Exploring the Fundamental Factors Behind Algal-Bacterial Symbiosis and Their Impact on Ecological Interactions
- 1Tianjin Key Laboratory of Clean Energy and Pollution Control, School of Energy and Environmental Engineering, Hebei University of Technology, Tianjin, China
- 2Institute of Ecological and Environmental Sciences, Sichuan Agricultural University, Chengdu, China
- 3Hebei Key Laboratory of Close-to-Nature Restoration Technology of Wetlands, School of Eco-Environment, Hebei University, Baoding, China
This study focused on important factors including light intensity, light/dark cycles, and the biomass of algae/bacteria to explore the relationship between algae and bacteria, aiming to obtain the optimal performance in the algae–sludge membrane bioreactor (AS-MBR). It was found that 3000 lux was considered to be the appropriate light intensity that could improve algal biomass and nitrogen removal among the chosen light intensities. Further analysis of results indicated that a higher or lower light intensity could not simultaneously promote algal energy absorption and nitrifying bacterial activity. Moreover, the highest average growth rate of algae (0.16 mg/L d−1) and the removal efficiency of NH4+-N (96.4 ± 1.5%) were both observed during the 12-h light/12-h dark cycle, respectively. Meantime, the appropriate algal proportion would mitigate membrane fouling compared with the conventional MBR. The investigation of the mechanism suggested that light intensity, light–dark cycle, and algal proportion were significantly associated with algal photosynthesis (key proteins, chromophores, and nucleic acids), the characteristics of functional bacteria, and the underlying cognition of cell-to-cell signaling between algae and bacteria, which would further influence the reactor performance.
1 Introduction
Algal–bacterial biomass has been preferred over conventional activated sludge for treating wastewater due to its excellent N/P removal efficiencies, high-potential bio-product production, and low intensity or no aeration (Godos et al., 2009; Zhen et al., 2016). These characteristics tend to facilitate the algal–bacterial technologies for wastewater treatment from urban and industrial activities, and livestock. However, poor settleability as a result of small algal cells would cause the biomass washout inartificially, leading to the system failure and impairment of effluent quality, which is the main obstructive factor for the widespread application of the algal–bacterial technology. Therefore, an effective and sustainable strategy for keeping the algal–bacterial biomass stale is highly desirable.
Although membrane filtration has already become a better choice for algal–bacterial biomass washout because of its smaller membrane pore than the algae cell (Bilad et al., 2014), a few studies have proposed an integrated system of algal–bacterial technique and membrane bioreactor (MBR) and obtained excellent wastewater treatment and membrane fouling mitigation (Xu et al., 2014; Sun et al., 2018). The relationship between algae and bacteria was closely associated with the activity of algae and bacteria responsible for good performance. In contrast to the achievement in searching for suitable pollutants and operation conditions in an algae–bacteria system, few studies focused on understanding the underlying mechanism related to the interaction of algae and bacteria. Especially, several factors are worthy of special attention, such as the illumination intensity, light/dark cycles, and algae/bacteria ratios, which are considered as the most important factors for the formation and interaction of algal–bacterial symbiosis (Sforza et al., 2012; Ye et al., 2018). Light is an important factor for the growth of algae because of its ability to absorb carbon dioxide (Agrawal, 2012). Various investigators observed that high light intensity may promote algal growth and pigment biosynthesis, which would influence the algal growth, biomass accumulation, and biodiesel production (Muller et al., 1998), while excess light absorption might cause the accumulation of TAGs (Singh and Singh, 2015). With regard to the important factors and further influence on the algal–bacterial consortia, the additional insights into manipulating the interactions between microalgae and bacteria might provide exciting tools to improve the environmental sustainability and economic feasibility of the algal–bacterial system. However, knowledge of the interaction mechanisms between the factors and the algal–bacterial symbiosis is still lacking in previous studies and real practice.
In this study, for the first time, in a novel algal–sludge MBR (AS-MBR) system, we aimed was (1) to determine the effect of illumination intensity, light/dark cycles, and the ratio (algae/bacteria) on the formation and performance of algal–bacterial symbiosis and (2) to explore the interaction mechanism between the factors and algal–bacterial consortia.
2 Materials and Methods
2.1 AS-MBR
An AS-MBR combining algae and sludge membrane bioreactors was designed to evaluate the impact of the algae on the membrane fouling and water treatment performance. Details of the fabrication and operation of the AS-MBR are described in the Supporting information (SI).
2.2 Microbial Activity Analysis
The microbial relative activity was represented by the specific oxygen uptake rate (SOUR) (Huang et al., 2015). Detailed methods of the SOUR are listed in the SI.
2.3 Extraction and Analysis of Extracellular Polymeric Substances and Chlorophyll-a
2.3.1 EPS
EPSs were considered as the total of soluble EPS (S-EPS) and bound EPS (B-EPS). The B-EPS includes the loosely bound EPS (LB-EPS) and tightly bound EPS (TB-EPS). The EPSs were extracted following the three-step heat treatment as documented in Hwang et al. (2010).
2.3.2 Chlorophyll-a
The samples were centrifuged and resuspended by 90% acetone with little CaCO3. Then, the mixture was vortex stirred and kept in a refrigerator at 4°C for 24 h under dark conditions. After that, the mixture was centrifuged, and the optical density (OD) value of the supernatant was measured at 750, 663, 645, and 630 nm using UV/V (UV-2550, Shimadzu, Japan) (Lee et al., 2015).
2.4 Water Quality Analysis
The total organic carbon (TOC) and the total nitrogen (TN) were determined via a TOC/TN analyzer (TOC-5000, Shimadzu, Japan). COD, NH4+-N, and PO43--P were measured in accordance with the APHA Standard Methods (2005). The analysis was conducted in triplicate, and their average values were reported.
3 Results and Discussion
3.1 Effect of Illumination Intensity on Algal Growth and Nitrification
Light intensity is closely associated with algal biomass and nitrate concentration because of its necessity for algal growth and the inhibition of the bacterial nitrification (Merbt et al., 2012). Chlorophyll-a concentrations under different light intensities are shown in Figure 1. Under the light intensities of 3000 and 4000 lux, the chlorophyll-a concentration increased at average rates of 0.10/mg/L d−1 and 0.14/mg/L d−1, respectively, along with the operation time. However, the increased rate of the chlorophyll-a concentration started to decrease from the third day under a light intensity of 2000 lux. (Because of inconstant light intensity under natural conditions, an artificial light source was used in the experiment.) According to He et al. (2015), light intensity could promote the accumulation of carbon partition among proteins, carbohydrates, and lipids, stimulating algal biomass production. Hence, the algal average growth rate is positively related to light intensity in a certain level.
From Table 1, the biological activity of ammonia-oxidizing bacteria (AOB) under 2000 lux was 22.9 and 19.12% higher than those under 3000 and 4000 lux, while with regard to nitrite oxidizing bacteria (NOB), the biological activity increased by 8.16 and 10.42% in comparison with those under 3000 and 4000 lux. It indicated that nitrifying bacteria were inhibited under light intensities of 3000 and 4000 lux. Accordingly, the phenomenon might be logical to cause an increase in NH4+-N concentration under the light intensities of 3000 and 4000 lux. However, NH4+-N concentrations in the effluent under both 3000 and 4000 lux were lower than those under 2000 lux, which might be attributed to the higher nitrogen removal activities of the algae under 3000 and 4000 lux. In addition, weak light intensity can induce low nutrient uptake and insufficient O2 supply of algae in the integrated system. Extreme light exposure led to the failure of the process due to the sensitiveness of the nitrifying bacteria. Therefore, a higher light intensity is beneficial for algal growth, while implies much more inhibition for nitrification. Thus, in this study, considering the energy consumption for lighting and the removal efficiency of ammonia nitrogen, 3000 lux was regarded as the appropriate light intensity.
3.2 Effect of Light and Dark Cycles on Algal Biomass and Wastewater Treatment
In this study, 8-h light/16-h dark (8 hL/16 hD), 12 hL/12 hD, and 16 hL/8 hD cycles were investigated to explore the effect of the light/dark cycle on the algal biomass and nutrient removal. From Table 2, average algal growth rates under both 12 hL/12 hD and 16 hL/8 hD cycles were higher than those under the 8 hL/16 hD cycle, indicating that algal growth could be promoted under long durations of light illumination time. However, algae exhibited the highest growth rate under the 12 hL/12 hD cycle, rather than the 16 hL/8 hD cycle. It indicated that the light illumination time could affect algal light and dark reactions and further improve or inhibit the algal growth under the different light/dark cycles.
As for the influence of the light/dark cycle on the nutrient removal (Table 2), the COD removal efficiency had little change among the three light/dark cycles. First, algae could assimilate polysaccharides through the diffusion dynamic mode, thus facilitating the uptake of external polysaccharides due to the enhancement of algae respiration under long dark conditions. Second, dissolved oxygen (DO) concentration under long illumination time was higher than that under the short light conditions, emphasizing the importance of DO concentration for bacterial respiration and polysaccharide mineralization. In consequence, with regard to light/dark cycles, the polysaccharide assimilation by algae under long dark conditions was more advantageous, while the mineralization of polysaccharides by bacteria under long light illumination time was higher, which resulted in the slight variation of COD removal efficiency among the three light/dark cycles. The removal efficiency of NH4+-N under 8 hL/16 hD, 12 hL/12 hD, and 16 hL/8 hD cycles was 91.3 ± 1.1%, 96.4 ± 1.5%, and 93.7 ± 1.4%, respectively. The variation trend of NH4+-N removal efficiency was similar to the growth of algae, where the 12 hL/12 hD cycle had the highest and the 8 hL/16 hD cycle had the lowest removal efficiency. It suggested that an appropriate light/dark cycle could enhance algae photosynthesis and promote NH4+-N assimilation. Moreover, the increase in DO concentration caused by the algae photosynthesis improved nitrification. Hence, NH4+-N removal efficiency and algal growth exhibited a similar variation trend. In addition, long duration of illumination might inhibit the activities of ammonium-oxidizing and nitrite-oxidizing bacteria, inducing the high NH4+-N concentration in the 16 hL/8 hD cycle compared with the 12 hL/12 hD cycle, which is consistent with the observation of Vergara’s study (2016). In summary, proper light illumination time is critical to the algae growth and nutrient removal, influencing the stable operation of the algae–bacteria synergistic system.
3.3 Effect of Algae/Sludge on Membrane Fouling and Microbial Metabolites
Previous studies on the effect of algae on the membrane fouling are contradictory. Some research studies demonstrate that algae have a positive influence on the membrane filtration (Xu et la., 2014; Huang et al., 2015), while others support its potential to cause dramatic membrane fouling (Qu et al., 2015; Matsumoto et al., 2014), in which the EPS was considered as the main principal contradiction. Thus, the algal biomass in the algal–bacterial symbiotic was investigated as one of the most important factors for membrane fouling in this coupled system AS-MBR. Figure 2 shows that the reactor with the ratio (algae/bacteria) of 1:5 exhibited the lowest average increasing rate of transmembrane pressure (dTMP/dt), while the reactor with a ratio of 1:1 exhibited the highest rate. Hence, compared with the control reactor (without algae), the reactor with different algal proportions exhibited membrane fouling aggravation or mitigation, and the influence of algae on the membrane permeability cannot be definitively determined, which depends on the relative content of algae and bacteria in the symbiotic system. Membrane fouling in the reactors with algae/bacteria ratios of 1:10, 1:5, and 1:1 and without algae all exhibited a distinct three-stage process. Generally, stage 1 (0-3d) consisted of a higher initial membrane fouling rate; stage 2 (intermediate stage) reflected a long-term rise of TMP with a lower membrane fouling rate; stage 3 occurred with the highest membrane fouling rate that is referred to as the TMP jump. Selecting the last membrane fouling cycle, the dTMP/dt of stages 2 and 3 in AS-MBR with a ratio of 1:5 were 0.5 and 2.83 kPa/d. Previous studies demonstrated that the membrane pore blockage and the dominant foulant were the major factors for the slow fouling stage (stage 2) and the rapid fouling stage (stage 3) (Cho and Fane, 2002). Thus, lower dTMP/dt in AS-MBR with a ratio of 1:5 indicated that the reduction of membrane pore blocking and/or the decrease in floc accumulation on the membrane surface may occur, contributing to the alleviation of membrane fouling.
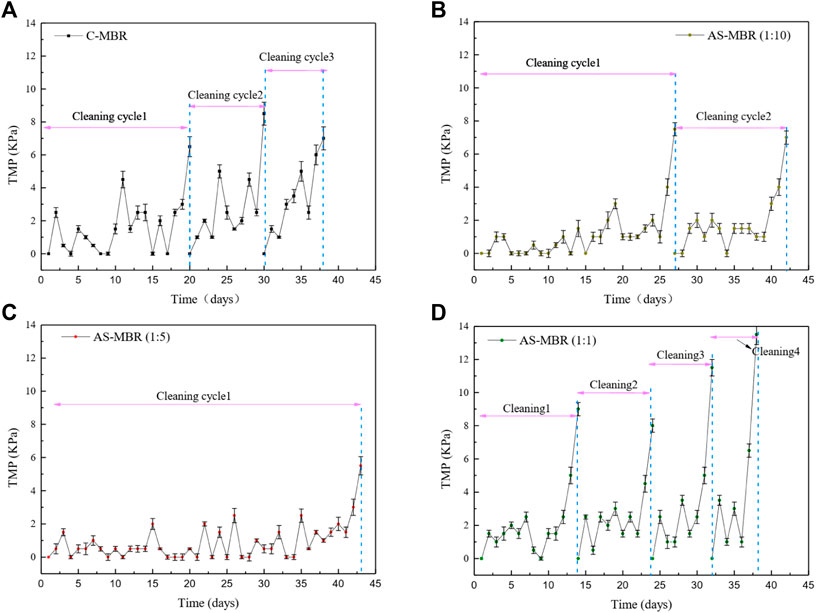
FIGURE 2. Profiles of dTMP/dT in the four reactors with different algae/bacteria inoculation ratios: (A) system without algae, (B) 1:10 ratio of algae and bacteria, (C) 1:5 ratio of algae and bacteria, and (D) 1:1 ratio of algae and bacteria.
In summary, different inoculation ratios (algae/activated sludge) have a significant influence on membrane fouling, and an inoculation ratio of 1:5 was conducive to membrane permeability.
3.4 Mechanism and Practical Implications
Light intensity, light–dark cycles, and algae/bacteria ratios were important factors for AS-MBR, and a greater insight into the consortia mechanism of algae and bacteria at the molecular level may promote its broad-spectrum applicability (Figure 3).
The algal oxygenic photosynthesis process can be divided into light and dark phases. Algal chlorophyll can absorb the photons and drive linear and cyclic electron transfer to form adenosine triphosphate (ATP), which powers the Calvin–Benson cycle to produce carbohydrates in the dark phase. As one of the most important functional units, photosystem II (PSII) is responsible for absorbing sunlight and utilizing excitation energy to oxidize water molecules into protons and molecular oxygen (Vecchi et al., 2020). Obviously, in the process of algal photosynthesis, light is the energy source received by algae and the pigment-binding proteins. Thus, light intensity could affect the algal energy absorption and the activity of key protein enzymes, thus influencing the algal light reaction (He et al., 2015). Lamare et al. (2019) demonstrated that high light intensity implies much more inhibition for photosystem PSII than low light intensity. However, extremely low light intensity may diminish the light energy absorption of algal chlorophyll. Therefore, proper light intensities should be considered for the algal energy requirement and activities of the key proteins of PSII process. On the other hand, light intensity has important influence on algae growth and activities of AOB and NOB. First, the enzyme ammonium oxygenase and its associated porphyrin cofactors would cause photo-oxidative damage during high light intensities (Vergara et al., 2016). Second, high light intensities can affect the synthesis of polypeptides and cytochrome-c, which are critical for the ammonia monooxygenase system and nitrifying process (Hyman and Arp, 1992; Guerrero and Jones, 1997). Finally, a previous study hypothesized that a prolonged exposure to high light intensities could also induce significant damage on key proteins, chromophores, and nucleic acids (Guerrero and Jones, 1997). Consequently, light tendency has contradictory effects on the growth of algae and the activity of nitrifying bacteria, which are closely associated with the interactions of algae and bacteria. Unfortunately, the current knowledge lacks convincing data of actual application for reconciling the response of algae and nitrifying bacteria to light.
The optimal alternation of the light/dark cycle, which, similar to the circulation cycle of anaerobic/aerobic switches in the sequencing batch reactor (SBR), is also beneficial to the efficient use of light energy by algae (Sforza et al., 2012). As discussed in Section 3.2, if the light/dark cycle was not appropriate, algae might undergo radiation damage, thus hindering the photosynthesis process. The light and dark phases were in charge of the conversion of light energy to chemical energy. In the dark phase, algae utilized NADPH/ATP from the light phase to convert the CO2 that is mostly produced by bacterial respiration, into sugar via the Calvin–Benson–Bassham cycle. Obviously, when cells are exposed to high-intensity illumination, PSII may be continually damaged and must be repaired by re-synthesis of damaged components. Li et al. (2009) reported that harmful reactive oxygen species (ROS) would be produced and induce oxidative stress under excess light. Accordingly, the damage of photosystems should be repaired and minimized. If the illumination time is short, most photons are utilized for photosynthesis, rather than for ROS formation. Conversely, longer exposure may lead to the generation of ROS and damage, and the abrupt changes in illumination are as harmful to the photosynthetic apparatus as constant high light. Thus, neither extremely long nor extremely short illumination time was beneficial for algal growth and nutrient removal under the same light intensity. Therefore, the light/dark cycle has a critical effect on the growth and the activity of algae, which influence the relationship between algae and bacteria, further resulting in the variation in nutrient removal efficiency.
In the algae–bacteria system, bacteria and algal cells would attach to each other and form a micro-environment with higher nutrient concentrations than those in the surrounding water. Moreover, algae provide an appropriate habitat for the colonization of bacterial communities on their surfaces and secrete organic substances as nutrients for bacterial proliferation, and the associated bacteria provide effective molecules (such as bioactive compounds and QS signaling molecules) as feedback, which are beneficial for morphogenesis, development, and host growth. However, in the competitive interaction of algae and bacteria, some algal metabolites present a bactericidal effect, and some bacteria can secrete toxic substances and affect the algal photosynthesis-related gene transcription. Accordingly, the microbial community was considered to play one of the most important roles in the relationship between algae and bacteria. Therefore, an appropriate ratio of algae and bacteria may have a selective power for particular membranes of bacterial communities, resulting in the formation of more factorable conditions. These functions include ability to degrade complex nutrients and stave off competition and provide benefits to each other. It requires metabolic activity linked to a complex signaling network to maintain the relationship, backed by fluid genetic machinery.
Finally, interactions between algae and bacteria are of potential utilization for the wastewater treatment and membrane fouling control. In addition, light intensity, light/dark cycle, and algae/bacteria ratio were considered to be closely associated with algal–bacterial relationship and the performance of AS-MBR. Unfortunately, the current knowledge lacks convincing data for the underlying cognition of cell-to-cell signaling between algae and bacteria. Consequently, more molecular biology research studies (such as QS signals) on in-depth analysis of the environment factors for algal–bacterial interaction mechanisms are necessary in the future in order to improve the performance of AS-MBR.
4 Conclusion
The influence of light and the proportion of algae on the algal–bacterial consortia were studied. The light intensity of 4000 lux exhibited the highest algal average growth rate of 0.14/mg/L d−1, and the highest NH4+-N removal efficiencies of 96.4% was achieved under 3000 lux light intensity. Moreover, AS-MBR achieved the highest algal growth rate and COD and NH4+-N removal efficiencies under 12 hL/12 hD cycle. The membrane fouling was mitigated with appropriate algae inoculation, which attributed to the reduction of membrane pore blocking and/or the decrease of floc accumulation on the membrane surface. Consequently, it is crucial to understand how the factors influence the algal and bacterial cells and further to create an optimal photo-biological formula for each algal–bacterial consortia.
Data Availability Statement
The original contributions presented in the study are included in the article/Supplementary Materials, further inquiries can be directed to the corresponding author.
Author Contributions
LS: conceptualization, methodology, experimental design, and writing—original draft. HL: supervision, writing—review and editing, and funding acquisition. YL: investigation, visualization, and data curation.
Funding
This study was supported by the National Natural Science Foundation of China (52100031), the Guangdong Basic and Applied Basic Research Foundation (2020A1515110309), and the Natural Science Foundation of Hebei Province (E2019201293).
Conflict of Interest
The authors declare that the research was conducted in the absence of any commercial or financial relationships that could be construed as a potential conflict of interest.
Publisher’s Note
All claims expressed in this article are solely those of the authors and do not necessarily represent those of their affiliated organizations, or those of the publisher, the editors, and the reviewers. Any product that may be evaluated in this article, or claim that may be made by its manufacturer, is not guaranteed or endorsed by the publisher.
References
Agrawal, S. C. (2012). Factors Controlling Induction of Reproduction in Algae-Review: the Text. Folia Microbiol. 57, 387–407. doi:10.1007/s12223-012-0147-0
APHA Standard Methods (2005). Standard Methods for the Examination of Water and Wastewater. Washington, DC: Am. Pub. Health Assoc..
Bilad, M. R., Arafat, H. A., and Vankelecom, I. F. J. (2014). Membrane Technology in Microalgae Cultivation and Harvesting: A Review. Biotechnol. Adv. 32 (7), 1283–1300. doi:10.1016/j.biotechadv.2014.07.008
Cho, B. D., and Fane, A. G. (2002). Fouling Transients in Nominally Sub-critical Flux Operation of a Membrane Bioreactor. J. Membr. Sci. 209, 391–403. doi:10.1016/s0376-7388(02)00321-6
Godos, I. d., Blanco, S., García-Encina, P. A., Becares, E., and Muñoz, R. (2009). Long-term Operation of High Rate Algal Ponds for the Bioremediation of Piggery Wastewaters at High Loading Rates. Bioresour. Tech. 100, 4332–4339. doi:10.1016/j.biortech.2009.04.016
Guerrero, M., and Jones, R. (1997). Light-induced Absorbance Changes Associated with Photoinhibition and Pigments in Nitrifying Bacteria. Aquat. Microb. Ecol. 13, 233–239. doi:10.3354/ame013233
He, Q., Yang, H., Wu, L., and Hu, C. (2015). Effect of Light Intensity on Physiological Changes, Carbon Allocation and Neutral Lipid Accumulation in Oleaginous Microalgae. Bioresour. Tech. 191, 219–228. doi:10.1016/j.biortech.2015.05.021
Huang, W., Li, B., Zhang, C., Zhang, Z., Lei, Z., Lu, B., et al. (2015). Effect of Algae Growth on Aerobic Granulation and Nutrients Removal from Synthetic Wastewater by Using Sequencing Batch Reactors. Bioresour. Tech. 179, 187–192. doi:10.1016/j.biortech.2014.12.024
Hwang, B.-K., Kim, J.-H., Ahn, C. H., Lee, C.-H., Song, J.-Y., and Ra, Y.-H. (2010). Effect of Disintegrated Sludge Recycling on Membrane Permeability in a Membrane Bioreactor Combined with a Turbulent Jet Flow Ozone Contactor. Water Res. 44, 1833–1840. doi:10.1016/j.watres.2009.12.009
Hyman, M. R., and Arp, D. J. (1992). 14C2H2- and 14CO2-labeling Studies of the De Novo Synthesis of Polypeptides by Nitrosomonas Europaea during Recovery from Acetylene and Light Inactivation of Ammonia Monooxygenase. J. Biol. Chem. 267 (3), 1534–1545. doi:10.1016/s0021-9258(18)45979-0
Lamare, P.-O., Aguillon, N., Sainte-Marie, J., Grenier, J., Bonnefond, H., and Bernard, O. (2019). Gradient-based Optimization of a Rotating Algal Biofilm Process. Automatica 105, 80–88. doi:10.1016/j.automatica.2019.02.043
Lee, C. S., Lee, S.-A., Ko, S.-R., Oh, H.-M., and Ahn, C.-Y. (2015). Effects of Photoperiod on Nutrient Removal, Biomass Production, and Algal-Bacterial Population Dynamics in Lab-Scale Photobioreactors Treating Municipal Wastewater. Water Res. 68, 680–691. doi:10.1016/j.watres.2014.10.029
Matsumoto, T., Yamamura, H., Hayakawa, J., Watanabe, Y., and Harayama, S. (2014). Influence of Extracellular Polysaccharides (EPS) Produced by Two Different green Unicellular Algae on Membrane Filtration in an Algae-Based Biofuel Production Process. Water Sci. Technol. 69, 1919–1925. doi:10.2166/wst.2014.104
Merbt, S. N., Stahl, D. A., Casamayor, E. O., Martí, E., Nicol, G. W., and Prosser, J. I. (2012). Differential Photoinhibition of Bacterial and Archaeal Ammonia Oxidation. Fems Microbiol. Lett. 327 (1), 41–46. doi:10.1111/j.1574-6968.2011.02457.x
Muller-Feuga, A., Le Guédes, R., Hervé, A., and Durand, P. (1998). Comparison of Artificial Light Photobioreactors and Other Production Systems Using Porphyridium Cruentum. J. Appl. Phycol 10, 83–90. doi:10.1023/a:1008046814640
Qu, F., Du, X., Liu, B., He, J., Ren, N., Li, G., et al. (2015). Control of Ultrafiltration Membrane Fouling Caused by Microcystis Cells with Permanganate Preoxidation: Significance of In Situ Formed Manganese Dioxide. Chem. Eng. J. 279, 56–65. doi:10.1016/j.cej.2015.05.009
Sforza, E., Simionato, D., Giacometti, G. M., Bertucco, A., and Morosinotto, T. (2012). Adjusted Light and Dark Cycles Can Optimize Photosynthetic Efficiency in Algae Growing in Photobioreactors. PLoS One 7 (6), e38975. doi:10.1371/journal.pone.0038975
Singh, S. P., and Singh, P. (2015). Effect of Temperature and Light on the Growth of Algae Species: A Review. Renew. Sust. Energ. Rev. 50, 431–444. doi:10.1016/j.rser.2015.05.024
Sun, L., Tian, Y., Zhang, J., Cui, H., Zuo, W., and Li, J. (2018). A Novel Symbiotic System Combining Algae and Sludge Membrane Bioreactor Technology for Wastewater Treatment and Membrane Fouling Mitigation: Performance and Mechanism. Chem. Eng. J. 344, 246–253. doi:10.1016/j.cej.2018.03.090
Vecchi, V., Barera, S., Bassi, R., and Dall’Osto, L. (2020). Potential and Challenges of Improving Photosynthesis in Algae. Plants 9 (1), 67. doi:10.3390/plants9010067
Vergara, C., Muñoz, R., Campos, J. L., Seeger, M., and Jeison, D. (2016). Influence of Light Intensity on Bacterial Nitrifying Activity in Algal-Bacterial Photobioreactors and its Implications for Microalgae-Based Wastewater Treatment. Int. Biodeterioration Biodegradation 114, 116–121. doi:10.1016/j.ibiod.2016.06.006
Xu, M., Bernards, M., and Hu, Z. (2014). Algae-facilitated Chemical Phosphorus Removal during High-Density Chlorella Emersonii Cultivation in a Membrane Bioreactor. Bioresour. Tech. 153, 383–387. doi:10.1016/j.biortech.2013.12.026
Ye, J., Liang, J., Wang, L., Markou, G., and Jia, Q. (2018). Operation Optimization of a Photo-Sequencing Batch Reactor for Wastewater Treatment: Study on Influencing Factors and Impact on Symbiotic Microbial Ecology. Bioresour. Tech. 252, 7–13. doi:10.1016/j.biortech.2017.12.086
Keywords: algal–sludge membrane bioreactor, fundamental factors, algal–bacterial symbiosis, microbial ecology, wastewater treatment
Citation: Sun L, Lei Y and Li H (2022) Exploring the Fundamental Factors Behind Algal-Bacterial Symbiosis and Their Impact on Ecological Interactions. Front. Environ. Sci. 10:893412. doi: 10.3389/fenvs.2022.893412
Received: 10 March 2022; Accepted: 31 March 2022;
Published: 14 June 2022.
Edited by:
Xiaodong Xin, Dongguan University of Technology, ChinaReviewed by:
Lingwe Meng, Northeast Electric Power University, ChinaFang Zhang, Fujian Agriculture and Forestry University, China
Copyright © 2022 Sun, Lei and Li. This is an open-access article distributed under the terms of the Creative Commons Attribution License (CC BY). The use, distribution or reproduction in other forums is permitted, provided the original author(s) and the copyright owner(s) are credited and that the original publication in this journal is cited, in accordance with accepted academic practice. No use, distribution or reproduction is permitted which does not comply with these terms.
*Correspondence: Hui Li, lihui2006.cool@163.com