC, et al. Phase I study of the matrix metalloproteinase inhibitor, BAY12-9566. Ann Oncol (2001) 12(3):389–95. doi: 10.1023/a:1011183905848
319. Heath EI, O'Reilly S, Humphrey R, Sundaresan P, Donehower RC, Sartorius S, et al. Phase I trial of the matrix metalloproteinase inhibitor BAY12-9566 in patients with advanced solid tumors. Cancer Chemother Pharmacol (2001) 48(4):269–74. doi: 10.1007/s002800100330
320. Rizvi NA, Humphrey JS, Ness EA, Johnson MD, Gupta E, Williams K, et al. A phase I study of oral bms-275291, a novel nonhydroxamate sheddase-sparing matrix metalloproteinase inhibitor, in patients with advanced or metastatic cancer. Clin Cancer Res (2004) 10(6):1963–70. doi: 10.1158/1078-0432.ccr-1183-02
321. Mohammadzadeh M, Faramarzi E, Mahdavi R, Nasirimotlagh B, Asghari Jafarabadi M. Effect of conjugated linoleic acid supplementation on inflammatory factors and matrix metalloproteinase enzymes in rectal cancer patients undergoing chemoradiotherapy. Integr Cancer Ther (2013) 12(6):496–502. doi: 10.1177/1534735413485417
322. Farsad-Naeimi A, Alizadeh M, Esfahani A, Darvish Aminabad E. Effect of fisetin supplementation on inflammatory factors and matrix metalloproteinase enzymes in colorectal cancer patients. Food Funct (2018) 9(4):2025–31. doi: 10.1039/c7fo01898c
323. Levin M, Udi Y, Solomonov I, Sagi I. Next generation matrix metalloproteinase inhibitors - novel strategies bring new prospects. Biochim Biophys Acta Mol Cell Res (2017) 1864(11 Pt A):1927–39. doi: 10.1016/j.bbamcr.2017.06.009
324. Zucker S, Cao J, Chen WT. Critical appraisal of the use of matrix metalloproteinase inhibitors in cancer treatment. Oncogene (2000) 19(56):6642–50. doi: 10.1038/sj.onc.1204097
325. Shi Y, Ma X, Fang G, Tian X, Ge C. Matrix metalloproteinase inhibitors (MMPIS) as attractive therapeutic targets: Recent progress and current challenges. NanoImpact (2021) 21:100293. doi: 10.1016/j.impact.2021.100293
Keywords: matrix metalloproteinases (MMPs), colitis associated cancer (CAC), immunomodulation, inflammation, extracellular matrix (ECM)
Citation: He L, Kang Q, Chan KI, Zhang Y, Zhong Z and Tan W (2023) The immunomodulatory role of matrix metalloproteinases in colitis-associated cancer. Front. Immunol. 13:1093990. doi: 10.3389/fimmu.2022.1093990
Received: 09 November 2022; Accepted: 07 December 2022;
Published: 19 January 2023.
Edited by:
Shicheng Guo, University of Wisconsin-Madison, United StatesReviewed by:
Bing Feng, Pennington Biomedical Research Center, United StatesFeng He, Stanford University, United States
Xinxin Chi, Harvard Medical School, United States
Yan Yao, Columbia University, United States
Wentao Liang, Wake Forest University, United States
Man Zhao, Stanford University, United States
Zhaohua Hou, Memorial Sloan Kettering Cancer Center, United States
Copyright © 2023 He, Kang, Chan, Zhang, Zhong and Tan. This is an open-access article distributed under the terms of the Creative Commons Attribution License (CC BY). The use, distribution or reproduction in other forums is permitted, provided the original author(s) and the copyright owner(s) are credited and that the original publication in this journal is cited, in accordance with accepted academic practice. No use, distribution or reproduction is permitted which does not comply with these terms.
*Correspondence: Zhangfeng Zhong, zfzhong@um.edu.mo; Wen Tan, tanwen@lzu.edu.cn
†These authors have contributed equally to this work