Heavy metal levels and sources in suspended particulate matters of the glacier watersheds in Northeast Tibetan Plateau
- 1School of Geography and Ecotourism, Southwest Forestry University, Kunming, China
- 2State Key Laboratory of Cryosphere Sciences/Northwest Institute of Eco- Environment and Resources, Chinese Academy of Sciences, Lanzhou, China
- 3Southwest Research Center of Ecological Civilization (State Forestry and Grassland Administration), Kunming, China
- 4Department of Watershed Sciences, Utah State University, Logan, UT, United States
This study collected summer meltwater runoff samples from several glacier watersheds of the northeast Tibetan Plateau during June-July 2017, and measured the concentrations of 17 trace elements (Li, Be, Sc, V, Cr, Co, Ni, Cu, Zn, Ga, Rb, Mo, Cd, In, Sb, Cs, Ba) in meltwater suspended particulate matter (SPM), in order to reveal the elemental concentration, spatial distribution, and water quality in remote glacier watershed under regional anthropogenic activities. Results showed that, the concentration of heavy metal elements was relatively high in Yuzhufeng Glacier basin, ranging from 0.57 μg/L (In) to 1,551.6 μg/L (Ba), whereas in Qiyi Glacier basin it was the lowest, ranging from 0.02 to 85.05 μg/L; and relatively medium in other glacier watersheds, with total elemental concentration varying from 1,503.9 to 1726.2 μg/L. Moreover, enrichment factors (EFs) of SPM heavy metals showed significantly higher value in the downstream than that of upper glacier region of the watershed. Most heavy metals with low EFs mainly originated from crust dust, while others with higher EFs (e.g., Cd, Sb) probably originated from anthropogenic sources. Spatially, the EFs of heavy metals were higher in Yuzhufeng and Laohugou Glacier basins; while in other regions the EFs were relatively low, which may be caused by regional land-surface and atmospheric environmental differences surrounding the various glacier watersheds. Compared with other remote locations in global range, heavy metals level (e.g., Cu, Ni, and Zn) in this region is relatively higher. Meanwhile, we find that, though the water quality of the glacier basin in northeast Tibetan Plateau was relatively clean and pollution-free, it is still obviously affected by regional anthropogenic activities. Mining activities, transportation and natural weathering and erosion processes in the study areas have important effects on the content of heavy metal pollutants of river-water SPM in the glacier watershed. Moreover, backward air-mass trajectories demonstrated the potential atmospheric pollutants transport from the surrounding cities and suburbs, to deposit in the snowpack and glaciers, and then melted out and released into meltwater runoff. This study provides a new perspective on more complete view of heavy metals distribution in glacier watershed, and new understanding for the cryosphere water environment evaluation in the Tibetan Plateau region.
Introduction
In the hydrosphere cycle, chemical phases are mainly distributed in three important sections as: dissolved or filterable (d < 0.45 µm), suspended particulate matter (abbreviated to SPM, d > 0.45 µm), sediment (Fergusson, 1990). The SPM in river water is normally derived from the weathering crust and soil of the catchment area, and is the product of the river basin surface process such as weathering, pedogenic, and surface erosion process (Qu et al., 1984; Liu et al., 2007; Hao et al., 2010; Li X. et al., 2021; Liu et al., 2021). The chemical characteristics of SPM may indicate the source area and provide a basis for the pollution sources of rivers (Hao et al., 2010). Thus it has acted as an important medium for studying surface geochemistry characteristics and influencing factors (Liu et al., 2007). The preliminary studies of large rivers in the world have found that SPM (e.g., mineral dust and oxide or hydroxide particles of aluminum, manganese and iron) is the main transport carrier for heavy metals in rivers (Wang and Zhang, 1994; McBride, 2000; Zhou et al., 2002; Woitke et al., 2003; He et al., 2003; Gao et al., 2011; Miles et al., 2021; Liu et al., 2021). Environmental pollution caused by excessive heavy metal elements in river water will seriously affect water quality (Li X. et al., 2021).
In general, trace metals (including heavy metals) occur naturally in the atmosphere, terrestrial and aquatic environments, and anthropogenic activities can lead to the release of heavy metals. Many factors can influence the anthropogenic pollutant release behavior of heavy metal elements in the environment, such as chemical smelting processes, land-use changes, and metal mining activities (Huser et al., 2011). Ross and Sherrell, (1999) have revealed that the concentration of dissolved trace metals was usually correlated with water flow. Colloidal and particle size fractions of trace metals (such as Fe, Cu, Zn, Pb) change independently with time, while other metals (e.g., V, As) were often associated with colloidal or particulate iron fractions (Wällstedt et al., 2010). Research on the distribution of dissolved heavy metals and particulate metals in Swedish river water has shown that some metal elements (Cu, Zn, Cd, Cr and Ni) in particulate components are close to or less than 25% of the total metal concentration (Köhler, 2010).
The Tibetan Plateau (TP), known as “Asian water tower,” is sensitive to anthropogenic impact due to its unique landform, fragile ecosystem, and specific climate and environment. It is the headwaters of the main large rivers in Asia, which are the water sources for about 40% of the world’s population. Many investigations on metal elements have been widely carried out in different environmental media on the TP. Previous studies have found that there are elevated concentrations of potentially harmful metals in the TP water environment that human beings depend on for survival (Huang et al., 2018). Moreover, a large amount of studies have observed that heavy metals widely exist in various environment factors of the TP such as ice core and snow (Wei et al., 2019; Gabrielli et al., 2020; Potocki et al., 2022), aerosols (Lee et al., 2008; Liu et al., 2011), wet precipitation of rainfall and snowfall (Cong et al., 2010; Liu et al., 2013), river and lake deposition (Guo et al., 2018; Yan et al., 2018; Zhao et al., 2018). However, there is still a lack of relevant studies on glacial meltwater runoff (Li et al., 2012; Dong and Kang, 2017). During recent years, with the accelerated glacier melting under global warming, the changes of various chemical components and their climate environment effect on glacial meltwater runoff on the TP have gradually become a hot topic (Li et al., 2020). After heavy metal elements enter rivers, the change of their fate, occurrence form, content and composition and possible impacts are uncertain (Cong et al., 2007), which will inevitably lead to water quality and downstream aquatic ecosystem change. Therefore, study of SPM heavy metals in the glacier watershed of northern TP can provide an important basis for the understanding and evaluation of the water quality environment in the source region of the Alpine river (Cong et al., 2007; Zhu, 2014; Du et al., 2015).
The Qilian Mountains and Kunlun Mountains in northern TP are climate-sensitive and complex environments. In the high-altitude glacier area, they are the main water sources for the three inland river basins (Shiyang River, Heihe River, and Shule River) in Hexi, surrounded by large deserts and Gobi, with the unique topography, the production and emission of adjacent urban and rural regions, and the fragile ecosystem (Du et al., 2017; Li et al., 2018; Wei et al., 2019). At the same time, the exposed bedrock and strong physicochemical weathering in the glacier region, will lead to bedrock fragmentation and the dissolution of a large number of heavy metals and other chemical elements into the glacier basin, which will influence the water quality supply to downstream oasis and related human production and life.
In this study, SPM heavy metals in glacier meltwater runoff samples are investigated in a wide range of glacier watershed in different areas of the northern TP, including the glaciers located both in Qilian Mountains and Kunlun Mountains. The total concentrations and EFs of trace elements (including heavy metals) are measured. Moreover, the study region is divided into five regions, typically including the Dabanshan snowpack basin (DS) and Lenglongling Glacier basin (LG), Heihe River upper glacier basin (HG), Qiyi Glacier basin (QY), Laohugou Glacier No.12 basin (LG12) and Yuzhufeng Glacier basin (YG). This work aims to investigate the concentration, enrichment factors (EFs), and spatial distribution characteristics of heavy metals in SPM of glacier watershed in northeast TP, and then to evaluate the general water quality and water environment through heavy metals element composition and their content level analysis.
Fieldwork sampling and lab analysis
Study area and sample collection
In this study, the glacier watersheds in the Qilian Mountains (including the Dabanshan snowpack basin, Lenglongling Glacier basin, Heihe River basin, Qiyi Glacier basin, Laohugou Glacier basin) in the northern TP are selected as the main study region, while the Yuzhufeng basin in the Kunlun Mountains is used as the reference study region for spatial comparison (Figure 1). A total of 31 SPM samples were collected from meltwater rivers in remote glacier and snowpack basins (Table 1 and Figure 1), which are mainly located in the mid-latitude north temperate zone, deep inland, far from the ocean. The climate type belongs to the continental alpine semi-humid mountain climate, winter is long and cold dry, summer is short and cool humid, annual precipitation is concentrated from May to September. The main water storage in the Qilian Mountains is the glacier and snowpacks, and glacier meltwater flows out to form the Qilian Mountains drainage system. The sampling time was from June to July 2017, and the sampling points were arranged in the mainstream of the source region, where the river was open and the water flow was gentle. These samples are representative enough to reflect the overall distribution level of heavy metals and water quality in the studied glacier regions for the following reasons: (1) These six research areas cover the alpine areas of the northeastern TP and have relatively complete spatial and climatic representation; (2) by selecting the glaciers in the Qilian Mountains, which are close to human settlements such as the Hexi Corridor, and comparing them with Yuzhufeng Glacier basin, which is far away from the influence of human activities, can comprehensively evaluate the impact degree of human activities on the levels of heavy metals and water quality in the meltwater of remote glaciers in the northeastern TP; (3) For each watershed, the glacial meltwater samples cover different hydrological sections (upstream, midstream, and downstream), which can reflect the distribution conditions of heavy metal levels in the overall glacier watershed.
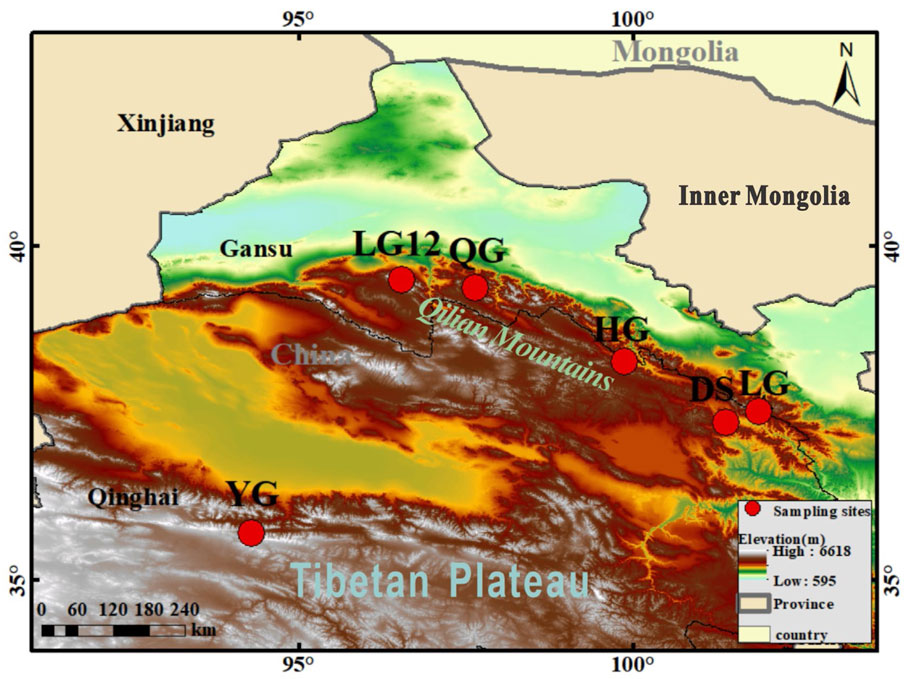
FIGURE 1. Study region and sampling points in the glacier watersheds of the northeast Tibetan Plateau.
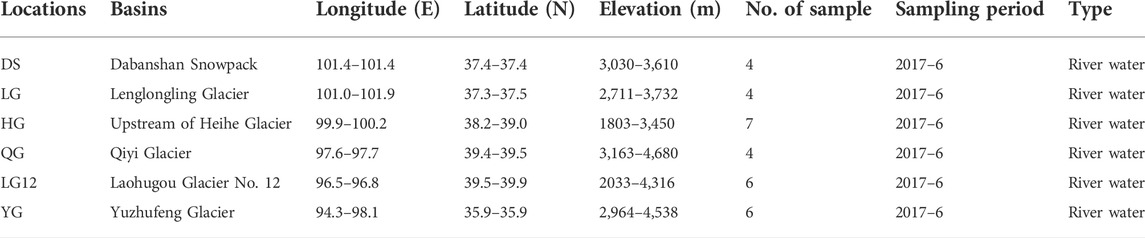
TABLE 1. Location information of river suspended particulate matter sampling points in glacier basins of different regions in the northeast Tibetan Plateau.
All sampling tools and bottles were strictly cleaned according to standard procedures. To prevent sample contamination and ensure the accuracy of experimental measurements, researchers must wear specially tailored clean polypropylene protective suits and gloves throughout the sampling process. Then, the samples can be placed in low-density polyethylene (LDPE) bottles (Thermo Scientific). Of course, when collecting water samples, we removed floating or immersed branches, dead leaves, and then filtered through filter membranes (polyethersulfone membrane, pore size 0.45 µm) before extraction of SPM. The filter membrane should be dried, weighed, and recorded in the room before use. After cleaning and wetting the filter membrane with distilled water, the filter membrane was tiled in the Bush funnel. Shaking the water sample well every time to avoid the SPM at the bottom of the container. After filtration, the filter membrane was dried to a semi-dry state, and a blank filter membrane was left for blank analysis, then, which will be sent to the laboratory for analysis.
Before measurement, all samples were acidified by ultrapure nitric acid (0.5% V/V) to dissolve trace elements related to atmospheric particles and effectively prevent their adsorption on the bottle wall. Finally, the collected samples were sealed and frozen until they were analyzed by the Inductively Coupled Plasma Mass Spectrometer (ICP-MS, Elemental/XR). To ensure the accuracy and reliability of laboratory measurements, we need quality assurance and quality control processes, and prevent contamination of samples during sampling and laboratory analysis. Therefore, the experimenters must wear clean, dust-free plastic gloves, masks, and clean clothes throughout the process. For the concentration analysis of each element, the external standard method was used for quantitative analysis, and after the initial calibration, every 10 samples carried out for standard analysis. The detection limit of this method was 3 times of the standard deviation of repeated blank experiment. After that, the analytical laboratory of the Beijing Institute of Geology of Nuclear Industry used ICP-MS to determine the heavy metals and trace elements in SPM. The accuracy of the analysis scheme was determined by repeated measurements of an externally certified reference solution (AccuTrace™ reference standard solution). And the accuracy of this method was between 85% Cr and 105% Ni. To improve the analysis accuracy, the RSD values corresponding to the determined concentration of each element in the standard sample were all less than 10%. Finally, we determined the major chemical elements including Li, Be, Sc, V, Cr, Co, Ni, Cu, Zn, Ga, Rb, Mo, Cd, In, Sb, Cs, and Ba in the river water SPM samples from Qilian and Kunlun mountains of TP.
Enrichment factors analysis
Enrichment factors (EFs) is an important method to indicate the degree of element enrichment in environmental media and to judge and evaluate the source of elements (Huang et al., 2018). According to the size of enrichment factors, the natural and anthropogenic effects of element enrichment in river suspended particulate matter can be distinguished (Fan and Jiang, 2016). The EF is defined as the concentration ratio of a given element to that of a crustal element (e.g., Al and Sc), which is normalized to the same concentration ratio of topsoils of the TP (Li et al., 2009). The Sc element is used as the reference element in this study to calculate the EF coefficient of heavy metal elements in river suspended particulate matter (SPM). The calculation for the EF is as follows:
In Formula 1, Cx represents the concentration of heavy metal element x, Cref represents the concentration of reference element, sample and baseline represent sample and background, respectively. Previous work has showed that, when EF < 10, this element mainly originates from crustal weathering. We here considered elements with EF values between 1 and 10 as non-enriched elements, and EF values between 10 and 100 as moderately enriched elements, indicating that the concentration of this element is higher than the expected concentration of crustal elements (Dong et al., 2015; Dong and Kang, 2017; Huang et al., 2018; Dong et al., 2019; Jiao et al., 2021). Moreover, according to Sutherland, (2000), the heavy metal pollution level is divided as: EF < 2 represents the minimum enrichment, indicating no or minimum pollution; 2 ≤ EF < 5 represents slight enrichment, indicating slight pollution; 5 ≤ EF < 20 represents significant pollution; 20 ≤ EF < 40 represents high enrichment, indicating serious pollution; EF ≥ 40 represents extreme enrichment, indicating extreme pollution.
Meteorological data analysis
To further identify the migration sources and paths of atmospheric sources of heavy metal pollution in glacier basins, the meteorological reanalysis data (wind field data and air mass trajectory analysis) of the National Center for Environmental Prediction (NCEP) and National Center for Atmospheric Research (NCAR) are used. The backward air mass trajectory clustering was carried out for the five main sampling basins divided from the northeast TP in May 2017, and the wind field around the study area was simulated in four seasons from 2015 to 2017.
Results and discussion
Concentration and regional distribution of SPM heavy metals in various glacier watersheds
Average concentration, maximum (Max) and minimum (Min) of metal elements in SPM at different locations in northeast TP were calculated and compared, the results are shown in Table 2. The concentration of heavy metal elements in the five regions is as follows: Li: 4.68–90.58 μg/L; Be: 0.56–9.25 μg/L; Sc: 4.42–42.19 μg/L; V: 85.05–338.55 μg/L; Cr: 42.10–197.65 μg/L; Co: 2.72–53.99 μg/L; Ni: 9.00–127.76 μg/L; Cu: 7.72–324.92 μg/L; Zn: 34.21–533.00 μg/L; Ga: 3.06–73.89 μg/L; Rb: 16.71–471.32 μg/L; Mo: 0.27–5.39 μg/L; Cd: 0.06–3.42 μg/L; In: 0.02–0.57 μg/L; Sb: 0.54–7.88 μg/L; Cs: 1.31–124.49 μg/L; Ba: 68.68–1,551.60 μg/L. As shown in Table 2, the averaged concentrations of trace elements (Li, Be, Sc, V, Cr, Co, Ni, Cu, Zn, Ga, Rb, Mo, Cd, In, Sb, Cs, and Ba) in SPM span four order of magnitude ranging from 0.3 (In) to 990.4 (Ba) μg/L. (Table 2 and Figure 2).
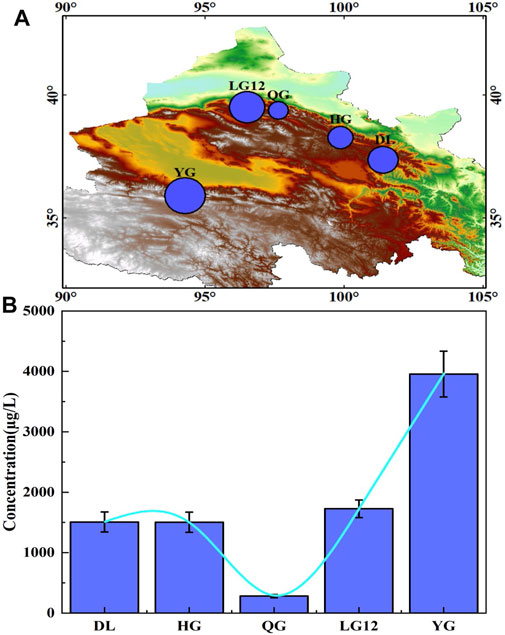
FIGURE 2. Map showing the total concentration distribution of trace elements in each glacier watershed area. (note: error bars and error lines are shown in the figure)
The total concentrations of all heavy metals measured in this study generally reflect a heterogeneous geographic distribution that varies from the northern to southern sampling locations (Figure 2). We found that the northern locations of west Kunlun Mountains (YG) had the highest total concentrations, whereas the Qilian Mountains locations (e.g., LG12, HG, QG, DL) displayed a relatively lower content (Figure 2B). Compared with the northern Qilian Mountains, the concentration of SPM heavy metals in the YG are more enriched with the highest value, and that in LG12, DS, and HG, and the QG basin decrease gradually. The spatial distribution of the total concentrations in SPM of the Qilian Mountains locations shows the order of LG12 > DL > HG > QG (see Figure 2), and the change trend of heavy metals in each region is similar (Figure 3). Such spatial heterogeneity showed the potential different environmental characteristics at the sampling locations, which were closely related to the regional geology, human activity, and land-use conditions between the various sampling locations (Pant et al., 2020). Moreover, the backward air mass trajectories showed that there were remarkable differences in the air mass origins and transport paths among the various study areas, which can explain the differences of spatial distribution in SPM metals from the perspective of atmospheric transport (Figure 10).
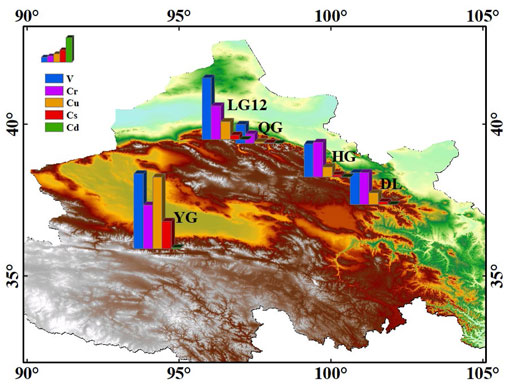
FIGURE 3. Distribution of each trace element concentration in SPM of various glacier watersheds of the study region.
The difference in the elemental concentrations is about 2–18 times between various glacier watersheds. For example, Cu, Cd, and Cs display the largest variability, as the maximum/minimum concentration ratios are 42.09, 53.42, and 94.85, respectively (Table 2 and Figure 3). By contrast, V, Cr, and Sc display the smallest variation, as the maximum/minimum concentration ratios are 3.98, 4.69, and 9.55, respectively. The origins and spatial distribution of trace elements in different glacier basins are controlled by a complex interplay of multiple factors, including aeolian dust sources, pollutants particles (e.g. Coal and biomass combustion) and land surface process. The high-altitude locations are close to the large deserts and Gobi in central Asia and can receive large amounts of aeolian dust, which could provide trace metals to the glacier basins (Dong et al., 2020). In addition, coal and biomass combustion could release various pollutants into the atmosphere, which is enriched with trace metals. Coal fly ash is characterized by Ba, Bi, Co, Cr, Cs, Mo, Ni, and, particularly, As, Cd, Ga, Pb, Sb, Ti, and Zn (Gabrielli et al., 2020), which may be an important anthropogenic contributor to SPM metals in glacier rivers through atmospheric deposition in this study.
Comparing the upstream and downstream differences of heavy metal elements in various glacier watersheds
Generally, in the remote upperstream areas of the glacier basin, the content of SPM heavy metals is usually affected by local geological structure, bedrock type, and aeolian dust deposition (Li R. et al., 2021). Besides, the regional environmental pollutants are also transported to remote areas through atmospheric circulation and enter glacial basins through wet and dry deposition (Dong et al., 2020). In this study, as shown in Figure 4, a relatively high average concentration appears in the meltwater of DS basin and the upperstream of YG basin with a relatively small anthropogenic activity, indicating that these elements may be enriched from the local bedrock or derived from aeolian dust or pollutants deposition (Pant et al., 2020). We found that there were significant differences in heavy metal elements in SPM at different glacial watersheds between upstream of high-altitude regions and downstream of low-altitude regions.
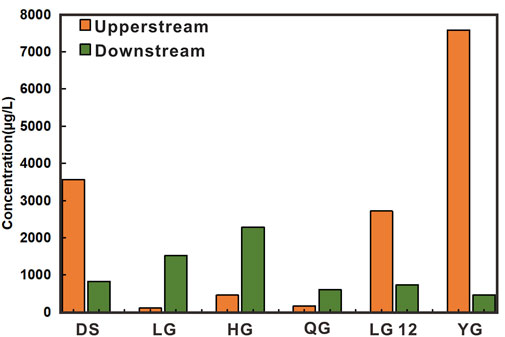
FIGURE 4. Concentrations of major heavy metals distributed in the upstream and the downstream segment of each glacier watershed basin.
In the high alpine regions, strong chemical weathering and pedogenic processes caused by glacier erosion and freezing-thawing penetration action play an important role in increase of elemental concentrations in meltwater (Dong et al., 2015; Paudyal et al., 2016a; Singh et al., 2017; Pant et al., 2020). We found that the concentration of heavy metal elements in the downstream locations of YG basin in Kunlun Mountains was higher than that in the upperstream glacier basin, which is likely to be affected by riverbed chemical weathering along the watershed, or by human activities in the nearby residential environment such as mineral water plants and highway maintenance stations.
Similarly, the heavy metal elements level in the downstream locations in QG basin in western Qilian Mountains is significantly higher than that of the upperstream. Moreover, the elements level in LG basin of eastern Qilian Mountains are also generally lower than that of the downstream locations. Such distribution in the glacier watersheds may be related to the regional geological mineralogy and human activity influence in the basin. For example, there is a huge NW-trending iron, copper, manganese and phosphorus ore belt in the geological structure of the Lenglongling (LG) area. Also, there are several mining facilities around the mountain areas. In addition, the inflow of tributaries also affects the concentration of heavy metals in the mainstream (Hao et al., 2010). Hulugou Glacier small watershed in the upper Heihe River belongs to the first-level tributary area, with glaciers, forests, grasslands, and other typical cold areas underlying surfaces (Huang et al., 2018). We found that the contents of heavy metal elements in the river water samples of Babao River (Heihe River tributary) in the Qilian Mountains were also significantly higher than that of upperstream Hulugou Glacier basin.
In addition, the concentration of heavy metals in the LG12 glacial basin in this study is lower than that in the downstream area, because most of the SPM could be carried into the middle and lower mainstream (Figure 4). In the meantime, the strong physical and chemical erosion in the migration process leads to an increase in the concentration of various chemical elements, indicating that the inflow of tributaries has an impact on the chemical element content in the mainstream. Therefore, this study confirmed that, on the whole, the content of SPM trace elements in glacier basins showed an increasing trend from upstream to downstream, mainly affected by bedrock chemical-erosion or enrichment in the catchment area, thereby causing the increased trace metals concentration. From the mainstream to the lower midstream segment, the SPM content of the river presents an irregular trend, and the influence degree of anthropogenic activities increased.
Comparison on heavy metals level of meltwater SPM with the upper glacier snowpack and other surrounding areas
In arid and semi-arid areas of northwest China, glacier/snow melt has a huge impact on runoff in glacier basins. Compared with the heavy metals concentration in the glacier basin of this work and other surrounding glacier areas in TP and Asia, we found that the upper glacier/snowpack melting has large influences on the meltwater runoff in the glacier watersheds. For example, the runoff segmentation of Baishui Glacier No.1 in Yulong Snow Mountain, Yuzhufeng Glacier in the Kunlun Mountains, and Qingbingtan Glacier No. 72 in Tianshan mountains in Xinjiang showed that the recharge of glacier melting to runoff is 53.4%, 74.8%, and 56.64% respectively (Song et al., 2015; Yang et al., 2016). Thus the release of atmospheric deposited materials stored in glacier/snowpack may have a large effect on the concentration and occurrence form of trace metal elements in meltwater runoff. Chemical matters released from snow-ice melting may account for 50%–80% of the total release during the glacier melting period (Johannessen et al., 1978). However, the contribution in trace element concentrations of meltwater runoff and snow-ice still need sufficient data for further discussion.
As shown in Table 3, the levels of heavy metals in samples close to anthropogenic sources are much higher than those in remote glacier/snow and glacier-watershed samples. Table 3 and Figure 5 show that the heavy metal elements in high altitude rivers of most regions are higher than those in glacier/snowpack (e.g. QG, LG12, YG), except that the concentration of Co, Cu, Zn, Cd, and Mo in the LG snowpack are slightly higher than those in rivers. Such distribution may imply that the glacier meltwater has an important contribution to the runoff river in the glacier watersheds; Meanwhile, large amounts of SPM have also originated from local sources (e.g., riverbed erosion) to the river water runoff. For example, the content of Ba in snowpack samples in QG is higher than that in river samples. Also, in the Zelonglong Glacier located at Namcha Barwa Peak, heavy metal elements in rivers are slightly higher than those in snowpack, which together indicated that the sources of SPM in glacier watershed is not only from atmospheric aeolian dust/pollutants released from glacier snowmelt water, but also from other local sources, such as the riverbed weathering crust, rock and land surface sediments.
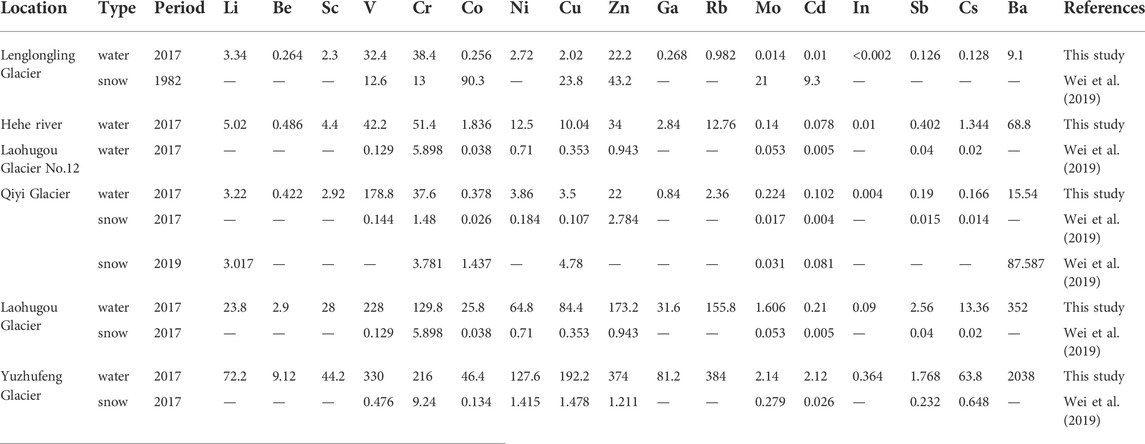
TABLE 3. Heavy metal level in river water and nearby glacier snowpack and ice of sampling locations in glacier basin of the northeast Tibetan Plateau (unit: µg/L).
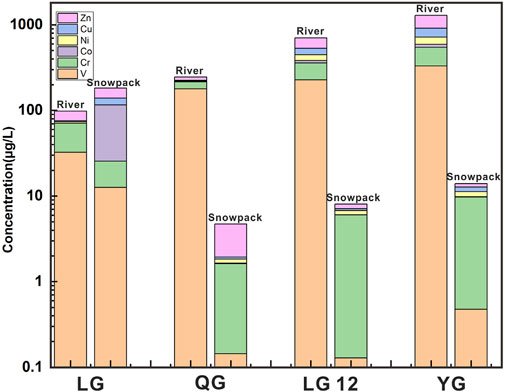
FIGURE 5. Distribution and variation of major heavy metal element concentrations in the glacier snowpack and rivers in glacier regions.
Spatially, compared with YG in the Kunlun Mountains and other locations in eastern China, the heavy metal elements level in the glacier basins of Qilian Mountains was lower, which reflects a relatively clean environment (Supplementary Table S1 and Figure 6). On the whole, it can represent water environment characteristics of these remote areas, but there are also still strong effects from local anthropogenic pollutants inputs. Some heavy metal elements appear obviously enrichment with high concentrations (e.g., Zn, Cu Ni), and there are local elements inputs from anthropogenic pollution areas and exposed mine rich areas.
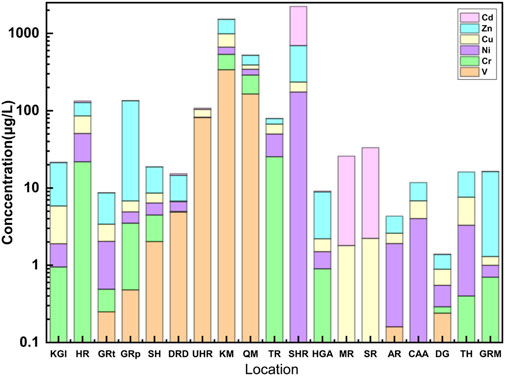
FIGURE 6. Comparison with other rivers in the world, with the major heavy metal elements level of King George Island (KGI), Hooghly River (HR), Gandaki River (GR), Southern Himalayas (SH), Dan River Drainage (DRD), Upper Han River (UHR), Kunlun Mountains (KM), Qilian Mountains (QM), Tigris River (TR), Songhua River (SHR), Haut Glacier d'Arolla (HGA), Marne River (MR), Seine River (SR), Athabasca River and its tributaries (AR), Canadian Arctic Islands (CAA), Dunkemadi Glacier (DG), Thames (TH), and the global average of rivers (GRM).
When compared with global locations, the content of heavy metal elements in the Qilian Mountains glacier watersheds is significantly higher than that in the Canadian Arctic archipelago and the Antarctic region, indicating that the Qilian Mountains region is influenced more by exposed surface dust substances and anthropogenic activities. Heavy metals level in the Hooghly River is relatively high (e.g., Cd), indicating that the influence of anthropogenic pollutant emissions in the region is significantly higher than that in TP. The general concentration of TP locations in this work shows relatively higher value in the global range. By contrast, it further reflects the high level heavy metals of the water environment in the glacial watersheds of the northeast TP.
Figure 7 shows the general comparison and distribution of typical heavy metal elements (e.g. Ni, Cu, and Zn) in northeast TP and other global watershed locations. We found that the concentration of typical heavy metals (Cu, Ni, Zn) in the glacier basin of the Qilian Mountains is relatively high when compared with different regions in the world (Figure 7), indicating that the local surface environment of the Qilian Mountains surrounded by Asian dust sources (the combined effect of the natural deserts and anthropogenic environment), accounting for 50% of the total concentration, and the proportions of Ni, Cu, and Zn are 62%, 63%, and 44%, respectively (Figure 8). Because of elements Ni, Cu, and Zn are three common trace elements in heavy metal pollution, most of that are generated from industrial emissions such as mineral exploitation and the smelting process.
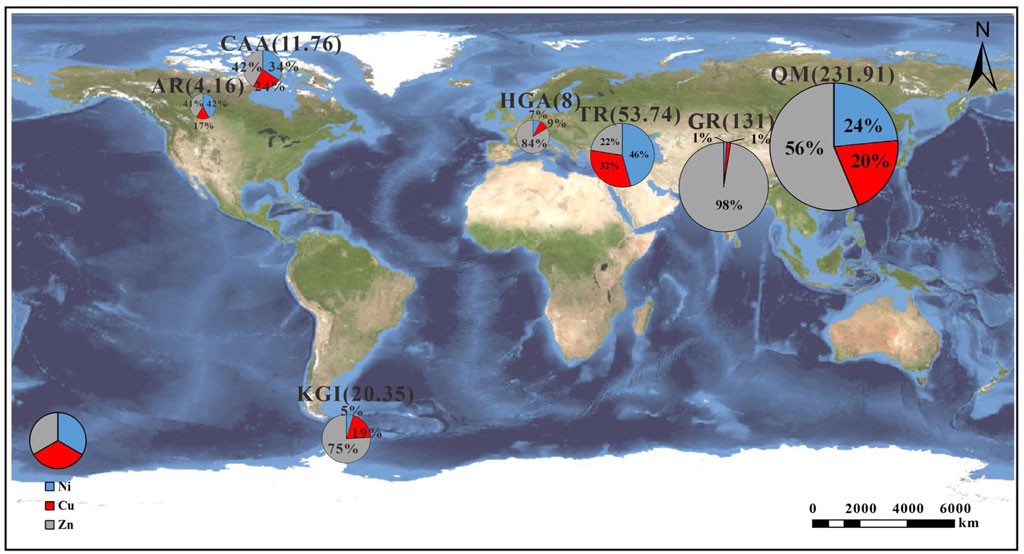
FIGURE 7. Comparison of the proportion of Ni, Cu, and Zn elements in other watersheds and their global distribution. Including the Qilian Mountains (QM), Arctic Islands watershed of Canada (CAA), Gandaki River (p) (GR), Athabasca River and its tributaries (AR), Tigris River (TR), Haut Glacier d'Arolla (HGA), and King George Island (KGI). The units are µg/L. Brackets indicate the total amount of three elements in the area.
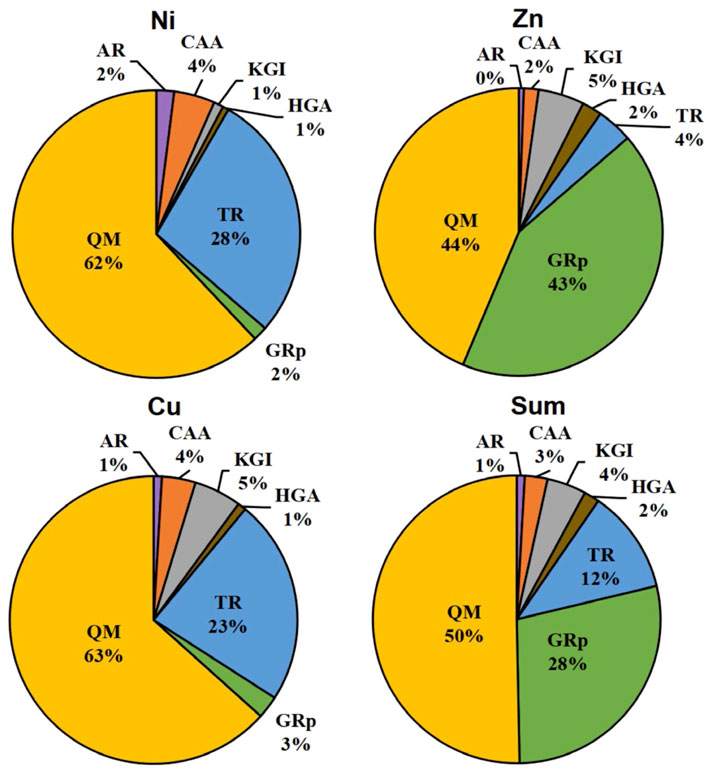
FIGURE 8. Composition proportion of typical heavy metals (Ni, Cu, Zn) in the northeast Tibetan Plateau and other surrounding watersheds showed in the Panel.
Overall, heavy metals level in SPM of rivers in the Qilian and Kunlun Mountains glacier basin, is higher than that in the clean area located in the European, Antarctic and Arctic, but is still significantly lower than that in most rivers in East Asia (Supplementary Table S1 and Figure 6), especially when compared with the enrichment factors (with general low EFs) of heavy metals as discussed below (Figure 7). It showed that the water quality and water environment of most glacier basins in the NETP are relatively clean, but there is also anthropogenic activity. The content of heavy metals in local areas is high, and some elements are even higher than the global, illustrating that the Qilian Mountains may have rich minerals, unique regional environment characteristics, or local anthropogenic activity influences.
Discussion of the enrichment factors and sources of heavy metals in the glacier watersheds of the northeast Tibetan Plateau
Generally, trace element mainly originate from natural or anthropogenic sources, in which natural sources mainly include rock and soil dust, sea salt and marine biological sources, volcanic activity, terrestrial biological sources, biological combustion (e.g., forest wild fires) (Huang et al., 2018). This study region is far from the ocean and at a high altitude, the contribution of sea salt and marine biological activities can be ignored. During the sampling period, major volcanic eruptions with no significant impact occurred. Low vegetation coverage and poor environmental conditions limit the metabolic activities of animals and plants. Although the influence of biomass combustion cannot be ruled out, the proportion of biomass combustion is very low compared to the superposition of fossil fuel combustion and human industrial activities (Huang et al., 2018).
Therefore, we calculated the average EF values of heavy metal elements in the glacier watersheds (Figure 9). Results shows that, the EF value of trace elements is generally low except the Sb (EF = 6) and Cd (EF = 13), indicating that the general enrichment degree of heavy metal elements is relatively low and the water environment conditions are relatively natural and clean. Moreover, comparing glacier basins of the Kunlun Mountains and Qilian Mountains, EF values have large regional differences. The total value of EF of all elements in the YG is 2.21 times than that in Qilian Mountains glacier watershed, the minimum ratio is 1.00 (Cr) times, and the maximum ratio is 3.39 (Cd) times.
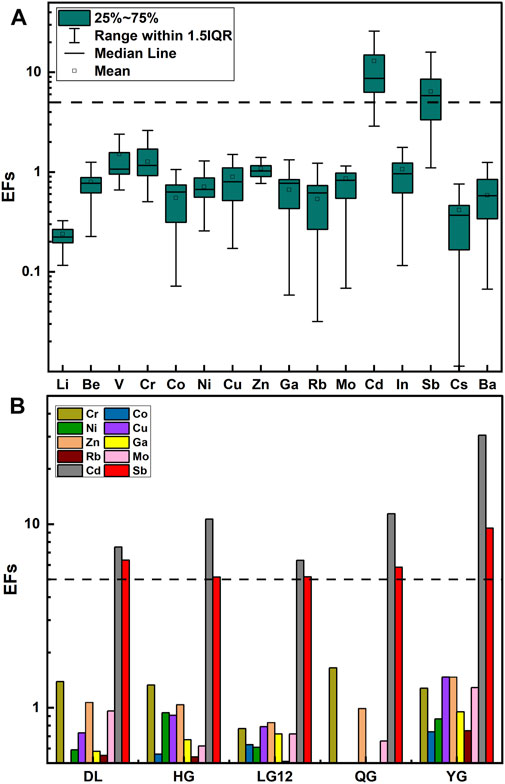
FIGURE 9. (A) Enrichment factors (EFs) of heavy metal elements in suspended particles of glacial rivers in different regions; (B) Comparing the average enrichment factors (EFs) of heavy metal elements in SPM of glacial rivers in different regions.
Specifically for the study of the Glacier watersheds in this work (Figure 9), Cd and Sb elements shows EF ≥ 5 in the Dabanshan snowpack basin, Lenglongling Glacier basin and upper Heihe River basin. Mo (EF = 2.88) element is locally enriched in the Lenglongling Glacier basin, while Cd and Sb chemical elements are mainly anthropogenic sources (EF ≥ 5). The Cd and Sb elements in the Qiyi Glacier are greatly affected by anthropogenic activities, and even the concentration of heavy metal elements in some samples reach severe pollution level [Cd (EF = 11.41), Sb (EF = 5.83)]. Because there are mines and factories nearby, EFs of Sb element in glacier area and downstream of the Laohugou Glacier basin indicate that the downstream are more affected by anthropogenic activities than those in the upper glacier area.
The EF coefficients of heavy metals such as V, Cr, Ni, Zn, Cd, Sb, Cs, and other heavy metal elements are relatively high in the downstream of YG basin and the glacier basin, and Cd and Sb may be severely polluted. Previous studies suggested that Ni, As, Se, Cd, and Pb are often derived from fossil fuel combustion (Mattigod and Page, 1983; Robarge, 1999). Pollutants particles are emitted into the atmosphere through anthropogenic combustion activities, and their transport conditions depend on meteorological conditions, aerosol size, form, and chemical properties. Long range and local dust/pollutants could migrate and settle to the glacier area through the large range air circulation and local inputs, resulting in the enrichment of SPM heavy metal elements in the glacier basins and watersheds. For example, both the heavy metal concentration and EFs in the YG region (e.g. Sb, Cs, and Cr) are much higher value than other regions, which is probably related to the nearby mining and the Qinghai-Tibet highway, as well as the mineral water factory nearby the YG glacier basin.
Overall, we found that the Li, Be, Co, Ga, Rb, Mo, Ba, V, Cr, Ni, Cs and other heavy elements in SPM at all sampling locations have limited pollution (EF < 2), and these elements probably mainly originate from aeolian dust, riverbed crust and soils; while Sb, and Cd have obvious enrichment in various regions (with EF > 5), and these elements may have anthropogenic sources to the glacier basins. Aerosols are one of the important sources of heavy metal elements in high-altitude glacier areas, and their chemical compositions are very important for understanding the long-distance transport process of pollutants (Préndez and Adriana Carrasco, 2003). In alpine and glacier areas, in addition to the local surface chemical weathering and pedogenic processes affect the concentration of SPM in rivers, atmospheric transport of aerosols with dust and pollutants can cause dust particles deposition and accumulate in the glacier/snowpack, thereby increasing the concentration of SPM in glacial meltwater (Singh et al., 2017). As many heavy metals (such as Zn and Cd) can only transport as the particulate matter (Hayharth and Jones, 1992).
The geological conditions of the TP are complex. The central and northern part of the Plateau is predominantly composed of volcanic clastic sandstone shales and marl (Sheng et al., 2012). The zonal soil, such as soil-yellow brown soil, is widely distributed in cold desert soil, meadow soil and grassland, with a low degree of general development and easy weathering and erosion. It was found that Cd and Sb in the surface soil of the northeastern TP exceeded the standard significantly (Yang et al., 2020). Consistent with the results of EF enrichment in the river SPM in the glacier basin in this paper, indicating that erosion and weathering in the study area have an important influence on the river SPM. In addition, studies have shown that Cd content is high in surface soil along highways in the Tibetan Plateau (Zhang et al., 2015), which reflects the export of heavy metal elements by transit. Moreover, the existence of antimony deposits in the study area and the frequent mining, tourism and transportation activities in Qinghai also reflect the influence of mining and transportation on the source of heavy metals (Mattigod and Page, 1983; Robarge, 1999; Wu et al., 2016; Yang et al., 2020). Therefore, the sources of Sb and Cd pollution in rivers in the northeast of the TP are affected by a combination of mining, natural and traffic factors under regional weathering and erosion.
Although atmospheric transport has little influence on chemical elements in rivers, the TP, as a sink of Asian aerosols (Préndez and Adriana Carrasco, 2003), and accumulates on the surface of glacier snow and soil through dry/wet deposition in the mountain and glacier area. In addition to the local surface chemical weathering and pedogenic process affects rivers SPM concentration, long distance and local dust/pollutants through a wide range of air circulation and local input will lead to dust particles in the ice/snow subsidence and accumulation, thus increasing the glacier melt water concentrations of SPM (Hayharth and Jones, 1992; Singh et al., 2017). Mainly in the northeast of the TP from the north branch of central and western China strong westerlies (Figure 10), the influence of melting during the summer period is affected by the east Asian summer monsoon. Therefore, man-made pollutants from northwest China in South Asia and east Asia may through the atmospheric circulation to the ice valley, bring a lot of heavy metals, and the glacier basin deposition. The concentration and EFs (e.g., Sb, Cs, and Cr) of heavy metals in YG basin are higher than those in other areas, which may be influenced by more aerosols in the process of atmospheric transport.
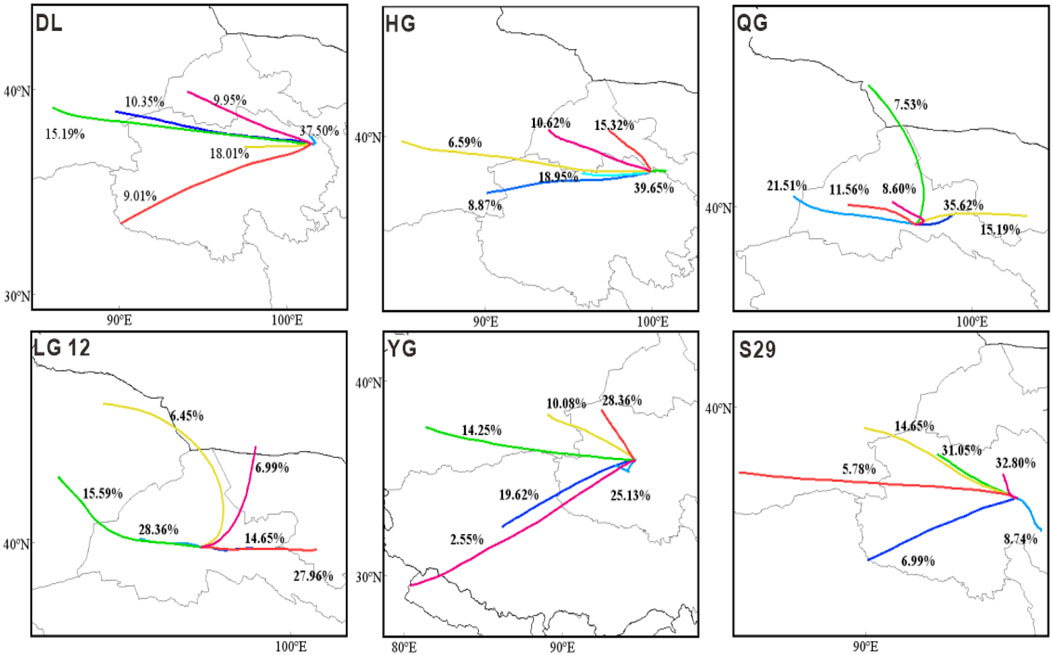
FIGURE 10. The typical backward trajectory of five glacier basin areas in May 2017. The clustering of all trajectory air masses for 31 days in May is calculated at an interval of 1 h every day at a height of 500 m.
Due to the differences of climate and surrounding environment, the origins, transport and deposition of heavy metals are different in the north (Qilian Mountains) and south (Himalaya Mountains) of the TP. Previous studies have observed that the Himalayan region is seriously polluted by anthropogenic aerosols based on the high enriched heavy metals such as Cr, Co, Ni, Cu, Zn, Cd, Pb, As, Mo, Sn and Sb in ice core/precipitation records indicates that. These polluted metals are mainly derived from natural mineral dust and anthropogenic emissions such as stationary fossil fuel combustion and nonferrous metals production in South Asia (particularly in India) (Hong et al., 2009; Tripathee et al., 2014). By contrast, our data shows that SPM heavy metals of the high-altitude glacier rivers in the Qilian and Kunlun Mountains display slightly enrichment and are easily affected by the surrounding human activities.
Conclusion
In this study, we investigated the concentration and spatial variation of heavy metal elements in the SPM in the glacier watershed in northeast TP. Results showed that the concentration of heavy metals is relatively high in the Yuzhufeng glacier area, ranging from 0.57 μg/L (In) to 1,551.6 μg/L (Ba). The concentration in the Qiyi Glacier region is the lowest, ranging from 0.02 μg/L to 85.05 μg/L. While the Lenglongling Glacier, Dabanshan snowpack basin, upstream Heihe River glacier basin, and Laohugou Glacier basin showed the middle, and the total concentration varies from 1,503.94 μg/L to 1726.2 μg/L. The enrichment factors (EFs) analysis showed that the content of heavy metal elements in the river SPM in the river-water is higher than that in upper glacier snowpack. Moreover, most of trace elements mainly originated from crustal dust, while other metals (e.g., Cd, Sb) have certain sources of anthropogenic pollutants. The enrichment factors (EFs) of heavy metal elements in the SPM of the river in the Yuzhufeng Glacier area, the downstream segment of the Laohugou Glacier area, and the Laohugou Glacier area are relatively high. However, the enrichment factors of heavy metal elements in other regions, such as the Heihe source area, upstream segment and mountain exit, and the Qiyi Glacier are very low, which may be derived from the natural mineral resources on the surface of the glacier basin. Compared with the global locations, the concentration of heavy metals (e.g., Cu, Ni, and Zn) in this region is relatively high on the whole. Meanwhile, the river water environment in the glacier basin in the northeast TP is close to the natural and clean state, but it is also affected by certain anthropogenic activities. Mining activities, transportation and natural weathering and erosion processes in the study area have important effects on the content of heavy metal pollutants of rivers SPM in the glacier watershed. The analysis of backward air mass trajectory indicated the potential environmental pollutants transport from surrounding cities and suburbs to the remote glaciers, and then melted out and released into the meltwater runoff glacier watersheds.
Heavy metals have ecotoxicology implications and may act on the human body and accumulate to cause chronic poisoning. Glaciers are as the largest water reserves distributed in the TP and its surrounding areas. As the water tower of Asia, TP glaciers are closely related to human health and production, thus the water quality and water environment in this region is very important. Therefore, this study provides a new perspective on the complete view of heavy metals levels distribution, source and general water quality in glacier watershed, and thus provides the new understanding for the cryosphere water environment evaluation in the TP region.
Data availability statement
The original contributions presented in the study are included in the article/Supplementary Material, further inquiries can be directed to the corresponding authors.
Author contributions
RW: Writing and Editing; ZD: Supervision, Resource, Investigation, Writing, Review and Editing; XC: Supervision, Writing, Review and Editing; JB: Review and Editing; XJ: Investigation, Visualization; LW: Review and Editing.
Funding
This work was funded by the National Natural Science Foundation of China (42022002), and the State Key Laboratory of Cryosphere Sciences (SKLCS-ZZ-2022).
Acknowledgments
The authors would like to thank the NCEP/NCAR for sharing the regional and global wind field data, and also many thanks to the fieldwork team who worked in the harsh and cold environment on the Tibetan Plateau region during 2017–2020.
Conflict of interest
The authors declare that the research was conducted in the absence of any commercial or financial relationships that could be construed as a potential conflict of interest.
Publisher’s note
All claims expressed in this article are solely those of the authors and do not necessarily represent those of their affiliated organizations, or those of the publisher, the editors and the reviewers. Any product that may be evaluated in this article, or claim that may be made by its manufacturer, is not guaranteed or endorsed by the publisher.
Supplementary material
The Supplementary Material for this article can be found online at: https://www.frontiersin.org/articles/10.3389/fenvs.2022.918514/full#supplementary-material
References
Cong, Z., Kang, S., Liu, X., and Wang, G. (2007). Elemental composition of aerosol in the nam co region, Tibetan Plateau, during summer monsoon season. Atmos. Environ. 41, 1180–1187. doi:10.1016/j.atmosenv.2006.09.046
Cong, Z., Kang, S., Zhang, Y., and Li, X. (2010). Atmospheric wet deposition of trace elements to central Tibetan Plateau. Appl. Geochem. 25 (9), 1415–1421. doi:10.1016/j.apgeochem.2010.06.011
Dong, Z., Brahney, J., Kang, S., Elser, J., Wei, T., Jiao, X., et al. (2020). Aeolian dust transport, cycle and influences in high-elevation cryosphere of the Tibetan Plateau region: New evidences from alpine snow and ice. Earth-Science Rev. 211, 103408. doi:10.1016/j.earscirev.2020.103408
Dong, Z., Kang, S., Qin, D., Qin, X., Yan, F., Du, W., et al. (2017). Temporal and diurnal analysis of trace elements in the Cryospheric water at remote Laohugou basin in northeast Tibetan Plateau. Chemosphere 171, 386–398. doi:10.1016/j.chemosphere.2016.12.088
Dong, Z., Kang, S., Qin, X., Li, X., Qin, D., and Ren, J. (2015). New insights into trace elements deposition in the snow packs at remote alpine glaciers in the northern Tibetan Plateau, China. Sci. Total Environ. 529, 101–113. doi:10.1016/j.scitotenv.2015.05.065
Dong, Z., Qin, D., Li, K., Kang, S., Wei, T., and Lu, J. (2019). Spatial variability, mixing states and composition of various haze particles in atmosphere during winter and summertime in northwest China. Environ. Pollut. 246, 79–88. doi:10.1016/j.envpol.2018.11.101
Du, S., Yong-qiu, W., and Wen-min, H. (2015). Research progress on analysis methods and their application of aeolian sediment sources. Arid Zone Res. 32, 184–191. doi:10.13866/j.azr.2015.01.26
Du, Z., Xiao, C., Liu, Y., Yang, J., and Li, C. (2017). Natural vs. anthropogenic sources supply aeolian dust to the Miaoergou Glacier: Evidence from Sr-Pb isotopes in the eastern Tienshan ice core. Quat. Int. 430, 60–70. doi:10.1016/j.quaint.2015.11.069
Fan, X. T., and Jiang, Y. X. (2016). Selection of a reference element for enrichment factor: A case study on the pollution evaluation of heavy metals in the sediment of yuan river. Acta Sci. Circumst. 36, 3795–3803. doi:10.13671/j.hjkxxb.2016.0044
Fergusson, J. E. (1990). The heavy elements: Chemistry, environmental impact and health effects. Environ. Int. 17, 501–502. Available at: https://search.crossref.org/?q=The+heavy+elements:+Chemistry,+environmental+impactand+health+effects.&from_ui=yes. doi:10.1016/0160-4120(91)90309-e
Gabrielli, P., Wegner, A., Sierra-Hernández, M. R., Beaudon, E., Davis, M., Barker, J. D., et al. (2020). Early atmospheric contamination on the top of the Himalayas since the onset of the European Industrial Revolution. Proc. Natl. Acad. Sci. U.S.A. 117 (8), 3967–3973. doi:10.1073/pnas.1910485117
Gao, W., Li, Z., and Zhang, M. (2011). Study on particle-size properties of suspended load in glacier runoff from the tomor Peak. Arid Zone Res. 28, 449–454. doi:10.13866/j.azr.2011.03.028
Guo, B., Liu, Y., Zhang, F., Hou, J., Zhang, H., and Li, C. (2018). Heavy metals in the surface sediments of lakes on the Tibetan Plateau, China. Environ. Sci. Pollut. Res. 25 (4), 3695–3707. doi:10.1007/s11356-017-0680-0
Hao, L. B., Sun, L. J., Lu, J. L., Sun, S. M., and Pan, Z. H. (2010). Distribution characteristics of the heavy metals of the suspended solids in the upper and middle reaches of the Second Songhua River. J. Jilin Univ. (Earth Sci. Ed. 40, 327–330. doi:10.13278/j-cnki.jjuese.2010.02.009
Hayharth, P. M., and Jones, K. C. (1992). “Atmospheric deposition of metals to agricultural surfaces,” in Biogeochemistry of trace metals. Editor C. A. Domy (Boca Raton, USA: Lewis Publishers), 249–276.
He, J., Wang, X., and Chao-sheng, I. I. (2003). Pollution character of heavy metals in the water sediment system from Baotou section of the Yellow River. Acta Sci. Circumstantiae 23, 53–57. doi:10.13671/j.hjkxxb.2003.01.011
Hong, S., Lee, K., Hou, S., Hur, S. D., Ren, J., Burn, L. J., et al. (2009). An 800-year record of atmospheric As, Mo, Sn, and Sb in central Asia in high-altitude ice cores from Mt. Qomolangma (Everest), Himalayas. Environ. Sci. Technol. 43 (21), 8060–8065. doi:10.1021/es901685u
Huang, J., Li, Y., Li, Z., and Xiong, L. (2018). Spatial variations and sources of trace elements in recent snow from glaciers at the Tibetan Plateau. Environ. Sci. Pollut. Res. 25, 7875–7883. doi:10.1007/s11356-017-0904-3
Huang, J., Wu, G., Zhang, X., and Zhang, C. (2020). New insights into particle-bound trace elements in surface snow, Eastern Tien Shan, China. Environ. Pollut. 267, 115272. doi:10.1016/j.envpol.2020.115272
Huser, B. J., Köhler, S. J., Wilander, A., Johansson, K., and Fölster, J. (2011). Temporal and spatial trends for trace metals in streams and rivers across Sweden (1996-2009). Biogeosciences 8, 1813–1823. doi:10.5194/bg-8-1813-2011
Jiao, X., Dong, Z., Kang, S., Li, Y., Jiang, C., and Rostami, M. (2021). New insights into heavy metal elements deposition in the snowpacks of mountain glaciers in the eastern Tibetan Plateau. Ecotoxicol. Environ. Saf. 207, 111228. doi:10.1016/j.ecoenv.2020.111228
Johannessen, M., and Henriksen., A. (1978). Chemistry of snow meltwater: Changes in concentration during melting. Water Resour. Res. 14, 615–619. doi:10.1029/wr014i004p00615
Köhler, S. (2010). Comparing filtered and unfiltered metal concentrations in some Swedish surface waters. Uppsala, Sweden: Department of Aquatic Sciences and Assessment, Swedish University of Agricultural Sciences.
Lee, K., Hur, S. D., Hou, S., Hong, S., Qin, X., Ren, J., et al. (2008). Atmospheric pollution for trace elements in the remote high-altitude atmosphere in central Asia as recorded in snow from Mt. Qomolangma (Everest) of the Himalayas. Sci. Total Environ. 404, 171–181. doi:10.1016/j.scitotenv.2008.06.022
Li, C., Kang, S., and Zhang, Q. (2009). Elemental composition of Tibetan Plateau top soils and its effect on evaluating atmospheric pollution transport. Environ. Pollut. 157 (8-9), 2261–2265. doi:10.1016/j.envpol.2009.03.035
Li, C., Chen, P., Kang, S., Yan, F., Tripathee, L., Wu, G., et al. (2018). Fossil fuel combustion emission from south Asia influences precipitation dissolved organic carbon reaching the remote Tibetan plateau: Isotopic and molecular evidence. J. Geophys. Res. Atmos. 123, 6248–6258. doi:10.1029/2017jd028181
Li, M., Sun, X., and Li, S. (2020). Advances on inorganic hydrochemistry of glacial meltwater runoff in the Qinghai-Tibet Plateau and its surrounding areas. J. Glaciol. Geocryol. 42, 562–574.
Li, R., Shen, B., and Wu, X. (2021). Spatial distribution and source analysis of trace elements in typical mountain glaciers on the Qinghai-Tibet Plateau. J. Glaciol. Geocryol. 43, 1277–1289. (in Chinese). doi:10.7522/j.issn.1000-0240.2021.0082
Li, X., Zhang, Y., and Hou, L. (2021). Characteristics and risk assessment of heavy metals pollution in sediments of Chongli District reaches of Qingshui River Basin. J. Cap. Normal Univ. Nat. Sci. Ed. 42, 54–61. doi:10.19789/j.1004‐9398.2021.01.010
Li, X., Qin, D., and Jing, Z. (2012). Diurnal variation of trace elements in qiyi glacier basin of qilian mountain and its influence on unfiltered after sampling. Sci. China Earth Sci. 42 (11), 1745–1756. doi:10.1360/zd-2012-42-11-1742
Liu, B., Kang, S., Sun, J., Zhang, Y., Xu, R., Wang, Y., et al. (2013). Wet precipitation chemistry at a high-altitude site (3,326 m a.s.l.) in the southeastern Tibetan Plateau. Environ. Sci. Pollut. Res. 20 (7), 5013–5027. doi:10.1007/s11356-012-1379-x
Liu, G.-d., Zhang, L., and Yang, Z. (2021). Distribution characteristics of heavy in the water and suspended substance of Nenjiang River basin: Environmental Implication. Geol. Resour. 30, 53–61. doi:10.13686/j.cnki.dzyzy.2021.01.007
Liu, W., Xu, S. J., and Yang, J. D. (2007). Mineralogical characteristics of suspended matters and sediments in the Jinshajiang River and their superficial geochemical significance. Bull. Mineralogy. Petrology Geochem. 26, 164–169. doi:10.3969/j.issn.1007-2802.2007.02.012
Liu, Y., Hou, S., Hong, S., Hur, S.-D., Lee, K., and Wang, Y. (2011). Atmospheric pollution indicated by trace elements in snow from the northern slope of Cho Oyu range, Himalayas. Environ. Earth Sci. 63 (2), 311–320. doi:10.1007/s12665-010-0714-0
Mattigod, S. V., and Page, A. L. (1983). Assessment of metal pollution in soils. Appl. Environ. Geochem. . Editor I. Thornton Academic Press Geology Series, 355–394.
McBride, M. B. (2020). Chemisorption and precipitation reactions. Handbook of Soil Science. Editor M. E. Sumner (Boca Raton, FL: CRC Press, B265–B302.
Miles, E., McCarthy, M., Dehecq, A., Kneib, M., Fugger, S., and Pellicciotti, F. (2021). Health and sustainability of glaciers in high mountain Asia. Nat. Commun. 12, 2868–2910. doi:10.1038/s41467-021-23073-4
Pant, R. R., Zhang, F., Rehman, F. U., Koirala, M., Rijal, K., and Maskey, R. (2020). Spatiotemporal characterization of dissolved trace elements in the Gandaki River, central himalaya Nepal. J. Hazard. Mater. 389, 121913. doi:10.1016/j.jhazmat.2019.121913
Paudyal, R., Kang, S., Sharma, C. M., Tripathee, L., Huang, J., Rupakheti, D., et al. (2016a). Major ions and trace elements of two selected rivers near Everest region, southern Himalayas, Nepal. Environ. Earth Sci. 75, 1–11. doi:10.1007/s12665-015-4811-y
Potocki, M., Dixon, D. A., Kurbatov, A. V., Casassa, G., Zamora, R., Handley, M. J., Grigholm, B., Korotkikh, E. V., Birkel, S. D., Clifford, H., and Mayewski, P. A. (2022). Trace metal emission history captured in a Chilean ice core. Atmos. Environ. 276, 119002. doi:10.1016/j.atmosenv.2022.119002
Préndez, M., and Adriana Carrasco, M. (2003). Elemental composition of surface waters in the Antarctic Peninsula and interactions with the environment. Environ. Geochem. Health 25, 347–363. Available at: https://search.crossref.org/?q=Short-term+variability+of+dissolved+trace+element+concentrations+in+the+Marne+and+Seine+Rivers+near+Paris.+&from_ui=yes.
Qu, C. H., Zheng, J. Z., and Yang, S. J. (1984). Chemical composition of suspended matter and its restriction factors in control stations of the lower reaches of the Yellow River, the Yangtze River and the Pearl River. Chin. Sci. Bull. 29, 1063–1066.
Robarge, W. P. (1999). Environmental soil and water chemistry: Principles and applications. Soil Sci. 164, 609–610. doi:10.1097/00010694-199908000-00008
Ross, J. M., and Sherrell, R. M. (1999). The role of colloids in tracemetal transport and adsorption behavior in New Jersey Pinelands streams. Limnol. Oceanogr. 44, 1019–1034. doi:10.4319/lo.1999.44.4.1019
Sheng, J., Wang, X., Gong, P., Tian, L., and Yao, T. (2012). Heavy metals of the Tibetan top soils. Environ. Sci. Pollut. Res. 19 (8), 3362–3370. doi:10.1007/s11356-012-0857-5
Singh, S. M., Avinash, K., Sharma, P., Mulik, R. U., Upadhyay, A. K., and Ravindra, R. (2017). Elemental variations in glacier cryoconites of Indian himalaya and spitsbergen, arctic. Geosci. Front. 8, 1339–1347. doi:10.1016/j.gsf.2017.01.002
Song, M., Li, Z., and Jin, S. (2015). Characteristics of water isotopes and hydrograph separation in the glacier No.72 of qingbingtan, tomur Peak. J. Arid Land Resour. Environ. 29, 156–160. doi:10.13448/j.cnki.jalre.2015.097
Sutherland, R. A. (2000). Bed sediment-associated trace metals in an urban stream, Oahu, Hawaii. Environ. Geol. 39, 611–627. doi:10.1007/s002540050473
Taylor, S. R., and McLennan, S. M. (1995). The geochemical evolution of the continental crust. Rev. Geophys. 33, 241–265. doi:10.1029/95rg00262
Tripathee, L., Kang, S., Huang, J., Sharma, C. M., Sillanpää, M., Guo, J., et al. (2014). Concentrations of trace elements in wet deposition over the central Himalayas, Nepal. Atmos. Environ. 95, 231–238. doi:10.1016/j.atmosenv.2014.06.043
Wällstedt, T., Björkvald, L., and Gustafsson, J. P. (2010). Increasing concentrations of arsenic and vanadium in (southern) Swedish streams. Appl. Geochem. 25, 1162–1175. doi:10.1016/j.apgeochem.2010.05.002 Available at: https://search.crossref.org/?q= Increasing+concentrations+ of+arsenic+and+vanadium+in+(southern)+Swedish+streams.&from_ui=yes.
Wang, W., and Zhang, J. (1994). Analysis on the mineral compositions of suspended matter from Rhone and some main Chinese rivers. Oceanol. Limnol. Sin. 25, 3l9–327.
Wei, T., Dong, Z., Kang, S., Zong, C., Rostami, M., and Shao, Y. (2019). Atmospheric deposition and contamination of trace elements in snowpacks of mountain glaciers in the northeastern Tibetan Plateau. Sci. Total Environ. 689, 754–764. doi:10.1016/j.scitotenv.2019.06.455
Woitke, P., Wellmitz, J., Helm, D., Kube, P., Lepom, P., and Litheraty, P. (2003). Analysis and assessment of heavy metal pollution in suspended solids and sediments of the river Danube. Chemosphere 51, 633–642. doi:10.1016/s0045-6535(03)00217-0
Wu, J., Duan, D., Lu, J., Luo, Y., Wen, X., Guo, X., et al. (2016). Inorganic pollution around the qinghai-tibet plateau: An overview of the current observations. Sci. Total Environ. 550, 628–636. doi:10.1016/j.scitotenv.2016.01.136
Yan, L., Sun, M., and Yao, X. (2018). Lake water in the tibet plateau: Quality change and current status evaluation. Acta Sci. Circumstantiae 38 (3), 900–910. doi:10.13671/j.hjkxxb.2017.0390
Yang, A., Wang, Y. H., Hu, J., Liu, X. L., and Li, J. (2020). Evaluation and source of heavy metal pollution in surface soil of qinghai-tibet plateau. Huan Jing Ke Xue 41 (2), 886–894. doi:10.13227/j.hjkx.201907195
Yang, Y., Wu, Q., and Jin, H. (2016). Evolutions of water stable isotopes and the contributions of cryosphere to the alpine river on the Tibetan Plateau. Environ. Earth Sci. 75–11. doi:10.1007/s12665-015-4894-5
Zhang, H., Wang, Z., Zhang, Y., Ding, M., and Li, L. (2015). Identification of traffic-related metals and the effects of different environments on their enrichment in roadside soils along the Qinghai-Tibet highway. Sci. Total Environ. 521-522, 160–172. doi:10.1016/j.scitotenv.2015.03.054
Zhao, G., Li, W., Li, F., Zhang, F., and Liu, G. (2018). Hydrochemistry of waters in snowpacks, lakes and streams of Mt. Dagu, eastern of Tibet Plateau. Sci. Total Environ. 610-611, 641–650. doi:10.1016/j.scitotenv.2017.08.088
Zhou, D. M., Chen, H. M., and Zheng, C. R. (2002). Heavy metals in water bodies purified by suspended substrate of rivers. J. Environ. Sci. (China) 14, 44–48.
Keywords: trace metals level, SPM, glacier watershed, Northeast Tibetan Plateau, source appointments
Citation: Wu R, Dong Z, Cheng X, Brahney J, Jiao X and Wu L (2022) Heavy metal levels and sources in suspended particulate matters of the glacier watersheds in Northeast Tibetan Plateau. Front. Environ. Sci. 10:918514. doi: 10.3389/fenvs.2022.918514
Received: 12 April 2022; Accepted: 27 June 2022;
Published: 03 August 2022.
Edited by:
Tirthankar Banerjee, Banaras Hindu University, IndiaReviewed by:
Vishnu Murari, Indian Institute of Technology Kanpur, IndiaLekhendra Tripathee, Northwest Institute of Eco-Environment and Resources (CAS), China
Copyright © 2022 Wu, Dong, Cheng, Brahney, Jiao and Wu. This is an open-access article distributed under the terms of the Creative Commons Attribution License (CC BY). The use, distribution or reproduction in other forums is permitted, provided the original author(s) and the copyright owner(s) are credited and that the original publication in this journal is cited, in accordance with accepted academic practice. No use, distribution or reproduction is permitted which does not comply with these terms.
*Correspondence: Zhiwen Dong, dongzhiwen@lzb.ac.cn; Xiping Cheng, xipingcheng2012@163.com