Host Plant Volatile Lures Attract Apanteles polychrosidis (Hymenoptera: Braconidae) to Ash Trees Infested With Caloptilia fraxinella (Lepidoptera: Gracillariidae)
- 1City of Edmonton Pest Management Lab, Edmonton, AB, Canada
- 2Department of Biological Sciences, University of Alberta, Edmonton, AB, Canada
Caloptilia fraxinella Ely (Lepidoptera: Gracillariidae), the ash leaf-cone roller, is an aesthetic pest of horticultural ash trees (Oleaceae, Genus Fraxinus) in prairie communities across Canada. Because pesticide use is undesirable in urban centers, biological control of C. fraxinella is a preferred approach. The native parasitoid wasp, Apanteles polychrosidis Vierek (Hymenoptera: Braconidae), has shifted hosts and is the primary parasitoid of C. fraxinella in Edmonton, Alberta, Canada, and has potential as a biological control agent. Here, in an effort to increase parasitism of C. fraxinella, lures releasing methyl salicylate (MeSA) and two green leaf volatiles (GLVs), [(Z)-3-hexenol, and (Z)-3-hexenyl-acetate], at both low and high release rates, were tested to enhance attraction and retention of A. polychrosidis in infested ash trees. Attraction of A. polychrosidis to baited trees was measured by capture on yellow sticky cards positioned in the tree canopy, and wasp activity was assessed by the parasitism rate of C. fraxinella. More male and female A. polychrosidis were captured on yellow sticky traps positioned in trees baited with the low dose of both MeSA + GLVs than to unbaited, infested ash trees. The increased attraction of wasps did not correlate with an increase in parasitism of C. fraxinella. The high release rate lures did not enhance attraction of A. polychrosidis to infested ash trees. Parasitism rate, however, was negatively correlated with host density in both field experiments. There was no evidence of close-range attraction to lures in an olfactometer assay. Synthetic HIPVs attract A. polychrosidis to ash trees infested with C. fraxinella, but the effect of wasp attraction on parasitism rate requires further research if HIPVs are to be used to enhance biological control in this system.
Introduction
Plants produce a variety of volatile organic chemicals (VOCs). Many of these compounds are by-products of plant metabolism and play a number of roles in plant fitness, including above and below ground herbivore defense, plant-plant communication, and attraction of herbivores and their natural enemies (Dudareva et al., 2006). Parasitoids, in particular, use herbivore-induced plant volatiles (HIPV) released by host plants attacked by herbivores as long-range signals for insect host-finding (Turlings et al., 1991). The ability of parasitoids to perceive differences in HIPVs seems linked to the level of host specialization, with those having a narrow host range better able to detect variation than generalist parasitoids (De Moraes et al., 1998; Röse et al., 1998; Ngumbi et al., 2009). While chemicals produced by the insect host, such as frass or cuticular compounds are reliable signals due to their specificity, they are often less detectable to foraging parasitoids at long range (Vet et al., 1991). Since the discovery of the important role of HIPVs in the foraging behavior of parasitoids of herbivorous insects (Turlings et al., 1991), research efforts have examined the response of potential biological control agents to synthetic HIPVs in lab and then field experiments (Rodriguez-Saona et al., 2012; Turlings and Erb, 2018). Relatively few studies have determined if enhanced attraction of parasitoids to HIPVs subsequently leads to higher parasitism rates and better biological control (Kaplan, 2012; Rodriguez-Saona et al., 2012).
Methyl salicylate (MeSA) is a HIPV that is released by the plant when fed upon by insects. Methyl salicylate plays many roles in both direct and indirect plant defenses (Garrido et al., 2009; Tang et al., 2015). As an indirect defense, MeSA attracts a broad range of predatory and parasitic insects of herbivores to infested plants (Rodriguez-Saona et al., 2011). Synthetic MeSA lures attract braconid wasps in maize, Zea mays (Poaceae) (Simpson et al., 2010) and grapes (James and Price, 2004). The aphid parasitoid, Aphidius ervi (Hymenoptera: Braconidae), is attracted to MeSA, and response increases with dose (Sasso et al., 2009). Varieties of maize that release more MeSA enhance parasitism of Spodoptera frugiperda (Smith) (Lepidoptera: Noctuidae) by the generalist parasitoid Cotesia marginiventris (Cresson) (Hymenoptera: Braconidae) (Degen et al., 2012).
Green leaf volatiles (GLVs) are ubiquitous, constitutive, short-chain (C6) alcohol, acetate and aldehyde components released by plants. They are released continuously in small amounts and are emitted in much higher amounts immediately after leaf damage (Turlings and Erb, 2018). Green leaf volatiles are implicated in the regulation of plant defense genes, and defense priming in a variety of plants (Engelberth et al., 2004; Farag et al., 2004), and also the attraction of beneficial insects (Scala et al., 2013). Cotesia marginiventris orients to varieties of maize that release high amounts of the GLVs (Hoballah et al., 2002). In addition, C. marginiventris prefers artificially damaged cotton plants, Gossypium hirsutum (Malvaceae), that release more GLVs than undamaged plants (Röse et al., 1998). This species is also attracted, in a dose dependent manner, to a blend of volatiles comprised of over 70% GLVs (Turlings et al., 1991). A specialist braconid parasitoid, C. glomerata (L.) is attracted to Arabidopsis sprayed with the synthetic GLVs (Z)-3-hexenal, (Z)-3-hexenol, and (Z)-3-hexenyl acetate (Shiojiri et al., 2006).
There is evidence that a blend of volatile chemicals released from herbivore-infested plants is important for parasitoid attraction. Tamiru et al. (2015) found that, although Cotesia sesamiae (Cameron), detect 13 HIPVs, only three of those compounds are behaviorally active alone. Cotesia marginiventris is attracted to both the natural and synthetic blend of plant volatiles released from plants fed on by beet armyworm, Spodoptera exigua (Hübner) (Lepidoptera: Noctuidae), and the response is dose-dependent (Turlings et al., 1991). In the field, HIPV dispensers emitting a synthetic blend of volatiles which mimics the compounds emitted from herbivore-damaged cabbage, Brassica oleracea (Brassicales: Brassicaceae), attract Cotesia vestalis (Haliday) (Hymenoptera: Braconidae) (Uefune et al., 2011). A spray application of a mixture of several HIPVs to different crops attracts a variety of parasitic Hymenoptera (Simpson et al., 2010).
Here, we tested MeSA and two GLVs as lures to attract a native parasitoid Apanteles polychrosidis Vierek (Hymenoptera: Braconidae), to increase parasitism of an invasive pest of ash trees, the ash leaf-cone roller, Caloptilia fraxinellla Ely (Lepidoptera: Gracillariidae) in Edmonton, Alberta, Canada. The ash leaf-cone roller was first recorded in Edmonton in 1999 (Pohl et al., 2004). The ash leaf-cone roller overwinters as an adult in reproductive diapause (Evenden et al., 2007). In the spring, moths emerge from overwintering sites, females produce a sex pheromone signal (Evenden and Gries, 2008), and mating occurs prior to orientation to newly flushed ash (Fraxinus) trees for oviposition (Evenden, 2009). Moths are constrained to oviposit on newly flushed ash leaflets as hatched larvae must penetrate the leaf tissue for leafmining. Young larvae are leafminers, but larvae emerge from leaflets at the fourth instar and disperse on silken threads to new leaflets which are rolled by mature larvae into aesthetically displeasing cones (Pohl et al., 2004; Evenden, 2009). Fourth instar larvae are nuisance pests when they hang from silk threads to disperse. Pupation occurs within the leafrolls and adults emerge in mid-July and aestivate prior to dispersing to overwintering sites in the fall (Evenden, 2009).
The primary parasitoid of C. fraxinella in Edmonton is A. polychrosidis (Wist and Evenden, 2013). This solitary, konobiont parasitoid has a broad Nearctic range (Fernández-Triana and Huber, 2010) and is native to Edmonton. Previous host records of A. polychrosidis include larvae from the moth family Tortricidae, including Choristoneura rosaceana (Harris), Choristoneura fumiferana Walsingham, Pandemis limitata Robinson, Pandemis pyrusana Kearfott, Platynota idaeusalis (Walker), and Endopiza viteana Clemens (all Lepidoptera:Tortricidae) (Seaman et al., 1990; Biddinger et al., 1994; Li et al., 1999; Cossentine et al., 2004; Fernández-Triana and Huber, 2010; Pfannenstiel et al., 2012). In Edmonton, A. polychrosidis shifted hosts to exploit the introduced pest, C. fraxinella, as its larval host during the summer. Field host-association studies suggest that A. polychrosidis target dispersing fourth-instar C. fraxinella larvae for oviposition (Wist and Evenden, 2013). There are two generations per year of A. polychrosisdis in Edmonton, a generation in which larvae overwinter in native caterpillar hosts and a summer generation in which the larvae use C. fraxinella as a host and emerge as adults in mid-late summer to mate and lay eggs in the native overwintering host (Wist and Evenden, 2013). Apanteles polychrosidis females can parasitize ∼60 Choristoneura rosaceana larvae under optimal temperature conditions (Cossentine et al., 2005), but it is not known how many C. fraxinella larvae are parasitized by a single female.
Apanteles polychrosidis detect and orient to VOCs released from ash trees. The headspace volatiles from intact and damaged green ash seedlings contain 13 VOCs that elicit a response from both male and female A. polychrosidis antennae (Wist et al., 2015). Those VOCs were identified as: (1) (Z)-3-hexenal, (2) (Z)-3-hexenol, (3) (Z)-3-hexenyl-acetate, (4) ocimene, (5) linalool, (6) (E)-4,8-dimethyl-1,3,7-non-atriene, (7) methyl salicylate, (8) bourbonene, (9) (E)-β-farnesene, (10) α-farnesene, and (11) dendrolasin. Two other compounds detected by wasp antennae were not identified. Intact, mechanically damaged, and leaf-mined green ash leaflets produce different VOC profiles (Wist et al., 2015). Release of GLVs [(Z)-3-hexenal, (Z)-3-hexenol, (Z)-3-hexenyl acetate], decreases after leaf-mining, but increases after mechanical damage of leaflets (Wist et al., 2015). Methyl salicylate release is greater in both mined and mechanically damaged green ash leaflets than from intact leaflets (Wist et al., 2015). Black ash leaves mined by C. fraxinella larvae attract more A. polychrosidis than unmined leaflets, indicating that the wasp is attracted to HIPVs (Wist et al., 2015). The GLVs (Z)-3-hexenol, and (Z)-3-hexenyl acetate, and the HIPV, MeSA, may have potential to attract A. polychrosidis, as they are attractive to other braconid parasitoids, and other parasitic Hymenoptera (James and Grasswitz, 2005). We evaluated MeSA and two GLVs known to be detected by A. polychrosidis, as lures to attract wasps and increase parasitism of C. fraxinella in infested ash trees. We considered lure release rate and combinations of HIPVs as long and short-range attractants and measured levels of parasitism of C. fraxinella in treated trees. Attraction of other non-target parasitic Hymenoptera in treated trees was also recorded. We also tested the short-range response of A. polychrosidis to these same compounds in an olfactometer study.
Materials and Methods
We tested synthetic lures releasing MeSA and two GLVs [(Z)-3-hexenol, and (Z)-3-hexenyl acetate] both alone and in combination on the attraction of, and parasitism by A. polychrosidis in horticultural green ash trees (Fraxinella pennsylvanica) infested with C. fraxinella. Field experiments were conducted with both low and high release rate lures (Table 1).
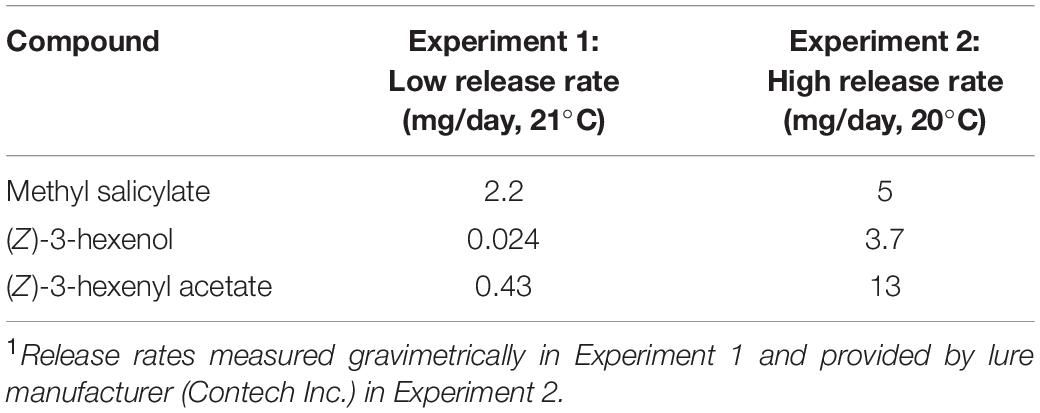
Table 1. Release rates1 of synthetic HIPV lures placed in Caloptilia fraxinella infested green ash trees for Experiments 1 (2016) and 2 (2017).
Experiment 1: Low Release Rate HIPV Lures Field Study (2016)
In the summer of 2016, ten horticultural green ash sites were selected in Edmonton, Alberta, Canada (53.5444° N, 113.4909° W) at least 600 m apart, on boulevards or in parks in areas known to have C. fraxinella infestation. Trees were approximately 10–15 years old with a canopy that could be sampled from the ground using 1.8 and 2.4 m long ladders. At each site, one tree was randomly assigned one of the following treatments: (1) one 250 μl Eppendorf lure containing 200 μl of MeSA (99% purity, Fisher Scientific, Ottawa, ON, Canada); (2) one 250 μl Eppendorf lure of each of the GLVs [(Z)-3-hexenol and (Z)-3-hexenyl acetate] containing 200 mg of each individual compound (Contech Enterprises Inc., Delta, BC, Canada); (3) both MeSA and GLV lures together, or (4) a blank control without lures. Experimental trees at each site were separated by 14–35 m, depending on the tree planting regime at each site. Each individual lure, or set of lures for combined treatments, was secured with wire on a random side of the tree trunk in the low to mid-canopy. To assess the attraction of A. polychrosidis to each of the treated trees, three yellow sticky cards (18 cm x 9cm, 2cm x 2cm grid) (Alphascents Insect Monitoring Systems, West Linn, OR) were equidistantly positioned in the low-mid canopy in each tree at each site. Previous work has shown that the number of A. polychrosidis wasps captured on yellow sticky cards does not vary with position within the tree canopy (Wist and Evenden, 2013). Sticky cards were positioned in trees after bud flush when it is expected that C. fraxinella oviposition will have already occurred (Evenden, 2009). Sticky cards were changed weekly from 10 May through 21 July, 2016. This trapping period encompasses capture of the generation of A. polychrosidis, that parasitize C. fraxinella, and the generation that emerge from parasitized C. fraxinella in July (Evenden, 2009). After collection, the traps were wrapped in plastic and frozen until they could be processed. Wasps were identified as A. polychrosidis based on wing characters and femur colour (Fernández-Triana and Huber, 2010), under a dissecting scope at 40X magnification. Apanteles polychrosidis were separated by sex based on the presence of an ovipositor in females. Trap catch per tree was based on the average trap catch from all traps in each tree. In the 2016 experiment, some of the other parasitic Hymenoptera captured on traps were also identified and counted. These included Sympiesis sericeicornis and other Sympiesis (Hymenoptera: Eulophidae) wasps that are known to parasitize C. fraxinella and be hyperparasitoids of A. polychrosidis (Wist and Evenden, 2013). Wasps in the genus Diadegma (Hymenoptera: Ichneumonidae) that have also been recorded to parasitize C. fraxinella (Wist and Evenden, 2013), were also identified and counted.
The percent parasitism of C. fraxinella by A. polychrosidis on each experimental tree at each site was assessed after wasp emergence, in August 2016. One hundred leaf rolls, or all the leaf rolls on trees with fewer than 100, were haphazardly collected throughout the tree canopy. The C. fraxinella and A. polychrosidis pupal cases were identified (after Wist et al., 2015) per each 100-roll sample to obtain the percent parasitism as the number of A. polychrosidis/total pupal cases sampled. The overall density of C. fraxinella infestation was visually estimated in August by scanning one side of the canopy for ∼10 s to obtain the percentage of ash leaflets rolled per treated tree, as per City of Edmonton monitoring protocols (BC Ministry of Forests, 1998).
Experiment 2: High Release Rate HIPV Lures Field Study (2017)
In 2017, a similar experiment was conducted at the same field sites as in 2016. Attraction of the generation of A. polychrosidis that parasitizes C. fraxinella was targeted from 3 May to 21 June, 2017. The HIPV lures were bubble packs (Contech Enterprises Inc., Delta BC, Canada) which released compounds at a higher release rate than lures used in the 2016 experiment (Table 1). Apanteles polychrosidis wasps were captured and identified as in Experiment 1, but they were not separated by sex. Measures of C. fraxinella density and parasitism rate of C. fraxinella by A. polychrosidis were obtained as in 2016. The non-target parasitic Hymenoptera were not identified or counted in 2017.
Olfactometer Bioassay
An olfactometer bioassay was conducted under laboratory conditions to test close range attraction of A. polychrosidis to the same semiochemicals tested in the field studies.
Insects
Apanteles polychrosidis were collected between 13 and 28 June, 2016 from sites across Edmonton. Leaf rolls without emergence “windows” were selected following the protocol of Wist et al. (2015) to identify rolls that contained parasitoids. Leaf rolls were placed individually in 36 ml plastic cups positioned on trays. Ten-15 trays were stacked in black plastic bags with moistened paper towels to maintain humidity and maintained at room temperature in the laboratory (∼22°C). Leaf rolls were examined daily for wasp emergence and newly emerged males and females were paired within a single cup. Each pair of wasps was provided with a sucrose solution (25%, v/v) through a dental wick. Paired wasps were maintained at room temperature (∼22°C) positioned next to the window to provide a natural ambient light cycle. Female wasps were assumed to be mated 24 h after pairing, as they mate shortly after eclosion (Wist and Evenden, 2013). Wasps were maintained as pairs until females were used in trials at 9–11 days old, as A. polychrosidis is capable of parasitism up to 20 days post eclosion (Cossentine et al., 2005).
Bioassay Methods
Methyl salicylate (Sigma-Aldrich, 99% purity) was diluted to 1 μg/μl in HPLC-grade hexane. Each of the two GLVs, were extracted from unused Eppendorf tube lures (described in field experiments above) and diluted to 1 μg/μl in HPLC-grade hexane. Five μl of each solution was applied with a micropipette to 1 × 1 cm filter paper (Whatman no. 1) on a straight pin, and allowed to evaporate for ∼10 s in a fume hood. The treatments were 5 μg MeSA, 5μg of each GLV (separate filter paper squares on 2 pins), 5 μg MeSA + 5 μg each GLV (separate filter paper squares on 3 pins), or 5 μl of the solvent, hexane, as the clean air control. After solvent evaporation, filter papers were positioned in separate arms of a six-arm olfactometer and a female wasp was introduced to the bioassay within 1 min.
The six-arm olfactometer (Analytical Research Systems Inc., Gainsville, FL.) had two airflow passages blocked to provide access to four odor sources. A circular hole in the center of the arena served as both the entrance point for the insect, as well as the attachment point for the air outflow. Airflow into the arena was 1 L/min from each separate arm containing each of the four odor sources, and outflow at the center of the arena was 3L/min, as regulated with a vacuum pump airflow system that filters and purifies air entering the bioassay (Model OLFM-6C-ADS + VAC, Analytical Research Systems Inc., Gainsville, FL.). Six 100-watt incandescent light bulbs diffused through white paper provided bright (600–650 lux), even light throughout the bioassay arena. Females were individually tested in 15 min trials conducted between 9 am and 4 pm. Odor sources were replaced for each wasp tested. A Logitech web camera was adhered to a ring clamp and positioned on a clamp stand at 50 cm above the platform, with the lens aiming downward at 45°, to record the wasp movement in each assay. The stand with the camera was placed to have the whole olfactometer arena in the frame, but with the camera out of view of the arena. Following each day of trials, the olfactometer was cleaned with 70% ethanol, glassware was rinsed three times with acetone, and three times with hexane, and dried in the oven at 60°C overnight. Treatment positions were randomly rearranged for trials conducted on each of 3 days. Each wasp was only used once (n = 21).
Statistical Analyses
Field Studies
All statistical analyses were conducted in R version 3.4.2 (R Core Team, 2017 Vienna, Austria). Data were evaluated visually for normality and heteroscedasticity with Q-Q plots, and residual variance plots. In Experiment 1 conducted in 2016, the average number of A. polychrosidis captured on all yellow sticky traps positioned in trees baited with different low release HIPV lures over the wasp generation that attacks C. fraxinella (May 10 to June 8, 2016) was square-root transformed to approach normality. Average A. polychrosidis count captured on all yellow sticky cards among trees was the dependent variable in a linear mixed-effects model. Fixed explanatory variables were semiochemical treatment and the sex of A. polychrosidis. Site was included as a random variable. Non-significant interaction terms were removed from the original model: lmer[sqrt(avg#wasps) ∼ HIPV treatment ∗ sex + (1 | site)]. Analyses were conducted using lme4 package version 1.1-11 (Bates et al., 2015). Post hoc multiple comparison Tukey tests were conducted with multcomp, version 1.4-8 (Hothorn et al., 2008), to determine differences between significant factors.
A second linear mixed model compared average A. polychrosidis counts in trees treated with the high release rate HIPV lures, in Experiment 2 conducted in 2017. The average wasp count was square-root transformed. The fixed explanatory variable was semiochemical treatment, and site was included as a random variable. Analysis was conducted using lme4 package version 1.1-11 (Bates et al., 2015).
The parasitism rates of C. fraxinella by A. polychrosidis in ash trees treated with the different low (2016) and high (2017) HIPV lure release rates, were compared with separate generalized linear mixed-effects models with a binomial error distribution in lme4 package version 1.1-11 (Bates et al., 2015). A vector (y), was constructed, binding the number of parasitized larvae, and the number of unparasitized larvae into a single object, to act as the response variable (Crawley, 2012), with HIPV lure treatment as the explanatory variable, host density as a covariate, and site specified as a random factor. The model including interaction between HIPV treatment and host density had a higher AIC than without the interaction for Experiment 1, and the interaction was removed from the model. Without the interaction term, HIPV treatment was marginally significant, the host density significantly affected parasitism, and multiple comparisons Tukey test was conducted using lsmeans package (version 2.27-2, Lenth 2016). For Experiment 2, the interaction between explanatory variables was significant, and multiple comparisons Tukey test was conducted using lsmeans package (version 2.27-2, Lenth 2016). Linear models for both Experiments 1 and 2 were used to show the relationship between parasitism level and host density.
A Welch’s t-test compared the overall mean number of first-generation A. polychrosidis captured between years.
Olfactometer Bioassay
A grid dividing the arena into 6 triangular segments was superimposed to each recording with Adobe Photoshop (Adobe Creative Cloud) and the time spent in each sector was recorded. Female A. polychrosidis that did not move from their initial position were counted as non-responders (n = 3). The proportion of time each female spent in the sector of each treatment was calculated and standardized over the observation period of 900 s (15 min). The number of seconds spent by each female wasp in the segment closest to each HIPV treatment was cube-root transformed, and compared in a linear mixed model using lme4 package version 1.1-11 (Bates et al., 2015).
Results
Experiment 1: Low Release Rate HIPV Lures Field Study (2016)
Semiochemical treatment affected capture of A. polychrosidis on yellow sticky cards positioned in ash trees baited with the various low release rate lures in 2016 (HIPV treatment: χ2 = 14.80, df = 3, p = 0.002). Trap catch also varied by wasp sex (sex: χ2 = 34.84, df = 1, p < 0.0001) (Figure 1), as more females were captured than males. Post hoc analysis showed more wasps were captured in trees baited with low release rate MeSA + GLVs lures, compared to control trees without lures (Figure 1). The number of A. polychrosidis attracted to trees baited with MeSA or GLV lures alone was intermediate. Sticky traps positioned in the variously treated trees in 2016 also captured other parasitic Hymenoptera including Sympiesis sericeicornis (80), other Sympiesis spp. (309), and Diadegma spp. (91) (Table 2).
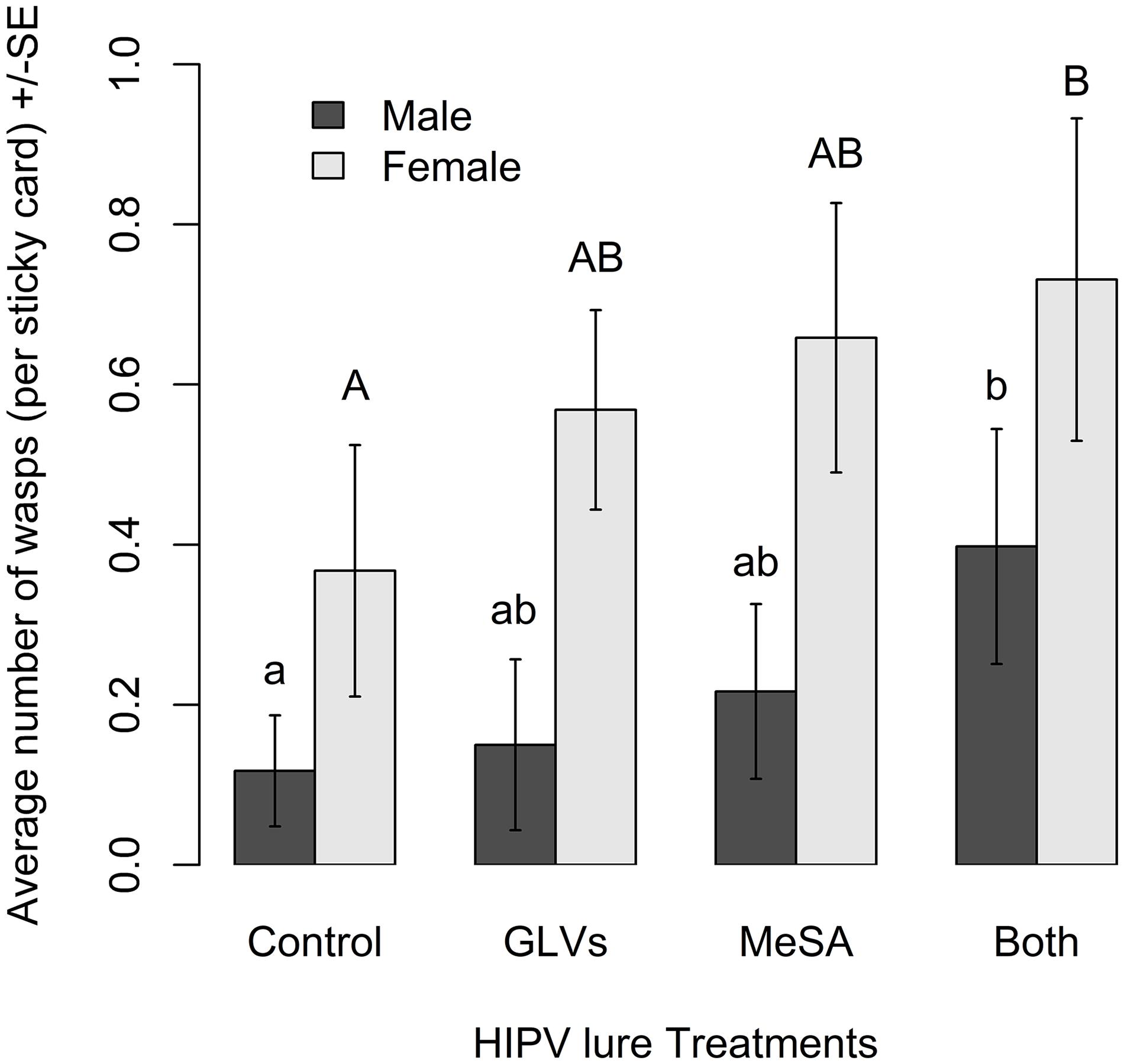
Figure 1. Mean number of Apanteles polychrosidis captured in trees baited with low release rate HIPV lures in Experiment 1 (2016). One green ash tree at each site (n = 10) was treated with a single low release rate lure releasing (1) methyl salicylate (MeSA) (2.2 mg/day 21°C): (2) green leaf volatiles (GLVs) (Z)-3-hexenol (0.024 mg/day, 21°C) and (Z)-3-hexenyl acetate (0.43 mg/day, 21°C); (3) both MeSA and GLVs (MeSA and GLVs); or (4) no lures (Control). The mean number of wasps captured on sticky traps in experimental trees were compared with a linear mixed model. Significantly more female than male wasps were captured. Significant differences are indicated by different lower-case letters for male wasps and upper-case letters for females (Tukey pairwise comparisons, p < 0.05).
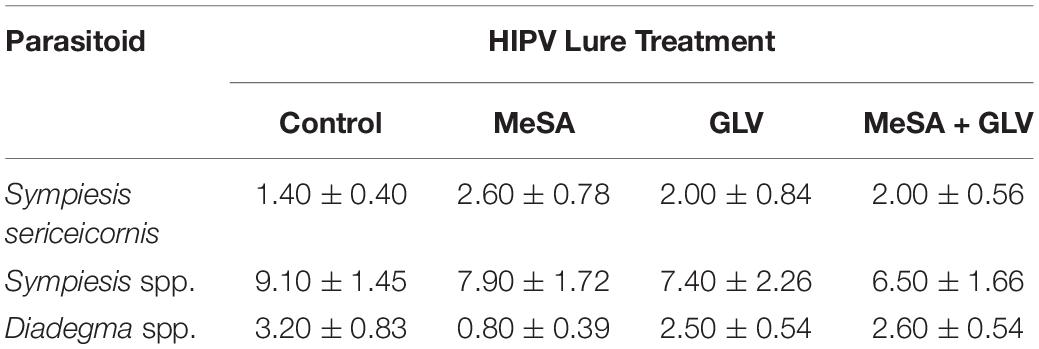
Table 2. Mean (+SE) capture of non-target parasitoids on yellow sticky cards positioned in Caloptilia fraxinella infested green ash trees baited with synthetic HIPV lures in Experiment 1 (2016).
Although more A. polychrosidis were attracted to trees baited with the MeSA + GLV combined lures compared to unbaited trees in 2016, the parasitism rate of C. fraxinella by A. polychrosidis was only marginally affected by HIPV treatment. Parasitism in trees treated with GLV lures (30.9 ± 7.78%) was marginally higher than that in trees treated with MeSA (21.83 ± 6.12%) lures (p = 0.059). Trees treated with the low release rate MeSA had the lowest parasitism rate of the experimental trees. Parasitism by A. polychrosidis was negatively correlated with C. fraxinella density (y = 33.027−0.49x, p = 0.0028, adjusted R2 = 0.20) in 2016 (Figure 2).
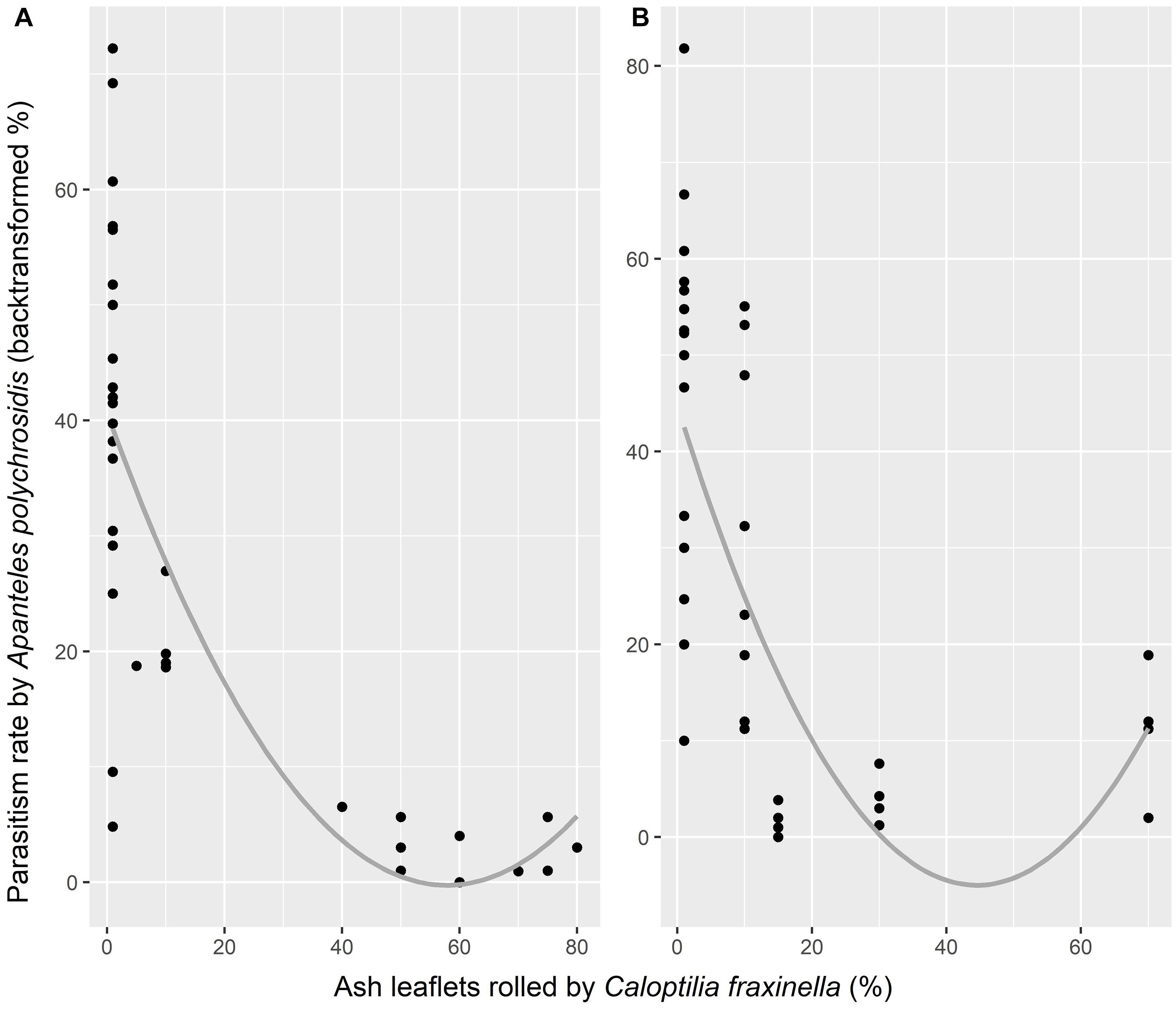
Figure 2. Parasitism by Apanteles polychrosidis, as affected by density of Caloptilia fraxinella in green ash trees in Experiment 1 (A) and Experiment 2 (B). Parasitism rate is the backtransformed value of the weighted vector, y = (parasitized, not parasitized). Points represent parasitism in all experimental trees for each experiment with a smoothed mean line. (A) p = 0.0028, adjusted R2 = 0.20 (B) p = 0.0001, adjusted R2 = 0.29.
Experiment 2: High Release Rate HIPV Lures Field Study (2017)
There were no significant differences among treatments in the number of A. polychrosidis captured on yellow sticky cards positioned in ash trees treated with the high release rate lures in 2017 (χ2 = 1.82, df = 3, p = 0.61) (Figure 3). Wasp capture was highly variable in trees treated with the high release rate lures. There was a non-significant trend of less wasp capture on traps positioned in unbaited control trees than in treated trees.
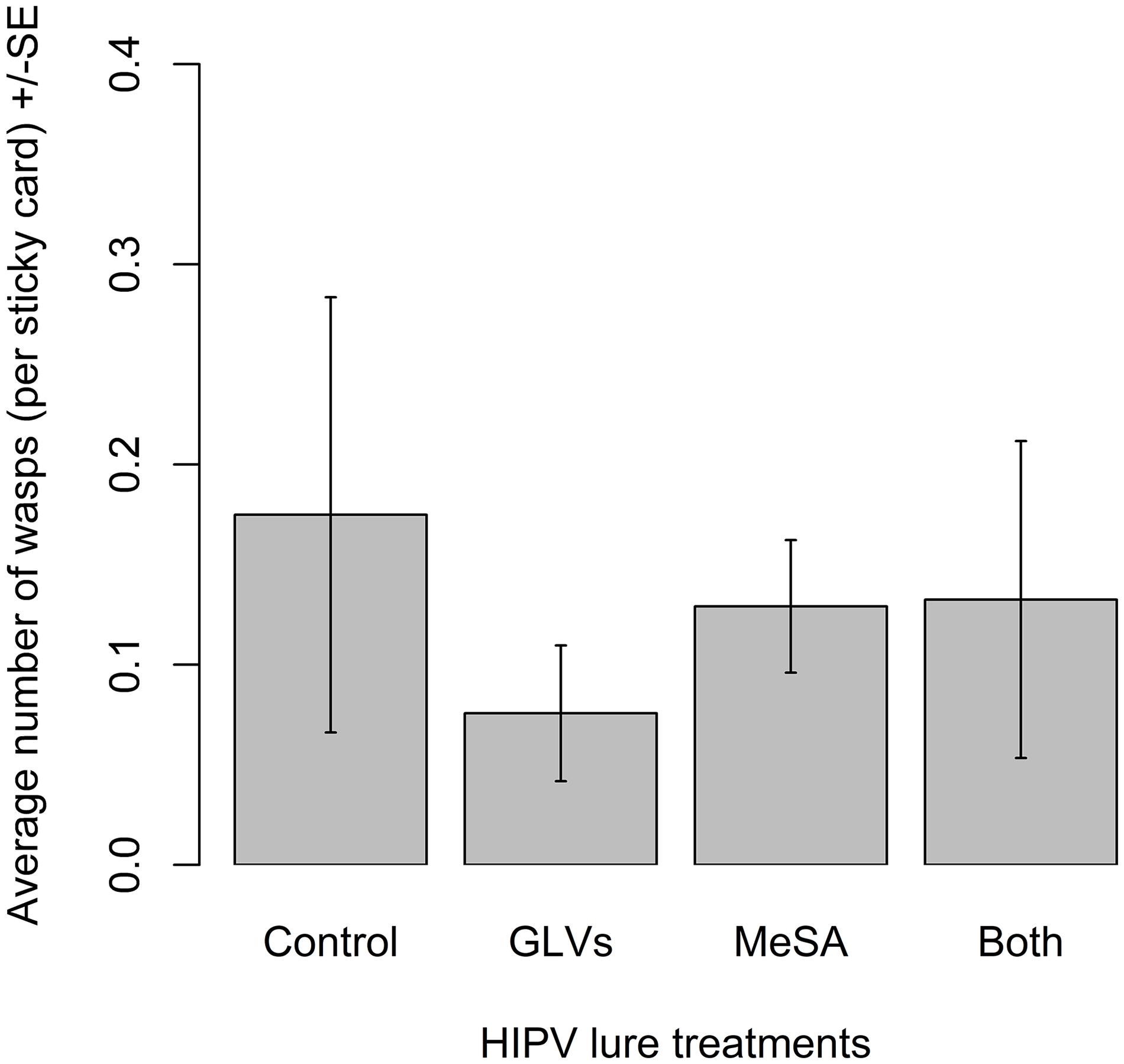
Figure 3. Mean number of Apanteles polychrosidis captured in trees baited with high release rate HIPV lures in Experiment 2 (2017). One green ash tree at each site (n = 10) was treated with a single high release rate lure releasing (1) methyl salicylate (MeSA) (5 mg/day 20°C): (2) green leaf volatiles (GLVs) (Z)-3-hexenol (3.7 mg/day, 20°C) and (Z)-3-hexenyl acetate (13 mg/day, 20°C); (3) both MeSA and GLVs (MeSA and GLVs); or (4) no lures (Control). The mean number of wasps captured on sticky traps in experimental trees were compared with a linear mixed model, but a statisitcally equal number of wasps were captured in the variously treated trees.
There was a significant interaction between high release rate HIPV treatment in 2017 and host density (χ2 = 11.607, df = 3, p = 0.0089) that affected the parasitism rate of C. fraxinella by A. polychrosidis (Figure 4). Host density could not be directly impacted by HIPV treatment because lures were placed in trees following host orientation by C. fraxinella. Trees treated with the high release rate GLV lures alone had the lowest parasitism rates at higher host density. Parasitism rate was least affected by host density in trees with high release rate MeSA lures alone, and MeSA decreased the negative effect of high host density on parasitism rate in the trees with both lure types. As in Experiment 1, the overall linear model showed parasitism rate to be negatively correlated with host density (y = 36.7–0.54x, p < 0.0001, adjusted R2 = 0.29) in Experiment 2 (Figure 2).
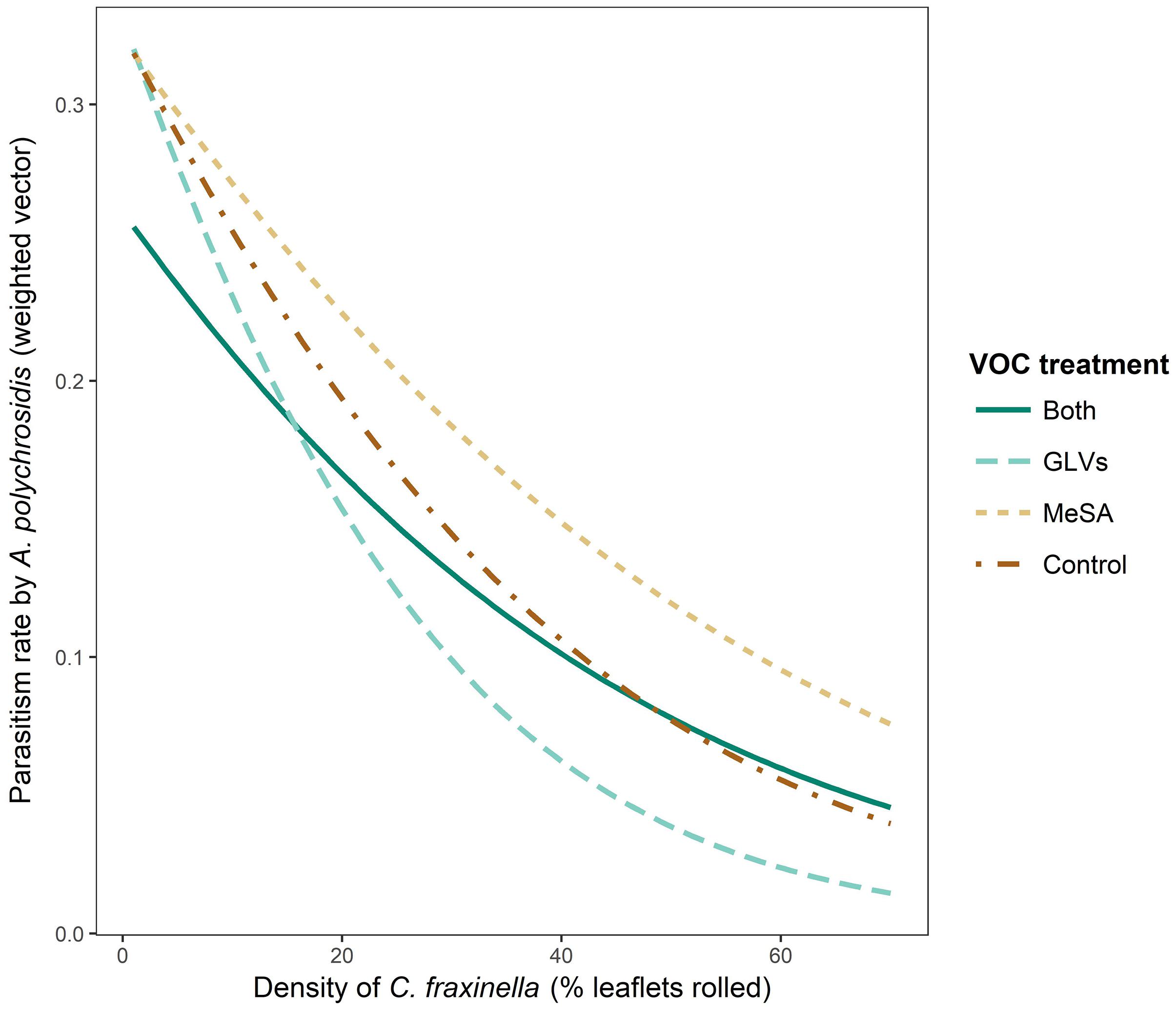
Figure 4. Interaction plot of parasitism rate by Apanteles polychrosidis, as affected by Caloptilia fraxinella density and high dose HIPV lure treatment in green ash trees in Experiment 2. Rate of parasitism is the value of the weighted vector, y = (parasitized, not parasitized). Green ash trees were treated in 2017 with HIPV high release rate lures of either methyl salicylate (MeSA) (5 mg/day, 20°C), green leaf volatiles (GLVs) (Z)-3-hexenol (3.7 mg/day, 20°C) and (Z)-3-hexenyl acetate (13 mg/day, 20°C), both MeSA and green leaf volatiles (Both), or no lures (Control).
The number of A. polychrosidis wasps captured while orienting to infested ash trees in 2016 was significantly higher than that in 2017 (t = -9.14, df = 196.83, p < 0.0001).
Olfactometer Bioassay
Wasps in the olfactometer exhibited detection and orientation behaviors (Klomp, 1981; Colazza et al., 1999) to the tested odors including arrestment, antennal drumming and frequent turning. There were, however, no significant differences in the time spent by mated female wasps in the various sections of the olfactometer supplied with the different odor treatments or blank controls in the 15 min assay. There was a trend toward less time spent in the GLV-treated segment, but it was not significant (χ2 = 3.24, df = 3, p = 0.36) (Figure 5).
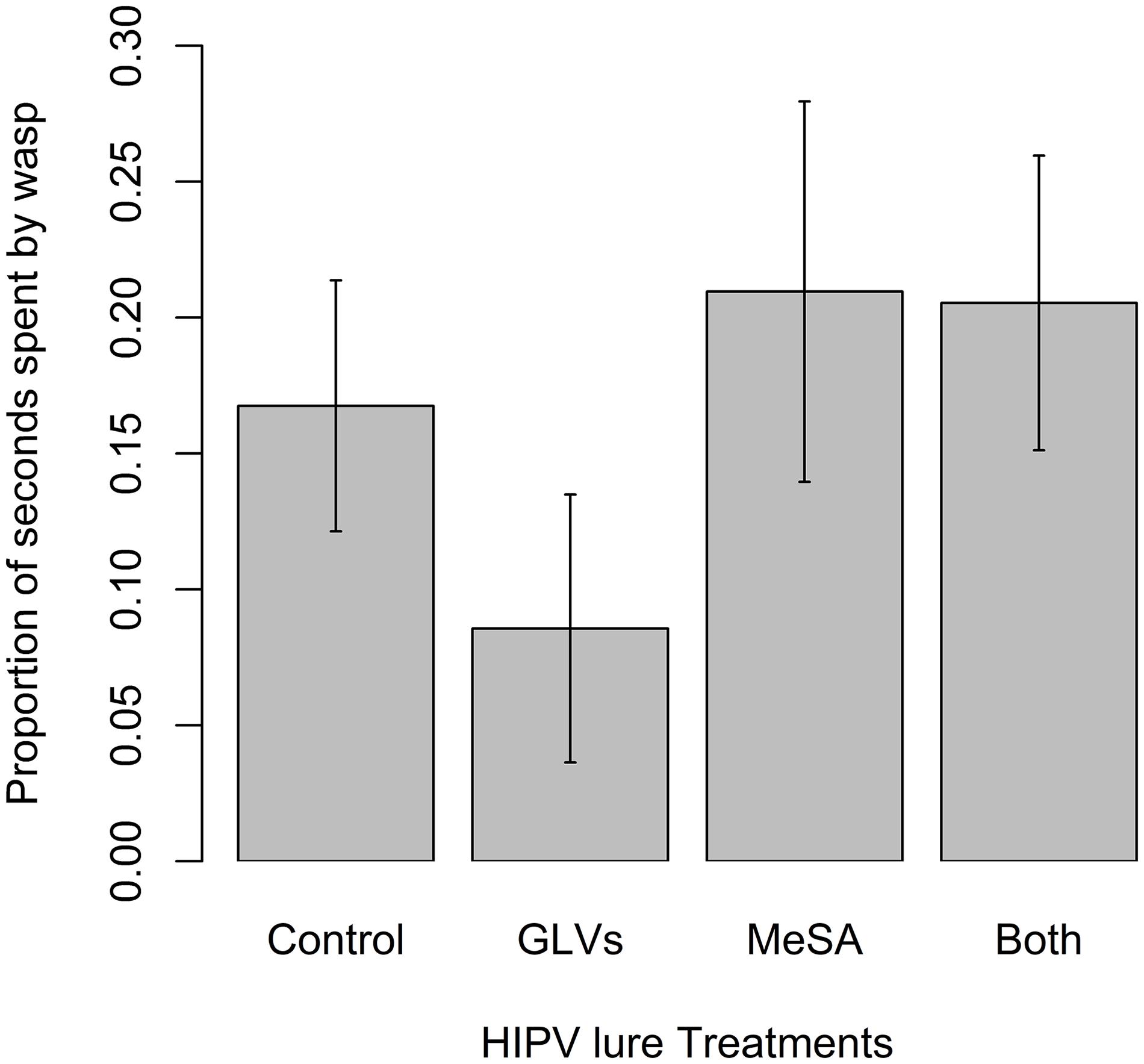
Figure 5. Mean (± SE) number of seconds spent by mated female Apanteles polychrosidis (n = 20) in proximity to various HIPV treatments presented in an olfactometer. A linear mixed model compared the mean time spent closest to each HIPV treatment. Herbivore-induced plant volatile treatments were: (1) 5 μg methyl salicylate (MeSA); (2) 5 μg of each GLV [(Z)-3-hexenol and (Z)-3-hexenyl acetate]; (3) 5 μg MeSA + 5 μg of each GLV; or (4) 5 μl of HPLC grade hexane (Control). Females were in the trial for 900 s. There were no significant differences in time spent by female wasps in proximity to the treatments.
Discussion
We illustrated that both male and female A. polychrosidis are attracted to trees baited with the low release rate combined MeSA and GLV lures, but not the high release rate lures. Release rate of HIPVs from individual lures or per treated area is known to affect natural enemy recruitment (Kaplan, 2012). Many studies illustrate that natural enemy recruitment increases with release rate of the attractant, but some studies on parasitoid response to HIPVs indicate that optimal attraction occurs at intermediate release rates (Whitman and Eller, 1992). The difference in results obtained by the low and high release rate lures in our study, might also be attributable to other factors, like climate, as the experiments were conducted in separate years. The mechanism by which A. polychrosidis are attracted to HIPV-treated trees cannot fully be determined from this study, as yellow sticky cards were positioned within the tree canopy and not directly adjacent to the lure. Lures can directly attract natural enemies or act as indirect attractants that trigger plants to alter their volatile profile (Kaplan, 2012; Rodriguez-Saona et al., 2012). Both MeSA (Ozawa et al., 2000) and GLVs (Farag et al., 2004) can induce HIPV production in plants. Gaseous application of MeSA to lima bean plants induces VOC production similar to HIPVs released by feeding spider mites, Tetranychus urticae Koch (Trombidiformes: Tetraychidae) (Ozawa et al., 2000). Exogenous application of (Z)-3-hexenol on corn plants upregulates production of (Z)-3-hexenyl acetate and MeSA (Farag et al., 2004). Tomato plants exposed to (Z)-3-hexanol produce and release the HIPVs 2-carene, and β-phellandrene (Farag and Paré, 2002). The synthetic lures used in these experiments cannot, however, act through the mechanism of herbivore attraction and increased prey density (Kaplan, 2012) in the treated trees, as oviposition by C. fraxinella females occurs at bud flush (Evenden, 2009), prior to lure placement in trees.
Our results indicate that HIPV attraction of A. polychrosidis is best to a combination of low release rate lures releasing MeSA and GLVs. More wasps were captured in trees treated with the combined lures than in the unbaited control trees, and yellow sticky cards positioned in trees treated with the MeSA or GLV lures alone caught an intermediate number of wasps. Yu et al. (2008) tested various HIPVs alone and together for attraction of different cotton pest natural enemies, and found differential response to compounds depending on the species of natural enemy. A combined lure releasing both nonanol and (Z)-3-hexenol attracted more Microplitis mediator (Halliday) (Hymenoptera: Braconidae) wasps than the same compounds alone (Yu et al., 2008). As both specialist and generalist parasitoids are known to use complex information provided by blends of HIPVs for foraging (Turlings and Erb, 2018), it is likely that a combination of HIPVs will be more effective than the constituent compounds for long range attraction of parasitoids (Kaplan, 2012). There was no visible close-range attraction to HIPVs by mated female A. polychrosidis in the olfactometer study, and it is possible that the HIPVs tested do not mediate short range attraction. Lures releasing HIPVs in the field are presented in the background of natural semiochemicals released by infested ash trees. A blend of plant compounds may be required to elicit oriented attraction in this species (D’Alessandro et al., 2009). Most efforts to manipulate natural enemy behavior with HIPVs to augment biological control have evaluated response to individual compounds separately (Rodriguez-Saona et al., 2012), but the results of the current study suggest that testing a combination of HIPVs warrants further attention. Herbivore-induced plant volatile blends that more specifically target attraction of A. polychrosidis may be worth further development in this system, as we detected hyperparasitoids in the genus Sympiesis in trees treated with the tested HIPV lures. An increased density of hyperparasitoids as a result of HIPV treatment could negatively impact parasitoid population density and subsequent biological control.
Few studies have illustrated the effect of natural enemy recruitment to a managed ecosystem on subsequent biological control (see Yu et al., 2010 for exception). Our experimental design allowed us to both monitor attraction to baited trees and to measure parasitism of C. fraxinella by A. polychrosidis (Wist et al., 2015). Enhanced attraction of male and female A. polychrosidis to trees treated with the combined low release rate HIPV lures did not correlate to higher parasitism in treated ash trees. Increased aggregation of parasitoids at a host patch does not always lead to more parasitism of insect hosts in the patch (Morrison and Strong, 1980). More encounters with conspecifics in a host patch can lead to more time spent making oviposition decisions (Visser et al., 1999). Time may also be spent avoiding encounters with conspecifics. Venturia canescens (Gravenhorst) (Hymenoptera: Ichneumonidae) avoid conspecific wasps with or without the presence of host kairomones (Castelo et al., 2003). Competition between conspecific females decreases longevity of Diaeretiella rapae (McIntosh) (Hymenoptera: Braconidae), a parasitoid of the cabbage pest Brevicoryne brassicae (Linaeus) (Hemiptera: Aphididae) (Kant and Minor, 2017). It is also possible that the attraction of more A. polychrosidis to trees treated with HIPVs results in superparasitism of larval hosts by more than one female (Godfray, 1994). Detection and selection of unparasitized hosts increases foraging time and may decrease the efficiency of the female parasitoid under levels of high competition (Visser et al., 1992).
Parasitism was marginally lower in trees treated with low release rate MeSA lures, compared to those with the GLV lures. Methyl salicylate is an important volatile signaling compound in plant-plant communication (Shulaev et al., 1997) and plant-produced MeSA can signal herbivore host quality to parasitoids. Koinobiont parasitoids rely on high quality host larvae for development (Quicke, 2015), and poor plant quality that negatively affects herbivore performance can also impact the third trophic level (Godfray, 1994). Parasitoid larval growth and adult weight of two ichneumonid koinobiont parasitoids of Plutella xylostella (Linnaeus) (Lepidoptera: Plutellidae) larvae were reduced when the host larvae were reared on herbivore-damaged cabbage (Bukovinszky et al., 2012). The amount of synthetic MeSA released from lures in this study may induce further release from infested ash trees and signal low quality C. fraxinella to A. polychrosidis. Methyl salicylate lures attract predatory insects such as lacewings (Order Neuroptera), ladybird beetles (Coleoptera: Coccinellidae), and syrphid flies (Diptera: Syrphidae) (James and Price, 2004; Mallinger et al., 2011). The quality of the prey item is less important to insect predators than parasitoids as they generally gain nutrition from more than one prey item. Although MeSA lures are attractive to other braconid wasps (James and Price, 2004), they also repel at least one species of ichneumonid parasitoid (Snoeren et al., 2010).
Parasitism by A. polychrosidis was negatively correlated with the density of C. fraxinella in both Experiments 1 and 2. These findings support previous parasitism studies in this system in which parasitism of C. fraxinella was negatively correlated with host density on green ash, but not black ash trees (Wist et al., 2015). Laboratory experiments suggest that the differential parasitism of C. fraxinella on the two ash hosts is mediated by HIPVs (Wist et al., 2015). It is possible that this negative correlation occurs because parasitoids are swamped by high host numbers (Lessells, 1985), that result in an overall decrease in parasitism rate with host density. High levels of HIPV released by plants attacked by many herbivores may potentially mask foraging cues (Schröder and Hilker, 2008), and inhibit close-range foraging behavior of parasitoids. The olfactometer study illustrated that A. polychrosidis detected the HIPVs but did not orient to odor sources at close range.
The high release rate HIPV lures interacted with host density to affect parasitism rate in Experiment 2. The high release rate GLV lures alone led to further decreased parasitism rates in trees with high C. fraxinella density, compared to other treated trees. In the olfactometer study, mated female wasps spent marginally less time close to the GLV sources compared to other treatments. Defense chemical pathways are induced in plants exposed to a number of GLVs. It may be that the higher doses of GLVs in Experiment 2 induced the production of multiple HIPVs by trees, which affect A. polychrosidis foraging and parasitism behavior, and this effect is amplified with HIPV release as a result of high herbivore attack on the tree. In contrast, high release rate MeSA lures modulated the negative relationship with host density on parasitism rates. Methyl salicylate is well known to induce defenses against plant pathogens (Deng et al., 2004) and sucking insects (Saad et al., 2015). Further research is needed to determine if plant pathogen pathways may have been induced in ash trees exposed to high release MeSA lures and if this may have affected parasitism levels in this experiment. Cross-talk between the salicylic and jasmonic acid pathways (Turlings and Erb, 2018), affect pathogen-herbivore interactions that have been linked to effects on parasitism in some systems. Tischeria ekebladella Bjerkander (Lepidoptera: Tischeridae), a leaf miner on oak, experiences higher parasitism on mildew-infected than uninfected oak leaves (Tack et al., 2012). The induction of plant pathogen pathways in ash trees exposed to high release MeSA lures may have affected parasitism levels in this experiment.
It is clear that long range attraction of A. polychrosidis to ash trees infested with C. fraxinella can be enhanced with synthetic HIPVs. Attractiveness of HIPV lures depends on both the chemical constituents and the release rate, and further work is necessary to produce an optimally attractive lure that targets A. polychrosidis. The relationship between wasp attraction and close-range foraging behavior that leads to parasitism also needs to be explored further if HIPVs are to be employed to enhance parasitism of C. fraxinella.
Data Availability Statement
The raw data supporting the conclusions of this article will be made available by the authors, without undue reservation.
Author Contributions
SM assisted in experimental study design, conducted the research, analyzed the data, and lead the writing of the manuscript. ME conceived of the study, obtained the funding for the project, led the experimental design of the study, and participated in editing and writing the manuscript. Both authors contributed to the article and approved the submitted version.
Funding
Funding for this study was provided by the Alberta Crop Industry Development Fund (2015F034R). SM was supported through a TAship from the Department of Biological Sciences at the University of Alberta. In-kind support was provided by the City of Edmonton.
Conflict of Interest
The authors declare that the research was conducted in the absence of any commercial or financial relationships that could be construed as a potential conflict of interest.
Acknowledgments
We thank members of the Evenden Lab in the Department of Biological Sciences at the University of Alberta. In particular, Rebecca Pain assisted with field work, Matthew Russell identified the non-Apanteles parasitic wasps captured on yellow sticky cards, and Ronald Batallas assisted with statistical analyses. The City of Edmonton provided in-kind support, and project input from Mike Jenkins was especially valuable. Suggestions from two reviewers and Cesar Rodriguez-Saona greatly improved this manuscript.
References
Bates, D., Maechler, M., Bolker, B., and Walker, S. (2015). Fitting linear mixed-effects models using lme4. J. Stat. Softw. 67, 1–48. doi: 10.18637/jss.v067.i01
BC Ministry of Forests (1998). Field Manual for Describing Terrestrial Ecosystems. Victoria, BC: Forest Science Program, 16. Land Management Handbook Number 25. ISSN 0229-1622.
Biddinger, D. J., Felland, C. M., and Hull, L. A. (1994). Parasitism of tufted apple bud moth (Lepidoptera: Tortricidae) in conventional insecticide and pheromone-treated Pennsylvania apple orchards. Environ. Entomol. 23, 1568–1579. doi: 10.1093/ee/23.6.1568
Bukovinszky, T., Gols, R., Smid, H. M., Bukovinszkiné Kiss, G. B., Dicke, M., and Harvey, J. A. (2012). Consequences of constitutive and induced variation in the host’s food plant quality for parasitoid larval development. J. Insect Physiol. 58, 367–375. doi: 10.1016/j.jinsphys.2011.12.017
Castelo, M. A., Corley, J. C., and Desouhant, E. (2003). Conspecific avoidance during foraging in Venturia canescens (Hymenoptera: Ichneumonidae): the roles of host presence and conspecific densities. J. Insect Behav. 16, 307–318. doi: 10.1023/a:1023928204793
Colazza, S., Salerno, G., and Wajnberg, E. (1999). Volatile and contact chemicals released by Nezara viridula (Heteroptera:Pentatomidae) have a kairomonal effect on the egg parasitoid Trissolcus basalis (Hymenoptera: Scelionidae). Biol. Control 16, 310–317. doi: 10.1006/bcon.1999.0763
Cossentine, J. E., Deglow, E. K., Jensen, L. B. M., and Goulet, H. (2005). Biological assessment of Macrocentrus linearis and Apanteles polychrosidis (Hymenoptera: Braconidae) as parasitoids of the obliquebanded leafroller. Choristoneura rosaceana (Lepidoptera: Tortricidae). Biocontrol. Sci. Techn. 15, 711–720. doi: 10.1080/09583150500136022
Cossentine, J. E., Jensen, L., Deglow, E., Goulet, H., Huber, J. T., and O’Hara, O. (2004). The parasitoid complex affecting Choristineura rosaceana and Pandemis limitata in organically managed apple orchards. BioControl 49, 359–372. doi: 10.1023/B:BICO.0000034603.56877.0b
D’Alessandro, M., Brunner, V., Mérey, G. V., and Turlings, T. C. J. (2009). Strong attraction of the parasitoid Cotesia marginiventris towards minor volatile compounds of maize. J. Chem. Ecol. 35, 999–1008. doi: 10.1007/s10886-009-9692-7
De Moraes, C. M. D., Lewis, W. J., Paré, P. W., Alborn, H. T., and Tumlinson, J. H. (1998). Herbivore-infested plants selectively attract parasitoids. Nature 393, 570–573. doi: 10.1038/31219
Degen, T., Bakalovic, N., Bergvinson, D., and Turlings, T. C. J. (2012). Differential performance and parasitism of caterpillars on maize inbred lines with distinctly different herbivore-induced volatile emissions. PLoS One 7:e47589. doi: 10.1371/journal.pone.0047589
Deng, C., Zhang, X., Zhu, W., and Qian, J. (2004). Investigation of tomato plant defence response to tobacco mosaic virus by determination of methyl salicylate with SPME-capillary GC-MS. Chromatographia 59, 263–268. doi: 10.1365/S10337-003-0144-1
Dudareva, N., Negre, F., Nagegowda, D. A., and Orlova, I. (2006). Plant volatiles: recent advances and future perspectives. Crit. Rev. Plant Sci. 25, 417–440. doi: 10.1080/073526806008999973
Engelberth, J., Alborn, H. T., Schmelz, E. A., and Tumlinson, J. H. (2004). Airborne signals prime plants against insect herbivore attack. PNAS 101, 1781–1785. doi: 10.1073/pnas.0308037100
Evenden, M. L. (2009). Biology of Caloptilia fraxinella (Lepidoptera: Gracillariidae) on ornamental green ash, Fraxinus pennsylvanica (Oleaceae). Can. Entomol. 141, 31–39. doi: 10.4039/n08-036
Evenden, M. L., Armitage, G., and Lau, R. (2007). Effects of nutrition and methoprene treatment upon reproductive diapause in Caloptilia fraxinella (Lepidoptera: Gracillariidae). Physiol. Entomol. 32, 275–282. doi: 10.1111/j.1365-3032.2007.00581.x
Evenden, M. L., and Gries, R. (2008). Plasticity of male response to sex pheromone depends on physiological state in a long-lived moth. Anim. Behav. 75, 663–672. doi: 10.1016/j.anbehav.2007.07.020
Farag, M. A., Fokar, M., Abd, H., Zhang, H., Allen, R. D., and Par, P. W. (2004). (Z)-3-Hexenol induces defense genes and downstream metabolites in maize. Planta 220, 900–909. doi: 10.1007/s00425-004-1404-5
Farag, M. A., and Paré, P. W. (2002). C6-Green leaf volatiles trigger local and systemic VOC emissions in tomato. Phytochem 61, 545–554. doi: 10.1016/s0031-9422(02)00240-6
Fernández-Triana, J. L., and Huber, J. T. (2010). Braconid parasitoids (Hymenoptera: Braconidae) of Nearctic Choristoneura species (Lepidoptera: Tortricidae), with a summary of other parasitoid families attacking Choristoneura. Can. Entomol. 142, 295–343. doi: 10.4039/n10-025
Garrido, I., Espinosa, F., and Álvarez-Tinaut, M. C. (2009). Oxidative defence reactions in sunflower roots induced by methyl-jasmonate and methyl-salicylate and their relation with calcium signalling. Protoplasma 237, 27–39. doi: 10.1007/s00709-009-0069-0
Godfray, H. J. C. (1994). Parasitoids: Behavioral and Evolutionary Ecology. Princeton, NJ: Princeton University Press.
Hoballah, M. E. F., Tamo, C., and Turlings, T. C. J. (2002). Differential attractiveness of induced odors emitted by eight maize varieties for the parasitoid Cotesia marginiventris: is quality or quantity important? J. Chem. Ecol. 28, 951–968. doi: 10.1023/a:1015253600083
Hothorn, T., Bretz, F., and Westfall, P. (2008). Simultaneous inference in general parametric models. Biom. J. 50, 346–363. doi: 10.1002/bimj.200810425
James, D. G., and Grasswitz, T. (2005). Synthetic herbivore-induced plant volatiles increase field captures of parasitic wasps. BioControl 50, 871–880. doi: 10.1007/s10526-005-3313-3
James, D. G., and Price, T. S. (2004). Field-testing of methyl salicylate for recruitment and retention of beneficial insects in grapes and hops. J. Chem. Ecol. 30, 1613–1628. doi: 10.1023/B:JOEC.0000042072.18151.6f
Kant, R., and Minor, M. A. (2017). Parasitoid Diaeretiella rapae (Hymenoptera: Braconidae) adjusts reproductive strategy when competing for hosts. Environ. Entomol. 46, 521–527. doi: 10.1093/ee/nvx057
Kaplan, I. (2012). Attracting carnivorous arthropods with plant volatiles: the future of biocontrol or playing with fire? Biol. Control 60, 77–89. doi: 10.1016/j.biocontrol.2011.10.017
Klomp, H. (1981). Parasitic wasps as sleuthhounds: response of an ichneumon wasp to the trail of its host. Neth. J. Zool. 31, 762–772.
Lessells, C. M. (1985). Parasitoid foraging: should parasitism be density dependent? J. Anim. Ecol. 54, 27–41. doi: 10.2307/4618
Li, S., Fitzpatrick, S., Troubridge, J., Sharkey, M., Barron, J., and O’Hara, J. (1999). Parasitoids reared from the obliquebanded leafroller (Lepidoptera: Tortricidae) infesting raspberries. Can. Entomol. 131, 399–404. doi: 10.4039/Ent131399-3
Mallinger, R. E., Hogg, D. B., and Gratton, C. (2011). Methyl salicylate attracts natural enemies and reduces populations of soybean aphids (Hemiptera: Aphididae) in soybean agroecosystems. J. Econ. Entomol. 104, 115–124. doi: 10.1603/ec10253
Morrison, G., and Strong, D. R. (1980). Spatial variations in host density and the intensity of parasitism: some empirical examples. Environ. Entomol. 9, 149–152. doi: 10.1093/ee/9.2.149
Ngumbi, E., Chen, L., and Fadamiro, H. Y. (2009). Comparative GC-EAD responses of a specialist (Microplitis croceipes) and a generalist (Cotesia marginiventris) parasitoid to cotton volatiles induced by two caterpillar species. J. Chem. Ecol. 35, 1009–1020. doi: 10.1007/s10886-009-9700-y
Ozawa, R., Arimura, G.-I., Takabayashi, J., Shimoda, T., and Nishioka, T. (2000). Involvement of jasmonate- and salicylate-related signaling pathways for the production of specific herbivore-induced volatiles in plants. Plant Cell Physiol. 41, 391–398. doi: 10.1093/pcp/41.4.391
Pfannenstiel, R. S., Mackey, B. E., and Unruh, T. R. (2012). Leafroller parasitism across an orchard landscape in central Washington and effect of neighboring rose habitats on parasitism. BioControl 62, 152–161. doi: 10.1016/jbiocontrol.2012.04.006
Pohl, G. R., Saunders, C., Barr, W. B., Wartenbe, M. D., and Fownes, S. L. (2004). Caloptilia fraxinella (Lepidoptera: Gracillariidae), a new pest of ash (Oleaceae: Fraxinus spp.) on the Canadian Prairies. Can. Entomol. 136, 733–736. doi: 10.4039/n03-101
Quicke, D. L. J. (2015). The Braconid and Ichneumonid Parasitoid Wasps: Biology, Systematics, Evolution and Ecology. New York, NY: Wiley-Blackwell.
R Core Team (2017). R: A Language and Environment for Statistical coMputing. Vienna: R Foundation for Statistical Computing.
Rodriguez-Saona, C., Blaauw, B. R., and Isaacs, R. (2012). “Manipulation of natural enemies in agroecosystems: habitat and semiochemicals for sustainable insect pest control,” in Integrated Pest Management and Pest Control -Current and Future Tactics, eds M. L. Larramendy and S. Solenski (London: IntechOpen), 89–126. doi: 10.5772/30375
Rodriguez-Saona, C., Kaplan, I., Braasch, J., Chinnawamy, D., and Williams, L. (2011). Field responses of predaceous arthropods to methyl salicylate: a meta-analysis and case study in cranberries. Biol. Control 59, 294–303. doi: 10.1016/j.biocontrol.2011.06.017
Röse, U., Lewis, J., and Tumlinson, J. H. (1998). Specificity of systemically released cotton volatiles as attractants for specialist and g eneralist parasitic wasps. J. Chem. Ecol. 24, 303–319. doi: 10.1023/A:1022584409323
Saad, K., Mohamad Roff, M. N., Hallett, R., and Idris, A. B. (2015). Aphid-induced defences in chilli affect preferences of the whitefly, Besmisia tabaci (Hemiptera: Aleyrodidae). Sci. Rep. 5:13697. doi: 10.1038/srep13697
Sasso, R., Iodice, L., Woodcock, C. M., Pickett, J. A., and Guerrieri, E. (2009). Electrophysiological and behavioural responses of Aphidius ervi (Hymenoptera: Braconidae) to tomato plant volatiles. Chemoecol 19, 195–201. doi: 10.1007/s00049-009-0023-9
Scala, A., Allmann, S., Mirabella, R., Haring, M. A., and Schuurink, R. C. (2013). Green leaf volatiles: a plant’s multifunctional weapon against herbivores and pathogens. Int. J. Mol. Sci. 14, 17781–17811. doi: 10.3390/ijms140917781
Schröder, R., and Hilker, M. (2008). The relevance of background odor in resource location by insects: a behavioral approach. BioScience 58, 308–316. doi: 10.1641/B580406
Seaman, A. J., Nyrop, J. P., and Dennehy, T. J. (1990). Egg and larval parasitism of the grape berry moth (Lepidoptera: Tortricidae) in three grape habitats in New York. Environ. Entomol. 19, 764–770. doi: 10.1093/ee/19.3.764
Shiojiri, K., Ozawa, R., Matsui, K., Kishimoto, K., Kugimiya, S., and Takabayashi, J. (2006). Role of the Lipoxygenase/lyase pathway of host-food plants in the host searching behavior of two parasitoid species, Cotesia glomerata and Cotesia plutellae. J. Chem. Ecol. 32, 969–979. doi: 10.1007/s10886-006-9047-6
Shulaev, V., Silverman, P., and Raskin, I. (1997). Airborne signalling by methyl salicylate in plant pathogen resistance. Nature 385, 718–721. doi: 10.1038/385718a0
Simpson, M., Gurr, G. M., Simmons, A. T., Wratten, S. D., James, D. G., Leeson, G., et al. (2010). Insect attraction to synthetic herbivore-induced plant volatile-treated field crops. Agric. For. Entomol. 13, 45–57. doi: 10.1111/j.1461-9563.2010.00496.x
Snoeren, T. A. L., Mumm, R., Poelman, E. H., Yang, Y., Pichersky, E., and Dicke, M. (2010). The herbivore-induced plant volatile methyl salicylate negatively affects attraction of the parasitoid Diadegma semiclausum. J. Chem. Ecol. 36, 479–489. doi: 10.1007/s10886-010-9787-1
Tack, A. J. M., Gripenberg, S., and Roslin, T. (2012). Cross-kingdom interactions matter: fungal-mediated interactions structure an insect community on oak. Ecol. Lett. 15, 177–185. doi: 10.1111/j.1461-0248.2011.01724.x
Tamiru, A., Bruce, T. J. A., Woodcock, C. M., Birkett, M. A., Midega, C. A. O., Pickett, J. A., et al. (2015). Chemical cues modulating electrophysiological and behavioural responses in the parasitic wasp Cotesia sesamiae. Can. J. Zool. 93, 281–287. doi: 10.1139/cjz-2014-0266
Tang, F., Fu, Y.-Y., and Ye, J.-R. (2015). The effect of methyl salicylate on the induction of direct and indirect plant defense mechanisms in poplar (Populus× euramericana ‘Nanlin 895’). J. Plant Interact. 10, 93–100. doi: 10.1080/17429145.2015.1020024
Turlings, T. C. J., and Erb, M. (2018). Tritrophic interactions mediated by herbivore-induced plant volatiles: mechanisms, ecological relevance, and application potential. Annu. Rev. Entomol. 63, 433–452. doi: 10.1146/annurev-ento-020117-043507
Turlings, T. C. J., Tumlinson, J. H., Heath, R. R., Proveaux, A. T., and Doolittle, R. E. (1991). Isolation and identification of allelochemicals that attract the larval parasitoid, Cotesia marginiventris (Cresson), to the microhabitat of one of its hosts. J. Chem. Ecol. 17, 2235–2251. doi: 10.1007/BF00988004
Uefune, M., Choh, Y., Abe, J., Shiojiri, K., Sano, K., and Takabayashi, J. (2011). Application of synthetic herbivore-induced plant volatiles causes increased parasitism of herbivores in the field. J. Appl. Entomol. 136, 561–567. doi: 10.1111/j.1439-0418.2011.01687.x
Vet, L. E., Dicke, M., and Wäckers, F. L. (1991). How to hunt for hiding hosts: the reliability-detectability problem in foraging parasitoids. Neth. J. Zool. 41, 202–213.
Visser, M. E., Hefin Jones, T., and Driessen, G. (1999). Interference among insect parasitoids: a multi-patch experiment. J. Anim. Ecol. 68, 108–120. doi: 10.1046/j.1365-2656.1999.00269.x
Visser, M. E., van Alphen, J. M., and Nell, H. W. (1992). Adaptive superparasitism and patch time allocation in solitary parasitoids: the influence of pre-patch experience. Behav. Ecol. Sociobiol. 31, 163–171. doi: 10.1007/BF00168643
Whitman, D. W., and Eller, F. J. (1992). Orientation of Microplitis croceipes (Hymenoptera: Braconidae) to green leaf volatiles: dose-response curves. J. Chem. Ecol. 18, 1743–1753. doi: 10.1007/BF02751099
Wist, T. J., and Evenden, M. L. (2013). Parasitoid complex and bionomics of Apanteles polychrosidis (Hymenoptera: Braconidae) on the ash leaf-cone roller (Lepidoptera: Gracillariidae). Can. Entomol. 145, 416–429. doi: 10.4039/tce.2013.26
Wist, T. J., Gries, R., and Evenden, M. L. (2015). Differential parasitism by a generalist parasitoid is mediated by volatile organic chemicals of the herbivore’s host. Arthropod Plant Int. 9, 515–527. doi: 10.1007/s11829-015-9393-9
Yu, H., Zhang, Y., Wu, K., Gao, X. W., and Guo, Y. Y. (2008). Field-testing of synthetic herbivore-induced plant volatiles as attractants for beneficial insects. Environ. Entomol. 37, 1410–1415. doi: 10.1603/0046-225x-37.6.1410
Keywords: parasitoid, herbivore-induced plant volatile, conservation biological control, horticulture, urban forest
Citation: McPike SM and Evenden ML (2021) Host Plant Volatile Lures Attract Apanteles polychrosidis (Hymenoptera: Braconidae) to Ash Trees Infested With Caloptilia fraxinella (Lepidoptera: Gracillariidae). Front. Ecol. Evol. 9:701954. doi: 10.3389/fevo.2021.701954
Received: 28 April 2021; Accepted: 16 June 2021;
Published: 09 July 2021.
Edited by:
Cesar Rodriguez-Saona, Rutgers, The State University of New Jersey, United StatesReviewed by:
Jian J. Duan, United States Department of Agriculture, United StatesTherese Marie Poland, United States Department of Agriculture, United States
Copyright © 2021 McPike and Evenden. This is an open-access article distributed under the terms of the Creative Commons Attribution License (CC BY). The use, distribution or reproduction in other forums is permitted, provided the original author(s) and the copyright owner(s) are credited and that the original publication in this journal is cited, in accordance with accepted academic practice. No use, distribution or reproduction is permitted which does not comply with these terms.
*Correspondence: Maya L. Evenden, mevenden@ualberta.ca