Phthalate Acid Esters in Soil, Plastic Shed Film, and Ginseng Tissues of Different Ages From Farmland: Concentration, Distribution, and Risk Assessment
- 1Institute of Special Animal and Plant Sciences, Chinese Academy of Agricultural Sciences, Changchun, China
- 2Key Laboratory of Agro-Product Quality and Safety, Institute of Quality Standards and Testing Technology for Agro-Products, Chinese Academy of Agricultural Sciences, Beijing, China
- 3College of Chinese Medicinal Materials, Jilin Agricultural University, Changchun, China
Plastic shed film used in ginseng cultivation could increase phthalate acid esters (PAEs) residues in ginseng and its planting soil. This study recorded the situation of 19 PAEs in ginseng, soil, and plastic shed film samples from eight ginseng cultivation bases in Jilin Province, China. The results showed that 6 PAEs are omnipresent contaminants in ginseng cultivation bases. The Σ19 PAEs residue ranged from 0.69 to 3.30 mg kg−1 in the soil and from 32.40 to 96.20 mg kg−1 in the plastic shed film. Plastic shed film is possibly one source of PAEs in ginseng bases. In addition, PAEs concentrations in different ginseng tissues (roots, stems, and leaves) showed significant differences (p < 0.05). The residues of Σ19 PAEs in ginseng roots and stems were 0.71–2.10 mg kg−1 [dry weight (dw)] and 1.56–4.69 mg kg−1 (dw), respectively, lower than 2.37–6.82 mg kg−1 (dw) in leaves (p < 0.05). PAEs more readily accumulated in ginseng leaves than in roots and stems. Cultivation age also influenced PAEs accumulation in ginseng. PAEs residues in 3-year-old ginseng plants were higher than those in annual and biennial ginseng (p < 0.05). The noncancer and carcinogenic risk assessments of the target PAEs in ginseng indicated acceptable levels for adult intake. This study provides data for understanding the concentration, distribution, and potential risk of PAEs in ginseng and its cultivated soil.
Introduction
Phthalate acid esters (PAEs), a kind of common plasticizer, are widely added to chemical plastics and agricultural films to increase the plasticity and toughness of these plastic products (Zolfaghari et al., 2014; Steinmetz et al., 2016). The application of plastic film in facility agriculture rose to 2.60 million tons in 2006 (Zhou et al., 2020), and some thick films (over 50 mm polypropylene) are used in facility-based agriculture, especially plastic greenhouses, playing an important role in the adjustment of environmental conditions. PAEs are still easily released into the environment due to sunlight, rain erosion, and other natural phenomena because PAEs are bonded to polymer chains by hydrogen bonding and van der Waals forces (Arfaeinia et al., 2019). PAEs have potential endocrine-disrupting effects in humans (Lu et al., 2020). Moreover, PAEs are known as “reproductive arsenic” in Europe. Dimethyl phthalate (DMP), diethyl phthalate (DEP), dibutyl phthalate (DBP), butyl benzyl phthalate (BBP), di-(2-ethylhexyl) phthalate (DEHP), and di-n-octyl phthalate (DnOP) were defined as priority control pollutants by the United States Environmental Protection Agency (Niu et al., 2014).
PAEs residues in many types of agricultural soils and various crops across China are among the most concerning organic pollutants in agricultural fields (Ma et al., 2013; Wang et al., 2015; Du et al., 2020; Li et al., 2020). Previous studies have provided information on PAEs occurrence in plants in greenhouse facilities affected by varieties, different temperatures, and cultivation patterns (Cai et al., 2015; Li et al., 2016; Cheng et al., 2020; Li et al., 2020; Zhao et al., 2022). However, few studies have characterized the PAEs residues and sources in the Chinese herbal medicine ginseng according to different cultivation ages and tissues.
In China, ginseng, as a perennial herb (mostly requiring 3–5 years to harvest), is planted in greenhouses with plastic shed films to avoid strong light and modify the microclimate. However, plastic shed films easily release PAEs into the air and soil, which results in higher PAEs residues in greenhouse soil than in open fields (Zhou et al., 2021). Plastic film is believed to be one of the important sources of PAEs in farmland (Wang et al., 2015). Some reports also show that PAEs can accumulate in plants at concentrations even higher than those in the corresponding soils. Leaf vegetables contain more PAEs than root vegetables (Zeng et al., 2020; Zhao et al., 2022). Ginseng requires multiple years of cultivation, which means there is a long time to accumulate pollution in soil. Ginseng plants have roots, stems, and leaves. Roots are grown underground. Stems and leaves are grown aboveground. Analysis of whole ginseng plants (roots, stems, and leaves) can help to clarify the distribution and potential uptake sources of PAEs. Most importantly, compared with vegetables grown for several months, the perennial herb ginseng can be especially well suited to research pollution accumulation.
Jilin, located in northeast China, is China’s largest ginseng suppling province, contributing to 85% of the ginseng production in the country (Wang et al., 2018). With the continuous expansion of ginseng demand, wild ginseng is not enough to meet supply requirements, and the level of agricultural productivity must meet the demands of capital development. To increase ginseng output, Jilin Province has used farmland as the main means to cultivate ginseng. After several years of rapid promotion, a large-scale farmland cultivation base has been devoted to ginseng cultivation. However, limited data have been reported about PAEs pollution in ginseng cultivation bases. This study aimed to explore PAEs pollution characteristics in a ginseng cultivation environment and evaluate the noncarcinogenic and carcinogenic risks to adults via dietary consumption of ginseng.
Materials and Methods
Sample Collection
Based on geographic location, eight large-scale ginseng cultivation bases ranging from 25 to 500 ha were selected for research in Jilin Province, China. These bases were located in the farmland of four areas, namely, Baishan city, Dunhua city, Jilin city, and Tonghua city (Figure 1).
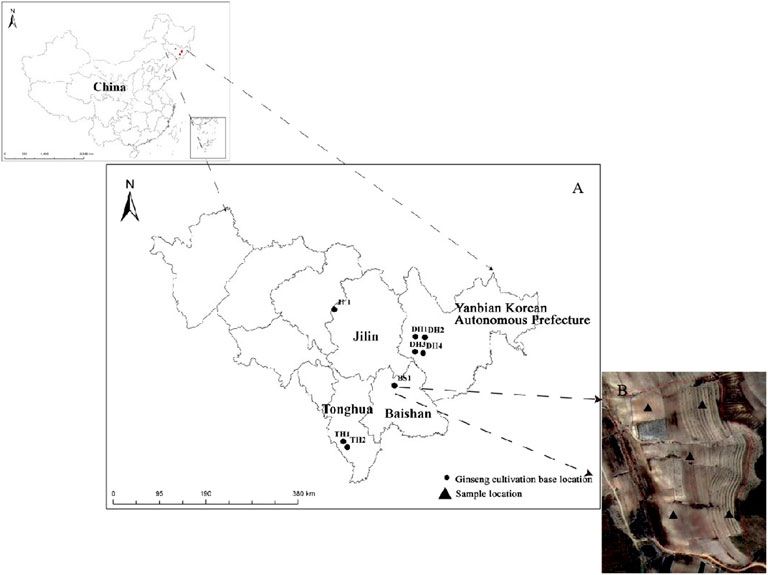
FIGURE 1. Map showing the eight ginseng cultivation bases of Jilin Province, China. (A) Research areas within Jilin Province. (B) Satellite imagery showing the ginseng sampling layout at the BS1 site as an example. The ginseng cultivation base names were BS1, DH1, DH2, DH3, DH4, JL1, TH1, and TH2 (BS, Baishan city; DH, Dunhua city; JL, Jilin city; TH, Tonghua city).
The fresh ginseng, soil (0–20 cm), and shed films were combined from the eight ginseng cultivation bases in July–August 2021. The detailed collection process can be found in Li et al. (2016). Fifteen soil samples from farmland used to cultivate garden ginseng were collected (Supplementary Table S1). Every composite soil sample was collected from five points using a stainless steel auger and sealed in brown paper bags before being transported back to the lab. Then, the soil samples were freeze-dried, ground, sifted through an 80-mesh stainless steel sieve, and stored in glass bottles.
A total of 23 ginseng samples (including roots, stems, and leaves) under different cultivation ages (annual, biennial, 3-year-old, 4-year-old, and 5-year-old) were cultivated in greenhouses covered by plastic shed films. They were separated into roots, stems, and leaves and rinsed clean with deionized water. Then, the samples were freeze-dried, ground, and sieved through a 60-mesh stainless steel sieve. Regarding the ginseng sampling strategy, we mainly considered the ginseng planting years of different bases. A total of 1–5 ginseng samples were collected from each ginseng cultivation base (Supplementary Table S1). Each composite ginseng sample was randomly collected from five different sites in the same bases and homogenized as one sample.
An inserted map displays the location of Jilin Province, including the plastic films and nets, and these materials were also collected for potential source analysis. A total of 1–3 film samples were collected from each ginseng cultivation base. In total, 15 plastic shed film samples were collected from eight cultivation bases (Supplementary Table S1). These film samples were washed with deionized water, air-dried, cut into 4 mm × 4 mm fragments, mixed and kept in sealed kraft paper.
Standard and Reagents
A mixed standard solution (1,000 mg L−1) of 19 target PAEs was purchased from Alta Scientific Co., Ltd. (Tianjin, China). Dimethyl phthalate (DMP), diethyl phthalate (DEP), diisopropyl phthalate (DIPrP), diallyl phthalate (DAP), dipropyl phthalate (DPrP), diisobutyl phthalate (DIBP), dibutyl phthalate (DBP), bis (2-Methoxyethyl) phthalate (DMEP), bis (4-Methyl-2-pentyl) phthalate (BMPP), di (2-ethoxyethyl) phthalate (DEEP), diamyl phthalate (DPP), dihexyl phthalate (DHXP), butyl benzyl phthalate (BBP), bis(2-n-butoxyethyl) phthalate (DBEP), dicyclohexyl phthalate (DCHP), di-(2-ethylhexyl)phthalate (DEHP), dipentyl phthalate (DPhP), di-n-octyl phthalate (DnOP), and dinonyl phthalate (DNP). Acetone, acetonitrile, n-hexane, and other chemicals were all HPLC grade and purchased from Fisher (Pittsburgh, United States).
Sample Processing
PAEs were extracted following a QuEChERS method with slight modification (Zhao et al., 2022). A total of 2.5 g ginseng root, stem or leaf powder in a 50 ml glass centrifuge tube with 2 g sodium chloride was mixed with 10 ml acetonitrile for ginseng sample extraction. The polypropylene cap was screwed onto the tube with aluminum foil, and the glass tube was swirled and subjected to ultrasound treatment for 30 min, followed by centrifugation at 5,000 rpm for 5 min. Five milliliters of the extracts was transferred to a new glass column containing 30 mg of GCB and 100 mg of PSA for the clean-up procedure. This extraction process was repeated twice. The eluate was concentrated to nearly dry, and 1.0 ml hexane was added and redissolved for GC–MS analysis.
The PAEs extraction method in the soil and shed film was performed according to a previous study with some modifications (Li et al., 2021). A total of 2.5 g of soil or 0.1 g of plastic shed film in a glass centrifuge tube was mixed with 40 ml hexane and acetone (V: V = 1:1) for 24 h. The samples were vortexed and treated ultrasonically for 15 min, followed by centrifugation at 5,000 rpm for 5 min. The supernatant was separated through centrifugation. The extraction procedure was repeated twice, and the supernatant was combined. The total extracts were transferred to a new glass column containing 2 g of anhydrous magnesium sulfate, 6 g of neutral alumina, and 12 g of silica gel for the clean-up procedure. Next, 15 ml n-hexane and 15 ml n-hexane:acetone (V:V = 4:1) were used to prewash the column in turn. The extract was eluted with 40 ml of n-hexane and acetone (V: V = 4:1). Then, the leachate was concentrated to nearly dry and diluted to a final volume of 1.0 ml with n-hexane. All the final extracts were tested with external standards by GC-MS.
GC-MS Analysis
The determination of PAEs was done using an ISQ gas chromatography triple quadrupole mass spectrometry (GC–MS) system (Thermo Fisher, United States) and separation by an HP-5MS capillary column (30 m × 0.25 mm×0.25 μm) with selected ion monitoring (SIM) mode for quantitative determination. The MS/MS transitions and parameters for the compounds used in this study are shown in Supplementary Table S2. The carrier gas helium was supplied with a flow rate of 1.0 ml min−1. The column temperature program was initiated at 60°C for 1.0 min, ramped up to 220°C at a rate of 20°C·min−1, held for 1.0 min, further increased to 290°C at 5°C·min−1, and held for 2 min. Then, the temperature was held at 285°C for 2 min. The ion source temperature and transfer line in GC–MS were all set at 280°C. The injector temperature was set at 260°C. One microliter of extract was injected by splitless injection mode in GC-MS.
Quality Control and Analysis
Plastic materials were not used during sampling and processing to avoid PAE contamination. All glassware was baked at 450°C for 8 h to remove all organic matter. The linearity range and equation, determination coefficient (R2), limit of detection (LOD), and limit of quantification (LOQ) of the 19 PAEs are shown in Supplementary Table S3. Five-point standard calibration curves were employed with R2 values ranging from 0.9939 to 0.9999. The LODs of the 19 PAEs ranged from 0.125 to 1.150 μg L−1, with a signal-to-noise ratio of 3. The matrix-spiked method was used to ensure the validity of the method by calculating the recoveries of target PAEs accuracies in soil, ginseng, and shed film matrix-spiked samples. The spiked levels were set according to the residues of PAEs in the samples. The results shown in Supplementary Tables S4, S5 indicate that three spiked levels, that is, low (0.02 mg kg−1), medium (0.10 mg kg−1), and high (0.50 mg kg−1), were added for recovery of each compound of the 19 PAEs in ginseng and soil: 73.0%–109.1% and 70.2%–113.0%, respectively. The recoveries of 19 PAEs in the shed film samples ranged from 70.5 to 114.5% as shown in Supplementary Table S6. Solvent blank samples, duplicates, and matrix-spiked samples were used to ensure the stability and accuracy of the instrument during the injection process. Because it is difficult to find blank ginseng free of PAEs, the slope comparison method was used to evaluate the ginseng matrix effect. The slope ratios (slope matrix/slope solvent) for each compound were obtained considering a signal enhancement or suppression effect as acceptable if the slope ratio ranged from 80 to 120%. Only small amounts of DMP, DIBP, and DHXP were detected in the solvent blank from 0.0002 to 0.006 mg kg−1, and the final results of all samples were blank corrected.
Health Risk Assessment
The risk of PAEs to consumer health caused by ingestion of garden ginseng was determined through methods recommended by the US EPA (2013). Among the detected PAEs in ginseng root, DBP, DEP, DMP, and DEHP are recognized as noncancerous pollution compounds for human health, while DEHP is carcinogenic (Kluwe et al., 1982). The average daily doses (ADDs) were calculated following Eq. 1 via the ginseng intake route. Noncancer risk was calculated by the hazard index (HI) via the intake of garden ginseng. The HI was used to calculate the sum of hazard coefficients of exposure to various chemicals having the same toxic mechanism. HI was defined by the daily maximum allowable contaminant level (RfD) and the ratio of average daily dietary intake doses (ADD) as shown in Eq. 2. If the HI is above 1, it is believed that noncancer risk cannot be ignored.
where C is the concentration of PAEs detected in the ginseng sample (mg·kg−1), I is the daily ingestion of ginseng root (mg·person−1day−1), EF is the exposure frequency (days·year−1), ED is the exposure cycle (year), BW is the body weight (kg), and AT is the average lifetime exposure (days).
where RfD is the daily maximum permissible level of contaminants (mg·kg−1·day −1).
where CFS is the slope factor of carcinogenic (kg·day·mg−1).
Carcinogenic risks (Girkin et al., 2018) were calculated using the ADD multiplied by the CSF, as shown in Eq. 3. The CFS values for DEHP are 0.014 mg kg−1 day−1 (Kluwe et al., 1982). These values of the parameters for the above formula are shown in Supplementary Table S7. Carcinogenic risks were regarded as acceptable if the CR < 10–6 (USEPA, 2013).
Results and Discussion
Occurrence of Phthalate Acid Esters in Soils From Ginseng Cultivation Bases
The concentrations and detection frequencies of PAEs in soils, films and ginseng roots are listed in Supplementary Table S9 and Figure 2. PAEs were detected in all the soil samples from eight cultivation bases. The total 19 PAEs concentrations (Σ19PAEs) in soils ranged from 0.69 to 3.30 mg kg−1 (mean 1.65 mg kg−1). These results were similar to those of other studies, in which the average Σ16PAEs (DMP, DEP, DiBP, DnBP, DOP, DMEP, BMPP, DEEP, DPP, DHXP, BBP, DBEP, DCHP, DEHP, DPhP, and DnOP) in plastic greenhouse soil ranged from 0.12 to 5.76 mg kg−1 (mean: 1.86 mg kg−1) (Zeng et al., 2020) and Σ15PAEs (DMP, DEP, DiBP, DnBP, DMEP, BMPP, DEEP, DAP, DHXP, BBP, DBEP, DCHP, DEHP, DPhP, and DnOP) ranged from 0.14 to 2.13 mg kg−1 (mean 0.99 mg kg−1) (Li et al., 2016). Among the 19 PAEs, 7 PAEs compounds, including DMP, DEP, DIBP, DBP, DHXP, DEHP, and DNOP, were detectable. DMP, DIBP, DBP, DHXP, and DEHP occurred in all samples, accounting for approximately 94% of Σ19PAEs. PAEs detected from eight bases showed the order of DBP > DIBP > DEHP > DnOP > DMP = DNP > DEP. This distribution was different from that found for vegetable agricultural bases in China (Wang et al., 2014; Ma et al., 2015).
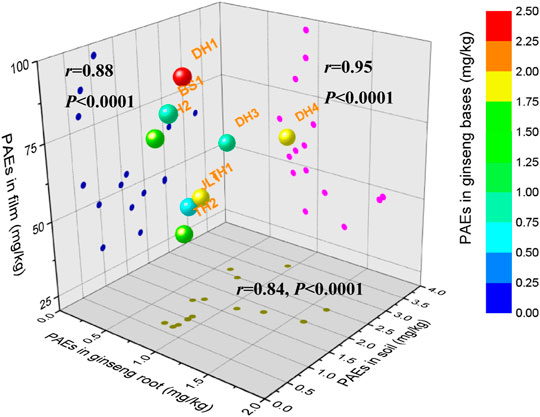
FIGURE 2. PAEs distributions and correlations among soil, shed film, and ginseng root. The color ball represents the total concentration of 19 PAEs in soils from eight ginseng cultivation bases (mg·kg−1); r: Pearson correlation coefficient, p: p value.
According to New York State Department of Environmental Conservation (2010) guidelines about soil cleanup in the United States, six PAEs, including DMP, DEP, DBP, BBP, DnOP, and DEHP, should be limited to 0.020, 0.071, 0.081, 1.125, 1.200, and 4.350 mg kg−1, respectively. In the eight ginseng cultivated bases, the DEP, BBP, DnOP, and DEHP residue levels in the soil samples met the standards. However, DBP was over the recommended allowable values, ranging from 0.22 to 1.86 mg kg−1. The concentrations of DMP in a vast majority of ginseng base soil samples (93%) except those of BS1 surpassed the recommended allowable values. Therefore, DBP and DMP in farmland soil in ginseng cultivation bases should receive attention.
However, the concentrations of PAEs in the soil varied significantly among the different ginseng cultivation bases (Figure 3). The residue of Σ7PAEs in the DH1 ginseng cultivation bases was higher than those in the other bases. The Σ7PAEs detected in soils was 2.47 mg kg−1. Lower PAEs residues were found in JL1 located in Zuojia town, Jilin city, with a mean concentration of 0.69 mg kg−1. JL1 is an experimental farmland, and compared with other bases, the farming intensities and agricultural activities were relatively low in this area. This might be the reason why PAEs residues were accordingly lower.
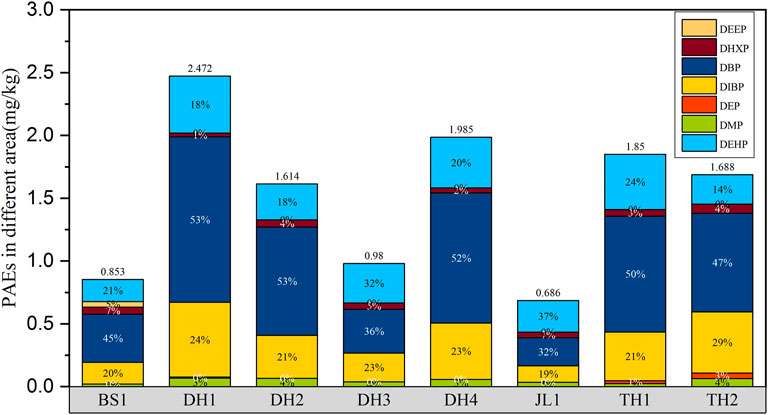
FIGURE 3. Concentrations and distribution of seven PAEs detected in soils from eight ginseng cultivation bases.
In recent years, soil organic matter (SOM) and pH have been reported to affect PAEs fate in soil (Xu et al., 2008; Chang et al., 2009; Ren et al., 2016). SOM content crucially influenced the adsorption process of PAEs, and the soil solution pH and its concentration of dissolved organic matter (DOM) affected biodegradation by providing nutrients, which supported the growth of bacteria and improved overall enzyme activity (Tang et al., 2020). In fact, a slight positive correlation between PAEs residues and pH was confirmed in agricultural soils (Katsoyiannis, 2006; Zeng et al., 2008), and no correlations between PAEs and total organic carbon were detected in green vegetable production base soils (Chai et al., 2014). In this study, the SOM content ranged from 30.2 to 119.0 g kg−1 and the pH ranged from 3.70 to 5.80 in soil from eight large ginseng cultivation bases. However, the correlation of SOM and pH with PAEs concentration in soil was evaluated. SOM was negatively correlated with the concentrations of 19 PAEs in soils (r = −0.63, p = 0.022), and no clear correlation was found with pH (r = 0.08, p = 0.79), as shown in Supplementary Table S8 and Supplementary Figure S1. Niu et al. (2014) also reported that pH has no clear correlation with the level of PAEs residues in Chinese soil, and a positive correlation was shown with DMP and SOM.
Phthalate Acid Esters Concentrations in Plastic Shed Films From Ginseng Cultivation Bases
Plastic shed films in ginseng cultivation bases are mainly composed of shading nets made by high-density polyethylene (HDPE) and plastic films made by polypropylene, which have different colors to absorb different kinds of light. To determine the level of PAEs in shading films used in the cultivation bases of ginseng, 15 plastic film samples were detected, as shown in Supplementary Table S9 and Figure 2. Σ19 PAEs were detected in shed plastic film (thick film) from 32.4 to 96.2 mg kg−1 (mean 56.80 mg kg−1), which are higher than those in mulching films (thin films) from 3.66 to 30.8 mg kg−1 (mean 12.1 mg kg−1) (Li et al., 2016). In addition, the results showed that DEHP, DIBP, and DBP were the most omnipresent congeners, accounting for N88% of Σ19PAEs, and DMP, DEP, and DHXP remained at 12%, having a similar content ratio in the PAEs components detected in corresponding farmland soils. PAEs components in plastic polymers can easily enter the environment because PAEs rely on hydrogen bonding and van der Waals forces to bind with the chemical structure of the plastic (Wang et al., 2014; Zolfaghari et al., 2014; He et al., 2015). The PAEs levels in the plastic shed films were 10–30 times higher than those in the soils. To better understand the potential sources of PAEs residues in ginseng cultivated soil, Pearson’s correlation coefficients were used to calculate the correlations of residual levels of PAEs between soil and shed films. The correlation coefficient was 0.88, between 0.8 and 1.0, indicating a strong correlation. This result indicates that PAEs concentrations in farmland soil were significantly correlated with the type and amount of plasticizer in the shed film. Therefore, plastic shed films may result in enhanced PAEs contamination in the environment.
Distribution of Phthalate Acid Esters in Ginseng Roots Under Different Cultivation Ages
The application of PAEs additives could cause plants, such as vegetables and crops, to absorb PAEs from the environment (Cai et al., 2015). However, there are few studies on the accumulation of PAEs in plants with longer growing periods (more than 1 year). In this study, 23 ginseng roots with five different cultivation ages (annual, biennial, 3-year-old, 4-year-old, and 5-year-old) were collected from eight large cultivation bases, and the levels of 19 PAEs were determined. The contents of individual PAEs in ginseng samples are shown in Supplementary Table S10 and Figure 4A. Seven PAEs were found in ginseng samples, ranging from 0.71 to 2.10 mg·kg−1 (1.16 mg kg−1 on average). The annual, biennial, 3-year-old, 4-year-old, and 5-year-old averages of Σ19PAEs in ginseng roots were 0.71, 0.78, 1.58, 1.02, and 0.87 mg kg−1, respectively. Lower PAEs residues in ginseng samples were observed in the annual ginseng and biennial ginseng, which showed a significant difference (p < 0.05) from those in 3-year-old ginseng. With the growth of ginseng, the 4-year-old and 5-year-old ginseng groups showed a decreasing trend for total PAEs residues. Plant root exudates and their associated microbiota have an important impact on the behavior of soil contaminants (Rodríguez-Garrido et al., 2020). Ginseng has rich root exudates that can provide carbon and energy sources for soil microbes (Girkin et al., 2018). These microbes could promote the degradation or dissipation of organic contaminants (Turkovskaya and Muratova, 2019). The ginseng endophyte Paenibacillus polymyxa was reported to degrade pesticide residues (fluazinam, benzene hexachloride, pentachloronitrobenzene, chlorpyrifos, and dichloro-diphenyl-trichloroethane) (Zhang et al., 2019). Ginseng root exudates and the associated genera of microbes became diverse with the growth of ginseng (4year-old and 5-year-old), which probably enhances the degradation and bioremediation of phthalate acid esters. Similar results have been reported in rice, whose root exudates enhance the desorption of PAEs in soil (Du et al., 2020).
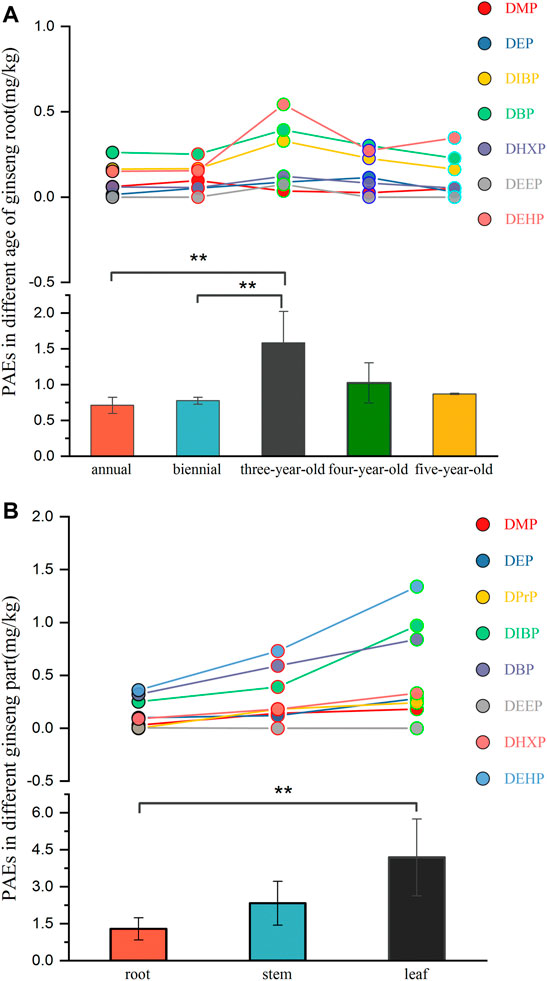
FIGURE 4. Concentrations and distribution of PAEs in ginseng roots under five cultivation ages (annual, biennial, 3-year-old, 4-year-old, and 5-year-old) (A) and ginseng tissues (root, stem, and leaf) (B). ** in the picture indicates significant differences (p < 0.05).
The most abundant PAEs were DIBP, DBP, and DEHP in ginseng, which were also observed from soil and shed films. The ginseng, soil, and shed film samples had nearly the same PAEs, but the PAEs contents in the shed films were greater than those in the soil and ginseng samples. More importantly, the coefficient of determination showed that the level of PAEs residues in ginseng was strongly correlated with the detected PAEs in the soil and film (r = 0.84 and 0.95, respectively; p < 0.01). Compared with other potential sources of PAEs pollution, such as water, air or fertilizers, total concentrations of the main PAEs have been reported to be up to 4.04 μg L−1 in surface water (He et al., 2013), 4,393 ng/m3 in air (∑16 PAEs) (Zeng et al., 2020) and 0.25 mg kg−1 in 22 fertilizers (∑6 PAEs) (Mo et al., 2008). These reported values were much lower than those of PAEs from plastic shed films (average 56.8 mg kg−1). In addition, PAEs can be absorbed by air, water, and soil, especially in plastic greenhouses, and this could threaten crops (Zhou et al., 2021). Therefore, plastic films may be the primary source of PAEs in ginseng samples and their cultivated soils.
Distribution of Phthalate Acid Esters in Ginseng Roots, Stems, and Leaves
Concentrations of the eight detected PAEs in ginseng (root, stem, and leaf) showed significant differences (p < 0.05). The Σ19PAEs in ginseng leaves were 2.37–6.82 mg kg−1 (dw) and 1.56–4.69 mg kg−1 (dw) in ginseng stems and 0.71–2.10 mg kg−1 (dw) roots. Supplementary Table S11 and Figure 4B indicate that the concentrations of DMP, DEP, DPrP, DIBP, DBP, DHXP, and DEHP in ginseng leaves were higher than those in stems and roots. DEEP was only found in individual ginseng roots. A similar distribution of PAEs in celery tissues was reported by Zhao et al. (2022), and the total content of 16 PAEs (DiBP, DBP, DEHP, DEP, DMP, DMEP, BMPP, DPP, DHXP, DEEP, BBP, DCHP, DBEP, DnOP, DNP, and DPhP) was 0.089–1.13 mg kg−1 (dw) in celery stems and 0.16–2.73 mg kg−1 (dw) in celery leaves. Wang et al. (2014) also reported that stem vegetables (celery and lettuce) and leaf vegetables (spinach, cabbage, and pot herb mustard) accumulated more PAEs than root vegetables (radish). Among other environmental pollutants, brominated flame retardants (BFRs) and organophosphate flame retardants (OPFRs) were reported to be taken up by plants through partitioning to root lipids and through gaseous and particle-bound deposition to the leaves (Zhang et al., 2021). This result shows that discrepancies in plant tissue distribution indicated different absorption pathways of PAEs pollutants.
To research the uptake pathways in different tissues of ginseng, the distribution of the concentration of PAEs in ginseng roots, stems, and leaves at different ages is presented in Figure 5 and Supplementary Table S10. Ginseng roots can bioaccumulate PAEs with the years of cultivation, but PAEs residues in ginseng stems and leaves exhibited higher levels in the first year and second year. This result indicated that the accumulation of PAEs in ginseng aboveground tissue caused by root or soil migration is difficult. Most PAEs are hydrophobic compounds with low translocation factors, and DEHP and its metabolite MEHP were proven to have a poor translocation ability from roots to aboveground tissues with a translocation factor below 1 (Cheng et al., 2020). A similar result was found in stem and leaf vegetables, which accumulated more PAEs (Wang et al., 2015; Li et al., 2020). Therefore, PAEs released by plastic shed film were transferred to soil and leaves through atmospheric deposition, which may be the main exposure route by which aboveground ginseng tissues are contaminated. The PAEs contamination of ginseng roots was mainly from the soil. There was a strong correlation between the type and content of PAEs contaminants and ginseng root growth under the ground. Different ginseng tissues have different pollutant exposure pathways and accumulation behavior, but the mechanisms underlying transport pathways require further investigation. Plastic shed films are still one main source of PAEs in cultivation environments. In addition, humans may accumulate PAEs through the food chain. Therefore, it is necessary to assess the health risk of potential hazards from PAEs in edible cultivated ginseng.
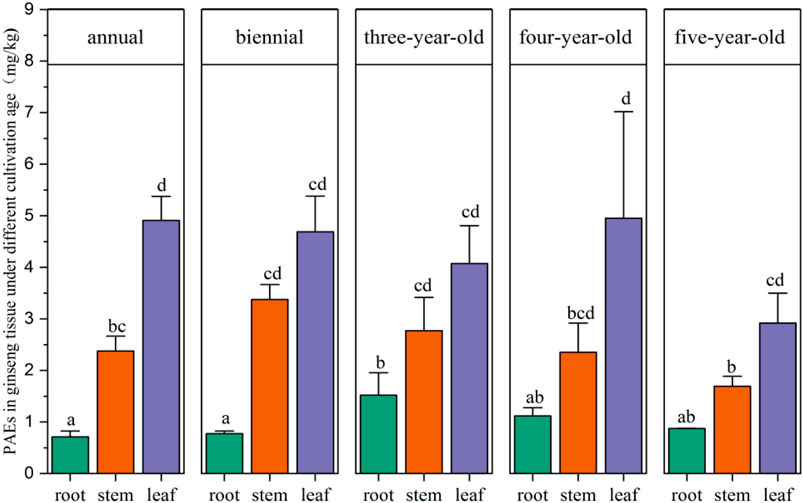
FIGURE 5. Concentrations of PAEs in ginseng roots, stems, and leaves according to cultivation age (annual, biennial, 3-year-old, 4-year-old, and 5-year-old). Labels containing the same letters indicate no significant difference (p > 0.05). Labels in different letters indicate significant difference (p < 0.05).
Human Health Risk Assessments for Ingested Ginseng
Since 2012, the Ministry of Health has issued “the Announcement on the Approval of Ginseng (Artificially Cultivated) as a New Resource Food” Restrictions on ginseng use will be lifted, and adults can eat no more than 3 g of ginseng per day. According to US EPA 2013 guidelines, DMP, DEP, DBP, and DEHP may result in noncarcinogenic risk through the maximal reference doses as recommended, while DEHP was related to cancer risks. The calculated HIs of the target PAEs from the collected cultivated ginseng samples (n = 23) are shown in Figure 6. This result showed that the noncarcinogenic risk of PAEs to consumer health was slight for the ginseng cultivation bases in Jilin Province, and DEHP was the main phthalate posing noncarcinogenic risk. The CR of DEHP through ginseng ingestion ranged from 1.80×10–8 to 1.58×10–7, as shown in Supplementary Table S12, and values below 1×10–6 are negligible for humans. In total, the results of PAEs risk assessments for ginseng indicated that cultivated ginseng from the Jilin Province farmland cultivation bases was acceptable.
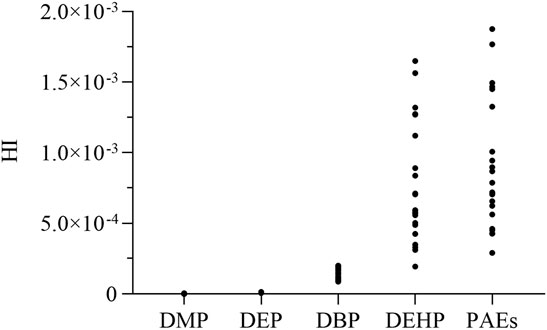
FIGURE 6. The hazard index (HI) was calculated to assess the noncancer risk posed by the intake of phthalate acid esters (PAEs) in ginseng roots. Black dots represent the HI value of the target PAEs per ginseng sample.
Conclusion
This study explored the occurrence of PAEs residues in soils, shed films, and ginseng tissues of plants of different ages from the ginseng cultivation bases in Jilin Province. The predominant PAEs were DEHP, DIBP, and DBP, with over 88%, 94%, and 80% of Σ19PAEs in soil, plastic shed film, and ginseng roots, respectively. As for soils, the concentration of DBP (0.83 mg kg−1 on average) and DMP (0.05 mg kg−1 on average) reveals a serious situation of PAEs pollution in soil from ginseng cultivation bases in Jilin Province. The PAEs concentration in ginseng roots exhibited a closer relationship with those in soil and plastic films, indicating that the widespread use of plastic shed films is possibly the main potential source of PAEs in ginseng cultivation bases. In addition, the accumulation of PAEs in ginseng roots was easily affected by cultivation years. Three-year-old ginsengs had higher PAEs residues than annual and biennial ginsengs (p < 0.05). In addition, PAEs were more readily accumulated in ginseng leaves than in roots and stems (p < 0.05), which indicated different uptake pathways of PAEs contaminants in different ginseng tissues. Risk assessment showed that the noncarcinogenic risk was mainly due to DEHP. Carcinogenic and noncarcinogenic risks via ginseng dietary routes were within acceptable levels. Overall, this work could be useful for understanding the characteristics and distribution of PAEs pollution in ginseng cultivation areas.
Data Availability Statement
The original contributions presented in the study are included in the article/Supplementary Material; further inquiries can be directed to the corresponding authors.
Author Contributions
Y-SL: investigation, validation, formal analysis, methodology, and writing-original draft. Y-YX: methodology, writing-review, and editing. Y-TZ: formal analysis and methodology. Z-BL: methodology. WL: resources. Y-SS: conceptualization, supervision, and resources.
Conflict of Interest
The authors declare that the research was conducted in the absence of any commercial or financial relationships that could be construed as a potential conflict of interest.
Publisher’s Note
All claims expressed in this article are solely those of the authors and do not necessarily represent those of their affiliated organizations, or those of the publisher, the editors, and the reviewers. Any product that may be evaluated in this article, or claim that may be made by its manufacturer, is not guaranteed or endorsed by the publisher.
Acknowledgments
This work was supported by Natural Science Foundation of Jilin Province (YDZJ202201ZYTS437) and Technology Innovation Project of Chinese Academy of Agricultural Sciences(CAAS-ASTIP-ISAPS-2021-010).
Supplementary Material
The Supplementary Material for this article can be found online at: https://www.frontiersin.org/articles/10.3389/fenvs.2022.917508/full#supplementary-material
References
Arfaeinia, H., Fazlzadeh, M., Taghizadeh, F., Saeedi, R., Spitz, J., and Dobaradaran, S. (2019). Phthalate Acid Esters (PAEs) Accumulation in Coastal Sediments from Regions with Different Land Use Configuration along the Persian Gulf. Ecotoxicol. Environ. Saf. 169, 496–506. doi:10.1016/j.ecoenv.2018.11.033
Cai, Q.-Y., Xiao, P.-Y., Chen, T., Lü, H., Zhao, H.-M., Zeng, Q.-Y., et al. (2015). Genotypic Variation in the Uptake, Accumulation, and Translocation of Di-(2-ethylhexyl) Phthalate by Twenty Cultivars of Rice (Oryza Sativa L.). Ecotoxicol. Environ. Saf. 116, 50–58. doi:10.1016/j.ecoenv.2015.02.038
Chai, C., Cheng, H., Ge, W., Ma, D., and Shi, Y. (2014). Phthalic Acid Esters in Soils from Vegetable Greenhouses in Shandong Peninsula, East China. Plos One 9 (4), e95701. doi:10.1371/journal.pone.0095701
Chang, B. V., Lu, Y. S., Yuan, S. Y., Tsao, T. M., and Wang, M. K. (2009). Biodegradation of Phthalate Esters in Compost-Amended Soil. Chemosphere 74 (6), 873–877. doi:10.1016/j.chemosphere.2008.10.003
Cheng, Z., Yao, Y., and Sun, H. (2020). Comparative Uptake, Translocation and Subcellular Distribution of Phthalate Esters and Their Primary Monoester Metabolites in Chinese Cabbage (Brassica Rapa Var. Chinensis). Sci. Total Environ. 742, 140550. doi:10.1016/j.scitotenv.2020.140550
Du, P.-P., Huang, Y.-H., Lü, H., Xiang, L., Li, Y.-W., Li, H., et al. (2020). Rice Root Exudates Enhance Desorption and Bioavailability of Phthalic Acid Esters (PAEs) in Soil Associating with Cultivar Variation in PAE Accumulation. Environ. Res. 186, 109611. doi:10.1016/j.envres.2020.109611
Girkin, N. T., Turner, B. L., Ostle, N., Craigon, J., and Sjögersten, S. (2018). Root Exudate Analogues Accelerate CO2 and CH4 Production in Tropical Peat. Soil Biol. Biochem. 117, 48–55. doi:10.1016/j.soilbio.2017.11.008
He, L., Gielen, G., Bolan, N. S., Zhang, X., Qin, H., Huang, H., et al. (2015). Contamination and Remediation of Phthalic Acid Esters in Agricultural Soils in China: a Review. Agron. Sustain. Dev. 35 (2), 519–534. doi:10.1007/s13593-014-0270-1
He, W., Qin, N., Kong, X., Liu, W., He, Q., Ouyang, H., et al. (2013). Spatio-temporal Distributions and the Ecological and Health Risks of Phthalate Esters (PAEs) in the Surface Water of a Large, Shallow Chinese Lake. Sci. Total Environ. 461-462, 672–680. doi:10.1016/j.scitotenv.2013.05.049
Katsoyiannis, A. (2006). Occurrence of Polychlorinated Biphenyls (PCBs) in the Soulou Stream in the Power Generation Area of Eordea, Northwestern Greece. Chemosphere 65 (9), 1551–1561. doi:10.1016/j.chemosphere.2006.04.004
Kluwe, W. M., Haseman, J. K., Douglas, J. F., and Huff, J. E. (1982). The carcinogenicity of dietary di(2‐ethylhexyl) phthalate (DEHP) in fischer 344 rats and B6C3F1mice. J. Toxicol. Environ. Health 10 (4-5), 797–815. doi:10.1080/15287398209530296
Li, C., Chen, J., Wang, J., Han, P., Luan, Y., Ma, X., et al. (2016). Phthalate Esters in Soil, Plastic Film, and Vegetable from Greenhouse Vegetable Production Bases in Beijing, China: Concentrations, Sources, and Risk Assessment. Sci. Total Environ. 568, 1037–1043. doi:10.1016/j.scitotenv.2016.06.077
Li, Q., Zeng, A., Jiang, X., and Gu, X. (2021). Are Microplastics Correlated to Phthalates in Facility Agriculture Soil? J. Hazard. Mater. 412, 125164. doi:10.1016/j.jhazmat.2021.125164
Li, Y., Yan, H., Li, X., Ge, J., Cheng, J., and Yu, X. (2020). Presence, Distribution and Risk Assessment of Phthalic Acid Esters (PAEs) in Suburban Plastic Film Pepper-Growing Greenhouses with Different Service Life. Ecotoxicol. Environ. Saf. 196, 110551. doi:10.1016/j.ecoenv.2020.110551
Lu, Y.-S., Yao, G.-X., Wang, X.-L., Liu, J.-X., Yu, J., Qiu, J., et al. (2020). A Comprehensive Analysis of Metabolomics and Transcriptomics Reveals New Biomarkers and Mechanistic Insights on DEHP Exposures in MCF-7 Cells. Chemosphere 255, 126865. doi:10.1016/j.chemosphere.2020.126865
Ma, T. T., Christie, P., Luo, Y. M., and Teng, Y. (2013). Phthalate Esters Contamination in Soil and Plants on Agricultural Land Near an Electronic Waste Recycling Site. Environ. Geochem Health 35 (4), 465–476. doi:10.1007/s10653-012-9508-5
Ma, T. T., Wu, L. H., Chen, L., Zhang, H. B., Teng, Y., and Luo, Y. M. (2015). Phthalate Esters Contamination in Soils and Vegetables of Plastic Film Greenhouses of Suburb Nanjing, China and the Potential Human Health Risk. Environ. Sci. Pollut. Res. 22 (16), 12018–12028. doi:10.1007/s10653-012-9508-510.1007/s11356-015-4401-2
Mo, C.-H., Cai, Q.-Y., Li, Y.-H., and Zeng, Q.-Y. (2008). Occurrence of Priority Organic Pollutants in the Fertilizers, China. J. Hazard. Mater. 152 (3), 1208–1213. doi:10.1016/j.jhazmat.2007.07.105
Niu, L., Xu, Y., Xu, C., Yun, L., and Liu, W. (2014). Status of Phthalate Esters Contamination in Agricultural Soils across China and Associated Health Risks. Environ. Pollut. 195, 16–23. doi:10.1016/j.envpol.2014.08.014
Ren, L., Jia, Y., Ruth, N., Qiao, C., Wang, J., Zhao, B., et al. (2016). Biodegradation of Phthalic Acid Esters by a Newly Isolated Mycobacterium Sp. YC-RL4 and the Bioprocess with Environmental Samples. Environ. Sci. Pollut. Res. 23 (16), 16609–16619. doi:10.1007/s11356-016-6829-4
Rodríguez-Garrido, B., Balseiro-Romero, M., Kidd, P. S., and Monterroso, C. (2020). Effect of Plant Root Exudates on the Desorption of Hexachlorocyclohexane Isomers from Contaminated Soils. Chemosphere 241, 124920. doi:10.1016/j.chemosphere.2019.124920
Steinmetz, Z., Wollmann, C., Schaefer, M., Buchmann, C., David, J., Tröger, J., et al. (2016). Plastic Mulching in Agriculture. Trading Short-Term Agronomic Benefits for Long-Term Soil Degradation? Sci. Total Environ. 550, 690–705. doi:10.1016/j.scitotenv.2016.01.153
Tang, J., Rong, X., Jin, D., Gu, C., Chen, A., and Luo, S. (2020). Biodegradation of Phthalate Esters in Four Agricultural Soils: Main Influencing Factors and Mechanisms. Int. Biodeterior. Biodegrad. 147, 104867. doi:10.1016/j.ibiod.2019.104867
Turkovskaya, O., and Muratova, A. (2019). Plant-Bacterial Degradation of Polyaromatic Hydrocarbons in the Rhizosphere. Trends Biotechnol. 37 (9), 926–930. doi:10.1016/j.tibtech.2019.04.010
Wang, J., Bo, L., Li, L., Wang, D., Chen, G., Christie, P., et al. (2014). Occurrence of Phthalate Esters in River Sediments in Areas with Different Land Use Patterns. Sci. Total Environ. 500-501, 113–119. doi:10.1016/j.scitotenv.2014.08.092
Wang, J., Chen, G., Christie, P., Zhang, M., Luo, Y., and Teng, Y. (2015). Occurrence and Risk Assessment of Phthalate Esters (PAEs) in Vegetables and Soils of Suburban Plastic Film Greenhouses. Sci. Total Environ. 523, 129–137. doi:10.1016/j.scitotenv.2015.02.101
Wang, K., Li, L., Zhao, M., Li, S., Sun, H., Zhang, M., et al. (2018). Characterization of the Complete Chloroplast Genome of Jilin Ginseng (Panax Ginseng C. A. Meyer) Using Next Generation Sequencing. Mitochondrial DNA Part B 3 (2), 685–686. doi:10.1080/23802359.2018.1462122
Xu, G., Li, F., and Wang, Q. (2008). Occurrence and Degradation Characteristics of Dibutyl Phthalate (DBP) and Di-(2-ethylhexyl) Phthalate (DEHP) in Typical Agricultural Soils of China. Sci. Total Environ. 393 (2-3), 333–340. doi:10.1016/j.scitotenv.2008.01.001
Zeng, F., Cui, K., Xie, Z., Wu, L., Liu, M., Sun, G., et al. (2008). Phthalate Esters (PAEs): Emerging Organic Contaminants in Agricultural Soils in Peri-Urban Areas Around Guangzhou, China. Environ. Pollut. 156 (2), 425–434. doi:10.1016/j.envpol.2008.01.045
Zeng, L.-J., Huang, Y.-H., Chen, X.-T., Chen, X.-H., Mo, C.-H., Feng, Y.-X., et al. (2020). Prevalent Phthalates in Air-Soil-Vegetable Systems of Plastic Greenhouses in a Subtropical City and Health Risk Assessments. Sci. Total Environ. 743, 140755. doi:10.1016/j.scitotenv.2020.140755
Zhang, Q., Yao, Y., Wang, Y., Zhang, Q., Cheng, Z., Li, Y., et al. (2021). Plant Accumulation and Transformation of Brominated and Organophosphate Flame Retardants: A Review. Environ. Pollut. 288, 117742. doi:10.1016/j.envpol.2021.117742
Zhang, X., Gao, Y., Zang, P., Zhao, Y., He, Z., Zhu, H., et al. (2019). Study on the Simultaneous Degradation of Five Pesticides by Paenibacillus Polymyxa from Panax Ginseng and the Characteristics of Their Products. Ecotoxicol. Environ. Saf. 168, 415–422. doi:10.1016/j.ecoenv.2018.10.093
Zhao, F., Ma, Z., Ping, H., He, Z., Li, B., Gao, Y., et al. (2022). Tissue Distribution of Phthalates in Celery under Different Cultivation Patterns and Associated Dietary Exposure. Environ. Pollut. 292 (Pt B), 118391. doi:10.1016/j.envpol.2021.118391
Zhou, B., Zhao, L., Sun, Y., Li, X., Weng, L., and Li, Y. (2021). Contamination and Human Health Risks of Phthalate Esters in Vegetable and Crop Soils from the Huang-Huai-Hai Region of China. Sci. Total Environ. 778, 146281. doi:10.1016/j.scitotenv.2021.146281
Zhou, B., Zhao, L., Wang, Y., Sun, Y., Li, X., Xu, H., et al. (2020). Spatial Distribution of Phthalate Esters and the Associated Response of Enzyme Activities and Microbial Community Composition in Typical Plastic-Shed Vegetable Soils in China. Ecotoxicol. Environ. Saf. 195, 110495. doi:10.1016/j.ecoenv.2020.110495
Keywords: phthalate acid esters, soil, ginseng tissue, cultivation age, risk assessment
Citation: Lu Y-S, Xu Y-Y, Zhang Y-T, Liu Z-B, Li W and Sun Y-S (2022) Phthalate Acid Esters in Soil, Plastic Shed Film, and Ginseng Tissues of Different Ages From Farmland: Concentration, Distribution, and Risk Assessment. Front. Environ. Sci. 10:917508. doi: 10.3389/fenvs.2022.917508
Received: 11 April 2022; Accepted: 23 May 2022;
Published: 01 July 2022.
Edited by:
Jesús Rodrigo-Comino, University of Granada, SpainReviewed by:
Wenjie Ren, Institute of Soil Science (CAS), ChinaXiaojing Li, Agro-Environmental Protection Institute (CAAS), China
Copyright © 2022 Lu, Xu, Zhang, Liu, Li and Sun. This is an open-access article distributed under the terms of the Creative Commons Attribution License (CC BY). The use, distribution or reproduction in other forums is permitted, provided the original author(s) and the copyright owner(s) are credited and that the original publication in this journal is cited, in accordance with accepted academic practice. No use, distribution or reproduction is permitted which does not comply with these terms.
*Correspondence: Yin-Shi Sun, sunyinshi2015@163.com