- 1Department of Obstetrics, Women’s Hospital, School of Medicine, Zhejiang University, Hangzhou, China
- 2School of Public Health, Sun Yat-sen University, Guangzhou, China
Context: Maternal lipid levels affect birthweight and the long-term health of the offsprings. However, this association could be influenced by genetic and other common factors.
Objective: This work aimed to explore the relationship between maternal lipid levels and birthweight of two pregnancies in the same mother.
Methods: In this population-based cohort study, 705 women and their 1 410 offsprings were included. From an initial sample of women with more than one singleton birth in the database, we made the following exclusions: missing data for pre-pregnancy BMI, pregnancy weight gain, birthweight and lipid values; maternal age less than 19 or older than 44 years old; gestational age < 37 weeks or > 41weeks, gestational diabetes mellitus/diabetic. In the second and third trimesters, serum samples were collected for the determination of fasting total cholesterol (TC), triglycerides (TG), high-density lipoprotein cholesterol (HDL-C), and low-density lipoprotein cholesterol (LDL-C) levels. Then we assessed the association between maternal lipids and birthweight.
Results: Infants of women whose 2nd-trimester TC increased by 10th-20th percentile (-0.92~-0.56 mmol/L) from 1st to 2nd pregnancy were 239.69 (62.32~417.06) g lighter at birth than were infants of women those of 40th-50th percentile (-0.20~-0.03 mmol/L). Parity, gestational age, neonatal gender, maternal pre-pregnancy body mass index, maternal weight gain, and 3rd-trimester TC and HDL-C were all associated with higher birth weight. Every unit increase in TC in the third trimester increases birthweight by 53.13 (14.32 ~91.94) g.
Conclusion: Maternal TC level is associated with birthweight independent of shared genes. TC may be used to guide diet and predict birthweight combined with ultrasound and other indicators.
Introduction
Association between birthweight and fetal health in the short- and long-term has been confirmed. This fetal origin of adult disease was first proposed by Baker and colleagues (1) and has been confirmed by a large number of human studies. For instance, too low or too high infant birthweight both increase the risk for metabolic diseases in adulthood including obesity, hypertension, cardiovascular diseases, type 2 diabetes mellitus, insulin resistance and hyperlipidemia (2, 3). With the onset of the epidemic of metabolic diseases in offsprings, many experiments on animals have confirmed that maternal lipid metabolism disorders led to metabolic related diseases in offsprings (4, 5). Research also demonstrated that the effects of perinatal dyslipidemia would persist after birth (6).
During the normal gestational period, the level of blood lipid profiles including TG, TC, LDL-C and HDL-C gradually increase from the 12th week of gestation and this increasing trend continues to the third trimester (7–9). These changes in lipids are needed to maintain the growth and development of the fetus. The two main changes in fat metabolism during pregnancy are fat accumulation and hyperlipidemia (4). Maternal TG levels in late pregnancy of women diagnosed with gestational diabetes mellitus (GDM) are found to have a significant positive correlation with neonatal birthweight, fat mass and body mass index (BMI) (10–12). One previous study in China showed that high TG and low HDL-C values in late pregnancy could be considered as predictors of a high risk of large for gestational age (LGA) and macrosomia, regardless of infant gender, which was coincident with our results (13). A study including women with normal glucose tolerance discovered that pre-pregnancy BMI and maternal TG values in the last trimester were associated with newborn birthweight independently (14). In a prospective population-based cohort study, higher maternal TG levels were positively correlated with higher birthweight and that was found in normal weight women only (15). It remains controversial whether the association between maternal lipid levels and newborn birthweight appears only in pregnant women with normal glucose or also in GDM/diabetic pregnancies Our previous study excluded pregnancies with relevant complications like GDM, diabetes, pre-eclampsia(PE) and concluded that high HDL-C levels during the third trimester were significantly associated with small for gestational age (SGA), independent of gestational weight gain (16).
Direct association between maternal lipid profiles during pregnancy and birthweight have generally been shown in previous studies. However, interindividual comparisons conducted in these observational studies have a major characteristic limitation from genetic and environmental confoundings. In a large population-based cohort study published in Lancet (17) examined how differences in weight gain during two or more pregnancies for the same mother predicted the birthweight of her offspring. Therefore in our study, we aimed to explore the association between maternal lipids and birthweight by comparing outcomes from two pregnancies of each woman. This within-family research was designed to reduce genetic confounding and other potential individual factors.
Materials and methods
Study design and population
Data for this retrospective study were from natality records covering all births in Women’s Hospital, Zhejiang University School of Medicine from 1 Jan 2014 and 31 Dec 2020. Records of birth outcomes, maternal characteristics and prenatal healthcare are available from the hospital information system. Children born to the same mother were recorded under the unique patient’s identification number and we have checked the fathers’ identification number to ensure children born to the same father.
We made the following exclusions from an initial sample of singleton births: missing data for pre-pregnancy BMI, pregnancy weight gain, birthweight and lipid values; maternal age less than 19 or older than 44 years old; gestational age < 37 weeks or > 41weeks; maternal diabetes; inherited metabolic diseases before pregnancy; malignant tumor and chromosomal abnormalities; consumed alcohol or drugs, used tobacco and systemic infection during pregnancy that may influence lipid metabolism; birthweight > 7000g or < 500g (extreme values that could come from data export error). The study was conducted under an approval from the hospital’s Clinical Research Ethics Committee.
Data collection
All the data of birth outcomes, maternal characteristics and lipid values were obtained from the hospital information system of Women’s Hospital, Zhejiang University School of Medicine. The variables included were: gravidity, parity, maternal age, husband age, gestational age, education background, pre-pregnancy weight, height, pregnancy weight gain, child gender, and lipid values in the second and third trimester. Data for birth outcomes and maternal characteristics were recorded by midwives and checked with obstetricians, which made it highly reliable. Maternal pre-pregnancy BMI was calculated from pre-pregnancy height and weight, and categorized into overweight (≥25.0 kg/m2), normal weight (18.5-24.9 kg/m2), and underweight (<18.5 kg/m2) groups on the basis of the World Health Organization BMI classification (18).
Mothers retained in the sample were all maintained regular prenatal healthcare in our hospital and venous blood samples were taken from all the mothers after eight hours fasting at the second (24-26 gestational weeks) and third (30–32 gestational weeks) trimester of pregnancy. All the blood samples were assayed for LDL-C, HDL-C, TG and TC concentrations. An automatic biochemical analyzer was used to perform the measurements and the specific methods had been described in our previous study (16).
Statistical analysis
We expected that the maternal TC level is positively associated with birthweight and HDL-C level has a negatively relationship with birthweight after adjustment for potential confounders as reported previously. Our inclusion and exclusion criteria were set up to maximum eliminate some sources of potential confounding, like gestational age, maternal diabetes, and extremes in birth weight. For residual confounding by measured (smoking) and unmeasured (shared genetic and environmental) covariates, we adopted the strategy of comparing two pregnancies in the same mother and including measured covariates in the statistical models. Thus, we kept the effects of differences between individuals to a minimum.
In the mixed-effects model, we take ID number of pregnant women as random effects terms, and maternal age, pre-pregnancy BMI, gestational weight gain, gestational age at birth, parity, infant gender, the interval of two pregnancies were regarded as fixed effects terms.
Linear regression analysis was applied to examine the associations between changes in maternal lipid values and changes in birthweight between two pregnancies for each mother. First, we classified the changes of maternal lipid values (TC, TG, HDL-C, LDL-C) by percentiles, from the minimum value (min) to maximum value (max). We then regressed changes in birthweight (continuous variable) on categories of changes in maternal serum levels (min-P10, P10-20, and so on, until P80-90, P90-max). In the multivariable adjusted model, maternal age, parity, gestational weight gain, pre-pregnancy BMI, gestational age at birth, infant gender, the interval of two pregnancies and the difference in gestational age were regarded as confounding variables. The variable values refer to the values of the second observed pregnancy minus the value of the first observed pregnancy for each mother.
All statistical analyses were conducted using the R software, version 4.0.1 for Windows (The R Project; https://www.r-project.org). P values < 0.05 were considered statistically significant. All data were presented as means and SDs for maternal cohort characteristics or 95% CIs for outcome data.
Results
From an initial sample size of 2144 singleton births from 1072 mothers who had two records of birth from 1 Jan 2014 and 31 Dec 2020, exclusions were as follows: gestational diabetes mellitus (GDM)/diabetic (440births), gestational age < 37 weeks or >41 weeks (168 births); missing data for pre-pregnancy BMI, pregnancy weight gain, birthweight and lipid values (102 births); maternal age less than 19 or older than 44 years old (24 births); birthweight < 500g or > 7000g (0 birth). Finally, the study population was consisted of 1 410 singleton births of 705 women. No malignant tumor, chromosomal abnormalities, or metabolic diseases before pregnancy were recorded. And no one experienced serious infection, used tobacco, or consumed alcohol or drugs.
Table 1 showed descriptive characteristics of mothers and infants in each pregnancy. All newborns included in our analysis had a mean (SD) birth weight of 3405 (407.50) g, and the gestational age at birth was 39.19 (1.12) weeks. In addition, 691 (49%) infants were boys. For maternal lipid values, we can see that TG in the second trimester and HDL-C values in the second and third trimesters have significant differences. Figure 1 shows the distribution of maternal lipid values in the second trimester and birthweight of the first and second pregnancy.
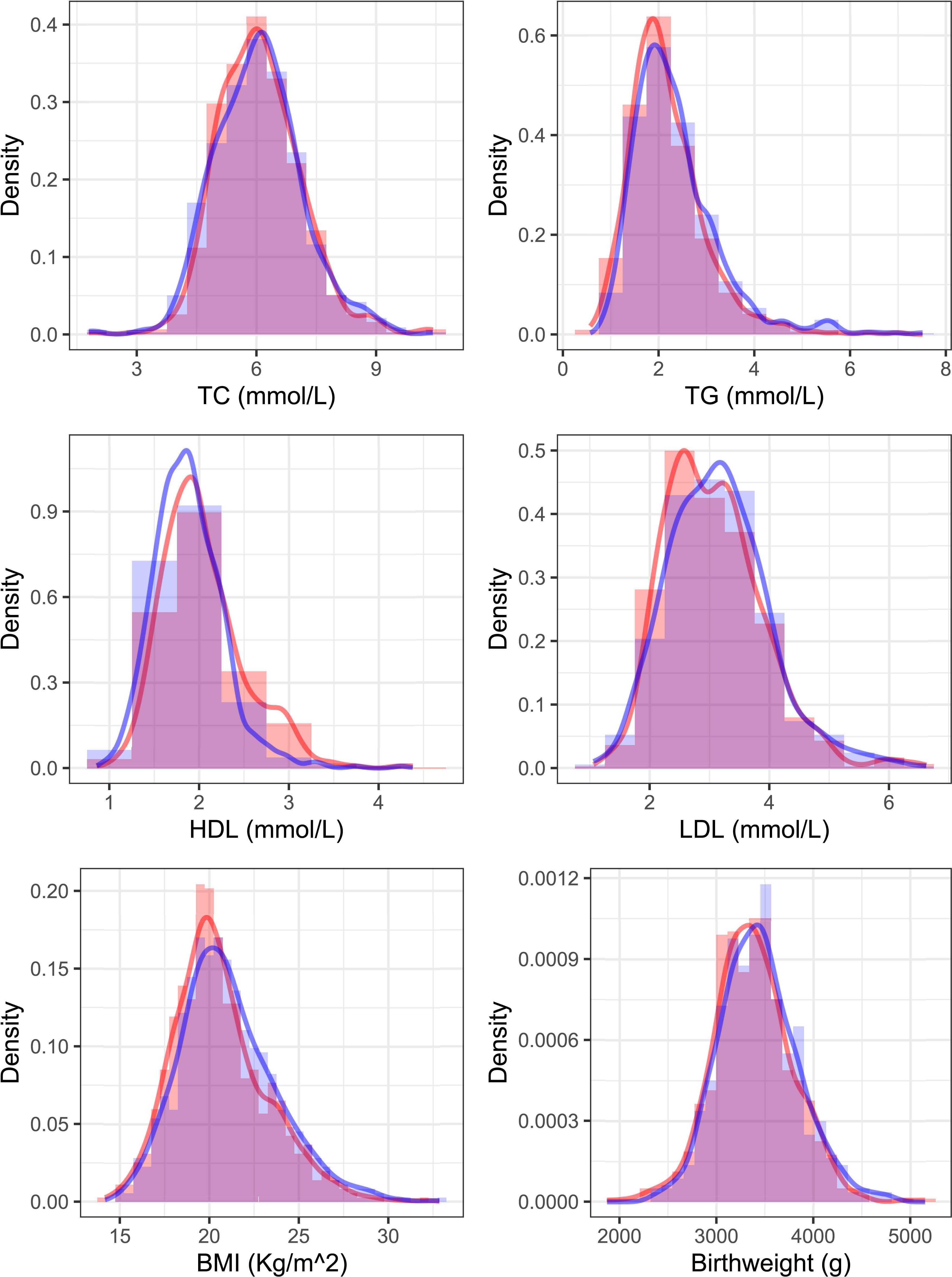
Figure 1 Distribution of 2nd-trimester serum lipids and birthweight of the first and second pregnancy. The red color shows the distribution curve in an older sibling and blue shows the next youngest sibling.
Table 2, using a mixed-effects model, showed the adjusted associations between maternal factors, lipid values and difference in birthweight. Gestational age, parity, neonatal gender, maternal pre-pregnancy BMI, maternal weight gain, and third-trimester TC were all positively related to higher birthweight. Third-trimester HDL-C was negatively associated with birthweight. Every unit increase in TC in the third trimester increases birthweight by 53.13 (14.32 ~91.94) g.
Linear regression models involving maternal lipid values as a categorical variable were analyzed and only one significant result was found (shown in Table 3). The 10th -20th percentiles of the difference in 2nd-trimester TC were statistically significant compared with the 40th to 50th percentile. Infants of women whose 2nd-trimester TC increased by 10th-20th percentile (-0.92~-0.56 mmol/L) from 1st to 2nd pregnancy were 239.69 (62.32~ 417.06) g lighter at birth than were infants of women those of 40th-50th percentile (-0.20~-0.03 mmol/L). Figure 2 plots coefficient estimates from the linear regression model (Table 3).
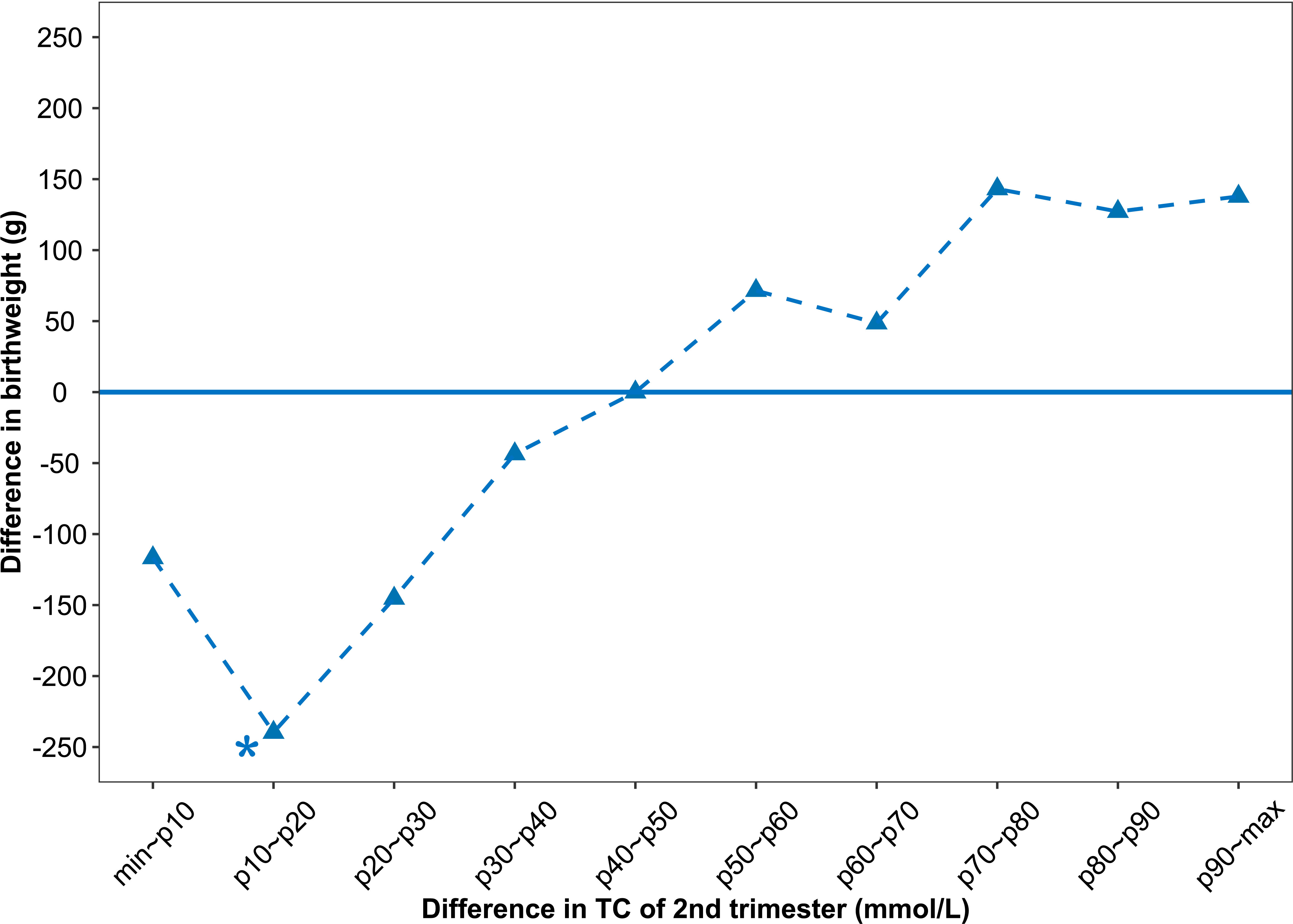
Figure 2 Associations between difference in 2nd-trimester TC and difference in birthweight. The difference in birthweight is relative to the reference group (infants of mothers whose 2nd-trimester TC increased by 40th-50th percentile (-0.20 ~ -0.03 mmol/L). The variable values refer to the values of the second observed pregnancy minus the value of the first observed pregnancy for each mother.
Discussion
Maternal lipid profiles have been associated with birthweight and adverse pregnancy outcomes. Our study, using singleton births from population-based data, provides evidence for a causal association that is independent of shared genes. We confirmed that the difference in TC in the second trimester was positively associated with the difference in birthweight in the two pregnancies of the same mother. We also noted that every unit increase in TC in the third trimester increases birthweight by about 50g. According to the literature available so far, this is the first study to show an independent association between maternal TC levels and birthweight using a within-family design to minimize confounding. In addition, we found that the 2nd trimester TG value significantly increased and HDL-C value significantly decreased in the second pregnancy, and the corresponding neonatal birthweight significantly increased. Because birthweight predicts adult BMI (19–23), our study suggests that maternal dyslipidemia could program the fetus for an increased long-term risk of obesity-related diseases.
According to existing research, the relationship of maternal lipids and birthweight has been studied among different countries and races. In a recently published large-scale birth cohort study of Japanese mothers with singleton birth at term, maternal TC levels in midpregnancy, independent of other potential confoundings including prenatal weight gain and pre-pregnancy BMI, are significantly correlated with the birth of SGA or LGA (24). In rural India, undernourished pregnant women with normal glucose, neonatal birthweight was significantly associated with maternal blood glucose levels and lipid concentrations at 18 and 28 weeks. Similarly, linear correlation was found between maternal TC and birth size (25). One cohort study in the United State reported that low maternal TC in midpregnancy was associated with SGA (26). Our study is in line with the above studies and further confirms these findings independent of shared genes and environmental facts. The results may provide evidence for further research and potential mechanism.
In early pregnancy, maternal cholesterol is fundamental to hormonal and physical changes (27–34) and is associated with the development of both embryonic and placental tissues. Circulating cholesterol is transported to the embryo and could affect the placenta transport function by changing the placental cholesterol concentrations (35). As gestation progresses, maternal total cholesterol levels gradually increase and this physiologic hypercholesterolemia especially comes up in the second and third trimesters (36–39), which maintain pregnancy and fetal growth. Maternal TG, which cannot cross the placenta, is hydrolyzed to fatty acids by placental lipases and then taken up by placental trophoblasts, where they can be metabolized or transported to fetal circulation (40, 41). These fatty acids are thought to be substrates for fetal adipogenesis or provide the energy needed for fetal growth (42, 43). The fetus can derive lipid from both endogenous and exogenous pathways. The fetus can synthesize lipid through their own metabolism, which called the endogenous pathway. And lipid obtained from the yolk sac or placenta is called the exogenous pathway. Any factors affecting these two pathways of lipid access could cause abnormal fat accumulation within the fetus (27). Too high or low fat intake can both affect fetal growth and even adipose tissue development, adipocyte differentiation in offspring, which have been shown in previous animal studies (44, 45). This mechanism may confirm the “fetal origin hypothesis” and an increased risk of cardiovascular disease in the adult (46–48). In addition, decreased or increased maternal TC levels have been reported to be associated with abnormal birthweight and adverse pregnancy complications. In our study, the difference in TC levels between two pregnancies of the same pregnant woman was positively correlated with the difference in newborn birthweight, which is worthy of further exploring the related factors affecting birthweight in the metabolism pathway.
One interesting finding of our study was that maternal pre-pregnancy BMI and birthweight were significantly increased in the second pregnancy. A recent study showed that a higher maternal pre-pregnancy BMI was associated with altered maternal early-pregnancy nonesterified fatty acids (49). In the second pregnancy, the TG value was significantly increased and the HDL-C value was significantly decreased, which may declare a higher risk of dyslipidemia. Diet and lifestyle may cause these changes and influence the birthweight and metabolism of offsprings. As reported, metabolic disturbance of maternal TG and non-esterified fatty acid were correlated with excessive birthweight as Herrera et al. (4, 30). The Amsterdam Born Children and their Development cohort study focused on the association between early pregnancy maternal TG concentrations and adverse pregnancy outcomes including LGA, preterm birth, pregnancy-induced hypertension, and PE and discovered they were linearly correlated (50). This also provides a basis for predicting birthweight.
The highlight of our research is the providing of attractive evidence regarding the association of maternal TC concentrations and birthweight within the same mother. The interaction between glucose and lipid metabolism during pregnancy is still controversial. No within-family studies have been conducted investigating the association between maternal lipid and birthweight before, and less attention has been paid to TC levels. Our study design excluded more confounding factors and provides a good complement to existing research. Some guidelines have proposed the importance of prepregnancy lipid screening, but an unified standard is still lacking. Our study can provide a basis for the formulation of the reference range of blood lipid during pregnancy. In routine clinical practice, dietary interventions/daily activities can be managed by maternal lipid levels.
However, there are still some limitations. The primary limitation of the study was that it was a single-center cohort study and our sample size was not large enough. Further research should expand the sample size and conduct a multi-center and multi-region prospective investigation. There is an outlier and missing data in lipid values, we think this confounding would also be decreased by enlarging the sample size. Additionally, only second and third-trimester lipid values were included in our study. Maternal lipids concentrations before pregnancy and through the whole pregnancy should better to be collected. Thus, we can move the point of intervention forward and establish a prediction model. Our within-family design has effectively eliminated confounding by genetic and other relevant factors especially environmental factors. In addition, the information that could be cobfoundings about the difference in physical activities during the two pregnancies and family history of diabetes was not obtained Furthermore, there is no measure of neonatal body fatness so the authors are unable to say whether the difference in lipids between pregnancies influences somatic growth or adipose tissue deposition in the offspring.
In conclusion, the difference in third trimester TC was positively associated with the difference of birthweight in the two pregnancies of the same mother independent of other potential confounding factors, mainly for prenatal weight gain and pre-pregnancy BMI. Every unit increase in TC in the third trimester increases birthweight by 53.13 (14.32 ~91.94) g. Given the apparent associations, TC may be used to guide diet and predict birthweight combined with ultrasound and other indicators. Further studies can explore optimal maternal serum cholesterol levels during pregnancy to prevent adverse pregnancy outcomes and adult obesity related diseases.
Data availability statement
The raw data supporting the conclusions of this article will be made available by the authors, without undue reservation.
Ethics statement
Written informed consent was obtained from the individual(s) for the publication of any potentially identifiable images or data included in this article.
Author contributions
QL and HH designed the study. QC was involved in the study design. MW and LQ enrolled the patients. HC analysed the data. QC and HC wrote the article. All other authors – FX, YJ, and ML critically reviewed and approved the final manuscript.
Funding
This work was supported by Scientific Research Foundation of the National Health Commission (WKJ-ZJ-2126), National Key Research and Development Program (2021YFC2700700), Key Laboratory of Gamete and Reproductive Tract Abnormalities, National Health Commission(NHC-2020-2).
Acknowledgments
The authors thank the Staff at the women’s hospital, Zhejiang University for technical assistance and facility support. We are also grateful to all the mothers and newborns that participated in the study and thank all the midwives and obstetricians who carefully gathered and recorded data.
Conflict of interest
The authors declare that the research was conducted in the absence of any commercial or financial relationships that could be construed as a potential conflict of interest.
Publisher’s note
All claims expressed in this article are solely those of the authors and do not necessarily represent those of their affiliated organizations, or those of the publisher, the editors and the reviewers. Any product that may be evaluated in this article, or claim that may be made by its manufacturer, is not guaranteed or endorsed by the publisher.
References
1. Hales CN, Barker DJ. Type 2 (non-insulin-dependent) diabetes mellitus: the thrifty phenotype hypothesis. Diabetologia (1992) 35(7):595–601. doi: 10.1007/BF00400248
2. Pallotto EK, Kilbride HW. Perinatal outcome and later implications of intrauterine growth restriction. Clin Obstet Gynecol (2006) 49(2):257–69. doi: 10.1097/00003081-200606000-00008
3. Barker DJ. Adult consequences of fetal growth restriction. Clin Obstet Gynecol (2006) 49(2):270–83. doi: 10.1097/00003081-200606000-00009
4. Herrera E, Ortega-Senovilla H. Lipid metabolism during pregnancy and its implications for fetal growth. Curr Pharm Biotechnol (2014) 15(1):24–31. doi: 10.2174/1389201015666140330192345
5. Smith CJ, Ryckman KK. Epigenetic and developmental influences on the risk of obesity, diabetes, and metabolic syndrome. Diabetes Metab Syndr Obes (2015) 8:295–302. doi: 10.2147/DMSO.S61296
6. Frantz E, Menezes HS, Lange KC, Abegg MP, Correa CA, Zangalli L, et al. The effect of maternal hypercholesterolemia on the placenta and fetal arteries in rabbits. Acta Cir Bras (2012) 27(1):7–12. doi: 10.1590/S0102-86502012000100002
7. Lippi G, Albiero A, Montagnana M, Salvagno GL, Scevarolli S, Franchi M, et al. Lipid and lipoprotein profile in physiological pregnancy. Clin Lab (2007) 53(3-4):173–7.
8. Husain F, Latif S, Uddin M, Nessa A. Lipid profile changes in second trimester of pregnancy. Mymensingh Med J (2008) 17(1):17–21.
9. Ghio A, Bertolotto A, Resi V, Volpe L, Di Cianni G. Triglyceride metabolism in pregnancy. Adv Clin Chem (2011) 55:133–53. doi: 10.1016/B978-0-12-387042-1.00007-1
10. Herrera E, Ortega-Senovilla H. Disturbances in lipid metabolism in diabetic pregnancy - are these the cause of the problem? Best Pract Res Clin Endocrinol Metab (2010) 24(4):515–25. doi: 10.1016/j.beem.2010.05.006
11. Schaefer-Graf UM, Graf K, Kulbacka I, Kjos SL, Dudenhausen J, Vetter K, et al. Maternal lipids as strong determinants of fetal environment and growth in pregnancies with gestational diabetes mellitus. Diabetes Care (2008) 31(9):1858–63. doi: 10.2337/dc08-0039
12. Ortega-Senovilla H, Schaefer-Graf U, Meitzner K, Abou-Dakn M, Herrera E. Decreased concentrations of the lipoprotein lipase inhibitor angiopoietin-like protein 4 and increased serum triacylglycerol are associated with increased neonatal fat mass in pregnant women with gestational diabetes mellitus. J Clin Endocrinol Metab (2013) 98(8):3430–7. doi: 10.1210/jc.2013-1614
13. Zhang G, Zeng G, Liang X-Z, Huang C. Increasing heat risk in china’s urban agglomerations. Environ Res Lett (2021) 16(6):64073.
14. Di Cianni G, Miccoli R, Volpe L, Lencioni C, Ghio A, Giovannitti MG, et al. Maternal triglyceride levels and newborn weight in pregnant women with normal glucose tolerance. Diabetes Med (2005) 22(1):21–5. doi: 10.1111/j.1464-5491.2004.01336.x
15. Misra VK, Trudeau S, Perni U. Maternal serum lipids during pregnancy and infant birth weight: the influence of prepregnancy BMI. Obes (Silver Spring) (2011) 19(7):1476–81. doi: 10.1038/oby.2011.43
16. Chen Q, Chen H, Xi F, Sagnelli M, Zhao B, Chen Y, et al. Association between maternal blood lipids levels during pregnancy and risk of small-for-gestational-age infants. Sci Rep (2020) 10(1):19865. doi: 10.1038/s41598-020-76845-1
17. Ludwig DS, Currie J. The association between pregnancy weight gain and birthweight: a within-family comparison. Lancet (2010) 376(9745):984–90. doi: 10.1016/S0140-6736(10)60751-9
18. Obesity: preventing and managing the global epidemic. report of a WHO consultation. World Health Organ Tech Rep Ser (2000) 894:1–253.
19. Warrington NM, Beaumont RN, Horikoshi M, Day FR, Helgeland Ø, Laurin C, et al. Maternal and fetal genetic effects on birth weight and their relevance to cardio-metabolic risk factors. Nat Genet (2019) 51(5):804–14. doi: 10.1038/s41588-019-0403-1
20. Jensen BW, Meyle KD, Madsen K, Sørensen TIA, Baker JL. Early life body size in relation to risk of renal cell carcinoma in adulthood: a Danish observational cohort study. Eur J Epidemiol (2020) 35(3):251–8. doi: 10.1007/s10654-020-00605-8
21. Araújo CL, Hallal PC, Nader GA, Neutzling MB, deFátima Vieira M, Menezes AM, et al. Effect of birth size and proportionality on BMI and skinfold thickness in early adolescence: prospective birth cohort study. Eur J Clin Nutr (2009) 63(5):634–9. doi: 10.1038/ejcn.2008.20
22. Gillman MW, Rifas-Shiman S, Berkey CS, Field AE, Colditz GA. Maternal gestational diabetes, birth weight, and adolescent obesity. Pediatrics (2003) 111(3):e221–6. doi: 10.1542/peds.111.3.e221
23. Wei JN, Li HY, Sung FC, Lin CC, Chiang CC, Li CY, et al. Birth weight correlates differently with cardiovascular risk factors in youth. Obes (Silver Spring) (2007) 15(6):1609–16. doi: 10.1038/oby.2007.190
24. Kaneko K, Ito Y, Ebara T, Kato S, Matsuki T, Tamada H, et al. Association of maternal total cholesterol with SGA or LGA birth at term: the Japan environment and children's study. J Clin Endocrinol Metab (2022) 107(1):e118–e29. doi: 10.1210/clinem/dgab618
25. Kulkarni SR, Kumaran K, Rao SR, Chougule SD, Deokar TM, Bhalerao AJ, et al. Maternal lipids are as important as glucose for fetal growth: findings from the pune maternal nutrition study. Diabetes Care (2013) 36(9):2706–13. doi: 10.2337/dc12-2445
26. Edison RJ, Berg K, Remaley A, Kelley R, Rotimi C, Stevenson RE, et al. Adverse birth outcome among mothers with low serum cholesterol. Pediatrics (2007) 120(4):723–33. doi: 10.1542/peds.2006-1939
27. Woollett LA. The origins and roles of cholesterol and fatty acids in the fetus. Curr Opin Lipidol (2001) 12(3):305–12. doi: 10.1097/00041433-200106000-00010
28. Petraglia F, Santuz M, Florio P, Simoncini T, Luisi S, Plaino L, et al. Paracrine regulation of human placenta: control of hormonogenesis. J Reprod Immunol (1998) 39(1-2):221–33. doi: 10.1016/S0165-0378(98)00024-2
29. Miller WL. Steroid hormone biosynthesis and actions in the materno-feto-placental unit. Clin Perinatol (1998) 25(4):799–817. doi: 10.1016/S0095-5108(18)30084-8
30. Herrera E. Lipid metabolism in pregnancy and its consequences in the fetus and newborn. Endocrine (2002) 19(1):43–55. doi: 10.1385/ENDO:19:1:43
31. Beachy PA, Cooper MK, Young KE, von Kessler DP, Park WJ, Hall TM, et al. Multiple roles of cholesterol in hedgehog protein biogenesis and signaling. Cold Spring Harb Symp Quant Biol (1997) 62:191–204. doi: 10.1101/SQB.1997.062.01.025
32. Mauch DH, Nägler K, Schumacher S, Göritz C, Müller EC, Otto A, et al. CNS synaptogenesis promoted by glia-derived cholesterol. Science (2001) 294(5545):1354–7. doi: 10.1126/science.294.5545.1354
33. Kelley RI, Herman GE. Inborn errors of sterol biosynthesis. Annu Rev Genomics Hum Genet (2001) 2:299–341. doi: 10.1146/annurev.genom.2.1.299
34. Goto M, Brickwood S, Wilson DI, Wood PJ, Mason JI, Hanley NA. Steroidogenic enzyme expression within the adrenal cortex during early human gestation. Endocr Res (2002) 28(4):641–5. doi: 10.1081/ERC-120016979
35. Sen A, Ghosh PK, Mukherjea M. Changes in lipid composition and fluidity of human placental basal membrane and modulation of bilayer protein functions with progress of gestation. Mol Cell Biochem (1998) 187(1-2):183–90. doi: 10.1023/A:1006839711587
36. Winkler K, Wetzka B, Hoffmann MM, Friedrich I, Kinner M, Baumstark MW, et al. Low density lipoprotein (LDL) subfractions during pregnancy: accumulation of buoyant LDL with advancing gestation. J Clin Endocrinol Metab (2000) 85(12):4543–50. doi: 10.1210/jcem.85.12.7027
37. Alvarez JJ, Montelongo A, Iglesias A, Lasunción MA, Herrera E. Longitudinal study on lipoprotein profile, high density lipoprotein subclass, and postheparin lipases during gestation in women. J Lipid Res (1996) 37(2):299–308. doi: 10.1016/S0022-2275(20)37617-3
38. Brizzi P, Tonolo G, Esposito F, Puddu L, Dessole S, Maioli M, et al. Lipoprotein metabolism during normal pregnancy. Am J Obstet Gynecol (1999) 181(2):430–4. doi: 10.1016/S0002-9378(99)70574-0
39. Qureshi IA, Xi XR, Limbu YR, Bin HY, Chen MI. Hyperlipidaemia during normal pregnancy, parturition and lactation. Ann Acad Med Singap (1999) 28(2):217–21.
40. Larqué E, Demmelmair H, Gil-Sánchez A, Prieto-Sánchez MT, Blanco JE, Pagán A, et al. Placental transfer of fatty acids and fetal implications. Am J Clin Nutr (2011) 94(6 Suppl):1908s–13s. doi: 10.3945/ajcn.110.001230
41. Brett KE, Ferraro ZM, Yockell-Lelievre J, Gruslin A, Adamo KB. Maternal-fetal nutrient transport in pregnancy pathologies: the role of the placenta. Int J Mol Sci (2014) 15(9):16153–85. doi: 10.3390/ijms150916153
42. Szabo AJ. Transferred maternal fatty acids stimulate fetal adipogenesis and lead to neonatal and adult obesity. Med Hypotheses (2019) 122:82–8. doi: 10.1016/j.mehy.2018.10.022
43. Larqué E, Pagán A, Prieto MT, Blanco JE, Gil-Sánchez A, Zornoza-Moreno M, et al. Placental fatty acid transfer: a key factor in fetal growth. Ann Nutr Metab (2014) 64(3-4):247–53. doi: 10.1159/000365028
44. Llopis M, Sánchez J, Priego T, Palou A, Picó C. Maternal fat supplementation during late pregnancy and lactation influences the development of hepatic steatosis in offspring depending on the fat source. J Agric Food Chem (2014) 62(7):1590–601. doi: 10.1021/jf405161e
45. Elahi MM, Cagampang FR, Mukhtar D, Anthony FW, Ohri SK, Hanson MA. Long-term maternal high-fat feeding from weaning through pregnancy and lactation predisposes offspring to hypertension, raised plasma lipids and fatty liver in mice. Br J Nutr (2009) 102(4):514–9. doi: 10.1017/S000711450820749X
46. Napoli C, Glass CK, Witztum JL, Deutsch R, D'Armiento FP, Palinski W. Influence of maternal hypercholesterolaemia during pregnancy on progression of early atherosclerotic lesions in childhood: Fate of early lesions in children (FELIC) study. Lancet (1999) 354(9186):1234–41. doi: 10.1016/S0140-6736(99)02131-5
48. Skilton MR, Evans N, Griffiths KA, Harmer JA, Celermajer DS. Aortic wall thickness in newborns with intrauterine growth restriction. Lancet (2005) 365(9469):1484–6. doi: 10.1016/S0140-6736(05)66419-7
49. Wahab RJ, Jaddoe VWV, Voerman E, Ruijter GJG, Felix JF, Marchioro L, et al. Maternal body mass index, early-pregnancy metabolite profile, and birthweight. J Clin Endocrinol Metab (2022) 107(1):e315–e27. doi: 10.1210/clinem/dgab596
Keywords: maternal lipid, body mass index, total cholesterol, birthweight, within-family comparison
Citation: Chen Q, Chen H, Wang M, Qiu L, Xi F, Jiang Y, Lv M, Huang H-F and Luo Q (2022) The association between alteration of maternal lipid levels and birthweight at term: A within-family comparison. Front. Endocrinol. 13:989663. doi: 10.3389/fendo.2022.989663
Received: 08 July 2022; Accepted: 16 September 2022;
Published: 30 September 2022.
Edited by:
Malgorzata Wojcik, Jagiellonian University Medical College, PolandReviewed by:
Malgorzata Waluś-Miarka, Jagiellonian University Medical College, PolandWei Zheng, Capital Medical University, China
Copyright © 2022 Chen, Chen, Wang, Qiu, Xi, Jiang, Lv, Huang and Luo. This is an open-access article distributed under the terms of the Creative Commons Attribution License (CC BY). The use, distribution or reproduction in other forums is permitted, provided the original author(s) and the copyright owner(s) are credited and that the original publication in this journal is cited, in accordance with accepted academic practice. No use, distribution or reproduction is permitted which does not comply with these terms.
*Correspondence: Qiong Luo, luoq@zju.edu.cn; He-Feng Huang, huanghefg@hotmail.com
†These authors have contributed equally to this work and share first authorship
‡ORCID: Qiong Luo, orcid.org/0000-0002-9541-9781
Hefeng Huang, orcid.org/0000-0002-0195-985X