Long-term effects of soft rock amendment on changes of soil aggregate cementing agents of sandy soil by SEM-EDS
- 1Technology Innovation Center for Land Engineering and Human Settlements, Shaanxi Land Engineering Construction Group Co., Ltd., and Xi’an Jiaotong University, Xi’an, China
- 2College of Life Sciences, Yulin University, Yulin, China
- 3Key Laboratory of Degraded and Unused Land Consolidation Engineering, Ministry of Natural and Resources of China, Xi’an, China
- 4School of Human Settlements and Civil Engineering, Xi’an Jiaotong University, Xi’an, China
Soil aggregates are a crucial constituent of soil and have a significant function in regulating water, nutrients, air, and heat within the soil. The development of soil aggregates is influenced by various factors, including the soil’s parent material and human activities. Understanding the formation and the mechanism of stabilization of soil aggregates is of great significance in the study of soil development, in regulating and managing organic carbon pools in soils, and in promoting soil fertility. In this study, aeolian sandy soil with a low degree of soil development and compound soil formed by combining soft rock and aeolian sandy soil were selected as the research objects. We selected three time points from 0 to 9 years after amendment by soft rock in order to investigate the changes of soil aggregate cementing agents. The shape of soil aggregates in both types of soils was analyzed by environmental scanning electron microscopy-energy dispersive spectroscopy (SEM-EDS), which were also used to assess the appearance of soil aggregates and quantify the composition of mineral elements on a cross section of the aggregate. The results show that when the soft rock and the aeolian sandy soil are compounded and mixed, the clay minerals in the soft rock change the microstructure of the original aeolian sandy soil from a single granular barrier to one characterized by a cumulative porous structure, indicating that clay minerals promote soil development and form aggregates with good structural properties. The cementing agents in the compounded soil aggregates are mainly clay minerals, aluminum, iron, and calcium. In comparison to aeolian sandy soils, the presence of iron and calcium in compounded soils is notably elevated. The iron oxides present in compounded soils serve a similar function to “bolts” in the formation of soil aggregates. These findings establish a theoretical foundation for investigating the process of soil aggregate formation and the mechanisms by which cementing agents contribute to their stabilization.
1 Introduction
Soil aggregates are an important component of soil and are widely regarded as the basic unit as well as an important parameter of soil structure (Barral et al, 1998; Hou et al, 2018). Aggregates play important roles in coordinating water, nutrients, air, and heat in soil, affecting the types and activities of soil enzyme, maintaining and stabilizing soil, and loosening and curing layers (Haynes et al, 1993; Jastrow et al, 1996). The formation of soil aggregates is a very complex process involving a range of physical, chemical, and biological effects that depend primarily on the quantity and nature of the various materials of composition in the soil (Chaney et.al., 1986a; Janalizadeh et al, 2019b; Lado et al, 2004). During the process of soil particle aggregation, not only organic compounds play an important role in particle cementation, but inorganic compounds such as iron and aluminum oxides and hydroxides, as well as silica and calcium carbonate also play important roles (Arya et al, 1972; Falsone et al, 2007; Wiesmeier et al, 2012). The parent material is the primary material that makes up soil, and has a great influence on the composition and action of inorganic soil cementing agents (Bosch-Serra et al, 2017; Oades et al, 1993). The formation of organic cementing agents is related to the quantity of microorganisms, their activity and metabolites, plant root exudates, and organic matter input (Chaney et.al., 1986b; Fakhrabadi et al, 2021; Gale et al, 2000).
Soil cementing agents may be inorganic, organic, or a combination of organic and inorganic (Giovannini et al, 1976; Shi et al, 2002) Although these three types of cementing agents may be found at the same time in different soil types, the composition of organic and inorganic cementing agents in the soil differs due to differences in parent materials, bioclimatic conditions, and agricultural management. In soils with high organic matter content and low clay and oxidized iron and aluminum, the role of organic matter is dominant (Guénet et al, 2016; Ren et al, 2011); in soils with low organic matter content, but high clay and oxidized iron and aluminum content, the formation of soil aggregates is mainly due to the cohesive force between clay particles and due to cementation by iron and aluminum oxides (Barral et al, 1998; Roshan et al, 2022; Skjemstad et al, 1993). Numerous studies have investigated the quantity, distribution, stability, and other factors that influence the formation of soil aggregates (Chaney et.al., 1986a; Terpstra et al, 1990; Diaz et al, 1994; Schomburg et al, 2018). However, such studies cannot describe the internal structure of soil aggregates, explain the process of aggregate formation, or the cementation mechanism. The basic physical indicators of aggregates contain very limited information and do not reflect the complex internal structural differences of the aggregates, but it is difficult to distinguish and discriminate the shape and internal composition of soil aggregates.
Soft rock, formed in continental clastic rock system of late Paleozoic Permian, Mesozoic Triassic, Jurassic, and Cretaceous (Sun and Han, 2018), has the characteristics of low diagenetic degree, poor intergrain bond and low structural intensity and is the fundamental reason for serious water loss and soil erosion in soft rock area. To solve the problems of the sand soil sharing common root but with different qualities and that it is hard for sandy to become soils, Han et al (2012) found that soft rock had great potentials as sandy remediating material. They prepared “new type soil” by mixing soft rock sandstone with sand at certain proportion, which not only could solve the problems of the sandy being unable to form colloidal materials due to poor resistance and fertility, but also could alleviate the trouble of nonlocal soil dressing. Applied in Mu Us Desert land consolidation projects, this technology helped increase the arable areas of more than 200,000 ha. Corns and potatoes have been planted in the “compound soil” with high yield. In this way, utilization of sand and soft rock is realized, which has increased effectively the arable areas and guaranteed food security with good ecologic, economic and social benefits (Sun et al, 2021). To study furthermore the improvement of soft rock of aeolian sandy soil as well as the formation of this new type of “artificial soil” mixed with soft rock and sand and to realize multi-purpose application of the compound soil, it is hard to diagnose and study relying only on some routine features of cross section form and the fundamental physical and chemical analysis; therefore, it requires also to study profoundly the micro morphology of the compound soil as well as the formation and development of the aggregate cementing materials in the compound soil.
With the advancement of soil morphology research, research on the structure of soil aggregates has evolved from qualitative observation by scanning electron microscopy (SEM) to quantitative analysis combined with image processing technology (Garbout et al, 2013; Hapca et al, 2015; Wang et al, 2012; Wilson et al, 2013). SEM has the characteristics of high magnification, high resolution, and large depth of field. It can be used to directly observe the original microstructure of soil aggregates and can be used together with X-ray diffraction spectroscopy to achieve simultaneous analysis of morphology at a microscopic scale and elemental composition (Sayen et al, 2009; Jiménez-Pinilla et al, 2016; Yu et al, 2017). It has become an important tool for the analysis of the morphology of clay mineral particles. The combined analysis of the qualitative and quantitative microstructure data provides information on the various nutrients, microorganisms, and cementing agents in soil aggregates and is an ideal method by which the state and mechanism of soil aggregate formation may be explored. For this research, the selected objects of study were aeolian sandy soil with low soil development and a compound soil created by combining aeolian sandy soil and soft rock. Understanding the nature of organic cementing materials, the concepts behind the formation of soil aggregates, the mechanisms of soil aggregate stability, and the mechanism for renewal and turnover can help to further explore changes in soil development and succession, organic carbon pool, water stability of soil aggregates, and other soil fertility indexes once virgin land is first opened for cultivation, as well as the mechanism of the influence of soil structure on soil fertility (Yu et al, 2017; Watteau et al, 2012; Zhang et al, 2015). The main purpose of our study is to determine, through the use of SEM-energy dispersive spectroscopy (EDS):
• the main composition of cementing agents on the surface of soil aggregates during soil development
• to quantify the distribution of major mineral elements on the surface of soil aggregates
• to elucidate the mechanism of action of cementing agents during the formation of soil aggregates.
2 Materials and methods
2.1 Sites and soil sampling
The aeolian sandy soil and compound soil selected for the experiment were collected from the Mu Us desert, Jingkeliang, Daji Khan Village, Yuyang District, Yulin City, China (38°27′53″N, 109°28′58 E). The area is located in northwestern Shaanxi at an altitude of 1,210 m above sea level and is a typical warm temperate monsoon climate. The annual average temperature is 6.0°C–8.5°C and the annual precipitation is 400–440 mm. The compound soil is composed of materials from soft rock (also called Pisha sandstone or Feldspathic Sandstone) and aeolian sandy soil in a volume ratio of 1:2 [Detailed field experiments design were shown in Sun et al (2018)]. The physical and chemical properties of the aeolian sandy soil and the soft rock materials are shown in Tables 1, 2. The soft rock is composed of Paleozoic Permian, Mesozoic Triassic, Jurassic and Cretaceous thick layer sandstones, sand shale, and argillaceous sandstones (Bazhenov et al, 1993; Martin et al, 1999). Its rock layer is thin, with low pressure, low diagenesis, and poor cementation between sand grains and poor structural strength (Wang et al, 2009). In recent years, some scholars have used the complementary properties of soft rock and aeolian sandy soil to form a new compound soil (the soil at this time is only at the beginning of its development) which is an improvement on aeolian sandy soil (Sun et al, 2018; Han et al, 2015; Han et al, 2012; Wang et al, 2013; Sun et al, 2019). As the years of cultivation increase, the compound soil tends to ripen. Therefore, this study selected aeolian sandy soil, compound soil that has undergone 3 years of planting, and compound soil that has undergone 9 years of planting as research materials. Undisturbed soil was collected from topsoil and the soil samples were taken back to the laboratory and naturally air-dried. The dry sieve method was used to screen the 1–2 mm soil aggregates that were the objects of observation in this study.

TABLE 1. Basic characteristic of sandy soil and soft rocka.
2.2 SEM and X-ray diffraction methods
The German LEO1430VP scanning electron microscopy (SEM) and the OXFORD7353 energy dispersive spectrometer (EDS) were used in combination. The SEM was operated with a test voltage of 30 kV, a secondary electron resolution of 3.5 nm, and a maximum magnification of 900,000 times. The EDS was used for quantitative analysis of the mineral elements in the sample, with an error of less than 5%. SEM-EDS was used to observe and analyze the soil aggregates (1–2 mm). The relatively flat interparticle cementation was used as the location of analysis for surface cementation, which standardized the locations where analyses were performed. In this study, 10 typical cementation points were selected on the surface of each observed soil aggregate for energy spectral point element analysis.
Soil aggregates 1.0–2.0 mm were selected for cross-sectional elemental analysis. The selected aggregates were embedded and fixed with polyester resin and sample columns with a diameter of 2 cm and a height of 1 cm were cut out, and the sample was mechanically polished. The cross section of the compound soil was observed by SEM, and the elemental distribution of the soil aggregate section was analyzed using the element mapping mode of EDS. Firstly, the typical cross section of the target aggregate was found at low magnification and then the overall element distribution analysis was performed by using the mapping mode. Finally, the overall regional elements are collected and analyzed quantitatively using pattern mode analysis. The SEM was set on fast mode, working distance 10 mm, acceleration voltage 15 kv, dead time is at 30%–50%, and then the result was subjected to zaf wt% normalization and correction.
2.3 Statistical analysis
Statistical analysis was performed using the PROC GLM process in SAS for equation analysis. The mean comparison was performed using the least significant difference (LSD) method with a significance level of p < 0.05 between treatments.
3 Analysis result
3.1 Aggregate trait analysis
The microaggregates of aeolian sandy soil are mainly composed of single particles of irregular shape, with high degree of rounding, smooth surface, almost no sharp edges and corners, and no bonding surface (Figures 1A, C). Compared with the aeolian sandy soil aggregates, the compound soil aggregates have a certain number of bridging barrier structures formed by the bonding of small mineral particles as cementing agents to the core structural particles; the coarse core structural particles are filled by fine particles, which either form filler-like junction structures filling the voids of the core structure or an envelope-like structure encapsulating core structural particles (Figures 1B, D). The differences in morphological characteristics between compound soil and aeolian sandy soil can be clearly observed on the surface of compound soil microaggregates, where 2–40 μm morphologically different pore structures can be observed (Figure 2A). The presence of hyphae was also observed on the surface of compound soil aggregates (Figure 2B), indicating that the compound soil has a good soil aggregate structure.
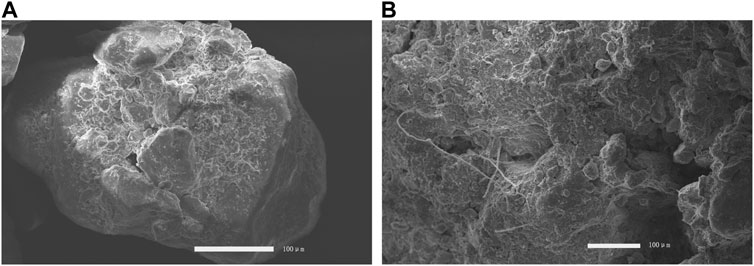
FIGURE 2. Morphology features of aggregates in compound soil. (A) Surface of compound soil aggregates; (B) hyphae in soil aggregates.
3.2 Soil aggregate cross-section elemental distribution
The aeolian sandy soil aggregate section has regions of significant Si and Al enrichment, and only a small amount of Fe and Ca (Figure 3). The distribution of Al, Ca, and Fe in aeolian sandy soil aggregates are intermittently in the soil aggregate cross section. Areas of highly concentrated Si, Al, Fe, and Ca appeared in the cross section of compound soil aggregates that has undergone 3 years of planting (Figure 4). The Al-Mg distribution zones in the compounded soil have strong correlation and connectivity, and run through the entire soil aggregate cross section and form the basic shape of the aggregate section. Al has obvious regions of enrichment that exist mainly as semi-joined points. The areas of Al enrichment are mostly concentrated on the pores in the cross section and at the edge of the core structural particles. Ca is distributed independently in some parts of the section, and is relatively far from the areas containing Al-Mg.
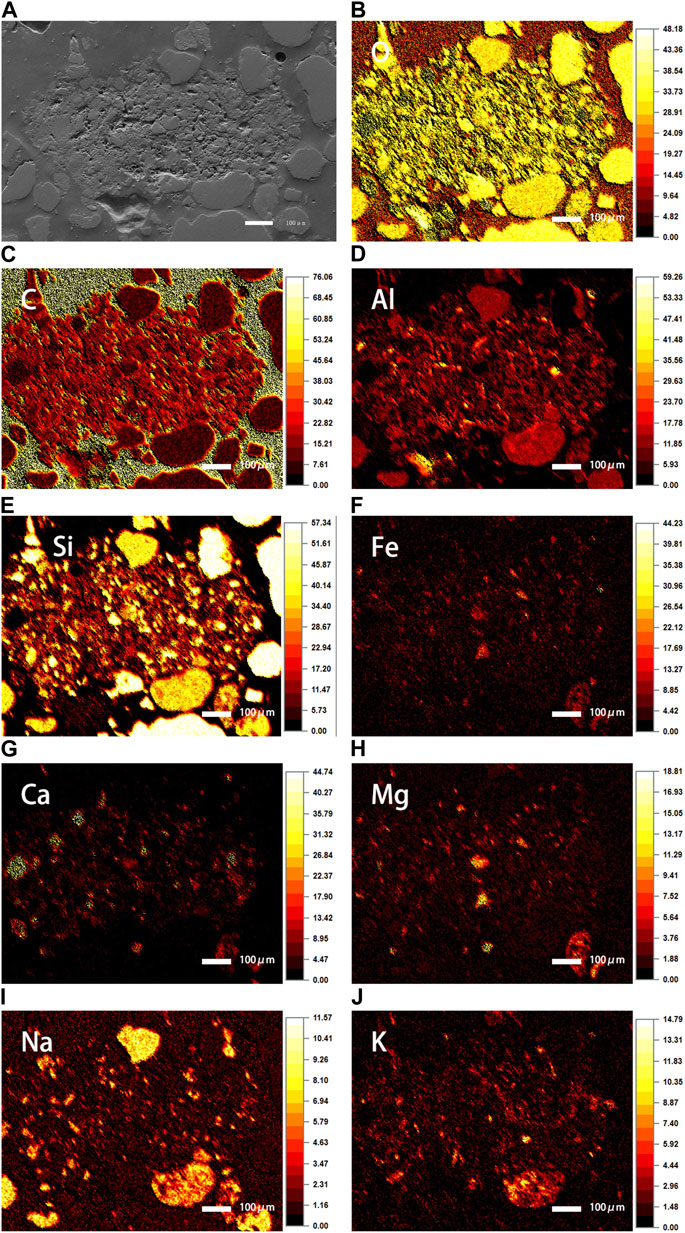
FIGURE 3. SEM–EDS analysis of aeolian sandy soil aggregate. SEM image of the sample (A), and corresponding X-ray maps of O (B), C (C), Al (D), Si (E), Fe (F), Ca (G), Mg (H), Na (I), and K (J).
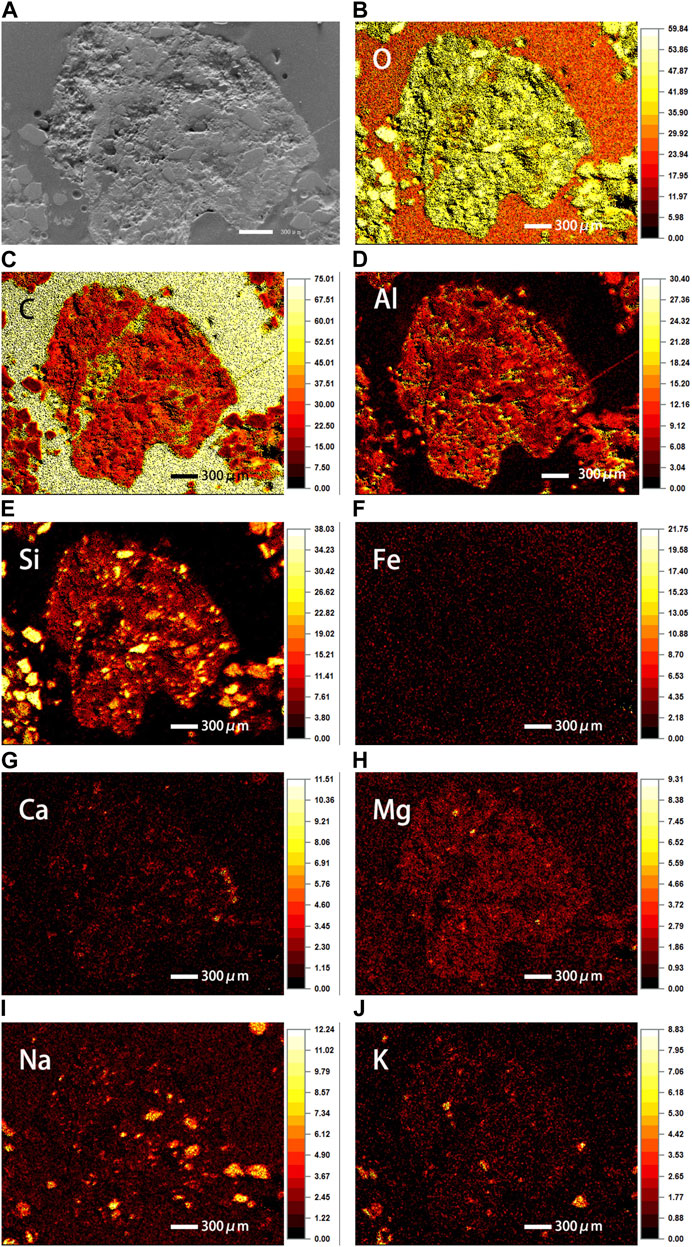
FIGURE 4. SEM-EDS analysis of compound soil aggregates that has undergone 3 years of planting. SEM image of the sample (A), and corresponding X-ray maps of O (B), C (C), Al (D), Si (E), Fe (F), Ca (G), Mg (H), Na (I), and K (J).
3.3 Analysis of elemental content on the surface of soil aggregates
The relative contents of Fe, Ca and Mg in the compound soil aggregates were significantly higher than those in the aeolian sandy soil (Table 3). It suggests that Fe, Ca, and Mg elements play an important role in the cementation process during the formation of soil aggregates. From this, it can be inferred that compared with aeolian sandy soil, the cementing materials on the surface of compound soil aggregates mainly include ferric oxide, calcium carbonate, and magnesium carbonate. After 9 years of cultivation, there are more iron cementation points on the surface of large aggregates in compound soil, and the content of Fe super-enriched zones is higher. The relative content of Fe increased from 7.27% to 10.74% and the distribution area is more extensive and tight. The Ca enrichment area also increased and the relative content of Ca increased from 3.47% to 4.40% (Table 3).
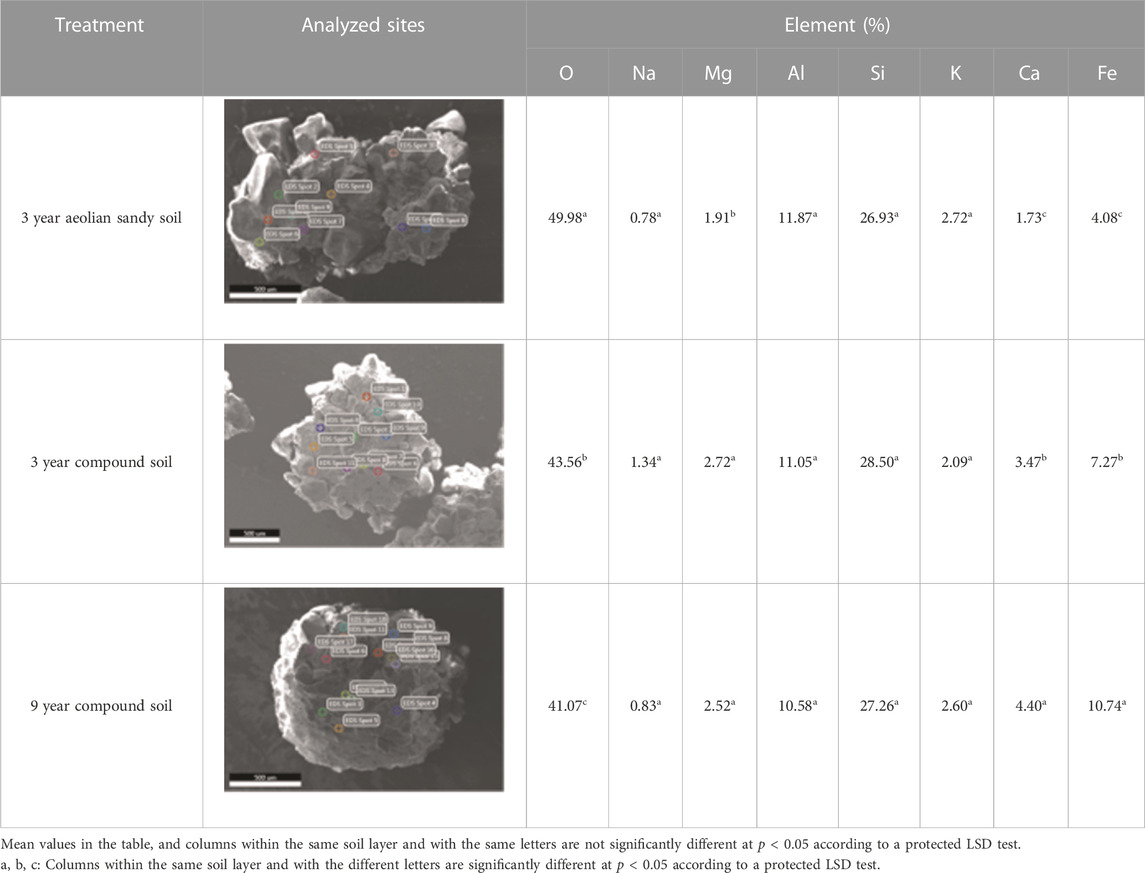
TABLE 3. Analytical results of X-ray energy spectrum characteristics of the aggregates in aeolian sandy soil and compound soils.
4 Discussion
Soil minerals are important constituents of soil and can be divided into three types: primary minerals, secondary minerals, and soluble minerals (Gislason et al, 1996; Ghadakpour et al, 2020). Primary minerals in soft rock and compound soils are silicate minerals such as quartz and feldspar. The main elements are Si, Al, and O. Secondary minerals include clay minerals, secondary oxides, and salts. The clay minerals are mostly in the form of cementing agents (Afrakoti et al, 2020; Roshan et al, 2020). The elemental composition is mostly Si, Al, O, Mg, K, Ca, and Fe. Secondary oxides are iron oxide or oxidized alumina, and its elemental composition is mostly O, Fe, and Al. Salt minerals include carbonates and other substances, the main elements of which are O, C, and Ca. In this study, the resin embedding technique was used for the cross-section analysis of the soil aggregates. The constituent elements of the resin were C and O. Therefore, C and O were not analyzed in this study.
This study analyzed the mapped image of the elements found on the cross-section of 1–2 mm soil aggregates in compound soil. According to the distribution characteristics of the Fe-rich region using iron oxide as the indicator, iron oxide is mainly in the form of an organic-inorganic composite formed by the iron oxide cemented by adsorption or co-precipitation with organic matter, and is mostly present around the core structural particles of the soil aggregate and at the pores as a clear semi-continuous envelope (Janalizadeh et al, 2019a; Koutenaei et al, 2021). Iron oxides act like “bolts” in the development of compound soil aggregates. In addition, the high degree of association between Fe-Ca distribution zones and Fe enrichment zones in the cross section of compound soil aggregates cultivated for 3 and 9 years indicates that good clay mineral-metal oxide-organic matter composite cementing agents is formed in the compound soil (Figures 4, 5). The composite cementing system is more stable as the years of cultivation increases.
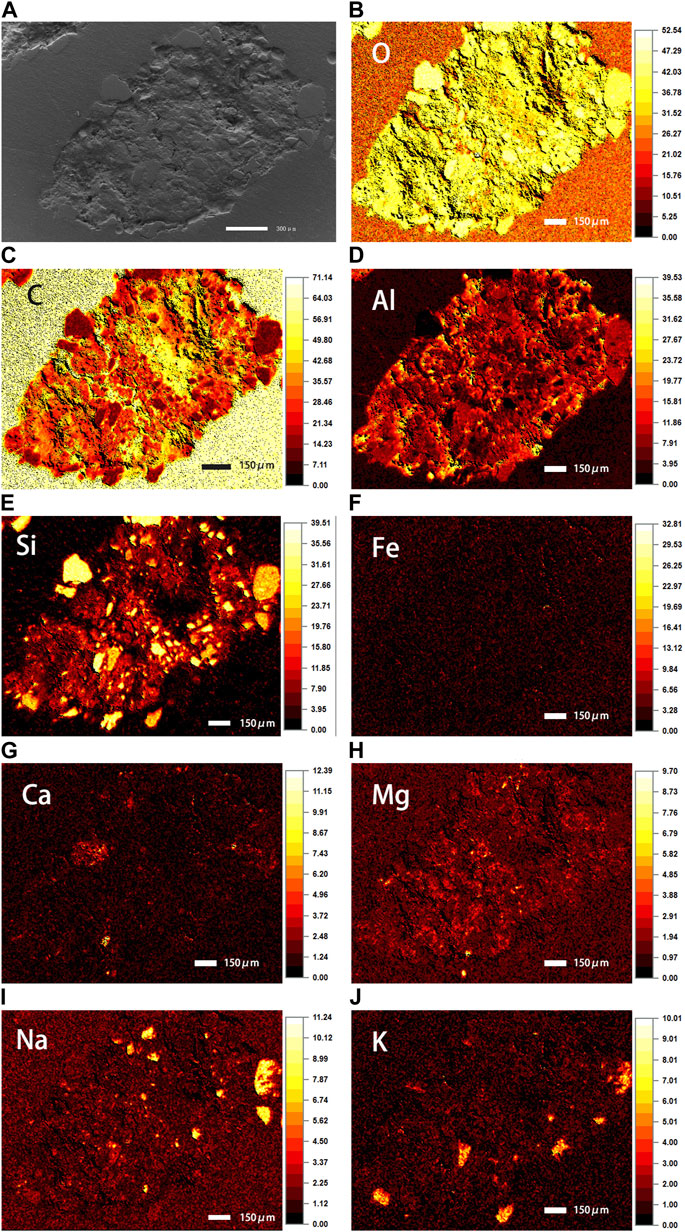
FIGURE 5. SEM-EDS analysis of compound soil aggregates that has undergone 9 years of planting. SEM image of the sample (A), and corresponding X-ray maps of O (B), C (C), Al (D), Si (E), Fe (F), Ca (G), Mg (H), Na (I), and K (J).
The interaction between iron-aluminum oxide and clay minerals is closely related to the cementation of soil aggregates (Barral et al, 1998; Amézketa et al, 1999; Duiker et al, 2003; Molina et al, 2001). For the new “artificial soil” with low organic matter content and high content of clay minerals and iron-aluminum oxides (Alc: 2.17–2.07 mg/kg; Fec: 4.92–3.97 mg/kg) in the compound soil (Table 4), the formation of soil aggregates mainly depends on the cohesive force within the clay and the cementation of iron-aluminum oxide (Barral et al, 1998; Choobbasti et al, 2017; Skjemstad et al, 1993). This study found that iron oxides have obvious distribution characteristics in the compound soil aggregates: Fe is mainly concentrated at the outside of the aggregates, and the surface Fe aggregates become more and more abundant with increased years of cultivation (Figure 5). The free iron oxide in the compound soil aggregates showed a small downward trend with the increase in years of cultivation (Table 4). This is mainly because the soft rock contains minerals rich in Fe, adding the soft rock to the aeolian sandy soil increases the content of free iron oxide in the compound soil. Since iron oxide is very active in the soil environment and can move, under the continuous action of soil roots, leaching and redox, free iron oxide continuously enters the soil solution and adsorbs to the surface of the aggregate, effectively promoting the cementation of soil aggregates (Choobbasti et al, 2015; Muggler et al, 1999; Barberis et al, 1991). On the other hand, as a redox sensitive element, Fe is continuously enriched at the surface of the aggregate due to the difference of redox conditions on the inside and the outside of the compound soil aggregates and due to the lack of pores in the aggregate (Yaghi et al, 2013; Huang et al, 2016). This also leads to positively charged iron oxides on the surface of the compound soil aggregates becoming more easily cemented together with the negatively charged aggregates by electrostatic forces, causing the soil aggregate particle size to increase (Choobbasti et al, 2018). With increased years of cultivation, the free iron oxide in the compound soil ages, the free iron oxide is continuously reduced, and the iron oxide in the cemented state is gradually increased, which also reveals the soil formation process of the compound soil. In general, iron plays an important role as a “bridge builder” in the development of compound soil aggregates (Giovannini et al, 1976; Jiang et al, 2015).
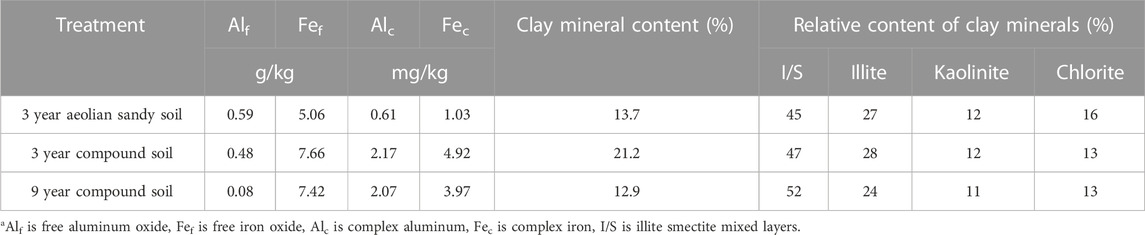
TABLE 4. Analytical results of cementing materials content in aeolian sandy soil and compound soilsa
Compared with compound soil, the distribution characteristics of clay minerals and aluminum oxides in aeolian sandy soils are not significantly different, but iron oxides exhibit a certain distribution on the surface and inside of aeolian sandy soil aggregates (Table 3), showing the difference in the distribution of cementing agents between the aeolian sandy soil and compound soil. The content of free iron oxide and complexed iron oxide is small, indicating that the aggregate cementation strength in aeolian sandy soil is low, which also illustrates in a different way the difference between the redox system and the pore channels inside soil aggregates from aeolian sandy soil and compound soil. When soft rock is compounded and mixed with the aeolian sandy soil, the micro-structural characteristics of the original aeolian sandy soil with a single-grain barrier are changed, which promotes the development of “new man-made soil” to form bridges, fill microaggregates, and promote good structural characteristics, mainly due to the positive effect of the cementing materials in the soft rock on soil formation and development of the compound soil.
With the same number of cultivation years, the clay mineral content in the compound soil is higher than that in the aeolian sandy soil, and the clay minerals in the compound soil and the aeolian sandy soil are mainly water-sensitive illite smectite mixed layers. This is mainly because soft rock contains abundant water-sensitive clay minerals (Li et al, 2014; Ma et al, 2016). These clay minerals have strong hydrophilicity, specific surface area and cation exchange capacity (Van et al, 1995; Joussein et al, 2004). The surface of the clay particles has strong hydrophilicity, which can absorb water and nutrients in the soil, forming a cohesive substance and promoting the formation of soil aggregates (Ghadakpour et al, 2020). In addition, clay particles can also provide support force to promote the stability of soil aggregates. After soft rock is used as a remediation material and compounded and mixed with aeolian sandy soil, under the action of continuous leaching and weathering, the soft rock gradually disintegrates, and the clay minerals are continuously released and redistributed (Li et al, 2017; Ribeiro et al, 2018). The clay minerals adsorb to the minerals in the aeolian sandy soil and improve its surface characteristics, which promotes the development of microaggregates in compound soil.
5 Conclusion
In this study, SEM-EDS was used to characterize the microstructure and elemental distribution of soil aggregates in aeolian sandy soils and compound soil containing a mixture of soft rock and aeolian sandy soil, and the formation and development and aggregation of cemented materials in compound soil aggregates were studied. The results show that when soft rock and aeolian sandy soil are compounded and mixed, the micro-structure of the original aeolian sandy soil, which is primarily single granular barrier, is changed, promoting the formation and development of soil and forms soil aggregates with good structural characteristics. Compared with aeolian sandy soil, the cementing agents in the compound solid aggregates are mainly clay minerals, aluminum cements, iron cements, and calcium cements. During soil development, many aggregates are further cemented into larger soil aggregates. Iron oxides are fixed by combining with organic matter, which further strengthens the stability and development of soil aggregates. The iron oxides in the compounded soil play a role similar to “bolts” during the development of soil aggregates. This study provides a theoretical basis for the study of the formation process and stabilization mechanism of soil aggregates driven by cementing agents. This study also has some shortcomings. It only analyzed the surface morphology characteristics of the complex soil, and did not detect the structures below the surface. Quantitative research needs to be strengthened in future studies.
Data availability statement
The original contributions presented in the study are included in the article/Supplementary Material, further inquiries can be directed to the corresponding author.
Author contributions
ZS and ZL wrote the main manuscript. JH and ZS designed the experiment. ZL performed the experiments and collected the data. HW and HZ prepared the figures. ZS and JY contributed the statistical analyses. All authors contributed to the article and approved the submitted version.
Funding
This research was supported by the Fund for Less Developed Regions of the National Natural Science Foundation of China (No. 42167039), the Technology Innovation Center for Land Engineering and Human Settlement Environment, Shaanxi Land Engineering Construction Group Co., Ltd., and Xi’an Jiaotong University (2021WHZ0091), and the Scientific Research Item of Shaanxi Provincial Land Engineering Construction Group (DJTD 2022-3, DJNY 2022-15, and DJNY 2022-35).
Acknowledgments
The authors gratefully acknowledge researchers at the Shaanxi Provincial Land Engineering Construction Group, for their help with the field experiments.
Conflict of interest
Authors ZS, ZL, JH, HW, and HZ were employed by Shaanxi Land Engineering Construction Group Co., Ltd.
The authors declare that this study received funding from Shaanxi Land Engineering Construction Group Co., Ltd. The funder had the following involvement in the study: study design and preparation of the manuscript.
The remaining author declares that the research was conducted in the absence of any commercial or financial relationships that could be construed as a potential conflict of interest.
Publisher’s note
All claims expressed in this article are solely those of the authors and do not necessarily represent those of their affiliated organizations, or those of the publisher, the editors and the reviewers. Any product that may be evaluated in this article, or claim that may be made by its manufacturer, is not guaranteed or endorsed by the publisher.
References
Afrakoti, M. T. P., Choobbasti, A. J., Ghadakpour, M., and Kutanaei, S. S. (2020). Investigation of the effect of the coal wastes on the mechanical properties of the cement-treated sandy soil. Constr. Build. Mater. 239, 117848. doi:10.1016/j.conbuildmat.2019.117848
Amézketa, E. (1999). Soil aggregate stability: A review. J. Sustain. Agric. 14, 83–151. doi:10.1300/j064v14n02_08
Arya, L. M., and Blake, G. R. (1972). Stabilization of newly formed soil aggregates. Agron. J. 64, 177–180. doi:10.2134/agronj1972.00021962006400020015x
Barberis, E., Marsan, F. A., Boero, V., and Arduino, E. (1991). Aggregation of soil particles by iron oxides in various size fractions of soil b horizons. J. Soil Sci. 42, 535–542. doi:10.1111/j.1365-2389.1991.tb00100.x
Barral, M., Arias, M., and Guerif, J. (1998). Effects of iron and organic matter on the porosity and structural stability of soil aggregates. Soil Tillage Res. 46, 261–272. doi:10.1016/s0167-1987(98)00092-0
Bazhenov, M. L., Chauvin, A., Audibert, M., and Levashova, N. (1993). Permian and triassic paleomagnetism of the southwestern tien Shan: Timing and mode of tectonic rotations. Earth Planet. Sci. Lett. 118, 195–212. doi:10.1016/0012-821x(93)90168-9
Bosch-Serra, À. D., Yagüe, M., Poch, R. M., Molner, M., Junyent, B., and Boixadera, J. (2017). Aggregate strength in calcareous soil fertilized with pig slurries. Eur. J. Soil Sci. 68, 449–461. doi:10.1111/ejss.12438
Chaney, K., and Swift, R. S. (1986b). Studies on aggregate stability 11 The effect of humic substances on the stability of re-formed soil aggregates. Eur. J. Soil Sci. 37, 337–343. doi:10.1111/j.1365-2389.1986.tb00036.x
Chaney, K., and Swift, R. (1986a). Studies on aggregate stability. I. Re-formation of soil aggregates. J. Soil Sci. 37, 329–335. doi:10.1111/j.1365-2389.1986.tb00035.x
Choobbasti, A. J., and Kutanaei, S. S. (2017). Microstructure characteristics of cement-stabilized sandy soil using nanosilica. J. Rock Mech. Geotechnical Eng. 9 (5), 981–988. doi:10.1016/j.jrmge.2017.03.015
Choobbasti, A. J., Vafaei, A., and Kutanaei, S. S. (2015). Mechanical properties of sandy soil improved with cement and nanosilica. Open Eng. 5, 111–116. doi:10.1515/eng-2015-0011
Choobbasti, A. J., Vafaei, A., and Soleimani, K. S. (2018). Static and cyclic triaxial behavior of cemented sand with nanosilica. J. Mater. Civ. Eng. 30 (10), 04018269. doi:10.1061/(ASCE)MT.1943-5533.0002464
Diaz, E., Roldan, A., Lax, A., and Albaladejo, J. (1994). Formation of stable aggregates in degraded soil by amendment with urban refuse and peat. Geoderma 63, 277–288. doi:10.1016/0016-7061(94)90069-8
Duiker, S. W., Rhoton, F. E., Torrent, J., Smeck, N. E., and Lal, R. (2003). Iron (hydr) oxide crystallinity effects on soil aggregation. Soil Sci. Soc. Am. J. 67, 606–611. doi:10.2136/sssaj2003.6060
Fakhrabadi, G. A., Ghadakpour, M., Choobbasti, A. J., and Kutanaei, S. S. (2021). Evaluating the durability, microstructure and mechanical properties of a clayey-sandy soil stabilized with copper slag-based geopolymer against wetting-drying cycles. Bull. Eng. Geol. Environ. 80, 5031–5051. doi:10.1007/s10064-021-02228-z
Falsone, G., Celi, L., and Bonifacio, E. (2007). Aggregate formation in chloritic and serpentinitic alpine soils. Soil Sscience 172, 1019–1030. doi:10.1097/ss.0b013e31815778a0
Guénet, H., Davranche, M., Vantelon, D., Pédrot, M., Al-Sid-Cheikh, M., Dia, A., et al. (2016). Evidence of organic matter control on as oxidation by iron oxides in riparian wetlands. Chem. Geol. 439, 161–172. doi:10.1016/j.chemgeo.2016.06.023
Gale, W., Cambardella, C., and Bailey, T. (2000). Root-derived carbon and the formation and stabilization of aggregates. Soil Sci. Soc. Am. J. 64, 201–207. doi:10.2136/sssaj2000.641201x
Garbout, A., Munkholm, L. J., and Hansen, S. B. (2013). Temporal dynamics for soil aggregates determined using x-ray ct scanning. Geoderma 204, 15–22. doi:10.1016/j.geoderma.2013.04.004
Ghadakpour, M., Choobbasti, A. J., and Kutanaei, S. S. (2020). Experimental study of impact of cement treatment on the shear behavior of loess and clay. Arabian J. Geoscience 13 (4), 184. doi:10.1007/s12517-020-5181-7
Giovannini, G., and Szqui, P. (1976). Iron and aluminium as cementing substances of soil aggregates: I. Acetylacetone in benzene as an extractant of fractions of soil iron and aluminium. J. Soil Sci. 27, 140–147. doi:10.1111/j.1365-2389.1976.tb01984.x
Gislason, S. R., Arnórsson, S., and Armannsson, H. (1996). Chemical weathering of basalt in southwest Iceland; effects of runoff, age of rocks and vegetative/glacial cover. Am. J. Sci. 296, 837–907. doi:10.2475/ajs.296.8.837
Han, J., Liu, Y., and Zhang, Y. (2015). Sand stabilization effect of feldspathic sandstone during the fallow period in mu us sandy land. J. Geogr. Sci. 25, 428–436. doi:10.1007/s11442-015-1178-7
Han, J., Xie, J., and Zhang, Y. (2012). Potential role of feldspathic sandstone as a natural water retaining agent in mu us sandy land, northwest China. Chin. Geogr. Sci. 22, 550–555. doi:10.1007/s11769-012-0562-9
Hapca, S., Baveye, P. C., Wilson, C., Lark, R. M., and Otten, W. (2015). Three-dimensional mapping of soil chemical characteristics at micrometric scale by combining 2d sem-edx data and 3d x-ray ct images. PloS One 10, e0137205. doi:10.1371/journal.pone.0137205
Haynes, R., and Francis, G. (1993). Changes in microbial biomass c, soil carbohydrate composition and aggregate stability induced by growth of selected crop and forage species under field conditions. J. Soil Sci. 44, 665–675. doi:10.1111/j.1365-2389.1993.tb02331.x
Hou, T., Berry, T. D., Singh, S., Hughes, M. N., Tong, Y., Papanicolaou, A. T., et al. (2018). Control of tillage disturbance on the chemistry and proportion of raindrop-liberated particles from soil aggregates. Geoderma 330, 19–29. doi:10.1016/j.geoderma.2018.05.013
Huang, X., Jiang, H., Li, Y., Ma, Y., Tang, H., Ran, W., et al. (2016). The role of poorly crystalline iron oxides in the stability of soil aggregate-associated organic carbon in a rice–wheat cropping system. Geoderma 279, 1–10. doi:10.1016/j.geoderma.2016.05.011
Janalizadeh, D. C., Soleimani, K. S., and Taslimi, P. A. M. (2019a). Modeling of compressive strength of cemented sandy soil. J. Adhesion Sci. Technol. 33 (8), 791–807. doi:10.1080/01694243.2018.1548535
Janalizadeh, F., Choobbasti, A., Farrokhzad, F., Nadimi, A., and Soleimani, K. S. (2019b). Effects of copper sludge on cemented clay using ultrasonic pulse velocity. J. Adhesion Sci. Technol. 33 (4), 433–444. doi:10.1080/01694243.2018.1471842
Jastrow, J. (1996). Soil aggregate formation and the accrual of particulate and mineral-associated organic matter. Soil Biol. Biochem. 28, 665–676. doi:10.1016/0038-0717(95)00159-x
Jiang, X., Bol, R., Willbold, S., Vereecken, H., and Klumpp, E. (2015). Speciation and distribution of p associated with fe and al oxides in aggregate-sized fraction of an arable soil. Biogeosciences 12, 6443–6452. doi:10.5194/bg-12-6443-2015
Jiménez-Pinilla, P., Mataix-Solera, J., Arcenegui, V., Delgado, R., Martín-García, J. M., Lozano, E., et al. (2016). Advances in the knowledge of how heating can affect aggregate stability in mediterranean soils: A xdr and sem-edx approach. Catena 147, 315–324. doi:10.1016/j.catena.2016.07.036
Joussein, E., Kruyts, N., Righi, D., Petit, S., and Delvaux, B. (2004). Specific retention of radiocesium in volcanic ash soils devoid of micaceous clay minerals. Soil Sci. Soc. Am. J. 68, 313–319. doi:10.2136/sssaj2004.3130
Koutenaei, E. R. Y., Choobbasti, A. J., and Kutanaei, S. S. (2021). Triaxial behaviour of a cemented sand reinforced with Kenaf fibres. Eur. J. Environ. Civ. Eng. 25 (7), 1268–1286. doi:10.1080/19648189.2019.1574607
Lado, M., Ben-Hur, M., and Shainberg, I. (2004). Soil wetting and texture effects on aggregate stability, seal formation, and erosion. Soil Sci. Soc. Am. J. 68, 1992–1999. doi:10.2136/sssaj2004.1992
Li, C., Zhang, T., and Wang, L. (2014). Mechanical properties and microstructure of alkali activated pisha sandstone geopolymer composites. Constr. Build. Mater. 68, 233–239. doi:10.1016/j.conbuildmat.2014.06.051
Li, T., Wang, H., Chen, X., and Zhou, J. (2017). Soil reserves of potassium: Release and availability to lolium perenne in relation to clay minerals in six cropland soils from eastern China. Land Degrad. Dev. 28, 1696–1703. doi:10.1002/ldr.2701
Ma, W., and Zhang, X. (2016). Effect of pisha sandstone on water infiltration of different soils on the Chinese loess plateau. J. Arid Land 8, 331–340. doi:10.1007/s40333-016-0122-8
Martin, M. W., Clavero, J., and Mpodozis, C. (1999). Late paleozoic to early jurassic tectonic development of the high andean principal cordillera, el indio region, Chile (29–30 s). J. S. Am. Earth Sci. 12, 33–49. doi:10.1016/s0895-9811(99)00003-6
Molina, N., Caceres, M., and Pietroboni, A. (2001). Factors affecting aggregate stability and water dispersible clay of recently cultivated semiarid soils of Argentina. Arid Land Res. Manag. 15, 77–87. doi:10.1080/15324980118369
Muggler, C. C., van Griethuysen, C., Buurman, P., and Pape, T. (1999). Aggregation, organic matter, and iron oxide morphology in oxisols from minas gerais, Brazil. Soil Sci. 164, 759–770. doi:10.1097/00010694-199910000-00007
Oades, J. (1993). The role of biology in the formation, stabilization and degradation of soil structure. Geoderma 56, 377–400. doi:10.1016/0016-7061(93)90123-3
Ren, W., Wang, M., and Zhou, Q. (2011). Adsorption characteristics and influencing factors of chlorimuron-ethyl in two typical Chinese soils. Soil Sci. Soc. Am. J. 75, 1394–1401. doi:10.2136/sssaj2010.0228
Ribeiro, S. P. d. S., Cescon, L. d. S., Ribeiro, R. Q. C. R., Landesmann, A., Estevão, L. R. d. M., and Nascimento, R. S. V. (2018). Effect of clay minerals structure on the polymer flame retardancy intumescent process. Appl. Clay Sci. 161, 301–309. doi:10.1016/j.clay.2018.04.037
Roshan, H. K., Choobbasti, A. J., and Kutanaei, S. S. (2020). Evaluation of the impact of fiber reinforcement on the durability of lignosulfonate stabilized clayey sand under wet-dry condition. Transp. Geotech. 23, 100359. doi:10.1016/j.trgeo.2020.100359
Roshan, H. K., Choobbasti, A. J., Kutanaei, S. S., and Fakhrabadi, A. (2022). The effect of adding polypropylene fibers on the freeze-thaw cycle durability of lignosulfonate stabilised clayey sand. Cold Regions Sci. Technol. 193, 103418. doi:10.1016/j.coldregions.2021.103418
Sayen, S., Mallet, J., and Guillon, E. (2009). Aging effect on the copper sorption on a vineyard soil: Column studies and sem–eds analysis. J. Colloid Interface Sci. 331, 47–54. doi:10.1016/j.jcis.2008.11.049
Schomburg, A., Verrecchia, E. P., Guenat, C., Brunner, P., Sebag, D., and Le Bayon, R. C. (2018). Rock-eval pyrolysis discriminates soil macro-aggregates formed by plants and earthworms. Soil Biol. Biochem. 117, 117–124. doi:10.1016/j.soilbio.2017.11.010
Shi, Y., Chen, X., and Shen, S. (2002). Mechanisms of organic cementing soil aggregate formation and its theoretical models. J. Appl. Ecol. 13, 1495–1498.
Skjemstad, J., Janik, L. J., Head, M., and McClure, S. G. (1993). High energy ultraviolet photo-oxidation: A novel technique for studying physically protected organic matter in clay-and silt-sized aggregates. J. Soil Sci. 44, 485–499. doi:10.1111/j.1365-2389.1993.tb00471.x
Sun, Z., and Han, J. (2018). Effect of soft rock amendment on soil hydraulic parameters and crop performance in mu us sandy land, China. Field Crops Res. 222, 85–93. doi:10.1016/j.fcr.2018.03.016
Sun, Z., Han, J., and Wang, H. (2019). Soft rock for improving crop yield in sandy soil in the mu us desert, China. Arid Land Res. Manag. 33, 136–154. doi:10.1080/15324982.2018.1522385
Sun, Z. H., Han, J. C., Wang, H. Y., Zhang, R. Q., Sun, Y. Y., Wei, J., et al. (2021). Use and economic benefit of soft rock as an amendment for sandy soil in Mu Us Sandy Land, China. Arid Land Res. Manag. 35, 15–31. doi:10.1080/15324982.2020.1765221
Terpstra, R. (1990). Formation of new aggregates and weed seed behaviour in a coarse-and in a fine-textured loam soil. A laboratory experiment. Soil Tillage Res. 15, 285–296. doi:10.1016/0167-1987(90)90085-r
Van Oss, C., and Giese, R. (1995). The hydrophilicity and hydrophobicity of clay minerals. Clays Clay minerals 43, 474–477. doi:10.1346/ccmn.1995.0430411
Wang, N., Xie, J., and Han, J. (2013). A sand control and development model in sandy land based on mixed experiments of arsenic sandstone and sand: A case study in mu us sandy land in China. Chin. Geogr. Sci. 23, 700–707. doi:10.1007/s11769-013-0640-7
Wang, W., Kravchenko, A., Smucker, A., Liang, W., and Rivers, M. (2012). Intra-aggregate pore characteristics: X-ray computed microtomography analysis. Soil Sci. Soc. Am. J. 76, 1159–1171. doi:10.2136/sssaj2011.0281
Wang, Y., Zheng, J., Zhang, X., and Zhang, Y. (2009). A structure-based investigation on the binding interaction of hydroxylated polycyclic aromatic hydrocarbons with DNA. Acta Agrestia Sin. 17, 250–257. doi:10.1016/j.tox.2009.06.015
Watteau, F., Villemin, G., Bartoli, F., Schwartz, C., and Morel, J.-L. (2012). 0–20 μm aggregate typology based on the nature of aggregative organic materials in a cultivated silty topsoil. Soil Biol. Biochem. 46, 103–114. doi:10.1016/j.soilbio.2011.11.021
Wiesmeier, M., Steffens, M., Mueller, C., Kölbl, A., Reszkowska, A., Peth, S., et al. (2012). Aggregate stability and physical protection of soil organic carbon in semi-arid steppe soils. Eur. J. Soil Sci. 63, 22–31. doi:10.1111/j.1365-2389.2011.01418.x
Wilson, C., Cloy, J. M., Graham, M. C., and Hamlet, L. (2013). A microanalytical study of iron, aluminium and organic matter relationships in soils with contrasting hydrological regimes. Geoderma 202, 71–81. doi:10.1016/j.geoderma.2013.03.020
Yaghi, N., and Hartikainen, H. (2013). Enhancement of phosphorus sorption onto light expanded clay aggregates by means of aluminum and iron oxide coatings. Chemosphere 93, 1879–1886. doi:10.1016/j.chemosphere.2013.06.059
Yu, X., Fu, Y., and Lu, S. (2017). Characterization of the pore structure and cementing substances of soil aggregates by a combination of synchrotron radiation x-ray micro-computed tomography and scanning electron microscopy. Eur. J. Soil Sci. 68, 66–79. doi:10.1111/ejss.12399
Keywords: aeolian sandy soil, compound soil, soil aggregate, cementing agent, environmental scanning electron microscopy
Citation: Sun Z, Liu Z, Han J, Wang H, Zhang H and Yan J (2023) Long-term effects of soft rock amendment on changes of soil aggregate cementing agents of sandy soil by SEM-EDS. Front. Environ. Sci. 11:1207781. doi: 10.3389/fenvs.2023.1207781
Received: 18 April 2023; Accepted: 29 May 2023;
Published: 15 June 2023.
Edited by:
Kaibo Wang, Chinese Academy of Sciences (CAS), ChinaReviewed by:
Guowei Pang, Northwest University, ChinaSaman Soleimani Kutanaei, Babol Noshirvani University of Technology, Iran
Yurui Li, Chinese Academy of Sciences (CAS), China
Copyright © 2023 Sun, Liu, Han, Wang, Zhang and Yan. This is an open-access article distributed under the terms of the Creative Commons Attribution License (CC BY). The use, distribution or reproduction in other forums is permitted, provided the original author(s) and the copyright owner(s) are credited and that the original publication in this journal is cited, in accordance with accepted academic practice. No use, distribution or reproduction is permitted which does not comply with these terms.
*Correspondence: Jichang Han, hanjc_sxdj@126.com