Effect of Hydrothermal Pretreatment on Anaerobic Digestion of Erythromycin Fermentation Dregs: Biogas Production, Antibiotic Resistance Gene Evolution, and Microbial Community Dynamics
- College of Environment and Safety Engineering, Qingdao University of Science and Technology, Qingdao, China
The utilization of erythromycin fermentation dregs (EFD), one kind of solid biowaste, is limited due to the high-level residue of antibiotics. Hydrothermal pretreatment (HT) has great potential to remove residual antibiotics. However, its harmless performance and influence on the EFD anerobic digestion (AD) process remains unclear. In this study, HT was conducted for erythromycin removal before EFD AD with the temperature ranging from 80 to 180°C. Moreover, changes in biogas yield, antibiotic resistance genes (ARGs), and microbial communities in the EFD AD process were compared among different treatments. The results showed that under the optimal hydrothermal temperature of 160°C, more than 85% of erythromycin was eliminated. In addition, HT significantly reduced the ARGs in the EFD AD process and ermT and mefA relative abundance decreased by one order of magnitude. Mobile genetic elements (IntI1 and Tn916/1545) also showed decreased tendency with the hydrothermal temperature elevation. The maximum methane production of 428.3 ml g−1 VS was obtained in the AD system of EFD with hydrothermal treatment at 160°C. It is attributed to the cooperation of hydrolysis and acidogenesis bacteria (e.g., Aminicenantales and Sedimentibacter) and methylotrophic methanogens (Candidatus_Methanofastidiosum and Methanosarcina), and they presented the highest relative abundance in this group. The results indicated that methylated substance reduction was the major methanogenesis route. Hydrothermal technology was of great potential to realize the harmless treatment of EFD and for recycling EFD via AD.
1 Introduction
Antibiotics have been widely used in clinical medicine, animal husbandry, and other fields (David et al., 2021; Gamble et al., 2022). Most antibiotics are manufactured by microbiological fermentation, which produces millions of tons of byproducts – antibiotic fermentation dregs (AFD) (Wang et al., 2022). AFD is one kind of organic solid waste and has great potential to be fertilizer or feedstuff because it contains lots of organic carbon such as protein and polysaccharides (Xiao et al., 2015; Zhang T. et al., 2015; Shen et al., 2019). However, residual unextracted antibiotics limit its use due to possible ecosystem risks (Kemper, 2008). Erythromycin fermentation dregs (EFD) with 640 mg kg−1 residual erythromycin and 90% water content have raised concerns among all the AFD (Zhang et al., 2019). It could not only pose threats to living species such as Daphnia magna and Selenastrum capricornutum but also present selecting pressure of antibiotic bacteria and promote the spread of antibiotic resistant genes, if without reasonable disposal (Zhang et al., 2019). Moreover, the EFD is the reservoir of ARGs, for example, the relative abundance of ermB is 6.82 × 10−4copies/16S rRNA copies (Zhang et al., 2019). The ARGs in the environment pose great threats to human health, especially those related to human pathogens. Thus, the development of suitable treatment methods is encouraged, as they will enable resource utilization and pollution control (erythromycin and ARGs) of EFD.
Anerobic digestion (AD) is of great advantage for the treatment of EFD as it can remove some contaminants and convert biomass into biogas at the same time (Syafiuddin and Boopathy, 2021). The AD process includes four stages, hydrolysis, acidogenesis, acetogenesis, and methanogenesis, and each stage requires specific bacteria and archaea (Pang et al., 2022). As AD involves multiple equilibria between microbial metabolism, the substance that affects the microbial activity could influence the AD performance, visualizing biogas production (Pang et al., 2021). Numerous studies have investigated the influence of antibiotics on the AD process. The biogas yield decreased significantly as the clarithromycin increased to 10 mg L−1 (Zeng et al., 2021). Florfenicol with a concentration of 36 mg L−1 could reduce 40% of methane production (Mitchell et al., 2013). In addition, antibiotics were the major driver of ARG propagation in some biological processes (Bai et al., 2019; Ni et al., 2020). Lu et al. (2021) confirmed the accumulation of antibiotics in agricultural soil, inducing the enrichment of ARGs. Li et al. (2022) found positive relationships between antibiotics and ARGs in swine manure composting process. According to previous research, antibiotics do not only inhibit methane production but also induce an increase in ARG. Moreover, the flocs and mycelium in EFD decreased the anaerobic biodegradability. Thus, to guarantee the favorable AD performance of EFD and maximum ARG reduction, a pretreatment must be performed preceding the AD processes.
Hydrothermal technology is one of the most promising pretreatment methods for EFD as it is highly efficient at antibiotic elimination and is environmentally friendly (Wang et al., 2018a). In addition, this technology could destroy floc structure and microbial cell walls, making refractory organic matters available for AD (Wang et al., 2018a). As reported, the hydrothermal temperature is the most important factor affecting pretreatment performance (Chen et al., 2020). Previous research has evaluated the temperature influences on sludge hydrolysis ranging from 60 to 270°C and confirmed that the effective condition was between 160 and 180°C (Pilli et al., 2015). Toutian et al. (2020) found some recalcitrant soluble organics, which would be produced as the temperature increased above 180°C and that reduced anaerobic biodegradability. Chen et al. (2018) reported that the sludge with hydrothermal treatment at 160°C for 30 min in the AD process increased 33.4% biogas production compared to no pretreatment. Moreover, Li and Jin (2015) investigated the properties with the thermal pretreatment (55–160°C) and found that the highest methane yield was obtained from those pretreated at 90 and 120°C for 70 and 50 min, respectively. However, the appropriate treatment temperatures of EFD were unclear. The influences of hydrothermal treatment on the EFD AD process such as methane production, antibiotics, and ARG removal remain to be investigated.
Therefore, the purpose of this study is to comprehensively explore the influence of hydrothermal treatment on the EFD AD process from the aspects of methane production, ARG abundance, and community composition of bacteria and archaea. First, the methane yield of EFD was evaluated at different hydrothermal temperatures (80, 120, 160, and 180°C). Then, the changes of typical ARGs and MEGs in the EFD AD process were monitored and the correspondent mechanisms were analyzed. Finally, the bacterial and archaeal community structures were investigated during the EFD AD process with HT.
2 Methods and Materials
2.1 Materials
In this study, the EFD was obtained from a pharmaceutical company in Xinjiang, China. The water content of EFD was about 90% and the residual concentration of erythromycin (ERY) in the raw EFD was around 720 ± 56 mg kg−1. Anerobic seed sludge was collected from a sewage treatment plant in Shanghai, China. It was cultured at 37°C for 14 days to recover the activity of microbial organisms before use. The basic physicochemical properties of the sludge and EFD were listed in Table1. Briefly, the total solid contents of EFD and inoculum sludge were 10 ± 0.9% and 13.6 ± 0.1%, respectively, and the VS contents were 85.2 ± 1.1% and 92.1 ± 1.2%, correspondingly.
2.2 HT Experiment
The hydrothermal experiment was conducted in a stainless steel columnar reactor with a Teflon liner of 300 ml volume. Five treatments including control and hydrothermally treated with different temperatures were carried out in the electric oven independently (Cai et al., 2020). The preset reaction temperatures are 80, 120, 160, and 180°C. In detail, 150 g of the homogenized EFD sample was added to each reactor and was allowed to react at the preset temperature for 60 min. The reaction was thought to start when the oven reached the preset temperature (80, 120, 160, and 180°C). After that, the sample was taken out of the oven and cooled naturally.
2.3 AD Test
4The AD experiment was conducted in 500-ml wide-mouthed bottles with 300 ml working volume. The mixture of hydrothermal pretreated EFD and seed sludge was inoculated in the bottles with a total volume of 200 ml. Particularly, the ratio of the inoculum to the substrate (treated EFD) was 2:1 based on the VS content. The tests with only 200 ml inoculum sludge were set as the control (Cai et al., 2021). The pH value of all the tests was adjusted to 6.8–7.2, and all the anerobic bottles were flushed with nitrogen for 5 min to remove oxygen, creating a suitable anerobic condition. The AD experiment was performed in a shaking incubator with a constant temperature of 37 ± 1°C. The shaking speed was 80 r min−1 in the total experiment. The generated gas was collected with a gas bag, and the concentration of ERY and routine parameters such as soluble protein, soluble carbohydrate total solid (TS), volatile solid (VS), chemical oxygen demand (COD), ammonia nitrogen (NH4+ - N), and total nitrogen (TN) were measured every day. The evolution of ARGs and microbial community was analyzed after being cultured for 15 and 30 days, respectively. Three parallel experiments were set up in each group.
2.4 Analytical Methods
TS, VS, COD, NH4+ - N, and TN were analyzed according to the standard APHA method (Cai et al., 2021). The Lowry–Folin method and Anthrone method were used to measure protein and carbohydrates, respectively (Classics Lowry et al., 1951; Raunkjær et al., 1994). The cumulative methane production was determined by the automatic flow measurement unit of Automatic Methane Potential Test System II (Bioprocess Control, Sweden). The TOC analyzer was used to analyze the dissolved organic carbon (DOC) content (TOCV, Shimadzu, Japan). The ERY concentration in the hydrothermally treated EFD and digestate was determined based on the study of Zhang G. et al. (2015). Briefly, the erythromycin in the EFD was ultrasonically extracted with the extraction solution (30 ml 0.01 M Tris-0.02 M CaCl2, pH = 6) for 30 min. Then, the supernatant obtained by centrifugation was further purified via liquid–liquid microextraction. After concentration, the extracts were reconstituted into 1 ml methanol and loaded into 2-ml screwcap amber glass vials for analysis. An Agilent 1210 liquid chromatography (Agilent, Palo Alto, CA, United States) equipped with a C18 chromatographic column (Waters-Xbridge C18, 3.5 μm, 4.6 × 150 mm) was used to analyze the erythromycin content. The mobile phase was made up of MeOH and 0.1% formic acid (55:45) with a flow rate of 1 ml min−1.
The total microbial genomic DNA was extracted via the FastDNA spin Kit (MP biomedical, United States) according to the manufacturer’s instructions and stored at −20°C before analysis. The quality of extracted DNA was checked by a NanoDrop spectrophotometer (Thermo Fisher Scientific, Waltham, MA, United States). The abundance of target resistance genes was quantified by real-time qPCR (Wegene Bio-technology, Shanghai). Seven erythromycin resistance genes, namely, ereA, ereB, ermA, ermB, ermT, mefA, and mphA were selected in this research. At the same time, two kinds of MGEs (IntI1 and Tn916/1545) and 16S rRNA genes were quantitatively analyzed. The relative abundance of target genes was standardized by the 16S RNA gene. Three repeats were conducted for the quality control. Moreover, the microbial communities among different AD treatments were analyzed via MiSeq sequencing.
2.5 Statistical Analysis
The relative abundance histogram of the target genes was drawn with OriginPro 9.0 (OriginLabInc., United States). SPSS was used for one-way analysis of variance (ANOVA). Principal component analysis (PCA) was conducted by R with the ggplot2 package. The co-occurrence network analysis of the ARGs and their potential hosts was performed and visualized by Gephi 0.9.1.
3 Results and Discussion
3.1 Erythromycin Degradation in Hydrothermal Treatment
Erythromycin concentration and removal rate after the hydrothermal treatment was examined (Figure 1). Erythromycin concentration decreased evidently as the temperature increased from 80 to 180°C. As shown in Figure 1, only 5% of erythromycin was removed in the hydrothermal treatment with the temperature of 80°C. Although the removal efficiency enhanced to 18.2% at 120°C, around 589 mg kg−1 erythromycin was left in the EFD. As the hydrothermal treatment temperature reached 160 and 180°C, the erythromycin removal rate increased to 88.5 and 90.1%, respectively. The results indicated that the erythromycin removal rate increased with the hydrothermal temperature which was in accordance with previous research (Zhang et al., 2019). It is attributed to the fact that the rising temperature increased the number of activated molecules and enhanced molecular mobility, resulting in elevated degradation rate constants (Wang et al., 2018b). To remove more erythromycin from the EFD, a higher hydrothermal temperature was encouraged. However, it means more energy consumption. In addition, the high temperature would lead to microbial inaccessibility as the tar and char would be produced at a temperature above 220°C for 30 min (Zhang et al., 2014). In this research, there was no significant removal efficiency improvement as the hydrothermal temperature increased from 160 to 180°C. Thus, to ensure considerable erythromycin removal and enough soluble organics in the EFD for microbial accessibility, the optimal hydrothermal temperature of 160°C was chosen.
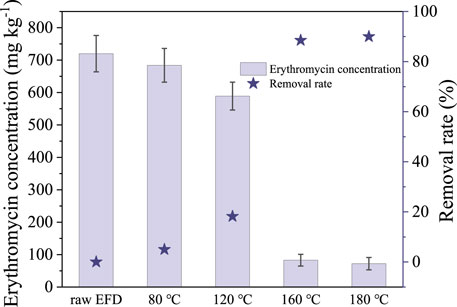
FIGURE 1. Changes of erythromycin concentration in the erythromycin fermentation dregs (EFD) with different hydrothermal treatments.
3.2 Methanogenic Performance
The cumulative and daily methane production of the EFD hydrothermally treated with different temperatures is presented in Figure 2. The methanogenesis was enhanced gradually with the treatment temperature increasing from 80 to 160°C (Figure 2A). However, as the treatment temperature reached 180°C, methane production decreased. It might be because of the Maillard effect which consumed the soluble compounds in the EFD at high temperature (Vhangani and Van Wyk, 2021). In addition, except for the raw sludge, the methane production of all the groups gradually leveled off in 10–15 days and moved toward the platform stage. The maximum accumulative methane yield (428.3 ml g VS−1) was obtained in the EFD with hydrothermal treatment at 160°C, which was 16% higher than that of the raw EFD. It was in line with the results in 3.1 that hydrothermal temperature at 160°C showed the largest erythromycin removal. The cumulative methane production of the raw EFD and EFD hydrothermally treated at 180, 120, and 80°C were 369.2, 360.6, 358.6, and 341.4 ml g VS−1, respectively. There was no significant difference in the cumulative biogas production between the raw EFD and those treated at 180 and 120°C. However, methane yield was lowest in EFD treated at 80°C, which might be because this condition could not destroy the super colloidal structure of EFD, hindering the dissolution of organic matters (Yang et al., 2019). However, few of the released organics absorbed by the EFD particles also inhibited methane production. As shown in Figure 2B, there were two prominent sites in the daily methane production. EFD treated at 160°C had the peak biogas production in both sites, which also led to the highest cumulative methane production. Overall, the methane production of all the groups increased in the first 2 days, followed by a gradual decrease on the third and fourth days due to the consumption of dissolved organic matters. After 5 days, all the groups presented positive methane production potential, possibly attributed to the alleviation inhibition of antibiotics which usually inhibited the activity of hydrolysis and acidogenesis bacteria (Tian et al., 2018). As the digestion progressed, the bacterial activity rebounded, and the organic macromolecule decomposes into small molecules, promoting methane production (Zhu et al., 2017).
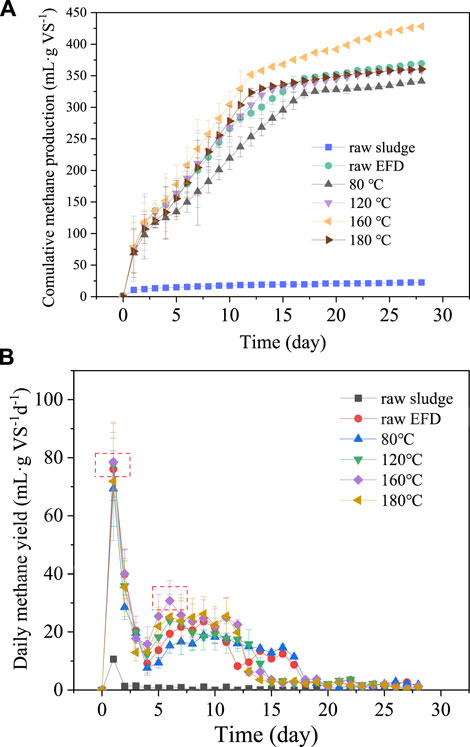
FIGURE 2. Cumulative methane production (A) and daily methane production (B) of the erythromycin fermentation dregs (EFD) with hydrothermal treatment at different temperatures.
3.3 ARG and MGE Evolution in the EFD AD Process After Different Hydrothermal Treatment
The changes of seven macrolide ARG relative abundances in the hydrothermally treated EFD AD system are described in Figure 3. The relative abundances of almost all the ARGs in the AD systems in 30 days were lower than those in 15 days. This might be due to the ARG host reduction in 30 days for the consumption of organics and nutrients in the digestate (Zarei-Baygi and Smith, 2021). As reported, esterase type I and II encoded by esterase genes (ereA, ereB, and mphA) could hydrolyze the erythromycin lactone ring, conferring the resistance of microorganisms to erythromycin (Morar et al., 2012). In this research, the relative abundance of ereA showed no significant difference among different hydrothermal treatments, while ereB presented the lowest amount in the EFD with 120°C hydrothermal treatment, indicating that this condition was not suitable for ereB proliferation. mphA relative abundance showed apparent positive relationships with the hydrothermal temperature because the high temperature means less residual erythromycin in the EFD. The rRNA methylase genes (ermB and ermT) exhibit resistance via encoding methylase that could prevent hydrogen bond formation and hold back the binding of erythromycin with the acting site and the efflux pump genes (mefA) via encoding the membrane-bound efflux protein to efflux antibiotics. All of these genes (ermB, ermT, and mefA) decreased in the EFD AD system with the increasing hydrothermal temperature. This was because HT destroyed the residual erythromycin in the EFD and mitigated the selective pressure (Gong et al., 2020). Based on the results given above, HT was available to inhibit the dissemination of ARGs in EFD during the AD process and promote the EFD recycling utilization.
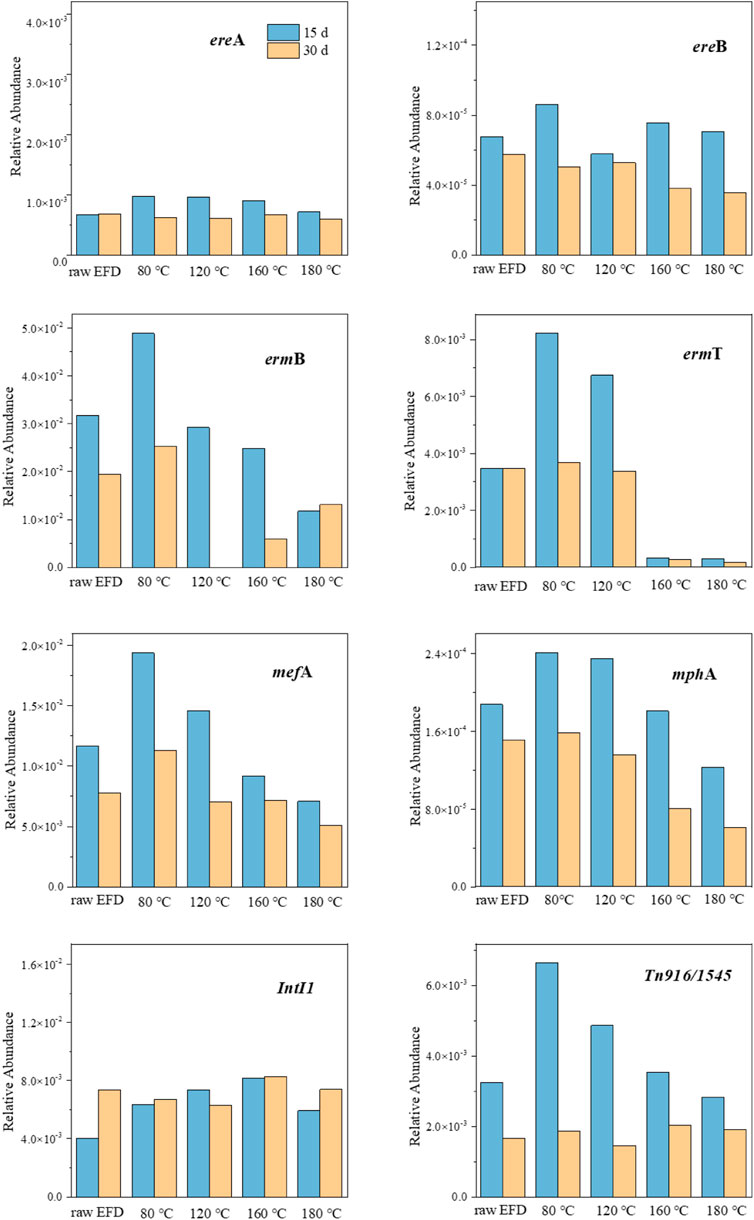
FIGURE 3. Relative abundance changes of antibiotic resistance genes (ARGs) and mobile genetic elements (MGEs) during AD of the erythromycin fermentation dregs (EFD) with different hydrothermal treatments.
Two MGEs (IntI1 and Tn916/1545) were selected to indicate the spread of potential risks of ARGs in the EFD. The relative abundance of IntI1 showed no significant difference in the AD process of EFD with different HT (Figure 3). In comparison, HT led to Tn916/1545 relative abundance, increasing in the first 15 days of the AD process, implying that Tn916/1545 has broad hosts and the decomposition of EFD super colloidal structure promoting their propagation (Guo et al., 2020). With the hydrothermal temperature elevating, all the substances in the EFD were destroyed and Tn916/1545 relative abundance presented decreased tendency. Moreover, as the digested time extended to 30 days, it exhibited no significant difference among all the groups, indicating that HT did not increase the HGT potential via Tn916/1545. Overall, HT eliminated the residual antibiotics in the EFD and decreased the ARG and MGE relative abundance in the subsequent AD process.
3.4 Variations in Microbial Communities During the EFD AD Process With Different Hydrothermal Treatments
3.4.1 Bacterial Community Analysis
Figure 4 showed the composition changes of the bacterial community during the AD process of EFD with different hydrothermal treatments. Firmicutes, Bacteroidetes, Proteobacteria, Chloroflexi, Acidobacteria, and Cloacimonetes were the dominant phyla (Figure 4A) that played a crucial role in the reaction process. Among them, Firmicutes presented the highest relative abundance. It is because most of the Firmicutes could produce endospores, which are resistant to dehydration and extreme environments (Filippidou et al., 2016). Thus, in the antibiotic concentrated EFD AD process, Firmicutes were abundant. However, its relative abundance decreased with the AD extended to 30 days. It might be because other phylum bacteria gradually adapted to the environment and caused niche competition (Peng et al., 2020). Moreover, the relative abundance of the top 20 bacteria was listed on the genus level (Figure 4B). Aminicenantales, Longilinea, Sedimentibacter, norank_o_DTU014, and Syntrophomonas were the dominant genera.
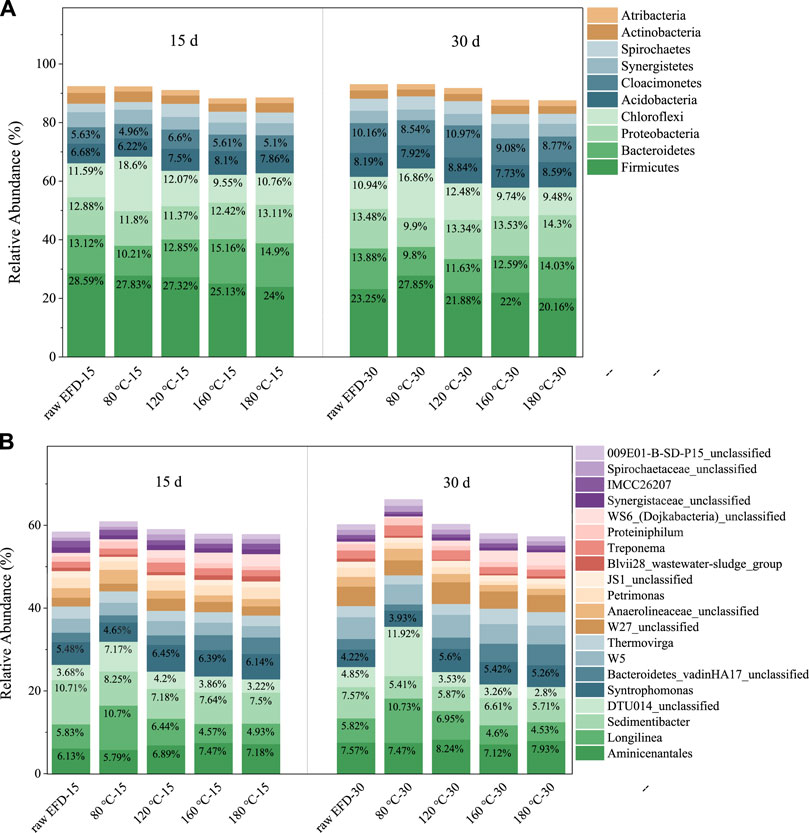
FIGURE 4. Changes of dominant phylum (A) and genus (B) during AD of the erythromycin fermentation dregs (EFD) with hydrothermal treatment at different temperatures.
Aminicenantales was the most abundant bacteria in all the AD systems. It belongs to Aminicenantes (candidate phylum OP8) which preferred the hydrocarbon-impacted environments, especially in low oxygen tension (Farag et al., 2014). Its relative abundance was highest in the 160°C hydrothermal treated group, indicating the best performance of hydrolysis and acidogenesis. Sedimentibacter, one kind of acidogenic genera, was the second abundant genus in the EFD AD system. It took part in the volatile fatty acid production and electron transfer to methanogens, therefore promoting methane yield (Regueiro et al., 2014). Moreover, Longilinea belonging to Anaerolineae, a type of microbiota that can use glucose, arabinose, and fructose, played significant roles in hydrolysis and acidogenesis (Chen et al., 2021). Some species of Anaerolineae could establish syntrophic associations with the hydrogenotrophic methanogens and use carbohydrates to produce methane (Xia et al., 2016). The anerobic system of the EFD with 80°C hydrothermal treatment had the most Longilinea but produced the lowest methane, suggesting that Longilinea could not promote biogas yield via syntrophic associations with the hydrogenotrophic methanogens. Hydrolysis and acidogenesis bacteria (e.g., Aminicenantales and Sedimentibacter) was the most abundant in the 160°C hydrothermal treated group compared to other groups which promoted the methanogenesis process.
Principal coordinates analysis (PCA) was performed to investigate the evolution of bacterial communities in the EFD AD systems with different HT (Figure 5). The bacteria clustered into four groups after AD for 15 days. The bacteria in the hydrothermally treated EFD AD system were significantly different from those of the raw EFD, implying that HT largely changed the bacterial community composition. Moreover, the bacterial communities in the group with different hydrothermal temperatures were much different. 160 and 180°C treated samples were far separated from 80 to 120°C. This might be attributed to the less antibiotic residual in the high temperature–treated systems and presented less selective pressure (Li et al., 2015). With the AD time prolonging, the bacteria adapted to the environment gradually, and erythromycin was biodegraded, presenting less selection pressure. Thus, after AD for 30 days, the distances of different treated groups were close, which means that the bacterial community composition difference was shrinking. This phenomenon was in line with the previous research that antibiotics were the major driver for bacterial community evolution (Burman and Bengtsson-Palme, 2021).
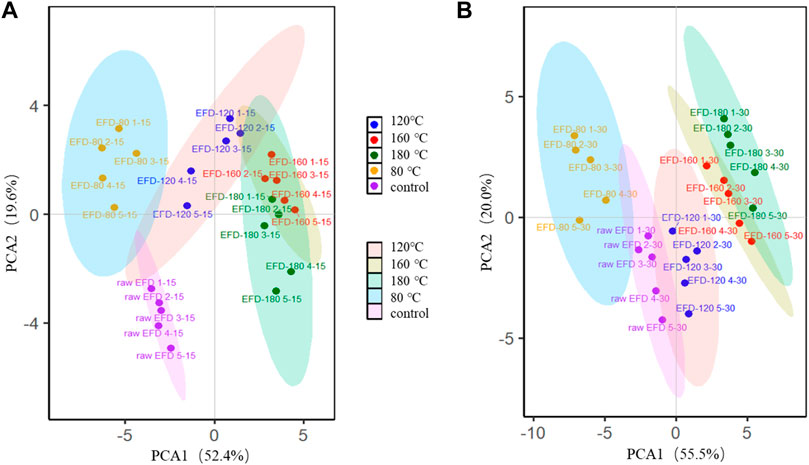
FIGURE 5. Principal component analysis (PCA) of bacterial genera during AD for 15 days (A) and 30 days (B) of the erythromycin fermentation dregs (EFD) after HT.
3.4.2 Archaeal Community Analysis
The Archaeal community was examined on the genus level to investigate the methanogenesis in the AD process of EFD with different HT (Figure 6). Candidatus_Methanofastidiosum and Methanosarcina played a dominant role in methane production. Candidatus_Methanofastidiosum, belonging to the sixth class of methanogens, could produce methane via methylated thiol reduction (Nobu et al., 2016). Their relative abundance in the raw EFD, 80, 120, and 160°C hydrothermally treated EFD was 24.87, 13.24, 22.55, 37.59, and 35.85%. The 160°C hydrothermally treated EFD AD system had the most Candidatus_Methanofastidiosum, indicating that methylated thiol reduction might be the major methane production route. Methanosarcina, the second abundant Archaea in all groups, could function as the mixotrophic (e.g., acetoclastic, hydrogenotrophic, and methylotrophic) methanogen (Wang et al., 2017). Their relative abundance in the 160 and 180°C treated EFD was 17.05 and 19.8%, respectively, which was higher than that in the low 80 and 120°C treated groups (11.03 and 16.07%). In addition, strictly hydrogenotrophic methanogens such as Methanoculleus also appeared in the EFD AD systems (Manzoor et al., 2016). But, their relative abundance decreased with the hydrothermally treated temperature increasing in the first 15 days of AD, which might be because of the limited substances. As the digested time extended to 30 days, Methanoculleus became the dominant methanogen, implying that the hydrogenotrophic methanogenesis pathway replaced other pathways in the late stage of AD. Based on the previous results, methylotrophic methanogenesis was dominant in the early phase of the EFD AD process, especially in the system with high temperature hydrothermal treatment, while with the digestion process, the hydrogenotrophic methanogenesis became the major route.
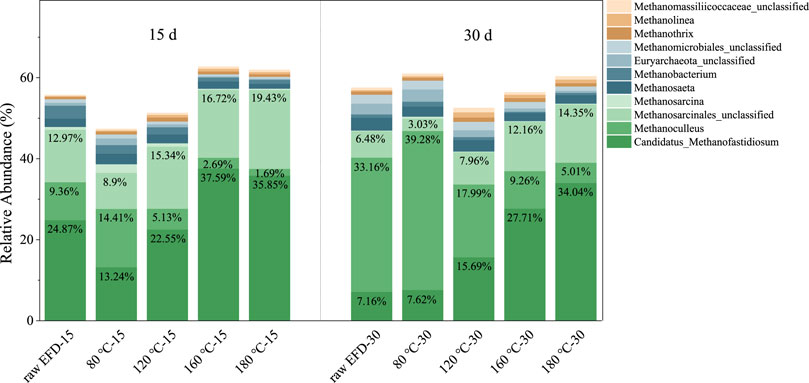
FIGURE 6. Changes of relative abundance of dominant Archaea genus during AD of the erythromycin fermentation dregs (EFD) with different hydrothermal treatment.
4 Conclusion
This study investigated the influence of HT on the AD performance of EFD at different temperatures. The results showed that erythromycin removal rates significantly increased with the hydrothermal temperature elevating, and under the optimal temperature of 160°C, more than 85% of erythromycin was eliminated. HT also led to the reduction of ARGs and MGEs in the EFD AD system. For example, the relative abundance of ermT and mefA decreased by one order of magnitude. Moreover, HT promoted methane production compared to the raw EFD. The relative abundance of hydrolysis and acidogenesis bacteria (e.g., Aminicenantales and Sedimentibacter) and methylotrophic methanogens (Candidatus_Methanofastidiosum and Methanosarcina) was enhanced after HT at 160°C, indicating that methylated substance reduction played a dominant role in methanogenesis.
Data Availability Statement
The original contributions presented in the study are included in the article/Supplementary Material, Further inquiries can be directed to the corresponding authors.
Author Contributions
YW: investigation, methodology, validation, data curation, visualization, and writing—original draft, SK: formal analysis, visualization, and writing—review and editing. MW: conceptualization, writing—review and editing, and funding acquisition.
Funding
This work was financially supported by the Natural Science Foundation of Shandong Province, China (Grant No. ZR2021QE100) and the Major Scientific and Technological Innovation Project of Shandong Province (Grant No. 2021CXGC011206).
Conflict of Interest
The authors declare that the research was conducted in the absence of any commercial or financial relationships that could be construed as a potential conflict of interest.
Publisher’s Note
All claims expressed in this article are solely those of the authors and do not necessarily represent those of their affiliated organizations, or those of the publisher, the editors, and the reviewers. Any product that may be evaluated in this article, or claim that may be made by its manufacturer, is not guaranteed or endorsed by the publisher.
References
Bai, Y., Xu, R., Wang, Q.-P., Zhang, Y.-R., and Yang, Z.-H. (2019). Sludge Anaerobic Digestion with High Concentrations of Tetracyclines and Sulfonamides: Dynamics of Microbial Communities and Change of Antibiotic Resistance Genes. Bioresour. Technol. 276, 51–59. doi:10.1016/j.biortech.2018.12.066
Burman, E., and Bengtsson-Palme, J. (2021). Microbial Community Interactions Are Sensitive to Small Changes in Temperature. Front. Microbiol. 12, 672910. doi:10.3389/fmicb.2021.672910
Cai, C., Hua, Y., Li, H., Li, L., Dai, L., Liu, H., et al. (2020). Hydrothermal Treatment of Erythromycin Fermentation Residue: Harmless Performance and Bioresource Properties. Resour. Conserv. Recycl. 161, 104952. doi:10.1016/j.resconrec.2020.104952
Cai, C., Li, L., Hua, Y., Liu, H., and Dai, X. (2021). Ferroferric Oxide Promotes Metabolism in Anaerolineae Other Than Microbial Syntrophy in Anaerobic Methanogenesis of Antibiotic Fermentation Residue. Sci. Total Environ. 758, 143601. doi:10.1016/j.scitotenv.2020.143601
Chen, S., Li, N., Dong, B., Zhao, W., Dai, L., and Dai, X. (2018). New Insights into the Enhanced Performance of High Solid Anaerobic Digestion with Dewatered Sludge by Thermal Hydrolysis: Organic Matter Degradation and Methanogenic Pathways. J. Hazard. Mater. 342, 1–9. doi:10.1016/j.jhazmat.2017.08.012
Chen, Z., Li, W., Qin, W., Sun, C., Wang, J., and Wen, X. (2020). Long-term Performance and Microbial Community Characteristics of Pilot-Scale Anaerobic Reactors for Thermal Hydrolyzed Sludge Digestion under Mesophilic and Thermophilic Conditions. Sci. Total Environ. 720, 137566. doi:10.1016/j.scitotenv.2020.137566
Chen, J., Liu, Y., Liu, K., Hu, L., Yang, J., Wang, X., et al. (2021). Bacterial Community Composition of Internal Circulation Reactor at Different Heights for Large-Scale Brewery Wastewater Treatment. Bioresour. Technol. 331, 125027. doi:10.1016/j.biortech.2021.125027
Classic Lowry, O., Rosebrough, N., Farr, A. L., and Randall, R. (1951). Protein Measurement with the Folin Phenol Reagent. J. Biol. Chem. 193, 265–275. doi:10.1016/s0021-9258(19)52451-6
David, J.-C., Buchet, A., Sialelli, J.-N., and Delouvée, S. (2021). The Use of Antibiotics in Veterinary Medicine: Representations of Antibiotics and Biosecurity by Pig Farmers. Prat. Psychol. 27, 159–170. doi:10.1016/j.prps.2020.06.003
Farag, I. F., Davis, J. P., Youssef, N. H., and Elshahed, M. S. (2014). Global Patterns of Abundance, Diversity and Community Structure of the Aminicenantes (Candidate Phylum OP8). PLoS One 9, e92139. doi:10.1371/journal.pone.0092139
Filippidou, S., Wunderlin, T., Junier, T., Jeanneret, N., Dorador, C., Molina, V., et al. (2016). A Combination of Extreme Environmental Conditions Favor the Prevalence of Endospore-Forming Firmicutes. Front. Microbiol. 7, 1707. doi:10.3389/fmicb.2016.01707
Gamble, K. C., Rose, D. T., and Sapozhnikov, J. (2022). Intravenous to Oral Antibiotics versus Intravenous Antibiotics: a Step-Up or a Step-Down for Extended Spectrum β-lactamase (ESBL)-producing Urinary Tract Infections without Concomitant Bacteraemia? Int. J. Antimicrob. Agents 59, 106541. doi:10.1016/j.ijantimicag.2022.106541
Gong, P., Liu, H., Wang, M., Dai, X., and Yao, J. (2020). Characteristics of Hydrothermal Treatment for the Disintegration of Oxytetracycline Fermentation Residue and Inactivation of Residual Antibiotics. Chem. Eng. J. 402, 126011. doi:10.1016/j.cej.2020.126011
Guo, H., Gu, J., Wang, X., Nasir, M., Yu, J., Lei, L., et al. (2020). Elucidating the Effect of Microbial Inoculum and Ferric Chloride as Additives on the Removal of Antibiotic Resistance Genes from Chicken Manure during Aerobic Composting. Bioresour. Technol. 309, 122802. doi:10.1016/j.biortech.2020.122802
Kemper, N. (2008). Veterinary Antibiotics in the Aquatic and Terrestrial Environment. Ecol. Indic. 8, 1–13. doi:10.1016/j.ecolind.2007.06.002
Li, Y., and Jin, Y. (2015). Effects of Thermal Pretreatment on Acidification Phase during Two-phase Batch Anaerobic Digestion of Kitchen Waste. Renew. Energy 77, 550–557. doi:10.1016/j.renene.2014.12.056
Li, C., Zhang, G., Zhang, Z., Ma, D., Wang, L., and Xu, G. (2015). Hydrothermal Pretreatment for Biogas Production from Anaerobic Digestion of Antibiotic Mycelial Residue. Chem. Eng. J. 279, 530–537. doi:10.1016/j.cej.2015.05.073
Li, Y., Gu, J., Wang, X., Song, Z., Hu, T., Xie, J., et al. (2022). The Fate of Antibiotic Resistance Genes and Their Influential Factors in Swine Manure Composting with Sepiolite as Additive. Bioresour. Technol. 347, 126727. doi:10.1016/j.biortech.2022.126727
Lu, Y., Li, J., Meng, J., Zhang, J., Zhuang, H., Zheng, G., et al. (2021). Long-term Biogas Slurry Application Increased Antibiotics Accumulation and Antibiotic Resistance Genes (ARGs) Spread in Agricultural Soils with Different Properties. Sci. Total Environ. 759, 143473. doi:10.1016/j.scitotenv.2020.143473
Manzoor, S., Schnürer, A., Bongcam-Rudloff, E., and Müller, B. (2016). Complete Genome Sequence of Methanoculleus Bourgensis Strain MAB1, the Syntrophic Partner of Mesophilic Acetate-Oxidising Bacteria (SAOB). Stand. Genomic Sci. 11, 80–89. doi:10.1186/s40793-016-0199-x
Mitchell, S. M., Ullman, J. L., Teel, A. L., Watts, R. J., and Frear, C. (2013). The Effects of the Antibiotics Ampicillin, Florfenicol, Sulfamethazine, and Tylosin on Biogas Production and Their Degradation Efficiency during Anaerobic Digestion. Bioresour. Technol. 149, 244–252. doi:10.1016/j.biortech.2013.09.048
Morar, M., Pengelly, K., Koteva, K., and Wright, G. D. (2012). Mechanism and Diversity of the Erythromycin Esterase Family of Enzymes. Biochemistry 51, 1740–1751. doi:10.1021/bi201790u
Ni, B.-J., Zeng, S., Wei, W., Dai, X., and Sun, J. (2020). Impact of Roxithromycin on Waste Activated Sludge Anaerobic Digestion: Methane Production, Carbon Transformation and Antibiotic Resistance Genes. Sci. Total Environ. 703, 134899. doi:10.1016/j.scitotenv.2019.134899
Nobu, M. K., Narihiro, T., Kuroda, K., Mei, R., and Liu, W.-T. (2016). Chasing the Elusive Euryarchaeota Class WSA2: Genomes Reveal a Uniquely Fastidious Methyl-Reducing Methanogen. ISME J. 10, 2478–2487. doi:10.1038/ismej.2016.33
Pang, H., He, J., Ma, Y., Pan, X., Zheng, Y., Yu, H., et al. (2021). Enhancing Volatile Fatty Acids Production from Waste Activated Sludge by a Novel Cation-Exchange Resin Assistant Strategy. J. Clean. Prod. 278, 123236. doi:10.1016/j.jclepro.2020.123236
Pang, H., Jiao, Q., He, J., Zhang, Z., Wang, L., Yan, Z., et al. (2022). Enhanced Short-Chain Fatty Acids Production through a Short-Term Anaerobic Fermentation of Waste Activated Sludge: Synergistic Pretreatment of Alkali and Alkaline Hydrolase Blend. J. Clean. Prod. 342, 130954. doi:10.1016/j.jclepro.2022.130954
Peng, H., Gu, J., Wang, X., Wang, Q., Sun, W., Hu, T., et al. (2020). Insight into the Fate of Antibiotic Resistance Genes and Bacterial Community in Co-composting Green Tea Residues with Swine Manure. J. Environ. Manag. 266, 110581. doi:10.1016/j.jenvman.2020.110581
Pilli, S., Yan, S., Tyagi, R. D., and Surampalli, R. Y. (2015). Thermal Pretreatment of Sewage Sludge to Enhance Anaerobic Digestion: a Review. Crit. Rev. Environ. Sci. Technol. 45, 669–702. doi:10.1080/10643389.2013.876527
Raunkjær, K., Hvitved-Jacobsen, T., and Nielsen, P. H. (1994). Measurement of Pools of Protein, Carbohydrate and Lipid in Domestic Wastewater. Water Res. 28, 251–262.
Regueiro, L., Carballa, M., and Lema, J. M. (2014). Outlining Microbial Community Dynamics during Temperature Drop and Subsequent Recovery Period in Anaerobic Co-Digestion Systems. J. Biotechnol. 192, 179–186. doi:10.1016/j.jbiotec.2014.10.007
Shen, Y., Zhuan, R., Chu, L., Xiang, X., Sun, H., and Wang, J. (2019). Inactivation of Antibiotic Resistance Genes in Antibiotic Fermentation Residues by Ionizing Radiation: Exploring the Development of Recycling Economy in Antibiotic Pharmaceutical Factory. Waste Manag. 84, 141–146. doi:10.1016/j.wasman.2018.11.039
Syafiuddin, A., and Boopathy, R. (2021). Role of Anaerobic Sludge Digestion in Handling Antibiotic Resistant Bacteria and Antibiotic Resistance Genes - A Review. Bioresour. Technol. 330, 124970. doi:10.1016/j.biortech.2021.124970
Tian, Z., Zhang, Y., and Yang, M. (2018). Chronic Impacts of Oxytetracycline on Mesophilic Anaerobic Digestion of Excess Sludge: Inhibition of Hydrolytic Acidification and Enrichment of Antibiotic Resistome. Environ. Pollut. 238, 1017–1026. doi:10.1016/j.envpol.2018.02.023
Toutian, V., Barjenbruch, M., Unger, T., Loderer, C., and Remy, C. (2020). Effect of Temperature on Biogas Yield Increase and Formation of Refractory COD during Thermal Hydrolysis of Waste Activated Sludge. Water Res. 171, 115383. doi:10.1016/j.watres.2019.115383
Vhangani, L. N., and Van Wyk, J. (2021). Heated Plant Extracts as Natural Inhibitors of Enzymatic Browning: A Case of the Maillard Reaction. J. Food Biochem. 45, e13611. doi:10.1111/jfbc.13611
Wang, M., Zhou, J., Yuan, Y.-X., Dai, Y.-M., Li, D., Li, Z.-D., et al. (2017). Methane Production Characteristics and Microbial Community Dynamics of Mono-Digestion and Co-digestion Using Corn Stalk and Pig Manure. Int. J. Hydrogen Energy 42, 4893–4901. doi:10.1016/j.ijhydene.2016.10.144
Wang, M., Zhang, B., Cai, C., Xin, Y., and Liu, H. (2018a). Acidic Hydrothermal Treatment: Characteristics of Organic, Nitrogen and Phosphorus Releasing and Process Optimization on Lincomycin Removal from Lincomycin Mycelial Residues. Chem. Eng. J. 336, 436–444. doi:10.1016/j.cej.2017.12.041
Wang, M., Zhang, B., Wang, J., Cai, C., Xin, Y., and Liu, H. (2018b). Degradation of Lincomycin in Aqueous Solution with Hydrothermal Treatment: Kinetics, Pathway, and Toxicity Evaluation. Chem. Eng. J. 343, 138–145. doi:10.1016/j.cej.2018.03.008
Wang, M., Ren, P., Wang, Y., Cai, C., Liu, H., and Dai, X. (2022). Erythromycin Stimulates rather Than Inhibits Methane Production in Anaerobic Digestion of Antibiotic Fermentation Dregs. Sci. Total Environ. 807, 151007. doi:10.1016/j.scitotenv.2021.151007
Xia, Y., Wang, Y., Wang, Y., Chin, F. Y., and Zhang, T. (2016). Cellular Adhesiveness and Cellulolytic Capacity in Anaerolineae Revealed by Omics-Based Genome Interpretation. Biotechnol. Biofuels 9, 111–113. doi:10.1186/s13068-016-0524-z
Xiao, R., Sun, X., Wang, J., Feng, J., Li, R., Zhang, Z., et al. (2015). Characteristics and Phytotoxicity Assay of Biochars Derived from a Zn-Rich Antibiotic Residue. J. Anal. Appl. Pyrolysis 113, 575–583. doi:10.1016/j.jaap.2015.04.006
Yang, W., Shang, J., Sharma, P., Li, B., Liu, K., and Flury, M. (2019). Colloidal Stability and Aggregation Kinetics of Biochar Colloids: Effects of Pyrolysis Temperature, Cation Type, and Humic Acid Concentrations. Sci. Total Environ. 658, 1306–1315. doi:10.1016/j.scitotenv.2018.12.269
Zarei-Baygi, A., and Smith, A. L. (2021). Intracellular versus Extracellular Antibiotic Resistance Genes in the Environment: Prevalence, Horizontal Transfer, and Mitigation Strategies. Bioresour. Technol. 319, 124181. doi:10.1016/j.biortech.2020.124181
Zeng, S., Sun, J., Chen, Z., Xu, Q., Wei, W., Wang, D., et al. (2021). The Impact and Fate of Clarithromycin in Anaerobic Digestion of Waste Activated Sludge for Biogas Production. Environ. Res. 195, 110792. doi:10.1016/j.envres.2021.110792
Zhang, G., Ma, D., Peng, C., Liu, X., and Xu, G. (2014). Process Characteristics of Hydrothermal Treatment of Antibiotic Residue for Solid Biofuel. Chem. Eng. J. 252, 230–238. doi:10.1016/j.cej.2014.04.092
Zhang, G., Li, C., Ma, D., Zhang, Z., and Xu, G. (2015a). Anaerobic Digestion of Antibiotic Residue in Combination with Hydrothermal Pretreatment for Biogas. Bioresour. Technol. 192, 257–265. doi:10.1016/j.biortech.2015.05.014
Zhang, T., Yang, Y., and Pruden, A. (2015b). Effect of Temperature on Removal of Antibiotic Resistance Genes by Anaerobic Digestion of Activated Sludge Revealed by Metagenomic Approach. Appl. Microbiol. Biotechnol. 99, 7771–7779. doi:10.1007/s00253-015-6688-9
Zhang, Y., Liu, H., Xin, Y., Shen, Y., Wang, J., Cai, C., et al. (2019). Erythromycin Degradation and ERY-Resistant Gene Inactivation in Erythromycin Mycelial Dreg by Heat-Activated Persulfate Oxidation. Chem. Eng. J. 358, 1446–1453. doi:10.1016/j.cej.2018.10.157
Zhu, H., Qin, L., Hu, Y., Wei, D., Hai, Z., Li, A., et al. (2017). Occurrence and Transformations of Carbon, Nitrogen, and Phosphorus Related to Particle Size Fraction of Sweet Potato Starch Wastewater during Hydrolytic Acidification Processes. Environ. Sci. Pollut. Res. 24, 20717–20724. doi:10.1007/s11356-017-9724-8
Keywords: erythromycin fermentation dregs, antibiotic resistance genes, anerobic digestion, methane production, microbial community
Citation: Wang Y, Kuang S and Wang M (2022) Effect of Hydrothermal Pretreatment on Anaerobic Digestion of Erythromycin Fermentation Dregs: Biogas Production, Antibiotic Resistance Gene Evolution, and Microbial Community Dynamics. Front. Environ. Sci. 10:905494. doi: 10.3389/fenvs.2022.905494
Received: 27 March 2022; Accepted: 19 April 2022;
Published: 31 May 2022.
Edited by:
Xiaodong Xin, Dongguan University of Technology, ChinaReviewed by:
Shuli Liu, North China University of Water Resources and Electric Power, ChinaZhao Yuxiao, Shandong Academy of Sciences, China
Copyright © 2022 Wang, Kuang and Wang. This is an open-access article distributed under the terms of the Creative Commons Attribution License (CC BY). The use, distribution or reproduction in other forums is permitted, provided the original author(s) and the copyright owner(s) are credited and that the original publication in this journal is cited, in accordance with accepted academic practice. No use, distribution or reproduction is permitted which does not comply with these terms.
*Correspondence: Mengmeng Wang, wangmm@qust.edu.cn; Shaoping Kuang, kuangshaoping@126.com