Water relations and yield characteristics of mungbean as influenced by foliar application of gibberellic acid (GA3)
- 1Department of Agronomy, Hajee Mohammad Danesh Science and Technology University, Dinajpur, Bangladesh
- 2Agronomy Division, Pulse Research Centre (BARI), Ishurdi, Bangladesh
- 3Department of Agricultural Extension, Agricultural Training Institute, Gaibanda, Bangladesh
- 4Department of Agricultural Chemistry, Hajee Mohammad Danesh Science and Technology University, Dinajpur, Bangladesh
- 5Department of Agronomy, Faculty of Agriculture, University of Poonch Rawalakot, Rawalakot, Pakistan
- 6VIT School of Agricultural Innovations and Advanced Learning (VAIAL), Vellore Institute of Technology (VIT), Vellore, India
- 7Department of Agronomy, Faculty of Agriculture and Environment, The Islamia University of Bahawalpur, Bahawalpur, Pakistan
- 8Plant Production Department, College of Food and Agriculture Sciences, King Saud University, Riyadh, Saudi Arabia
- 9College of Pastoral Agriculture Science and Technology, Lanzhou University, Lanzhou, China
- 10Department of Biotechnology, Graduate School of Engineering, Osaka University, Osaka, Japan
- 11Department of Agronomy, Faculty of Agriculture, Kafrelsheikh University, Egypt
Optimizing the dose and foliar application frequency of growth regulators such as gibberellic acid (GA3) may play an important role in ensuring food security under changing climate scenarios by boosting grain yield of food legumes such as mungbean. A trial was conducted to evaluate the growth promoting effect of foliage applied gibberellic acid (GA3) at various spraying frequencies for mungbean crop. The employed treatments included four gibberellic acid levels (0, 100, 200, and 300 ppm) and two application frequencies (single spray at 30 days after sowing DAS, and two sprays at 30 and 40 DAS). Water relations, yield contributing characteristics and mungbean grain yield were among the response variables investigated. The research findings revealed that GA3 (200 ppm applied twice at pre-flowering and post-flowering stages) significantly improved the water relations, morphological and yield attributes of mungbean. This treatment combination remained unmatched by producing the highest relative water content in the stem (85.52), water retention capacity in the stem (17.24), and water uptake capacity in the stem and leaf (2.35). Furthermore, the same treatment combination resulted in the maximum plant height (50.04 cm), pods per plant (11.07), pod length (6.62 cm), grains number per pod (11.00), 100-grains weight (3.78 g), grain yield per plant (4.57 g), and a minimum water saturation deficit. Thus, GA3 foliage application at 200 ppm at 30 and 60 DAS has the potential to stimulate growth and increase the yield attributes and grain yield of mungbean. However, additional in-depth field trials with various doses of GA3 sprayed at a higher frequency may be required before recommending GA3 for general adoption to mungbean growers.
1. Introduction
Globally, legumes are being cultivated as crop rotation crops with cereals for imparting sustainability to the modern commercial-oriented farming systems because these have potential to reduce the environmental concerns due to limited nitrogen (N) needs. In the root nodules, legumes fix atmospheric N through a symbiotic fixation process carried out by host-specific strains of rhizobia (Islam et al., 2022). Symbiotic rhizobia can boost yields by accelerating flowering and fruit ripening along with improving soil N in a biologically viable way (Iqbal et al., 2021b; Islam et al., 2021a). Mungbean [Vigna radiata (L.) Wilczek] is an important food legume crop in South Asian countries like India, Pakistan and particularly in Bangladesh (Islam et al., 2017a,b), where it ranks first in terms of acreage and production (Iqbal et al., 2021a). It occupies pivotal position in Bangladesh owing to high dietary and nutritional value as well as low-cost source of easily digestible dietary protein (Islam et al., 2017b). Its grains are enriched with protein (25.67%), fat (1–3%), carbohydrates (5.4%), fibers (3.5–4.5%), and ash (4.5–5.5%; Islam et al., 2017c; Iqbal et al., 2018, 2019). Mungbean protein is highly digestible because it contains low sulfur containing amino acids especially lesser methionine is present than lysine (Islam et al., 2017b; Iqbal et al., 2021a). It may become a major source of protein for the rapidly growing population of Bangladesh, which heavily relies on low protein cereal-based diet leading to malnutrition. Because the country relies on rice to meet its basic food needs, the cultivation of mungbean is being shifted to less fertile and/or problematic soils where sub-optimal management practices are carried out. As a result, mungbean grain yield in Bangladesh continues to remain very low in comparison to neighboring countries. This situation necessitates developing farmer-friendly, biologically viable and scientifically tested agronomic practices to increase growth and yield of mungbean in a sustainable manner.
The plant growth hormones (PGHs) are organic substances produced in extremely high, concentrations within the plant which regulate cellular activities in response to signals. The PGHs also regulate and standardize the growth and development of flowers, stems, leaves, leaf shedding, and grain maturity. Contrastingly, plants could have remained a clump of undifferentiated cells if hormones were absent or present in very small amounts. The foliar applied PGHs aided in the restoration of water content retardation in mungbean plants subjected to a water deficit condition (Das et al., 1994). Gibberellic acid (GA3) is one of the most PGHs which synergistically improved crop growth and grain yield of wheat (Islam et al., 2014), and mungbean (Chakraborti and Mukherji, 2003; Islam et al., 2021b). Additionally, GA3 also remained effective in improving soybean growth, development, and yield when it was applied as seed priming agent before crop sowing (Deotale et al., 1998) or as foliar application for various crops (Naidu and Swamy, 1995; Haque and Haque, 2002).
Interestingly, gibberellins (GAs) effectively promoted the seedling emergence, plant growth and development in soybean (Maske et al., 1997), common bean, cowpea and pigeon pea (Khafagi et al., 1986), black gram and horse gram (Chauhan et al., 2009). Furthermore, GAs tend to breakdown seed dormancy and initiate the mobilization endosperm reserves (Mahmoody and Noori, 2014). Likewise, GAs trigger stem elongation by stimulating cell division, flower formation and fruit development in many legume crops (Silva, 2004; Taiz and Zeiger, 2010). Moreover, GA3 improved crop performance and productivity under normal and sub-optimal growth conditions (Chakraborti and Mukherji, 2003), increased the size and production of grapevine fruit (Abu-Zahra, 2010) and improved the productivity and quality of lily cut flowers (Sajid et al., 2009). Besides, it increased the fiber yield of cotton (Copur et al., 2010) protein content of field peas (Bora and Sarma, 2006), chemical constituents in Croton (Soad et al., 2010), fruit size in Molina (Vwioko and Longe, 2009), floral buds in Jojoba (Prat et al., 2008), and suppressed the undesirable compounds in mungbean (Chakrabarti and Mukherji, 2002). Additionally, GA3 increased the leaf area index, dry weight, crop growth rate, relative growth rate, net assimilation rate and yield in Allium cepa (Hore et al., 1988), tree species (Naidu and Swamy, 1995), and mungbean (Haque and Haque, 2002; Islam et al., 2021b).
More importantly, it increased the pigment content of Vicia faba (Aldesuquy and Gaber, 1993), and the water use efficiency of wheat (Aldesuquy and Ibrahim, 2001) by alleviating the adverse effects of salinity. Another vital role of GA3 is promotion of photosynthesis by increasing the carboxylase activity of Rubisco in broad bean and soybean (Yuan and Xu, 2001), along with boosting the rates of cyclic and non-cyclic phosphorylations in tree species (Naidu and Swamy, 1995), and regulated thetransport of ions in plants (Karmoker, 1984). It increased water uptake in plant tissues, causing cell expansion and diluting the sugars in the tissues under water scant conditions (Salisbury and Ross, 1996). The hydrolysis of starch into reducing sugars by GA3 led to accumulation of sugar which improved the water balance and osmotic potential of gerbera cut flowers. Moreover, the GA3 decreased transpiration and triggered transition from juvenile to mature stage along with induction of flowering in cut flowers (Emongor, 2004), as well as sex determination and fruit set establishment in horticultural crops (Taiz and Zeiger, 2004). Likewise, GA3 tend to boost protein content by increasing nitrate reductase activity in cowpea (Singh and Sharma, 1996), wheat (Aldesuquy and Ibrahim, 2001), black cumin (Shah, 2004), and mungbean (Islam et al., 2021b). It has been reported to be directly linked with the regulation of flower senescence in cut flowers (Halevy and Mayak, 1981). Contrastingly, GA3 decreased the number of flowers and fruit set by boosting vegetative biomass, which led to higher transmission of photo-assimilates to fruits during the pre-blooming stage of soybean (Birnberg and Brenner, 1987). This findings are supported by King et al. (2000), who recorded increased stem growth in Fuschia hibrida and Pharbitis nil, which inhibited flowering.
Thus, the exogenous application of growth regulators like GA3 may become a viable tool for increasing crop productivity in a biologically viable manner. However, the efficiency of GA3 is dependent on the concentration and frequency of application in relation to crop growth stage, and there is very little information available on the exogenous application of GA3 for mungbean. We hypothesized that exogenous application of GA3 at various concentrations and frequencies hold potential to boost grain yield of mungbean by improving water relations and yield attributes. As a consequence, the current study was designed to evaluate the effects of GA3 and spraying frequency on mungbean growth and yield with an ultimate aim to optimize the dose of GA3 and sort out the most productive application frequency.
2. Materials and methods
2.1. Plant genetic material
The mungbean variety BARI Mung-8 was obtained from the Pulse Research Center, Regional Agricultural Research Station, Ishurdi, Pabna, Bangladesh. It was developed in 2015 by the Bangladesh Agricultural Research Institute andis being grown widely due to growth robustness during all seasons (Rabi, Kharif-1, and Kharif-2), short life span (58–60 days), higher plant height (55–60 cm), moderate grain size (TSW 25–32 g), deeply yellow grain color, high tolerance to yellow mosaic virus and leaf spot diseases, and higher grain yield (1.6–1.7 t ha−1; BARI (Bangladesh Agricultural Research Institute), 2019).
2.2. Experimental site and design
An experiment was carried out at the Agronomy Pocket House, Department of Agronomy, Hajee Mohammad Danesh Science and Technology University (HSTU), Dinajpur, Bangladesh to determine the effect of gibberellic acid on the growth, physiology and yield of mungbean. The earthen pots were used to grow mungbean plants until they were harvested. The study was planned according to completely randomized design (CRD), and replicated five times of each treatment. The experiment was completed within the period of March to May 2018.
2.3. Experimental treatments
The study included two factors of factor A: four gibberellic acid (GA3) levels (0, 100, 200, and 300 ppm), and factor B: two spraying frequencies (one time at 30 days after sowing: DAS, and two times at 30 and 40 DAS). Gibberellic acid was obtained from Nanjing Loyal Chemical Co. Ltd., China imported by M/S Rahman Scientific Store, Balubari, Dinajpur, Bangladesh, was used as a plant growth regulator.
2.4. Meteorological features of the study site
The crop was grown during Kharif-1 season, and the weather data including temperature, rainfall and relative humidity (RH) were recorded at the HSTU Meteorological Station, HSTU, Dinajpur during the experimental period, and are shown in Table 1. The average monthly temperatures (minimum and maximum), relative humidity, and rainfall gradually increased with from March to May. The maximum rainfall occurred between 55 and 61 DAS, corresponding to the grain filling period.

Table 1. Monthly average temperature (minimum, maximum, and average), relative humidity (%), and rainfall (mm) during the experiment.
2.5. Soil
The soil samples were collected from the Agronomy Research Field at HSTU, Dinajpur, and analyzed by following the prescribed procedure. The soil was sandy loam with a low nutrients status. Total soil N was 0.07 per cent, available K was 0.10 meq 100 g−1 soil, and available P and S were 10.43 and 7.46 ppm, respectively. According to the critical value, the experimental soil had a low N and K content, a moderate available S content, and a high P content. The organic carbon, organic matter content, and soil pH values were 0.479, 0.925, and 5.61%, respectively. The physical and chemical properties of the soil are shown in Table 2.
2.6. Execution of trial
Each plastic pot (17 cm × 19.5 cm) was filled up with approximately 10 kg of well-pulverized air dried soil which was sandy loam type with 25.8% field capacity and 1.49 g/cc bulk density. The soil was mixed with the recommended doses of fertilizers viz., 50, 90, 40 and 55 kg/ha from Urea, TSP, MOP, and gypsum, respectively (BARI (Bangladesh Agricultural Research Institute), 2019). Fertilizers were calculated on basis of pot size and applied into the soil as a basal dose. Fifteen mungbean seeds were sown separately in each pot at the desired moisture level after sterilizing with 0.1% mercuric chloride solution for 2 min, and subsequently washing with tap water (Saminathan, 2013). Each pot was irrigated with a watering can in the evening, as needed. Excess or stagnant water was effectively drained away by leaking the pots at the bottom. Numerous intercultural operations like thinning, weeding were conducted to improve growth and development of the mungbean following seedling establishment. To ensure optimal growth and development, the first weeding occurred at 20 DAS followed by second at 35 DAS. Thinning was done gradually in accordance with plant development, with the goal of retaining three homogenous plants for harvesting. Plant protection measures were taken as necessary such as Caterpillars infested the pots, which were successfully eradicated by hand spraying Ripcord (15 g/L Cypermethrin) twice at a concentration of 20 ml/500 ml of clean water. Various doses of GA3 (0, 100, 200, and 300 ppm) were applied as foliar sprays at 30 and 40 DAS according to treatment specifications. The crop was harvested manually from each pot on 31 May, 2018 at full maturity. The harvested crop from each plot was bundled together, tagged and transported to the threshing floor. Mungbean seeds were harvested, threshed and cleaned with care.
2.7. Measurement of plant water status
The shoots of mungbean were separated at 45 DAS and fresh weights of fully expanded third trifoliate leaves and stems were taken. The plant parts were immersed in distilled water for 24 h at room temperature in the dark. The turgid weights of those plant parts were measured, and then all of the materials were oven-dried at 80°C for 72 h to obtain dry weight. The fresh, turgid and dry weights of the plant segments were used to calculate the relative water content (RWC), water saturation deficit (WSD), water retention capacity (WRC) and water uptake capacity (WUC) according to Saneoka et al. (1995):
2.8. Data collection
At the harvesting stage, plant height was measured from the base to the top of the plant. Three plant samples were taken from each pot and means were calculated. The number of pods per pot was manually counted at harvest, and their average was calculated. The length of pods was measured from the base to the tip using a measuring tape. The number of grains in each pod was counted. In that case, each pod from each pot was removed and the means were computed. The number of grains was counted from 10 randomly selected pods of three plants from each plot, and the mean number was expressed per pod. A total of hundred cleaned sun dried grains were counted and weighted using an electrical balance. The grains were separated by threshing them in pots. The sample was then sun dried and weighed. Grain yield was adjusted to a moisture content of 12% (Hellevang, 1995), and grain yield per plant was calculated by dividing the value by the number of plants in each pot. The harvest index was calculated by dividing the seed yield and biological yield (seed + stover yield) from the same area and multiplying the result by 100 (Gardner et al., 1985).
2.9. Functional relationship
Grain yield and GA3 levels were analyzed using regression analysis (Payne et al., 2001), and the relationship were quadratic by polynomial function defined as Y = a + bx − cx2. There are four coefficients of regression in this equation: Y = dependent variable (yield attributes), a = Intercept (constant), b and c = Regression coefficients, and x = Independent variable (nutrient element). The polynomial regression equation developed by Gomez and Gomez (1984) was used to calculate yield the optimum level of GA3 for maximum grain yield with the help of formula as given below:
Grain yield was also predicted against optimum GA3 levels using a functional relationship that was developed.
2.10. Statistical analysis
The statistical analysis of recorded data was performed using computer-run statistical package of MSTAT-C (version 2.1, Michigan State University, United States), and the results were presented as mean ± SE (n = 3). Subsequently, Duncan’s Multiple Range Test (DMRT) was employed at a 5% level of probability for determining the significance among treatments (Gomez and Gomez, 1984).
3. Results and discussion
3.1. Water relations of mungbean
3.1.1. Relative water content
The relative water content (RWC) of leaves is an important characteristic, which has significant relationship with yield and plant stress tolerance (Merah, 2001; Almeselmani et al., 2012). The results revealed that GA3 foliar application in different concentrations and frequencies significantly increased RWC in different parts of the mungbean (leaf and stem; Table 3). The highest RWC (85.52%) in mungbean was recorded for twice application of GA3 (200 ppm) compared to the control (75.06%). This treatment combination resulted in 9.94% increase in RWC than control. These findings are consistent with the conclusion made by Hossain et al. (2015) who inferred that GA3 application at different growth stages had a positive effect on relative water content of chick pea (Lobato et al., 2009). Likewise, spraying of GA3 increased RWC in plant tissues causing cell expansion and diluting the sugars in the tissues (Salisbury and Ross, 1996). The hydrolysis of starch into reducing sugars caused by GA3 results in sugar accumulation which tend to improve the water balance and osmotic potential of gerbera cut flowers. Moreover, it has also been inferred that GA3 application decreasing transpiration in cut flowers which might be ascribed to higher RWC and productivity (Emongor, 2004). In this study, twice foliar spraying of GA3 remained more effective than single spray and these findings are in concurrence with number of previously reported research findings (Aldesuquy and Ibrahim, 2001; Ashraf and Foolad, 2007).
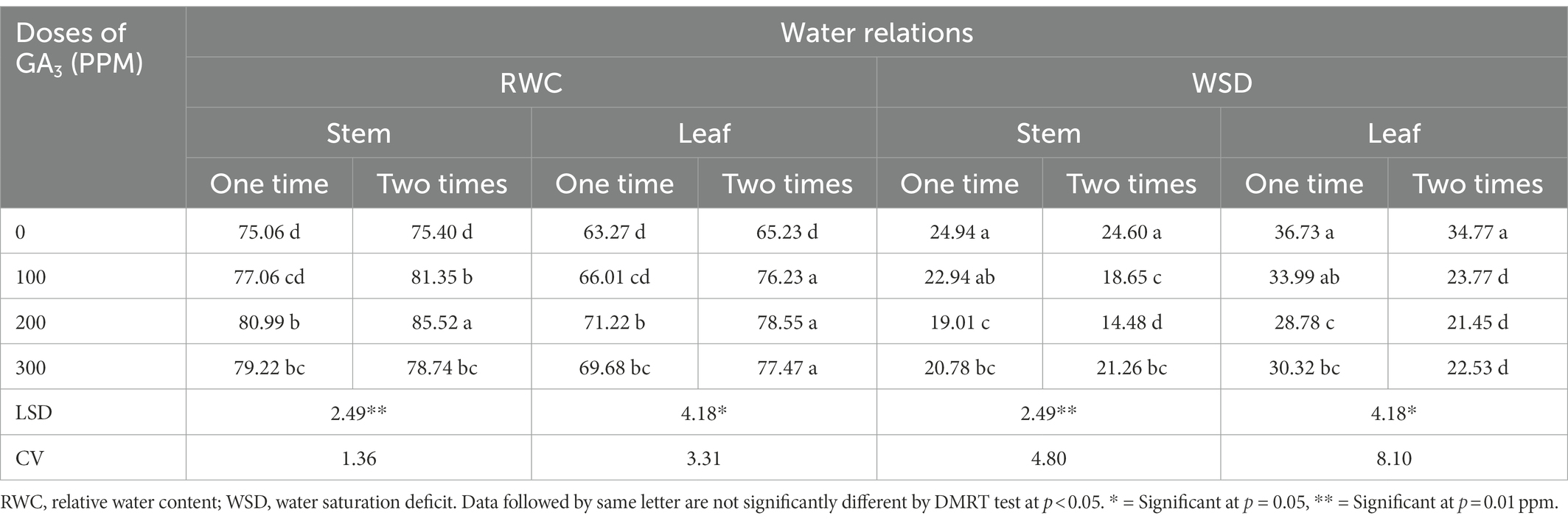
Table 3. The RWC and WSD in different plant parts of mungbean in response to exogenously applied different concentrations of GA3 at varying frequencies.
3.1.2. Water saturation deficit
Water saturation deficit (WSD) exhibits an inverse relationship with RWC and indicates the degree of water deficit in plants (Islam, 2001). The application of GA3 at various growth stages significantly reduced the water saturation deficit (WSD) in leaf and stem of mungbean (Table 3). The highest WSD (36.73) was found in the leaves and stems under control conditions (without application of GA3), while the lowest (21.45) was found after foliar spraying GA3 at 200 ppm levels at 30 and 40 DAS. The lowest WSD values indicated relatively vigorous plant growth, which resulted in significantly higher mungbean yield. Previously, it was reported that GA3 application as seed priming remained effective in increasing seed water uptake, germination and early seedling growth in sugar beet under salt stress (Jamil and Rha, 2007). Besides that, GA3 counteracted the negative effects of saline by increasing water uptake in Vicia faba seeds (Shukry and El-Bassiouny, 2002). Furthermore, GA3 increased water absorption in plant tissue, and reduced water saturation deficit which aided in boosting growth traits and grain yield of food legumes (Salisbury and Ross, 1996).
3.1.3. Water retention capacity
Water retention capacity (WRC) is a turgid weight: dry weight ratio which depicts the capacity of a plant cell to retain water at a specific growth stage and thus it can be used as a critical and reliable indication of plant growth and health (Islam, 2001). The WRC of mungbean leaves and stems increased significantly in our trial as a result of foliar application of GA3 and spraying frequencies (Table 4). The WRC of mungbean grew up to 200 ppm when GA3 was sprayed on it, and thereafter it dropped significantly. Spraying GA3 at 30 and 40 DAS resulted in higher WRC values than one time spraying (at 30 DAS) for all treatments, including control. The highest WRC (17.24) was observed after two sprayings of GA3 at a concentration of 200 ppm, while the lowest (7.54) was evidenced at control and the rate of increment was approximately 129% over control. According to Hossain et al. (2015), GA3 application gradually improved water retention capacity of cowpea. In addition, GA3 remained effective in increasing K uptake which improved water and nutrient transport through the xylem and thus influenced a variety of biochemical and physiological traits such as photosynthesis, respiration, protein synthesis, cell extension, and wall thickness and stability (Abbas et al., 2011). Furthermore, it tends to activate various enzymes and facilitates adenosine triphosphate (ATP) biosynthesis, which in turn regulates the rate of photosynthesis (Atkin and Macherel, 2009). The plant transport system uses gibberellin to promote growth by stimulating cells for quick division, as well as elongation which is followed, by hydrolysis of starch to sugar, resulting in a reduced water potential and allowing water to enter the cell. The growth promoting effect of GA3 on germination and seedling growth may be attributed to its indirect effect on membrane permeability to water (Shivanna et al., 2007) as water uptake was significantly improved by GA3 exogenous application.
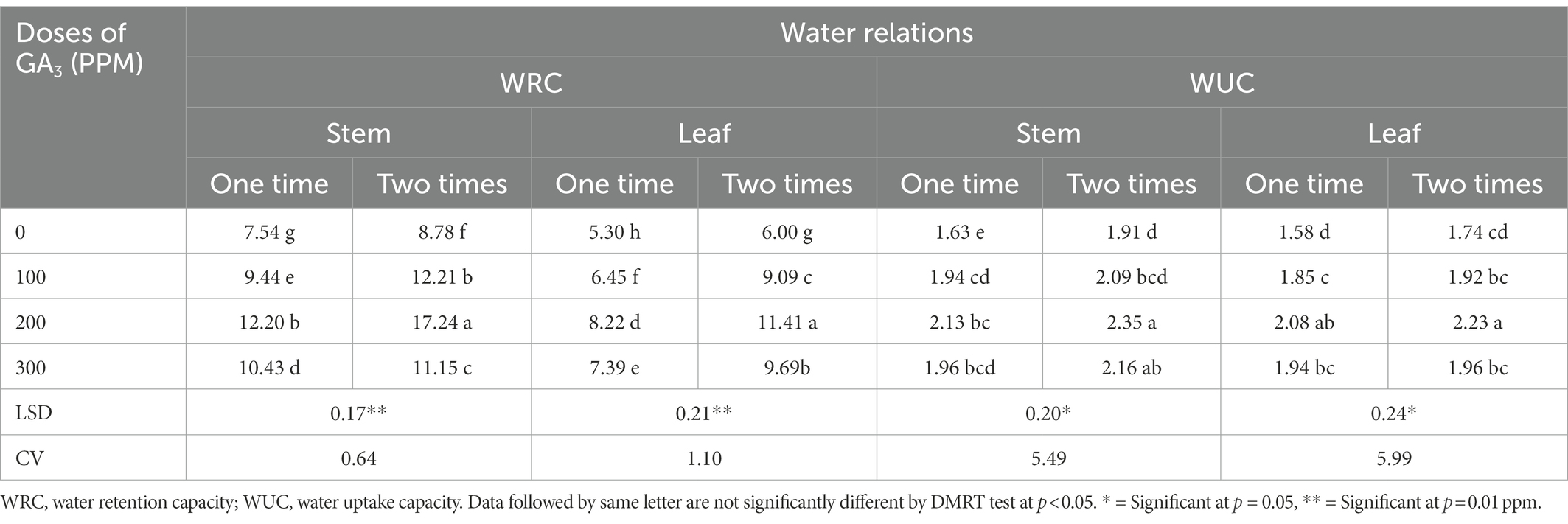
Table 4. The WRC and WUC in different plant parts of mungbean in response to exogenously applied GA3 in different doses and frequencies.
3.1.4. Water uptake capacity
The WUC measures the capacity of plant cells to absorb water per unit dry weight at a specific growth stage of plants (Islam, 2001). The GA3 foliar sprays significantly increased the WUC of mungbean plants. The dose of 200 ppm of GA3 applied twice at 30 and 40 DAS resulted in the highest WUC (Table 4). These findings are in concurrence with previously reported conclusions where GA3significantly increased the WUC in wheat (Aldesuquy and Ibrahim, 2001), mustard (Banyal and Rai, 2003), and cut flowers (Emongor, 2004). According to Salisbury and Ross (1996), GA3 effectively increased water uptake in plant tissue by lowering the water potential of the cells and allowing water to enter the cells more quickly. Furthermore, with the application of GA3, the NPK content in the leaves and pods increased significantly, which could be a sign of increased water uptake in pea plant (Fouda, 2017).
3.2. Morphological and yield components of mungbean
3.2.1. Plant height
The plant height of mungbean was significantly affected by the GA3 application at different frequencies, while 200 ppm dose applied twice remained unmatched by producing the tallest (50.0 cm) plant (Table 5). GA3 application near the terminal bud of the plant stimulated growth and development. It might be inferred that taller plants by foliar application of GA3might have triggered cell division which led to increased plant height. Similar growth stimulating effects were observed in mungbean (Abdel et al., 2011; Keykha et al., 2014; Islam et al., 2017a), chickpea (Iqbal et al., 2001), cowpea (Emongor, 2007), and field peas (Singh et al., 2015). Likewise, the growth promoting effect of GA3 on the plant height has also been reported in soybean (Leite et al., 2003), black gram and horse gram (Chauhan et al., 2009), and linseed (Ayala-Silva et al., 2005; Rastogi et al., 2013). It has been suggested that the impact of GA3 may be due to increased internode length as a result of increased cell multiplication and cell number. In this study, GA3 had pronounced stimulatory effect on plant water status, such as RWC, RUC and WRC (Tables 3, 4), which could be due to improved nutrient transport through the xylem, influencing photosynthesis, respiration, and cell extension, and thus increasing plant height. The results revealed that two applications of GA3 are preferable than one in terms of mungbean plant height which might be attributed to GA3 critical role in regulating cell division in meristematic tissues, resulting in the creation ion of new cells and accelerated plant development (Emongor, 2007). It has been reported earlier that plant growth and development was regulated by various exogenous and endogenous factors, including the growth regulators (Taiz and Zeiger, 2010). The GA3 are found in those tissues that are elongating the most rapidly, such as stems, petioles, and flower inflorescences (Ayala-Silva et al., 2005). Ross et al. (2003) noted that GA3 promotes stem elongation by increasing the physiological levels of auxin, either by increasing auxin production or decreasing the destruction of auxin.
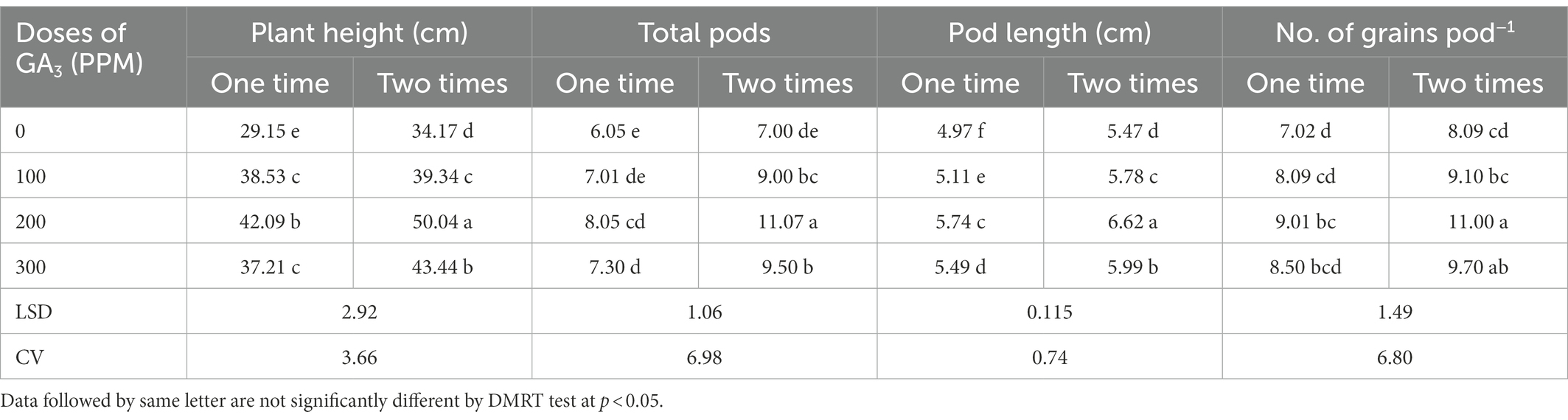
Table 5. Reproductive yield attributes of mungbean as influenced by exogenously applied GA3 in different concentrations and frequencies.
3.2.2. Total pods per plant
The results of this trial explored that the GA3 spraying at different doses and frequencies had a significant effect on the total pods plant−1 in mungbean (Table 5). The total pods increased up to 200 ppm and then decreased significantly. At 100 and 200 ppm of GA3, two applications increased total pods plant−1 by 28 and 37%, respectively, compared to single application. The maximum number of pods plant−1 (11.07) were recorded at 200 ppm GA3 with two applications, while the minimum number of pods plant−1 (6.053) was recorded for control treatment. These findings are consistent with those reported for mustard (Khan et al., 1988), pea (Bora and Sarma, 2006; Fouda, 2017), cowpea (Emongor, 2007), and mungbean (Islam et al., 2021b). It has been inferred that GA3 @ 100 ppm produced the highest pods per plant (8.49% increase over control) in mungbean (Abdel et al., 2011), while @ 200 ppm produced the highest pods per plant in peas (Singh et al., 2015). This could be attributed to crop genetic variability. The exogenous GA3 application increased vegetative and reproductive growth parameters especially total pods per plant as per data recordings of this study which is consistent with the findings of Ilias et al. (2007). Through intermingling with other growth promoting hormones in mungbean, the GA3 appears to have demonstrated the performance of leaf growth and reproductive organs (Abdel et al., 2011). On the contrary, it has been determined that GA3 decreased the total pods per plant in common bean (Emongor, 2002).
3.2.3. Pod length
The length of pods varied significantly due to exogenous application of GA3 in various concentrations and frequencies. The dose of 200 ppm applied twice and control treatment produced the longest (6.617 cm) and the shortest (4.97 cm) pod lengths, respectively (Table 5). The pod enlargement might be due to active cell division induced by exogenously applied GA3 (Inglese et al., 2005). Similar findings have been reported for garden pea (Singh et al., 2015) and mungbean (Islam et al., 2021b). According to Emongor (2007), the application of GA3 to cowpea plants significantly increased pod length. The GA3 spraying significantly increased pod lengths in mungbean (Bhadra, 2004) and rape (Ancha and Morgan, 1996). In contrast, Abdel et al. (2011) inferred that the increase in pod length (3.71% over control) with the application of GA3 in mungbean was statistically significant with control treatment. Emongor (2002) also stated that GA3 treatments had no effect on the pod lengths of bean plant. The GA3 treatment at 50 and 100 ppm, on the other hand, resulted in a significant reduction in pea yield traits (El-Shraiy and Hegazi, 2009). These contrasting findings might be interpreted by varying plant-growth regulator-environment interaction which resulted in contradictory findings (Hedden and Thomas, 2012).
3.2.4. Grains per pods
The different concentrations of foliar applied GA3 at different frequencies significantly influenced the number of grains pod−1 of mungbean. The maximum number of grains pod−1 (11.07) was obtained for twice application of GA3 in200 ppm concentration, while the minimum number (7.05) was acquired for the control treatment (Table 5). Nonetheless, the increase in grains pod−1 exaggerated by GA3 was significantly greater in dual spraying during the growing season than in single spraying. GA3 @ 200 ppm application resulted in the highest number grains pod−1 in mungbean (Islam et al., 2021b), and in garden pea (Singh et al., 2015). Rastogi et al. (2013) confirm that exogenous GA3 application significantly increased grain yield in linseed. Cowpea yielded similar results (Emongor, 2007). Gibberellin stimulates flowering and can cause fruit set by initiating fruit growth, which is followed by pollination (Gupta and Gupta, 2005). Gibberellin has been shown to play a significant role in tomato fruit development and gene expression (Jong et al., 2009). The GA3 also played an important role in stem elongation and leaf expansion, as well as reducing maturation time, and increasing flower and fruit set in food legumes (Roy and Nasiruddin, 2011).
3.2.5. 100-grains weight
The 100-grains weight was also influenced by the GA3 doses and frequency of exogenous application. The highest 100-grain weight (3.78 g) was obtained when GA3 was applied twice at a concentration of 200 ppm, while the lowest corresponding value (2.79 g) was obtained for control treatment (Table 6). However, GA3 @ 200 ppm applied as two foliar sprays remained unmatched by resulting in the greatest 100-seed weight (35.48%). Similar to our findings, Groot et al. (1987) found that the exogenous application of GA3 increased grain weight gradually. As reported by Bora and Sarma (2006), the seed index was gradually increased with the foliage applied GA3 up to 250 ppm. Similar trends have also been reported in mungbean (Bhadra, 2004; Islam et al., 2021b) and cowpea (Emongor, 2007). It has been reported that GA3 application exogenously contributed to increase the endogenous GA3 level in developing grains that might have increased the 100 seed weight (Nagar et al., 2021). GA3 also enhances the grain filling duration which ensures long time transportation of photo-assimilates to the grain (sink), consequently increases the 100 grain weight (Wang et al., 2006).
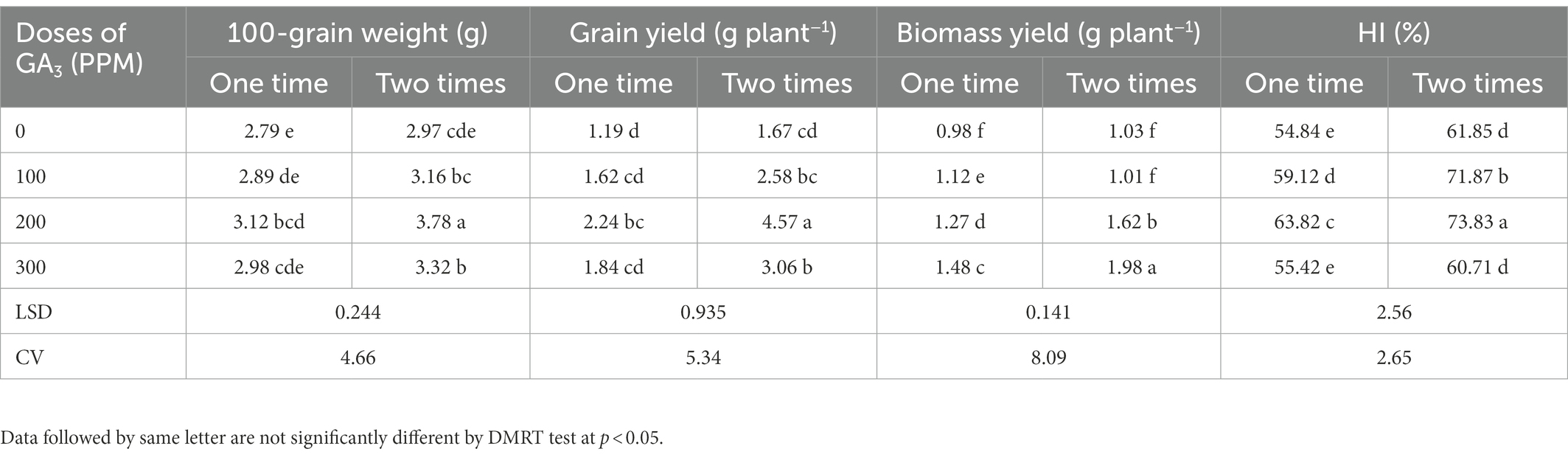
Table 6. The 100-grain weight, grain and biomass yield, and harvest index of mungbean in response to foliage applied GA3 in varying concentrations and frequencies.
3.3. Grain and biomass yield, and harvest index
3.3.1. Grain yield per plant
The application of GA3 at various concentrations and frequencies significantly increased grain yield plant−1 which ranged from 1.19 to 4.57 g plant−1 (Table 6). The maximum and minimum grain yields plant−1 were recorded for foliar applied GA3 @ 200 ppm in two sprays and the control treatments, respectively. The findings also revealed that GA3 remained effective in increasing the grain yield up to a certain concentration (200 ppm), and that increasing spraying frequencies (two times) always promoted grain yield more than one time spraying. These findings corroborate with those of previously reported results where GA3 foliar application significantly promoted seed development and yield in mungbean (Bhadra, 2004; Abdel-Mouty and El-Greadly, 2008; Keykha et al., 2014; Islam et al., 2021b). Exogenous application of GA3 also remained effective in increasing grain yield of black gram (Islam et al., 2010), field peas (Swain et al., 1997; Bora and Sarma, 2006), cowpea (Emongor, 2007), and linseed (Faizanullah et al., 2010; Rahimi et al., 2011; Rastogi et al., 2013). Our results revealed that the GA3 improved the yield attributes such as the plant height, total pods plant−1, pod length, number of grains pod−1 and 100-grains weight which ultimately increased the grain yield of mungbean. Rastogi et al. (2013) found that linseed seed yield was strongly related to the plant height, and Copur et al. (2010) inferred that plant height increment indirectly affects seed yield in cotton via number of nodes and sympodia branches development. Foliar application of GA3 at pre-flowering, increased sunlight trapping, which increased mustard dry matter and grain yield (Khan et al., 1988). These improved traits by foliar applied GA3 tend to increase assimilates production which is directly related to yield. The application of GA3 at 10−6 M on 30 days old plants increased mustard seed yield (Hayat et al., 2001), but GA3 100 ppm concentration produced the highest soybean seed yield (Maske et al., 1997; Deotale et al., 1998).
3.3.2. Biomass yield
The frequency and doses of GA3 applied to the foliage had a significant impact on the biomass yield of mungbean (Table 6). GA3 @ 300 ppm produced the highest biomass yield as compare to other doses of GA3, and two times application exhibited higher biomass yield than one time application. Nonetheless, the maximum biomass yield (1.98 g plant−1) was obtained after twice applying GA3 @ 300 ppm, while the minimum biomass yield (0.98 g plant−1) was obtained for the control treatment. It was reported in many studies that GA3 enhanced the plant growth by increasing fresh and dry weights of leaves, number of leaves per plant (Azab, 2018; Iftikhar et al., 2020), plant height, biomass, stem weight, stem diameter, and the number of stems (Ullah et al., 2017). Previously, a significant effect of GA3 on the biological yield, stover yield and harvest index was observed in mungbean (Beall et al., 1996; Uddin, 1999; Alam et al., 2018; Rahman et al., 2018).
3.3.3. Harvest index (%)
The frequent application of GA3 gradually influenced the harvest index (HI) of mungbean. Application of GA3 gradually increased the HI up to 200 ppm concentration, and this level of GA3 showed the highest HI, while the lowest was in 0 ppm (control). On the other hand, frequency of application had significant effect on the HI. However, the highest harvest index (73.83%) was obtained from two applications of GA3 @ 200 ppm, while the lowest (54.84%) was obtained from the control (Table 6). The GA3 application on a regular basis had a significant impact on mungbean harvest index, as proven in mungbean (Uddin, 1999; Alam et al., 2018), and bean (Beall et al., 1996). The present finding are conformity with Verma et al. (2016) where they found 12.79% increment of HI due to addition of 150 ppm GA3 over control condition. Contrariwise, this finding is not consistent with the results of Nagar et al. (2021) who reported that GA3 is unable to increase the HI remarkably of wheat.
3.4. Coefficient of correlation
The varying doses and frequency of GA3 foliar application had a significant influence on the correlation coefficient of yield and yield components of mungbean (Figure 1). Positive correlation was found between the plant height and total pods plant−1 (p < 0.01). In addition, significantly positive correlation existed between the total pods plant−1, pod length, grains plant−1, hundred grain weight and grain yield. The hundred grain weight and grain yield were positively correlated with the pods plant−1 and grains plant−1. Similar to the current study, a positive correlation between harvest mode and seed yield was observed in mungbean (Malik et al., 1986; Khan, 1988; Natarajan et al., 1988; Patil and Deshmukh, 1988; Singh et al., 1988; Naidu and Satyanarayana, 1993), and in mash (Vigna mungo L.; Patil and Narkhede, 1987; Ghafoor et al., 1990). A significant positive correlation between plant height and seeds pod−1 was found in mungbean (Naidu and Satyanarayana, 1993), lending support to the current study.
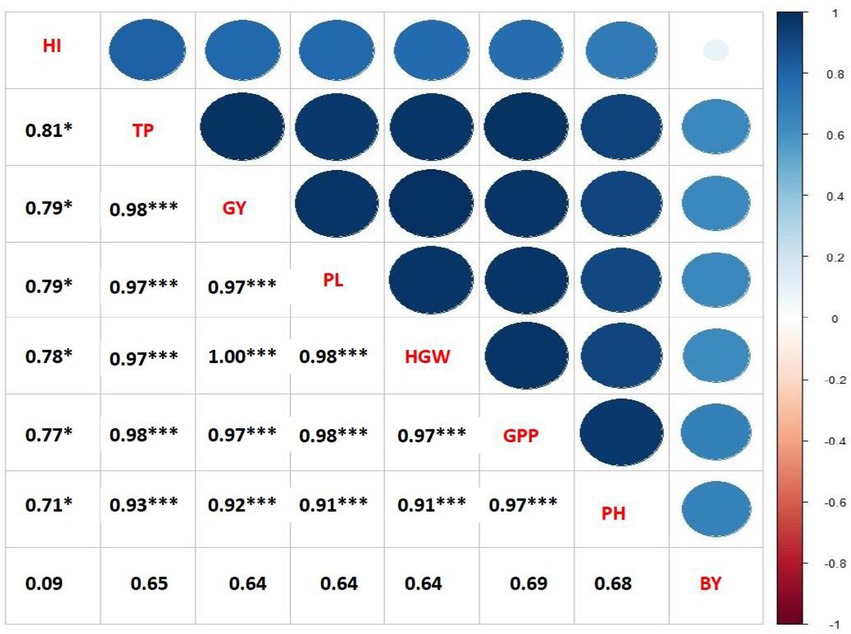
Figure 1. Correlation coefficients of seed yield per plant with other yield contributing characteristics of mungbean under different concentrations and frequencies of GA3 (PH, plant height; TP. total pods plant−1; PL. pod length; GPP, grains pod−1; HGW, hundred grains weight; GY, grain yield).
3.5. Functional relationship between grain yield and applied GA3 level
The functional equation fits the grain yield data as well as it was influenced by the different levels and frequencies of GA3 application (Figure 2). Estimated optimum doses of GA3 for maximum grain yield and their corresponding yields were calculated using the two regression equations (one and two times application of GA3; Table 7). The coefficients of determination R2 = 87 and 76, respectively, expressed the yield response of mungbean to GA3 application of approximately 87 and 76 per cent. The application of 1 ppm GA3 increased grain yield by 0.008 and 0.024 g plant−1, respectively.
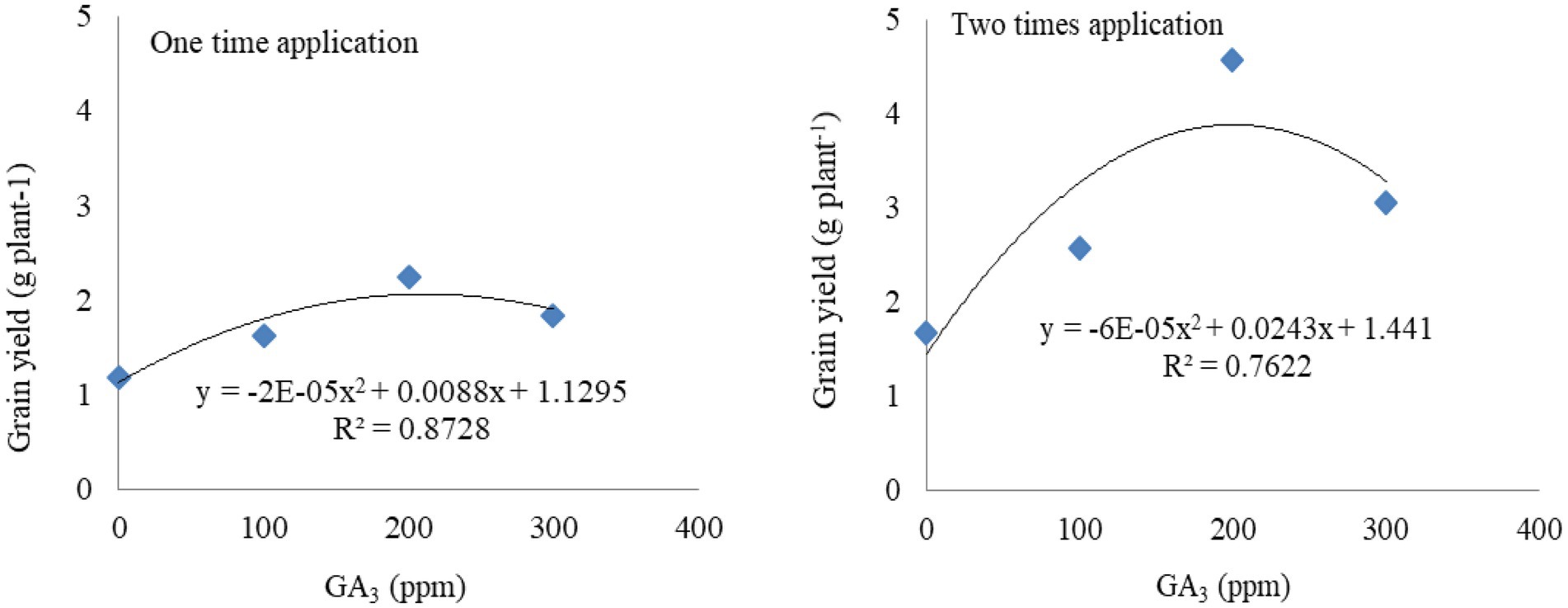
Figure 2. Functional relationship between GA3 (concentrations and frequencies) and grain yield of mungbean.
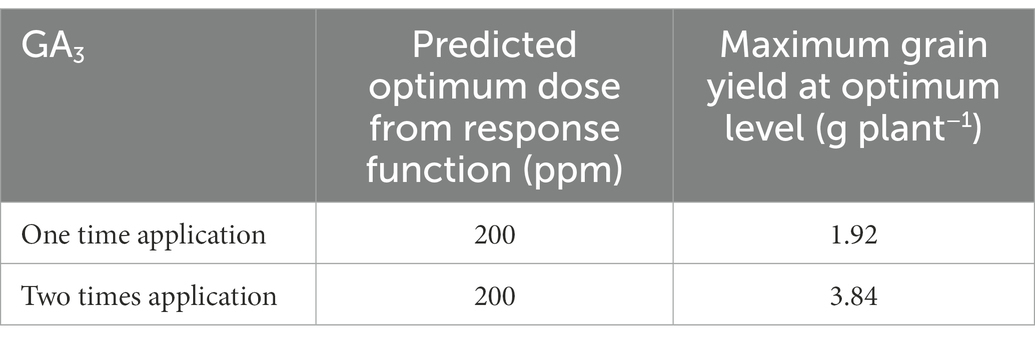
Table 7. The impact of different doses of GA3 applied at varying frequencies on grain yield of mungbean.
3.6. Optimum level of GA3
Based on the developed function, the estimated optimum dose of GA3 was 200 ppm when applied once and twice, and the maximum grain yield was 1.92 and 3.84 g plant−1, respectively (Table 7). It implies that the grain yield was increased with increasing GA3 dose up to 200 ppm, respectively, and that further application of higher doses tend to reduce mungbean grain yield.
4. Conclusion
Based on recorded findings, it is inferred that results remained in concurrence with the postulated hypothesis remained asGA3 stimulated various morpho-physiological parameters of mungbean, including water relations (RWC, WSD, WRC, and WUC), growth and yield contributing characteristics (plant height, total pods plant−1, pod length, grains per pod, total grains plant−1, and 100-grains weight) of mungbean. Moreover, the GA3 foliar application significantly increased grain yield and biomass productivity along with harvest index of mungbean. According to the findings, the exogenous application of GA3 in 200 ppm concentration twice during the pre-flowering and post-flowering stages preserved critical water status and yield attributes, resulting in significantly higher mungbean grain yield. As a result, it may be recommended for general use by mungbean growers; however, additional in-depth trials for optimizing GA3 doses and application frequency for mungbean are recommended.
Data availability statement
The original contributions presented in the study are included in the article/supplementary materials, further inquiries can be directed to the corresponding authors.
Author contributions
MSI and MKH prepared the manuscript, analyzed data, and developed maps and Figures. MSI conceptualized the study, designed the methodology, and reviewed the manuscript. MKH and MRI developed code for data analysis, maps and Figures. MKC, MHP, MAI, KR, RI, WS, MK, LL, and AES attained funding for project conduction and reviewed the manuscript. All authors contributed to the article and approved the submitted version.
Funding
This research was funded by the Researchers Supporting Project number (RSP2023R390), King Saud University, Riyadh, Saudi Arabia. The current work was funded by Institute of Research and Training (IRT) Project number 4829, HSTU, Dinajpur, Bangladesh.
Acknowledgments
The authors extend their appreciation to Researchers Supporting Project number (RSP2023R390), King Saud University, Riyadh, Saudi Arabia. We thank Shafiqul Islam Sikdar, Chairman, Department of Agronomy, HSTU, Dinajpur, Bangladesh for the help in the experimentation.
Conflict of interest
The authors declare that the research was conducted in the absence of any commercial or financial relationships that could be construed as a potential conflict of interest.
Publisher’s note
All claims expressed in this article are solely those of the authors and do not necessarily represent those of their affiliated organizations, or those of the publisher, the editors and the reviewers. Any product that may be evaluated in this article, or claim that may be made by its manufacturer, is not guaranteed or endorsed by the publisher.
References
Abbas, G., Aslam, M., Malik, A. U., Abbas, Z., Ali, M., and Hussain, F. (2011). Potassium sulfate effects on growth and yield of mungbean (Vigna radiata L.) under arid climate. Int. J. Agric. Appl. Sci. 3, 72–75.
Abdel, C. G., Murad, I., and Al-Rawi, T. (2011). Response of mungbean (Vigna radiata L., Wilczek) to gibberellic acid (GA3) rates and varying irrigation frequencies. Int. J. Biosci. 1, 85–92.
Abdel-Mouty, M. M., and El-Greadly, N. H. M. (2008). The productivity of two okra cultivars as affected by gibberellic acid, organic N, rock phosphate and feldspar application. J. Appl. Sci. Res. 4, 627–636.
Abu-Zahra, T. R. (2010). Berry size of Thompson seedless as influenced by the application of gibberellic acid and cane girdling. Pak. J. Bot. 42, 1755–1760.
Alam, M. J., Ahmed, K. S., Sultana, A., Firoj, S. M., and Hasan, I. M. (2018). Ensure food security of Bangladesh: analysis of post-harvest losses of maize and its pest management in stored condition. J. Agric. Eng. Food Technol. 5, 26–32.
Aldesuquy, H. S., and Gaber, A. M. (1993). Effect of growth regulators on Vicia faba plants irrigated by sea water: leaf area, pigment content and photosynthetic activity. Biol. Plant. 35, 519–527. doi: 10.1007/BF02928026
Aldesuquy, H. S., and Ibrahim, A. H. (2001). Interactive effect of seawater and growth bio-regulators on water relations, absicisic acid concentration and yield of wheat plants. J. Agron. Crop Sci. 187, 185–193. doi: 10.1046/j.1439-037x.2001.00522.x
Almeselmani, M., Saud, A., Al-Zubi, K., Abdullah, F., Hareri, F., Nassan, M., et al. (2012). Physiological performance of different durum wheat varieties grown under rainfed condition. Global J. Sci. Front. Res. 12, 55–63.
Ancha, S., and Morgan, D. G. (1996). Growth and development of the pod wall in spring rape as related to the presence of seeds and exogenous phytohormones. J. Agric. Sci. 127, 487–500. doi: 10.1017/S0021859600078710
Ashraf, M., and Foolad, M. R. (2007). Roles of glycinebetaine and proline in improving plant abiotic stress resistance. Environ. Exp. Bot. 59, 206–216. doi: 10.1016/j.envexpbot.2005.12.006
Atkin, O. K., and Macherel, D. (2009). The crucial role of plant mitochondria in orchestrating drought tolerance. Ann. Bot. 103, 581–597. doi: 10.1093/aob/mcn094
Ayala-Silva, T., Akin, D. E., Foulk, J., and Dodd, R. B. (2005). Effect of growth regulators on yield and fiber quality and quantity in flax (Linum usitatissimum L.). PGRSA Q. 33, 90–100.
Azab, E. S. (2018). Seed pre-soaking on gibberellic acid (GA3) enhance growth, histological and physiological traits of sugar beet (Beta vulgaris L.) under water stress, Egyptian. J. Agron. 40, 119–132. doi: 10.21608/AGRO.2018.2944.1095
Banyal, S., and Rai, V. K. (2003). Reversal of osmotic stress effects by gibberellic acid in Brassica campestris. Recovery of hypocotyl growth, protein and RNA level in the presence of GA. Physiol. Plant. 59, 111–114. doi: 10.1111/J.1399-3054.1983.TB06580.X
BARI (Bangladesh Agricultural Research Institute) (2019). Krish Projukti Hatboi (Handbook on Agro-Technology), 8th Edn. Bangladesh Agricultural Research Institute, Gazipur, Bangladesh.
Beall, F. D., Young, E. C., and Pharis, R. P. (1996). Far-red light stimulates internode elongation, cell division, cell elongation and gibberellin levels in bean. Can. J. Bot. 74, 743–752. doi: 10.1139/b96-093
Bhadra, A. K. (2004). Effect of GA3 on growth and yield attributes of mungbean. M.S. thesis. Mymensingh, Bangladesh: Dept. of Crop Botany, Bangladesh Agricultural University.
Birnberg, P. R., and Brenner, M. L. (1987). Effect of gibberellic acid on pod set in soybean. Plant Growth Regul. 5, 195–206. doi: 10.1007/BF00024695
Black, C. A. (1965). Methods of Soil Analysis Part 1. Agronomy Monograph 9. Madison, WI: ASA Madison.
Bora, R. K., and Sarma, C. M. (2006). Effect of gibberellic acid and clycocel on growth, yield and protein content of pea. Asian J. Plant Sci. 5, 324–330. doi: 10.3923/ajps.2006.324.330
Bray, R. H., and Kurtz, L. T. (1945). Determination of total, organic, and available forms of phosphorus in soils. Soil Sci. 59, 39–46. doi: 10.1097/00010694-194501000-00006
Bremner, J. M., and Mulvaney, C. S. (1982). Methods of Soil Analysis. Part 2. Chemical and Microbiological Properties. Madison, WI: Soil Science Society of America. pp. 595–624.
Chakrabarti, N., and Mukherji, S. (2002). Effect of phytohormone pretreatment on metabolic changes in Vigna radiata under salt stress. J. Environ. Biol. 23, 295–300.
Chakraborti, N., and Mukherji, S. (2003). Effect of phytohormone pretreatment on nitrogen metabolism in Vigna radiata under salt stress. Biol. Plant. 46, 63–66. doi: 10.1023/A:1022358016487
Chauhan, J. S., Tomar, Y. K., Singh, I. K., Ali, S., and Debarati, T. (2009). Effects of growth hormones on seed germination and seedling growth of black gram and horse gram. J. Am. Sci. 5, 79–84.
Copur, O., Demirel, U., and Karakus, M. (2010). Effects of several plant growth regulators on the yield and fiber quality of cotton (Gossypium hirusutum L.). Not. Bot. Horti. Agrobot. Cluj Napoca 38, 104–110. doi: 10.15835/nbha3834588
Das, G. P., Das, D., and Mukherji, S. (1994). Role of phytohormones in the reversal of stressinduced alteration in growth turgidity and proline accumulation in mungbean (Vigna radiata L. Wilczek) plants. Indian J. Biol. 26, 343–348.
Deotale, R. D., Maske, V. G., Sorte, N. V., Chimurkar, B. S., and Yerne, A. Z. (1998). Effect of GA and NAA on morphological parameter of soybean. J. Soil Crops 8, 91–94.
El-Shraiy, A. M., and Hegazi, A. M. (2009). Effect of acetylsalicylic acid, indole-3- bytric acid and gibberellic acid on plantgrowth and yield of pea (Pisum sativum L.). Aust. J. Basic Appl. Sci. 3, 3514–3523.
Emongor, V. E. (2002). Effect of benzyladenine and gibberellins on growth, yield and yield components of common bean (Phaseolus vulgaris). UNISWA Res. J. Agric. Sci. Technol. 6, 65–72. doi: 10.4314/uniswa-rjast.v6i1.4648
Emongor, V. E. (2004). Effects of gibberellic acid on postharvest quality and vaselife life of gerbera cut flowers (Gerbera jamesonii). J. Agron. 3, 191–195. doi: 10.3923/ja.2004.191.195
Emongor, V. E. (2007). Gibberellic acid (GA3) influence on vegetative growth, nodulation and yield of cowpea (Vigna unguiculata L. Walp). J. Agron. 6, 509–517. doi: 10.3923/ja.2007.509.517
Faizanullah, A., Bano, A., and Nosheen, A. (2010). Role of plant growth regulators on oil yield and biodiesel production of linseed (Linum usitatissimum L.). J. Chem. Soc. Pak. 32, 668–671.
Fouda, K. F. (2017). Effect of phosphorus level and some growth regulators on productivity of Faba bean (Vicia Faba L.). Egypt. J. Soil Sci. 57, 73–87. doi: 10.21608/ejss.2017.3593
Fox, R. L., Olson, R. A., and Rhoades, H. F. (1964). Evaluating the sulfur status of soil by plants and soil tests. Soil Sci. Am. Proc. 28, 243–246. doi: 10.2136/sssaj1964.03615995002800020034x
Gardner, F. P., Pearce, R. B., and Mitchell, R. L. (1985). Physiology of Crop Plants. Iowa: Iowa State Univ. Press. p. 66.
Ghafoor, A., Zubair, M., and Malik, B. A. (1990). Path analysis in mash (Vigna mungo L.). Pak. J. Bot. 22, 160–167.
Gomez, K. A., and Gomez, A. A. (1984). Statistical Procedures for Agricultural Research. 2nd Edn. Singapore: A Wiley Interscience Publication, John Wiley and Sons, 302–307.
Groot, S. P. C., Bruinsma, J., and Karssen, C. M. (1987). The role of endogenous gibberellin in seed and fruit development of tomato: studies with a gibberellin deficit mutant. Physiol. Plant. 71, 184–190. doi: 10.1111/j.1399-3054.1987.tb02865.x
Gupta, N. K., and Gupta, S. (2005). “Growth regulators” in Plant Physiology. eds. N. K. Gupta and S. Gupta (New Delhi, India: Oxford and IBH Publishing), 286–349.
Halevy, A. H., and Mayak, S. (1981). Senescence and postharvest physiology of cut flowers, part 2. Hortic. Rev. 3, 59–143. doi: 10.1002/9781118060766.ch3
Haque, M. M., and Haque, M. S. (2002). Effects of gibberllic acid (GA3) on physiological contributing characters of mungbean (Vigna radiate L.). Pakistan. J. Biol. Sci. 5, 401–403. doi: 10.3923/pjbs.2002.401.403
Hayat, P., Gupta, R. K., and Singh, S. S. (2001). Effect of planofix (NAA) and GA3 on the yield and quality of mustard. Pesticides J. 25, 10–12.
Hedden, P., and Thomas, S. G. (2012). Gibberellin biosynthesis and its regulation. Biochem. J. 444, 11–25. doi: 10.1042/BJ20120245
Hellevang, K. J. (1995). Grain moisture content effects and management. Department of Agricultural and Biosystems Engineering, North Dakota State University. Available online: http://www.ag.ndsu.edu/pubs/plantsci/crops/ae905w.htm (Accessed September 1, 2022).
Hore, J. K., Paria, M. C., and Sen, S. K. (1988). Effect of pre-sowing seed treatment on germination growth and yield of Allium cepa ver. Red Globe. Harayana J. Hort. Sci. 17, 83–87.
Hossain, M. I., Mannan, M. A., and Karim, M. A. (2015). Salicylic acid and gibberelic acid ameliorates the adverse effects of salinity on chickpea. Bangladesh Agron. J. 18, 81–88. doi: 10.3329/baj.v18i1.25571
Iftikhar, A., Rizwan, M., Adrees, M., Ali, S., Ur Rehman, M. Z., Qayyum, M. F., et al. (2020). Effect of gibberellic acid on growth, biomass, and antioxidant defense system of wheat (Triticum aestivum L.) under cerium oxide nanoparticle stress. Environ. Sci. Pollut. Res. 27, 33809–33820. doi: 10.1007/s11356-020-09661-9
Ilias, I., Ouzounidou, G., Giannakoula, A., and Papadopoulou, P. (2007). Effects of gibberellic acid and prohexadione-calcium on growth, chlorophyll fluorescence and quality of okra plant. Biol. Plant. 51, 575–578. doi: 10.1007/s10535-007-0126-5
Inglese, P., Chessa, I., Lamantia, T., and Nieddu, G. (2005). Evolution of endogenous gibberellin at different stage of flowering in relation to return bloom of cactus pear. Sci. Hortic. 71, 45–51. doi: 10.17660/ActaHortic.1997.438.7
Iqbal, M. A., Hamid, A., Ahmad, A., Hussain, I., Ali, S., Ali, A., et al. (2019). Forage sorghum-legumes intercropping: effect on growth, yields, nutritional quality and economic returns. Bragantia 78, 82–95. doi: 10.1590/1678-4499.2017363
Iqbal, M. A., Imtiaz, H., Abdul, H., Bilal, A., Saira, I., Ayman, S., et al. (2021b). Soybean herbage yield, nutritional value and profitability under integrated manures management. An. Acad. Bras. Cienc. 93:e20181384. doi: 10.1590/0001-3765202120181384
Iqbal, M. A., Iqbal, A., Ahmad, Z., Raza, A., Rahim, J., Imran, M., et al. (2021a). Cowpea [Vigna unguiculata (L.) Walp] herbage yield and nutritional quality in cowpea-sorghum mixed strip intercropping systems. Rev. Mex. Cienc. Pecu. 12, 402–418. doi: 10.22319/rmcp.v12i2.4918
Iqbal, M. A., Siddiqui, M. H., Afzal, S., Ahmad, Z., Maqsood, Q., and Khan, R. D. (2018). Forage productivity of cowpea [Vigna unguiculata (L.) Walp] cultivars improves by optimization of spatial arrangements. Rev. Mex. Cienc. Pecu. 9, 203–219. doi: 10.22319/rmcp.v9i2.4335
Iqbal, H. F., Tahir, A., Khalid, M. N., Haque, I. I., and Ahmad, A. N. (2001). Response of chickpea (Cicer arietinum L.) growth towards the foliar application of gibberellic acid at different stages. Pakistan. J. Biol. Sci. 4, 433–434. doi: 10.3923/pjbs.2001.433.434
Islam, M. S. (2001). Morpho-physiology of blackgram and mungbean as influemced by salinity. MS Thesis. Gazipur, Bangladesh: Dept. of Agronomy, Bangabandhu Sheikh Mujibur Rahman Agricultural University.
Islam, S., Chakrabortty, S., Uddin, M. J., Mehraj, H., and Jamal Uddin, A. F. M. (2014). Growth and yield of wheat as influenced by GA3 concentrations. Int. J. Bus. Soc. Sci. Res. 2, 74–78.
Islam, M. S., EL Sabagh, A., Hasan, K., Akhter, M., and Barutçular, C. (2017c). Growth and yield response of mungbean (Vigna radiata L.) as influenced by sulphur and boron application. Sci. J. Crop Sci. 6, 153–160. doi: 10.14196/sjcs.v6i1.2383
Islam, M. S., Fahad, S., Hossain, A., Chowdhury, M. K., Iqbal, M. A., Dubey, A., et al. (2021a). “Legumes under drought stress: plant responses, adaptive mechanisms and management strategies in relation to nitrogen fixation” in Engineering Tolerance in Crop Plants Against Abiotic Stress. eds. S. Fahad, O. Sönmez, S. Saud, D. Wang, C. Wu, and M. Adnan, et al. (Boca Raton, USA: CRC Press, Taylor & Francis Grou), 179–208.
Islam, M. S., Hasan, M. K., Islam, B., Renu, N. A., Hakim, M. A., Islam, M. R., et al. (2021b). Responses of water and pigments status, dry matter partitioning, seed production, and traits of yield and quality to foliar application of GA3 in mungbean (Vigna radiata L.). Front. Agron. 2:596850. doi: 10.3389/fagro.2020.5968500
Islam, M. S., Hasan, K., Sarkar, N. A. A., EL Sabagh, A., Rashwan, E., and Barutçular, C. (2017a). Yield and yield contributing characters of mungbean as influenced by zinc and boron. Sci. J. Agric. Adv. 6, 391–397. doi: 10.14196/aa.v6i1.2362
Islam, M. S., Hasan, K., Shaddam, O., and El Sabagh, A. (2017b). Effect of storage periods and containers on the germinablity of mungbean seeds. Sci. J. Agric. Adv. 6, 418–424. doi: 10.14196/aa.v6i7.2468
Islam, M. S., Muhyidiyn, I., Islam, M. R., Hasan, M. K., Golam Hafeez, A. S. M., Hosen, M. M., et al. (2022). Soybean and sustainable agriculture for food security. Soybean-Recent Advances in Research and Applications. IntechOpen.
Islam, M. R., Prodhan, A. K. M. A., Islam, M. O., and Uddin, M. K. (2010). Effect of plant growth regulators (GABA) on morphological characters and yield of black gram (Vigna mungo L.). Aust. J. Agric. Res. 48, 73–80.
Jamil, M., and Rha, E. S. (2007). Gibberellic acid (GA3) enhance seed water uptake, germination and early seedling growth in sugar beet under salt stress. Pak. J. Biol. Sci. 10, 654–658. doi: 10.3923/pjbs.2007.654.658
Jong, M. D., Mariani, C., and Vriezen, W. H. (2009). The role of auxin and gibberellin in tomato fruit set. J. Exp. Bot. 60, 1523–1532. doi: 10.1093/jxb/erp094
Karmoker, J. L. (1984). “Hormonal regulation of ion transport in plants” in Hormonal Regulation of Plant Growth and Development. Vol. 1. ed. S. S. Purohit (India: Agro Botanical Publishers), 219–263.
Keykha, M., Ganjali, H. R., and Mobasser, H. R. (2014). Effect of salicylic acid and gibberellic acid on some characteristics in mungbean (Vigna radiata). Int. J. Biosci. 5, 70–75. doi: 10.12692/ijb/5.11.70-75
Khafagi, O. A., Khalaf, S. M., and El-Lawendy, W. I. (1986). Effect of GA3 and CCC on germination and growth of soybean, common bean, cowpea and pigeon pea plants grown under different levels of salinity. Ann. Agric. Sci. 24, 1965–1982.
Khan, I. A. (1988). Path coefficient analysis of yield attributes in mungbean (Vigna radiate L.). Legum. Res. 11, 41–43.
Khan, N. A., Ansari, H. R., and Sanaullah, A. H. (1988). Effect of gibberellic acid spray during ontogeny of mustard on growth, nutrient uptake and yield characteristics. J. Agron. Crop Sci. 181, 61–63. doi: 10.1111/j.1439-037X.1998.tb00399.x
King, R. W., Seto, H., and Sachs, R. M. (2000). Response to gibberellin structural variants shows that ability to inhibit flowering correlates with effectiveness for promoting stem elongation of some plant species. Plant Growth Regul. 19, 437–444. doi: 10.1007/s003440000015
Leite, M. V., Rosolem, C. A., and Rodrigues, J. D. (2003). Gibberelin and cytokinin effects on soybean growth. Sci. Agric. 60, 537–541. doi: 10.1590/S0103-90162003000300019
Lobato, A. K. S., Filho, B. G. S., Costa, R. C. L., Goncalves-Vidigal, M. C., Moraes, E. C., Oliveira Neto, C. F., et al. (2009). Morphological, physiological and biochemical responses during germination of the cow pea seeds under salt stress. World J. Agric. Sci. 5, 590–596.
Mahmoody, M., and Noori, M. (2014). Effect of gibberellic acid on growth and development plants and its relationship with abiotic stress. Int. J. Farm. Allied Sci. 3, 717–721.
Malik, B. A., Tahir, M., Hussain, S. A., and Chaudhary, A. H. (1986). Identification of physiologically efficientgenotypes in mungbean. Pak. J. Agric. Res. 7, 41–43.
Maske, V. G., Dotale, R. D., Sorte, P. N., Tale, B. D., and Chore, C. N. (1997). Germination, root and shoot studies in soybean as influenced by GA3 and NAA. J. Soil. Crops 7, 147–149.
Merah, O. (2001). Potential importance of water status traits for durum wheat improvement under Mediterranean conditions. J. Agric. Sci. 137, 139–145. doi: 10.1017/S0021859601001253
Nagar, S., Singh, V. P., Arora, A., Dhakar, R., Singh, N., Singh, G. P., et al. (2021). Understanding the role of gibberellic acid and paclobutrazol in terminal heat stress tolerance in wheat. Front. Plant Sci. 12:692252. doi: 10.3389/fpls.2021.692252
Naidu, N. V., and Satyanarayana, A. (1993). Heterosis for yield and its components in mungbean (Vigna radiate (L.) Wilczek). Indian J. Pulses Res. 6, 102–105.
Naidu, C. V., and Swamy, P. M. (1995). Effect of gibberllic acid in growth, biomass production and associated physiological parameters in some selected tree species. Indian J. Plant Physiol. 5, 401–403. doi: 10.3923/pjbs.2002.401.403
Natarajan, C., Thyagarajan, K., and Rathanswamy, R. (1988). Association and genetic diversity in greengram. Madras Agric. J. 75, 238–245.
Page, A. L., Miller, R. H., and Keeney, D. R. (1982). Methods of Soil Analysis. Part 2. 2nd Edn. Madison, WI: American Society of America.
Patil, H. S., and Deshmukh, R. B. (1988). Correlation and path coefficient analysis in mungbean. J. Maharashtra Agric. Univ. 13, 183–185.
Patil, H. S., and Narkhede, B. N. (1987). Variability association and path analysis in black gram. J. Maharashtra Agric. Univ. 12, 289–292.
Payne, W. A. P. I., Ramussen, C. C., and Raming, R. F. (2001). Assessing simple wheat and pea models using data from a long-term tillage experiments. Agron. J. 93, 250–260. doi: 10.2134/agronj2001.931250x
Piper, C. S. (1966). Soil and Plant Analysis. Adelaide University Press. Available at: https://www.adelaide.edu.au/library/ (Accessed September 10, 2022).
Prat, L., Batti, C., and Fichet, T. (2008). Effect of plant growth regulators on floral differentiation and seed production in jojoba [Simmondsia chinesis (link) Schneider]. Ind. Crop. Prod. 27, 44–49. doi: 10.1016/j.indcrop.2007.07.001
Rahimi, M. M., Zarei, M. A., and Arminian, A. (2011). Selection criteria of flax (Linum usitatissimum L.) for seed yield and yield components and biochemical composition under various planting dates and nitrogen. Afr. J. Agric. Res. 6, 3167–3175. doi: 10.5897/AJAR11.382
Rahman, A. B. M., Khan, M., Hasan, M. M., Banu, L. A., and Howlader, M. H. K. (2018). Effect of foliarapplication of gibberellic acid on different growth contributing characters of mungbean. Progress. Agric. 29, 233–238. doi: 10.3329/pa.v29i3.40008
Rastogi, A., Siddiqui, A., Mishra, B. K., Srivastava, M., Pandey, R., Misra, P., et al. (2013). Effect of auxin and gibberellic acid on growth and yield components of linseed (Linum usitatissimum L.). Crop Breed. Appl. Biotechnol. 13, 136–143. doi: 10.1590/S1984-70332013000200006
Ross, J. J., O’Neill, D. P., and Rathbone, D. A. (2003). Auxin-gibberellin interactions in pea: integrating the old with the new. J. Plant Growth Regul. 22, 99–108. doi: 10.1007/s00344-003-0021-z
Roy, R., and Nasiruddin, K. M. (2011). Effect of different level of GA3 on growth and yield of cabbage. J. Environ. Sci. Nat. Res. 4, 79–82. doi: 10.3329/jesnr.v4i2.10138
Sajid, G. M., Mahmoona, K., and Ahmad, Z. (2009). Foliar application of plant growth regulators (PGRs) and nutrients for improvement of lily flowers. Pak. J. Bot. 41, 233–237. Available at: https://www.pjbot.org
Salisbury, F. B., and Ross, C. W. (1996). Plant Physiology. 6th Edn. California, USA: Wadsworth. pp. 247–277.
Saminathan, B. (2013). Effect of chromium studies on germination and biochemical content of black gram (Vigna mungo (L.) Hepper.). Int. J. Adv. Res. 1, 216–222.
Saneoka, H., Hamid, A., and Hashem, A. (1995). Soil and plant water relations. IPSA-JICA Project reports. Salna, Gazipur, Bangladesh. 20.
Shah, S. H. (2004). Morpho-physiological response of black cumin (Nigella sativa) to nitrogen, gibberellic acid and kinetin application. PhD Thesis. Aligarh, India: Aligarh Muslim University.
Shivanna, H., Balachandra, H. C., and Suresha, N. L. (2007). Influence of growth regulators and pre-sowing chemicals on germination and growth parameters of Prosopis cineraria L. Druce. J. Agric. Sci. 20, 328–329.
Shukry, W. M., and El-Bassiouny, H. M. S. (2002). Gibberellic acid effects on protein pattern, hydrolytic enzyme activities and ionic uptake during germination of Vicia faba in sea water. Acta Bot. Hungar. 44, 145–162. doi: 10.1556/ABot.44.2002.1-2.11
Silva, I. R. (2004). Praias da Costa do Descobrimento: uma contribuição para a gestão ambiental. Salvador. Tese de Doutorado em Geologia, Instituto de Geociências, Universidade Federal da Bahia. 227.
Singh, M., John, S. A., Rout, S., and Patra, S. S. (2015). Effect of GA3 and NAA on growth and quality of garden pea (Pisum sativum L.) cv. Arkel. 10, 381–383.
Singh, D. B., and Sharma, T. V. R. (1996). Effect of GA3, NAA and 2,4-D on growth and yield of cowpea (Vigna unguiculata L.) Walf variety arka garima. Flora Fauna Jhansi 2, 5–6.
Singh, I. S., Singh, B. D., Singh, R. P., and Singh, K. K. (1988). Inter relationship of yield and its components in F3 progenies of a cross in mungbean. Crop. Improv. 15, 146–150.
Soad, M. M. I., Lobna, T. S., and Farahat, M. M. (2010). Vegetative growth and chemical constituents of croton plants as affected by foliar application of benzyl adenine and gibberellic acid. J. Am. Sci. 6, 126–130.
Swain, S. M., Reid, J. B., and Kamiya, Y. (1997). Gibberellins are required for embryo growth and seed development in pea. Plant J. 12, 1329–1338. doi: 10.1046/j.1365-313x.1997.12061329.x
Taiz, L., and Zeiger, E. (2004). Plant Physiology. 3rd Edn. Sunderland, MA: Sinauer Associates, Inc. 494–505.
Taiz, L., and Zeiger, E. (2010). “Gibberellins: regulators of plant height” in Plant Physiology. ed. E. Zeiger (Hard Cover: Sinauer Associates, Incorporated), 461–493.
Uddin, M. H. (1999). Effect of plant growth regulators on flowering, pod set and yield attributesin mungbean. M.S. Thesis. Gazipur: Department of Crop Botany, Bangabandhu Sheikh Mujibur Rahman Agricultural University, 4–36.
Ullah, S., Anwar, S., Rehman, M., Khan, S., Zafar, S., Lijun Liu, L., et al. (2017). Interactive effect of gibberellic acid and NPK fertilizer combinations on ramie yield and bast fibre quality. Sci. Rep. 7:10647. doi: 10.1038/s41598-017-09584-5
Verma, U. S., Kumar, A., and Tripathi, D. K. (2016). Yield performance of mungbean variety following application of growth regulators. Biochem. Cell. Arch. 16, 157–161.
Vwioko, E. D., and Longe, M. U. (2009). Auxin and gibberellin effects on growth and fruit size in Lagenaria siceraria (Molina standley). Biosci. Biotechnol. Res. Commun. 21, 263–271.
Wang, F., Cheng, F., and Zhang, G. (2006). The relationship between grain filling and hormone content as affected by genotype and source-sink relation. Plant Growth Regul. 49, 1–8. doi: 10.1007/s10725-006-0017-3
Keywords: gibberellic acid (GA3), growth regulators, water relations, grain yield, functional relationship, mungbean
Citation: Islam MS, Hasan MK, Islam MR, Chowdhury MK, Pramanik MH, Iqbal MA, Rajendran K, Iqbal R, Soufan W, Kamran M, Liyun L and El Sabagh A (2023) Water relations and yield characteristics of mungbean as influenced by foliar application of gibberellic acid (GA3). Front. Ecol. Evol. 11:1048768. doi: 10.3389/fevo.2023.1048768
Edited by:
Dariusz Piesik, University of Science and Technology (UTP), PolandReviewed by:
Arshad Javaid, University of the Punjab, PakistanKarthikeyan Adhimoolam, Jeju National University, Republic of Korea
Copyright © 2023 Islam, Hasan, Islam, Chowdhury, Pramanik, Iqbal, Rajendran, Iqbal, Soufan, Kamran, Liyun and El Sabagh. This is an open-access article distributed under the terms of the Creative Commons Attribution License (CC BY). The use, distribution or reproduction in other forums is permitted, provided the original author(s) and the copyright owner(s) are credited and that the original publication in this journal is cited, in accordance with accepted academic practice. No use, distribution or reproduction is permitted which does not comply with these terms.
*Correspondence: Mohammad Sohidul Islam, ✉ shahid_sohana@yahoo.com; Ayman El Sabagh, ✉ ayman.elsabagh@agr.kfs.edu.eg