- 1The 2nd Affiliated Hospital of Guangzhou University of Chinese Medicine, Guangzhou, China
- 2The Second Clinical Medical College of Guangzhou University of Chinese Medicine, Guangzhou, China
- 3School of Pharmaceutical Sciences, Guangzhou University of Chinese Medicine, Guangzhou, China
Purpose: To explore the molecular mechanism of luteolin in the treatment of osteoporosis (OP) by network pharmacological prediction and experimentation.
Methods: The target proteins of luteolin were obtained with the Traditional Chinese Medicine Systems Pharmacology Database and Analysis Platform (TCMSP). OP-related proteins were extracted from the Comparative Toxicogenomics Database (CTD) and GeneCards and DisGeNET databases. We imported the common protein targets of luteolin and OP into the STRING database to obtain the relationships between the targets. The common target proteins of luteolin and OP were assessed by KEGG and GO enrichment analyses with the DAVID database. Animal experiments were conducted to verify the effect of luteolin on bone mineral density in ovariectomised (OVX) rats. Finally, the effects of luteolin on key signalling pathways were verified by cell experiments in vitro.
Results: Forty-four targets of luteolin involved in the treatment of OP, including key target proteins such as TP53, AKT1, HSP90AA1, JUN, RELA, CASP3, and MAPK1, were screened. KEGG enrichment analysis found that luteolin inhibits OP by regulating the PI3K-Akt, TNF, oestrogen and p53 signalling pathways. The results of animal experiments showed that bone mass in the low-dose luteolin group (Luteolin-L group, 10 mg/kg), high-dose luteolin group (Luteolin-H group, 50 mg/kg) and positive drug group was significantly higher than that in the OVX group (P<0.05). Western blot (WB) analysis showed that the protein expression levels of Collagen I, Osteopontin and RUNX2 in bone marrow mesenchymal stem cells (BMSCs) cultured with 0.5, 1 and 5 μM luteolin for 48 h were significantly higher than those in the dimethyl sulfoxide (DMSO) group (P<0.05). In vitro cell experiments showed that the p-PI3K/PI3K and p-Akt/Akt expression ratios in BMSCs cultured with 0.5, 1 and 5 μM luteolin for 48 h were also significantly higher than those in the DMSO group (P<0.05).
Conclusions: Luteolin has multitarget and multichannel effects in the treatment of OP. Luteolin could reduce bone loss in OVX rats, which may be due to its ability to promote the osteogenic differentiation of BMSCs by regulating the activity of the PI3K-Akt signalling pathway.
Introduction
Osteoporosis (OP) is a systemic bone disease involving decreased bone density and bone quality induced by human ageing, menopause and other factors, and the main characteristics of this disease are the destruction of bone microstructure and an increase in bone brittleness (1, 2). With the ageing of the global population, OP and its complications will become a major public global health problem (3, 4). It is predicted that by 2050, the medical expenses for OP-related fractures in China will reach 25.4 billion USD, nearly 30 times greater than those in 2010 (5). At present, OP is mainly treated by drug therapy, physical therapy and exercise therapy (6, 7). Although great progress has been made in the treatment of OP, therapeutic effects still do not meet clinical expectations. First, many newly developed anti-OP drugs are expensive (8), limiting their wide clinical application; in addition, adverse drug reactions (9) eventually affect patient drug compliance.
In the theoretical traditional Chinese medicine system, OP is called “Gu Wei”, which refers to osteopenia caused by kidney deficiency. Traditional Chinese medicine has a long history of using herbal medicines to tonify the kidney and replenish Qi for the prevention and treatment of “Gu Wei”. In the application of herbal traditional Chinese medicines, many kidney-tonifying herbs contain flavonoids or flavonoid monomers (10). Luteolin is a natural flavonoid found in various medicinal materials, vegetables and fruits, such as honeysuckle, Schizonepeta tenuifolia and Perilla, in its glycosylated form (11). Many studies have shown that flavonoids, such as icariin, naringin and soybean isoflavones, can inhibit OP through a variety of pathways and targets (10, 12–14). Luteolin, a flavonoid monomer, may also have great potential for the treatment of OP. Studies have focused on the mechanism of luteolin in the treatment of OP, but clarification of this mechanism is still needed (15, 16). This study aimed to screen the targets of luteolin in the treatment of OP through network pharmacology to study the network relationship among the drug, its targets and related signalling pathways. Some key targets were experimentally verified, providing a scientific basis for the mechanism of luteolin in the treatment of OP and drug development. A flow chart describing this study is shown in Figure 1.
Materials and Methods
Network Pharmacology
Obtaining Potential Targets of Luteolin
The Traditional Chinese Medicine Systems Pharmacology Database and Analysis Platform (TCMSP) (https://tcmspw.com/tcmsp.php) was used to obtain the targets of luteolin. Human genes were identified from the target information with the UniProt database (https://www.UniProt.org/).
Acquisition of OP Target Genes
OP-related genes were obtained from the Comparative Toxicogenomics Database (CTD) (http://ctdbase.org/) and GeneCards (https://www.genecards.org/) and DisGeNET (http://www.disgenet.org/web/DisGeNET/menu/home) databases with “osteoporosis” as the keyword. The target genes of luteolin and OP were introduced into Venny 2.1 to screen genes related to the use of luteolin in the treatment of OP.
Construction of a Protein–Protein Interaction (PPI) Network and Screening of Core Targets
Common target genes of luteolin and OP were imported into the STRING online database (https://string-db.org) for PPI analysis. The species in the database was set to “Homo sapiens”, and the screening confidence was ≥ 0.90. With the above parameters, a PPI network interaction diagram was obtained, and the core gene was then obtained based on the number of node connections.
Construction of a “Luteolin-Target” Network Diagram
The targets of luteolin in OP were visualized with Cytoscape 3.7.1.
Gene Function and Biological Pathway Enrichment Analyses
The target genes of luteolin in the treatment of OP were uploaded to the DAVID data analysis platform for KEGG pathway enrichment and GO biological process enrichment analyses. The species was limited to “Homo sapiens” in the database. Pathways and biological processes for which P < 0.05 were extracted.
Experimental Verification
Animals and Experimental Groups
A total of 20 SPF-grade female SD rats aged 1.5 months with a body weight of (200 ± 10) g were used. The above rats were purchased from the Experimental Animal Center of Southern Medical University. The animal certificate number is 44002100030321. The twenty rats were raised in an SPF environment at the Experimental Animal Center of Guangdong College of Traditional Chinese Medicine. The experimental protocol was implemented after approval by the Animal Ethics Committee of the Guangdong Hospital of Traditional Chinese Medicine (animal experimental ethics approval No. 2021028). Relevant experimental procedures were implemented in accordance with regulations related to the administration of experimental animals approved by the State Council of the People’s Republic of China.
Construction of an OP Rat Model of Bilateral Ovariectomy and Drug Treatment
Twenty rats were divided into five groups (4 rats in each group), namely, the sham group, ovariectomised (OVX) group, low-dose luteolin group (Luteolin-L group), high-dose luteolin group (Luteolin-H) and positive drug group. After isoflurane was used to anaesthetise the rats, the rats in all groups except the sham group underwent surgery on both ovaries. For rats in the sham group, the bilateral ovaries were separated, and some adipose tissue around the ovaries was removed. Drug gavage was started 4 weeks after the operation, and luteolin was dissolved in carboxymethylcellulose sodium (CMC-na, C304951, Aladdin, Shanghai, China) to prepare a suspension. Alendronate sodium and vitamin D3 tablets (J201440144, Merck Sharp & Dohme Ltd., UK) were dissolved in normal saline. Experimental studies have proved that [16], luteolin at 25, 50, and 100 mg/kg doses can alleviate bone loss associated with glucocorticoid-induced osteoporosis induced by gavage in SD rats over 2 months. Based on the principle of reduction and optimisation in the “3R principle” of animal experiments, effective experimental data are obtained on the premise of using as few animals as possible. In this experiment, the bone mass loss of the OP model constructed by the OVX method progressed slowly. Therefore, a high dose of 50 mg/kg was selected to observe the effect of luteolin on the bone mass of OVX rats, and a lower dose of luteolin (10 mg/kg) was used to further explore whether a lower dose of luteolin can also increase the bone mass of OVX rats, representing a safer dose choice for the future treatment of OP with luteolin. Rats in the Luteolin-L group (10 mg/kg body weight) and Luteolin-H group (50 mg/kg body weight) underwent gavage once a day for 8 weeks. Rats in the positive drug group underwent gavage with alendronate sodium and vitamin D3 tablets (6.3 mg/kg) once a week for 8 weeks. Rats in the other two groups were given the same dose of drug dissolved in solvent by gavage. After 8 weeks of gavage, the rats were anaesthetised and euthanised, and the long bones of the limbs were collected for further analysis.
Micro-CT Analysis
After 4% paraformaldehyde fixation, a micro-CT scanner (ZKKS-MCT-Sharp, Guangzhou, China) was used to scan and analyse the morphology of the femur. During scanning, the femur was fixed in the fixator along the long axis. The scanning voltage was set to 70 kV, and the current was set to 100 μA. The power was 7 W, 4 frames were superimposed, the angle gain was 0.72 degrees, the scanning was carried out by rotating for one cycle, and the scanning layer was 15 μm thick. ZKKS-MicroCT 4.1 software was used to analyse the bone morphological parameters of the femoral metaphysis.
Extraction, Culture, and Identification of Rat Primary BMSCs
Bone marrow fluid was collected from the rat long shaft, and rat BMSCs were extracted by whole-bone-marrow cell culture. The cells were cultured in OriCell® rat bone marrow mesenchymal stem cell (BMSC) complete medium. Expression of the cell surface markers CD29, CD44, CD45 and CD90 was detected by flow cytometry. Osteogenic differentiation of the BMSCs was induced by rat BMSCs with an Osteogenic Differentiation Medium Kit (batch No. RASMX-90021, Saiye (Suzhou) Biotechnology Co., Ltd., Suzhou, China). Cell mineralisation was observed by Alizarin red staining. Adipogenic differentiation of the BMSCs was induced by rat BMSCs with an Adipogenic Differentiation Medium kit (batch No. RASMX-90031, Saiye (Suzhou) Biotechnology Co., Ltd., Suzhou, China). Fat droplets in the cells were observed by oil red O staining.
Cell Viability Assay
BMSCs were plated at a density of 2.5 × 103 cells/well in a 96-well plate. After culturing the cells for 24 h, 0.05 μM, 0.1 μM, or 5 μM luteolin (S2320, Selleck, USA) was added, and the cells were then incubated for a further 24, 48, or 72 h. Dimethyl sulfoxide (DMSO, D2650, Sigma, USA) was used to treat the control group. At the end of luteolin treatment, 10 μL of reagent from a Cell Counting Kit-8 (CCK-8, C0038, Beyotime, China) was added to each well, and the cells were incubated for 2 h at 37°C. The optical density (OD) at 450 nm was determined using a microplate reader (M1000 Pro, Tecan, Switzerland); four independent experiments were carried out.
Western Blot (WB) Analysis
After BMSCs were treated with the corresponding drugs, cell lysis buffer for WB and IP (P0013, Beyotime Biotechnology, China) was used to collect the total protein from the cells, and a bicinchoninic acid (BCA) quantification kit (P0012, Beyotime Biotechnology, China) was used to detect the protein concentration. A total of 20 μg of total protein was subjected to SDS–PAGE electrophoresis and then transferred to PVDF membranes. The membranes were blocked with 5% skimmed milk at room temperature for 1 h, and the following diluted antibodies were then added: anti-collagen I (1:1000, ab260043, Abcam), anti-osteopontin (1:1000, ab63856, Abcam), anti-RUNX2 (1:1000, 12556, CST), anti-β-actin (1:2000, 3700, CST), anti-PI3K (1:1000, 3358, CST), anti-Akt (1:1000, 4691, CST), anti-phospho-Akt (Ser473) (1:1000, 4058, CST), and anti-PI3K p85 (1:1000, ab182651, Abcam). The PVDF membranes were washed 3 times with 1× TBST. Finally, the samples were incubated in a refrigerator at 4°C overnight. HRP-conjugated goat anti-rabbit or anti-mouse antibodies (7074, 7076, CST, USA) were added and incubated at room temperature for 1 h. Finally, the membranes were incubated with ECL reagent (WBKLS0100, Merck, Germany) and scanned with a chemiluminescence system (Bio–Rad, USA).
Data Analysis
The data were analysed using one-way analysis of variance (ANOVA) followed by Tukey’s multiple comparison test (GraphPad Prism version 5.0 for Windows).
Results
Common Targets of Luteolin and OP
A total of 57 luteolin targets (including 2 invalid targets) were retrieved with the TCMSP. We obtained 36180 gene targets of OP from the CTD, 1098 gene targets from the DisGeNET database and 2916 gene targets from the GeneCards database. After removing the duplicates, 23531 OP gene targets were obtained. After mapping the targets of luteolin and OP with Venny 2.1, 54 intersecting targets were obtained (Figure 2).
“Luteolin-Target” Network Diagram
Using Cytoscape 3 7.1 software, a “luteolin-target” network diagram was drawn (Figure 3). In the figure, the red node is luteolin, and each green node is a target.
PPI Network Construction
A PPI network consisting of common targets was obtained by using the STRING 11.0 data analysis platform with the confidence set to ≥0.90. After hiding the isolated protein, a PPI network diagram was obtained. A total of 54 proteins were obtained (Figure 4). The degree value is often used to indicate the importance of network nodes. The larger the degree value is, the more important the node is in the network is. The top 10 most important targets in the PPI network based on degree value were TP53, AKT1, HSP90AA1, JUN, IL6, MAPK1, RELA, RB1, CASP3, and CDKN1A (Figure 5).
Results of GO Enrichment Analysis
The intersecting targets of luteolin and OP were analysed for GO functional enrichment with the DAVID data analysis platform, and the top 10 most significantly enriched biological process (BP), cell composition (CC) and molecular function (MF) terms were selected to form a GO enrichment bar graph (Figure 6). BP enrichment included the response to drugs, negative regulation of apoptotic processes, positive regulation of nitric oxide biosynthetic processes, and positive regulation of transcription from RNA polymerase II promoter. CC enrichment included the cytosol, nucleus, nucleoplasm, membrane raft, etc. MF enrichment included identical protein binding, enzyme binding, protein binding, protein heterodimerisation activity, and protein phosphatase binding.
Results of KEGG Enrichment Analysis
The enrichment of KEGG pathways in the intersecting targets of luteolin and OP were analysed with the DAVID data analysis platform. Ultimately, a total of 109 signalling pathways were obtained. After excluding obviously unrelated disease pathways, such as tumour- and liver disease-related pathways, the top 15 most significantly enriched pathways were selected and used to form a bubble diagram consisting of enriched KEGG signalling pathways (Figure 7). The results showed that luteolin may inhibit OP by regulating the T cell receiver, PI3K-Akt, TNF, HIF-1, oestrogen, FoxO and p53 signalling pathways.
Luteolin Alleviated Bone Loss in an OP Model by Promoting the Osteogenic Differentiation of BMSCs
To verify the mechanism of luteolin in the treatment of OP, we administered luteolin (low-dose group: 10 mg/kg, high-dose group: 50 mg/kg) to OVX model rats by gavage and used alendronate D3 tablets as the positive control. After 8 weeks of gavage, the femurs were collected from the rats in each group, and the distal femur was scanned by micro-CT. The imaging results showed that bone mass was significantly decreased for rats in the OVX group compared with those in the sham group. However, bone mass was significantly greater for the Luteolin-L group, Luteolin-H group and positive drug group compared with the OVX group (Figure 8A). An analysis of bone morphological parameters showed that the bone mineral density (BMD), relative bone volume fraction (BV/TV) and trabecular number (Tb.N) were significantly lower for the OVX group than for the sham group, but the values of the Luteolin-L group, Luteolin-H group and positive drug group were significantly higher than those of the OVX group. The BMD and Tb.N values were greater for the Luteolin-H group than for the positive drug group. Trabecular separation (Tb.Sp) was significantly greater for the OVX group than for the sham group. Furthermore, the Tb.Sp of the Luteolin-H group was smaller than that of the OVX group and smaller than that of the positive drug group. There was no significant difference in trabecular thickness (Tb.Th) of the femur between the groups (Figure 8B). We collected long bone marrow from the rats in each group, extracted BMSCs and examined the isolated and cultured primary BMSCs. The results showed that the BMSCs grew normally after separation, and the cells were long and spindle-shaped under an inverted microscope (Figure 9A). After osteogenic induction, the results of alizarin red staining suggested the presence of a large number of mineralised nodules stained red (Figure 9B). After lipid induction, oil red O staining showed a large number of lipid droplets dyed orange (Figure 9C). Through fluorescent flow cytometry analysis of CD29, CD44, CD45 and CD90, the results showed that the CD29, CD44, CD45 and CD90 positivity rates were 99.00%, 99.08%, 0.08% and 98.17%, respectively (Figures 9D–G), in line with the biological characteristics of BMSCs. We extracted total protein from the BMSCs in each group and analysed osteogenic differentiation-related protein levels by WB. The results showed that the protein expression levels of collagen I, osteopontin and RUNX2 in BMSCs of the OVX group were significantly lower than those of the sham group, but the values of the Luteolin-L group, Luteolin-H group and positive drug group were significantly higher than those of the OVX group, and the expression of RUNX2 in the Luteolin-H group was also higher than that in the positive drug group (Figures 8C, D). To verify the effect of luteolin on the osteogenic differentiation of BMSCs, we further analysed the effect of luteolin on the viability of BMSCs and the expression of osteogenic differentiation-related proteins through in vitro cellular experiments. The results of CCK-8 assays showed that the viability of the BMSCs was not affected after 72 h of culture with luteolin at a concentration of 5 μM (Figure 8E). The results of WB showed that the protein expression levels of collagen I, osteopontin and RUNX2 in BMSCs cultured with luteolin at concentrations of 0.5, 1 and 5 μM were significantly higher than those in the DMSO group (Figures 8F, G). The above results show that luteolin could reduce bone loss in OVX rats, inhibit the progression of OP, and promote the osteogenic differentiation of BMSCs.
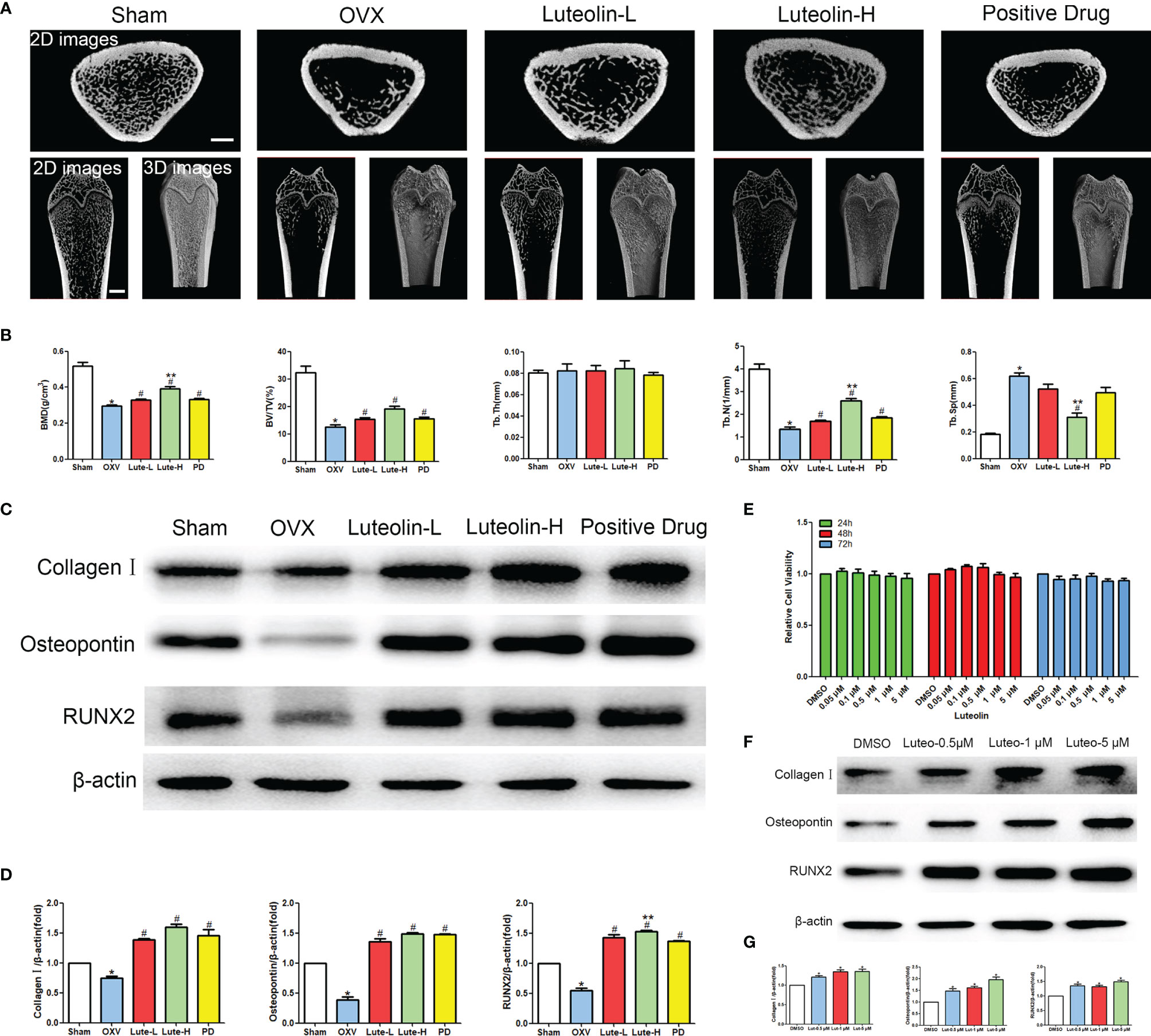
Figure 8 Luteolin alleviated bone loss in OVX rats by promoting the osteogenic differentiation of BMSCs. (A) Micro-CT image of the femoral diaphysis (scale bars, 1 mm). (B) Quantitative presentation of the femoral microarchitectural parameters BMD, BV/TV, Tb.Th, Tb.N, and Tb.Sp expressed as the mean ± SD from four independent experiments. Compared with the sham group, *p < 0.05; compared with the OVX group, #p < 0.05; compared with the DG group, **p < 0.05. (C) The expression levels of osteogenesis-related marker proteins (collagen I, osteopontin and RUNX2). (D) Comparative analysis of grey values for relevant protein bands; data are expressed as the mean ± SD from three independent experiments. Compared with the sham group, *p < 0.05; compared with the OVX group, #p < 0.05; compared with the DG group, **p < 0.05. (E) BMSCs were treated with luteolin at increasing concentrations (0.05 μM, 0.1 μM, 0.5 μM, 1 μM, and 5 μM) for 24, 48 and 72 h, after which the OD value was determined by CCK-8 assay. Cell viability is expressed as the mean ± SD from four independent experiments. There was no significant difference. (F) BMSCs were treated with luteolin at increasing concentrations (0.5 μM, 1 μM, and 5 μM) for 48 h, after which osteogenesis-related marker protein (collagen I, osteopontin, RUNX2) expression was analysed with WB. (G) Comparative analysis of grey values from relevant protein bands; data are expressed as the mean ± SD from three independent experiments. Compared with the DMSO group, *p < 0.05.
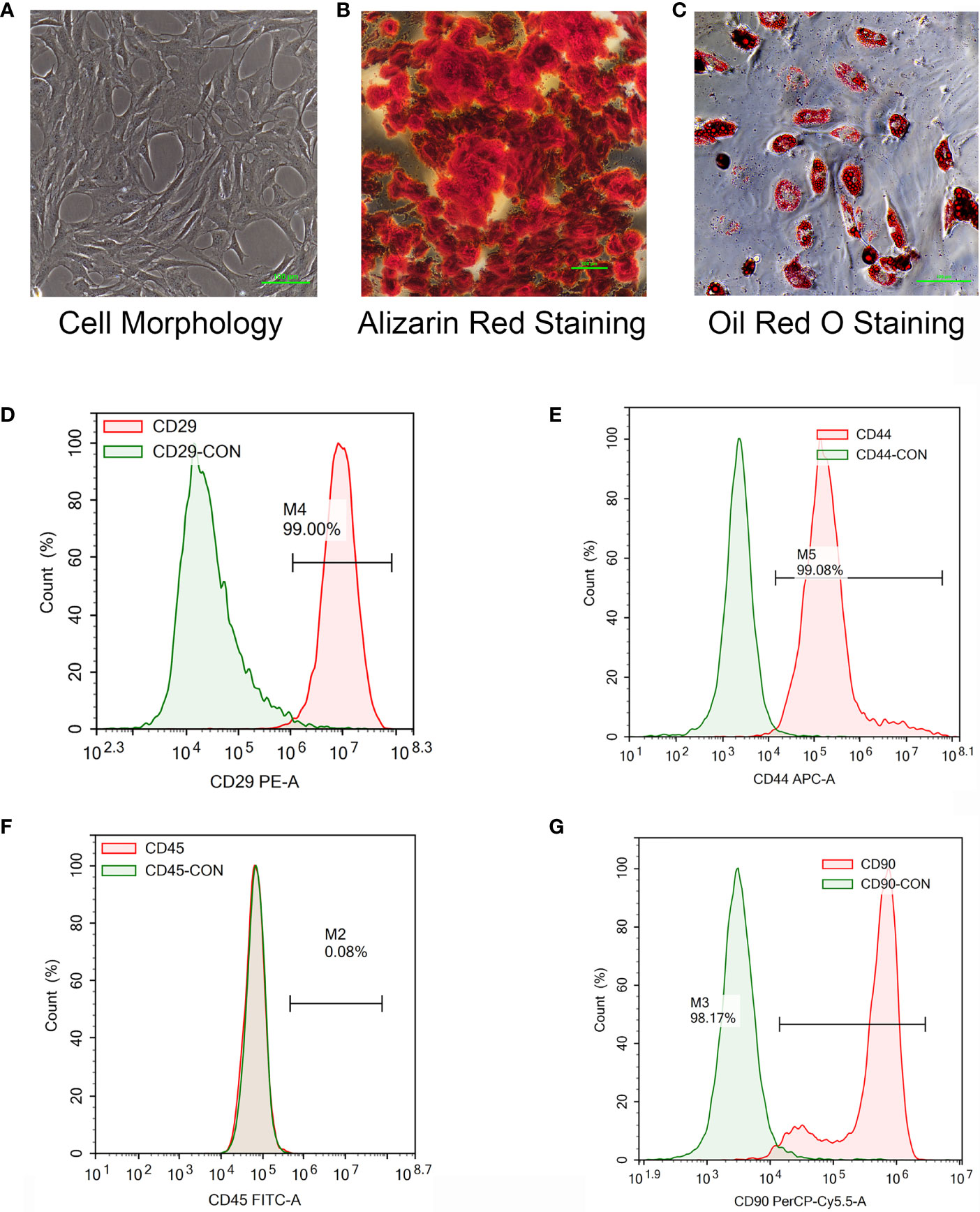
Figure 9 Isolation and culture of rat primary BMSCs, the induction of osteogenic and adipogenic differentiation and the detection of cell surface markers. (A) The morphology of primary BMSCs from third-generation rats was observed under a microscope (×40). (B) After the osteogenic differentiation of third-generation BMSCs was induced with an osteogenic differentiation-inducing agent (Alizarin red staining), the formation of mineralized nodules was observed under a microscope (×100). (C) The adipogenic differentiation of third-generation BMSCs was induced by an adipogenic differentiation-inducing agent, and lipid droplets were enlarged and made rounder by continuous culture with maintenance medium (stained with oil red O) and observed under a microscope (×200). (D–F) The primary BMSCs of third-generation rats were collected and stained for CD29 (D), CD44 (E), CD45 (F) and CD90 (G) for 30 min. Control staining was simultaneously carried out. Fluorescence was detected with a flow cytometer, and the results were analysed.
Luteolin Promotes the Osteogenic Differentiation of BMSCs by Activating the PI3K/Akt Signalling Pathway
Our animal experiments suggested that luteolin can promote the osteogenic differentiation of BMSCs, and the results of a network pharmacological analysis show that luteolin can regulate the PI3K/Akt signalling pathway. Therefore, we further explored the effect of luteolin on PI3K/Akt signalling pathway activity. The results of WB showed that the levels of phosphorylated PI3K and Akt (p-PI3K/PI3K and p-Akt/Akt) in BMSCs of the OVX group were significantly lower than those of BMSCs of the sham group. The levels BMSCs of in the Luteolin-L group, Luteolin-H group and positive drug group were higher than those in BMSCs of the OVX group (Figures 10A, B). After BMSCs were cultured with luteolin at concentrations of 0.5, 1 and 5 μM for 48 h, the relative expression of phosphorylated PI3K and Akt (p-PI3K/PI3K and p-Akt/Akt) was also significantly higher than that in the DMSO group (Figures 10C, D). To verify the role of the PI3K/Akt signalling pathway in the ability of luteolin to promote the osteogenic differentiation of BMSCs, we used the PI3K inhibitor LY294002 to inhibit the activity of the PI3K/Akt signalling pathway in BMSCs. The results of WB showed that the p-Akt/Akt expression ratio in BMSCs treated with 20 μM LY294002 was significantly lower than that in the DMSO group, indicating that LY294002 inhibited the activity of the PI3K/Akt signalling pathway in the BMSCs. LY294002 also reduced the protein expression levels of collagen I, osteopontin and RUNX2 in the BMSCs; the protein expression levels of collagen I, osteopontin and RUNX2 in BMSCs cultured with both 20 μM LY294002 and 5 μM luteolin were significantly lower than those in BMSCs cultured with 5 μM luteolin alone (Figures 10E, F), suggesting that inhibiting the activity of the PI3K/Akt signalling pathway reduced the ability of luteolin to promote the osteogenic differentiation of BMSCs. The above results suggest that luteolin may promote the osteogenic differentiation of BMSCs by enhancing the activity of the PI3K/Akt signalling pathway.
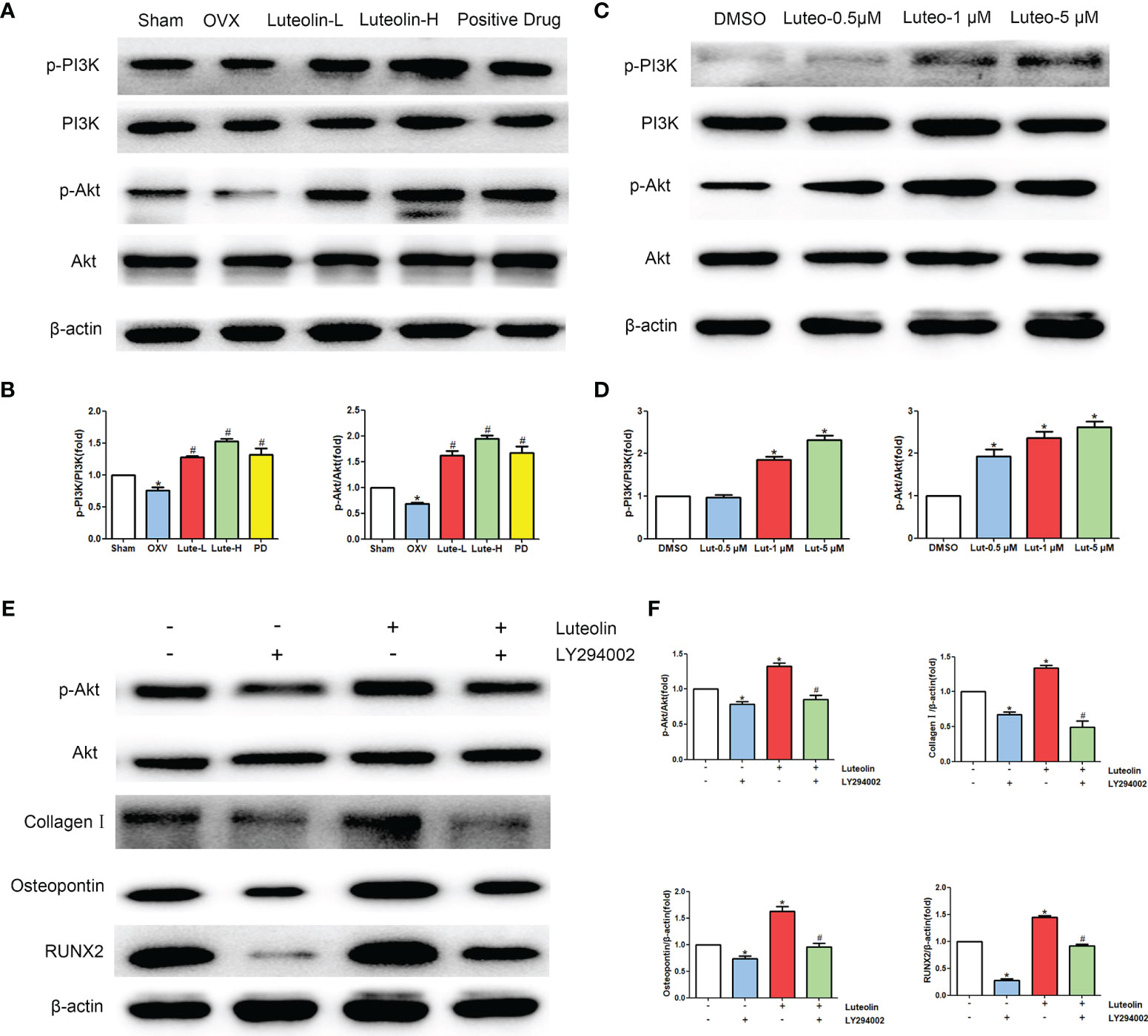
Figure 10 Luteolin promotes the osteogenic differentiation of BMSCs by activating the PI3K/Akt signalling pathway. (A) The protein expression of p-PI3K, PI3K, p-Akt and Akt was detected by WB. (B) Comparative analysis of grey values from relevant protein bands; data are expressed as the mean ± SD from three independent experiments. Compared with the sham group, *p < 0.05; compared with the OVX group, #p < 0.05. (C) BMSCs were treated with luteolin at increasing concentrations (0.5 μM, 1 μM, and 5 μM) for 48 h, followed by an analysis of p-PI3K, PI3K, p-Akt and Akt protein expression levels with WB. (D) Comparative analysis of grey values from relevant protein bands; data are expressed as the mean ± SD from three independent experiments. Compared with the DMSO group, *p < 0.05. (E) BMSCs were incubated with or without 20 μM LY294002 (S1105, Selleck, USA) and 5 μM luteolin for 48 h, followed by an analysis of collagen I, osteopontin, RUNX2, p-Akt and Akt protein expression levels by WB. (F) Comparative analysis of grey values from relevant protein bands; data are expressed as the mean ± SD from three independent experiments. Compared with the DMSO group, *p < 0.05; compared with the luteolin only group, *p < 0.05.
Discussion
Studies suggest that luteolin can reduce bone loss by reducing osteoclast activity and differentiation function (17, 18). Therefore, based on the large number of sources of luteolin and its great potential for the treatment of OP, in this study, we combined network pharmacology and experimental verification to explore the mechanism of luteolin in the treatment of OP. Through the prediction of luteolin and disease-related targets by network pharmacology, 54 potential targets of luteolin in OP were screened. PPI network analysis showed that luteolin may inhibit OP by regulating TP53, AKT1, JUN, RELA, CASP3, MAPK1, RB1 and CDKN1A. GO enrichment analysis found that the biological processes enriched in the targets of luteolin against OP include the response to drugs, negative regulation of apoptotic processes, and positive regulation of nitric oxide biosynthetic processes. The results of KEGG pathway enrichment analysis showed that luteolin may play a key role in regulating the PI3K-Akt, TNF, HIF-1, oestrogen and p53 signalling pathways. These results suggest that luteolin can regulate the biological processes of OP through multiple targets and pathways. The results of GO enrichment and KEGG pathway enrichment analyses showed that the degree to which the PI3K-Akt signalling pathway is enriched in targets of luteolin and OP is high, which may have significance and value for treating OP.
The results of animal experiments show that luteolin could reduce bone loss in OVX rats and upregulate the protein expression of collagen I, osteopontin and RUNX2 in BMSCs to promote osteogenic differentiation. In vitro cellular studies showed that luteolin promoted the osteogenic differentiation of BMSCs by increasing the p-PI3K/PI3K and p-Akt/Akt expression ratios, subsequently enhancing the activity of the PI3K/Akt signalling pathway. One study (19) showed that activation of the PI3K/Akt signalling pathway could stimulate the proliferation and differentiation of osteoblasts and inhibit their apoptosis. In addition, PI3K can stimulate the formation of osteoclast actin filaments and regulate cell chemotaxis, adhesion and diffusion. Inhibiting PI3K expression can reduce bone resorption of mature osteoclasts (19). Luteolin is a flavonoid monomer. Other research into the use of flavonoids for the treatment of OP has revealed that flavonoids, such as epimedium and drynaria, can regulate the activity balance of osteoblasts and osteoclasts by activating the PI3K/Akt signalling pathway (20, 21). The results of PPI analysis showed that AKT1 and TP53 are key targets of luteolin in the treatment of OP. Compared with that in normal mice, bone mass in AKT1- and AKT2-knockout mice are significantly decreased, suggesting that this pathway is related to enhanced bone formation (22). The PI3K/Akt signalling pathway is also negatively regulated by several factors. For example, TP53 can negatively regulate the PI3K/Akt signalling pathway and promote the dephosphorylation of members of the PI3K/Akt signalling pathway through a series of biological effects (23, 24). Studies have shown that the PI3K-Akt signalling pathway can affect bone formation and bone cell survival, thereby controlling BMD balance (25, 26). Based on this information and our network pharmacology and experimental research results, we believe that luteolin may enhance the activity of the PI3K-Akt signalling pathway by interfering with target proteins such as AKT1 and TP53 to inhibit OP.
This study has the following limitations, and further theoretical and experimental research is needed in the future. First, public databases are dynamic, so the target data associated with the results of this study may change in the future. Additionally, this study did not comprehensively verify the targets and pathway results, which were theoretically derived. Other pathway and target data that were not verified in this study need to be further studied in future work.
Conclusions
Based on network pharmacology experiments and an established OP rat model, this study discusses the mechanism of luteolin in the treatment of OP, and the PI3K-Akt signalling pathway was selected for further experimental verification. The results show that luteolin can reduce bone loss in OVX rats, and upregulate the protein expression of collagen I, osteopontin and RUNX2 in BMSCs, promoting osteogenic differentiation. The possible mechanism by which luteolin acts against osteoporosis is the promotion of BMSC osteogenic differentiation via PI3K Akt signalling pathway activation regulation. This study provides new insights for the experimental and clinical treatment of OP in the future.
Data Availability Statement
The original contributions presented in the study are included in the article/Supplementary Material. Further inquiries can be directed to the corresponding authors.
Ethics Statement
The animal study was reviewed and approved by the Experimental Animal Center of Guangdong College of Traditional Chinese Medicine.
Author Contributions
GL, JZ, LZ, and WY contributed to the concept and design of this study. GL, JZ, YD, YY, ZZ, and RZ performed the computational analyses and performed the in vivo experiments. GL, JZ, YD, DZ, YY, and ZZ performed the experimental analysis. GL and JZ drafted the manuscript. All the authors read, revised, and approved the final manuscript.
Funding
This work was supported by the National Natural Science Foundation of China (No. No.82004383, No. 81974574), the National key research and development program (2021YFC1712804), the Project of Administration of Traditional Chinese Medicine of Guangdong Province (No.20201129, No.20225016, No.20225025), the Project of Guangdong Provincial Department of Finance (No. [2014]157), the Medical Science Research Foundation of Guangdong Province (No.A2020105, No.B2019091), the Science and Technology Planning Project of Guangzhou (No. 202102010273) and the Science and Technology Research Project of Guangdong Provincial Hospital of Chinese Medicine (No.YN2019ML08).
Conflict of Interest
The authors declare that the research was conducted in the absence of any commercial or financial relationships that could be construed as a potential conflict of interest.
Publisher’s Note
All claims expressed in this article are solely those of the authors and do not necessarily represent those of their affiliated organizations, or those of the publisher, the editors and the reviewers. Any product that may be evaluated in this article, or claim that may be made by its manufacturer, is not guaranteed or endorsed by the publisher.
Supplementary Material
The Supplementary Material for this article can be found online at: https://www.frontiersin.org/articles/10.3389/fendo.2022.866641/full#supplementary-material
References
1. Gkastaris K, Goulis DG, Potoupnis M, Anastasilakis AD, Kapetanos G. Obesity, Osteoporosis and Bone Metabolism. J Musculoskelet Neuronal Interact (2020) 20(3):372–81.
2. Fardellone P, Salawati E, Monnier LL, Goëb V. Bone Loss, Osteoporosis, and Fractures in Patients With Rheumatoid Arthritis: A Review. J Clin Med (2020) 9:3361. doi: 10.3390/jcm9103361
3. Johnston CB, Dagar M. Osteoporosis in Older Adults. Med Clin N Am (2020) 104:873–84. doi: 10.1016/j.mcna.2020.06.004
4. Rozenberg S, Bruyère O, Bergmann P, Cavalier E, Gielen E, Goemaere S, et al. How to Manage Osteoporosis Before the Age of 50. Maturitas (2020) 138:14–25. doi: 10.1016/j.maturitas.2020.05.004
5. Si L, Winzenberg TM, Jiang Q, Chen M, Palmer AJ. Projection of Osteoporosis-Related Fractures and Costs in China: 2010–2050. Osteoporosis Int (2015) 26:1929–37. doi: 10.1007/s00198-015-3093-2
6. Polzonetti V, Pucciarelli S, Vincenzetti S, Polidori P. Dietary Intake of Vitamin D From Dairy Products Reduces the Risk of Osteoporosis. Nutrients (2020) 12:1743. doi: 10.3390/nu12061743
7. Zhu Y, Huang Z, Wang Y, Xu W, Chen H, Xu J, et al. The Efficacy and Safety of Denosumab in Postmenopausal Women With Osteoporosis Previously Treated With Bisphosphonates: A Review. J Orthop Transl (2020) 22:7–13. doi: 10.1016/j.jot.2019.08.004
8. Clynes MA, Harvey NC, Curtis EM, Fuggle NR, Dennison EM, Cooper C. The Epidemiology of Osteoporosis. Brit Med Bull (2020) 133(1):105–17. doi: 10.1093/bmb/ldaa005
9. Iolascon G, Moretti A, Toro G, Gimigliano F, Liguori S, Paoletta M. Pharmacological Therapy of Osteoporosis: What’s New? Clin Interv Aging (2020) 15:485–91. doi: 10.2147/CIA.S242038
10. Zhang G, Qin L, Hung WY, Shi YY, Leung PC, Yeung HY, et al. Flavonoids Derived From Herbal Epimedium Brevicornum Maxim Prevent OVX-Induced Osteoporosis in Rats Independent of Its Enhancement in Intestinal Calcium Absorption. Bone (2006) 38:818–25. doi: 10.1016/j.bone.2005.11.019
11. Yan H, Wei P, Song J, Jia X, Zhang Z. Enhanced Anticancer Activity in Vitro and in Vivo of Luteolin Incorporated Into Long-Circulating Micelles Based on DSPE-PEG2000 and TPGS. J Pharm Pharmacol (2016) 68:1290–8. doi: 10.1111/jphp.12598
12. Mu P, Hu Y, Ma X, Shi J, Zhong Z, Huang L. Total Flavonoids of Rhizoma Drynariae Combined With Calcium Attenuate Osteoporosis by Reducing Reactive Oxygen Species Generation. Exp Ther Med (2021) 21:618. doi: 10.3892/etm.2021.10050
13. Shi X, Liu K, Wu L. Interventional Value of Total Flavonoids From Rhizoma Drynariae on Cathepsin K, a Potential Target of Osteoporosis. Chin J Integr Med (2011) 17:556–60. doi: 10.1007/s11655-010-0792-1
14. Wang J, Jiang J, Xie Y, Wei X, Li J, Duan J, et al. Population Pharmacokinetics of Naringin in Total Flavonoids of Drynaria Fortunei (Kunze) J. Sm. In Chinese Women With Primary Osteoporosis. Chin J Integr Med (2012) 18:925–33. doi: 10.1007/s11655-012-1296-0
15. Zheng L. Luteolin Stimulates Proliferation and Inhibits Late Differentiation of Primary Rat Calvarial Osteoblast Induced by High-Dose Dexamethasone via Sema3A/NRP1/Pleixin A1. Curr Pharm Biotechnol (2021) 22:1538–45. doi: 10.2174/1389201021666201216150442
16. Jing Z, Wang C, Yang Q, Wei X, Jin Y, Meng Q, et al. Luteolin Attenuates Glucocorticoid-Induced Osteoporosis by Regulating ERK/Lrp-5/GSK-3β Signaling Pathway In Vivo and In Vitro. J Cell Physiol (2019) 234:4472–90. doi: 10.1002/jcp.27252
17. Li N, Cornelissen D, Silverman S, Pinto D, Si L, Kremer I, et al. An Updated Systematic Review of Cost-Effectiveness Analyses of Drugs for Osteoporosis. Pharmacoeconomics (2021) 39:181–209. doi: 10.1007/s40273-020-00965-9
18. Kim T, Jung JW, Ha BG, Hong JM, Park EK, Kim H, et al. The Effects of Luteolin on Osteoclast Differentiation, Function In Vitro and Ovariectomy-Induced Bone Loss. J Nutr Biochem (2011) 22:8–15. doi: 10.1016/j.jnutbio.2009.11.002
19. Shi D, Dong M, Lu Y, Niu W. PI3K/Akt Signaling Pathway and Bone Destruction: Problems and Mechanisms. Chin J Tissue Eng Res (2020) 24:3716–22. doi: 10.3969/j.issn.2095-4344.2728
20. Qu H, Zheng J. Research Progress on the Anti-Osteoporosis Effect of Plant Flavones. Chin J Osteoporos (2021) 27:1529–33. doi: 10.3969/j.issn.1006-7108.2021.10.024
21. Zhang J, Ren Y, Zhang S. Recent Advances in Anti-Osteoporosis Effects and Drug Delivery System of Icaritin. Chin Pharm J (2021) 56:781–4. doi: 10.11669/cpj.2021.10.001
22. Peng XD, Xu PZ, Chen ML, Hahn-Windgassen A, Skeen J, Jacobs J, et al. Dwarfism, Impaired Skin Development, Skeletal Muscle Atrophy, Delayed Bone Development, and Impeded Adipogenesis in Mice Lacking Akt1 and Akt2. Genes Dev (2003) 17:1352–65. doi: 10.1101/gad.1089403
23. Diehl N, Schaal H. Make Yourself at Home: Viral Hijacking of the PI3K/Akt Signaling Pathway. Viruses (2013) 5:3192–212. doi: 10.3390/v5123192
24. Nielsen-Preiss SM, Silva SR, Gillette JM. Role of PTEN and Akt in the Regulation of Growth and Apoptosis in Human Osteoblastic Cells. J Cell Biochem (2003) 90:964–75. doi: 10.1002/jcb.10709
25. Roncevic J, Djoric I, Selemetjev S, Jankovic J, Dencic TI, Bozic V, et al. MMP-9-1562 C/T Single Nucleotide Polymorphism Associates With Increased MMP-9 Level and Activity During Papillary Thyroid Carcinoma Progression. Pathology (2019) 51:55–61. doi: 10.1016/j.pathol.2018.10.008
Keywords: Traditional Chinese medicine, luteolin, osteoporosis, network pharmacology, signalling pathway, experimental verification
Citation: Liang G, Zhao J, Dou Y, Yang Y, Zhao D, Zhou Z, Zhang R, Yang W and Zeng L (2022) Mechanism and Experimental Verification of Luteolin for the Treatment of Osteoporosis Based on Network Pharmacology. Front. Endocrinol. 13:866641. doi: 10.3389/fendo.2022.866641
Received: 31 January 2022; Accepted: 14 February 2022;
Published: 08 March 2022.
Edited by:
Bo Liu, Guangdong Provincial Hospital of Chinese Medicine, ChinaReviewed by:
Chen Chao, Southern Medical University, ChinaAnna Piotrowska, University School of Physical Education in Krakow, Poland
Yikai Li, Southern Medical University, China
Copyright © 2022 Liang, Zhao, Dou, Yang, Zhao, Zhou, Zhang, Yang and Zeng. This is an open-access article distributed under the terms of the Creative Commons Attribution License (CC BY). The use, distribution or reproduction in other forums is permitted, provided the original author(s) and the copyright owner(s) are credited and that the original publication in this journal is cited, in accordance with accepted academic practice. No use, distribution or reproduction is permitted which does not comply with these terms.
*Correspondence: Weiyi Yang, gdszyyywy@sina.com; Lingfeng Zeng, lfzeng6778@163.com
†These authors have contributed equally to this work