Diet Drives Differences in Reproductive Synchrony in Two Sympatric Mountain Ungulates in the Himalaya
- 1National Centre for Biological Sciences, Bengaluru, India
- 2Centre for Wildlife Studies, Bengaluru, India
- 3Manipal University, Manipal, India
- 4Laboratory for the Conservation of Endangered Species, Council of Scientific and Industrial Research (CSIR)-Centre for Cellular and Molecular Biology, Hyderabad, India
Ungulates in higher latitudes and altitudes experience sharp seasonal changes in forage abundance and quality. In response, ungulates show varying degrees of synchrony in reproduction. Diet type has been hypothesized to be a determinant of differences in reproductive synchrony. Analyses at global scales using proxies of plant phenology such as climate, latitude and Normalized Difference Vegetation Index (NDVI) have found no evidence in support because such proxies do not capture differences in phenology among plant taxa at local scales. We compared seasonal variations in diet quality with reproductive synchrony in the Himalayan musk deer (Moschus chrysogaster), a browser, and the Himalayan goral (Naemorhedus goral), a grazer, in mid-altitude Himalaya. We also compared seasonal variations in physiological stress using fecal glucocorticoid metabolite (FGM). We identified different stages of female reproductive cycle using fecal concentrations of metabolites of estradiol, pregnanediol-3-glucuronide (PdG) and testosterone and used fecal crude protein (CP) as an indicator of diet quality. In musk deer, fecal estradiol and PdG concentrations showed a dispersed estrous and parturition, respectively. Goral had a more synchronized estrous and parturition. Estrous cycles in both species occurred when diet quality was poor, but parturition occurred when diet quality was high. Greater seasonality in reproduction in goral is driven by sharp phenological changes in graminoids on which it feeds, compared to slow changes in browse on which musk deer feeds. Thus, we show that diet type drives the differences in reproductive synchrony in these two sympatric species. Spring and summer with highest diet quality were times of highest stress in both the ungulates. We hypothesize predation pressure from feral dogs and resource competition with livestock as plausible explanations for this, which need to be tested in future. Our findings also highlight the need for studying relationships among plant phenology, diet type and reproductive biology of ungulates at local scales if we are to understand species responses to global phenomena such as climate change.
Introduction
Ungulates in higher latitudes and altitudes experience sharp seasonal changes in environmental conditions and forage abundance and quality (Post, 2003; Bronson, 2009; English et al., 2012; Stoner et al., 2016). A shortening of the season with palatable forage makes reproduction metabolically challenging for these ungulates (Rutberg, 1987). In female ungulates, nutritional demands are highest during late gestation and lactation, the latter being greater than the former (Oftedal, 1985; Clutton-Brock et al., 1989). Mating season in these ungulates is separated from the birth season by an over winter gestation period, thus maximizing the chance of late gestation and births coinciding with peak nutritional availability in spring or early summer (Loe et al., 2005; Moyes et al., 2011; Owen-Smith and Ogutu, 2013). In addition to meeting lactational requirements in spring and summer, females must gain nutritional reserves to carry out breeding in late autumn or early winter (Parker et al., 2005; Pettorelli et al., 2006). The acquisition of these nutritional reserves depends on the plant growth forms on which they primarily feed (Iversen et al., 2014). Plant growth forms strongly influence their phenology, especially in higher latitudes and altitudes. Grasses and forbs uptake nutrients faster immediately following winter compared to shrubs and other evergreens, and therefore show faster phenological changes (Iversen et al., 2009). Thus, a grazer faces more drastic seasonal changes in forage quality, compared to a browser in the same environment, which can influence the scheduling of reproduction by them (Rutberg, 1987; Owen-Smith and Ogutu, 2013). Grazers in highly seasonal environments need to build up a capital of nutrients during the peak in the abundance and quality of their forage, which is in spring and summer, and then expend it during a harsh winter and gestation. Such breeders have been termed capital breeders (Jonsson, 1997; Stephens et al., 2009). Browsers living in the same environment can mostly meet their resource needs for gestation and lactation concurrently, as the quality and abundance of their forage show relatively less drastic seasonal changes. These have been called income breeders (Jonsson, 1997). Thus, in the same habitat, grazers are likely to be at the capital breeder end of the spectrum, with greater synchrony in their reproduction, and the browsers toward income breeder end with lower synchrony. English et al. (2012) analyzed birth season length in 38 ungulates and found that the synchrony in births increased with seasonality and inter-annual constancy in Normalized Difference Vegetation Index (NDVI), an indicator of resource availability. Rutberg (1987) found climate to be a major driver of birth season length in 27 species of ungulates. Both these studies did not find any evidence for the influence of diet type on birth season length, although it was a predictor in their analyses. As English et al. (2012) have noted, global drivers of reproductive synchrony can hide the influence of local drivers, especially the differences among plant taxa in phenology which have implications on ungulate reproduction depending on their diet type.
Most ungulates in high altitudes and latitudes switch their diet from the growing season to winter when there is severe resource crunch. Grazers switch from graminoids to browse or lichens, due to drastic decline in nutritive value of graminoids as well as limited access due to snow cover. Examples include woodland caribou (Rangifer tarandus tarandus) (Thompson et al., 2015), blue sheep (Pseudois nayaur) (Suryawanshi et al., 2010) and mountain goat (Oreamnos americanus) (Fox and Smith, 1988). Such dietary switch is followed by a drastic dip in dietary nitrogen (N) (Klein, 1990; Barboza et al., 2018) which is the most limiting nutrient for wild ungulates (Robbins, 1993; White, 1993). Studies suggest that 5–9% of crude protein (CP) content is needed to maintain minimum protein balance in wild adult ruminants (Robbins, 1993). The inability to accomplish this would result in high nutritional stress which is in turn associated with physiological stress [indicated by Fecal Glucocorticoid Metabolite (FGM) levels] in barren-ground caribou (Rangifer tarandus groenlandicus) (Joly et al., 2015), and in red deer (Cervus elaphus) (Huber et al., 2003). In barren-ground caribou stress levels were greater in pregnant females (Joly et al., 2015). Such stress is expected to be greater in grazers which have to switch from a monocot diet in spring and summer to dicots in winter, in addition to having to depend on body reserves during gestation in winter. In contrast, ungulates that feed primarily on browse switch only from one dicot growth form to another during winter, e.g., mule deer (Odocoileus hemionus) from forbs to shrubs and elk (Cervus elaphus) from shrubs to tree foliage (Hosten et al., 2007). Therefore, they may be under less nutritional and physiological stress. However, comparable data on physiological stress do not exist for browsers, to the best of our knowledge. Nonetheless, we expect physiological stress to be associated with gestation in grazers more than in browsers. Another factor that influences the seasonal patterns in reproduction of these ungulates is the photoperiod or daylength (Bronson, 1989; Zerbe et al., 2012). In highly seasonal breeders, photoperiodic stimulus triggers estrous and thus synchrony in conception (Spinage, 1973; Zerbe et al., 2012). However, unlike higher latitudes where seasonal change in daylength is drastic, it is not so in tropical latitudes. Since grazers are expected to show greater synchrony in reproduction, we can expect the onset of estrous in them to be more correlated with photoperiod than in browsers in the same environment.
In this study, we compared reproductive synchrony and physiological stress in two sympatric ungulates in mid-altitude Himalaya, in relation to seasonal variation in their diet quality. With different body sizes and phylogenies, the two species have different feeding habits (Green, 1987a), with the smaller Himalayan musk deer being a browser feeding primarily on dicots and the Himalayan goral feeding on graminoids, except in winter when dicots form a significant part of their diet (Srivastava, 2019). In their habitat, dicots have higher CP than graminoids throughout the year without a distinct peak, while the graminoids have a distinct peak in the growing season and a rapid decline in autumn (Srivastava, 2019). The gestation period which mostly coincides with winter ranges from 183 to199 days in Himalayan musk deer (Mithileshwari et al., 2016) and 170 to 218 days in Himalayan goral (Duckworth and MacKinnon, 2008). We therefore expected seasonal reproductive patterns in both species to exhibit strong associations with seasonal variations in fecal CP, an indicator of diet quality in ungulates (Leslie et al., 2008). We expected the fecal glucocorticoid metabolite (FGM) concentration, a widely used indicator of physiological stress (Keay et al., 2006), to be negatively related to the fecal CP in both the species and positively correlated with gestation. Finally, since seasonal changes in daylength are more gradual in the sub-tropics compared to higher latitudes, we hypothesized poor association of daylength with the reproductive seasonality in both the ungulates. We specifically asked—(1) How do the variations in fecal metabolites of estradiol, progesterone and glucocorticoids relate to different reproductive stages, including estrous, gestation and parturition? (2) Do the reproductive stages correlate with diet quality, physiological stress and daylength? (3) Which is more closely related to increased stress levels – reproductive stages or diet quality?
Materials and Methods
Study Area
We conducted this study in and around Kyongnosla Alpine Sanctuary in Sikkim, India (Figure 1A), from December 2014 to December 2015. The Sanctuary encompasses an area of 31 km2 with an elevational range of 3,200–4,200 m. Three wild ungulates occur here—the Himalayan musk deer, Himalayan goral and Himalayan serow (Capricornis sumatraensis thar). Musk deer and goral have overlapping distribution, with the former mostly occurring >3,300 m and goral mostly <3,600 m. Although serow is distributed across the altitude gradient, it is less abundant (Srivastava and Kumar, 2018). Musk deer is solitary and territorial while goral occurs in small groups of 4–6 animals, including males and females (Menon, 2014).
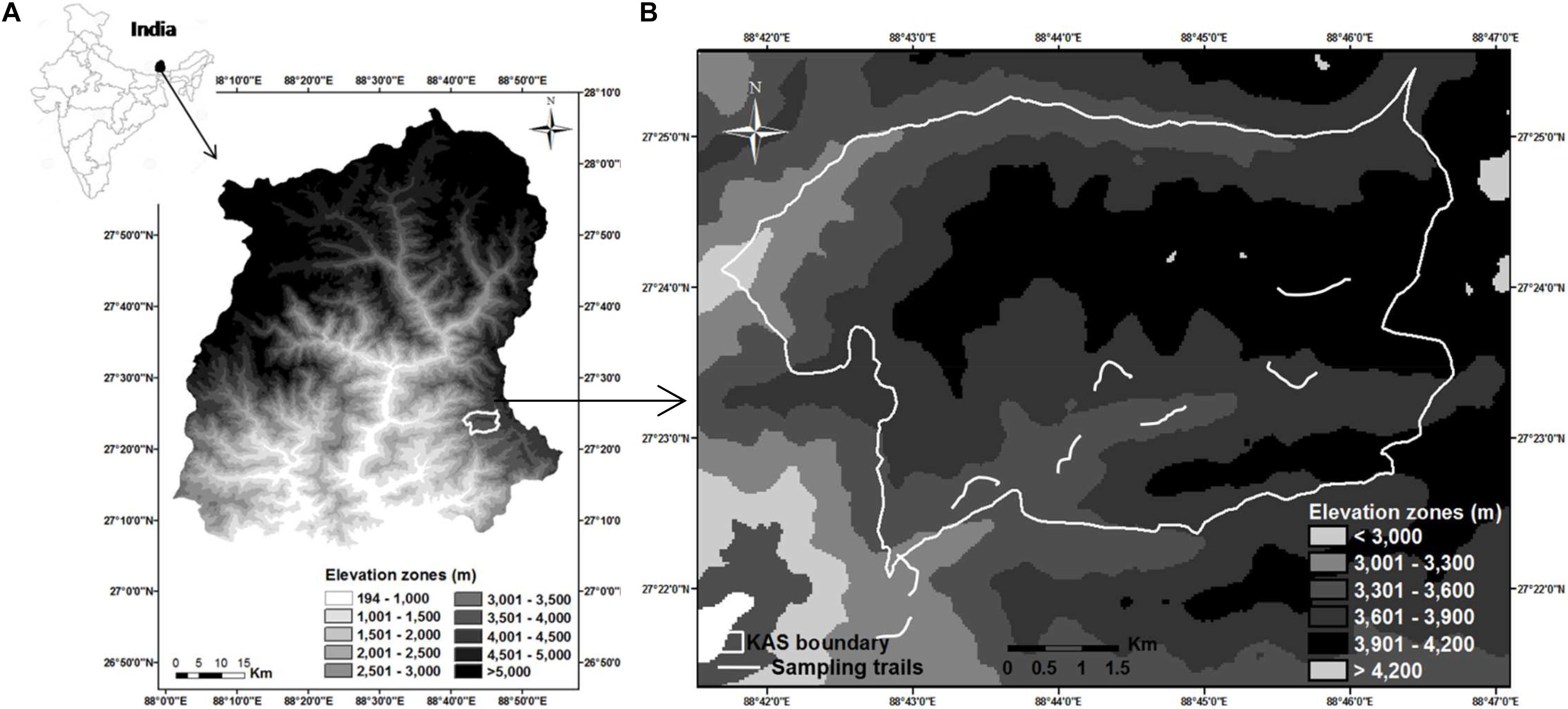
Figure 1. Study area map showing (A) location of Kyongnosla Alpine Sanctuary in Sikkim (B) classification of elevation zones and location of sampling trails—Trails located in elevation zones below 3,600 m are for goral and those above 3,600 m are for musk deer.
The area experiences a highly seasonal climate with the maximum temperature recorded during this study as 18.50 ± 0.70°C in July below 3,600 m and minimum temperature being -8.27 ± 0.52°C in January above 3,600 m. Areas < 3,600 m had snowfall from January to April, with 80% snow cover in January, while areas >3,600 m remained snow covered from November till May, with the snow cover between 50 and 100%. Based on the mean minimum and maximum temperatures recorded and the data on vegetation phenology in the area, we could group the months into four seasons: spring (April–June), summer (July–September), autumn (October–December), and winter (January–March) (Srivastava, 2019).
Fecal Sample Collection
We marked five permanent trails in musk deer habitat and four trails in goral habitat (Figure 1B) ranging from 600 to 900 m in length (Table 1). We spaced the trails at least 2–2.5 km apart from each other so that the home ranges of different animals in case of musk deer (0.47–1.81 km2 in Moschus chrysogaster and M. moschiferous, Green, 1987b; Zaitsev et al., 2015) and different groups of goral (1.08–1.46 km2, Cho et al., 2015), did not overlap. This minimized the probability of sampling the same animal on different trails. We monitored each of these trails in the morning at an interval of 4–5 days, from January to December 2015, to collect only fresh fecal samples. From each pellet group encountered, we collected around 10 pellets. Also, to avoid pseudo replication, we either removed or marked the pellet groups collected. The fecal pellets of the two species were identified visually as they were of different sizes and shapes (Figure 2). To avoid steroid hormone degradation in the fecal samples collected, they were oven-dried at 60°C for 48 h on the same day of collection in the field and then stored at -20°C until further laboratory analysis.
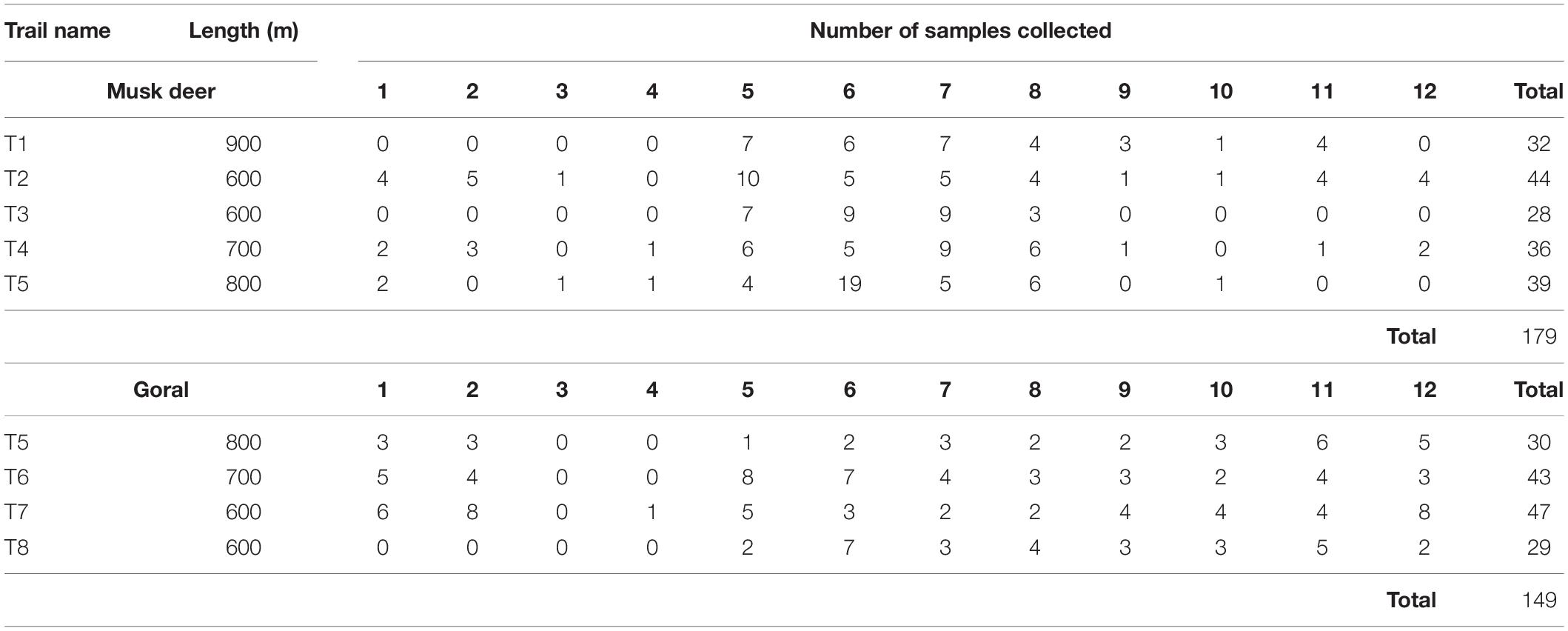
Table 1. The number of fecal samples of musk deer and goral collected from different trails in different months starting from January to December coded as 1 to 12.
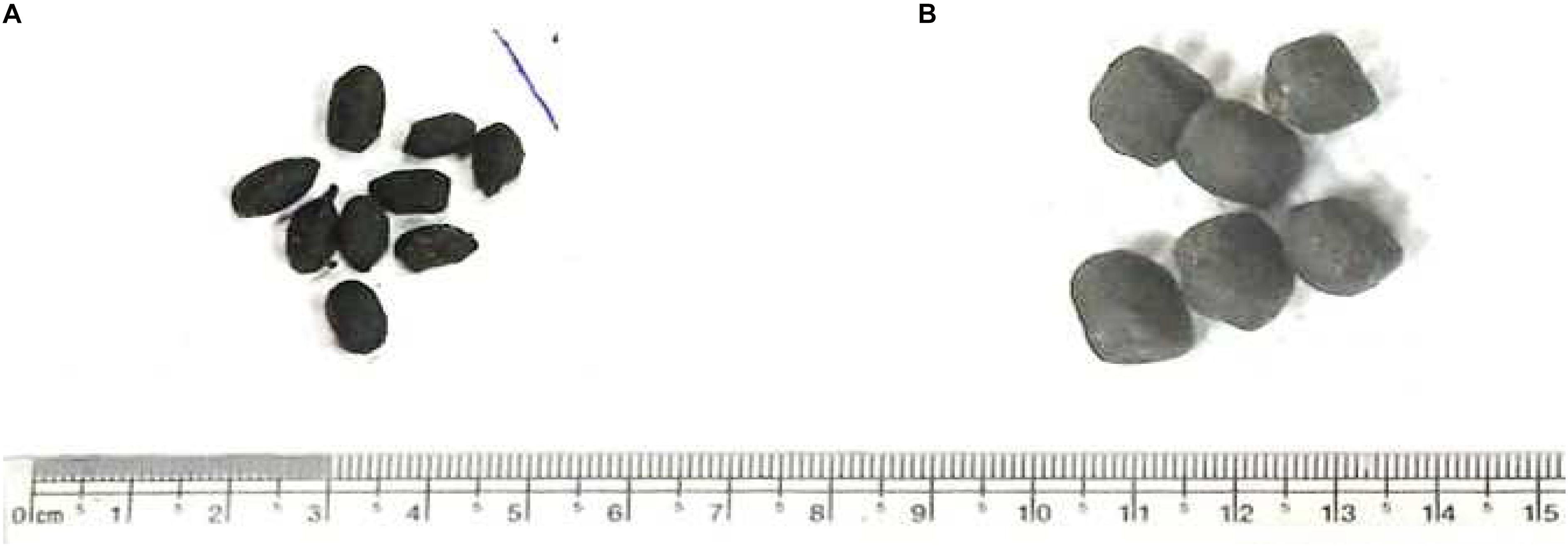
Figure 2. Photographs of fecal pellets of (A) musk deer and (B) goral, for size and shape comparisons.
Estimation of Fecal Hormone Metabolites
We measured the concentrations of fecal metabolites of estradiol, pregnanediol-3-glucuronide (PdG), testosterone and glucocorticoids. Estrogen metabolites have been used for determining the onset of estrous and length of estrous cycles in ungulates (Plotka et al., 1980; Conception et al., 2011). Metabolites of progesterone are widely used for pregnancy detection in many ungulates (Shivaji et al., 2003; Cain et al., 2012; Khonmee et al., 2014a; Mithileshwari et al., 2016) and in the identification of estrous and luteal phases (Kirkpatrick et al., 1992; Schwartz et al., 1995; Kapke et al., 1999). Typically, estrogen peaks at the time of ovulation and troughs during diestrus and pregnancy. Progesterone levels trough during estrous, remain high during luteal phase, but reach the highest concentrations during pregnancy, with a sharp drop at parturition (Budithi et al., 2016; Mithileshwari et al., 2016). We used FGM to assess stress levels as used in other ungulates (Saltz and White, 1991; Huber et al., 2003; Keay et al., 2006). We used testosterone in combination with estradiol and PdG to assign sex to the collected fecal samples (Oates et al., 2002; Bajra et al., 2008, 2011).
Extraction of Fecal Steroid Metabolites
We extracted fecal steroid metabolites (Estradiol, PdG, testosterone and glucocorticoids) following standard procedures (Umapathy et al., 2013; Budithi et al., 2016). Dried pellet samples were pulverized and 0.2 gm of each was boiled in 5 ml of 90% ethanol for 20 min, then centrifuged at 500 g for 10 min and the supernatant was transferred into a fresh glass tube. The pellet was re-suspended in 5 ml of 90% ethanol, vortexed for 1 min and centrifuged again. Both the supernatants were pooled, dried at 40°C in oven and re-suspended in 1 ml of absolute ethanol and stored at -20°C until assayed.
Hormone Assays and Validation
We estimated fecal estradiol concentration using estradiol polyclonal antibody (R0008; Dr. Coralie Munro lab, University of California, Davis, CA, United States). Estradiol antibody cross-reacts 100% with estradiol, 3% with estrone and 1% with others (Umapathy et al., 2015). We estimated fecal PdG concentration using PdG polyclonal antibody (R13904; Dr. Coralie Munro lab, University of California, Davis, CA, United States). PdG antibody cross-reacts 100% with PdG, 45% with 20α hydroxy-4-pregnen-3-one and less than 10% with other progestagens (O’Connor et al., 2011).
We measured fecal testosterone concentrations using testosterone polyclonal antibody (R156/7; Dr. Coralie Munro lab University of California, Davis). Cross-reactivity of testosterone antibody with testosterone 100%, dihydrotestosterone was 57.4%, <0.3% with androstenedione and < 0.1% with androsterone, dihydroepiandrosterone, β-estradiol and progesterone (Dloniak et al., 2004; Kumar et al., 2014). We measured FGM concentration using cortisol polyclonal antibody R4866; Dr. Coralie Munro lab University of California, Davis). The cortisol antibody cross-reacts with cortisol 100%, prednisolone 9.9%, prednisone 6.3% cortisone 5% and <1% with corticosterone, desoxycorticosterone, 21 -desoxycortisone, testosterone, androstenedione, androsterone and 11-desoxycortisol (Young et al., 2004; Kumar et al., 2014, 2019). We followed previously validated methods in our laboratory for all the Enzyme Immunoassays (EIAs) including PdG (Mithileshwari et al., 2016), estradiol (Munro and Lasley, 1988; Umapathy et al., 2015), testosterone (Kumar et al., 2014), and cortisol (Kumar et al., 2014, 2019). We have also biologically validated these hormones in musk deer for PdG (Mithileshwari et al., 2016) estradiol, testosterone and cortisol (Unpublished data). For goral, we could not perform the physiological validation due to non-availability animals in captivity, however these hormones have been validated in other species i.e., Chinese goral (Naemorhedus griseus) (Khonmee et al.,2014a,b).
We drew parallel displacement curves to demonstrate parallelism between the pooled fecal extracts of musk deer and goral (endogenous hormone) and their respective standards (exogenous hormone) to determine the optimum fecal sample dilution at 50% binding and validated immunogenicity of endogenous hormone with respective assay antibodies (Kumar and Umapathy, 2019). The pooled fecal extracts of musk deer and goral and standard curves showed similar slopes for all the hormones (within r = 0.90). We calculated assay sensitivities at 90% binding and found them to be 1.95 pg/well for estradiol, 39 pg/well for PdG, 1.17 pg/well for testosterone and 1.95pg/well for cortisol. The respective intra and inter-assay coefficients of variation (CV) were 3.82 and 6.01% (n = 10) for estradiol, 6.81 and 8.42% (n = 10) for PdG, 3.87 and 6.57% (n = 10) for cortisol and 6.83 and 8.54% for testosterone (n = 10). Recovery of known amount of unlabeled hormones were 90 ± 3.83%, 85.1 ± 2.4%, 87.3 ± 5.3%, and 83.6 ± 4.3% for PdG, estradiol, testosterone and cortisol, respectively. Moreover, the correlation (r2) and slope (m) values for the recovery of hormones were r2 = 0.99, m = 0.92; r2 = 0.99, m = 0.96; r2 = 0.98, m = 0.94; and r2 = 0.98, m = 0.92 for PdG, estradiol, testosterone and cortisol, respectively. We performed the EIAs for PdG (Mithileshwari et al., 2016), estradiol (Umapathy et al., 2015), testosterone (Kumar et al., 2014), and cortisol (Budithi et al., 2016) as described previously.
Fecal CP Estimation
We used fecal CP content as an indicator of the quality of ungulate diet (Leslie et al., 2008). We used a subsample from each dried pellet group to estimate fecal CP. We ground the dried subsamples in a Willey mill at 0.20 mm mesh size to estimate total Carbon (C) and Nitrogen (N) using a CN analyzer (Leco Instruments, St Joseph, MI, United States). Following this, we multiplied the N values with 6.25 to obtain CP content (Klein, 1990; Parker et al., 2005).
Data Analyses
Sex Assignment to Samples
We were unable to identify sex of the collected fecal samples using molecular genetics due to logistic constraints. We, therefore, used the concentrations of testosterone + PdG + estradiol (T + P + E) and the testosterone/PdG (T/P) ratio to assign sex to each fecal sample using k-means conglomerate analysis (using package stats in R), both of which have shown higher values in males in many mammals (Bajra et al., 2008, 2011). We additionally used estradiol/testosterone (E/T) ratio as reported in echidnas Tachyglossus aculeatus for sexing the samples (Oates et al., 2002). Previously, population level estimates of fecal hormones without assigning sex to the collected samples have been used by Creel et al. (2009) to conclude over aspects of reproductive and stress physiology of elk. We expect that our method has excluded the female calves with low concentrations of estradiol and PdG from the analysis by classifying them as males, thus further refining the results.
Prior to statistical analysis, we tested the dataset for normal distribution using Kolmogorov-Smirnov test of normality (using package ks in R). We used Mann-Whitney-Wilcoxon statistic to test for differences between the two groups in the concentrations of hormone metabolites.
Identification of Peaks in Hormone Metabolite Concentrations
For samples that we identified as those of females, we plotted the concentrations of estradiol and PdG against day of collection, and set mean + 2SD as a threshold to estimate baseline and peak values (Brown et al., 2004). We also examined monthly variations in fecal concentrations of estradiol, PdG and FGM. We used linear regression to examine the relationships of response variables i.e., the monthly estradiol and PdG concentrations with the explanatory variables i.e., fecal CP, fecal FGM, and daylength (calculated using the Geosphere package in R). All the analyses were done with statistical software R 3.0.1 (R Development Core Team, 2013).
Results
As both the study species were rare in the area (Srivastava and Kumar, 2018), even after continuous repeated monitoring of the trails we were able to collect only a limited number of samples. The number of samples collected varied greatly between different trails as well as months (Table 1). In all, we collected 179 fecal samples of musk deer and 149 samples of goral.
Sex Assignment
The k-means conglomerate analysis for the variables T + P + E, T/P ratio and E/T ratio revealed that these samples belonged to two groups with significantly different T + P + E concentrations (Mann-Whitney-Wilcoxon Test, p < 0.000) for both the species. Group 1 comprised of samples with significantly lower T + P + E concentration and higher E/T ratio and assigned as females (Table 2). Group 2 had samples with high T + P + E concentration and low E/T ratio and were assigned as those of males (Oates et al., 2002; Bajra et al., 2011). We did not find any difference in the T/P ratio between the two groups of both the species. Based on the analysis, 161 fecal samples of musk deer and 111 samples of goral were assigned as females.

Table 2. Fecal concentrations (ng/g) (Mean ± SE) of T + P + E, T/P ratio and E/T ratio in musk deer and goral.
Reproductive Synchrony
We examined reproductive synchrony with reference to concentrations of fecal metabolites of estradiol and PdG in individual female samples as well as monthly means across all female samples. In musk deer, individual estradiol concentrations showed values above the threshold (mean + 2SD) between February and May representing cycling females (Figure 3A). The monthly mean concentrations of estradiol between January and May were also higher compared to other months clearly showing estrous (Figure 5). Individual as well as monthly mean PdG concentrations in musk deer were also higher than the threshold between January and March and also sporadically between August and October indicating dispersed pregnancies (Figures 3B, 5). However, the lowest concentrations of fecal PdG were in May and June, indicating parturition. Thus, both estradiol and PdG concentrations in individual samples and monthly means showed a dispersed estrous, gestation and parturition. In goral, individual estradiol concentrations above the threshold occurred in October on all trails reflecting synchronized estrous (Figure 4A). Although we did not have fecal samples for goral in March and April, the highest mean monthly concentration of estradiol was also in October (Figure 4). Individual PdG concentrations above threshold were in December, January, and February reflecting pregnancies (Figure 4B). The mean monthly PdG levels showed an increase from October indicating pregnancies, with a trough in May–June indicating parturition (Figure 5). Thus, the estradiol and PdG levels in goral show greater synchrony in estrous and parturition, respectively.
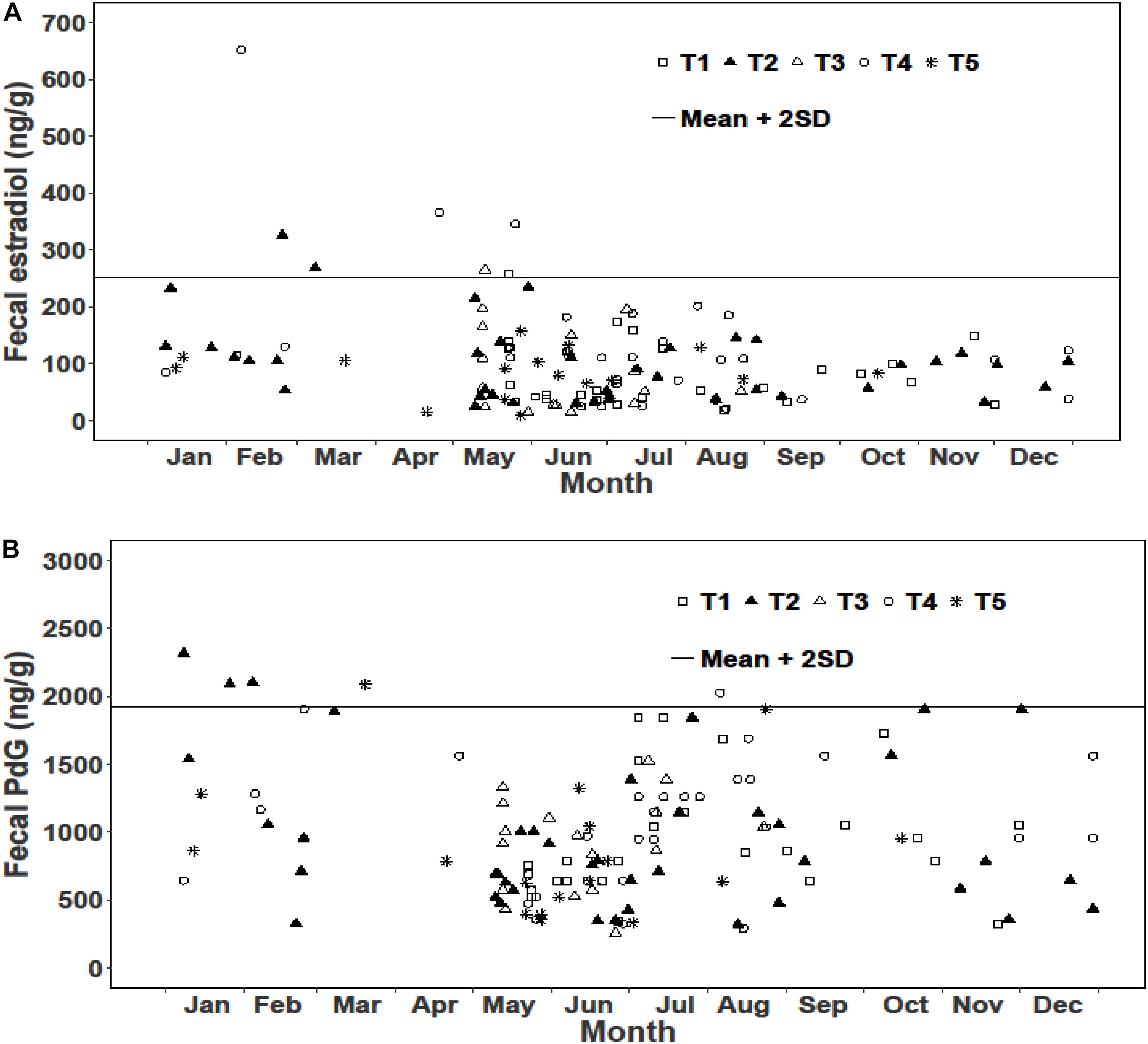
Figure 3. Fecal hormone metabolite concentrations in musk deer in various sampling trails (T1, T2, T3, T4, and T5) (A) Estradiol; (B) PdG.
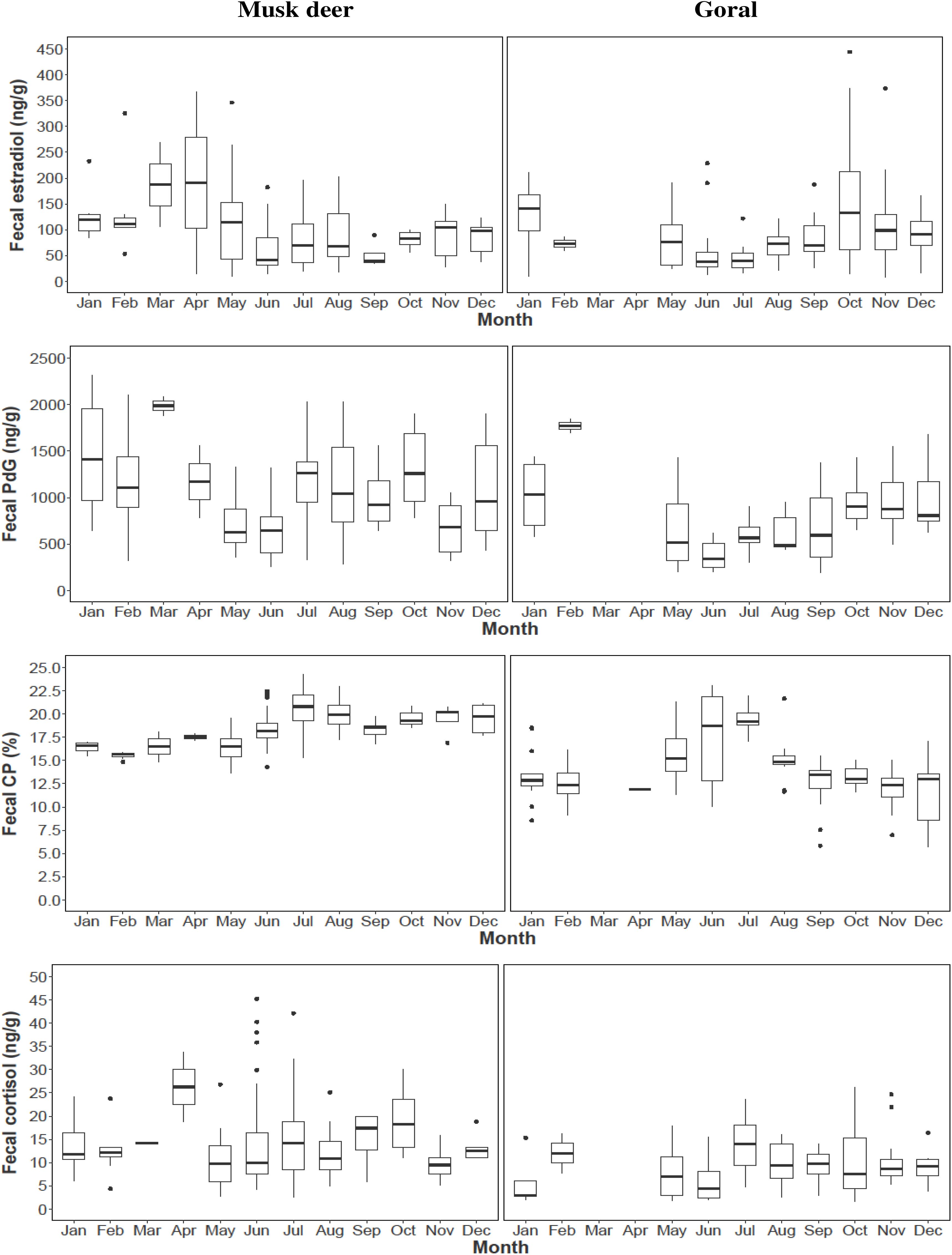
Figure 4. Fecal hormone metabolite concentrations in goral in different sampling trails (T5, T6, T7, and T8) (A) Estradiol; (B) PdG.
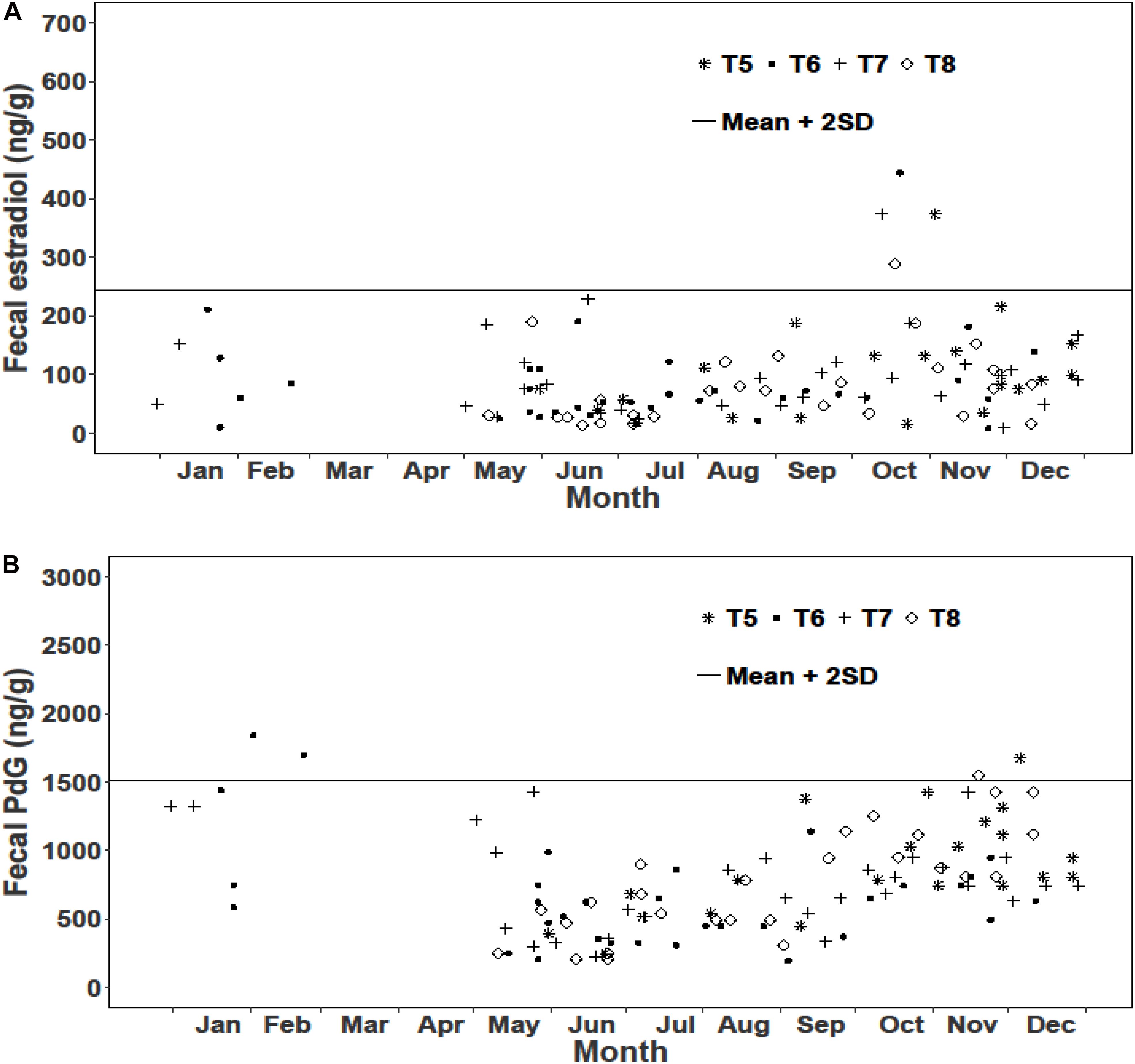
Figure 5. Monthly variations in fecal hormone metabolite concentrations and fecal CP in musk deer and goral. Box represents the interquartile range; horizontal line in the middle of the box is the median and the whiskers are the variability outside the upper and lower quartiles.
Diet Quality, Physiological Stress, and Daylength
In musk deer, diet quality, measured as fecal CP, was the lowest in January-April coinciding with estrous period (Figure 5). Fecal CP showed a sharp rise from May to July and remained high in the second half of the year coinciding with parturition and lactation. Monthly estradiol concentration showed a significant negative relationship with fecal CP (Table 3), indicating that the estrous cycles occurred when diet quality was poor. PdG concentration was not correlated with fecal CP. In goral, the fecal CP was lower than that in musk deer and showed a sharp increase from April to July, followed by an equally sharp decline reaching <15% in winter (Figure 5). The highest levels in fecal CP coincided with parturition and lactation as indicated by the lowest levels of PdG. Thus, in both species parturition and lactation coincided with high diet quality.
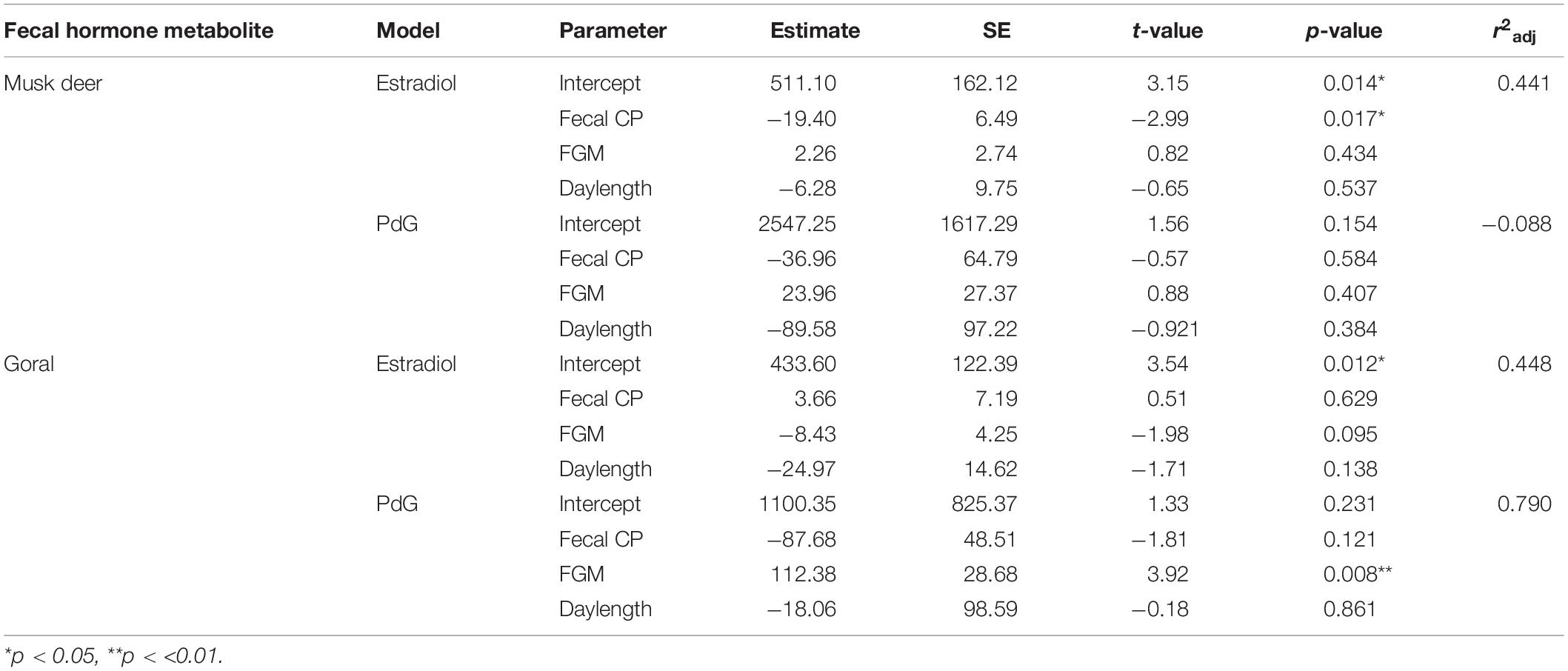
Table 3. Model parameter estimates and adjusted R-squared values (r2adj) of linear regression models used to fit fecal hormone metabolites (estradiol and PdG) of musk deer and goral against fecal CP, FGM and daylength.
In musk deer, FGM concentrations were higher in spring and summer months between April and July which is also the period of peak in fecal CP (Figure 5), however it was not correlated with fecal estradiol or PdG concentrations (Table 3). In goral, fecal estradiol concentration did not show a significant relationship with any of the predictor variables (Table 3). Fecal PdG, on the other hand, showed significantly positive relationship FGM (Table 3 and Figure 5), indicating that goral had gestation related stress which was not evident in musk deer. Daylength was not correlated with any of the hormonal metabolites in the both species, indicating a lack of its influence on reproductive phenology.
Discussion
To the best of our knowledge this is the first study to show differences in reproductive synchrony in relation to diet type and quality in sympatric ungulates. Previous studies have identified climate (Rutberg, 1987) and NDVI (English et al., 2012) as major drivers of birth season length in ungulates at a global scale, with diet type having no influence at that scale. In addition, we also examined the variation in physiological stress which in many female ungulates is related either to nutrition or reproduction.
Reproductive Synchrony
Because estrogen shows sharp peaks and troughs over a few days, compared to progesterone which shows high levels during luteal phase and pregnancy, it is easier to detect estrous using estradiol than to detect pregnancy using PdG. For example, in captive female Himalayan musk deer PdG levels started rising soon after conception, with a significant increase from the third month till parturition when it dropped sharply to baseline levels (Mithileshwari et al., 2016). High estradiol concentrations in musk deer from January to April indicate a diffused estrous season. While the elevated levels of PdG from January to May indicate gestation in the population and low levels in May and June indicate parturition, the elevated levels in the following months indicate gestation in females that were in estrous in April and May. Therefore, musk deer in the Himalayan subtropical forests seems to have a prolonged mating season from late winter to early spring, and an equally long birth season in summer and autumn. Similar patterns have also been reported by Qi et al. (2011) in captive forest musk deer (Moschus berezoski), where the mating season extended from October till early March and the fawning season from April till August.
In goral, peak estradiol concentrations in October on all the trails clearly show high estrous synchrony and a short mating season, as is also reported in both wild and captive Chinese goral (Lekagul and McNeely, 1988; Khonmee et al., 2014a). Rutting and mating activities in October have previously been observed in wild Himalayan goral (Lovari and Apollonio, 1994). Low concentrations of PdG in May and June indicate synchronized parturition. Unlike in musk deer, PdG concentration remained low in goral after May–June till the next estrous in October, indicating anestrous.
The dispersed estrous cycles and births in musk deer and the relatively high sychrony in goral correlate with the differences in their diet composition and quality. Partly overlapping habitats of musk deer and goral have similar biomass in graminoids, forbs and shrubs across seasons, with forbs forming about 70% of the biomass (Srivastava, 2019). However, in all months musk deer which fed primarily on dicots had higher fecal CP indicating better quality diet than goral which fed primarily on graminoids except in winter when it also fed on browse (Srivastava, 2019). Fecal CP remained high in musk deer in summer and autumn, during their long birth season. Fecal CP was the lowest during winter and early spring, coinciding with dispersed estrous. Thus fecal CP in musk deer was negatively correlated with estradiol concentration. In goral, there was a sharp increase in fecal CP from spring, peaking in May to July, which coincided with the lowest PdG levels indicating parturition during peak diet quality. Fecal CP levels remained <15% in late autumn and winter, during estrous and early gestation. In goral, a negative correlation between PdG and fecal CP, and the rise in fecal CP from April followed by probable parturition in May and June indicate that goral timed parturition to coincide with sharp increase in diet quality. Ungulates in seasonal environments are known to time parturition and lactation to capture the period of maximum forage quality and productivity (Sadlier, 1969; Parker et al., 2009; Kerby and Post, 2013a; Stoner et al., 2016). While this is true for both the species in this study, we also show that the duration of high diet quality can vary among sympatric ungulates depending on their primary diet type and this is reflected in the synchrony of reproduction in terms of duration of mating and birth seasons. This influence of diet type on reproductive synchrony, due to differences in phenology of plant growth forms, hypothesized by Rutberg (1987) would not be captured by global scale analyses that also include climate (Rutberg, 1987), latitude and NDVI (English et al., 1987).
This difference has implications on the impact of climate change on reproduction in these two species. A trophic mismatch between peak abundance of food resources and peak demand by consumers, often at breeding time, due to changes in plant phenology caused by climate change has been reported in many species (Thackeray et al., 2010). Income and capital breeders have been shown to respond differently to perturbations in resource abundance due to climate change. For example, reproductive performance in caribou, an income breeder, decreased due to trophic mismatch in the concurrent year, while that of the sympatric muskoxen (Ovibos moschatus), a capital breeder, was sensitive to resource conditions in the previous years (Kerby and Post, 2013b). Moreover, caribou was more likely to experience calf mortality while muskoxen skipped births. We found that musk deer was in the income breeder end of the spectrum, with relatively long estrous and birth seasons, and goral to be at the capital breeder end with much shorter estrous and birth seasons. By analogy with Kerby and Post (2013b), we would expect must deer to respond to concurrent perturbations in plant phenology due to climate change, and goral to respond to perturbations in previous years. These two species provide an ideal system to explore the differential impact that climate change can have on sympatric species.
Physiological Stress
In musk deer, neither fecal estradiol nor PdG was related to FGM concentrations, thus indicating that it did not exhibit stress related to reproduction or to low diet quality (Budithi et al., 2016; Tyagi et al., 2019). In goral, on the other hand, levels of fecal PdG and FGM were positively correlated indicating high stress co-inciding with gestation. Increased levels of stress hormones during late gestation or birthing period have been reported in pregnant barren-ground caribou (Joly et al., 2015), mandrills (Mandrillus sphinx) (Charpentier et al., 2018), sifaka (Propithecus verreauxi) (Brockman et al., 2009), and in red pandas (Ailurus fulgens) (Budithi et al., 2016).
Since the highest concentrations of FGM in both musk deer and goral were recorded in spring and summer when diet quality was also the highest, there might be other reasons for high stress levels. One is the presence of livestock during summer when forage quality was the highest (Srivastava, 2019). With yaks (Bos grunniens) grazing in the area, both species of ungulates might be facing some level of resource competition, when they have to meet their late gestation and lactation requirements. Second is the presence of a large population of feral dogs (Canis familiaris) in the village settlements and army camps just outside the sanctuary. From our camera trap data, we found that during spring and summer the dog capture rates were almost equal in both goral (13.95 ± 4.75 capture/100 days) and musk deer (14.26 ± 3.20 capture/100 days) habitats. More importantly, the capture rate of dogs increased in goral habitat (21.50 ± 7.27 capture/100 days) during winter coinciding with its gestation period and resulting in elevated stress levels as evident from the FGM concentrations. In musk deer habitat, dogs decreased during winter (2.69 ± 1.32 capture/100 days), mostly due to heavy snow cover in higher elevations. In our camera traps, we could record pack of dogs following gorals. During the study period, a few ungulate kills reportedly by feral dogs were also recorded (unpublished data). Feral dogs have recently been recognized as a major threat to wildlife, especially wild mammals, primarily due to predation (Doherty et al., 2017; Home et al., 2017). They are also known to increase stress levels not only by directly killing them, but also by harassing and chasing them (Lenth et al., 2008; Young et al., 2011). Stress levels in wild ungulates do not get elevated due to the presence of natural predators (Creel et al., 2009; Zbyryt et al., 2017), but anthropogenic factors could increase stress levels in ungulates due to unpredictable nature of occurrence (Zbyryt et al., 2017). The presence of predators can also cause disruption of reproductive cycles in ungulates, as has been reported in elk (Creel et al., 2009). These two hypotheses need to be tested in future.
Finally, the lack of a relationship between reproductive hormones and daylength in both the species, despite both being seasonal breeders, could be attributed to the location of the study area in the sub-tropical latitude where daylength might not be as important a factor in triggering reproductive stages as reported in higher northern latitudes (Owen-Smith and Ogutu, 2013).
Conclusion
Ungulates are expected to differ in synchrony of reproduction depending on phenology of the plant taxa that they primarily feed on. In this study we have shown that the Himalayan musk deer, a browser, has long estrous and birth seasons both of which are much shorter in the Himalayan goral, a grazer. This difference closely reflects seasonal changes in diet quality, that of goral showing a much sharper seasonal peak than that of musk deer. This in turn reflects seasonal phenology of the plant taxa that they primarily feed on. Previous studies at a global scale using proxies such as climate, latitude and NDVI have found no relationship between diet type and reproductive synchrony, since these proxies do not capture local scale differences in phenology among plant taxa. Our results imply that sympatric ungulates can differ in their response to climate change which influence plant taxa differently. We also found that physiological stress in the both the species was highest during spring and summer, when diet quality was also high, and not in winter when stress levels in ungulates in higher latitudes are highest. We hypothesize that grazing by domestic yak and harassment and predation by feral dogs are potential causes.
This study, however, had some limitations within which we have interpreted the results. We have used low T + P + E and high E/T ratio to separate fecal samples of females. While it is unlikely that we falsely included males, it is likely that this excluded some female samples. In spite of continuous monitoring of trails, fecal samples were not always found. Therefore, it is also likely that in some cases we have missed the peaks in estradiol as they last for a day or two. Finally, we could not identify individuals, therefore, samples from different sampling ocassions could have come from different individuals. This is especially true in the case of goral which lives in groups of three to five. In contrast, musk deer is solitary and territorial and therefore samples from a trail could be mostly of the same individual. Despite these limitations, this study provides information for the first time on seasonality of reproduction in these species in the wild and the timing of different stages with seasonal variations in diet quality. More importantly, our findings highlight the importance of studying the relationship among reproductive biology, diet quality and plant phenology at local scales in order to understand species differences in response to global phenomena such as climate change. We highlight the importance of studying whether livestock and feral dogs increase stress levels in these two species and disrupt their reproductive cycles, apart predation.
Data Availability Statement
The original contributions presented in the study are included in the article/supplementary material, further inquiries can be directed to the corresponding author/s.
Ethics Statement
Ethical review and approval was not required for the animal study because we collected the data non-invasively in protected areas and due permits for the same were procured as and when needed.
Author Contributions
TS and AK conceived the ideas and designed the study and its methods, performed data analyses, and wrote the manuscript. TS conducted the fieldwork. VK and TS conducted the laboratory analysis. TS, AK, GU, and VK interpreted the results and edited the manuscript to its final submitted draft. All authors contributed to the article and approved the submitted version.
Funding
This work was supported by the Department of Biotechnology (DBT), Government of India (Ref. BT/001/NE/PS/NCBS/09 to AK); the Council of Scientific and Industrial Research (CSIR), New Delhi, Government of India (Institutional support to GU and VK); and Rufford Small Grant Foundation, UK (Ref No. 19670-1 to TS).
Conflict of Interest
The authors declare that the research was conducted in the absence of any commercial or financial relationships that could be construed as a potential conflict of interest.
Acknowledgments
We thank Sonam and Kalu Singh Rai for their assistance in sample collection in the field; Sunita Khatiwara for assistance at different stages of the project; and the Department of Forests, Environment and Wildlife Management, Government of Sikkim, India for research permits.
References
Bajra, I., Silvan, G., Rosellini, S., Pineiro, A., Illera, M. J., and Illera, J. C. (2008). Quantification of sexual steroid hormones in faeces of Iberian wolf (Canis lupus signatus): a noninvasive sex typing method. Reprod. Domest. Anim. 43, 701–707. doi: 10.1111/j.1439-0531.2007.00974.x
Bajra, I., Silvan, G., Martinez-Fernandez, L., and Illera, J. C. (2011). Physiological stress responses, fecal marking behavior, and reproduction in wild European pine martens (Martes martes). J. Chem. Ecol. 37, 253–259. doi: 10.1007/s10886-011-9928-1
Barboza, P. S., Van Someren, L. L., Gustine, D. D., and Bret-Harte, M. S. (2018). The nitrogen window for arctic herbivores: plant phenology and protein gain of migratory caribou (Rangifer tarandus). Ecosphere 9, 1–17. doi: 10.1002/ecs2.2073
Brockman, D. K., Cobden, A. K., and Whitten, P. L. (2009). Birth season glucocorticoids are related to the presence of infants in sifaka (Propithecus verreauxi). Proc. R. Soc. B. 276, 1855–1863. doi: 10.1098/rspb.2008.1912
Bronson, F. H. (1989). Mammalian Reproductive Biology. Chicago, IL: The University of Chicago Press, 51–52.
Bronson, F. H. (2009). Climate change and seasonal reproduction in mammals. Philos. T. R. Soc. B 364, 3331–3340. doi: 10.1098/rstb.2009.0140
Brown, J. L., Walker, S. L., and Moeller, T. (2004). Comparative endocrinology of cycling and non-cycling Asian ((Elaphus maximus) and African (Loxodonta africana) elephants. Gen. and Comp. Endocr. 36, 360–370. doi: 10.1016/j.ygcen.2004.01.013
Budithi, N. R. B., Kumar, V., Yalla, S. K., Rai, U., and Umapathy, G. (2016). Non-invasive monitoring of reproductive and stress hormones in the endangered red panda (Ailurus fulgens fulgens). Anim. Reprod. Sci. 172, 173–181. doi: 10.1016/j.anireprosci.2016.07.016
Cain, S. L., Higgs, M. D., Roffe, T. J., Monfort, S. L., and Berger, J. (2012). Using fecal progestagens and logistic regression to enhance pregnancy detection in wild ungulates: a bison case study. Wildl. Soc. Bull. 36, 631–640. doi: 10.1002/wsb.178
Charpentier, M. J. E., Givalois, L., Faurie, C., Soghessa, O., Simon, F., and Kappeler, P. M. (2018). Seasonal glucocorticoid production correlates with a suite of small-magnitude environmental, demographic, and physiological effects in mandrills. Am. J. Phys. Anthrop. 165, 20–33. doi: 10.1002/ajpa.23329
Cho, C., Kim, K., and Kwon, G. (2015). Habitat altitude and home range of the endangered long-tailed goral (Naemorhedus caudatus): seasonal and monthly home range and altitude change. Mammalia 80, 481–489. doi: 10.1515/mammalia-2015-0024
Clutton-Brock, T. H., Albon, S. D., and Guinness, F. E. (1989). Fitness costs of gestation and lactation in wild mammals. Nature 337, 260–262.
Conception, B., Perez-Garnelo, S. S., Delclaux, M., Martinez, E., and Fuente, J. D. (2011). Fecal steroid evaluation to monitor reproductive status in wild ungulate females using enzyme immunoassay commercial kits. J. Zoo. Wildlife Med. 42, 537–551. doi: 10.1638/2009-0187.1
Creel, S., Winnie, J. A., and Christianson, D. (2009). Glucocorticoid stress hormones and the effect of predation risk on elk reproduction. PNAS 106:0902235106. doi: 10.1073/pnas.0902235106
Dloniak, S. M., French, J. A., Place, N. J., Weldele, M. L., Glickman, S. E., and Holekamp, K. E. (2004). Non-invasive monitoring of faecal androgens in spotted hyenas (Crocuta crocuta). Gen. Comp. Endocr. 135, 51–61. doi: 10.1016/j.ygcen.2003.08.011
Doherty, T. S., Dickman, C. R., Glen, A. S., Newsome, T. M., Nimmo, D. G., Ritchie, E. G., et al. (2017). The Global impacts of domestic dogs on threatened vertebrates. Biol. Conserv. 210, 56–59. doi: 10.1016/j.biocon.2017.04.007
Duckworth, J. W., and MacKinnon, J. (2008). IUCN Red List of Threatened Species. Naemorhedus. goral. 30:2018.
English, A. K., Chauvenet, A. L. M., Safi, K., and Pettorelli, N. (2012). Reassessing the determinants of breeding synchrony in ungulates. PLoS One 7:0041444. doi: 10.1371/journal.pone.0041444
Fox, J. L., and Smith, C. A. (1988). Winter mountain goat diets in Southeast Alaska. J. Wildlife Manage 52, 362–365. doi: 10.2307/3801249
Green, M. J. B. (1987a). Diet composition and quality in Himalayan musk deer based on fecal analysis. J. Wildlife Manage 51, 880–892. doi: 10.2307/3801755
Green, M. J. B. (1987b). “Some ecological aspects of a Himalayan population of musk deer,” in Biology and Management of the Cervidae, ed. C. M. Wemmer (Washington, D. C: Smithsonian Institution Press), 307–319.
Home, C., Vanak, A. T., and Bhatnagar, Y. V. (2017). Canine Conundrum: Domestic dogs as an invasive species and their impacts on wildlife in India. Anim. Conserv. 2017:12389. doi: 10.1111/acv.12389
Hosten, P. E., Whitridge, H., and Broyles, M. (2007). Diet overlap and social interactions among cattle, horses, deer and elk in the Cascade-Siskiyou National Monument, southwest Oregon. U.S. Department of the Interior, Bureau of land management, Medford district.
Huber, S., Palme, R., and Arnold, W. (2003). Effects of season, sex, and sample collection on concentrations of faecal cortisol metabolites in red deer (Cervus elaphus). Gen. Comp. Endocr. 130, 48–54. doi: 10.1016/s0016-6480(02)00535-x
Iversen, M., Brathen, K. A., Yoccoz, N. G., and Ims, R. A. (2009). Predictors of plant phenology in a diverse high-latitude alpine landscape: growth forms and topography. J. Veg. Sci. 20, 903–915. doi: 10.1111/j.1654-1103.2009.01088.x
Iversen, M., Fauchald, P., Langeland, K., Ims, R. A., Yoccoz, N. G., and Brathen, K. A. (2014). Phenology and cover of plant growth forms predict herbivore habitat selection in a high latitude ecosystem. PLoS One 9:01007780. doi: 10.1371/journal.pone.0100780
Joly, K., Wasser, S. K., and Booth, R. (2015). Non-invasive assessment of the interrelationships of diet, pregnancy rate, group composition and physiological and nutritional stress of Barren-ground caribou in late winter. PLoS One 10:0127586. doi: 10.1371/journal.pone.0127586
Jonsson, K. I. (1997). Capital and income breeding as alternative tactics of resource use in reproduction. Oikos 78, 57–66. doi: 10.2307/3545800
Kapke, C. A., Arcese, P., Ziegler, T. E., and Scheffler, G. R. (1999). Estradiol and progesterone metabolite concentration in white-tailed deer (Odocoileus virginianus) feces. J. Zoo. Wildlife Med. 30, 361–371.
Keay, J. M., Singh, J., Gaunt, M. C., and Kaur, T. S. (2006). Fecal glucocorticoids and their metabolites as indicators of stress in various mammalian species: A literature review. J Zoo Wildlife Med 37, 234–244. doi: 10.1638/05-050.1
Kerby, J., and Post, E. (2013a). “Reproductive phenology of large mammals,” in Phenology: an integrative environmental science, Second Edition, ed. M. D. Schwartz (Dordrecht: Springer), 467–479.
Kerby, J., and Post, E. (2013b). Capital and income breeding traits differentiate trophic match–mismatch dynamics in large herbivores. Phil. Trans. R. Soc. B 368:0484. doi: 10.1098/rstb.2012.0484
Khonmee, J., Brown, J. L., Taya, K., Rojanasthien, S., Punyapornwithaya, V., Thumasanukul, D., et al. (2014a). Assessment of ovarian activity in captive goral (Naemorhedus griseus) using noninvasive fecal steroid monitoring. Theriogenology 82, 997–1006. doi: 10.1016/j.theriogenology.2014.07.012
Khonmee, J., Brown, J. L., Rojanasthien, S., Thumasanukul, D., Kongphoemphun, A., and Siriaroonrat et al. (2014b). Seasonality of fecal androgen and glucocorticoid metabolite excretion in male goral (Naemorhedus griseus) in Thailand. Anim. Reprod. Sci. 146, 70–78. doi: 10.1016/j.anireprosci.2014.02.008
Kirkpatrick, J. F., Bancroft, K., and Kincy, V. (1992). Pregnancy and ovulation detection in bison (Bison bison) assessed by means of urinary and fecal steroids. J. Wildl. Dis. 28, 590–597. doi: 10.7589/0090-3558-28.4.590
Klein, D. R. (1990). Variation in quality of caribou and reindeer forage plants associated with season, plant part, and phenology. Rangifer 3, 123–130. doi: 10.7557/2.10.3.841
Kumar, V., Reddy, V. P., Kokkiligadda, A., Shivaji, S., and Umapathy, G. (2014). Non-invasive assessment of reproductive status and stress in captive Asian elephants in three south Indian zoos. Gen. Comp. Endocr. 201, 37–44. doi: 10.1016/j.ygcen.2014.03.024
Kumar, V., Pradheeps, M., Kokkiligadda, A., Niyogi, R., and Umapathy, G. (2019). Non-invasive assessment of physiological stress in captive Asian elephants. Animals 9:553. doi: 10.3390/ani9080553
Kumar, V., and Umapathy, G. (2019). Non-invasive monitoring of steroid hormones in wildlife for conservation and management of endangered species - A review. Indian J. Exp. Biol. 57, 307–314.
Leslie, D. M., Bowyer, R. T., and Jenks, J. A. (2008). Facts from feces: Nitrogen still measures up as a nutritional index for mammalian herbivores. J. Wildlife Manage. 72, 1420–1433.
Lenth, B., Knight, R., and Brennan, M. E. (2008). The effects of dogs on wildlife communities. Nat. Areas J. 28, 218–227. doi: 10.3375/0885-8608200828
Loe, L. E., Bonenfant, C., Mysterud, A., Gaillard, J. M., Langvatn, R., Klein, F., et al. (2005). Climate predictability and breeding phenology in red deer: timing and synchrony of rutting and calving in Norway and France. J. Anim. Ecol. 74, 579–588. doi: 10.1111/j.1365-2656.2005.00987.x
Lovari, S., and Apollonio, M. (1994). On the rutting behaviour of the Himalayan goral Naemorhedus goral (Hardwicke, 1825). J. Ethol. 12, 25–34. doi: 10.1007/BF02350077
Mithileshwari, C., Srivastava, T., Kumar, V., Kumar, A., and Umapathy, G. (2016). Non-invasive assessment of fecal progestagens and pregnancy detection in Himalayan musk deer (Moschus chrysogaster). Theriogenology 85, 216–233. doi: 10.1016/j.theriogenology.2015.09.009
Moyes, K., Nussey, D. H., Clements, M. N., Guinness, F. E., Morris, A., Morris, S., et al. (2011). Advancing breeding phenology in response to environmental change in a wild red deer population. Glob. Change Biol. 17, 2455–2469. doi: 10.1111/j.1365-2486.2010.02382.x
Munro, C. J., and Lasley, B. L. (1988). “Non-radiometric methods for immunoassay of steroid hormones,” in Non-radiometric assays: technology and application in polypeptide and steroid hormone detection, eds B. D. Albertson and F. P. Haseltine (New York, NY: Alan R. Liss Inc), 289–329.
Oates, J. E., Bradshaw, F. J., Bradshaw, S. D., and Lonsdale, R. A. (2002). Sex identification and evidence of gonadal activity in the short-beaked echidna (Tachyglossus aculeatus) (Monotremata: Tachyglossidae): non-invasive analysis of faecal sex steroids. Aust. J. Zool. 50, 395–406. doi: 10.1071/ZO02017
O’Connor, K. A., Brindle, E., Shofer, J., Trumble, B., Aranda, J. D., Rice, K., et al. (2011). The effects of a long-term psychosocial stress on reproductive indicators in the baboon. Am. J. Phys. Anthropol. 145, 629–638. doi: 10.1002/ajpa.21538
Oftedal, O. T. (1985). “Pregnancy and lactation,” in Bioenergetics of Wild Herbivores, eds R. J. Hudson and R. G. White (Boca Raton: CRC Press, Inc), 216–238.
Owen-Smith, N., and Ogutu, J. O. (2013). Controls over reproductive phenology among ungulates: allometry and tropical-temperate contrasts. Ecography 36, 256–263. doi: 10.1111/j.1600-0587.2012.00156.x
Parker, K. L., Barboza, P. S., and Stephenson, T. R. (2005). Protein conservation in female caribou (Rangifer tarandus): Effects of decreasing diet quality in winter. J. Mammal. 86, 610–622. doi: 10.1644/1545-1542200586[610:PCIFCR]2.0.CO;2
Parker, K. L., Barboza, P. S., and Gillingham, M. P. (2009). Nutrition integrates environmental responses of ungulates. Nutrit. Ecol. 23, 57–69. doi: 10.1111/j.1365-2435.2009.01528.x
Pettorelli, N., Gaillard, J.-M., Mysterud, A., Duncan, P., Stenseth, N. C., Delorme, D., et al. (2006). Using a proxy of plant productivity (NDVI) to find key periods for animal performance: the case of roe deer. Oikos 112, 565–572.
Plotka, E. D., Seal, U. S., Verme, L. J., and Ozoga, J. J. (1980). Reproductive steroids in deer. III. Luteinizing hormone, estradiol and progesterone around estrus. Biol. Reprod. 22, 576–581. doi: 10.1093/biolreprod/22.3.576
Post, E. (2003). “Timing of reproduction in large mammals,” in Phenology: An Integrative Environmental Science. Tasks for Vegetation Science, Vol. 39, ed. M. D. Schwartz (Dordrecht: Springer), doi: 10.1007/978-94-007-0632-3_27
Qi, W. H., Li, J., Zhang, X. Y., Wang, Z. K., Li, X. X., and Yang, C. Z. (2011). The reproductive performance of female Forest musk deer (Moschus berezovskii) in captivity. Theriogenology 76, 874–881. doi: 10.1016/j.theriogenology.2011.04.018
R Development Core Team (2013). A Language and Environment for Statistical Computing. Vienna: R Foundation for Statistical Computing.
Rutberg, A. T. (1987). Adaptive hypotheses of birth synchrony in ruminants: An interspecific test. Am. Nat. 130, 692–710.
Sadlier, R. M. F. S. (1969). The ecology of reproduction in domestic and wild mammals. London: Methuen.
Saltz, D., and White, G. C. (1991). Urinary cortisol and urea nitrogen responses to winter stress in mule deer. J. Wildlife Manage. 55, 1–16. doi: 10.2307/3809235
Schwartz, C. C., Monfort, S. L., Dennis, P. H., and Hundertmark, K. J. (1995). Fecal progestogen concentration as an indicator of the estrous cycle and pregnancy in moose. J. Wildlife Manage. 59, 580–583. doi: 10.2307/3802466
Shivaji, S., Kholkute, S. D., Verma, S. K., Gaur, A., Umapathy, G., Singh, A., et al. (2003). Conservation of wild animals by assisted reproduction and molecular marker technology. Indian J. Exp. Biol. 41, 710–723.
Spinage, C. A. (1973). The role of photoperiodism in the seasonal breeding of tropical African ungulates. Mammal Rev. 3, 71–83. doi: 10.1111/j.1365-2907.1973.tb00174.x
Srivastava, T. (2019). Ecology of feeding and reproduction in mid-altitude wild ungulates in Sikkim Himalaya. Manipal: Manipal University, 1–178. PhD thesis.
Srivastava, T., and Kumar, A. (2018). Seasonal habitat use in three species of wild ungulates in Sikkim Himalaya. Mamm. Biol. 88, 100–106.
Stephens, P. A., Boyd, I. L., McNamara, J. M., and Houston, A. I. (2009). Capital breeding and income breeding: their meaning, measurement and worth. Ecology 90, 2057–2067. doi: 10.1890/08-1369.1
Stoner, D. C., Sexton, J. O., Nagol, J., Bernales, H. H., and Edwards, T. C. Jr. (2016). Ungulate reproductive parameters track satellite observations of plant phenology across latitude and climatological regimes. PLoS One 112:e0148780. doi: 10.1371/journal.pone.0148780
Suryawanshi, K. R., Bhatnagar, Y. V., and Mishra, C. (2010). Why should a grazer browse? Livestock impact on winter resource use by bharal Pseudois nayaur. Oecologia 162, 453–462. doi: 10.1007/s00442-009-1467-x
Thackeray, S. J., Sparks, T. H., Frederiksen, M., Burthe, S., Bacon, P. J., Bell, J. R., et al. (2010). Trophic level asynchrony in rates of phenological change for marine, freshwater and terrestrial environments. Glob. Change Biol. 16, 3304–3313. doi: 10.1111/j.1365-2486.2010.02165.x
Thompson, I. D., Wiebe, P. A., Mallon, E., Rodgers, A. R., Fryxell, J. M., Baker, J. A., et al. (2015). Factors influencing the seasonal diet selection by woodland caribou (Rangifer tarandus tarandus) in boreal forests in Ontario. Can. J. Zool. 93, 87–98. doi: 10.1139/cjz-2014-0140
Tyagi, A., Kumar, V., Kittur, S., Reddy, M., Naidenko, S., Ganswindt, A., et al. (2019). Physiological stress responses of tigers due to anthropogenic disturbance especially tourism in two central Indian tiger reserves. Conserv. Physiol. 7:45. doi: 10.1093/conphys/coz045
Umapathy, G., Kumar, V., Kabra, M., and Shivaji, S. (2013). Detection of pregnancy and fertility status in big cats using an enzyme immunoassay based on 5α-pregnan-3α-ol-20-one. Gen. Comp. Endocr. 180, 33–38. doi: 10.1016/j.ygcen.2012.10.009
Umapathy, G., Deepak, V., Kumar, V., Mithileshwari, C., and Vasudevan, K. (2015). Endocrine profiling of endangered tropical Chelonians using noninvasive faecal steroid analyses. Chelonian Conserv. Biol. 14, 108–115. doi: 10.2744/ccab-14-01-108-115.1
White, T. C. R. (1993). The inadequate environment. Nitrogen and the abundance of animals. Berlin: Springer-Verlag.
Young, J. K., Olson, K. A., Reading, R. P., Amgalanbaatar, S., and Berger, J. (2011). Is wildlife going to the dogs? Impacts of feral and free-roaming dogs on wildlife populations. BioScience 61, 125–132. doi: 10.1525/bio.2011.61.2.7
Young, K. M., Walker, S. L., Lanthier, C., Waddell, W. T., Monfort, S. L., and Brown, J. L. (2004). Noninvasive monitoring of adrenocortical activity in carnivores by fecal glucocorticoid analyses. Gen. Comp. Endocr. 137, 148–165. doi: 10.1016/j.ygcen.2004.02.016
Zaitsev, V. A., Seryodkin, I. V., Maksimova, D. A., and Soutyrina, S. V. (2015). Study of the musk deer population structure in Sikhote-Alin Reserve. Achiev. Life Sci. 9, 83–86. doi: 10.1016/j.als.2015.11.001
Zerbe, P., Clauss, M., Codron, D., Lackey, L. B., Rensch, E., Streich, J. W., et al. (2012). Reproductive seasonality in captive wild ruminants: implications for biogeographical adaptation, photoperiodic control, and life history. Biol. Rev. 87, 965–990. doi: 10.1111/j.1469-185X.2012.00238.x
Keywords: Himalayan goral, Himalayan musk deer, reproduction, reproductive stages, fecal hormones, diet, forage quality, stress
Citation: Srivastava T, Kumar A, Kumar V and Umapathy G (2021) Diet Drives Differences in Reproductive Synchrony in Two Sympatric Mountain Ungulates in the Himalaya. Front. Ecol. Evol. 9:647465. doi: 10.3389/fevo.2021.647465
Received: 29 December 2020; Accepted: 11 May 2021;
Published: 17 June 2021.
Edited by:
John Cockrem, Massey University, New ZealandReviewed by:
Jaruwan Khonmee, Chiang Mai University, ThailandEdward Narayan, The University of Queensland, Australia
Sanjeeta Pokharel, Smithsonian Conservation Biology Institute (SI), United States
Copyright © 2021 Srivastava, Kumar, Kumar and Umapathy. This is an open-access article distributed under the terms of the Creative Commons Attribution License (CC BY). The use, distribution or reproduction in other forums is permitted, provided the original author(s) and the copyright owner(s) are credited and that the original publication in this journal is cited, in accordance with accepted academic practice. No use, distribution or reproduction is permitted which does not comply with these terms.
*Correspondence: Tanushree Srivastava, tanushree.wildlife@gmail.com
†Present address: Tanushree Srivastava, Laboratory for the Conservation of Endangered Species (LaCONES), CSIR-Centre for Cellular and Molecular Biology, Hyderabad, India