Occurrences, Sources, and Human Health Risk Assessments of Polycyclic Aromatic Hydrocarbons in Marine Organisms From Temperate Coastal Area
- 1College of Marine Sciences, Shanghai Ocean University, Shanghai, China
- 2College of Ocean Science and Engineering, Shanghai Maritime University, Shanghai, China
- 3State Key Laboratory of Pollution Control and Resources Reuse, Tongji University, Shanghai, China
- 4National Engineering Research Center for Oceanic Fisheries, Shanghai, China
- 5Institute of Industrial Science, The University of Tokyo, Tokyo, Japan
The pollution characteristics of 16 polycyclic aromatic hydrocarbons (PAHs) in marine organism species (6 species of fishes and 2 species of crustaceans) from the coastal area of the East China Sea were determined. The concentrations of 16 PAHs in the studied organisms ranged from 29.73 to 87.02 ng/g dw and 2- and 3-ring PAHs were the most abundant compounds in the aquatic organisms. The habitat, diet and predator-prey relationship have posed potential effects on the PAH accumulation in marine organisms. The source identification of PAHs was performed by using the molecular diagnostic ratios and principal component analysis (PCA). The results showed that the main sources of PAHs in the marine organisms were coal combustion, followed by mixture of gasoline combustion, oil combustion, crude oil spill and vehicle emissions. The incremental lifetime cancer risk of human via ingestion process of marine organisms in this sea area was also estimated and the assessment showed that it posed an acceptable but non-negligible risk to human health.
Introduction
Polycyclic aromatic hydrocarbons (PAHs) have been widely concerned because of their teratogenicity, carcinogenicity and mutagenicity. With the development of social economy, PAHs from industrial production, agricultural production, transportation and domestic pollution sources are increasing gradually (Hu et al., 2008; Jiao et al., 2012; Yoo et al., 2014; Tong et al., 2019). They can enter marine environment through various ways, such as wastewater discharge, atmospheric deposition, surface runoff and crude oil leakage (Heemken et al., 2000). It is worth noting that PAHs tend to accumulate in aquatic organisms through the biological lipid membrane (Billiard et al., 2002) since their lipophilic and hydrophobic properties, and their toxicity can be amplified through the food chain/web, finally causing toxic effects on human health via ingestion (Charles et al., 1992; Chen et al., 2002).
Located in a temperate zone with humid climate and abundant precipitation, the coastal area of the East China Sea owns massive biological resources, especially Zhejiang coastal waters. Zhejiang Province covers a sea area of ∼260,000 square kilometers and its continental coastline and island shoreline extend for ∼6,500 km, accounting for 20.3% of the total length of China’s coastline. The convergence zone of coastal current and Taiwan warm current makes the coastal waters of Zhejiang rich in fishery resources because of its low salinity, great seasonal changes and abundant nutrients. From 2017 to 2019, the average annual marine fishing yield of Zhejiang Province accounted for more than 27% of the that in China (CSF, 2019, 2020). However, the increasing human maritime activities have caused more concerns about the quality of marine environment. As reported in a previous study (Wang X. Y. et al., 2015), the sum of 16 PAH concentrations in the surface sediments samples collected from Zhoushan Archipelago and Xiangshan Harbor ranged from 3.67 to 31.30 ng/g and 11.58 to 481.44 ng/g, respectively. To date, there are still few studies and information which address the current situation of PAH pollution in the marine organisms of this sea area and its potential risk to human health.
The main tasks of this study can be described as follows: (1) Marine organisms will be collected from the temperate coastal area of the East China Sea and used to determine the residual level of 16 priority PAHs; (2) Diagnostic ratios and Principal Component Analysis (PCA) will be applied for identification of the possible sources of PAHs; (3) The safety risk to human health via ingestion of these aquatic products will be clearly evaluated. The purpose of this study is to further understand the level of pollutants in coastal aquatic products in the East China Sea, to provide useful information for the prevention and control of PAH pollution and an assessment for the potential risk of consuming PAH-contaminated seafood in this area.
Materials and Methods
Sample Collection
The study area was set in Zhejiang coastal waters in the East China Sea (Figure 1) and the marine organisms were sampled by bottom trawl at nine sites in April 2019. Eight kinds of high-economic-value marine organisms were selected, including six fishes and two crustaceans. The basic biological information is shown in Supplementary Table 1. After collection, the samples were washed with ultrapure water, stored in PE sealed bags and then transported to the laboratory at –20°C until analysis.
Experimental Section
Materials
Silica gel (60∼100 mesh for chromatography column), anhydrous sodium sulfate, 98% concentrated sulfuric acid, acetone, dichloromethane, n-hexane and other chromatographic pure solvents were purchased from Sinopharm Chemical Reagent Co., Ltd. (Shanghai, China). The silica gel needs to be activated for use according to previous protocol (Wang et al., 1994). The 16 USEPA priority PAH standard mixture including Naphthalene (Nap), Acenaphthylene (Acy), Acenaphthene (Ace), Fluorene (Fl), Phenanthrene (Phe), Anthracene (Ant), Fluoranthene (Flu), Pyrene (Pyr), Benzo[a]Anthracene (BaA), Chrysene (Chr), Benzo[b]fluoranthene (BbF), Benzo[k]fluoranthene (BkF), Benzo[a]pyrene (BaP), Indeno[1,2,3-cd]pyrene (InP), Dibenz[a,h]anthracene (DahA), Benzo[g,h,i]perylene (BghiP) and internal standard (p-Terphenyl-d14) were purchased from Supelco, Inc. (Bellefonte, PA, United States).
Sample Pretreatment
The sample pretreatment method was based on the determination of specified sixteen polycyclic aromatic hydrocarbons in aquatic products by gas chromatography-mass spectrum in Chinese aquatic industry standards (SC/T 3042–2008) and previously established protocols (Yu et al., 2016, 2019). In brief, muscle tissue (0.2–5 g, depending on amount available) was freeze-dried for 24 h, grinded, mixed with 18 mL n-hexane/dichloromethane (2:1, v/v), transferred into 50 mL centrifuge tubes, and vortex mixed for 1 min. Samples were then extracted in an ultrasonic extractor for 30 min, and centrifuged at a rate of 4,800 rad/min for 3 min. The extraction solution was concentrated to 5 mL, followed by washing with sulfuric acid solution (60%, v/v) for derosination and centrifuged at a 4,800 rad/min rate for 3 min, then transferred into a chromatographic column filled with 3 g silica gel and 1 g anhydrous sodium sulfate. The columns were eluted with 12 mL n-hexane/dichloromethane (1:1, v/v). The PAH-containing eluents were collected and placed in a nitrogen stream; the final solution was adjusted to 1 mL by hexane and spiked with p-terphenyl-d14 as an internal standard before instrument analysis.
Gas Chromatography-Mass Spectrometry Conditions
Gas chromatography–mass spectrometry (GC-MS) (Agilent 7890A-5975C) was used for the determination of 16 PAHs. A DB-5 capillary column (15 m × 0.25 mm × 0.25 μm) was used for PAH mixture separation. The inlet temperature was set at 250°C. The initial temperature of GC oven was set at 50°C, held for 3 min, increased to 180°C at a rate of 15°C/min, heated to 300°C at a 6°C/min rate, and held for 2 min. The electron impact (EI) ion source and quadrupole temperatures were set at 230 and 150°C, respectively. The 16 PAHs were detected by selected ion monitoring (SIM) mode and the target ions of PAH compounds were selected based on our previous study (Yin et al., 2015, 2021). Helium (purity > 99.9%) was used as a carrier gas with a flow rate of 1 mL/min. Pulsed splitless injection of 1 μL of the sample solutions was performed.
Quality Assurance/Quality Control
The linear regression coefficients for calibration curves based on five standard concentrations (10, 50, 100, 200, and 500 ng/mL) were greater than 0.99. The limit of detection (LOD) was defined as the concentrations of target compounds that gave rise to a peak with a signal-to-noise ratio (S/N) of 3 (Chen et al., 2007), which varied from 0.1 and 1.9 ng/g. The results showed that no target compounds were detected (below LOD) in the procedure blanks. The measured recoveries of matrix-spiked samples were 61.4 ± 8.8%, 70.9 ± 3.4%, 74.8 ± 6.9%, 122.7 ± 8.2% and 118.6 ± 6.5% for Naphthalene-d8, Acenaphthene-d10, Phenanthrene-d10, Chrysene-d12 and Pyrene-d12, respectively.
Results and Discussion
Concentrations and Composition Patterns of Polycyclic Aromatic Hydrocarbons
The PAH concentrations in marine organisms from the coastal area of Zhejiang in the East China Sea ranged from 29.73 ng/g dw in Konosirus punctatus to 87.02 ng/g dw in Cynoglossus robutus, with an average concentration of 53.35 ng/g dw. Detailed data were summarized in Supplementary Table 2. And the ranges of the concentration levels of Σ16PAHs were illustrated in Figure 2. Varied concentrations of PAHs in the studied marine organisms could be observed among different species and even among the individuals of the same species. According to previous studies, the species-specific differences and individual differences may be attributed to the different feeding behavior, metabolic capability, trophic level and biomagnification ability of each species and individuals (Rahmanpour et al., 2014; de Albergaria-Barbosa et al., 2018; Santana et al., 2018; Yu et al., 2019). As shown in Table 1, the average concentration of Σ16PAHs (53.35 ng/g) in the marine organisms of this study was close to those from Nansha and Xisha Islands (67.79 and 71.69 ng/g, Li et al., 2019), lower than those from South China Sea (289.86 ng/g, Ke et al., 2017), Ghana coast of West Africa (192 ng/g, Bandowe et al., 2014c), Tanmen of China (161.46 ng/g, Li et al., 2021) and Dongfang and Yachen gas fields and the Pearl River Delta of China (386 ng/g, Yu et al., 2019), but higher than those from Zhuhai of China (31.21 ng/g, Li et al., 2021) and the northern Bering Sea Shelf and Chukchi Sea Shelf (34.97 ng/g, Ma et al., 2020). The comparison of PAH concentrations with this respect indicated that the marine organisms from the coastal area of Zhejiang in the East China Sea were moderately contaminated by PAHs.
The composition pattern of PAHs in the marine species was presented in Figure 3. The relative compositions of PAHs were similar to each other, 2- and 3-ring PAHs were predominant in the marine organism tissues, accounting for 42.69 and 49.46%, respectively; followed by 4- and 5-ring PAHs, accounting for 5.90 and 1.93%, respectively. It is reported that low molecular weight polycyclic aromatic hydrocarbons (LMW PAHs) have higher gill-water transfer efficiency due to higher water solubility, while high molecular weight polycyclic aromatic hydrocarbons (HMW PAHs) tend to combine with particles (Baumard et al., 1999; Sun et al., 2016). Also, previous studies have shown that the removal or biotransformation rate of HMW PAHs is higher than that of LMW PAHs (Baumard et al., 1998). This may explain the reason why marine organisms are prone to the occurrence of LMW PAHs. Furthermore, it is stated that the habitats can be an important factor to affect the distribution characteristics of PAHs in marine organisms (Snyder et al., 2015). The coastal areas of Zhejiang Province including Zhoushan Archipelago and Xiangshan Harbor, and some nearby cities with high industrialization, such as Shanghai and Nantong, have built many oil refineries, coal power plants and petrochemical plants (Wang X. Y. et al., 2015). And the pollution emissions from these plants will affect the composition of PAHs in the water bodies and surface sediments of the surrounding sea. Jiang et al. (2014) sampled seawater and surface sediments from Zhoushan coastal area, and their results showed that 2- and 3-ring PAHs in seawater and sediment samples were predominant. This probably leads to higher accumulation of LMW PAHs in the studied aquatic products in this region (Badreddine et al., 2016; Margherit et al., 2018; Webster et al., 2018). Our results showed the composition of PAHs in the marine organism samples posed a potential correlation between the compositions of PAHs in the organisms and their habitat. Differences in physiology and diet also likely account for the differences in PAH exposure for aquatic organisms (Snyder et al., 2015). As shown in Supplementary Table 1, C. robutus, which has the highest PAH concentrations, is a typical benthic fish that is in contact with bottom sediments for long periods of time. Some studies have shown that soil and sediment are the greatest sinks for PAHs (Huang et al., 2018), hence C. robutus may accumulate more PAHs from sediments. This is consistent with some existing findings that benthic organisms in contact with the sediments tend to have a stronger accumulation capacity for PAHs than aquatic organisms in contact with the water (Nakata et al., 2003). Furthermore, the least abundant PAHs was detected in K. punctatus, which mainly feed on phytoplankton, and higher PAH contents were detected in carnivorous fishes that feed on other small fishes. It may suggest that the carnivorous fish species exhibited higher PAH concentrations than the herbivorous ones. This result is consistent with a previous study on the accumulation potential of persistent organic pollutants (POPs) such as polychlorinated biphenyls (PCBs) in freshwater fish species (Eqani et al., 2013). The food web also affects the concentration levels of PAH compounds. For example, Nap accounted for the highest proportion in the two crustaceans, which may cause a relatively high proportion of Nap in Pennahia argentata that feed on crustaceans.
Source Appointment
Molecular Diagnostic Ratios
The molecular diagnostic ratio method has been commonly used to discriminate the source of PAH pollution. Since PAH compounds that are isomers often have similar thermodynamic partition coefficients and kinetic mass transfer coefficients, the content ratio of single-component compounds of PAHs has been widely used to identify the sources of PAH contamination in environmental samples (Hellou et al., 2005; Nasr et al., 2010; Tobiszewski and Namiesnik, 2012; Zhang et al., 2021), even these ratios may not be stable in organisms due to the complexity of metabolic processes and the particularity of different hydrocarbons.
The commonly used molecular diagnostic ratios include Ant/(Phe + Ant) and Flu/(Flu + Pyr). When Ant/(Phe + Ant) < 0.1 or Flu/(Flu + Pyr) < 0.4, the main source of PAHs is petroleum source; when Ant/(Phe + Ant) > 0.1, the main source of PAHs is combustion source; when 0.4 < Flu/(Flu + Pyr) < 0.5, the main source of PAHs is natural gas, diesel, oil and other combustion; when Flu/(Flu + Pyr) > 0.5, the main sources of PAHs are biomass and coal combustion (Yunker et al., 2002). The cross plot of Ant/(Phe + Ant) and Flu/(Flu + Pyr) was selected to draw the PAH source of the studied samples. As indicated in Figure 4, all of the collected fish samples had PAHs mainly from combustion. Since the samples were collected in the nearshore of Zhejiang coastal area of the East China Sea, they are susceptible to land-based sources of production and domestic pollutant emissions.
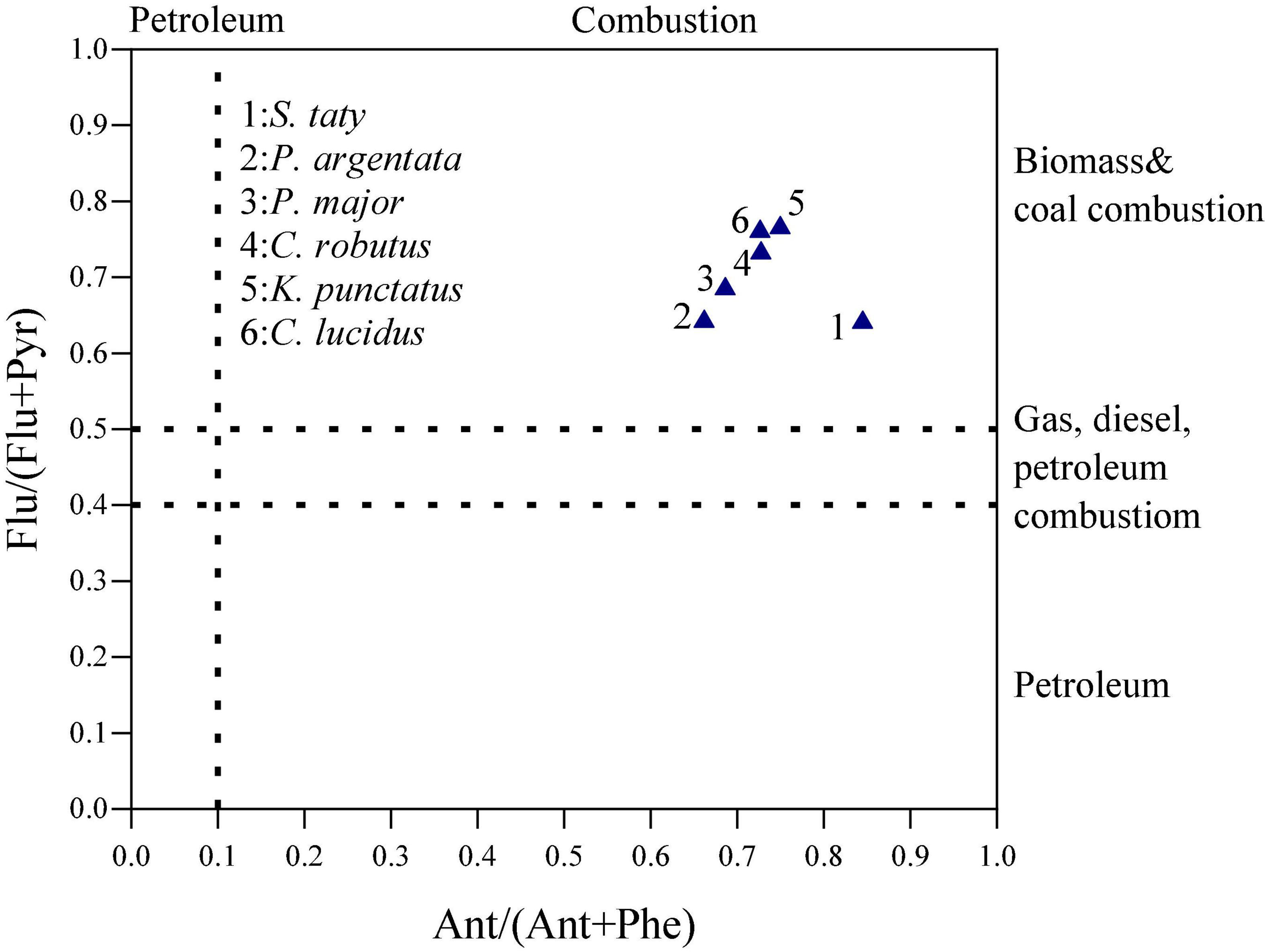
Figure 4. Cross plot of the molecular diagnostic ratios of Flu/(Flu + Pyr) and Ant/(Phe + Ant) in the marine organisms.
Principal Component Analysis
PCA is a multivariate analytical tool for receptor modeling in environmental source identification studies, which can represent the total variability of the original PAH data with a minimum number of factors (Liu et al., 2009). It provides a dimensionality reduction method to reduce the number of variables, reflecting most of the information of the original variables (Javed et al., 2008). The main advantage of this method is that it requires little information of the pollutant source or predetermined characteristics of the source (Sofowote et al., 2008). The concentrations of PAH variables can be considered as a linear combination of many potential factors, and the samples are identified by classifying them through several comprehensive characteristic indicators and comparing the loadings to infer the source of contamination (Liu et al., 2009). In this study, source analysis of the 11 detected PAHs was performed by using IBM SPSS Statistics 25.0 to identify three principal components (PC1, PC2, and PC3), calculate the cumulative variance contribution of the principal components, and obtain the correlated factor loading matrix (see Supplementary Table 3).
The results of PCA in Figure 5 and Supplementary Table 3 showed that the cumulative contribution of the three factors reached 81.487%. The contribution of PC1 was 54.698%, where Ace, Phe, Ant, Flu, Pyr, and BbF had large loading values, and Phe, Ant, Flu and Pyr are mainly emitted by coal combustion (Harrison et al., 1996; Simoneit, 2002; Yunker et al., 2002; Li et al., 2006; Tian et al., 2009), indicating that PC1 was coal combustion. The contribution of PC2 was mainly composed of Fl and BaP, explaining 16.064% of the total variance. Fl is an important tracer of coking (Sofowote et al., 2008; Wang C. H. et al., 2015), and BaP is mainly from gasoline combustion (Simoneit, 2002; Yunker et al., 2002; Sofowote et al., 2008) and vehicles (Randolph and Joel, 2003; Li et al., 2006), hence PC2 is a mixed source of coking, gasoline combustion, and vehicle emissions. PC3 only has a high percentage of Nap, and studies show that Nap is a characteristic indicator of crude oil spill (Patrolecco et al., 2010; Deng et al., 2013) and oil combustion (Dong and Lee, 2009; Khairy and Lohmann, 2013). Therefore, PC3 was identified as a mixed source of oil combustion and crude oil spill.
Combining the analysis results of two PAH source identification methods above, it is found that the results of these two methods are basically consistent. The results determined that the most important source of PAHs in the near-shore marine organisms in the study area was coal combustion, followed by a mixture of gasoline combustion, oil combustion, crude oil spill, and vehicle emissions. A possible explanation for this result was that marine organisms in the coastal waters of Zhejiang province were affected by the pollution from coal-powered plants, petroleum refineries and petrochemical plants in surrounding cities. This finding was consistent with previous field investigations in the highly industrialized harbor and sea areas (Bandowe et al., 2014a; Benali et al., 2017). It should be noted that most of the marine organisms captured by trawling was not migratory but stationary. The observed results of PAHs in the marine organisms may reflect the pollution situation around the study site.
Health Risk Assessment
Diet is one of the main ways that people are exposed to PAHs in their daily life. Numerous international studies have evaluated the combined carcinogenic effect of PAHs to human health, and the Incremental Lifetime Cancer Risk (ILCR) has been widely used to evaluate the risk of PAHs. To gain the carcinogenic risk level, the toxic equivalent factor (TEF) of 16 PAHs to BaP have been commonly used to assess the risk of PAHs in organisms by calculating their toxic equivalent concentration (TEQ) of BaP. The commonly applied TEFs have been proposed by Nisbet and LaGoy (Nisbet, 1992; see Supplementary Table 4). The equations for TEQ and ILCR are shown as follows:
where TEFi is the toxicity equivalence factor of this compound i relative to that of BaP; Ci is the concentration of an individual PAH compound i in marine organisms tissue.
where CSF is the oral cancer slope factor of BaP of 7.3 mg/kg/day; IR is daily intake rate of different organisms. The average daily consumption is assumed to be 59, 35, and 49 g/day for fish, mollusk, and crustacean species, respectively (Zhang et al., 2013). EF is exposure frequency (365 days/year); ED is exposure duration, taken as the average human life span of 70 years (Bandowe et al., 2014b). BW is average bodyweight, assumed to be 60 kg (Adomako et al., 2011). AT is average exposure time, assumed as 25,550 days in this study.
After calculation, the ILCR values for marine organisms from the coastal area of the East China Sea are shown in Figure 6, ranging from 4.32 × 10–7 (P. trituberculatus) to 2.54 × 10–5 (P. major). According to the fish advisory committees and safe eating guidelines from the USEPA, an ILCR of 1 × 10–5 is regarded as the maximum acceptable risk level (ARL), while the priority risk level of 1 × 10–4 is considered as serious (USEPA, 2000). Except S. taty and P. major, the values of other samples in the present study were well below the ARL, indicating low carcinogenic risk to local residents near the East China Sea.
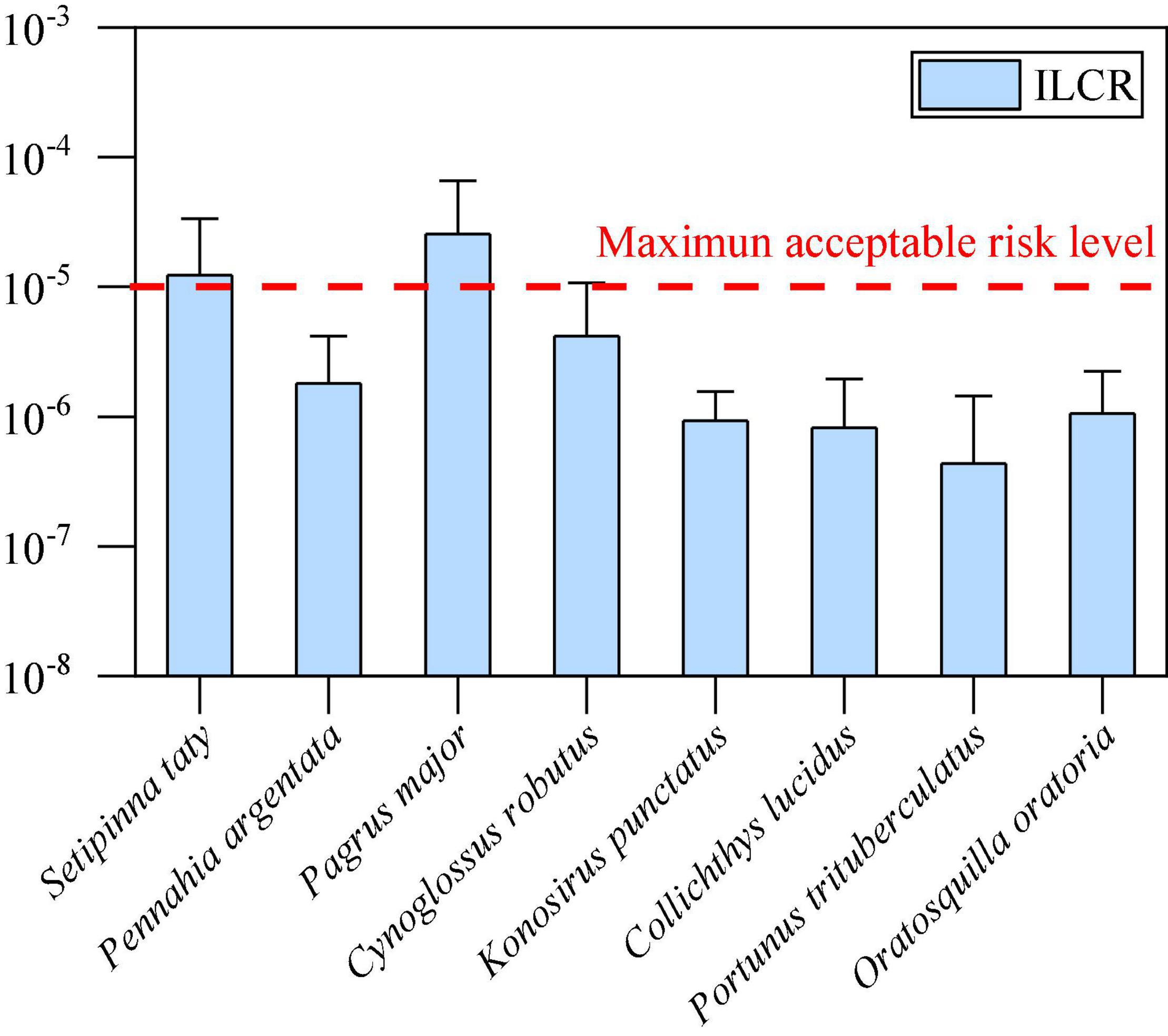
Figure 6. Ranges of the estimated ILCRs by consuming the marine organisms collected from this study area.
Conclusion
This study investigated the concentrations and compositional patterns of 16 PAHs in the marine organisms from the coastal area of the East China Sea. The PAHs with 2- and 3-ring were the dominance, then followed by 4-ring. The marine organisms in the study area were moderately contaminated by PAHs when compared with the marine organisms from different sea areas. The PAH accumulation in marine organisms has shown discernable connections with their habitats, diets and predator-prey relationships. The results of the source identification showed that PAH pollution in marine organisms from the Zhejiang coastal area of the East China Sea mainly originated from coal combustion. The incremental lifetime cancer risk from PAH-contaminated seafood consumption was significantly lower than the priority risk level (10–4), but potential risks still should be carefully considered in the study sea area. The results are expected to provide useful baseline information of the PAH pollution in the coastal aquatic products from the temperate coastal area of China, and to further support the control of PAH pollution and environmental management in this area.
Data Availability Statement
The original contributions presented in the study are included in the article/Supplementary Material, further inquiries can be directed to the corresponding author/s.
Author Contributions
SJ: conceptualization, methodology, data curation, writing—original draft, and writing—review and editing. FY: resources, writing—review and editing, supervision, and funding acquisition. WZ: writing—review and editing. ZS: data curation. BQ: investigation. PS: resources. JZ: writing—review and editing, resources, and supervision. DK: writing—review and editing and supervision. All authors contributed to the article and approved the submitted version.
Funding
This research was partly supported by the National Natural Science Foundation of China (grant no. 41807341) and the National Key Research and Development Program of China (grant no. 2019YFC0312104).
Conflict of Interest
The authors declare that the research was conducted in the absence of any commercial or financial relationships that could be construed as a potential conflict of interest.
Publisher’s Note
All claims expressed in this article are solely those of the authors and do not necessarily represent those of their affiliated organizations, or those of the publisher, the editors and the reviewers. Any product that may be evaluated in this article, or claim that may be made by its manufacturer, is not guaranteed or endorsed by the publisher.
Supplementary Material
The Supplementary Material for this article can be found online at: https://www.frontiersin.org/articles/10.3389/fevo.2022.850247/full#supplementary-material
References
Adomako, E. E., Williams, P. N., Deacon, C., and Meharg, A. A. (2011). Inorganic arsenic and trace elements in Ghanaian grain staples. Environ. Poll. 159, 2435–2442. doi: 10.1016/j.envpol.2011.06.031
Badreddine, B., Yassine, E. M., Christelle, C., Walid, B. A., Sabrine, M., Sondes, B., et al. (2016). Occurrence of polycyclic aromatic hydrocarbons (PAHs) in mussel (Mytilus galloprovincialis) and eel (Anguilla anguilla) from Bizerte lagoon, Tunisia, and associated human health risk assessment. Continent. Shelf Res. 124, 104–116. doi: 10.1016/j.csr.2016.05.012
Bandowe, B. A. M., Bigalke, M., Boamah, L., Nyarko, E., Saalia, F. K., and Wilcke, W. (2014a). Polycyclic aromatic compounds (PAHs and oxygenated PAHs) and trace metals in fish species from Ghana (West Africa): bioaccumulation and health risk assessment. Environ. Int. 65, 135–146. doi: 10.1016/j.envint.2013.12.018
Bandowe, B. A. M., Meusel, H., Huang, R. J., Ho, K., Cao, J., Hoffmann, T., et al. (2014b). PM2.5-bound oxygenated PAHs, nitro-PAHs and parent-PAHs from the atmosphere of a Chinese megacity: Seasonal variation, sources and cancer risk assessment. Sci. Total Environ. 473-474, 77–87. doi: 10.1016/j.scitotenv.2013.11.108
Bandowe, B. A. M., Bigalke, M., Boamah, L., Nyarko, E., and Wilcke, W. (2014c). Polycyclic aromatic compounds (PAHs and oxygenated PAHs) and trace metals in fish species from Ghana (West Africa): Bioaccumulation and health risk assessment. Environ. Int. 65, 135–146.
Baumard, P., Budzinski, H., Garrigues, P., Dizer, H., and Hansen, P. D. (1999). Polycyclic aromatic hydrocarbons in recent sediments and mussels (Mytilus edulis) from the Western Baltic Sea: occurrence, bioavailability and seasonal variations. Mar. Environ. Res. 47, 17–47.
Baumard, P., Budzinski, H., Garrigues, P., Sorbe, J. C., Burgeot, T., and Bellocq, J. (1998). Concentrations of PAHs (polycyclic aromatic hydrocarbons) in various marine organisms in relation to those in sediments and to trophic level. Mar. Poll. Bull. 36, 951–960.
Benali, I., Boutiba, Z., Grandjean, D., de Alencastro, L. F., Rouane-Hacene, O., and Chevre, N. (2017). Spatial distribution and biological effects of trace metals (Cu, Zn, Pb, Cd) and organic micropollutants (PCBs, PAHs) in mussels Mytilus galloprovincialis along the Algerian west coast. Mar. Poll. Bull. 115, 539–550. doi: 10.1016/j.marpolbul.2016.12.028
Billiard, S. M., Hahn, M. E., Franks, D. G., Peterson, R. E., Bols, N. C., and Hodson, P. V. (2002). Binding of polycyclic aromatic hydrocarbons (PAHs) to teleost aryl hydrocarbon receptors (AHRs). Comparat. Biochem. Physiol. Part B 133, 55–68. doi: 10.1016/s1096-4959(02)00105-7
Charles, A. M., Bonnie, B. P., and Joseph, S. (1992). Exposure to carcinogenic PAHs in the environment. Environ. Sci. Technol. 26, 1278–1284.
Chen, S. Y., Wang, L. Y., Lunn, R. M., Tsai, W. Y., Lee, P. H., Lee, C. S., et al. (2002). Polycyclic aromatic hydrocarbon-DNA adducts in liver tissues of hepatocellular carcinoma patients and controls. Int. J. Cancer 99, 14–21. doi: 10.1002/ijc.10291
Chen, Y. Y., Zhu, L. Z., and Zhou, R. B. (2007). Characterization and distribution of polycyclic aromatic hydrocarbon in surface water and sediment from Qiantang River, China. J. Hazard. Materials 141, 148–155. doi: 10.1016/j.jhazmat.2006.06.106
CSF (2019). 2019 China fisheries statistical yearbook. Ministry of Agriculture and Rural Affairs of hte People’s Republic of China. Beijing: CSF.
CSF (2020). 2020 China fisheries statistical yearbook. Ministry of Agriculture and Rural Affairs of hte People’s Republic of China. Beijing: CSF.
de Albergaria-Barbosa, A. C. R., da Silva, D. A. M., da Silva Rocha, A. J., Taniguchi, S., Patire, V. F., Dias, J. F., et al. (2018). Evaluation of polycyclic aromatic hydrocarbons bioavailability on Santos Bay (Brazil) through levels of biliary metabolites. Mar. Poll. Bull. 129, 822–828. doi: 10.1016/j.marpolbul.2017.10.006
Deng, W., Li, X. G., Li, S. Y., Ma, Y. Y., and Zhang, D. H. (2013). Source apportionment of polycyclic aromatic hydrocarbons in surface sediment of mud areas in the East China Sea using diagnostic ratios and factor analysis. Mar. Poll. Bull. 70, 266–273. doi: 10.1016/j.marpolbul.2013.02.032
Dong, T. T. T., and Lee, B. K. (2009). Characteristics, toxicity, and source apportionment of polycylic aromatic hydrocarbons (PAHs) in road dust of Ulsan, Korea. Chemosphere 74, 1245–1253. doi: 10.1016/j.chemosphere.2008.11.035
Eqani, S. A., Malik, R. N., Cincinelli, A., Zhang, G., Mohammad, A., Qadir, A., et al. (2013). Uptake of organochlorine pesticides (OCPs) and polychlorinated biphenyls (PCBs) by river water fish: the case of River Chenab. Sci. Total Environ. 450-451, 83–91. doi: 10.1016/j.scitotenv.2013.01.052
Harrison, R. M., Smith, D. J. T., and Luhana, L. (1996). Source apportionment of atmospheric polycyclic aromatic hydrocarbons collected from an urban location in Birmingham, U.K. Environ. Sci. Technol. 30, 825–832.
Heemken, O. P., Stachel, B., Theobald, N., and Wenclawiak, B. W. (2000). Temporal variability of organic micropollutants in suspended particulate matter of the River Elbe at Hamburg and the River Mulde at Dessau, Germany. Arch. Environ. Contaminat. Toxicol. 38, 11–31. doi: 10.1007/s002449910003
Hellou, J., Steller, S., Leonard, J., Langille, M. A., and Tremblay, D. (2005). Partitioning of polycyclic aromatic hydrocarbons between water and particles compared to bioaccumulation in mussels: a harbour case. Mar. Environ. Res. 59, 101–117. doi: 10.1016/j.marenvres.2004.04.001
Hu, J., Liu, W. X., Chen, J. L., Fan, Y. S., Xing, B. S., Kang, H., et al. (2008). Distribution and property of polycyclic aromatic hydrocarbons in littoral surface sediments from the Yellow Sea, China. J. Environ. Sci. Health Part A 43, 382–389. doi: 10.1080/10934520701795582
Huang, Y. P., Sun, X., Liu, M., Zhu, J. M., Yang, J., Du, W. N., et al. (2018). A multimedia fugacity model to estimate the fate and transport of polycyclic aromatic hydrocarbons (PAHs) in a largely urbanized area, Shanghai, China. Chemosphere 217, 298–307. doi: 10.1016/j.chemosphere.2018.10.172
Javed, I., Edward, B. O., and David, G. (2008). Sources of polycyclic aromatic hydrocarbons in Louisiana rivers and coastal environments: Principal components analysis. Environ. Forens. 9, 310–319. doi: 10.1080/15275920802501838
Jiang, M., Le, H. T., Mei, W. P., Ruan, H. H., and Wu, H. (2014). Distribution of polycyclic aromatic hydrocarbons in water and sediment from Zhoushan Coastal Area, China(in Chinese). Environ. Sci. 35, 2672–2679.
Jiao, W. T., Wang, T. Y., Khim, J. S., Luo, W., John, P. G., Hu, W. Y., et al. (2012). PAHs in surface sediments from coastal and estuarine areas of the northern Bohai and Yellow Seas, China. Environ. Geochem. Health 34, 445–456. doi: 10.1007/s10653-011-9445-8
Ke, C. L., Gu, Y. G., Liu, Q., Li, L. D., Huang, H. H., Cai, N., et al. (2017). Polycyclic aromatic hydrocarbons (PAHs) in wild marine organisms from South China Sea: Occurrence, sources, and human health implications. Mar. Poll. Bull. 117, 507–511. doi: 10.1016/j.marpolbul.2017.02.018
Khairy, M. A., and Lohmann, R. (2013). Source apportionment and risk assessment of polycyclic aromatic hydrocarbons in the atmospheric environment of Alexandria, Egypt. Chemosphere 91, 895–903. doi: 10.1016/j.chemosphere.2013.02.018
Li, J., Zhang, G., Li, X. D., Qi, S. H., Liu, G. Q., and Peng, X. Z. (2006). Source seasonality of polycyclic aromatic hydrocarbons (PAHs) in a subtropical city, Guangzhou, South China. Sci. Total Environ. 355, 145–155. doi: 10.1016/j.scitotenv.2005.02.042
Li, Y. L., Wang, C. L., Zou, X. Q., Feng, Z. Y., Yao, Y. L., Wang, T., et al. (2019). Occurrence of polycyclic aromatic hydrocarbons (PAHs) in coral reef fish from the South China Sea. Mar. Poll. Bull. 139, 339–345. doi: 10.1016/j.marpolbul.2019.01.001
Li, Y., Guo, N., Zou, X., Li, P., Zou, S., Luo, J., et al. (2021). Pollution level and health risk assessment of polycyclic aromatic hydrocarbons in marine fish from two coastal regions, the South China Sea. Mar. Poll. Bull. 168:112376. doi: 10.1016/j.marpolbul.2021.112376
Liu, Y., Chen, L., Huang, Q. H., Li, W. Y., Tang, Y. J., and Zhao, J. F. (2009). Source apportionment of polycyclic aromatic hydrocarbons (PAHs) in surface sediments of the Huangpu River, Shanghai, China. Sci. Total Environ. 407, 2931–2938. doi: 10.1016/j.scitotenv.2008.12.046
Ma, Y. X., Sun, Y. R., Li, Y. K., Zheng, H. Y., and Mi, W. Y. (2020). Polycyclic aromatic hydrocarbons in benthos of the northern Bering Sea Shelf and Chukchi Sea Shelf. J. Environ. Sci. 97, 194–199. doi: 10.1016/j.jes.2020.04.021
Margherit, A. F., Guido, Z., Antonio, C., Chiara, C., Alfina, G., Maria, F., et al. (2018). PAHs in seafood from the Mediterranean Sea: An exposure risk assessment. Food Chemical Toxicol. 115, 385–390. doi: 10.1016/j.fct.2018.03.024
Nakata, H., Sakai, Y., Miyawaki, T., and Takemura, A. (2003). Bioaccumulation and toxic potencies of polychlorinated biphenyls and polycyclic aromatic hydrocarbons in tidal flat and coastal ecosystems of the Ariake Sea, Japan. Environ. Sci. Technol. 37, 3513–3521. doi: 10.1021/es021083h
Nasr, I. N., Arief, M. H., Abdel-Aleem, A. H., and Malhat, F. M. (2010). Polycyclic Aromatic Hydrocarbons (PAHs) in Aquatic Environment at El Menofiya Governorate, Egypt. J. Appl. Sci. Res. 6, 13–21.
Nisbet, I. C. T. (1992). Toxic equivalency factors (TEFs) for polycyclic aromatic hydrocarbons (PAHs). Regulat. Toxicol. Pharmacol. 16, 290–300.
Patrolecco, L., Ademollo, N., Capri, S., Pagnotta, R., and Polesello, S. (2010). Occurrence of priority hazardous PAHs in water, suspended particulate matter, sediment and common eels (Anguilla anguilla) in the urban stretch of the River Tiber (Italy). Chemosphere 81, 1386–1392. doi: 10.1016/j.chemosphere.2010.09.027
Rahmanpour, S., Farzaneh Ghorghani, N., and Lotfi Ashtiyani, S. M. (2014). Polycyclic aromatic hydrocarbon (PAH) in four fish species from different trophic levels in the Persian Gulf. Environ. Monit. Assess. 186, 7047–7053. doi: 10.1007/s10661-014-3909-0
Randolph, K. L., and Joel, E. B. (2003). Source apportionment of polycyclic aromatic hydrocarbons in the urban atmosphere: A comparison of three methods. Environ. Sci. Technol. 37, 1873–1881. doi: 10.1021/es0206184
Santana, M. S., Sandrini-Neto, L., Neto, F. F., Oliveira Ribeiro, C. A., Di Domenico, M., and Prodocimo, M. M. (2018). Biomarker responses in fish exposed to polycyclic aromatic hydrocarbons (PAHs): Systematic review and meta-analysis. Environ. Poll. 242, 449–461. doi: 10.1016/j.envpol.2018.07.004
Simoneit, B. R. T. (2002). Biomass burning—a review of organic tracers for smoke from incomplete combustion. Appl. Geochem. 17, 129–162. doi: 10.1016/s0883-2927(01)00061-0
Snyder, S. M., Pulster, E. L., Wetzel, D. L., and Murawski, S. A. (2015). PAH exposure in gulf of Mexico demersal fishes, post-Deepwater Horizon. Environ. Sci. Technol. 49, 8786–8795. doi: 10.1021/acs.est.5b01870
Sofowote, U. M., Mccarry, B. E., and Marvin, C. H. (2008). Source apportionment of PAH in Hamilton Harbour suspended sediments: comparison of two factor analysis methods. Environ. Sci. Technol. 42, 6007–6014.
Sun, R. X., Lin, Q., Ke, C. L., Du, F. Y., Gu, Y. G., Cao, K., et al. (2016). Polycyclic aromatic hydrocarbons in surface sediments and marine organisms from the Daya Bay, South China. Mar. Poll. Bull. 103, 325–332. doi: 10.1016/j.marpolbul.2016.01.009
Tian, F. L., Chen, J. W., Qiao, X. L., Wang, Z. D., Yang, P., Wang, D. G., et al. (2009). Sources and seasonal variation of atmospheric polycyclic aromatic hydrocarbons in Dalian, China: Factor analysis with non-negative constraints combined with local source fingerprints. Atmos. Environ. 43, 2747–2753.
Tobiszewski, M., and Namiesnik, J. (2012). PAH diagnostic ratios for the identification of pollution emission sources. Environ. Poll. 162, 110–119. doi: 10.1016/j.envpol.2011.10.025
Tong, Y. F., Chen, L., Liu, Y., Wang, Y. N., and Tian, S. Y. (2019). Distribution, sources and ecological risk assessment of PAHs in surface seawater from coastal Bohai Bay, China. Mar. Poll. Bull. 142, 520–524. doi: 10.1016/j.marpolbul.2019.04.004
USEPA (2000). Guidance for Assessing Chemical Contaminant Data for Use in Fish Advisories - Volume 2: Risk Assessment and Fish Consumption Limits Third Edition. Washington, D.C: USEPA.
Wang, C. H., Wu, S. H., Zhou, S. L., Wang, H., Li, B. J., Chen, H., et al. (2015). Polycyclic aromatic hydrocarbons in soils from urban to rural areas in Nanjing: Concentration, source, spatial distribution, and potential human health risk. Sci. Total Environ. 527-528, 375–383. doi: 10.1016/j.scitotenv.2015.05.025
Wang, X. Y., Xu, H. Z., Zhou, Y. D., Wu, C. W., and Kanchanopas-Barnette, P. (2015). Distribution and source apportionment of polycyclic aromatic hydrocarbons in surface sediments from Zhoushan Archipelago and Xiangshan Harbor, East China Sea. Mar. Poll. Bull. 101, 895–902. doi: 10.1016/j.marpolbul.2015.10.073
Wang, Z. D., Fingas, M., and Li, K. (1994). Fractionation of a light crude oil and identification and quantitation of aliphatic, aromatic, and biomarker compounds by GC-FID and GC-MS, Part II. J. Chromatogr. Sci. 32, 367–382. doi: 10.1093/chromsci/32.9.367
Webster, L., Russell, M., Shepherd, N., Packer, G., Dalgarno, E. J., and Neat, F. (2018). Monitoring of polycyclic aromatic hydrocarbons (PAHs) in Scottish Deepwater environments. Mar. Poll. Bull. 128, 456–459. doi: 10.1016/j.marpolbul.2018.01.049
Yin, F., John, G. F., Hayworth, J. S., and Clement, T. P. (2015). Long-term monitoring data to describe the fate of polycyclic aromatic hydrocarbons in Deepwater Horizon oil submerged off Alabama’s beaches. Sci. Total Environ. 508, 46–56. doi: 10.1016/j.scitotenv.2014.10.105
Yin, F., Song, Z., Wei, Q., Zhao, X., Su, P., and Huang, H. (2021). Characterization of polycyclic aromatic hydrocarbons in the surface sediments around the sunken Sanchi oil tanker. Mar. Pollut. Bull. 164:112098. doi: 10.1016/j.marpolbul.2021.112098
Yoo, M. Y., Lee, S. H., Kim, S. Y., Kim, S. J., Seo, H. Y., and Shin, D. B. (2014). A comparative study of the analytical methods for the determination of polycyclic aromatic hydrocarbons in seafood by high-performance liquid chromatography with fluorescence detection. Int. J. Food Technol. 49, 1480–1489. doi: 10.1016/j.talanta.2011.11.060
Yu, Z. L., Lin, Q., Gu, Y. G., Du, F. Y., Wang, X. H., Shi, F. Q., et al. (2019). Bioaccumulation of polycyclic aromatic hydrocarbons (PAHs) in wild marine fish from the coastal waters of the northern South China Sea: Risk assessment for human health. Ecotoxicol. Environ. Safety 180, 742–748. doi: 10.1016/j.ecoenv.2019.05.065
Yu, Z. L., Lin, Q., Gu, Y. G., Ke, C. L., and Sun, R. X. (2016). Spatial–temporal trend and health implications of polycyclic aromatic hydrocarbons (PAHs) in resident oysters, South China Sea: A case study of Eastern Guangdong coast. Mar. Poll. Bull. 110, 203–211. doi: 10.1016/j.marpolbul.2016.06.061
Yunker, M. B., Macdonald, R. W., Vingazan, R., Mitchell, R. H., Goyette, D., and Sylvestre, S. (2002). PAHs in the Fraser River basin: a critical appraisal of PAH ratios as indicators of PAH source and composition. Organic Geochem. 33, 489–515. doi: 10.1016/s0146-6380(02)00002-5
Zhang, B., Zhai, F. Y., Du, S. F., and Popkin, B. M. (2013). The China health and nutrition survey, 1989–2011. Obes. Rev. 15, 2–7 doi: 10.1111/obr.12119
Keywords: polycyclic aromatic hydrocarbons, marine organisms, source identification, health risk assessment, temperate coastal area
Citation: Ji S, Yin F, Zhang W, Song Z, Qin B, Su P, Zhang J and Kitazawa D (2022) Occurrences, Sources, and Human Health Risk Assessments of Polycyclic Aromatic Hydrocarbons in Marine Organisms From Temperate Coastal Area. Front. Ecol. Evol. 10:850247. doi: 10.3389/fevo.2022.850247
Received: 07 January 2022; Accepted: 18 February 2022;
Published: 15 March 2022.
Edited by:
Chuanbo Guo, Institute of Hydrobiology (CAS), ChinaReviewed by:
Shuwen Yan, Fudan University, ChinaYuling Han, Guangdong University of Technology, China
Copyright © 2022 Ji, Yin, Zhang, Song, Qin, Su, Zhang and Kitazawa. This is an open-access article distributed under the terms of the Creative Commons Attribution License (CC BY). The use, distribution or reproduction in other forums is permitted, provided the original author(s) and the copyright owner(s) are credited and that the original publication in this journal is cited, in accordance with accepted academic practice. No use, distribution or reproduction is permitted which does not comply with these terms.
*Correspondence: Junbo Zhang, zhangjunbo1985@gmail.com
†These authors have contributed equally to this work and share first authorship