Group-living decrease predation risk of individual: Evidence from behavior, hormones and reproduction of plateau pika
- 1Key Laboratory of Grassland Ecosystem of the Ministry of Education, College of Grassland Science, Gansu Agricultural University, Lanzhou, China
- 2Qinghai Academy of Animal Science and Veterinary Medicine, Qinghai University of Animal Science and Veterinary Medicine, Xining, Qinghai, China
Extensive research confirms that abiotic stressors like predation risk can profoundly affect animal condition. However, there is a lack of experimental research assessing the suite of physiological responses to risk. We increased predation risk in free-living plateau pikas (Ochotona curzoniae) by simulating natural chases using a model predator (UAV: unmanned aerial vehicle) and monitored behavior, physiology, and reproduction of pikas. We found that: Predation risk affects the body weight of plateau pika under different population density stress, but the effect is not significant. Compared with the non-predation risk treatment (control), the plateau pika under high population density stress mainly responded to the risk of predation by reducing the foraging time and increasing the vigilance time, while plateau pikas under low population density pressure not only reduce foraging time and appropriately increase vigilance time, but also focus on increasing the concealing time in the burrows. The corticosterone (CORT), cortisol and thyroid (T4) level of plateau pika with low population density under the predation risk was significantly higher than those in the control, and the testosterone (T), progesterone (PROG), leptin (LEP) and testicular weight of plateau pika with low population density in the predation risk was significantly lower than those in the control. There was no difference in the litter size of female pika between predation risk treatments and control. Broadly, our result show that predation risk had significant effects on the behavior, physiology and reproduction of plateau pikas with low population density, but had no significant effect with high population density, and the response of male plateau pika to predation risk was greater than that of female. Therefore, the prevention of plateau pika should focus on the control of population density and the protection of predators, so as to avoid the failure of ecological prevention methods such as natural enemies due to the increase of population density.
1. Introduction
Studying how biotic and abiotic stressors affect animal activity is essential to reveal the complex structure and function of physiological systems (Boudreau et al., 2019). Abiotic stressors include various challenges imposed on wildlife, including predation risks (Turner and Chislock, 2010). Animals respond to predation risks by increasing vigilance, changing group sizes, or finding safe habitat (Childress and Lung, 2003; Creel et al., 2014). Typically, these anti-predation behaviors will reduce the foraging rate of animals, change dietary patterns or cause physiological stress responses, and eventually affect the growth and reproduction of prey (Kotler et al., 2004; Yin et al., 2017). In recent years, the research on the response of animal acute and chronic stress to predation risk has been gradually improved (Clinchy et al., 2013). The predation stress theory states that under the risk of predation, chronic stressors cause the hypothalamus to release corticotropin-releasing factor (CRF) and other hormones, and promote the release of adrenocorticotropic hormone (ACTH) from the hypophysis, which in turn leads to the release of cortisol or corticosterone from the adrenal cortex. The increase of corticosteroid concentration can inhibit the activity of hypothalamus-pituitary-gonad axis through acute stress, If predation stress persists, higher glucocorticoid concentrations in chronic exposure can lead damage to mammals, including immunosuppression and weight loss (Christianson and Creel, 2010; Clinchy et al., 2013). Therefore, physiological changes related to predation risk are usually represented by corticosteroids (cortisol and corticosterone) and sex hormones (testosterone and progesterone) to represent the physiological response of animals (Hawlena and Schmitz, 2010; Zanette et al., 2011).
The level of predation risk is closely related to the population density of small mammals. Under the pressure of population density, the behavior strategy of small mammals is mainly affected by the tradeoff between risk pressure and energy demand. The greater the density pressure, the greater the weight of risk aversion behavior (concealing, vigilance, escape, etc.) in their behavior patterns, while foraging and social behaviors decrease accordingly (Bian and Fan, 1997). At present, domestic and foreign scholars make lots of research to verify the hypothesis of “many eyes effect” in many behavioral studies of small mammals. For example, with the increase of Octodon degus population density, the earlier it finds predators (Ebensperger and Wallem, 2002); In addition, group-living Microtus epiroticus, Clethrionomys glareolus and Apodemus flavicollis are less likely to be attacked and hunted by weasels than individuals living alone (Jędrzejewski et al., 1992). Cynomys ludovicianus and Cynomys leucurus with high population density can find predators earlier and avoid them effectively (Hoogland, 1981). Although numerous studies have demonstrated that increased population density is beneficial for small mammals to cope with predation risks, the physiological mechanism remains unclear.
We conducted a field experiment manipulating predation risk in free-living plateau pika (Ochotona curzoniae) to assess their physiological, behavior, reproduction response in a natural setting. Plateau pika (Ochotona curzoniae) are small, diurnal lagomorphs that inhabit alpine meadows on the Qinghai-Tibet Plateau (QTP), People’s Republic of China (Wilson and Smith, 2015). They have crucial ecological significance for biodiversity maintenance in alpine rangeland ecosystems and improving soil quality on the QTP (Zhang et al., 2016). Mammalian predators of pikas include Wolves (Canis lupis), Tibetan foxes (Vulpes ferrilata), Red foxes (V. vulpes); avian predators include Upland buzzards (Buteo hemilasius), Golden eagles (Aquila chrysaetos), Steppe eagles (A. nipalensis) and Saker falcons (Falco cherrug) (Smith et al., 2018). Therefore, Plateau pikas face high predation risk in alpine meadow ecosystem. Since the 1990s, some scholars have done a lot of research on the behavior and physiological stress response of plateau pikas under population density or predation pressure in laboratory and natural conditions, and the “predator sensitive food hypothesis,” “predator stress hypothesis” and “multi-eye effect hypothesis” were verified (Yang et al., 2007; Yin et al., 2017; Wei, 2019). Among them, Wei et al.’s study on the “multi-eye effect hypothesis” of plateau pikas shows that the higher the population density, the lower the predation risk of plateau pikas in the population, and the vigilance time decreases with the increase of population density. However, the above results are based on the fact that the population density of plateau pikas is within the range of 36–132 effective burrows per hectare, but under the higher population density of plateau pikas, the strategy of pikas to deal with predation risk is also consistent with the theory of “multi-eye hypothesis.” It’s not clear. We selected two plateau pika populations with different densities and used UAVs to simulate natural enemies to interfere with these two populations, and analyze the response of behavior, physiology and reproduction of plateau pika with different population densities to predation risk. We hypothesized that under the predation risk, the anti-predation behavior and physiological response of the high density population were weaker than those of the low density population, and the reproduction of the high density population did not decrease significantly. Based on the hypothesis, we predicted that (1) the plateau pika with low species density reduces the predation risk by increasing the vigilance time, while the plateau pika with high population density reduces the predation risk by increasing the concealing time in the burrows, (2) the predation risk can significantly reduce the contents of testosterone and progesterone in plateau pikas, and increase the contents of cortisol, corticosterone, thyroid hormone and leptin in plateau pikas, (3) regardless of the population density of plateau pika, the predation risk significantly decreased the testicular weight and the litter size of plateau pika.
2. Materials and methods
2.1. Study area
This study is located on the northern Qinghai-Tibet Plateau in Maqu Tibetan Autonomous County in Gansu Province, China (N 33°50′23″, E 102°08′48″). The climate belongs to continental monsoon type dominated by the southeastern monsoon and high pressure from Siberia. Winter is long and severe, and summer is short and cool (Wei and Zhang, 2018). Based on a survey of vegetation and topography in the study area, we selected the pasture of an alpine meadow with an altitude of 3,250 m. Plateau pikas are the main lagomorphs in the study area, with a relative population density of 232 ± 18 effective holes per hm2. Therefore, this area is an ideal place to study the behavior of plateau pikas.
2.2. Experimental and sampling design
On March 19, 2018 (the breeding start period of plateau pika). Four experimental plots (1 ha per plot) were selected in the study area. The study area was divided into two high population density and two low population density. The vegetation status (coverage, height, dominant plant species, etc.) and topography (slope, height, distance to the road, etc.) between the experimental group and the control group in the same plateau pika population density area are as consistent as possible, and the specific information of the experimental sample site is shown in Table 1. The average activity range of plateau pika was 114.2 ± 5.1 m in the study area, in order to avoid the interference between different sample plots, the distance between plots is more than 200 meters. Plots were divided into two temporary sampling plots (fecal sampling and weight measurement) and one behavior detection plot.
2.3. Animal marking
On March 19–23, 2018, plateau pika was caught in the study area trapped using string snares (Dobson et al., 1998). After each capture, the capture position, body weight, sex and reproductive status of each plateau pika were recorded. In order to identify the individual of plateau pika in behavior observation, dyeing agent was used. The head of male and backs of females was also marked with black hair dye, which can determine the sex of the individual at a long distance. Each marked pika was released in the original capture location. Following each capture, the weight, sex, and reproductive condition of each adult pikas were recorded. All procedures involved in animal treatment in this experiment were approved by the animal ethics committee of Gansu Agricultural University (GSC-IACUC-2018-0011).
2.4. Predation risk simulation experiment
In this study, the unmanned aerial vehicle (UAV) was used to simulate the predation risk of raptors to plateau pikas. Before simulating the predation risk experiment, we need to conduct two preliminary experiments to determine the optimal flight parameters of the UAV and the impact of UAV noise on the plateau pika.
2.4.1. Experiment 1
First, we need to determine the optimal flight altitude and velocity of UAV. We used the target animal observation method to record the response of the marked plateau pika to the different flight altitude and velocity of UAV. The ratio of the number of entering burrows and the total number of ground activities of plateau pika was used as the response efficiency to choose the best flight height and velocity of UAV. The result showed that the response efficiency is the most obvious when the height is 10 m and the velocity is 8 m/s (Details refer to the Supplementary Table 1).
2.4.2. Experiment 2
Secondly, we need to verify whether the sound generated by the UAV make an effect on plateau pika. We used PCM-D100 digital recorder (frequency response range: 0.04–1.5khz) and SY-322 external microphone of SONY company to record the sound generated by the UAV, and then imported the sound file into the sound playback device (Bose Sound Link Mini). Put the sound playing equipment into the experimental plot, and observed the behavior changes of plateau pika in the experimental plot and the control plot. Set three observation time points (0900, 1,200, 1,600) in a day, and observe for 20 min each time. The results showed that there was no significant difference in the behavior of plateau pika between the experimental and the control (Details refer to the Supplementary Table 1).
In the previous study on the activity pattern of plateau pika, it was found that the activities of plateau pika in March and April were unimodal, and the activities of the day were mainly concentrated in 9:00–17:00 (Zhou et al., unpublished data, 2022). Therefore, UAV interference is conducted from 9:00 to 17:00 every day. The official interference time starts on March 29 and ends on April 7.
2.5. Behavior variables and measurements
The recorded behaviors of plateau pikas are defined the following: (1) concealing: the animal hid inside the burrow, (2) foraging: gathering or chewing vegetation, and (3) vigilance: sitting in the meadow with neck outstretched, standing with front feet off the ground, or raising head during foraging, (4) others: other behavior except for the above behaviors in the observation period (Yang et al., 2007).
At the active peaks from 0900 to 1700, after most pikas were captured for 3 days, observers measured behaviors according to a focal-animal sampling rule and a continuous recording rule (Martin and Bateson, 1985). Focal individuals were randomly selected from the colony based on color of fur dyeing location. Binoculars were used to identify the focal animal and observe its behavior. During the observation period, in order to avoid the impact of observer, the observer sat at a distance of more than 30 m from the study area (Wei et al., 2019). Observe for 20 min per hour and change observation points every other hour to ensure that each labeled adult pika is observed at least once. Use a stopwatch to record the duration of each behavior of the focus animal every 20 min.
2.6. Feces collection and hormone measurement of plateau pika
We set the fecal collection time to March 29th, April 3rd, April 7th. To facilitate the quantitative collection of all voided feces without handling the animals, we selected 15 active latrines of plateau pikas based on their similar characteristics (Navarro-Castilla et al., 2019). Following the method of Calvete et al. (2004), only pellet groups with clear fecal accumulation and with multiple pellets were considered latrines. Individual characteristics (e.g., sex, age and breeding condition) usually influence physiological variation in animals (Cockrem, 2013). Recently, Lafferty et al. (2017) showed that subsamples derived from homogenized whole pellet groups from snowshoe hares (Lepus americanus) are the most appropriate and representative of the physiological state when the conditions are not fully controlled (e.g., unknown identity and multiple defecation events). Therefore, feces from the same latrine and same color fresh fecal particles were thawed and thoroughly mixed to minimize potential bias due to both sample and individual variation (Cockrem, 2013). There are many ways to determine the sex of animals by animal feces, one of which is to determine the sex of animals by sex chromosomes. Another is to determine whether male or female is based on the hormones in the feces. At present, it has been found that the ratio of Tragopan caboti to testosterone in the feces of female is significantly higher than that of male. This method can be used to successfully distinguish the sex of yellow-bellied pheasant. Therefore, we judged sex by the content of testosterone and progesterone in each sample (each plateau pika individual). Moreover, the sampling time is the early reproductive stage of plateau pikas, and there is a significant difference in the contents of testosterone and progesterone between male and female, which is also helpful for us to judge the sex of fecal samples.
The collected pellets were stored in the freezer at −20°C until analysis. Testosterone (T), progesterone (PROG), thyroid (T4), leptin (LEP), corticosterone (CORT) and cortisol concentrations in fences were assayed by rat-specific enzyme-linked immunoassay with reagents supplied by Hunan fengrui Biotech Inc. (Hunan, China), and according to the manufacturer’s instructions. The sensitivity, intraassay coefficients of variation and inter-assay coefficients of variation for PROG, T4, LEP, CORT, and cortisol were listed in Table 2. All samples were tested twice. Refer to Wang et al. (2012) for the main steps of fecal hormone extraction.
2.7. Data analysis
We used the growth rate (GR) of each indicator between months (March–May) to determine the changes in body weight and reproduction (litter size and testicular weight) of plateau pikas under predation risk. The formula as follows.
In the formula, GR indicates the growth rate of the indicator, valueMay indicates the value of each indicator in May, and valueMarch indicates the value of each indicator in March. i refers to the experimental group in high density area (EXH), the control in high density area (CH), the experimental group in low density area (EXL) and the control in low density area (CL).
The assumption of normality was tested by the Kolmogorov–Smirnov test, and the assumption of homogeneity of variances was tested with Levene’s test. If these assumptions were not met, data were logarithmic or square-root transformed. Considering the effects of individual differences on behavior and fecundity, we used a linear mixed effect model to analyze the responses of plateau pikas body weight (BW), testicular weight (TW), litter size (LS), foraging time (FT), vigilance time (VT), and concealing time (CT) to predation risk. BW, TW, LS, FT, VT, and CT were taken as response variables, sex, treatment and different population density as fixed effects, and plateau pika individual ID as random effects. we also used a linear mixed effect model to analyze the responses of plateau pikas thyroid (T4), leptin (LEP), testosterone (T) and progesterone (PROG), corticosterone (CORT) and cortisol to predation risk, taking T4, LEP, T, PROG and CORT and cortisol as response variables, and sex, treatment and different population density as fixed effects. The feacal sample ID of plateau pika was taken as a random effect. If the treatment effect is significant, then a simple effect analysis is carried out, and the differences between groups are obtained. The specific settings of fixed effects and random effects in the linear mixed effect model were listed in Table 3.
The linear mixed model is run by lmerTest package in R, and the simple effect analysis is carried out by emmeans package. All statistical analyzes were carried out using R5.5.3 (R Core Team, 2016). Ggplot2 package is used to draw the graph. The data were expressed by mean ± SD. The significant level was p < 0.05, and the extremely significant level was p < 0.01.
3. Results
3.1. Effect of predation risk on body weight of plateau pika with different population density
The results showed that the body weight of male plateau pika decreased in both the predation risk group and the control under different population density, and the growth rate (GREXH = −3.05%;GREXL = −6.06%) of the predation risk was higher than that of the control (GRCKH = −1.86%;GRCKL = −2.96%). The body weight of female plateau pika increased in both the predation risk group and the control under different population density, and the growth rate (GREXH = 8.92%;GREXL = 7.36%) of the predation risk was lower than that of the control (GRCKH = 14.46%;GRCKL = 12.80%, Figure 1). The results of liner mixed model (LMM) test showed that the body weight of plateau pikas was significantly different between sex (F1,81 = 4.513, p = 0.0362), but there was no significant difference between treatment (F1,81 = 0.301, p = 0.585), density (F1,81 = 0.003, p = 0.956) and the interaction of the above three factors (Supplementary Table 2). It can be seen that predation risk can affect the body weight of plateau pikas, but the effect is not significant. It indicates the risk of predation has effect on the body weight of plateau pikas, but the effect is not significant.
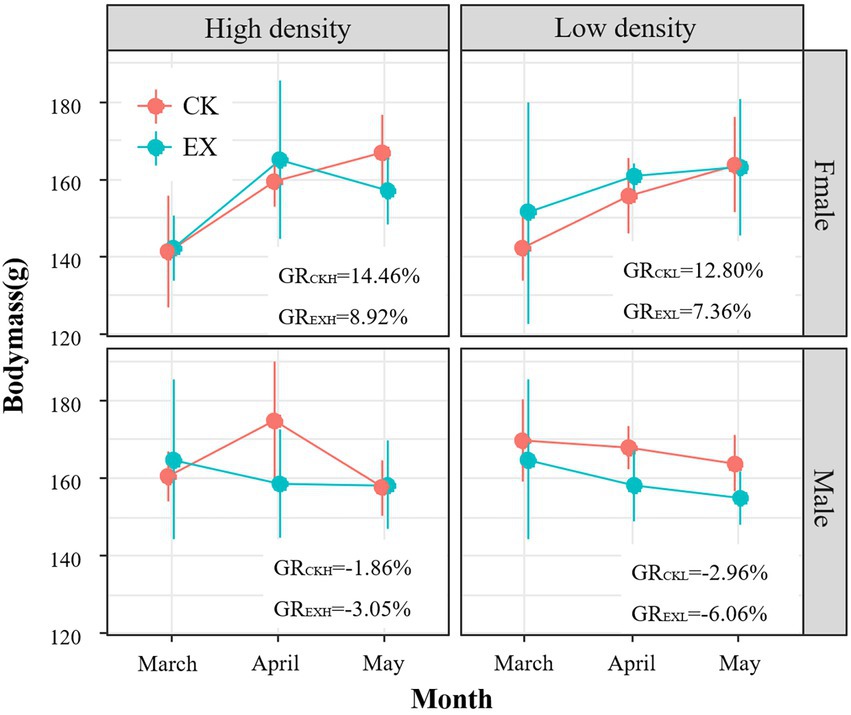
Figure 1. Mean (± SE) body mass (g) of adult plateau pikas from March to May between predation risk treatment group (n = 69 individuals) and control group (n = 58 individuals). The blue solid point represents the predation risk treatment group (EX), red solid point represents the control (CK); dot represents the high population density area (HIGH), triangle represents the low population density area (LOW); GR denotes the change rate.
3.2. Effect of predation risk on behavior of plateau pika with different population density
A total of 56 individuals were observed in this study (male: 35; female: 21). LMM test showed foraging of plateau pikas varied strongly with density (F1,56 = 18.852, p = 0.0001) and the interaction between treatment and density (F1,56 = 5.4981, p = 0.0238), but had no significant variance with sex, treatment and other interaction of the above three factors. Vigilance time of plateau pikas varied strongly with density (F1,56 = 16.831, p = 0.00013) and interactive between density and treatment (F1,56 = 6.5565, p = 0.0141). Concealing time of plateau pikas varied strongly with density (F1,56 = 22.727, p < 0.0001), treatment (F1,56 = 35.249,p < 0.0001) and the interactive between density and treatment (F1,56 = 9.4118,p = 0.0038, Supplementary Table 2). Simple effect analysis result showed foraging time of female plateau pikas in low-density plot decreased in predation risk group compared with no-predation risk group (p = 0.031). Foraging (p = 0.0042) and vigilance (p = 0.027) time of male plateau pikas in low-density plot decreased in predation risk group compared with no-predation risk group, but concealing time (p = 0.027) increased significantly (Figure 2). It can be seen that under the risk of predation, plateau pika in the high-density area reduced the time of foraging and increased the time of vigilance; while plateau pika in the low-density area increased the time of concealing time in the burrows in addition to reducing the time of foraging.
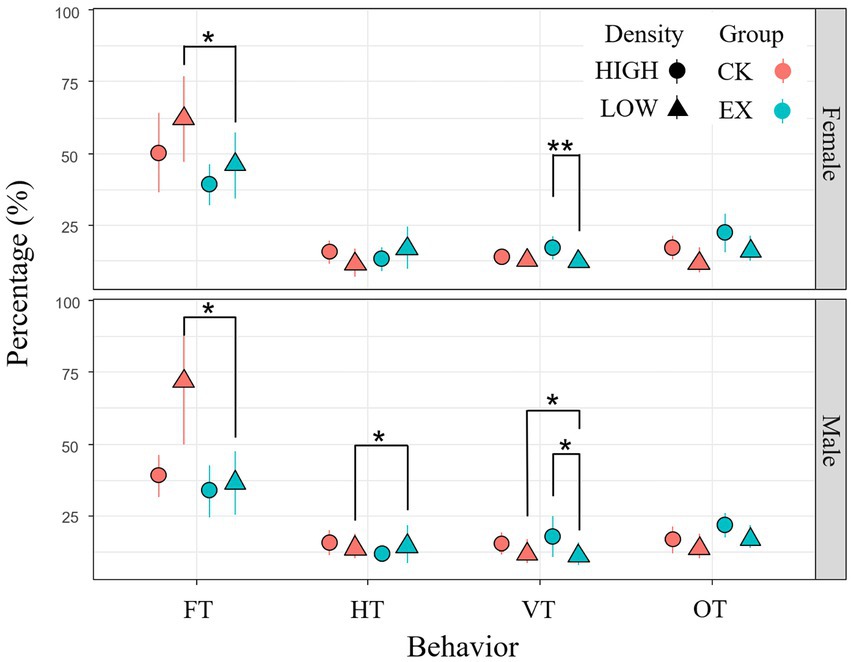
Figure 2. Mean time (± SE) allocation pattern of main behavior (FT-foraging time; CT-concealing time; VT-vigilance time; OT-other time) of the plateau pika in predation risk. The blue solid point represents the predation risk treatment group (EX), the red solid point represents the control (CK); dot represents the high population density area (HIGH), triangle represents the low population density area (LOW). *p < 0.05; **p < 0.01.
3.3. Effect of predation risk on hormone concentration of plateau pika with different population density
A total of 192 fences of individuals were observed in this study. LMM test also showed a significant difference in the treatment (F1,86 = 27.834, p < 0.001), interactive effects between sex and treatment (F1,86 = 6.1552, p = 0.0141) and interactive effects between density and treatment (F1,86 = 37.326,p < 0.001) in the fences T concentrations of male adult plateau pikas. The PROG concentrations in fences of plateau pika had no significant difference among densities, treatments, sex and its interactions. There was significant difference in T4 concentrations between density (F1,171 = 17.131,p < 0.001) and treatment (F1, 171 = 35.809,p < 0.001), but there was no significant difference between sex and other interactions of the density, treatments and sex. The LEP concentrations had significant difference among treatments (F1,171 = 12.7341,p = 0.0005) and the interaction between density and treatment (F1, 171 = 10.884,p = 0.0012). CORT concentrations of adult plateau pikas showed a significant difference in the interaction between treatment and density (F1, 171 = 9.593,p = 0.0023), and Cortisol concentrations showed a significant effects in the treatment (F1,171 = 16.579,p < 0.0001, Supplementary Table 2). Simple effect analysis result showed T concentrations of male adult plateau pikas in low-density plot significantly lower than that of high-density plot (p < 0.001). T (p < 0.001), T4 (p < 0.001), LEP (p = 0. 0017), CORT (p < 0.01), and Cortisol (p = 0.021) concentrations of adult plateau pikas in low-density plot significantly higher than that of high-density plot. There is no significant difference of these 6 hormones in high-density between predation risk group and control group. The results showed that predation risk had the most significant effect on CORT, cortisol, T4, T, and LEP of plateau pika in low-density, but had no significant effect on high-density plateau pika (Figure 3).
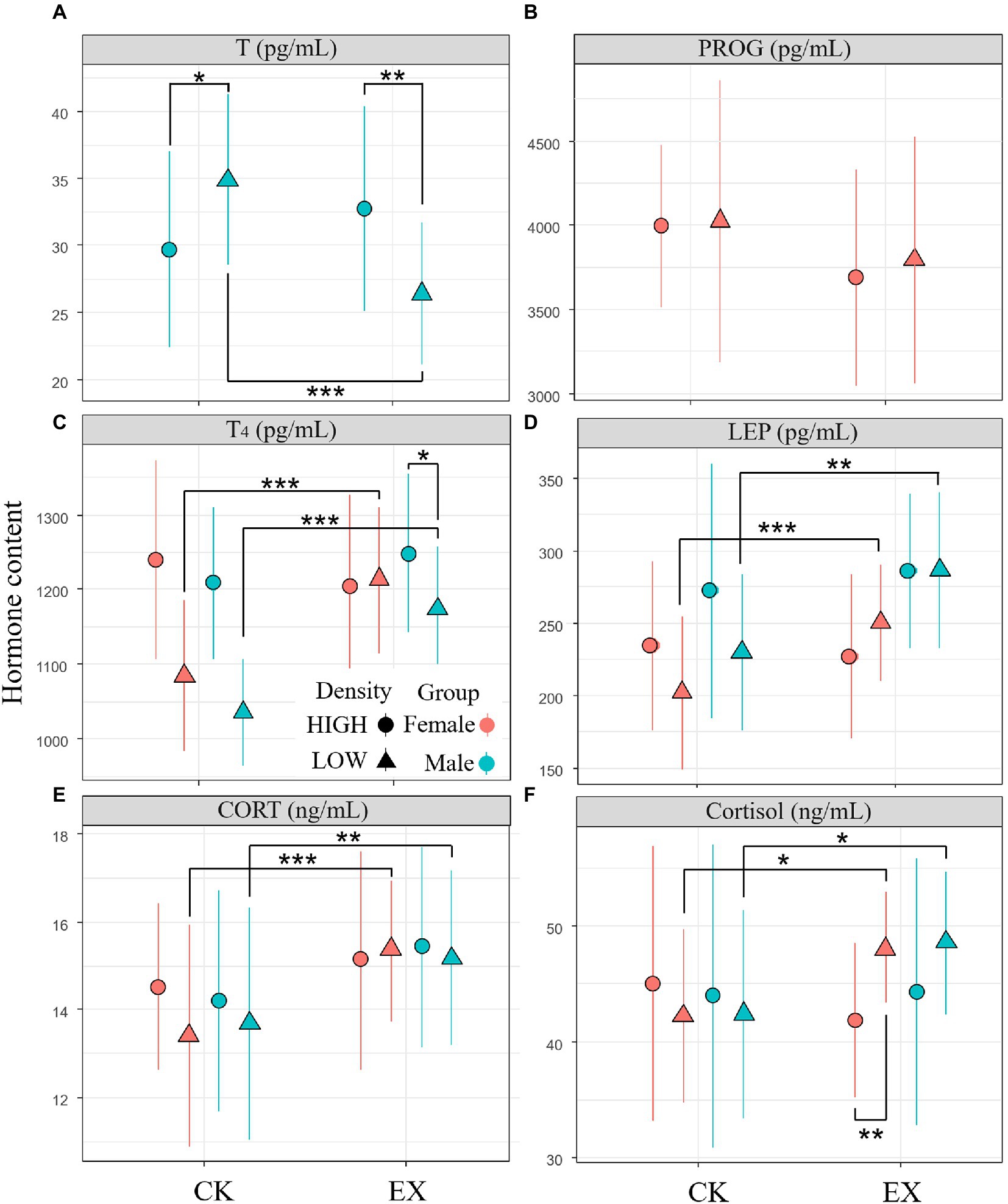
Figure 3. Mean concentration (± SE) of fences testosterone (T, n = 89 individual) in Figure 1A, progesterone (PROG, n = 89 individual) in Figure 1B, thyroid (T4, n = 179 individual) in Figure 1C, leptin (LEP, n = 179 individual) in Figure 1D, corticosterone (CORT, n = 179 individual) in Figure 1E, cortisol (n = 179 individual) in Figure 1F of adult plateau pikas between predation risk treatment group and control group. The blue solid point represents the predation risk treatment group (EX), red solid point represents the control (CK); dot represents the high population density area (HIGH), triangle represents the low population density area (LOW). *p < 0.05; **p < 0.01; ***p < 0.001.
3.4. Effect of predation risk on reproduction of plateau pika with different population density
The results showed that the testicular weight of male plateau pikas increased at first and then decreased under different population density, and the rate of change (GREXH = −23.73%; GREXL = 79.87%) in the treatment group was higher than that in the control (GRCKH = −6.92%; GRCKL = 79.65%), and the female litter size also increased at first and then decreased, but the rate of change in the treatment group (GREXH = −42.86%; GREXL = 31.43%) was lower than that in the control (GRCKH = −41.03%; GRCKL = 9.52%, Figure 4). LMM test also showed that the testicular weight of plateau pikas was significantly different in the density (F1,33 = 4.945,p = 0.0284). The litter size of female adult plateau pikas had no significant difference in the treatment, density and interaction between treatment and density (Supplementary Table 2). Simple effect analysis result showed testicular weights of male adult plateau pikas in low-density plot was significantly lower in the predation risk group than in control (p = 0.0053), and had no significant effects in high-density plot (Figure 4). In conclusion, predation risk has the most significant effect on the fertility of plateau pikas with low population density plot, but had no significant effect in high population density plot.
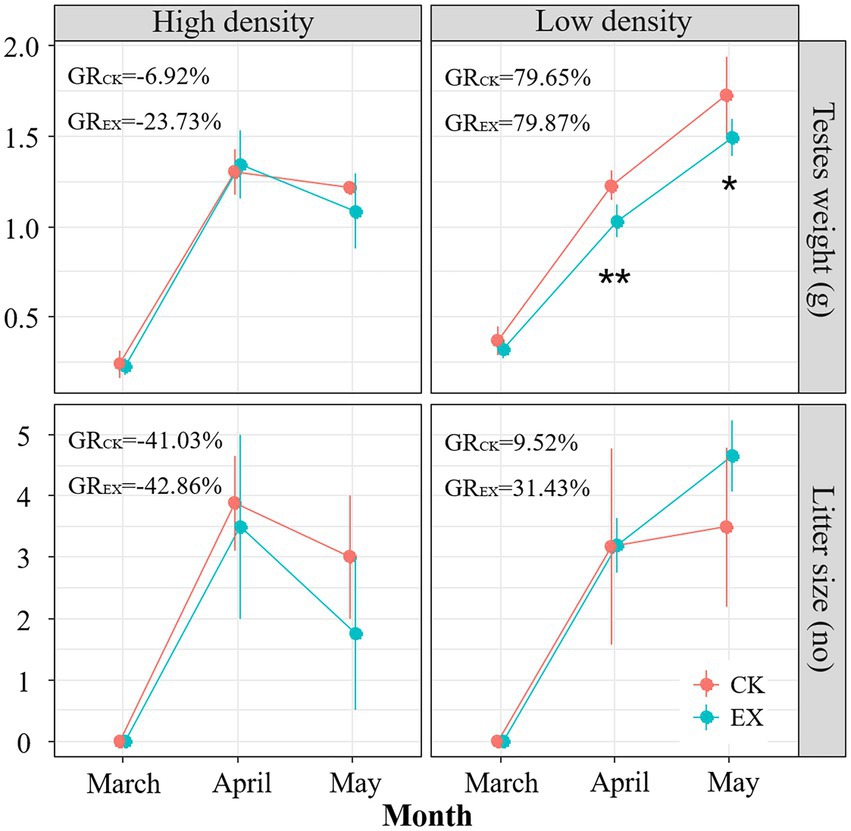
Figure 4. Changes in testicular weight and litter size in plateau pika under predation risk. The blue solid point represents the predation risk treatment group (EX), the red solid point represents the control (CK); dot represents the high population density area (HIGH), the triangle represents the low population density area (LOW). GR denotes the change rate. *p < 0.05; **p < 0.01.
4. Discussion
4.1. Predation risk has no significant effect on the weight of plateau pika
Ecological factors such as food resources, population density and predation risk have great effects on the body weight and somatotype of small mammals (Batzli et al., 1983). A large number of field studies have shown that under the predation risk, the foraging activity of animals is inhibited, the food intake decreases sharply or the metabolic process changes, resulting in the loss of animal weight, so as to make a trade-off between current and future resources (Lima and Dill, 1990; Mcnamara and Houston, 1990; Wei et al., 2004). Our research showed that under the predation risk, the body weight of female plateau pikas increased, while that of males decreased. This is mainly due to the fact that reproduction is an activity with high energy consumption of animals, and male plateau pikas increase their reproductive investment in order to find more mating opportunities or food sources, resulting in weight loss. However, due to the pregnancy during the breeding period, the weight change of the female was significantly higher than that of the male. We also found that predation risk had no significant difference in the effect of predation risk on the weight of plateau pika.
We can explain the result from the following two aspects. Firstly, we consider that it is mainly related to the type of predation risk. Predators are usually divided into three functional categories: resident specialist, nomadic specialist and generalist (Andersson and Erlinge, 1977). Mustela altaica and Mustela nivalis belong to resident specialist, Vulpes ferrilata belong to nomadic specialist, and Gyps himalayensis and Falco cherrug belong to generalist. Klemola et al. (1998) found that generalist predators had no significant effect on the growth rate of small mammals such as Clethrionomys glareolus and Microtus agrestis. Wei et al. (2004) carried out a series of studies on the actual effects of generalists on the behavior and physical condition of plateau pikas. The results showed that the generalist predator did not change the reproduction and bodyweight of the plateau pikas. On the contrary, the resident specialist predators such as Mustela altaica had significant effects on the plateau pika. These predators search, capture and kill prey according to the olfactory signals. In our study, the use of UAV as a predation risk to interfere with plateau pikas is actually a way of interfering with predators such as Gyps himalayensis. Generalists circulate in the sky before predation, which makes plateau pikas alert in advance and rely on complex burrow systems to avoid risks. Secondly, we speculate that the intensity of predation risk in our research had not reached the level that affects the bodyweight of plateau pikas. Like most small mammals, plateau pikas can weigh the risk of predation and make appropriate behavioral decisions. Although UAV increase the risk of plateau pikas, its strength may be within the bearable range of plateau pikas. Plateau pikas can compensate for the increased risk of predation by changing the time allocation of behaviors such as foraging and vigilance.
4.2. The behavior of plateau pika varied greatly among different density populations under the risk of predation
A large number of studies have shown that predation risk will also increase the cost of anti-predator behavior, even when direct killing is not apparent (Preisser and Deegan, 2005; Schmitz, 2008). In many cases, prey respond to high predation risk by reducing their foraging activity, general mobility and by increasing vigilance and their use of safer microhabitats (Apfelbach et al., 2005). When the prey is exposed to the risk of predation for a long time, the prey will still maintain moderate foraging activity in order to meet the minimum energy needs (Lima and Bednekoff, 1999). While the prey is avoiding the predator, it is necessary to increase the intensity of anti-predator. The behavior change induced by the predator increases the energy demand of the prey and seriously affects its foraging efficiency. The lack of energy intake eventually leads to the change of prey fecundity (Creel et al., 2007; Zanette et al., 2011). The results of this study show that under high density pressure, the plateau pika responded to the predation risk by reducing the foraging time and increasing the vigilance time outside the burrows, while the plateau pika in the low population density pressure responded to the predation risk by increasing concealing time in the burrows. And the foraging, vigilance and concealing behavior of plateau pika in high density pressure were not significantly different between the predation risk treatment group and the control, while these behavior in low population density area were significantly different. The reason may be that the increase of population density leads to the decrease of individual predation risk, that is, the “many-eye effect” under predation risk. According to the theory of multi-eye effect, the risk of predation varies with the size of the population, and the increase of population density is beneficial for individuals to find predators earlier. Under the pressure of high population density, plateau pikas are easier to perceive predation risk through information transmission between individuals, and take corresponding measures. This has been confirmed by many related studies in small mammals, such as the earlier human are found with the increase in the population density of Octodon degus.
4.3. Hormones concentration and fecundity of plateau pika in low population density were more affected by predation risk
The adjustment in antipredator responses to cope with predation risk levels might be driven by stress response (Monclús et al., 2009). If predation stress persists, higher glucocorticoid concentrations in chronic exposure can lead damage to mammals, including immunosuppression and weight loss (Mateo, 2007; Sheriff et al., 2009; Clinchy et al., 2011). Experimental data from laboratory studies confirm that predator-induced chronic stress will lead to the decrease of prey individual sex hormone content, and then affect their reproduction (Reeder and Kramer, 2005; Blas et al., 2007). In our research, it was found that under predation risk, the hormone levels of plateau pikas in the low population density were significantly different from those in the control, but the difference was not significant in the high population density. This indicated that predation risk did not affect the hormone secretion in high population density of plateau pika. There are two reasons for this result. One is that the increase of population density makes it easier for plateau pika to find predators and reduce the risk of predation, that is, the “many eyes effect.” Therefore, the plateau pika in high population density avoids the abnormal increase of stress hormones caused by the risk of predation; secondly, due to the high competitive pressure in the high population density of plateau pika, the levels of hormones such as CORT and cortisol have been kept at a high level, predation risk has little effect on hormone levels. But the competition pressure is smaller in the low population density, and the response to predation risk is more obvious. Our results on the behavior patterns of the plateau pikas under predation risk indicated that the foraging and vigilance time of the plateau pikas in the high-density areas were not significantly affected by predation risk. This is also the main reason why there is no significant change in endocrine hormone in plateau pikas. In addition, from the average plant height of different plateau pika population density areas in the study sites, it can be inferred that the average plant height in the low density area is higher than that in the high density area, which increases the predation risk of the plateau pika and further increases the pressure level of the pika.
Predation risk has a significant impact on the reproductive, pregnancy rate and mating activities of prey (Ylönen, 1989). Reproductive activities can increase the risk of predation at all stages of reproductive events (Magnhagen, 1991). Therefore, the presence of predation risk will reduce its reproductive, whereas overwintered small mammals with low residual reproductive value may make their ‘terminal investment’ in the second (and probably last) summer of their life (Korpimaki et al., 1994). In our study, it was found that the predation risk had the most significant effect on the testes weight of male plateau pika in low population density, but not on high density population. We suggested that plateau pika could assess predation risks and make decision to avoid them. Under the low-density population, the plateau pika not only has to deal with the external predation risk, but also face the competitive pressure within the population, such as protecting territory, fighting for mating and food resources, especially in the breeding period. Under high density pressure, plateau pikas maybe increase their inclusive fitness by altruistic behavior to other individuals within the population when predation risk increases, and reduced the risk of individuals within the population. In the study of plateau pika behavior and the response of endocrine hormones to predation risk, we also found that in the face of predation risk, plateau pikas with low population density were more likely to increase concealing time in the burrows, while high-density plateau pikas population tended to increase the vigilance time, and endocrine hormones of plateau pika had no significant effect on the risk of predation under high population density. Therefore, the plateau pika with high population density can reduce the predation risk through the vigilance behavior among individuals within the population, and effectively reduce the inhibition of predation risk on reproduction.
We also found that the effect of predation risk on the litter size of female plateau pikas had no significant difference under high and low population densities. We think that this may be related to the trade-off between reproduction and predation risk of female plateau pikas during breeding period. Sheriff et al. (2009) also found that compared with the control, the litter size of female snowshoe hare (Lepus americanus) under predation risk had no significant difference, but the stress hormone level and body weight of their offspring decreased significantly. In our study of progesterone in female plateau pikas, we also found that predation risk did not significantly inhibit progesterone secretion. This also indirectly indicates that female plateau pikas in the breeding period will not reduce their investment in reproduction under the risk of predation, so as to ensure the birth of offspring. However, this “maternal stress” can affect the entire life cycle of offspring through epigenetic modification (Sophie and McGowan, 2015). To sum up, we have come to the following conclusion. The body weight of the pikas was not significantly different between treatment and control plots. Under the high population density pressure, the plateau pika responded to the predation risk by reducing the foraging time and increasing the vigilance time outside the burrows, while the plateau pika under the low population density pressure responded to the predation risk by increasing concealing time in the burrows. Predation risk could significantly affect the behavior, endocrine hormones and fecundity of plateau pika with low population density, but not on high density population.
5. Application and prospect
In the recent years, due to overgrazing and the global climate change, the alpine meadow has degraded seriously. The degraded meadow provides the plateau pika with a better habitat and results in the overabundance of plateau pika (Liu et al., 2012). In order to manage pests, a variety of methods have been used. The commonly used one to control plateau pika is poisoning, which kills them with botulins of models C and D (Yang, 2017). These methods are limited to reduce the population density and the number of mammals and eliminate the species from the alpine rangeland ecosystem in a period of time and region. This pests control strategy “population elimination” ignores the function and position of the species in the ecosystem and eliminate the function of the species in the ecosystem. In addition, the high frequency and large-scale use of rodenticide has destroyed the integrity of the original food web of rangeland ecosystem, and the natural enemies of pests have plummeted or even become extinct. Thus, it creates more favorable habitat conditions for the occurrence of pests damage. The Chinese government has added these rodenticide types to the restricted list of pesticides, such as botulins of models C and D. Almost all of these rodenticides are prevention and control drugs for plateau pika. Therefore, it is essential to find an effective ecological control method. Over the past 30 years, the ecological control method of introducing natural enemies (eagles, Tibetan fox, etc.) into the field have also been widely used, and some results have been achieved. However, our study found that the ecological control method is limited to the low-density plateau pika population, and the effect on the population with high density is not obvious. Therefore, the prevention and control of pikas damage in alpine rangeland should have varying method of different population densities, that is the ecological control measures such as natural enemies were used in the low density area, and the artificial vegetation was restored in the high density area, which reduced the population density of plateau pika, and then use ecological control when the population density decreased. In this study, UAV was used as the source of predation risk, and the bionic principle was used to interfere with the plateau pika. This efficient, safe and environmental control method can not only control the population of plateau pika, but also achieve the purpose of protecting biodiversity, and has a broad application prospect.
Data availability statement
The original contributions presented in the study are included in the article/Supplementary material, further inquiries can be directed to the corresponding author.
Author contributions
RZ and LH contributed to the conceptualization, formal analysis, funding acquisition, investigation, methodology and project administration, software, supervision, and the writing original draft. RH and ZT contributed to the data curation. RZ contributed to the writing review and editing. All authors contributed to the article and approved the submitted version.
Funding
This work was financially supported by the Engineering and Technology Research Center for Alpine Rodent Pest Control, National Forestry and Grassland Administration; National key research and development program (No. 2017YFC0504803); Gansu province key research and development program (No. 17YF1NA059).
Conflict of interest
The authors declare that the research was conducted in the absence of any commercial or financial relationships that could be construed as a potential conflict of interest.
Publisher’s note
All claims expressed in this article are solely those of the authors and do not necessarily represent those of their affiliated organizations, or those of the publisher, the editors and the reviewers. Any product that may be evaluated in this article, or claim that may be made by its manufacturer, is not guaranteed or endorsed by the publisher.
Supplementary material
The Supplementary material for this article can be found online at: https://www.frontiersin.org/articles/10.3389/fevo.2023.1037377/full#supplementary-material
References
Andersson, M., and Erlinge, S. (1977). Influence of predation on rodent populations. Oikos 29, 591–597. doi: 10.2307/3543597
Apfelbach, R., Blanchard, C. D., Blanchard, R. J., Hayes, R. A., and McGregor, I. S. (2005). The effects of predator odors in mammalian prey species: a review of field and laboratory studies. Neurosci. Biobehav. Rev. 29, 1123–1144. doi: 10.1016/j.neubiorev.2005.05.005
Batzli, G. O., Pitelka, F. A., and Cameron, G. N. (1983). Habitat use by lemmings near barrow, Alaska. Ecography 6, 255–262. doi: 10.1111/j.1600-0587.1983.tb01089.x
Bian, J., and Fan, N. (1997). The effect of predation risk on animals' behavior and their decision. Chinese J. Ecol. 16, 34–39.
Blas, J., Perez-Rodriguez, L., Bortolotti, G., Vinuela, J., and Marchant, T. (2007). Testosterone increases bioavailability of carotenoids: insights into the honesty of sexual signaling. Proc. Natl. Acad. Sci. U. S. A. 103, 18633–18637. doi: 10.1073/pnas.0609189103
Boudreau, M. R., Seguin, J. L., Boonstra, R., Palme, R., Boutin, S., Krebs, C. J., et al. (2019). Experimental increase in predation risk causes a cascading stress response in free-ranging snowshoe hares. Oecologia 191, 311–323. doi: 10.1007/s00442-019-04500-2
Calvete, C., Estrada, R., Angulo, E., and Cabezas-Ruiz, S. (2004). Habitat factors related to wild rabbit conservation in an agricultural landscape. Landsc. Ecol. 19, 531–542. doi: 10.1023/B:LAND.0000036139.04466.06.
Childress, M. J., and Lung, M. A. (2003). Predation risk, gender and the group size effect: does elk vigilance depend upon the behaviour of conspecifics? Anim. Behav. 66, 389–398. doi: 10.1006/anbe.2003.2217
Christianson, D., and Creel, S. (2010). A nutritionally mediated risk effect of wolves on elk. Ecology 91, 1184–1191. doi: 10.1890/09-0221.1
Clinchy, M., Sheriff, M. J., and Zanette, L. Y. (2013). Predator-induced stress and the ecology of fear. Funct. Ecol. 27, 56–65. doi: 10.1111/1365-2435.12007
Clinchy, M., Zanette, L., Charlier, T. D., Newman, A. E. M., Schmidt, K. L., and Soma, B. K. K. (2011). Multiple measures elucidate glucocorticoid responses to environmental variation in predation threat. Oecologia 166, 607–614. doi: 10.1007/s00442-011-1915-2
Cockrem, J. F. (2013). Individual variation in glucocorticoid stress responses in animals. Gen. Comp. Endocrinol. 181, 45–58. doi: 10.1016/j.ygcen.2012.11.025
Creel, S., Christianson, D., Liley, S., and Winnie, J. A. (2007). Predation risk affects reproductive physiology and demography of elk. Science 315, 960–967. doi: 10.1126/science.1135918
Creel, S., Schuette, P., and Christianson, D. (2014). Effects of predation risk on group size, vigilance, and foraging behavior in an african ungulate community. Behav. Ecol. 25, 773–784. doi: 10.1093/beheco/aru050
Dobson, F. S., Smith, A. T., and Wang, X. G. (1998). Social and ecological influences on dispersal and philopatry in the plateau pika (Ochotona curzoniae). Behav. Ecol. 9, 622–635. doi: 10.1093/beheco/9.6.622
Ebensperger, L. A., and Wallem, P. K. (2002). Grouping increases the ability of the social rodent (Octodon degus) to detect predators when using exposed microhabitats. Oikos 98, 491–497. doi: 10.1034/j.1600-0706.2002.980313.x
Hawlena, D., and Schmitz, O. J. (2010). Herbivore physiological response to predation risk and implications for ecosystem nutrient dynamics. Proc. Natl. Acad. Sci. U. S. A. 107, 15503–15507. doi: 10.1073/pnas.1009300107
Hoogland, J. L. (1981). The evolution of coloniality in white-tailed and black-tailed prairie dogs (sciuridae: Cynomys leucurus and C. ludovicianus). Ecology 62, 252–272. doi: 10.2307/1936685
Jędrzejewski, W., Jędrzejewska, B., Okarma, H., and Ruprecht, A. L. (1992). Wolf predation and snow cover as mortality factors in the ungulate community of the bialowieża national park, Poland. Oecologia 90, 27–36. doi: 10.1007/BF00317805
Klemola, T., KorpimaÈki, E., and Norrdahl, K. (1998). Does avian predation risk depress reproduction of voles? Oecologia 115, 149–153. doi: 10.1007/s004420050501
Korpimaki, E., Norrdahl, K., and Valkama, J. (1994). Reproductive investment under fluctuating predation risk: microtine rodents and small mustelids. Evol. Ecol. 8, 357–368. doi: 10.1007/BF01238188
Kotler, B. P., Brown, J. S., and Bouskila, A. (2004). Apprehension and time allocation in gerbils: the effects of predatory risk and energetic state. Ecology 85, 917–922. doi: 10.1890/03-3002
Lafferty, D., Kumar, A., Whitcher, S., Hacklander, K., and Mills, S. (2017). Within-sample variation in snowshoe hare faecal glucocorticoid metabolite measurements. Conserv. Physiol. 5:cox068. doi: 10.1093/conphys/cox068
Lima, S. L., and Bednekoff, P. A. (1999). Temporal variation in danger drives antipredator behavior: the predation risk allocation hypothesis. Am. Nat. 153, 649–659. doi: 10.1086/303202
Lima, S. L., and Dill, L. M. (1990). Behavioral decisions made under the risk of predation: a review and prospectus. Can. J. Zool. 68, 619–640. doi: 10.1139/z90-092
Liu, M., Qu, J., Wang, Z., Wang, Y-I., Zhang, Y., and Zhang, Z. (2012). Behavioral mechanisms of male sterilization on plateau pika in the qinghai-tibet plateau. Behavioural processes 89, 278–285.
Magnhagen, C. (1991). Predation risk as a cost of reproduction. Trees 6, 183–186. doi: 10.1016/0169-5347(91)90210-O
Martin, P., and Bateson, P. (1985). The ontogeny of locomotor play behaviour in the domestic cat. Anim. Behav. 33, 502–510. doi: 10.1016/S0003-3472(85)80073-7
Mateo, J. M. (2007). Ecological and hormonal correlates of antipredator behavior in adult belding’s ground squirrels (Spermophilus beldingi). Behav. Ecol. Sociobiol. 62, 37–49. doi: 10.1007/s00265-007-0436-9
Mcnamara, J. M., and Houston, A. I. (1990). The value of fat reserves and the tradeoff between starvation and predation. Acta Biotheor. 38, 37–61. doi: 10.1007/BF00047272
Monclús, R., Palomares, F., Tablado, Z., Martínez-Fontúrbel, A., and Palme, R. (2009). Testing the threat-sensitive predator avoidance hypothesis: physiological responses and predator pressure in wild rabbits. Oecologia 158, 615–623. doi: 10.1007/s00442-008-1201-0
Navarro-Castilla, L., Sánchez-González, B., and Barja, I. (2019). Latrine behaviour and faecal corticosterone metabolites as indicators of habitat-related responses of wild rabbits to predation risk. Ecol. Indic. 97, 175–182. doi: 10.1016/j.ecolind.2018.10.016
Preisser, M. C., and Deegan, L. A. (2005). Effect of changing plant morphology on invertebrate susceptibility to predation in eelgrass beds. Biol. Bull. 189, 242–243. doi: 10.1086/BBLv189n2p242
R Core Team (2016). R: A language and environment for statistical computing [Computer software manual]. R Foundation for Statistical Computing, Vienna, Austria. Available at: http://www.R-project.org/
Reeder, D. M., and Kramer, K. M. (2005). Stress in free-ranging mammals: integrating physiology, ecology, and natural history. J. Mammal. 86, 225–235. doi: 10.1644/BHE-003.1
Schmitz, O. J. (2008). Effects of predator hunting mode on grassland ecosystem function. Science 319, 952–954. doi: 10.1126/science.1152355
Sheriff, M. J., Krebs, C. J., and Boonstra, R. (2009). The sensitive hare: sublethal effects of predator stress on reproduction in snowshoe hares. J. Anim. Ecol. 78, 1249–1258. doi: 10.1111/j.1365-2656.2009.01552.x
Smith, A. T., Wilson, M. C., and Hogan, B. W. (2018). Functional-trait ecology of the plateau pika Ochotona curzoniae (hodgson, 1858) in the Qinghai-Tibetan Plateau ecosystem. Integr. Zoo. 14, 87–103. doi: 10.1111/1749-4877.12300
Sophie, S. C., and McGowan, P. (2015). Programming of stress-related behavior and epigenetic neural gene regulation in mice offspring through maternal exposure to predator odor. Front. Behav. Neurosci. 9:145. doi: 10.3389/fnbeh.2015.00145
Turner, A. M., and Chislock, M. F. (2010). Blinded by the stink: nutrient enrichment impairs the perception of predation risk by freshwater snails. Ecol. Appl. 20, 2089–2095. doi: 10.1890/10-0208.1
Wang, D., Zhang, J., and Zhang, Z. (2012). Effect of testosterone and melatonin on social dominance and agonistic behavior in male Tscheskia triton. Behav. Process. 89, 271–277. doi: 10.1016/j.beproc.2011.12.010
Wei, W. (2019). The relationship between population density, vegetation community structure and predation risk of plateau pika. Acta Agrestia Sinica 27, 350–355. doi: 10.11733/j.issn.1007-0435.2019.02.011
Wei, W., Yang, S., Fan, N., and Zhou, L. (2004). The response of animal's foraging behaviour to predation risk. Chinese J. Zool. 39, 84–90. doi: 10.3969/j.issn.0250-3263.2004.03.019
Wei, W., and Zhang, W. (2018). Architecture characteristics of burrow system of plateau pika, Ochotona curzoniae. Pak. J. Zool. 50, 311–316. doi: 10.17582/journal.pjz/2018.50.1.311.316
Wei, H., Zhao, J., and Luo, T. (2019). The effect of pika grazing on stipa purpurea is amplified by warming but alleviated by increased precipitation in an alpine grassland. Plant Ecol. 220, 371–381. doi: 10.1007/s11258-019-00920-5
Wilson, M. C., and Smith, A. T. (2015). The pika and the watershed: the impact of small mammal poisoning on the ecohydrology of the Qinghai-Tibetan Plateau. Ambio 44, 16–22. doi: 10.1007/s13280-014-0568-x
Yang, S., Yin, B., Cao, Y., Zhang, Y., Wang, J., and Wei, W. (2007). Reproduction and behavior of plateau pikas (Ochotona curzoniae hodgson) under predation risk: a field experiment. Pol. J. Ecol. 55, 127–138.
Yang, X. (2017). Grassland rodents control and diet selection of plateau zokors in Qinghai province. Lanzhou University.
Yin, B., Yang, S., Shang, G., and Wei, W. (2017). Effects of predation risk on behavior, hormone levels, and reproductive success of plateau pikas. Ecosphere 8:e01643. doi: 10.1002/ecs2.1643
Ylönen, H. (1989). Weasels mustela nivalis suppress reproduction in cyclic bank voles clethrionomys glareolus. Oikos 55, 138–140. doi: 10.2307/3565886
Zanette, L. Y., White, A. F., Allen, M. C., and Clinchy, M. (2011). Perceived predation risk reduces the number of offspring songbirds produce per year. Science 334, 1398–1401. doi: 10.1126/science.1210908
Keywords: predation risk, anti-predator behavior, non-consumptive effects, stress, plateau pika (Ochotona curzoniae)
Citation: Zhou R, Hua R, Tang Z and Hua L (2023) Group-living decrease predation risk of individual: Evidence from behavior, hormones and reproduction of plateau pika. Front. Ecol. Evol. 11:1037377. doi: 10.3389/fevo.2023.1037377
Edited by:
Maria K. Oosthuizen, University of Pretoria, South AfricaReviewed by:
Yang Wang, Hebei Normal University, ChinaJiapeng Qu, Key Laboratory of Adaptation and Evolution of Plateau Biota, Northwest Institute of Plateau Biology (CAS), China
Copyright © 2023 Zhou, Hua, Tang and Hua. This is an open-access article distributed under the terms of the Creative Commons Attribution License (CC BY). The use, distribution or reproduction in other forums is permitted, provided the original author(s) and the copyright owner(s) are credited and that the original publication in this journal is cited, in accordance with accepted academic practice. No use, distribution or reproduction is permitted which does not comply with these terms.
*Correspondence: Limin Hua, hualm@gsau.edu.cn