Determining Timing of Births and Habitat Selection to Identify Lambing Period Habitat for Bighorn Sheep
- 1Department of Plant and Wildlife Sciences, Brigham Young University, Provo, UT, United States
- 2Department of Biology, Brigham Young University-Idaho, Rexburg, ID, United States
- 3Utah Division of Wildlife Resources, Salt Lake City, UT, United States
Timing of births in ungulates is influenced by ecological factors, and differences in seasonality of births have evolutionary implications for these mammals. Birthing habitat is one of the most important home-range attributes for ungulates, and disturbances during this time can decrease survival of young and population growth. We calculated timing of births and quantified habitat selected by nursery groups (i.e., females with young) of desert bighorn sheep (Ovis canadensis nelsoni) to produce and project a geographic information system (GIS) model of lambing period habitat (i.e., birthing locations and areas used up to 6 weeks post-parturition) in southeastern Utah, United States. We then applied that model to identify suitable lambing period habitat in an adjacent area for a population of Rocky Mountain bighorn sheep (O. c. canadensis). We monitored 19 global positioning system (GPS) collared females from 2012 to 2013 to delineate the birthing season. We used GPS locations during that time and logistic regression within a model-selection framework to differentiate between lambing period habitat and random locations based on habitat and anthropogenic covariates. We used model coefficients to produce and project a GIS model of lambing period habitat. Across both years, 89% of 45 births occurred in May (range = April 27–June 9). We quantified covariate values at 750 lambing period and 750 random locations during that time in the San Rafael study area. The top model included elevation, slope, terrain ruggedness, aspect, existing vegetation type, and distance to trails and roads. Those variables predicted lambing period habitat for desert bighorn sheep (rho = 0.99, P = 0.02). We then overlaid that model on 1,130 GPS locations from 17 female Rocky Mountain bighorns in the Green River study area. In both areas, a mean of 71% of locations were in the top two (of five) probability categories (San Rafael high = 37%, medium high = 31%; Green River high = 50%, medium high = 23%). Females in lambing period habitat preferred steep, north-facing slopes, rugged terrain, low elevation, and avoided roads. Our GIS model projected areas of lambing period habitat for adjacent desert and Rocky Mountain bighorns and provided land managers with a map of habitat in areas where resource extraction and recreation are increasing. Identifying timing of parturition, and high-probability lambing period habitat, will help managers mitigate temporal and spatial conflicts between bighorn sheep and anthropogenic activities. Similar data regarding timing of births and a GIS model can be applied to conserve habitat for other ungulates also.
Introduction
Timing of births in ungulates is influenced by many ecological factors (Sadleir, 1969; Bowyer, 1991; Loe et al., 2005), and differences in seasonality of births for these mammals can have evolutionary implications (Marshall and Cambridge, 1937; Rutberg, 1987; Ims, 1990), such as reduced survival of young and future reproductive potential (Clutton-Brock et al., 1987; Festa-Bianchet et al., 2000; Keech et al., 2000). For bighorn sheep (Ovis canadensis), latitude, elevation, growing season length, climate, nutrition, and photoperiod are factors influencing reproductive seasonality (Bunnell, 1982; Thompson and Turner, 1982; Whiting et al., 2012). Generally, bighorn sheep occupying northern latitudes and higher elevations give birth late in spring, because of a constricted birthing period and shortened growing season (Bunnell, 1982; Thompson and Turner, 1982). Conversely, bighorn sheep occupying southern latitudes can give birth during most months, likely because growing seasons are much less predictable (Lenarz, 1979; Thompson and Turner, 1982; Rubin et al., 2000). Understanding the timing of births for ungulates can guide conserving habitat by mitigating temporal conflicts between these mammals and anthropogenic activities (e.g., recreation and mining) during that time (Singh et al., 2010; Kaze et al., 2016).
Female ungulates select birth sites and habitat for nursery groups (i.e., females with young) based on a variety of trade-offs (Bowyer, 1991; Rachlow and Bowyer, 1991, 1994). These trade-offs include predation risk, exposure to extreme weather, and forage quality and availability for the mother to fulfill nutritional requirements during late gestation and lactation (Festa-Bianchet, 1988b; Berger, 1991; Severud et al., 2019). For example, female bighorn sheep often return to the same general area each year to give birth (Geist, 1971; Etchberger and Krausman, 1999; Whiting et al., 2012). Young are usually born in relatively flat areas of high elevation in rugged, steep terrain close to perennial water, on south and west facing slopes, and away from anthropogenic disturbance (Geist, 1971; Bangs et al., 2005; Smith et al., 2015). After giving birth, female bighorns generally form nursery groups with other females and young in rugged areas of high elevation and steep slopes (Geist, 1971; Bangs et al., 2005; Karsch et al., 2016). These groups then move cohesively among patches of nursery habitat for the subsequent months (Bangs et al., 2005; Whiting et al., 2012; Wiedmann and Bleich, 2014). In general, Rocky Mountain bighorn sheep (O. c. canadensis) give birth later in spring, and the birthing period is constricted allowing females to exploit the shortened growing season during summer (Bunnell, 1982; Thompson and Turner, 1982). Conversely, desert bighorn sheep (O. c. nelsoni) give birth in almost any month, ostensibly because of a less predictable growing season (Bunnell, 1982; Thompson and Turner, 1982; Rubin et al., 2000). Delineation of birthing habitat has important implications for the conservation, reproductive biology, and perpetuation of bighorn populations (Etchberger and Krausman, 1999; Wiedmann and Sargeant, 2014; Smith et al., 2015), and is a topic that is receiving increased attention for land-use planning (Severud et al., 2019).
Anthropogenic activities can influence habitat selection by female ungulates during birthing and when animals congregate in nursery groups (Stankowich, 2008; Dzialak et al., 2011; Kaze et al., 2016), which can reduce forage intake and suppress population growth (Ciuti et al., 2012b; Sproat et al., 2019). For example, Saiga antelope (Saiga tatarica) avoided areas of human use while selecting birthing sites (Singh et al., 2010). Parturient bison (Bison bison) selected birthing areas that were away from recreational trails, roads, and buildings (Kaze et al., 2016). In an area that was intentionally disturbed by humans during parturition, counts of young/female elk (Cervus canadensis) were 22.5% lower than in areas with undisturbed herds (Phillips and Alldredge, 2000). Populations of bighorn sheep can be negatively influenced by anthropogenic disturbances, especially during the birthing season (Papouchis et al., 2001; Wiedmann and Bleich, 2014; Smith et al., 2015). These disturbances can cause females to abandon previously used nursery habitat. Thereafter, females use less-suitable habitat (Longshore et al., 2013), which may increase predation risk for lambs (Papouchis et al., 2001), and lower recruitment of young, resulting in a declining population (Papouchis et al., 2001; Wiedmann and Bleich, 2014). Delineating birthing habitat and habitat for nursery groups of ungulates can reduce spatial conflict between these mammals and anthropogenic activities (Kaze et al., 2016; McLaren et al., 2017).
Conservation and land-use planning will increasingly rely on identifying preferred wildlife habitats in areas of varying levels of human use (Margules and Pressey, 2000; Dzialak et al., 2011; Harju et al., 2011). Biologists can reduce and mitigate disturbance from anthropogenic activities to female ungulates during birthing by identifying when and where animals give birth (Dzialak et al., 2011; Kaze et al., 2016; McLaren et al., 2017). Determining timing of parturition in ungulates to identify high probability nursery areas can help managers mitigate temporal and spatial conflicts (Kaze et al., 2016; McLaren et al., 2017), and guide habitat management (Severud et al., 2019). Global positioning system (GPS) data can effectively document such patterns at fine scales (Longshore et al., 2013), especially for parturient females (Smith et al., 2015; McLaren et al., 2017; Severud et al., 2019). We calculated timing of births to delineate habitat for nursery groups of GPS-collared desert bighorn sheep and Rocky Mountain bighorn sheep in two of the largest populations of those subspecies in Utah, United States. We used GPS locations during that time and logistic regression within a model-selection framework to differentiate between lambing period habitat (i.e., birthing locations and areas used up to 6 weeks post-parturition; Zeigenfuss et al., 2000) and random locations based on habitat and anthropogenic covariates. We then constructed a GIS model that projected lambing period habitat in both areas. That model provided biologists and managers with a map of high probability lambing period habitat. By identifying timing of parturition, and high-probability lambing period habitat, managers will be able to mitigate temporal and spatial conflicts between bighorn sheep and recreation, mining, and domestic livestock grazing. Data regarding timing of births and a similar GIS model can be applied to manage and conserve habitat for ungulate species in other areas as well.
MATERIALS AND METHODS
Study Areas
The population of desert bighorn sheep we studied occupied the North San Rafael Swell (hereafter, San Rafael). The San Rafael is located in Emery County, Utah (Figure 1; 38°58′N, 110°37′W). Bighorns were native and abundant in the San Rafael, but were likely extirpated from that area; the last confirmed sighting occurred in 1964 (Dalton and Spillett, 1971). That population was re-established in the 1970s and 1980s with five translocations of 57 bighorns from Canyonlands National Park and the San Juan Population, Utah (Utah Division of Wildlife Resources [UDWR], 2018). Those translocation efforts were successful, and in 2001 the UDWR estimated 543 animals in that population. However, bighorn sheep started to decline in the 2000s, and 1 month prior to our study, biologists estimated 143 individuals in that area.
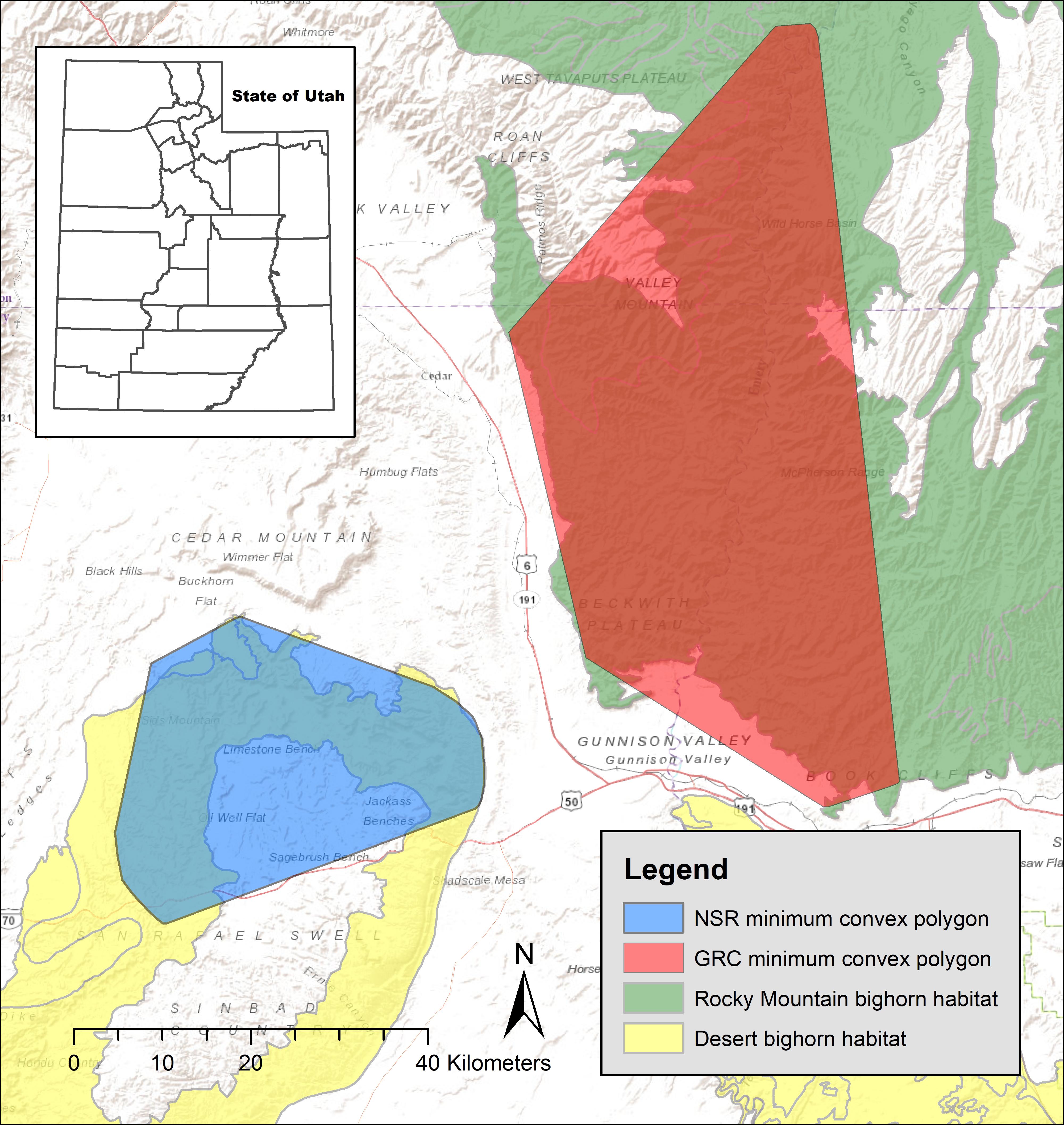
Figure 1. Study areas where we documented lambing period habitat of 36 GPS collared bighorn sheep in eastern Utah, United States, 2012–2013. GRC, Green River Corridor; NSR, North San Rafael Swell.
The San Rafael is characterized by steep canyons in the Wingate Formation with broad mesa tops in Navajo and Entrada Sandstone formations (Rigby and Beus, 1987). Desert bighorn sheep habitat in that area ranged in elevation from 1,700 to 2,100 m. Vegetation consisted of species typical of salt desert shrub environments. Common shrubs included blackbrush (Coleogyne ramosissima) and fourwing saltbush (Atriplex canescens). Pinyon pine (Pinus edulis) and juniper (Juniperus spp.) were predominate on mesa tops and on north facing canyon slopes. The San Rafael is dry with annual precipitation averaging <20 cm per year. Daily high temperatures during summer (June–September) averaged 31°C and often exceeded 35°C. Winters (November–February) were typified with daily low temperatures averaging −12°C. Native populations of pronghorn (Antilocapra americana) and mule deer (Odocoileus hemionus) inhabited the study area, although mule deer persisted at low densities. Wild burros (Equus asinus) and domestic cattle also occupied portions of the San Rafael. Mammalian predators included mountain lions (Puma concolor), bobcat (Lynx rufus), and coyotes (Canis latrans). The San Rafael study area was predominantly managed by the Bureau of Land Management (BLM). Recreation in the San Rafael included on and off-road travel, rock climbing, biking, hiking, camping, and hunting. Peak recreation occurred in spring during mild weather (Wade Paskett, pers. comm., UDWR).
We also studied a population of Rocky Mountain bighorn sheep in the Green River corridor (hereafter, Green River) located in Emery and Carbon counties, Utah (Figure 1). Bighorns were native to that area, but were extirpated by 1960 (Dalton and Spillett, 1971). Currently, bighorns in that area are a result of nine transplants beginning in 1970 with animals from Utah, Montana, Wyoming, and Colorado, United States and Alberta and British Columbia, Canada. Rocky Mountain bighorn in Green River form the largest metapopulation of this subspecies in Utah (Figure 1), and that area contains one of the largest contiguous sections of Rocky Mountain bighorn sheep habitat in Utah. An estimated 1,000 individuals occupied that area at the beginning of our study.
Elevation used by bighorn sheep in the Green River varied from 1,250 to 3,000 m. Riparian areas in that area were dominated by willow (Salix spp.), cottonwood (Populus fremontii), and box elder (Acer negundo) trees. General vegetation of the Green River was a desert environment dominated by salt shrubs, bunch grasses, pinyon, and juniper. Populations of pronghorn, mule deer, bison, elk, and domestic cattle occupied that area; and mammalian predators were the same as those that occurred in San Rafael, except the Green River has a large black bear (Ursus americanus) population. The Green River is of mixed land ownership, most of which is managed by the BLM. Recreation in the Green River included on and off-road travel, rock climbing, biking, hiking, camping, fishing, and hunting. The Green River corridor is also governed by the 1979 River Management Plan (Barry, 1979), which allows for private and commercial float permits and establishes a carrying capacity of 35,000 user days per year. The recreation season is divided into high and low use periods. High use occurred from May 15 through August 15. Total user days on the river have increased by 43% from 2003 to 2018 (Jaydon Mead, pers. comm., US BLM). Despite our two study populations being separated by <20 km, no genetic mixing has been documented between these populations.
Methods
In January 2012, 30 adult, female bighorn sheep were captured by aerial net gunning (Krausman et al., 1985) in the San Rafael, and 17 adult females were captured in the Green River. Efforts were made to sample a wide distribution of bighorns across the units by distributing collars proportionally to aerial count numbers and locations of bighorns established 2 months prior. Animals were equipped with Lotek 6000SD GPS/VHF collars (Lotek Wireless Inc., Ontario, Canada) with mortality transmitters and pre-programmable drop off mechanisms. Collars deployed in the San Rafael were programmed to acquire a GPS fix every 8 h, and collars deployed in the Green River were programmed to acquire a GPS fix on either a 2.5, 4, or 6 h schedule. In January 2013, eight additional females in the San Rafael and five in the Green River were captured and collared to replace mortalities, bringing the total number of collared females to 38 in the San Rafael and 22 in the Green River. Due to collar malfunctions and mortalities, we used data from 36 GPS collars (San Rafael = 19 and Green River = 17) for our GIS analyses; however, all females in the San Rafael with active collars at the time of lambing were observed for collection of data for parturition timing. We retrieved collars after mortalities or at the end of the study in January 2014, and GPS locations were uploaded from the onboard data storage of the collars. Wildlife biologists from the UDWR used care in capturing, handling, and attaching GPS collars to bighorns (Sikes et al., 2016). Using GPS data is an extremely effective way to document parturition sites in bighorn sheep, and these data should be used over other sources (i.e., VHF collars) when delineating critical birthing habitat for this species (Smith et al., 2015), especially when considering anthropogenic influences on habitat use (Longshore et al., 2013).
To determine timing of births, we monitored collared bighorn sheep in the San Rafael weekly using radio telemetry for 2 years until January 2014. We relocated collared and uncollared females with binoculars and spotting scopes to record birthdates from 25 April to 25 June during 2012 and 2013. We searched the San Rafael a mean (±SD) of every 2 days ± 1.6 days in 2012, and a mean of every 2 days ± 1.7 days in 2013. To estimate parturition dates, we observed the behavior of marked females before, during, and after parturition; as well as first sighting, motor skills, size, and behavior of neonates (Festa-Bianchet, 1988a; Whiting et al., 2008, 2011). To determine birthdates for neonates of uncollared females, we compared their young with neonates of estimated ages of collared females when all females congregated in nursery groups after parturition (Côté and Festa-Bianchet, 2001; Whiting et al., 2008, 2012). When female and lamb pairings were questionable, we waited until the lamb nursed to identify its mother (Festa-Bianchet, 1988a). We exercised care not to disturb females with young (Sikes et al., 2016).
We estimated birthdates of young, pooled them into sampling intervals and calculated corrected means (timing of births) and SD values (synchrony of births) for the San Rafael population in each year (Johnson et al., 2004; Whiting et al., 2011). This technique allowed robust calculations of unequal sampling intervals (bin sizes) in determining timing and synchrony of births (Johnson et al., 2004). We then calculated a date range using ±2 SDs from the mean for lambing period habitat for our spatial analyses using data from GPS collars in the San Rafael population. A mean of 88 (±3.6) adult females occupied the San Rafael during our study.
We did not relocate collared and uncollared females with binoculars and spotting scopes in the Green River during our study. The general estimate of the peak birthing period, however, for that population was from 25 May to 5 June (Whiting et al., 2011). We therefore used the date range of May 1–June 10 for our analyses of lambing period habitat in that population. A mean (±SD) of 462 (±69.3) adult females occupied the Green River during our study.
Resource Selection Functions
Using the appropriate spatial scale when defining habitat availability to animals is critical when making inferences about habitat selection at the population level, making it important to define an area biologically relevant to the species of interest (Johnson, 1980; Boyce, 2006). We used the reproducible home range (rhr) package in Program R 3.1 to delineate a 95% minimum convex polygon for each study area (Figure 1). We created those polygons using bighorn birthing and lambing period locations during the date range calculated for both study populations.
We evaluated bighorn lambing period habitat selection in those 95% minimum convex polygons using a resource selection function within a use-availability study design (Manly et al., 2002) where the response variable was 1 for a use location or 0 for a random site. Random points were extracted with the random points tool in ArcMap 10.3 (Esri, Redlands, CA, United States) and R (R Core Team, 2015). We used a mixed-effects, logistic regression with a random intercept for individual bighorn (radio collar ID) and analyzed covariates at use versus random locations. We evaluated lambing period habitat using locations only from the San Rafael study area. We then produced a model from those variables and applied it to the Green River to verify if our GIS model captured lambing period habitat for that population.
We performed a database query in ArcGIS 10.3 (Redlands, CA, United States) to eliminate locations with <3D accuracy for both study areas. Because GPS collars across both study areas were programmed at different sampling intervals, we used only one randomly selected location per collar per day both for resource selection function analysis (San Rafael) and model testing (Green River). After removing <3D locations and locations that occurred outside the 95% minimum convex polygon, use locations totaled 750 for the San Rafael, and testing locations totaled 1,130 for the Green River. We generated 750 random locations within the San Rafael to be equal to the number of use locations and assigned them equal weight. Because random locations were cast within the boundary of the study area and not associated with individual home ranges, our modeling of resource selection generally corresponded to Johnson’s second order of selection (Johnson, 1980). To ensure that 750 random locations adequately characterized our study area, we calculated the true mean values (i.e., mean of all pixels within the study area) for continuous variables and compared our sample means with 95% CIs to those values (Long et al., 2014). In every case, the confidence intervals of our samples overlapped the true mean values indicating that the 750 random locations were adequate to characterize our study area.
We selected the following landscape level features potentially influencing bighorn sheep habitat selection: slope, ruggedness, elevation, aspect, Landfire existing vegetation type (LANDFIRE1) (Smith et al., 1991; Bleich et al., 1997; Sappington et al., 2007; Rollins, 2009), and distance to trails and roads (Smith et al., 1991; Longshore et al., 2013; Wiedmann and Bleich, 2014). We extracted topographic features using a United States Geological Survey 30 m digital elevation model. We calculated slope using the slope tool in the Spatial Analyst Tools extension. We calculated ruggedness using the Vector Ruggedness Measure Tool in the Terrain Tools extension in ArcGIS (Sappington et al., 2007; Lowrey and Longshore, 2017). That tool measures terrain ruggedness as the variation in three-dimensional orientation of grid cells within a neighborhood. Vector Ruggedness Measure values can range from 0 (no terrain variation) to 1 (complete terrain variation). We calculated aspect using the aspect tool in the Spatial Analyst Tools extension and that variable was divided into the four cardinal directions (north, east, south, west). The LANDFIRE existing vegetation type layer consisted of five types (barren, sparse, herb, shrub, and tree). We calculated distance to roads and trails using the Generate Near Table Tool in the Analysis Tools extension. Distance to water sources was not included in our analysis. Because our study occurred in spring and due to the extensive nature of ephemeral water sources formed in holes in sandstone rock after rain or from seasonal seeps, it was not feasible to map water source locations accurately or comprehensively. Additionally, major rivers were in each study area. We evaluated explanatory variables for multi-collinearity and did not include any variables with a correlation coefficient |r| > 0.6.
We developed models using an information theoretic approach (Burnham and Anderson, 2002) within a mixed-effects logistic regression (Hosmer et al., 2013) and used a random intercept to account for individual heterogeneity. We used R package lme4 for mixed-effect modeling analysis (Bates et al., 2013). We used combinations of covariates (all permutations) to build models. All continuous covariates (slope, ruggedness, elevation, distance to trails, and distance to roads) were standardized before model development ( (Baxter et al., 2017). We used AICc values to identify the most supported models, and to identify which variables were informative (Burnham and Anderson, 2002; Arnold, 2010). We evaluated models based on AICc, ΔAICc values, and AICc model weight.
To assess predictive ability of our top model, we performed k-folds cross validation with k = 5 (Long et al., 2009; Villepique et al., 2015; Kaze et al., 2016) in the San Rafael. We randomly sorted observations into five partitions, with an equal number of locations in each partition. During each iteration of this procedure, we used four partitions (80% of the data) as the training set to estimate model coefficients and the remaining partition (20% of the data) to test model predictions. We repeated this procedure until all observations were used for the test set and part of the training set. We then applied the coefficients from the predictive model to calculate relative probability of use in the San Rafael and Green River study areas. We generated two predictive maps, one for the San Rafael and one for the Green River, by applying this procedure to each raster pixel in each study area. We then used five equal-area bins to categorize the relative probabilities of use for each pixel from low to high (Sawyer et al., 2007; Kaze et al., 2016).
Results
In 2012, we estimated birthdates for 29 young. Mean (±2 SDs) birthdate for bighorn sheep in the San Rafael during that year was 21 May (±19 days). In 2013, we estimated birthdates for 16 young, and mean birthdate for bighorn sheep in that area during that year was 20 May (±21 days). In both years, 89% of 45 births occurred in May (range = April 27–June 9). The date range we used for our analyses of lambing period habitat from GPS collar data for 2012 was 2 May–9 June. Whereas, for 2013 that range of dates was 29 April–10 June.
Our habitat analyses resulted in two top models that accounted for 99% of the AICc weight (Table 1). Because the top model accounted for 86% of AICc weight and included all covariates from the second model, we did not need to model average and only report parameter estimates from the top model (Table 2). That model included measures of elevation, slope, ruggedness, aspect, existing vegetation type, distance to trails, and distance to roads. Estimates for variables with evidence of selection (p < 0.05) were positive for barren vegetation, slope, ruggedness, north facing slopes, and distance to roads (indicating a preference for areas farther from roads). Estimates for variables with significant negative correlation were elevation (indicating a preference for lower elevations), south facing slopes, herb, shrub, and tree vegetation types, and distance to trails (indicating a preference for areas closer to trails).

Table 1. Model results (≥0.01 model weight) for habitat selection by 19 desert bighorn sheep females during lambing period in the North San Rafael Swell, Utah, United States, from 2012 to 2013.
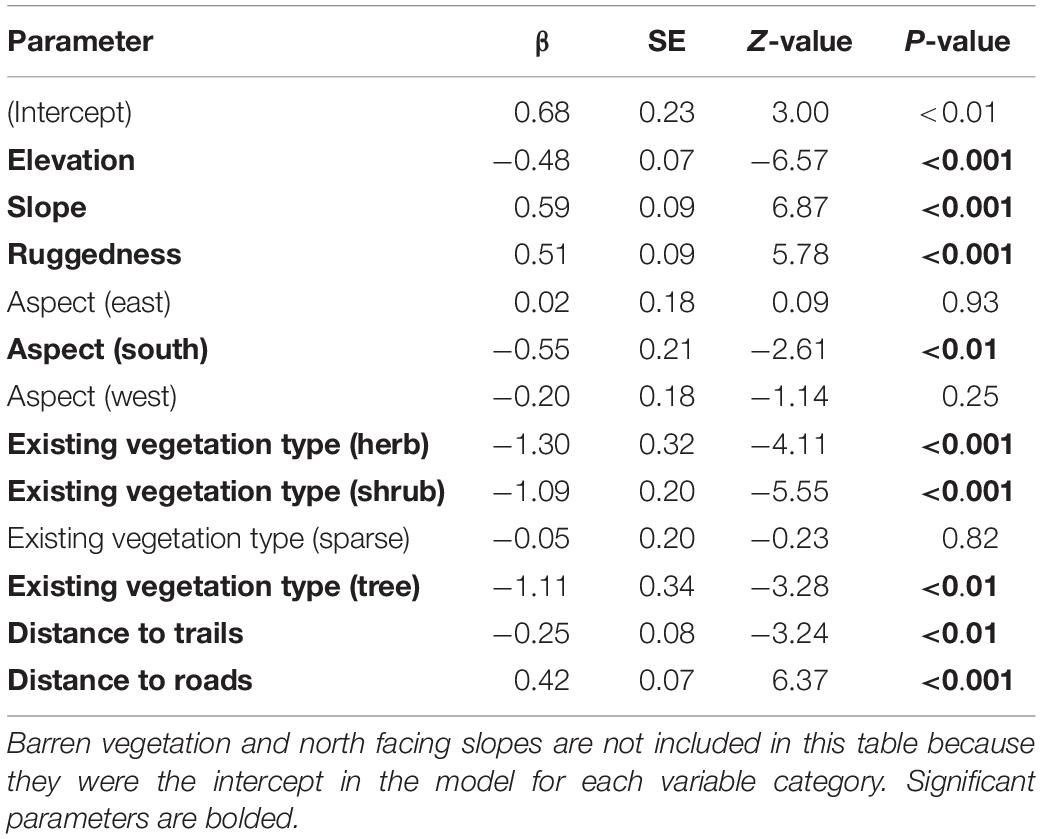
Table 2. Scaled β coefficients (which can be used to compare relative strength and importance of each variable) for resource selection of lambing period habitat of 19 desert bighorn sheep females in the North San Rafael Swell, Utah, United States, from 2012 to 2013.
Five-fold cross validation (rho = 0.99, P = 0.02) indicated that these variables predicted lambing period habitat of bighorns in our study area. In the San Rafael, predicted high-probability habitat was clumped around canyon rims surrounding the mesa top. Large areas of low-use predicted habitat occurred in that area, and locations of females during birthing were concentrated in small canyons (Figure 2). Of our 750 GPS locations, 68% were in the top two probability categories (high = 37%, medium high = 31%), 17% were within the medium category, 11% in the medium-low category, and 4% were within the low category (Figure 2). In the Green River, predicted high-probability habitat occurred along the Green River; however, some locations of females during birthing were concentrated in small canyon draws near areas of low habitat suitability (Figure 3). In the Green River, of our 1,130 GPS locations, 73% were in the top two categories of probability (high = 50%, medium high = 23%), 14% fell into the medium category, 10% fell within the medium-low category, and 3% fell within the low category (Figure 3).
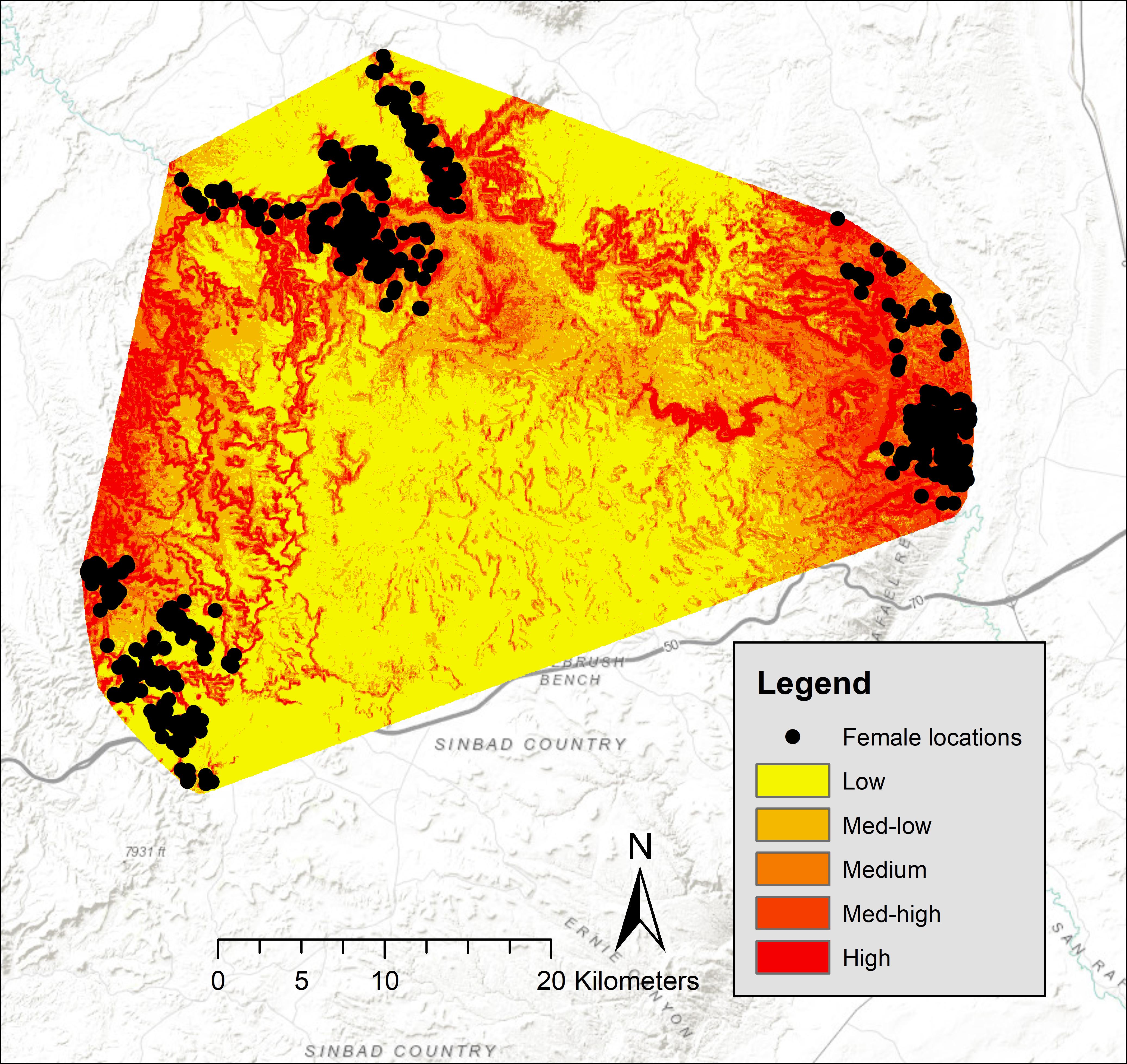
Figure 2. Probabilities of desert bighorn sheep lambing period habitat in the North San Rafael Swell, spring 2012–2013, Utah, United States.
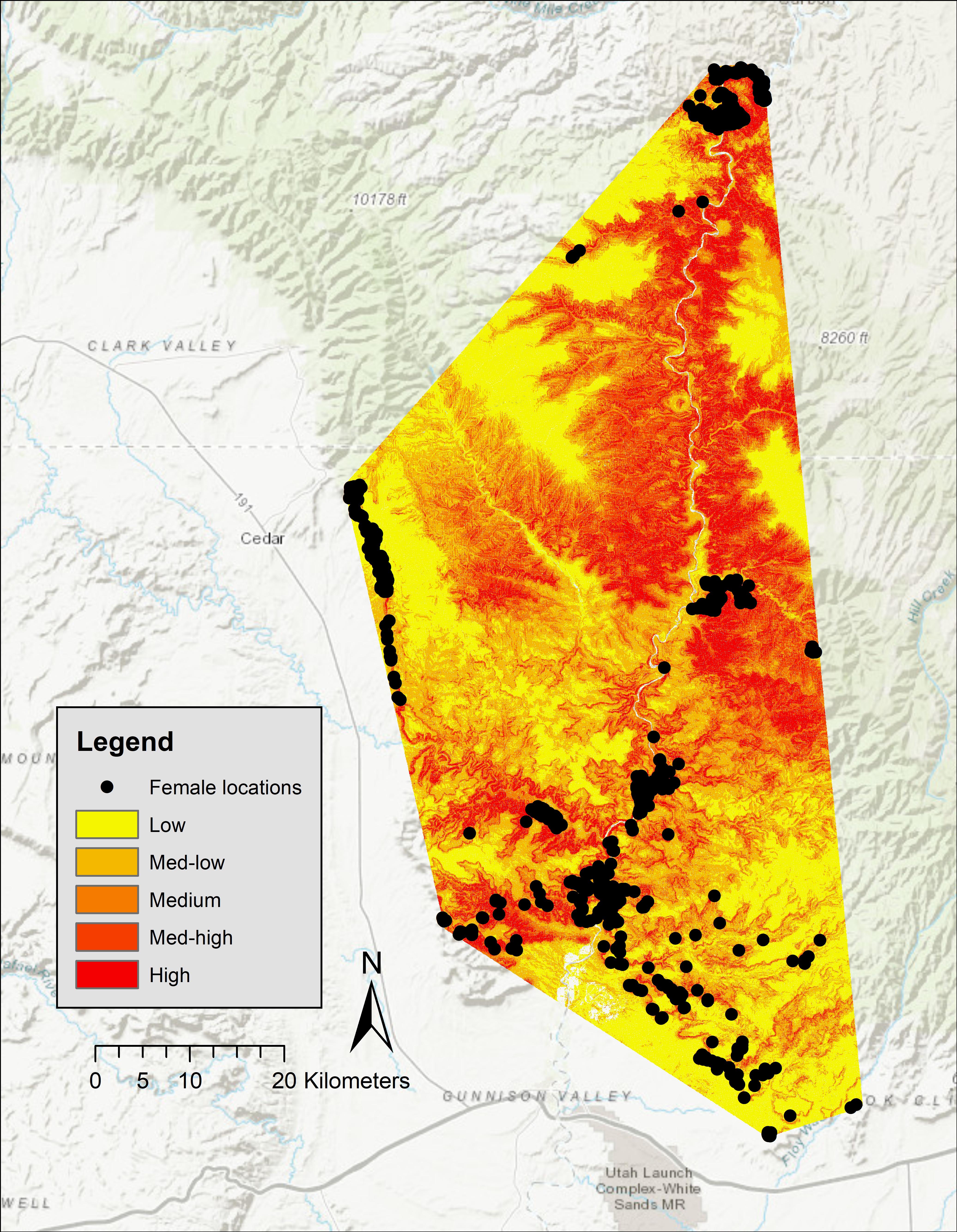
Figure 3. Probabilities of Rocky Mountain bighorn sheep lambing period habitat in the Green River Corridor, spring 2012–2013, Utah, United States.
Discussion
Management and conservation decisions for ungulates and their habitat are increasingly being made that emphasize birthing areas (Singh et al., 2010; Kaze et al., 2016; Severud et al., 2019). For bighorn sheep, additional research is needed to understand and predict habitat use during birthing and nursery periods (Bangs et al., 2005). Our description of timing of births and GIS model predicted lambing period habitat for adjacent populations of desert and Rocky Mountain bighorn sheep. In similar, adjacent habitat these methods could be applied to conserve and manage habitat for these ungulates and to identify lambing period habitat for reintroduction and translocation sites. Reintroduction and translocation continue to be useful management tools for bighorn conservation (Wiedmann and Sargeant, 2014; Boyce and Krausman, 2018; Robinson et al., 2019). In Utah >1,000 Rocky Mountain bighorn sheep and >850 desert bighorn sheep have been released in areas of historical habitat since 1966 (Olson et al., 2008; Shannon et al., 2008; Utah Division of Wildlife Resources [UDWR], 2018). Currently in Utah, 26 units/subunits are identified as potential sites for augmentation or reintroduction, and 27% of those units/subunits are in eastern Utah near our study area (Utah Division of Wildlife Resources [UDWR], 2018). Our results can provide a model that can identify potential lambing period habitat for both subspecies in these areas. Similar site-specific data could be used in other areas to identify birthing period habitat for other populations of adjacent ungulates.
Our GIS model showed where bighorn sheep gave birth and identified lambing period habitat; that model also indicated areas of low-probability lambing period habitat. These results can be important for land-use planning in our study areas. In these areas, outdoor recreation and energy development have increased in the last 40 years and are predicted to continually increase (Smith and Burr, 2011; Sproat et al., 2019). Several of the forms of recreation that can potentially effect bighorn sheep are off-highway vehicles, mountain biking, hiking, rafting, rock climbing, canyoneering, and camping near rivers (Papouchis et al., 2001; Longshore et al., 2013; Sproat et al., 2019). Hiking can have a pronounced effect because of the unpredictable locations of that activity and people approaching bighorn sheep, especially in spring when females are giving birth (Macarthur et al., 1979; Papouchis et al., 2001; Wiedmann and Bleich, 2014). Researchers recommend that known habitat for nursery groups of bighorn sheep be closed to hiking to benefit females and lambs (Papouchis et al., 2001). Our results can be used by biologists and land managers in land-use planning to identify areas of low-probability lambing period habitat that can be designated for increased recreation and energy development. There is an increase in demand for special recreation or special-use permits granted by land management agencies for commercial, competitive, or organized purposes, often for large groups. Given the concentrated level of anthropogenic activity generally associated with these permits, they can be especially disruptive to bighorn sheep. Our results can be helpful for land managers when reviewing permit applications for uses that overlap timing of births and lambing period habitat.
Expectedly, slope and ruggedness were significant variables in our models. Many other researchers indicate that these habitat components are important to minimize risk of predation for bighorn sheep with young (Smith et al., 1991; Bleich et al., 1997; Zeigenfuss et al., 2000; Bangs et al., 2005). Smith et al. (2015) also documented these components selected for at parturition sites and south and west facing slopes. Our model indicated, however, a preference for north facing slopes and an avoidance of south facing slopes. That response was due to a difference in latitude and temperature relative to different study areas, because bighorns are ostensibly selecting north-facing slopes in our study area to avoid warm, south-facing slopes during lactation. Smith et al. (2015) were also modeling parturition sites specifically, while we analyzed lambing period habitat. Lactating females have high water demands (Geist, 1971; Robbins, 2001), and travel of young lambs is restricted, necessitating the use of areas near water (Zeigenfuss et al., 2000). This could explain the preference for north facing slopes and lower elevation where water collects and is retained in our study area.
While our GIS model indicated that bighorn sheep avoided roads, largely corroborating past work (Krausman et al., 1989; Smith et al., 1991; Papouchis et al., 2001), that model also indicated a preference for areas near trails. That result was unexpected and likely occurred because most trails in the San Rafael traverse up the bottoms and around the side rims of canyons. The locations of those trails allow recreationists easy access to unobstructed views of the scenery and landscape. Many of those areas were also used by nursery groups of bighorn sheep. Trail traffic, however, was relatively low in the San Rafael. For example, a motion-sensor camera placed on a canyon of rim trail within high-use lambing period habitat recorded 316 spring visitor days by hikers and bikers. Previous researchers have reported an avoidance of areas by bighorn sheep with more intensive human recreation ( = 15,925 visitor days, SD = 6,038 visitor days) (Wiedmann and Bleich, 2014). Therefore, in the San Rafael the number of hikers might not be affecting bighorn sheep like other areas with high-use trails. We hypothesize that that effect will increase with increased recreation in these areas.
Our methods can be applied to other areas of desert and Rocky Mountain bighorn sheep habitat in Utah. For example, eastern Utah is the largest, contiguous area in the state consisting of important habitat for both subspecies of bighorn sheep (Utah Division of Wildlife Resources [UDWR], 2018). This area has been historically important bighorn sheep habitat (Flinders et al., 2002; Shannon et al., 2008), and some areas have experienced five times the growth in outdoor recreation since 1979 (Sproat et al., 2019), which often peaks in May (Papouchis et al., 2001). Oil and gas exploration and mining are also increasing industries in this area. Human disturbance has caused the abandonment of habitat by desert bighorn sheep (Etchberger et al., 1989; Lowrey and Longshore, 2017) and Rocky Mountain bighorn sheep (Wiedmann and Bleich, 2014), decreased population performance (Wiedmann and Bleich, 2014), and interrupted metapopulation dynamics for these animals (Epps et al., 2005; Bleich et al., 2016). The UDWR now has over 300 GPS collared bighorn sheep in 15 herds across the state. Our methods can be used in these areas to document lambing period habitat. Another important issue in southeastern Utah for which our GIS model can be used is in Grand Staircase-Escalante and Bears Ears national monuments. These areas are now being substantially reduced and opened for potential mining, livestock grazing, public access, and recreation (McBrayer and Roberts-Cady, 2018; Wilson et al., 2018; Reese, 2019). Timing of births data and a GIS model similar to ours could help inform natural resource managers about bighorn lambing period habitat in those areas facing immediate conservation and management changes.
Management of bighorn sheep habitat remains an important issue across much of western North America (Gutierrez-Espeleta et al., 2001; Lowrey and Longshore, 2017; Bleich, 2018; Boyce and Krausman, 2018). Proximity of bighorn sheep to domestic livestock allotments on public land (Cahn et al., 2011; Carpenter et al., 2014; O’Brien et al., 2014), and proximity of those wild ungulates to areas occupied by domestic sheep (O. aries) and goats (Capra hircus) on private land (Turner et al., 2004; Shannon et al., 2014), are controversial issues in the western United States. Domestic sheep and goats can transmit diseases to bighorn sheep (McClintock and White, 2007; Wehausen et al., 2011; Besser et al., 2012), and these issues are critical for the conservation and management of bighorn sheep populations (Cahn et al., 2011; Carpenter et al., 2014; O’Brien et al., 2014). Additionally, domestic cattle can affect habitat use by bighorns (Garrison et al., 2016). With female bighorn sheep showing general fidelity to birth sites and habitat for nursery groups (Etchberger and Krausman, 1999; Whiting et al., 2012), our data can be used to guide land-management decisions when assessing domestic livestock grazing allotments on public land, and when working with land owners on private land, which will help minimize conflict between these domestic and native ungulates.
Timing and synchrony of births in ungulates can have evolutionary implications (Marshall and Cambridge, 1937; Rutberg, 1987; Ims, 1990), such as reduced survival of young and reduced future reproductive potential (Clutton-Brock et al., 1987; Festa-Bianchet et al., 2000; Keech et al., 2000). Bighorn sheep from differing source populations that are released into adjacent areas can take up to 5 years to adapt timing of births to local environments, which can hinder population growth (Whiting et al., 2011). Further, females in those populations do not compensate for late births by increasing maternal care, which possibly reduces survival of young (Whiting et al., 2010). After released bighorn sheep have adapted to local environments, differences of up to 29 days can still exist in timing of births across adjacent populations, because of discrepancies in peak green-up of vegetation (Whiting et al., 2012). All that information underscores the importance of collecting site-specific birthing data to produce GIS models of lambing period habitat for bighorn sheep.
Documenting the effects of human disturbance on ungulates is important for the ecology, evolution, and conservation of these animals, especially in an increasingly human-dominated world (Dzialak et al., 2011; Ciuti et al., 2012a, b). As anthropogenic activity increases in these areas, conservation planning and habitat management will increasingly rely on identifying habitats used by wildlife in areas of varying levels of human use (Goodson et al., 1999; Margules and Pressey, 2000; Harju et al., 2011). Biologists can substantially reduce effects from human activities to female ungulates by identifying where and when animals give birth and rear young (Singh et al., 2010; Kaze et al., 2016; McLaren et al., 2017), and this is a current, pressing conservation issue (Dzialak et al., 2011). Identification of lambing period habitat is becoming increasingly more important for bighorn conservation (Smith et al., 2015). Additionally, with GPS data being more readily accessible in wildlife studies, our methods can be used with site-specific timing of births and abiotic data and applied to other areas occupied by Rocky Mountain and desert bighorn sheep – and other ungulates – to identify important lambing period areas, which will aid in conservation and land-use planning.
Data Availability Statement
The datasets analyzed for this study can be found by contacting the Utah Division of Wildlife Resources.
Ethics Statement
Ethical review and approval was not required for the animal study because all animal handling was done by the Utah Division of Wildlife Resources who held the pertinent permits to do such.
Author Contributions
RR, JW, and JS designed the study. RR performed the field work. JW, RR, and RL analyzed the data. RR, TS, and JW wrote an initial draft of the manuscript and all authors finalized it.
Conflict of Interest
The authors declare that the research was conducted in the absence of any commercial or financial relationships that could be construed as a potential conflict of interest.
Acknowledgments
We thank the UDWR, Utah Wild Sheep Foundation, and Brigham Young University for funding this project.
Footnotes
References
Arnold, T. W. (2010). Uninformative parameters and model selection using Akaike’s Information Criterion. J. Wildl. Manage. 74, 1175–1178. doi: 10.1111/j.1937-2817.2010.tb01236.x
Bangs, P. D., Krausman, P. R., Kunkel, K. E., and Parsons, Z. D. (2005). Habitat use by desert bighorn sheep during lambing. Eur. J. Wildl. Res. 51, 178–184. doi: 10.1007/s10344-005-0098-8
Barry, R. (1979). United States Bureau of Land Management Price River Resource Area. Moab, UT: United States Bureau of Land Management.
Bates, D., Maechler, M., Bolker, B., and Walker, S. (2013). lme4: Linear Mixed-Effects Models Using Eigen and S4. R Package Version 1.0–5. Available online at: https://github.com/lme4/lme4/
Baxter, J. J., Baxter, R. J., Dahlgren, D. K., and Larsen, R. T. (2017). Resource selection by Greater Sage-Grouse reveals preference for mechanically-altered habitats. Rangel. Ecol. Manage. 70, 493–503. doi: 10.1016/j.rama.2017.01.007
Berger, J. (1991). Pregnancy incentives, predation constraints and habitat shifts: experimental and field evidence for wild bighorn sheep. Anim. Behav. 41, 61–71.
Besser, T. E., Highland, M. A., Baker, K., Cassirer, E. F., Anderson, N. J., Ramsey, J. M., et al. (2012). Causes of pneumonia epizootics among bighorn sheep, western United States, 2008–2010. Emerg. Infect. Dis. 18, 406–414. doi: 10.3201/eid1803.111554
Bleich, V. C. (2018). Maintaining momentum for conservation: bighorn sheep as an example. Wildl. Soc. Bull. 42, 540–546. doi: 10.1002/wsb.914
Bleich, V. C., Bowyer, R. T., and Wehausen, J. D. (1997). Sexual segregation in mountain sheep: resources or predation? Wildl. Monogr. 134, 1–50.
Bleich, V. C., Whiting, J. C., Kie, J. G., and Bowyer, R. T. (2016). Roads, routes and rams: does sexual segregation contribute to anthropogenic risk in a desert-dwelling ungulate? Wildl. Res. 43, 380–388.
Bowyer, R. T. (1991). Timing of parturition and lactation in southern mule deer. J. Mammal. 72, 138–145. doi: 10.2307/1381988
Boyce, M. S. (2006). Scale for resource selection functions. Divers. Distrib. 12, 269–276. doi: 10.1111/j.1366-9516.2006.00243.x
Boyce, M. S., and Krausman, P. R. (2018). Controversies in mountain sheep management. J. Wildl. Manage. 82, 5–7. doi: 10.1002/jwmg.21400
Bunnell, F. L. (1982). The lambing period of mountain sheep: synthesis, hypotheses, and tests. Can. J. Zool. 60, 1–14. doi: 10.1139/z82-001
Burnham, K. P., and Anderson, D. A. (2002). Model Selection and Multimodel Inference: A Practical Information-Theoretic Approach. New York, NY: Springer-Verlag.
Cahn, M. L., Conner, M. M., Schmitz, O. J., Stephenson, T. R., Wehausen, J. D., and Johnson, H. E. (2011). Disease, population viability, and recovery of Endangered Sierra Nevada bighorn sheep. J. Wildl. Manage. 75, 1753–1766. doi: 10.1002/jwmg.232
Carpenter, T. E., Coggins, V. L., McCarthy, C., O’Brien, C. S., O’Brien, J. M., and Schommer, T. J. (2014). A spatial risk assessment of bighorn sheep extirpation by grazing domestic sheep on public lands. Prev. Vet. Med. 114, 3–10. doi: 10.1016/j.prevetmed.2014.01.008
Ciuti, S., Muhly, T. B., Paton, D. G., McDevitt, A. D., Musiani, M., and Boyce, M. S. (2012a). Human selection of elk behavioural traits in a landscape of fear. Proc. R. Soc. B Biol. Sci. 279, 4407–4416. doi: 10.1098/rspb.2012.1483
Ciuti, S., Northrup, J. M., Muhly, T. B., Simi, S., Musiani, M., Pitt, J. A., et al. (2012b). Effects of humans on behaviour of wildlife exceed those of natural predators in a landscape of fear. PLoS One 7:e50611. doi: 10.1371/journal.pone.0050611
Clutton-Brock, T. H., Major, M., Albon, S. D., and Guinness, F. E. (1987). Early development and population dynamics in red deer. I. Density-dependent effects on juvenile survival. J. Anim. Ecol. 56, 53–67.
Côté, S. D., and Festa-Bianchet, M. (2001). Birthdate, mass and survival in mountain goat kids: effects of maternal characteristics and forage quality. Oecologia 127, 230–238. doi: 10.1007/s004420000584
Dalton, L. B., and Spillett, J. J. (1971). The bighorn sheep in Utah–past and present. Trans. 1st North Am. Wild Sheep Conf. 1, 32–53.
Dzialak, M. R., Harju, S. M., Osborn, R. G., Wondzell, J. J., Hayden-Wing, L. D., Winstead, J. B., et al. (2011). Prioritizing conservation of ungulate calving resources in multiple-use landscapes. PLoS One 6:e0014597. doi: 10.1371/journal.pone.0014597
Epps, C. W., Palsboll, P. J., Wehausen, J. D., Roderick, G. K., Ramey, R. R., and McCullough, D. R. (2005). Highways block gene flow and cause a rapid decline in genetic diversity of desert bighorn sheep. Ecol. Lett. 8, 1029–1038. doi: 10.1111/j.1461-0248.2005.00804.x
Etchberger, R. C., and Krausman, P. R. (1999). Frequency of birth and lambing sites of a small population of mountain sheep. Southwest. Nat. 44, 354–360.
Etchberger, R. C., Krausman, P. R., and Mazaika, R. (1989). Mountain sheep habitat characteristics in the Pusch Ridge Wilderness, Arizona. J. Wildl. Manage. 53, 902–907.
Festa-Bianchet, M. (1988a). Birthdate and survival in bighorn lambs (Ovis canadensis). J. Zool. 214, 653–661. doi: 10.1007/s00442-008-1035-9
Festa-Bianchet, M. (1988b). Seasonal range selection in bighorn sheep: conflicts between forage quality, forage quantity, and predator avoidance. Oecologia 75, 580–586. doi: 10.1007/BF00776423
Festa-Bianchet, M., Jorgenson, J. T., and Réale, D. (2000). Early development, adult mass, and reproductive success in bighorn sheep. Behav. Ecol. 11, 633–639. doi: 10.1093/beheco/11.6.633
Flinders, J. T., Rogers, D. S., Webber-Alston, J. L., and Barber, H. A. (2002). Mammals of the Grand Staircase-Escalante National Monument: a literature and museum survey. Monogr. West. N. Am. Nat. 1, 1–64. doi: 10.3398/1545-0228-1.1.1
Garrison, K. R., Cain, J. W., Rominger, E. M., and Goldstein, E. J. (2016). Sympatric cattle grazing and desert bighorn sheep foraging. J. Wildl. Manage. 80, 197–207. doi: 10.1002/jwmg.1014
Geist, V. (1971). Mountain Sheep: A Study in Behavior and Evolution. Chicago, IL: The University of Chicago Press.
Goodson, N. J., Stevens, D. R., McCoy, K., and Cole, J. (1999). “Effects of river-based recreation and livestock grazing on desert bighorn sheep on the Navajo Nation,” in Proceedings of the 2nd North American Wild Sheep Conference, Reno, NV, 123–131.
Gutierrez-Espeleta, G. A., Hedrick, P. W., Kalinowski, S. T., Garrigan, D., and Boyce, W. M. (2001). Is the decline of desert bighorn sheep from infectious disease the result of low MHC variation? Heredity 86, 439–450. doi: 10.1046/j.1365-2540.2001.00853.x
Harju, S. M., Dzialak, M. R., Osborn, R. G., Hayden-Wing, L. D., and Winstead, J. B. (2011). Conservation planning using resource selection models: altered selection in the presence of human activity changes spatial prediction of resource use. Anim. Conserv. 14, 502–511. doi: 10.1111/j.1469-1795.2011.00456.x
Hosmer, D. W. Jr., Lemeshow, S., and Sturdivant, R. X. (2013). Applied Logistic Regression. Hoboken, NJ: John Wiley & Sons.
Ims, R. A. (1990). The ecology and evolution of reproductive synchrony. Trends Ecol. Evol. 5, 135–140. doi: 10.1016/0169-5347(90)90218-3
Johnson, D. H. (1980). The comparison of usage and availability measurements for evaluating resource preference. Ecology 61, 65–71. doi: 10.2307/1937156
Johnson, D. S., Barry, R. P., and Bowyer, R. T. (2004). Estimating timing of life-history events with coarse data. J. Mammal. 85, 932–939. doi: 10.1644/bfw-009
Karsch, R. C., Cain, J. W., Rominger, E. M., and Goldstein, E. J. (2016). Desert bighorn sheep lambing habitat: parturition, nursery, and predation sites. J. Wildl. Manage. 80, 1069–1080. doi: 10.1002/jwmg.21092
Kaze, J., Whiting, J. C., Freeman, E. D., Bates, S. B., and Larsen, R. T. (2016). Birth-site selection and timing of births in American bison: effects of habitat and proximity to anthropogenic features. Wildl. Res. 43, 418–428.
Keech, M. A., Bowyer, R. T., Ver Hoef, J. M., Boertje, R. D., Dale, B. W., and Stephenson, T. R. (2000). Life-history consequences of maternal condition in Alaskan moose. J. Wildl. Manage. 64, 450–462.
Krausman, P. R., Hervert, J. J., and Ordway, L. L. (1985). Capturing deer and mountain sheep with a net-gun. Wildl. Soc. Bull. 13, 71–73.
Krausman, P. R., Leopold, B. D., Seegmiller, R. F., and Torres, S. G. (1989). Relationships between desert bighorn sheep and habitat in western Arizona. Wildl. Monogr. 53, 1–66.
Lenarz, M. S. (1979). Social structure and reproductive strategy in desert bighorn sheep (Ovis canadensis mexicana). J. Mammal. 60, 671–678. doi: 10.2307/1380184
Loe, L. E., Bonenfant, C., Mysterud, A., Gaillard, J.-M., Langvatn, R., Klein, F., et al. (2005). Climate predictability and breeding phenology in red deer: timing and synchrony of rutting and calving in Norway and France. J. Anim. Ecol. 74, 579–588. doi: 10.1111/j.1365-2656.2005.00987.x
Long, R. A., Bowyer, R. T., Porter, W. P., Mathewson, P., Monteith, K. L., and Kie, J. G. (2014). Behavior and nutritional condition buffer a large-bodied endotherm against direct and indirect effects of climate. Ecol. Monogr. 84, 513–532. doi: 10.1890/13-1273.1
Long, R. A., Muir, J. D., Rachlow, J. L., and Kie, J. G. (2009). A comparison of two modeling approaches for evaluating wildlife-habitat relationships. J. Wildl. Manage. 73, 294–302. doi: 10.1111/gcb.13900
Longshore, K., Lowrey, C., and Thompson, D. B. (2013). Detecting short-term responses to weekend recreation activity: desert bighorn sheep avoidance of hiking trails. Wildl. Soc. Bull. 37, 698–706. doi: 10.1002/wsb.349
Lowrey, C., and Longshore, K. M. (2017). Tolerance to disturbance regulated by attractiveness of resources: a case study of desert bighorn sheep within the river mountains, Nevada. West. N. Am. Nat. 77, 82–99.
Macarthur, R. A., Johnston, R. H., and Geist, V. (1979). Factors influencing heart-rate in free-ranging bighorn sheep-physiological approach to the study of wildlife harassment. Can. J. Zool. 57, 2010–2021. doi: 10.1139/z79-265
Manly, B. F. J., McDonald, L. L., Thomas, D. L., McDonald, T. L., and Erickson, W. P. (2002). Resource Selection by Animals: Statistical Design and Analysis for Field Studies. Boston, MA: Kluwer Academic.
Margules, C. R., and Pressey, R. L. (2000). Systematic conservation planning. Nature 405, 243–253. doi: 10.1038/35012251
Marshall, F. H. A., and Cambridge, F. R. S. (1937). On the change over in the oestrous cycle in animals after transference across the equator, with further observations on the incidence of the breeding seasons and the factors controlling sexual periodicity. Proc. R. Soc. Lond. B 122, 413–428. doi: 10.1098/rspb.1937.0034
McBrayer, J., and Roberts-Cady, S. (2018). The case for preserving Bears Ears. Ethics Policy Environ. 21, 48–51. doi: 10.1080/21550085.2018.1448035
McClintock, B. T., and White, G. C. (2007). Bighorn sheep abundance following a suspected pneumonia epidemic in Rocky Mountain National Park. J. Wildl. Manage. 71, 183–189. doi: 10.2193/2006-336
McLaren, A., Benson, J., and Patterson, B. (2017). Multiscale habitat selection by cow moose (Alces alces) at calving sites in central Ontario. Can. J. Zool. 95, 891–899. doi: 10.1139/cjz-2016-0290
O’Brien, J. M., O’Brien, C. S., McCarthy, C., and Carpenter, T. E. (2014). Incorporating foray behavior into models estimating contact risk between bighorn sheep and areas occupied by domestic sheep. Wildl. Soc. Bull. 38, 321–331. doi: 10.1002/wsb.387
Olson, D. D., Shannon, J. M., Whiting, J. C., and Flinders, J. T. (2008). History, status, and population structure of California bighorn sheep in Utah. Proc. North. Wild Sheep Goat Counc. 16, 161–177.
Papouchis, C. M., Singer, F. J., and Sloan, W. B. (2001). Responses of desert bighorn sheep to increased human recreation. J. Wildl. Manage. 65, 573–582.
Phillips, G. E., and Alldredge, A. W. (2000). Reproductive success of elk following disturbance by humans during calving season. J. Wildl. Manage. 64, 521–530. doi: 10.2307/3803250
R Core Team (2015). A Language and Environment for Statistical Computing. Vienna: R Foundation for Statistical Computing.
Rachlow, J. L., and Bowyer, R. T. (1991). Interannual variation in timing and synchrony of parturition in Dall’s sheep. J. Mammal. 72, 487–492. doi: 10.2307/1382131
Rachlow, J. L., and Bowyer, R. T. (1994). Variability in maternal behavior by Dall’s sheep: environmental tracking or adaptive strategy. J. Mammal. 75, 328–337. doi: 10.2307/1382551
Rigby, J. K., and Beus, S. S. (1987). Stratigraphy and structure of the San Rafael Reef, Utah, a major Monocline of the Colorado Plateau. Decade N. Am. Geol. 2, 269–273. doi: 10.1130/0-8137-5402-x.269
Robinson, R. W., Whiting, J. C., Shannon, J. M., Olson, D. D., Flinders, J. T., Smith, T. S., et al. (2019). Habitat use and social mixing between groups of resident and augmented bighorn sheep. Sci. Rep. 9:14984. doi: 10.1038/s41598-019-51370-y
Rollins, M. G. (2009). LANDFIRE: a nationally consistent vegetation, wildland fire, and fuel assessment. Int. J. Wildl. Fire 18, 235–249. doi: 10.1071/wf08088
Rubin, E. S., Boyce, W. M., and Bleich, V. C. (2000). Reproductive strategies of desert bighorn sheep. J. Mammal. 81, 769–786. doi: 10.1644/1545-1542(2000)081<0769:rsodbs>2.3.co;2
Rutberg, A. T. (1987). Adaptive hypotheses of birth synchrony in ruminants: an interspecific test. Am. Nat. 130, 692–710. doi: 10.1086/284739
Sadleir, R. M. F. S. (1969). The role of nutrition in the reproduction of wild mammals. J. Reprod. Fertil. Suppl. 6, 39–48.
Sappington, J. M., Longshore, K. M., and Thompson, D. B. (2007). Quantifying landscape ruggedness for animal habitat analysis: A case study using bighorn sheep in the Mojave Desert. J. Wildl. Manage. 71, 1419–1426. doi: 10.2193/2005-723
Sawyer, H., Nielson, R. M., Lindzey, F. G., Keith, L., Powell, J. H., and Abraham, A. A. (2007). Habitat selection of Rocky Mountain elk in a nonforested environment. J. Wildl. Manage. 71, 868–874. doi: 10.2193/2006-131
Severud, W. J., DelGiudice, G. D., and Obermoller, T. R. (2019). Association of moose parturition and post-parturition habitat with calf survival. J. Wildl. Manage. 83, 175–183. doi: 10.1002/jwmg.21570
Shannon, J. M., Olson, D. D., Whiting, J. C., Flinders, J. T., and Smith, T. S. (2008). “Status, distribution, and history of Rocky Mountain bighorn sheep in Utah,” in Proceedings of the Northern Wild Sheep and Goat Council, (Heber, UT: Northern Wild Sheep and Goat Council), 178–195.
Shannon, J. M., Whiting, J. C., Larsen, R. T., Olson, D. D., Flinders, J. T., Smith, T. S., et al. (2014). Population response of reintroduced bighorn sheep after observed commingling with domestic sheep. Eur. J. Wildl. Res. 60, 737–748. doi: 10.1007/s10344-014-0843-y
Sikes, R. S., Animal Care and Use Committee of the American Society of Mammalogists (2016). 2016 Guidelines of the American Society of Mammalogists for the use of wild mammals in research and education. J. Mammal. 97, 663–688. doi: 10.1093/jmammal/gyw078
Singh, N. J., Grachev, I. A., Bekenov, A. B., and Milner-Gulland, E. J. (2010). Saiga antelope calving site selection is increasingly driven by human disturbance. Biol. Conserv. 143, 1770–1779. doi: 10.1016/j.biocon.2010.04.026
Smith, J., and Burr, S. (2011). Environmental attitudes and desired social-psychological benefits of off-highway vehicle users. Forests 2, 875–893. doi: 10.3390/f2040875
Smith, J. B., Grovenburg, T. W., and Jenks, J. A. (2015). Parturition and bed site selection of bighorn sheep at local and landscape scales. J. Wildl. Manage. 79, 393–401. doi: 10.1002/jwmg.843
Smith, T. S., Flinders, J. T., and Winn, D. S. (1991). A habitat evaluation procedure for Rocky Mountain bighorn sheep in the Intermountain West. Great Basin Nat. 51, 205–225.
Sproat, K. K., Martinez, N. R., Smith, T. S., Sloan, W. B., Flinders, J. T., Bates, J. W., et al. (2019). Desert bighorn sheep responses to human activity in South-eastern Utah. Wildl. Res. 47, 16–24.
Stankowich, T. (2008). Ungulate flight responses to human disturbance: a review and meta-analysis. Biol. Conserv. 141, 2159–2173. doi: 10.1016/j.biocon.2008.06.026
Thompson, R. W., and Turner, J. C. (1982). Temporal geographic variation in the lambing season of bighorn sheep. Can. J. Zool. 60, 1781–1793. doi: 10.1139/z82-231
Turner, J. C., Douglas, C. L., Hallam, C. R., Krausman, P. R., and Ramey, R. R. (2004). Determination of critical habitat for the endangered Nelson’s bighorn sheep in southern California. Wildl. Soc. Bull. 32, 427–448. doi: 10.2193/0091-7648(2004)32[427:dochft]2.0.co;2
Utah Division of Wildlife Resources [UDWR] (2018). Utah Bighorn Sheep Statewide Management Plan. Salt Lake City, UT: Utah Division of Wildlife Resources.
Villepique, J. T., Pierce, B. M., Bleich, V. C., Andic, A., and Bowyer, R. T. (2015). Resource Selection by an endangered ungulate: a test of predator-induced range abandonment. Adv. Ecol. 2015:357080.
Wehausen, J. D., Kelley, S. T., and Ramey, R. R. (2011). Domestic sheep, bighorn sheep, and respiratory disease: a review of the experimental evidence. Calif. Fish Game 97, 7–24. doi: 10.1016/j.prevetmed.2012.11.018
Whiting, J. C., Bowyer, R. T., and Flinders, J. T. (2008). Young bighorn (Ovis canadensis) males: can they successfully woo females? Ethology 114, 32–41. doi: 10.1111/j.1439-0310.2007.01442.x
Whiting, J. C., Bowyer, R. T., Flinders, J. T., and Eggett, D. L. (2011). Reintroduced bighorn sheep: fitness consequences of adjusting parturition to local environments. J. Mammal. 92, 213–220. doi: 10.1644/10-mamm-a-145.1
Whiting, J. C., Olson, D. D., Shannon, J. M., Bowyer, R. T., Klaver, R. W., and Flinders, J. T. (2012). Timing and synchrony of births in bighorn sheep: implications for reintroduction and conservation. Wildl. Res. 39, 565–572.
Whiting, J. C., Stewart, K. M., Bowyer, R. T., and Flinders, J. T. (2010). Reintroduced bighorn sheep: do females adjust maternal care to compensate for late-born young? Eur. J. Wildl. Res. 56, 349–357. doi: 10.1007/s10344-009-0323-y
Wiedmann, B. P., and Bleich, V. C. (2014). Demographic responses of bighorn sheep to recreational activities: a trial of a trail. Wildl. Soc. Bull. 38, 773–782. doi: 10.1002/wsb.463
Wiedmann, B. P., and Sargeant, G. A. (2014). Ecotypic variation in recruitment of reintroduced bighorn sheep: implications for translocation. J. Wildl. Manage. 78, 394–401. doi: 10.1002/jwmg.669
Wilson, J. S., Kelly, M., and Carril, O. M. (2018). Reducing protected lands in a hotspot of bee biodiversity: bees of Grand Staircase-Escalante National Monument. PeerJ 6:e6057. doi: 10.7717/peerj.6057
Keywords: GIS model, Ovis canadensis, reintroductions, timing of births, translocations
Citation: Robinson RW, Smith TS, Whiting JC, Larsen RT and Shannon JM (2020) Determining Timing of Births and Habitat Selection to Identify Lambing Period Habitat for Bighorn Sheep. Front. Ecol. Evol. 8:97. doi: 10.3389/fevo.2020.00097
Received: 18 December 2019; Accepted: 26 March 2020;
Published: 17 April 2020.
Edited by:
Paul Richard Krausman, University of Arizona, United StatesReviewed by:
Clinton Wakefield Epps, Oregon State University, United StatesMarco Festa-Bianchet, Université de Sherbrooke, Canada
Copyright © 2020 Robinson, Smith, Whiting, Larsen and Shannon. This is an open-access article distributed under the terms of the Creative Commons Attribution License (CC BY). The use, distribution or reproduction in other forums is permitted, provided the original author(s) and the copyright owner(s) are credited and that the original publication in this journal is cited, in accordance with accepted academic practice. No use, distribution or reproduction is permitted which does not comply with these terms.
*Correspondence: Jericho C. Whiting, whitingj@byui.edu