Formation of Solonchak in the Area of the Discharged Ancient Brine Wells (Perm Krai, Russia)
- 1Institute of Natural Science, Perm State National Research University, Perm, Russia
- 2Chemical Engineering Faculty, Perm National Research Polytechnic University, Perm, Russia
The change in soil-forming processes and, as a result, in the ecosystem due to nearly 300-year long outflow of highly saline waters from ancient brine wells is considered for Perm Krai in Russia for the first time. The study area is located in the high floodplain of the Usolka River valley. Sodium-chloride ancient brine wells with mineralization of about 30 g/L flow in streams over the soil surface and enter the Usolka River. The paper considers three soil types at various distances from a saline stream. The main pedogenic processes are the sod-forming process and gleying in alluvial soils. Soils in Gleyic Fluvisols (background)—Gleyic Fluvisols (Sulfatic) (transition)—Solonchak (affected by sodium-chloride brines) series were studied in terms of occurring soil-forming processes. All studied soils are formed under hydromorphic conditions. It is evidenced by gleization. However, the morphological and physicochemical properties of the soils differ. The presence of water-soluble ions in the solonchak suggests that salinization is occurring. The sum of toxic salts was 0.94% which was several times higher than in the transition and background soils. The sodium adsorption ratio (SAR) ranged from 10.4 to 21.6, with a рН of 8.3–9.1. The saline soils were more alkaline (pH average 8.8 and 9.3) than the background soil (pH 8.1). SAR decreased significantly in the Solonchak—transition—background soil series from 16.9 to 0.7. The aforementioned observations, as well as physical properties of the saline soil, clearly show the entry of sodium into the solonchak adsorption complex, indicating the solonization process. The study area is characterized by the presence of salt-tolerant species of herbaceous plants and an obligate halophyte. Salicornia perennans Willd, a halophyte, was discovered in the wells’ discharge area at a distance of 0.2–1.5 m from saline streams. The analysis of the long-term impact of highly mineralized waters on the natural environment will aid in revealing and forecasting ecosystem changes caused by potash mining in Perm Krai for environmental risk assessment for new and existing potash enterprises.
Introduction
Soil salinization of alluvial valleys with groundwater is a widespread process (Kovda, 1937; Pankova and Yamnova, 1993; Salama et al., 1999; Shishov and Pankova, 2006; Rodikova, 2007; Özcan et al., 2018). The depth from which groundwater can make the surface saline is determined by the dryness of the climate, mechanical composition of the soil, and its structure. The main indicator of solonchak is the constant presence of readily soluble salts in the profile of the surface horizon in an amount that suppresses plant growth (Kovda, 1937; Shishov and Pankova, 2006). In the 0–20 cm layer, the critical level of toxic salts that stops plant growth is 1% (Pankova and Yamnova, 1993). Solonchaks belong to the intrazonal soil type and can form in different natural areas. The main condition for the formation of solonchaks is inflow of salts from the groundwater or parent rock to the upper part of a profile (Kovda, 1937; Pankova and Yamnova, 1993; Everest and Özcan, 2019). For natural solonchaks, the quantitative results of salt accumulation are determined by groundwater mineralization, evaporation, and the time during which the ascending solutions move (Kovda, 1937; Pankova and Yamnova, 1993; Shishov and Pankova, 2006). The formation of solonchaks with chloride and sulfate salinization is associated with the accumulation of salts formed as a result of weathering of sedimentary rocks, aeolian salt supply, and deluvial salt supply (Pankova and Yamnova, 1993; Rodikova, 2007; Hulisz et al., 2013). The degree of salinity can range from moderate to very strong. The chemistry of salinity is determined by the composition of rocks and groundwater and is distinguished by the predominant anions and cations. Among natural solonchaks with anionic composition, chloride, sulfate, chloride-sulfate, sulfate-chloride, and sodic soils are distinguished. Among natural solonchanks with cationic composition, sodium, calcium, and magnesium soils are distinguished (Kovda, 1937; Pankova and Yamnova, 1993; Shishov and Pankova, 2006). The impact of highly mineralized waters of different chemical composition on the ecosystem is a relevant issue for many regions and countries (Hulisz et al., 2013; Lapinskaya and Basalay, 2014; Akpan et al., 2015; Hulisz et al., 2017; Kulizhskiy et al., 2017; Xie et al., 2019; Ditz et al., 2020; Yin et al., 2022). Changes in the chemical composition of surface water and groundwater affect the composition of vegetation species (Khayrulina, 2014; Khayrulina et al., 2017; Simonova et al., 2018; Simonova et al., 2020a). Soils are negatively affected, as evidenced by changes in their physical and chemical properties (Akpan et al., 2015; Hulisz et al., 2017; Simonova et al., 2018; Özcan et al., 2018). The formation of solonchaks in a humid zone with a leaching water regime is induced by the inflow of sodium chloride brines into the soil, which is normally caused by human activity (Khayrulina, 2014; Simonova et al., 2018). Groundwater and surface water redistribute accumulated salts in the soil profile, which leads to the emergence of solonchaks or secondary solonchaks in landscapes with a leaching water regime. Solonchaks formed under these conditions show the spatial variability of salinity, which is associated with soil heterogeneity and changes in the groundwater level (Salama et al., 1999; Rodikova, 2007; Khayrulina, 2014; Simonova et al., 2018).
Perm Krai (Russia) is famous for its huge salt deposit and ancient salt extraction via brine wells that have been influencing the environment until now. Most of the ancient brine wells are flooded by the waters of the Kama Reservoir, and their discharge occurs directly at the bottom of it (Kharitonov, 2015; Khayrulina et al., 2017). In the Usolka River valley, single brine wells remain. They act as the hydrodynamic channel, allowing groundwater to circulate from the overlying freshwater aquifers of the terrigenous-carbonate stratum.
The purpose of this paper is to study the transformation of soil properties in the direction of soil formation and changes in the plant community of the Usolka River valley where ancient brine wells have been discharging over 300 years. The research objectives are to study the chemical and morphological properties of alluvial soils in the Usolka River valley, to examine transformations in vegetation as a result of soil changes, and to identify dominant processes of soil formation due to prolonged exposure of soils to salt water.
The scientific novelty lies in the study of saline landscapes in a humid climate with a leaching water regime. The patterns of formation and transformation of taiga soil systems under the conditions of Na-Cl water influx are of particular interest. An examination of the long-term impact of highly mineralized waters on the environment, as well as changes in the direction of soil-forming processes, can help in identifying and forecasting ecosystem changes caused by the potash production at one of the largest deposits, the Verkhnekamskoe Potash Deposit.
Materials and Methods
Study Area
The study area is located in the north-east of the central part of Perm Krai on the territory of Yayvinsky ostrozhok, one of the first Russian settlements with salt production (59.140290°N, 57.170782° E). Yayvinsky ostrozhok was founded in 1570. This territory was a settlement until the 19th century. Now the lands in the study area are classified as agricultural lands. This area is used by the locals as hay meadows. The floodplain where the research points are located is not used for agriculture. Nowadays, the nearest settlement—Ust-Igum village with population of 450 people—is located downstream of the study area and has no effect on its hydrochemical parameters. The study area is remoted from any industrial enterprises.
Salt mining was carried out on the south-eastern periphery of the salt deposit and outside the potash deposit’s outline, within the Cl–Na water distribution zone of the Cis-Ural foredeep (Shestov et al., 1986). Sodium chloride waters are widespread in this area. They are most commonly found on the eastern edge of the Verkhnekamskoe salt deposit, among the Upper and Lower Permian deposits (Figure 1). The occurrence of salt stratum in some areas of the halogenic formation distribution is relatively shallow, especially in river valleys, and ranges between 30 and 50 m (Lepikhin and Miroshnichenko, 2008). With shallow occurrence of salt stratum, brines naturally come to the surface in the form of springs or karst springs (Shestov et al., 1986; Beltyukov, 2006).
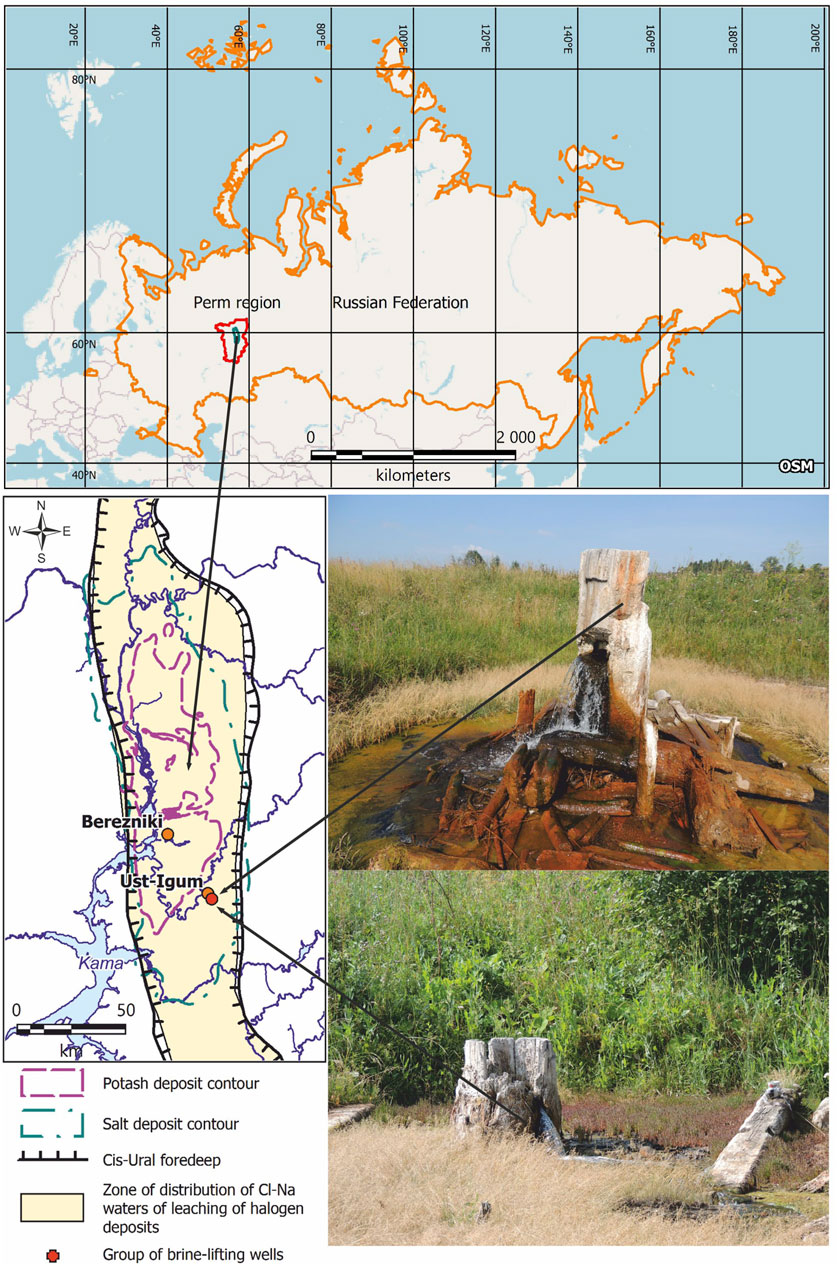
FIGURE 1. Distribution of potash and salt deposits of the Verkhnekamskoe potassium–magnesium salts deposit according to Shestov et al. (1986) and location of observation objects (photo by E. A. Khayrulina).
Since the 16th century, natural saline water outlets have contributed to the active development of the salt industry in Perm Krai. Initially, brine extraction was carried out from a depth of 30–40 m using brine pipes (Figure 1). The pipes were usually put in river valleys or depressions. The choice of wells location was based on salty taste of the water (Shestov et al., 1986). According to G. V. Beltyukov (Beltyukov, 2006), there were more than 200 wells with brine mineralization of 100–300 g/L within the deposit from 1430 to 1970. The brines were evaporated in large salt pans inside a special room where the salt was boiled out. These rooms were used for producing high-quality edible salt. The active development of salt production has led to salinization of pure water and soils (Beltyukov, 2006). The salt production was stopped in the 18th century.
The study area belongs to the East Russian landscape-geochemical province, specifically to the area of the denudation plain of the Cis-Urals and to the area of the Solikamsk plain, to the group of natural-technogenic floodplain-meadow landscapes (Gavrilov, 1992; Beltyukov, 2006). Currently, the study area is not under any anthropogenic influence. According to Kopylov (2014), the study area is characterized by increased geochemical values of Pb, Cr, Mn, Zn, Ni, Cu, Cd, and Zr. As a result of previous long-term industrial development (salt mining from the 16th to the 18th centuries), artificial geochemical anomalies have formed, including salt elements that are not characteristic of the previously existing natural landscapes of this territory.
The climate of the area is moderately continental, with an uneven distribution of precipitation and river water regime. The average annual precipitation is 600 mm; the average January temperature is −15, −16°C; the average July temperature is +17°C (Tartakovsky, 2012).
The relief of the territory is plain and low. The study area is located on the high floodplain of the Usolka River, which is lined with mantle loess-like loams and clays. In terms of geomorphological zoning, the boundaries of the study area are part of the Cis-Ural depression. According to hydrological zoning, the Usolka River belongs to the Kama-Vishera region, which has the following characteristics: lowland rivers, extensive forestry area, swampiness and karsting of basins, thick strata of easily eroded alluvial accumulations, small elevation differences, low river banks, wide valleys, and river bed meandering.
Geologically, the study area is located in the Cis-Ural foredeep, in the southern part of the Solikamsk depression, within the Ust–Igumsky salt swell. Lower Permian deposits of the Ufimian stage, represented by the Sheshmian and Solikamsk horizons, and the Kungurian horizon of the Filippovsky stage, lie directly under the quaternary deposits (Kudriashov, 2001) (Figure 2).
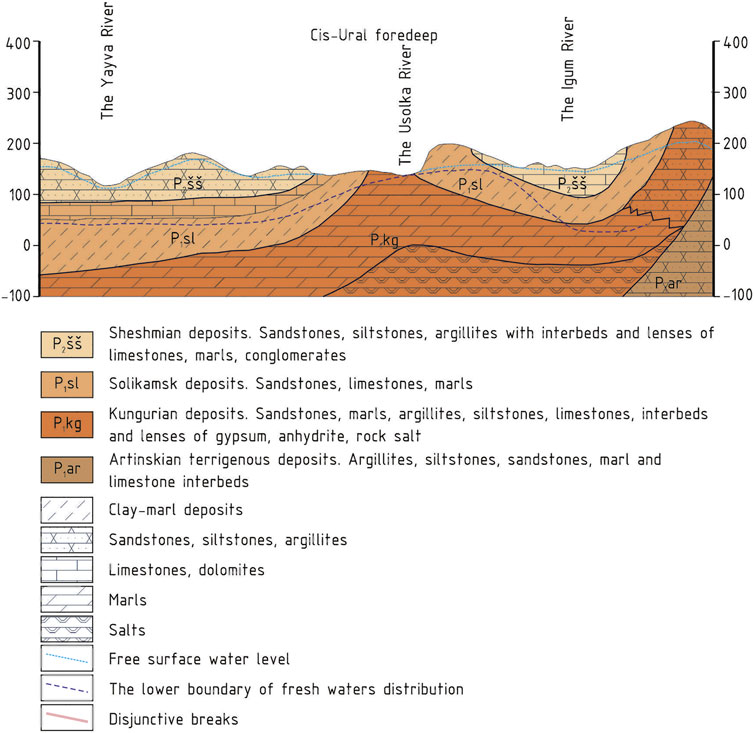
FIGURE 2. Geological section of the study area (Kudriashov, 2001).
The upper part of the Solikamsk horizon at the Verkhnekamskoe potassium salt deposit is presented by the terrigenous-carbonate stratum (an average thickness of about 110 m) (Kudriashov, 2001); its rocks have low permeability and low water content (Kopylov and Konoplev, 2013). The terrigenous-carbonate stratum is underlain by a salt-marl stratum in the lower part of the Solikamsk horizon, which is composed of marls and clays with anhydrite, gypsum, and rock salt interbeds in the lower part of the section. The lower part of the salt-marl stratum that contains rock salt beds is called the transition member. The top of the transition member is a salt table, which is the top of the first rock salt bed from the surface. The average thickness of the salt-marl stratum is 80 m, including the thickness of the transition member, which is 20 m (Kudriashov, 2001). According to Kopylov and Konoplev (2013), underground waters in the upper part of the salt-marl stratum have mostly sulfate-calcium chemical composition and mineralization of up to 3 g/L. Locally widespread brine horizon belongs to the transition member. The horizon is located on a salt table and contains sodium chloride brines with mineralization of up to 300 g/L. The waters of the brine horizon can be extremely pressurized. In some places, they discharge to the daylight surface in the form of highly mineralized springs through karst and tectonic cracks (Kopylov and Konoplev, 2013).
Water and Soil Sampling
A group of ancient brine wells is located on the right bank of the Usolka River (left tributary of the Yayva River). Four wells are located on a floodplain terrace at a distance of 80–120 m from the Usolka River. Wells form two streams that flow into the Usolka River. Some wells still have the remains of wooden structures (Figure 1). Water samples were collected from five brine wells and the Usolka River from 2015 to 2021. The studied territory has a total area of about 5 km2.
Samples of surface water, springs, and infiltration were collected four times a year in polymeric bottles during low-water levels in December and July and high-water levels in May and October. Water samples were collected in other polymeric bottles to determine the Fe total. HCl was added to each bottle to achieve a final concentration of 0.2–0.3 mol/L HCl for conservation, according to PND F 14.1: 2: 3.2–95 (PND F 14.1: 2: 3.2-95, 1995).
The soil was sampled in 2021 in the Usolka River floodplain under halophytes at the distances of 1 and 5 m from a saline stream bed, under salt-tolerant plant species, and on the Usolka River bank outside the impact area of saline stream water as a background Figure 3. In order to find and characterize soil properties, three soil sections up to 100 cm deep were made in 2021. The location coordinates of the sections are 59.140375°N 57.170823°E.
Laboratory Analyses
The pH of the water was determined by the potentiometric method;
In the soil samples, the following properties were determined: soil organic matter—by the wet combustion method using chromic acid and photometer, according to Tyurin (Vorobevа, 2006); pH-H2O and pH-KCl—by the potentiometric method using рН meter, according to GOST 26483–85 (GOST 26483-85, 1985a); hydrolytic acidity—by the Kappen method based on the treatment of a soil sample with sodium acetate at a concentration of 1 mol/L followed by titration of the soil extract with an alkali solution (Vorobevа, 2006); exchangeable cations—by the Kappen-Gilkowitz method based on the treatment of soil samples with a certain amount of 0.1 mol/L HCl followed by titration of the soil extract with 0.1 mol/L NaOH (Vorobevа, 2006); cation exchange capacity (CEC)—by the barium chloride method (Vorobevа, 2006); mobile compounds of phosphorus and potassium—by the Kirsanov method (Vorobevа, 2006) based on the extraction of mobile compounds of phosphorus and potassium from soil with 0.2 mol/L HCl solution; mobile phosphorus compounds in the form of a blue phosphorus-molybdenum complex—by the photoelectric colorimetric method; potassium compounds—by flame photometry (Workshop on agrochemistry and Mineev, 2001); exchangeable sodium—in the ammonium acetate extract using a flame photometer, according to GOST 26950–86 (GOST 26950-86, 1986); exchangeable calcium and exchangeable (mobile) magnesium—by complexometric titration with an acid chrome dark-blue indicator, according to CINAO methods (GOST 26487–85) (GOST 26487-85, 1985b); mobile iron—by the photometric method using o-Phenanthroline in sulfuric acid extract, according to GOST 27395–87 (GOST 27395-87, 1987); water soluble ions Na+ and K+—by flame photometry (Workshop on agrochemistry and Mineev, 2001); Cl−—by titration with silver nitrate (Workshop on agrochemistry and Mineev, 2001); Ca2+ and Mg2+—by the trilonometric method (Workshop on agrochemistry and Mineev, 2001); sulfates—by the turbidimetric method by means of precipitation with barium chloride (GOST 26426–85) (Workshop on agrochemistry and Mineev, 2001); the sum of toxic salts—by the calculation method (Bazilevich and Pankova, 1968).
In order to indicate sodium soils, we used the sodium adsorption ratio (SAR) which can also be used as an alternative to the exchangeable sodium percentage of cation exchange capacity. SAR is defined as the ratio of the Na+ concentration to the square root of the sum of Ca2+ and Mg2+; if SAR is greater than 13, the horizons are classified as solonetzic (Van Reeuwijk, 2006).
Ground vegetation was described in the area of brine wells outflow. We identified plant species using the herbarium of the Laboratory of Botany of Perm State National Research University. When studying the biodiversity of terrestrial ecosystems, we looked for salt tolerant plant species.
We used the soil-geochemical and landscape approaches to characterize natural components of the study area. Changes in the qualitative composition of interconnected landscape elements—surface water, groundwater, and relief—are inextricably linked to soil transformation processes.
We used the STATISTICA 7 and MC Excel software to calculate statistical indicators such as mean, coefficient of variation, and coefficient of determination. We used the Student’s t-test to estimate the statistical significance of a difference. In order to find dependencies between the indicators, we found the coefficients of correlation and determination; the coefficient of variation showed the variability of the studied values and properties.
Results
Chemical Composition of Water
Surface water bodies have a unique hydrochemical background due to highly mineralized waters erupting from brine wells. Currently, the mineralization of well waters is 30–34 g/L (Table 1); chloride and sodium ions prevail; the flowrate of the wells is 0.009–0.011 m3/s. The chemical composition of brine well water is stable. The
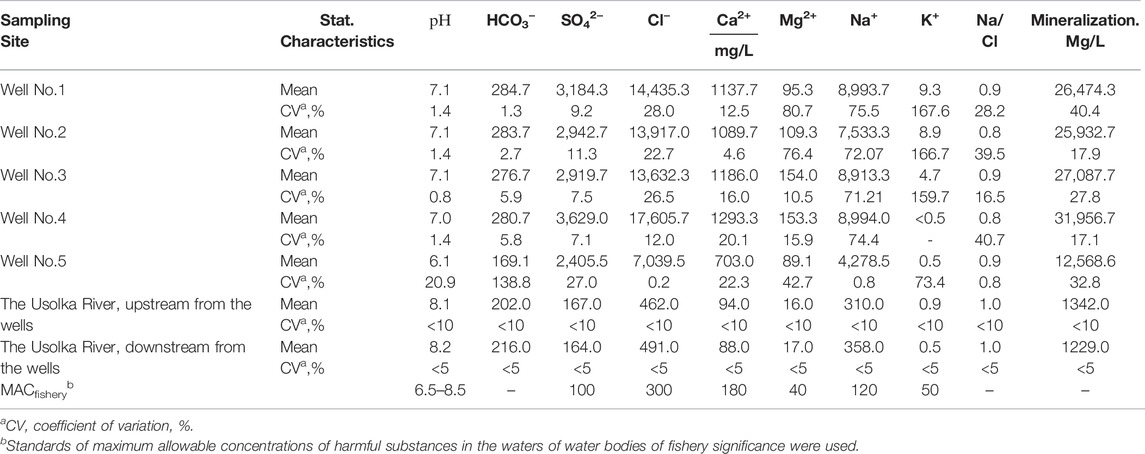
TABLE 1. The chemical composition of the waters of ancient brine wells at the site of the ancient settlement of Yayvinsky ostrozhok, 2015–2017.
The inflow of brines contributes to the Na–Cl composition of the river water. Mineralization of water in the Usolka River is much lower due to dilution with fresh water (1.2–1.3 g/L). The predominance of Na and Cl in the chemical composition of the Usolka River waters upstream of the brine wells confirms the presence of yet unknown salt springs (Table 1). The coefficient, defined as the ratio of the sodium ions to chloride ions (Na/Cl), indicates that halite dissolution is responsible for the chemical composition of brines.
Plant Species Composition
The vegetation cover of the study area is represented by species that are specific to floodplain meadow landscapes. Floodplain tallgrass of the study area includes meadow fescue Festuca pratensis Huds., alsike clover Trifolium hybridum L., common yarrow Achillea millefolium L., meadow vetchling Lathyrus pratensisL., cock’s-foot Dáctylis glomerata L., timothy-grass Phleum pratense L., meadow buttercup Ranunculus acris L., autumn hawkbit Leontodon autumnalis L., smooth meadow-grass Poa pratensis L., garden angelica Angelica officinalis Hoffm., meadowsweet Filipendula ulmaria (L.) Maxim, and parsnip Pastinaca sylvestris Mill. The plant community is represented mainly by salt tolerant plant species.
Salicornia perennans W. has appeared due to the high salinity of soils along the saline stream (Figure 4). This species was found in Perm Krai for the first time and was described only in the area of brine wells (Khayrulina et al., 2017). It is one of the obligate halophytes with the highest salt resistance, growing and developing well on saline soils and absorbing large amounts of salt from the soil. As a result of the high salt accumulation in the cell sap, the water potential of the cells is greatly reduced, and water enters the plants even from saline soil. The salt that is accumulated in vacuoles has no effect on the physiological processes of the plant (Ivanishchev, 2019).
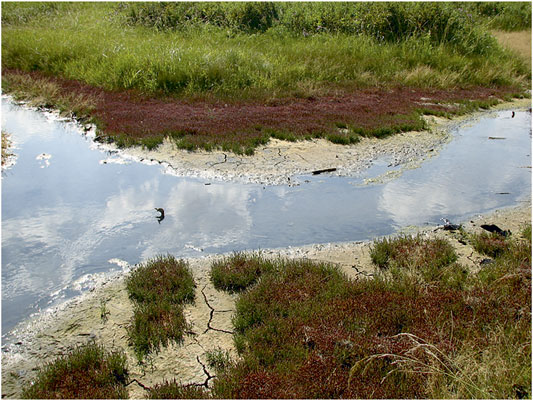
FIGURE 4. Overgrowth of the banks of a salt brook with saltwort Salicornia perennans W. (photo by E.A. Khayrulina).
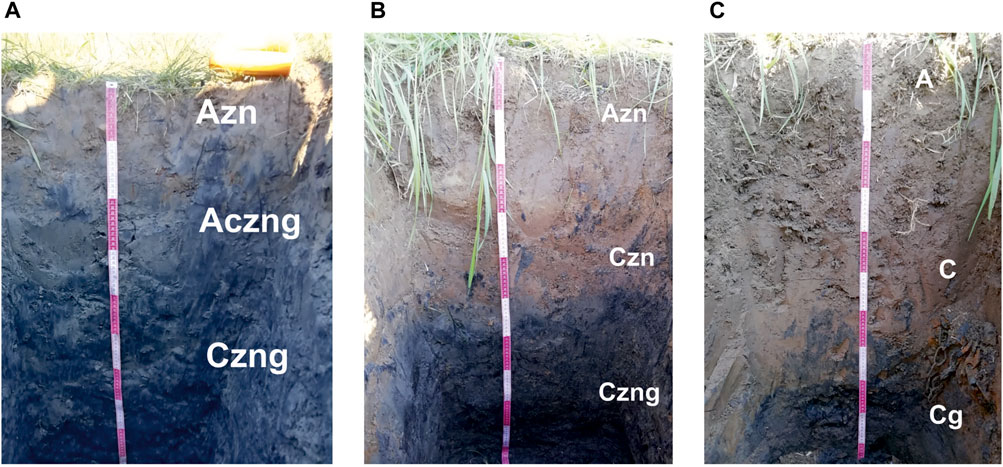
FIGURE 5. Studied soils (photo by N. V. Mitrakova): (A) Chloridic Gleyic Fluvic Solonchak (Humic, Loamic), (B) Gleyic Fluvisols (Humic, Loamic, Sulfatic), and (C) Gleyic Fluvisols (Humic, Loamic).
Halophytes typically grow on highly saline soils along sea coasts, along the shores of salt lakes, and in washes and gullies. Halophytes form red “glades” and “paths” in areas where highly mineralized waters are discharged. In this case, they grow along the banks of a stream formed by the waters of ancient brine wells. Typically, halophytes appear on saline soils (Lavrinenko et al., 2012; Sommer et al., 2020). In humid climate with leaching water regime, the appearance of halophytes is possible due to long-term impact of saline waters on soils. Typically, this process has anthropogenic origin.
Morphology, Agrochemical, and Chemical Properties of Soils
The soil types were determined according to the Classification and Diagnostics of Russian Soils (2004) (Classification and diagnostics of Russian Soils, 2004) and IUSS-WRB (2014) (World Reference Base for Soil Resources, 2015).
As a result of long-term inflow of highly mineralized groundwater onto the soil following its outflow from brine wells of Yayvinsky ostrozhok, a Chloridic Gleyic Fluvic Solonchak (Humic, Loamic) was formed in the floodplain of the Usolka River, on the bank of a saline stream (Figure 4) (secondary gley humus solonchak with sulfate-chloride sodium type of salinization (Classification and diagnostics of Russian Soils, 2004)). The studied Chloridic Gleyic Fluvic Solonchak (Loamic) was formed from the Gleyic Fluvisols (Humic, Loamic) (alluvial humus gley soil) that are typical for floodplains of taiga landscapes. The upper horizon is characterized by heavy granulometric composition, weakly acidic or neutral reaction of the soil medium, and low adsorption capacity (Classification and diagnostics of Russian Soils, 2004). Morphological properties of soils are shown in Table 2.
The content of organic matter in the topsoil of Chloridic Gleyic Fluvic Solonchak (Humic, Loamic) was the highest along the profile, at 3.56% (Table 3). Up to 70 cm depth, the content decreased slightly to a minimum value of 2.39% and then increased. The coefficient of variation showed an insignificant degree of data dispersion. The inflow of mineralized waters containing carbonates and sodium bicarbonates from brine wells explains the alkaline reaction of the soil. The value of cation exchange capacity depends on several factors, including the type of soil, cation composition of the soil adsorption complex, and granulometric composition (Rodikova, 2007). The studied Chloridic Gleyic Fluvic Solonchak (Humic, Loamic) had an average CEC of 23–31 mmol/100 g. The CEC value is associated with the content of organic matter and clay soil texture. We found no significant differences in the content of exchangeable calcium and sodium (with average content of 9.64 and 10.8 mmol/100 g, respectively). However, a coefficient of variation of about 35% for both indicates that the values vary along the soil profile. The exchangeable magnesium and mobile iron values were not greater than 2.3 mmol/100 g and 2.3 per mille, respectively. Starting at a depth of 20 cm, the sodium adsorption ratio (SAR) was more than 13, indicating a solonetz-formation process in the soil. The 70–80 cm layer had the highest SAR and exchangeable sodium values; these indicators can prove soil salinity (Klopp et al., 2020; Gharaibeh et al., 2021). The sum of toxic salts indicated severe soil salinization. The salinity increases with depth. NaCl, Na2SO4, and Na2CO3 are considered as predominant hypothetical salts.
The topsoil organic matter content in Gleyic Fluvisols (Humic, Loamic, Sulfatic) was 5.28%; it decreased with depth 1.5–2 times; the soil had an alkaline reaction and the average CEC according to Valkov (Valkov, 2004), which decreased with depth 1.2–1.7 times from 24 mmol/100 g, and it reached a minimum value of 14 mmol/100 g in the middle layers (Table 3). The content of exchangeable calcium ranged from 7.25 mmol/100 g in deep layers to 17.1 mmol/100 g in topsoil. At a depth of 70–100 cm, the value of exchangeable sodium increased with depth from 3 mmol/100 g to 7–8 mmol/100 g. We found that the content of exchangeable sodium increased, while the content of exchangeable calcium decreased. The impact of sodium chloride waters allowed exchangeable sodium to enter the soil adsorption complex. The average content of exchangeable magnesium was 1.89 mmol/100 g, ranging from 1.44 mmol/100 g in the 50–60 cm layer to 2.75 mmol/100 g in the topsoil. The coefficient of variation of mobile iron content was approximately 45%, showing the heterogeneity of the soil layers regarding this indicator. It could be associated with the redox environment. The SAR increased with depth from 3.3 to 10.3. These values indicate that the formation of solonetz has not occurred. The exchangeable sodium percentage of CEC indicated soil salinization. The sum of toxic salts in the topsoil was 0.13%, and it increased three times with depth. The soil was not saline up to 60 cm depth, and the salinity level was low.
The average content of organic matter in Gleyic Fluvisols (Humic, Loamic) was 4.7% up to 40 cm depth; the amount of Corg decreased slightly with depth. The coefficient of variation was 22.1%, indicating the heterogeneity of the Corg content along the profile (Table 3). The reaction of the soil varied from neutral in the upper 10 cm layer to slightly alkaline along the profile. The CEC varied insignificantly and was approximately 25 mmol/100 g. Up to 70 cm depth, the average amount of exchangeable calcium was 21 mmol/100 g. From a depth of 80 cm, the amount of calcium decreased by two times. The coefficient of variation (27.1%) reflects uneven calcium content. The exchangeable magnesium content was unevenly distributed along the profile as well, ranging from 3 mmol/100 g in the upper layer to 1.19 mmol/100 g in the lower layer. The amount of exchangeable sodium did not exceed 0.6 mmol/100 g. The SAR was less than 1.3, indicating the absence of exchangeable sodium in the soil adsorption complex, no salinity, and the transformation of the physical properties of the soil. The sum of toxic salts was less than 0.01%.
The sum of toxic salts indicated salinization of Chloridic Gleyic Fluvic Solonchak (Humic, Loamic) according to the criteria of N. I. Bazilevich and E. I. Pankova (Bazilevich and Pankova, 1968); sulfate-chloride sodium salinity was observed throughout the soil profile, which increased with depth (Figure 6A). Gleyic Fluvisols (Humic, Loamic, Sulfatic) had chloride-sulfate sodium salinity (Figure 6B) due to a change in the prevailing anions in the soil water extract. It appeared that in the transitional type of soil, at a distance of 5 m from the saline stream, sulfate ions accumulated in the profile, and chlorides were washed out, facilitating a non-toxic level of soil salinity. In general, the chemistry of the soil profile was determined by the waters of brine wells with sulfate-chloride salinity. Gleyic Fluvisols (Humic, Loamic) lacked salinity (Figure 6C), and the anions and cations content did not exceed 10–30 mg/100 g.
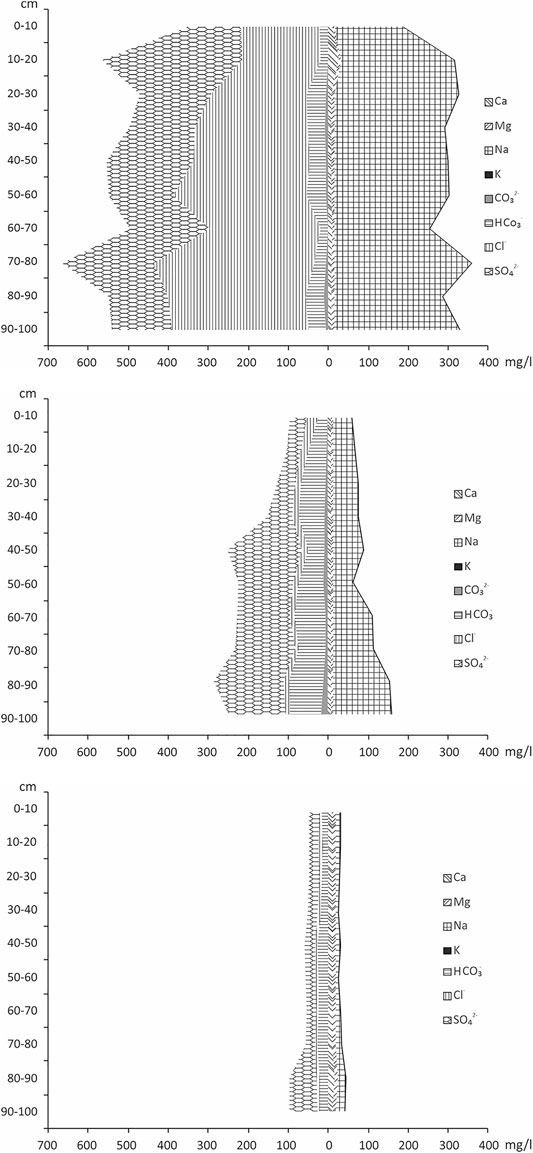
FIGURE 6. Salt profile of: (A) Chloridic Gleyic Fluvic Solonchak (Humic, Loamic), (B) Gleyic Fluvisols (Humic, Loamic, Sulfatic), and (C) Gleyic Fluvisols (Humic, Loamic).
The chlorides and sodium content increased with depth in Chloridic Gleyic Fluvic Solonchak (Humic, Loamic) (Figure 6A). We noted the highest content of Cl− and Na+ in the 70–80 cm layer, at 408 and 350 mg/100 g of soil, respectively. The 0–10 cm layer had the lowest amounts of chlorides and sodium—200 and 172 mg/100 g, respectively. In comparison to the topsoil, the content of chlorides and sodium increased 1.5–2 times with depth. The sum of calcium, magnesium, and potassium did not exceed 30 mg/100 g. The hydrocarbonate content was heterogeneous along the profile but did not exceed 50 mg/100 g of soil. The 10–20 cm layer had the highest sulfate content (350 mg/100 g), while the 0–10 cm layer had the lowest (130 mg/100 g).
The chloride content in Gleyic Fluvisols (Humic, Loamic, Sulfatic) did not exceed 35 mg/100 g. The sulfate content increased 3–4 times with depth; the lowest amount (40 mg/100 g) was in the 10–20 cm layer, and the highest amount (210 mg/100 g) was in the 40–50 cm layer. The content of hydrocarbonates and sodium also increased with depth, peaking in the 80–90 cm layer at 100 and 160 mg/100 g of soil, respectively.
Chloridic Gleyic Fluvic Solonchak (Humic, Loamic) contained 6–30 times less chlorides and slightly more hydrocarbonate ions than Gleyic Fluvisols (Humic, Loamic, Sulfatic). The content of sulfate ions at 40 cm depth was practically the same in both soils. The amount of sodium in the Solonchak was three times lower than in Fluvisols (Humic, Loamic, Sulfatic). In both solonchak and alluvial soil, the content of ions increased with depth. The content of calcium and magnesium in both soils did not exceed 10 and 5 mg/100 g of soil, respectively.
Gleyic Fluvisols (Humic, Loamic, Sulfatic) was characterized as the transitional type of soil between Chloridic Gleyic Fluvic Solonchak (Humic, Loamic) and Gleyic Fluvisols (Humic, Loamic).
Depending on the climatic conditions, the mineralization of well water ranged from 15 to 38 g/L. Sulfates (approximately 3,000 mg/L), chlorides (approximately 13,000 mg/L), and sodium ions (approximately 7,500 mg/L) were the most abundant ions in the water. The increased content of ions in water ensured their elevated content in the soil water extract and its salinity. The low filtration capacity of rocks and the clayey granulometric composition of soils contributed to significant salinization of the territory only along a 1.5–2 m wide saline stream. The high content of sodium sulfates and sodium chlorides in soil water extract explains the significant portion of exchangeable sodium in the soil adsorption complex. Spearman’s correlation coefficient revealed a positive correlation between the sodium content in the water extract and exchangeable sodium in saline soils, indicating the influence of mineralized waters on soil salinity.
Statistical analysis by means of the t-test showed differences in the properties of the studied soils. Thus, Chloridic Gleyic Fluvic Solonchak (Humic, Loamic) and Gleyic Fluvisols (Humic, Loamic, Sulfatic) had lower organic matter content and more alkaline soil reaction than background soil Gleyic Fluvisols (Humic, Loamic). The content of exchangeable calcium was 1.3–2 times lower; the content of exchangeable sodium was 6–24 times higher; the SAR value varied by an order of magnitude or more. The occurrence of the solonetz formation process is indicated by 1) the change in the percentage of exchangeable cations in the soil adsorption complex, resulting in the predominance of exchangeable sodium, 2) alkalization of the soil solution, and 3) the SAR value above 13. The level of toxic salts in Chloridic Gleyic Fluvic Solonchak (Humic, Loamic) and soil salinization with water-soluble salts indicates that sodium chloride brines affect the transformation of background soils and contribute to the formation of a new type of soil (solonchak) during the salinization process.
According to Shishov and Pankova (2006), the method of assessing soil salinity by the content of individual ions was used to obtain information on soil salinity. Depending on the composition of salts, different ions can carry the most accurate information on salinity. Thus, in our study, in the case of sodium chloride salinization of soils, chloride ion and sodium ion served as such ions. To assess soil salinity by individual ions, a correlation was found between the content of toxic salts and the content of chloride ions and sodium ions through the coefficient of determination (Figure 7). A very close link was found between the sum of toxic salts and the content of sodium ions in soil water extracts (R2 = 0.92; p = 0). For chloride ions, the bond was weaker (R2 = 0.58; p = 0.0155). We found no relationship between sulfate ions and the sum of toxic salts, indicating the sodium chloride type of salinity in the studied soil.
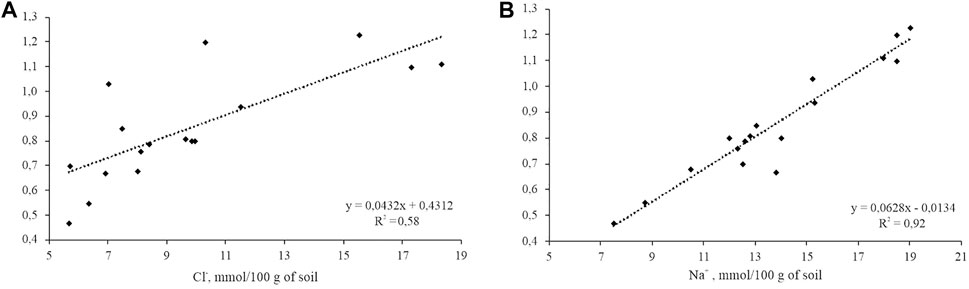
FIGURE 7. Dependence of the sum of toxic salts on the content of: (A) chloride ions and (B) sodium ions in the water extract of soils. R2 = coefficient of determination.
The regression coefficient, found between the chloride ions of the brine wells and the soil water extract, was 0.55 (p = 0.025). For sodium, the regression coefficient was 0.38 (p = 0.025). No regression relationship was found between the content of calcium ions and magnesium ions in water and soil.
Discussion
The forming streams with salinity levels of more than 30 g/L, flowing from the wells to the Usolka River, created a special geochemical background of this landscape. Highly mineralized brines entered the soil profile. An increase in sodium chlorides and sodium sulfates in soils has led to the appearance of salt-tolerant plants and the obligate halophyte instead of native vegetation.
Salinization negatively affects plant biochemical and physiological processes such as photosynthesis, stomatal activity, neutralization of reactive oxygen species, enzyme activity, and plant height and weight (Tavakkoli et al., 2010; Khudyaev and Banaev, 2012; Ivanishchev, 2019; Yin et al., 2021). Salt stress in plants suppresses the formation of proteins and increases the breakdown of already formed protein complexes (Vargas et al., 2017; Ivanishchev, 2019; Chaudhry et al., 2022). A decrease in protein synthesis shows itself in a noticeable decrease in growth and development rates of plants and disruption of metabolic processes. An increase in the degree of salinity leads to disruption of homeostatic water balance (Ivanishchev, 2019; Chaudhry et al., 2022). Numerous studies have shown that toxicity occurs at lower concentrations in the case of chloride salinity than in the case of sulfate salinity (Khudyaev and Banaev, 2012). Plants can withstand salt stress up to a certain level of salinity. However, once the salt concentration threshold is exceeded, cell death and plant death occur. The functioning, growth, and development of plants on saline soils suggest that they have special mechanisms of adaptation. The stability of phytocenosis during salinization is ensured by the replacement of plants with salt-tolerant species. The appearance of the obligate halophyte Salicornia perennans W. indicates a high degree of long-term salinization. Similar processes were found in Hulisz et al. (2013).
In Perm Krai, due to the humid type of climate and leaching water regime, the formation of saline soils is associated only with natural discharge of saline springs in floodplains of rivers in areas of salt mass bedding as well as with consequences of industrial extraction of potash salts.
The studied solonchak has a more alkaline reaction compared to the background alluvial soil from which it has formed (Figure 8). Due to the long-term effect of sodium chloride brines on soils, the content of exchangeable sodium increased many times over in comparison with the background soil. The content of water-soluble sodium and the sodium adsorption ratio (SAR) in solonchak indicated the transition of sodium into the soil adsorption complex. Sodium, displacing other cations, mainly calcium and magnesium, from the soil adsorption complex, contributed to the deterioration of the physicomechanical properties of the soil, which caused its viscosity, stickiness, lack of structure in the wet state, and firmness in the dry state.
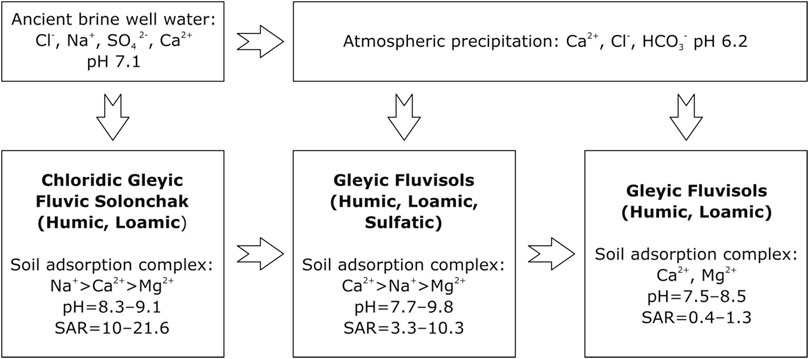
FIGURE 8. Schematic model of the transformation of soil characteristics under the influx of Cl–Na water.
The organic matter content of Chloridic Gleyic Fluvic Solonchak (Humic, Loamic) is significantly lower than that of Gleyic Fluvisols (Humic, Loamic). This is associated with the absence of herbaceous vegetation on solonchak and with a decrease in the solubility of carbon dioxide and oxygen in sodium chloride waters, according to Bogashova (2011). This entails a decrease in the mineralization of organic matter.
In the studied Chloridic Gleyic Fluvic Solonchak (Humic, Loamic), the chloride type of salinization predominates, which is associated with the inflow of brines from the wells. In natural solonchaks, the sulfate type of salinization predominates (Yamnova and Pankova, 2013), which is associated with the composition of underlying rocks. Gypsum was not found in the studied saline soils, but it was found in sulfate-type solonchaks, where its amount in the profile reaches 30% or more.
In arid saline soils, the content of sodium, chlorides, and sulfates is higher due to the underlying saline rocks and exudational water regime. In the studied solonchaks, the content of these ions is lower due to the rocks being alluvial and not saline. The humid climate contributes to the dilution and removal of salts. The main salinity factor is brines rising to the surface.
In Perm Krai, existing enterprises for the extraction of potassium–magnesium salts greatly affect the landscapes in addition to old brine wells (Baturin, 2012; Maksimovich and Pervova, 2012; Aleynikov et al., 2018; Khayrulina and Maksimovich, 2018; Mitrakova et al., 2020). The processes of transformation of alluvial soils into solonchaks were described in Siberia (Svanidze et al., 2014; Kazantseva and Svanidze, 2016), where the influence of old and abandoned exploration wells on floodplain soils was studied. The influx of sodium chloride water from the Artesian wells’ activated salinization process. This led to the transformation of soddy-ground-gley and alluvial soils into chloride-sodium solonchaks. In the Upper Volga basin, in a temperate climate in the area of salt sources discharge (the former center of salt formation), with a change in climatic conditions, saline soils with chloride-sulfate sodium-calcium salinity were formed (Simonova et al., 2020b). Sodium chloride chemical quality defines the alluvial soils in the impact area of highly mineralized water sources from artesian wells in the Yaroslavl Volga region (Yaroslavskoe Povolzhye). In a humid climate, soils accumulate water-soluble salts (Simonova et al., 2020a). This indicates that when chloride sodium waters enter the soil, saline soils with sodium chloride chemistry (solonchaks) are formed in a temperate climate.
The studied Chloridic Gleyic Fluvic Solonchak (Humic, Loamic) differed in the content of water-soluble ions from natural Mollic Solonchak (Loamic) of the Western Caspian region that formed under hydromorphic conditions of the arid zone (Saidov, 2008). The chloride ion and sodium ion content in natural Mollic Solonchak (Loamic) is 1.5 and 2 times higher than their content in Chloridic Gleyic Fluvic Solonchak (Humic, Loamic), respectively. It should be noted that the content of chlorides and sodium tends to decrease with depth in the compared soils, while the content of sulfates increases with depth. The chloride type of salinity prevailed in both soils. The gypsum content in Mollic Solonchak ranged from 0.2 to 3.7% from layer to layer. No gypsum was found in the Chloridic Gleyic Fluvic Solonchak (Loamic).
The Mollic Solonchak of the Eastern Siberia of the Tyva Republic has the sulfate type of salinity (Shishov and Pankova, 2006), as evidenced by the composition of the water extract. Chloridic Gleyic Fluvic Solonchak (Humic, Loamic), formed in the area of brine well outflow, has lower content of water-soluble ions, especially sulfates, calcium, magnesium, and gypsum. It is associated with the continuous flow of highly mineralized sodium chloride water to the soil, whereas salinization in natural solonchaks (Mollic Solonchak) is associated with salt accumulation caused by weathering of sedimentary rocks.
In humid climate, solonchaks are formed under similar conditions, mainly due to the influence of saline springs or wells. The Gleyic Gypsic Calcic Solonchak has formed in the Upper Volga basin in the depression of Lake Nero in the area of salt springs discharge—the former center of salt production, which was terminated several centuries ago (Simonova et al., 2020b). Gleyic Gypsic Calcic Solonchak, characterized by hydrogenic salt accumulation, undergoes significant salinity fluctuations depending on episodic salt rise. The sum of toxic salts in the upper layer of the Gleyic Gypsic Calcic Solonchak was twice as high as in the Chloridic Gleyic Fluvic Solonchak (Humic, Loamic). The indicator did not differ with soil depth. Formed as a result of the influence of ancient salt mining in humid climate, Gleyic Fluvic Solonchak (Loamic) differs in the type of salinity and the content of predominant ions and gypsum. It should be noted that the waters pouring out onto the surface in both cases had sodium chloride composition. Halophytes and salt-tolerant species (Juncus gerardii, Triglochin maritimum) found on the site are typical to moist saline meadows and solonchaks.
Thus, salinization of the studied soils occurs as a result of the inflow of highly mineralized sodium chloride waters into the soil via ancient brine wells. Wells act as the hydrodynamic channel for groundwater enriched in sodium chloride brines. The Chloridic Gleyic Fluvic Solonchak (Humic, Loamic) is formed from mature alluvial soil. Saline water, relief, climate, and the duration of the brine impact on the soil were the main soil-forming factors in solonchak formation in the Usolka River valley in the brine outlet area. These factors determined the occurrence of salinization in background alluvial soils, as well as solonization and soil gleization, which resulted in the formation of ferrous iron under reducing conditions. Rusty spots on the soil profile wall indicate the presence of ferric iron (Fe3+). The content of organic matter along the soil profile indicates the occurrence of humification processes. Taken together, environmental factors such as brine influx to the surface and active soil-forming processes led to the emergence of Chloridic Gleyic Fluvic Solonchak (Humic, Loamic). Despite the fact that saline waters are classified as eventual soil-forming factors (Kudejarov and Ivanov, 2015), they were the main cause of solonchak emergence in our study. Despite genetic and chemical changes in the soil, with the limitation or complete absence of brine influence, the soil evolution can go the opposite way, with desalinization of solonchaks occurring and the soil becoming alluvial humic once more.
Conclusion
Long-term discharge of ancient brine wells in the Usolka River floodplain in the area of Gleyic Fluvisols (Humic, Loamic) resulted in the formation of Chloridic Gleyic Fluvic Solonchak (Humic, Loamic) and Gleyic Fluvisols (Humic, Loamic, Sulfatic).
The studied solonchaks are characterized by sodium chloride salinization. The SAR and the amount of exchangeable sodium indicate that it has replaced exchangeable calcium and magnesium in the soil adsorption complex. The outflow of brines has led to soil alkalization. In its wet state, the soil has a viscous structure. In general, the physical and mechanical properties of the soil have deteriorated.
Salinization of the soil led to a change in plant species to more salt-tolerant ones. In the immediate vicinity of the streams flowing from the wells, the obligate halophyte Salicornia perennans W. appeared.
The formation of Chloridic Gleyic Fluvic Solonchak (Humic, Loamic) from Gleyic Fluvisols (Humic, Loamic) under the influence of brines is considered in the analysis of the transitional soil and the background soil.
The major soil-forming processes are solonization (based on the entry of exchangeable sodium into the soil adsorption complex), salinization (identified by the accumulation of water-soluble salts in the upper part of the profile due to the inflow of brines onto the surface), and gleization (which leads to the formation of clayey materials containing ferrous iron). The exchangeable sodium and alkaline reaction of the soil lead to mechanical destruction followed by crushing of the colloidal-silty part of the soil, implying a deterioration in the physical properties of the soil.
Future research will focus on the trace element composition in saline and background soils. Migration of trace elements, which have a high correlation with salt accumulation and are actively leached from soils and rocks in chloride brines, is not considered. The issue of biogeochemical accumulation of trace elements is of great interest. These studies have already begun and will be published in the near future.
Data Availability Statement
The original contributions presented in the study are included in the article/Supplementary Material, further inquiries can be directed to the corresponding author.
Author Contributions
Conceptualization, EK; methodology, EK and NM; software, AP; validation, EM and NM; formal analysis, PN and AP; investigation, EK and NM; writing—original draft preparation, EK; writing—review and editing, ME; visualization, NM; project administration, EK; funding acquisition, EK. All authors have read and agreed to the published version of the manuscript.
Funding
This research was funded by the Ministry of Science and Higher Education of the Russian Federation, project No. 2019-0858.
Conflict of Interest
The authors declare that the research was conducted in the absence of any commercial or financial relationships that could be construed as a potential conflict of interest.
Publisher’s Note
All claims expressed in this article are solely those of the authors and do not necessarily represent those of their affiliated organizations, or those of the publisher, the editors and the reviewers. Any product that may be evaluated in this article, or claim that may be made by its manufacturer, is not guaranteed or endorsed by the publisher.
Acknowledgments
The authors wish to thank Evgeniya Yakovleva for providing assistance in working on the text and Aleksey Denisov for organizing of and assistance in field work.
References
Akpan, A. E., Ebong, E. D., and Ekwok, S. E. (2015). Assessment of the State of Soils, Shallow Sediments and Groundwater Salinity in Abi, Cross River State, Nigeria. Environ. Earth Sci. 73, 8547–8563. doi:10.1007/s12665-015-4014-6
Aleynikov, А. A., Stenno, S. P., Tsiberkin, N. G., Mеlnichuk, A. F., and Sadovnikova, E. N. (2018). Influence of the Perm Salt Production in the 15th - 19th Centuries on forest Transformation: Impact Assessment. Rjee 3 (1). doi:10.21685/2500-0578-2018-1-2
A. M. Tartakovsky Editor (2012). Atlas of Perm Krai. (Perm: Perm State University), 124. (In Russian).
Baturin, E. N. (2012). Problems of the Development of the World Largest Potash Deposits. Mod. Probl. Sci. Educ. 6.
Bazilevich, N. I., and Pankova, E. I. (1968). Experience of Soil Classification According to Salinity. Eurasian Soil Sci. 11, 3–16. (In Russian).
Beltyukov, G. V. (2006). Karst and Supergene Processes in Evaporates. Perm, Russian: Perm State of University. dissertation/master’s thesis.
Bogashova, L. G. (2011). Influence of Halogen Processes on the Hydrosphere, Lithosphere, and Biosphere. Geochem. Int. 49, 925–936. doi:10.1134/S0016702911090023
Chaudhry, U. K., Neslihan, Z., Gokce, O., and Gokce, A. F. (2022). Salt Stress and Plant Molecular Responses» in « Plant Defense Mechanisms. London, United Kingdom: IntechOpen. doi:10.5772/intechopen.101513
Classification and diagnostics of Russian Soils, (2004). Classification and Diagnostics of Russian Soils. Smolensk: Oykumena Publ. (In Russian).
Ditz, L. Y., Dudina, T. N., Tsuskman, E. I., and Katunkina, E. V. (2020). Geoecological Problems of Oil Production Territories. Усе (Acnr) 3, 72–77. doi:10.17513/use.37348
Everest, T., and Özcan, H. (2019). Applying Multivariate Statistics for Identification of Groundwater Resources and Qualities in NW Turkey. Environ. Monit. Assess. 191, 47. doi:10.1007/s10661-018-7165-6
Gavrilov, D. V. (1992). Ecological Situation in the Verkhnekamsk Industrial Region in the Late 19th and Early 20th Centuries. Materials of the Republican Scientific Conference “Problems and Trends in the Development of Verkhnekamsk Region: History, Culture, Economy”. Berezniki, 43–44. (In Russian).
Gharaibeh, M. A., Albalasmeh, A. A., Pratt, C., and Hanandeh, A. E. (2021). Estimation of Exchangeable Sodium Percentage from Sodium Adsorption Ratio of Salt-Affected Soils Using Traditional and Dilution Extracts, Saturation Percentage, Electrical Conductivity, and Generalized Regression Neural Networks. Catena. V. 205. doi:10.1016/j.catena.2021.105466
Gost 26483-85 (1985a). Soils. Preparation of Salt Extract and Determination of its РН by CINAO Method.
Gost 26487-85 (1985b). Soils. Determination of Exchangeable Calcium and Exchangeable (mobile) Magnesium by CINAO Methods.
Gost 27395-87 (1987). Soils. Method for Determination of mobile Two-Trivalent Iron Compounds After Verigina-Arinushkina.
Gost 31957-2012 (2012). Water. Methods for Determination of Alkalinity and Mass Concentration of Carbonates and Hydrocarbonates.
Hulisz, P., Kwasowski, W., Pracz, J., and Malinowski, R. (2017). Coastal Acid Sulphate Soils in Poland: a Review. Soil Sci. Annu. 68 (1), 46–54. doi:10.1515/ssa-2017-0006
Hulisz, P., and Piernik, A. (2013). Soil Affected by Soda Industry in Inowrocław» in «Technogenic Soils of Poland. Editors P. Charzyński, P. Hulisz, and R. Bednarek (Toruń): Polish Society of Soil Science), 125–140.
Ivanishchev, V. V. (2019). About the Mechanisms of Plant Resistance to Salt and Specificity of Salinization Influence. Izvestiya Tulgu. Nat. Sci. 4, 76–88. (In Russian).
Kazantseva, M. N., and Svanidze, I. G. (2016). Transformation of forest Phytocenosis in Southern Taiga of West Siberia under the Action of Mineralized Formation Waters. TSU Herald. Nat. Resour. Use Ecol. 2, 71–81. doi:10.21684/2411-7927-2016-2-3-71-81
Kharitonov, T. V. (2015). Alternative Underground Leaching Salts of BSZ. Problems of Mineralogy, Petrography and Metallogeny. Scientific Readings in Memory of P. N. Chirvinsky., 375–382. (In Russian).
Khayrulina, E. A., Novoselova, L. V., Novoselova, L. V., and Poroshina, N. V. (2017). Natural and Anthropogenic Sources of Soluble Salts on the Territory of the Upper Kama Potash deposit. Геогр. Вестн. 1 (40), 93–101. (In Russian). doi:10.17072/2079-7877-2017-1-93-101
Khayrulina, E. A. (2014). Technogenic Transformation of Landscape-Geochemical Processes in the Area of Potassium and Magnesium Salts Mining. Theor. Appl. Ecol. 3, 41–45. (In Russian).
Khayrulina, E., and Maksimovich, N. (2018). Influence of Drainage with High Levels of Water-Soluble Salts on the Environment in the Verhnekamskoe Potash Deposit, Russia. Mine Water Environ. 37, 595–603. doi:10.1007/s10230-017-0509-6
Khudyaev, S. A., and Banaev, E. V. (2012). The Soil and Galogeochemical Conditions of Locations of the Species of the Nitraria (Nitrariaceae) Genus in the Southern Part of the Siberian Region. Contemp. Probl. Ecol. 5, 597–601. doi:10.1134/s199542551206008x
Klopp, H., Arriaga, F. J., and Bleam, W. (2020). Assessment of Soil Response to Exchangeable Sodium Percentage by Consistence and Water Retention Properties. Geoderma 376. doi:10.1016/j.geoderma.2020.114532
Kopylov, I. S. (2014). Geomorphological Landscapes as Basis Geoecological Zoning. Fundam. Res. 11 (10), 2196–2201. Available online: http://fundamental-research.ru/ru/article/view?id=35918 (Accessed on 09, 2021).
Kopylov, I. S., and Konoplev, A. V. (2013). Geological Structure and mineral Resources in the Atlas of Perm Krai. Bull. Perm Univ. Geology. 3 (20), 5–30. (In Russian).
Kulizhskiy, C. P., Rodikova, A. V., and Popova, S. V. (2017). Soil Formation in Contact Zones of Territorial and Aquatic Complexes of Shira Steppe. Vestnik Orenburg State. Univ. 12 (212), 51–54. doi:10.25198/1814-6457-212-51
Lapinskaya, V. O., and Basalay, I. A. (2014). Methods for Reducing Land Salinization in Areas of Potash Development. The Collection of Materials of the Youth Research Seminar "Social Ecology as the Basis for the Greening of Society. Kemerovo. (In Russian).
L.A. Vorobevа (Editor) (2006). Theory and Practice of Chemical Analysis of Soils (Moscow: GEOS), 400. (In Russian).
Lavrinenko, I. A., Lavrinenko, O. V., and Dobrynin, D. V. (2012). Long-term Dynamics and Destruction of Marsh Vegetation in the Kolokolkova Bay of the Barents Sea. Vegetation of Russia 21, 66–77. (In Russian). doi:10.31111/vegrus/2012.21.66
Lepikhin, A. P., and Miroshnichenko, S. A. (2008). Technogenic Impact of the Solikamsk-Bereznikovsky Industrial Hub on Surface Water Bodies. Gornyi Zhurnal 10, 92–96. (In Russian).
L. L. Shishov, and E. I. Pankova (Editors) (2006). Saline Soils of Russia (Moscow: IKC “Akademkniga”). (In Russian).
Maksimovich, N. G., and Pervova, M. S. (2012). Impact of saline Water Flows in Territory of Verkhnekamskoe Potassium-Magnesium Salts deposit on Subsurface Hydrosphere. Eng. Surv. (1), 22–28.
Mitrakova, N. V., and Khayrulina, E. A. (2020). «Formation of saline Technogenic Soils in the Zone of Influence of Potash Enterprises» in «Reflection Bio-, Geo-, Antroposferal Interactions in Soil and Soil Cover». Editor S.P. Kulizhskiy (Tomsk: Publishing House of Tomsk State University), 142–145. (In Russian).
Özcan, H., Cullu, M., Gunal, H., and Ekinci, H. (2018). “Solonchaks and Solonchak-like Soils,” in The Soils of Turkey. World Soils Book Series. Editors S. Kapur, E. Akça, and H. Günal (Cham: Springer), 267–283. doi:10.1007/978-3-319-64392-2_18
Pankova, E. I., and Yamnova, I. A. (1993). About Diagnostics of Solonchaks. Eurasian Soil Sci. 10, 28–38. (In Russian).
Pnd F 14.1: 2: 3.2-95 (1995). Quantitative Chemical Analysis of Waters. Method for Measuring the Mass Concentration of Total Iron in Natural and Wastewaters by the Photometric Method with O-Phenanthrolin.
Pnd F 14.1:2:4.261-10 (2010). Method for Measuring Mass Concentration Dry and Ignition Residue in Drinking, Natural and Waste Water Samples by the Gravimetric Method.
Rodikova, A. V. (2007). Genesis and Properties saline Soils of Khakasia. Tomsk State. Univ. J. Biol. 305, 208–210. (In Russian).
R. Vargas, E. Pankova, S. A. Balyuk, and P. Krasilnikov (Editors) (2017). Handbook for saline Soil Management. Food and Agriculture Organization of the United Nations (Moscow: MSU (FAO)). (In Russian).
Saidov, A. K. (2008). Alkali Soils of Water-Accumulated plains of Western Caspiy and Some Their Genetic Peculiarities (On the Example of Kizlyar Pastures). South Russia: Ecol. Dev. 2, 89–95. (In Russian).
Salama, R. B., Otto, C. J., and Fitzpatrick, R. W. (1999). Contributions of Groundwater Conditions to Soil and Water Salinization. Hydrogeology J. 7, 46–64. doi:10.1007/s100400050179
Shestov, I. N., Shurubor, A. V., and Shilyaeva, Z. A. (1986). Natural Outflows of Salt Waters and Their Role in the Development of Salt Production in the Kama Region. Materials of the Scientific Conference “Salt and the Development of the Region”. Solikamsk, 84–88. (In Russian).
Simonova, J. V., Rusakov, A. V., and Popov, A. I. (2020). Dynamics of Soil Salinization in the Nero Lake Depression (Upper Volga) in Connection with the Latest Climate Change. IOP Conf. Ser. Earth Environ. Sci. 438, 012026. doi:10.1088/1755-1315/438/1/012026
Simonova, J. V., Rusakov, A. V., Rusakov, A. V., and Rjumin, A. G. (2018). Salt-affected Soils of the Rostov Lowland (Yaroslavl' Region): Morphology, Genesis and Dynamics of Salinization in the Annual Hydrological Cycle. Dsb 93, 40–74. doi:10.19047/0136-1694-2018-93-40-74
Simonova, Y. V., Rusakov, A. V., Rusakov, A. V., and Korkina, E. A. (2020). Soil Salinization Processes in the Impact Zone of Mineralized Water Discharge by the Case Study in the Yaroslavl Volga Region, Russia. Vestnik Spbsu. Earth Sci. 65 (4). doi:10.21638/spbu07.2020.404
Sommer, V., Karsten, U., and Glaser, K. (2020). Halophilic Algal Communities in Biological Soil Crusts Isolated from Potash Tailings Pile Areas. Front. Ecol. Evol. 8, 46. doi:10.3389/fevo.2020.00046
Svanidze, I. G., Moiseenko, T. I., Yakimov, A. S., and Soromotin, A. V. (2014). Impact of Tecnogenic Halogenesis on Water Catchment Landscapes of River Valleys and Water Systems: Case Study of the South of Tyumen Province. Water Resour. 41, 105–114. doi:10.1134/s0097807814010114
Tavakkoli, E., Rengasamy, P., and McDonald, G. K. (2010). High Concentrations of Na+ and Cl- Ions in Soil Solution Have Simultaneous Detrimental Effects on Growth of Faba Bean under Salinity Stress. J. Exp. Bot. 61 (15), 4449–4459. doi:10.1093/jxb/erq251
Van Reeuwijk, L. P. (2006). Procedures for Soil Analysis. 7th Edition. Wageningen, Netherlands: ISRIC – World Soil Information. Technical Report 9.
V. N. Kudejarov, and I. V. Ivanov (Editors) (2015). Evolution of Soils and Soil Cover. Theory, Diversity of Natural Evolution and Anthropogenic Soil Transformations (Moscow: GEOS). (In Russian).
Workshop on agrochemistry (2001). Workshop on Agrochemistry. Editor V. G. Mineev (Moscow: Moscow State University Publ.), 689. (In Russian).
World Reference Base for Soil Resources, (2015). World Reference Base for Soil Resources. Rome: Food and agriculture organization of the United Nations.
Xie, X., Pu, L., Zhu, M., Xu, Y., and Wang, X. (2019). Linkage between Soil Salinization Indicators and Physicochemical Properties in a Long-Term Intensive Agricultural Coastal Reclamation Area, Eastern China. J. Soils Sediments 19, 3699–3707. doi:10.1007/s11368-019-02333-3
Yamnova, I. A., and Pankova, E. I. (2013). Gypsic Pedofeatures and Elementary Pedogenetic Processes of Their Formation. Eurasian Soil Sc. 46, 1117–1129. doi:10.1134/S1064229313120089
Yin, X., Feng, Q., Li, Y., Deo, R. C., Liu, W., Zhu, M., et al. (2022). An Interplay of Soil Salinization and Groundwater Degradation Threatening Coexistence of Oasis-Desert Ecosystems. Sci. Total Environ. 806, 150599. doi:10.1016/j.scitotenv.2021.150599
Keywords: solonchak, alluvial soil, brine wells, halophyte, salinization, gleization
Citation: Khayrulina E, Mitrakova N, Poroshina N, Menshikova E and Perminova A (2022) Formation of Solonchak in the Area of the Discharged Ancient Brine Wells (Perm Krai, Russia). Front. Environ. Sci. 10:858742. doi: 10.3389/fenvs.2022.858742
Received: 20 January 2022; Accepted: 21 March 2022;
Published: 28 April 2022.
Edited by:
Christophe Darnault, Clemson University, United StatesReviewed by:
Timuçin Everest, Çanakkale Onsekiz Mart University, TurkeySrinivasan Ramasamy, National Bureau of Soil Survey and Land Use Planning (ICAR), India
Copyright © 2022 Khayrulina, Mitrakova, Poroshina, Menshikova and Perminova. This is an open-access article distributed under the terms of the Creative Commons Attribution License (CC BY). The use, distribution or reproduction in other forums is permitted, provided the original author(s) and the copyright owner(s) are credited and that the original publication in this journal is cited, in accordance with accepted academic practice. No use, distribution or reproduction is permitted which does not comply with these terms.
*Correspondence: Natalya Mitrakova, mitrakovanatalya@mail.ru