- 1SERGAS (Servizo Galego de Saude), NEIRID Lab (Neuroendocrine Interactions in Rheumatology and Inflammatory Diseases), Research Laboratory 9, IDIS (Instituto de Investigación Sanitaria de Santiago), Santiago University Clinical Hospital, Santiago de Compostela, Spain
- 2Euro-Mediterranean Master in neuroscience and Biotechnology, Faculty of Science, Alexandria University, Alexandria, Egypt
- 3International PhD School of the University of Santiago de Compostela (EDIUS), Doctoral Program in Drug Research and Development, Santiago de Compostela, Spain
- 4International PhD School of the University of Santiago de Compostela (EDIUS), Doctoral Program in Medicine Clinical Research, Santiago de Compostela, Spain
- 5National Health Service, Local Health Authority ASL 3 Napoli Sud, Department of Pharmacy, Naples, Italy
- 6Hospital Universitario Marqués de Valdecilla, Epidemiology, Genetics and Atherosclerosis Research Group on Systemic Inflammatory Diseases, IDIVAL, University of Cantabria, Santander, Cantabria, Spain
- 7SERGAS, Santiago University Clinical Hospital, Division of Rheumatology, Santiago de Compostela, Spain
- 8SERGAS (Servizo Galego de Saude), IDIS (Instituto de Investigación Sanitaria de Santiago), Molecular and Cellular Cardiology Lab, Research Laboratory 7, Santiago University Clinical Hospital, Santiago de Compostela, Spain
- 9Faculty of Science, Alexandria University, Alexandria, Egypt
Adipose tissue malfunction leads to altered adipokine secretion which might consequently contribute to an array of metabolic diseases spectrum including obesity, diabetes mellitus, and cardiovascular disorders. Asprosin is a novel diabetogenic adipokine classified as a caudamin hormone protein. This adipokine is released from white adipose tissue during fasting and elicits glucogenic and orexigenic effects. Although white adipose tissue is the dominant source for this multitask adipokine, other tissues also may produce asprosin such as salivary glands, pancreatic B-cells, and cartilage. Significantly, plasma asprosin levels link to glucose metabolism, lipid profile, insulin resistance (IR), and β-cell function. Indeed, asprosin exhibits a potent role in the metabolic process, induces hepatic glucose production, and influences appetite behavior. Clinical and preclinical research showed dysregulated levels of circulating asprosin in several metabolic diseases including obesity, type 2 diabetes mellitus (T2DM), polycystic ovarian syndrome (PCOS), non-alcoholic fatty liver (NAFLD), and several types of cancer. This review provides a comprehensive overview of the asprosin role in the etiology and pathophysiological manifestations of these conditions. Asprosin could be a promising candidate for both novel pharmacological treatment strategies and diagnostic tools, although developing a better understanding of its function and signaling pathways is still needed.
1 Introduction
Adipose tissue operates as a central energy storage endocrine organ that produces a diversity of bioactive mediators known as adipokines (adipose-derived secreted factors) which possess proinflammatory or anti-inflammatory effects (1). Adipokines are mainly, but not exclusively, produced by lipid-rich cells termed adipocytes located in the white adipose tissue; the predominant type of fat in mammals (2). They can easily flow to the systemic circulation and exert their actions through an inter-cell communication network (autocrine, paracrine, endocrine). Moreover, they maintain the regulation of various aspects of the normal metabolic processes in the human body including glucose and lipid homeostasis, insulin sensitivity, and inflammatory response.
Metabolic syndrome (MetS) is a natural consequence of many risk factors including a sedentary lifestyle, uncontrolled nutrition, and excessive adiposity (3). Various scientific evidence suggests that obesity is the starting point of many metabolism-related diseases such as type 2 diabetes mellitus (T2DM), cardiovascular diseases, polycystic ovary syndrome (PCOS), and inflammatory rheumatic disorders (4, 5). Obesity usually provokes pathological acceleration of adipose tissue remodeling via alterations in the size (hypertrophy) and number (hyperplasia) of adipocytes (6, 7). Thus, it further causes adiposopathy, represented as an imbalance in the production and release of adipokines and consequent metabolic and cardiovascular disturbances (8, 9). During the past two decades, numerous profiling studies have identified growing number of adipokines and their roles in several signaling cascades in healthy and pathological conditions (10).
This review aimed to briefly discuss the discovery, synthesis, expression of asprosin and its cognate receptors. Additionally, it puts a spotlight on the multifunctional activity of asprosin and assesses the circulating levels of asprosin in several metabolism-related diseases obesity, T2DM, PCOS, and cancer.
2 Novel caudamin member: discovery and biosynthesis
Asprosin is a novel adipokine that recently joined the newly established protein hormone subclass caudamin (10). This subclass is synthesized differently from traditional protein hormones and peptides (11). Typically, members of this subclass are derived from a C-terminus cleavage event that yields a large protein while the alternative N-terminal segment renders a non-hormonal protein that does not associate with the hormone realm in terms of biosynthetic origin, properties, and function (11). As a caudamin member, asprosin is synthesized by cleaving the C-terminus of a parent protein called profibrillin-1 protein by the proteolytic enzyme furin (11, 12). This cleavage produces the 320 kDa glycoprotein fibrillin-1 in addition to the novel peptide hormone asprosin. Originally, profibrillin-1 is encoded by exons 65 and 66 of the fibrillin-1 gene (FBN1) located on chromosome 15q21.1. Mammalian asprosin is approximately a 30 kDa and 140 amino acids long protein (12).
Asprosin was first discovered at Baylor College of medicine by Romere et al. in 2016 while examining individuals with an inherited rare disorder named neonatal progeroid syndrome (NPS). In this disease, an elevated metabolic rate, low appetite, and insulin resistance (IR) lead to a generalized loss of subcutaneous fat and markedly low body weight, the leading cardinal features of NPS. The researchers found that the truncated heterozygous mutant form of the FBN1 gene caused a profound decreased plasma asprosin levels in NPS patients (12, 13). The same study also reported that adult IR obese mice have pathologically enhanced levels of asprosin during fasting. A single injection of recombinant asprosin in mice may cause an immediate increase in blood glucose and hyperinsulinemia (12). In addition, studies on humans with insulin resistance (IR) and high-fat diet mice models confirm the significant reduction of pathologically elevated asprosin levels after treatment with specific asprosin antibodies (13). Asprosin neutralization was reported to cause a concurrent decrease in food intake and body weight in mouse model of metabolic syndrome (14). These findings resulted in the characterization of asprosin as an orexigenic and glucogenic hormone.
3 Asprosin secretion and expression
Higher expression of FBN1 mRNA was detected in white adipose tissue suggesting it as the dominant producer of asprosin (12). A preclinical study showed lower plasma asprosin level in Bscl2_/_ deficient mice previously exposed to genetic resection of adipose tissue (≈ 65% decrease in adipose tissue) (15). Furthermore, asprosin may have a regulatory role in the browning process of primary adipocytes. Asprosin overexpression in subcutaneous white adipose tissue was revealed to downregulate browning-specific markers (UCP1) and accelerate lipid deposition via inhibition of the nuclear factor erythroid 2–related factor 2 (Nrf2) pathway in high-fat diet mice (16).
Other organs also appeared to produce this glucogenic peptide such as the heart, liver, pancreas, stomach, skeletal muscles, lungs, and brain (12, 17). Recent studies reported measurable amounts of asprosin in different body fluids such as saliva, breast milk, urine, serum, and plasma (18–20). Interestingly, asprosin was found to be expressed in the ovaries, placenta and human cartilage (19, 21, 22). A strong correlation was reported between serum asprosin levels and a specific marker for cartilage degradation termed cartilage oligomeric matrix protein (COMP) in patients subjected to total hip replacement surgery (19).
In humans and mice, asprosin is secreted into circulation in nanomolar concentration (12). Upon secretion, asprosin traffics via the circulation and is then recruited by the liver where it binds to the surface of hepatocytes and promotes blood glucose elevation which is vital for survival and brain function. The increased glucose production may serve as an inhibitor of asprosin secretion via a negative feedback loop (12).
4 Asprosin receptors
After secretion, circulating asprosin was found to exert peripheral and central modulating effects (15, 23). It acts as glucogenic and appetite stimulant hormone via the olfactory receptor 4M1 (OR4M1) and a cell surface receptor termed protein tyrosine phosphatase receptor δ (Ptprd), respectively (15, 23). OR4M1 (rhodopsin family member) is considered the primary asprosin receptor in humans, while OLFR734 is the mouse ortholog. Circulating asprosin binds to the OLFR734 receptor on the surface of hepatocytes to promote hepatic glucose production via activation of cyclic adenosine monophosphate (cAMP) second messenger system dependent protein kinase A (PKA) signaling pathway (12). OLFR734 is markedly distributed in the testis, liver, kidney, olfactory epithelium tissue, and olfactory bulb (15).
Centrally, asprosin hormone can pass across the blood-brain barrier and then activates the feeding neural circuitry to stimulate appetite (13). In the hypothalamic arcuate nucleus (ARH), asprosin acts directly on hunger-stimulating neurons known as agouti-related peptide neurons (AgRP) via the G protein-cAMP-PKA pathway (Figure 1) (13, 24). As previously mentioned, neutralization of serum asprosin with a monoclonal antibody in obese mice with pathologically elevated asprosin levels resulted in a decreased blood asprosin levels combined with reduced appetite and improved glycemic profile (13).
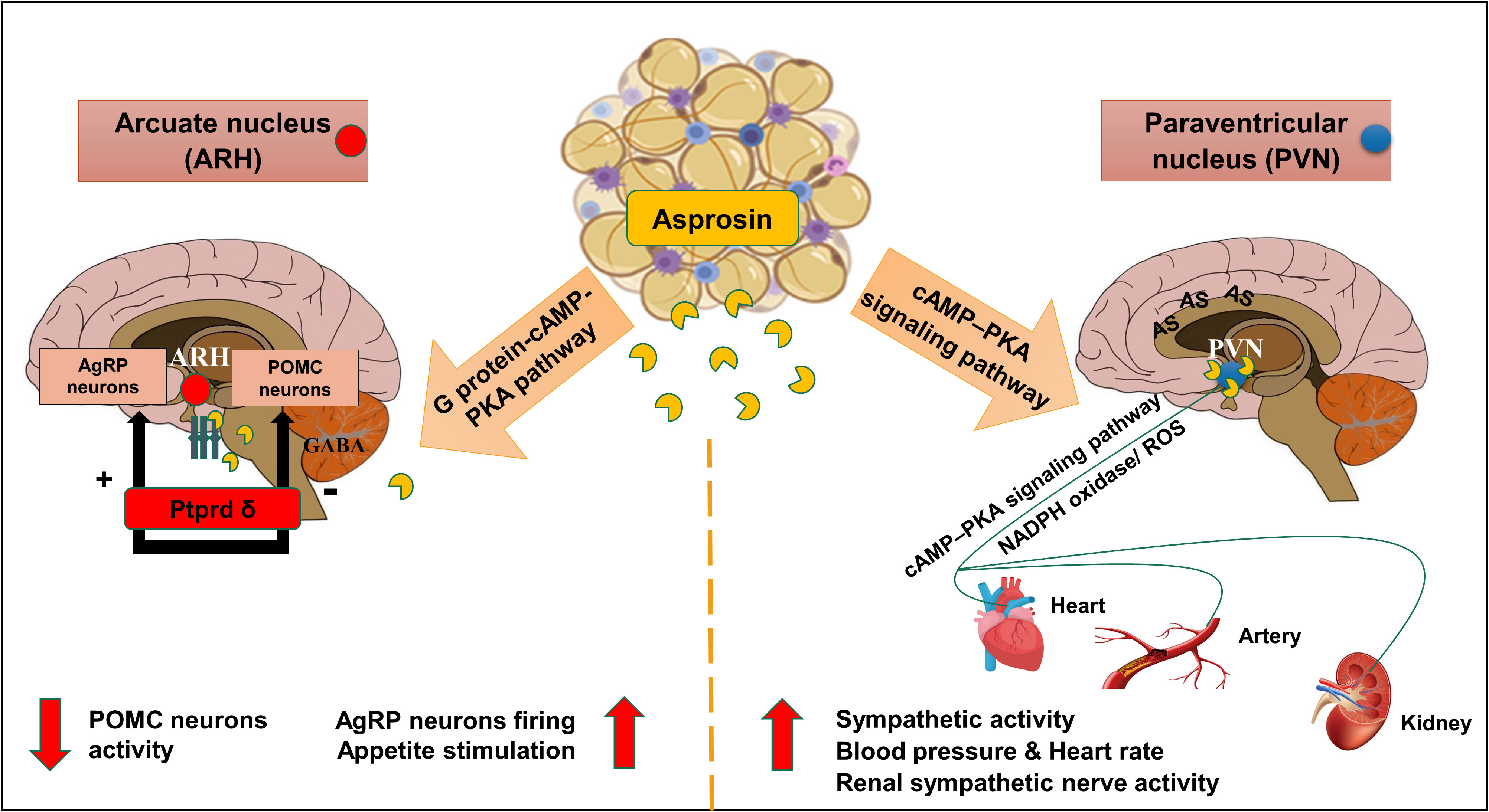
Figure 1 Schematic diagram of the central effects of asprosin in hypothalamic nuclei. Asprosin exerts its appetite stimulating effect by acting mainly via Protein tyrosine phosphatase receptor δ (Ptprd) in; Hypothalamic arcuate nucleus (ARH) causing stimulation of agouti-related protein neurons (AgRP) and inhibition of Pro-opiomelanocortin neurons (POMC). In paraventricular nucleus (PVN), asprosin increases the sympathetic outflow, blood pressure, and heart rate via promoting the cyclic adenosine monophosphate (cAMP) level, adenylyl cyclase (AC), and protein kinase A (PKA) activity mediated by nicotinamide adenine dinucleotide phosphate oxidase (NADPH oxidase) activity and superoxide production (ROS). (The illustration was created using the software: PowerPoint).
Simultaneously, asprosin was found to inhibit 85% of the appetite-suppressing neurons population pro-opiomelanocortin (POMC) in γ-Aminobutyric acid (GABA) dependent manner (13). Indeed, this inhibition can indirectly stimulate appetite, and adiposity accumulation (12, 13, 24). Recently, asprosin was found to have a high affinity to the cell surface receptor Ptprd, highly expressed in the hypothalamic AgRP neurons (23, 25). A preclinical study illustrated a significant reduction of appetite, blood glucose levels, and leanness after genetic excision of Ptprd receptors from AgRP neurons which suggested this receptor to be the orexigenic asprosin receptor (23). Notably, Ptprd membrane-bound receptors were not detected in the liver tissue which confirmed the absence of Ptprd role in the glucogenic function of asprosin (23).
As illustrated before, cAMP–PKA pathway is essential in AgRP neuronal activation that contributes to the orexigenic function of asprosin. This signaling pathway may also be implicated in the cardiovascular-related effects of asprosin. Recently, high expression levels of asprosin mRNA and protein were detected in another hypothalamic nucleus known as paraventricular nucleus (PVN) (26). Interestingly, asprosin induced the sympathetic outflow in the PVN via activation of the cAMP–PKA signaling pathway mediated by NADPH oxidase activation and subsequent reactive oxygen species (ROS) production (Figure 1) (26). Microinjection of asprosin protein in PVN of adult Sprague-Dawley rats caused increasing levels of heart rate, mean arterial pressure, and renal sympathetic nerve activity in a dose-dependent manner (26). However, neutralization of the endogenous asprosin with a specific antibody eliminated the previous asprosin-dependent actions (26).
Below the hypothalamus at the base of the brain, a master endocrine pituitary gland is located and charges several essential hormones. Abnormal excessive secretion of growth hormone from this gland due to pituitary cell adenoma develops a rare disease known as Acromegaly (AC). AC patients usually express uncontrolled glucose-lipid metabolism (insulin resistance, diabetes) with subsequent dysregulation of adipose tissue function and secretion. Patients with AC who have higher blood glucose and decreased fat mass were found to have significantly lower serum asprosin concentrations compared to healthy controls (27). Central administration of asprosin was reported to stimulate the hypothalamic-pituitary-testicular axis and significantly increase serum levels of pituitary-secreted hormones: luteinizing hormone (LH), follicle-stimulating hormone (FSH), and testosterone in rats (28).
5 Asprosin role in inflammatory response and cardiac related disease
As a characteristic feature of MetS, obesity and diabetes are closely related to severe chronic inflammation (29). In vitro studies have illustrated that asprosin may exert a pro-inflammatory role in pancreatic β-cells and skeletal muscle cells (Figure 2) (30–32). Palmitate is a saturated fatty acid that promotes IR and inflammation in peripheral tissue (33). Palmitate treatment of mouse insulinoma MIN6 cells and primary human islet caused significant expression of asprosin along with reduced insulin secretion and cell viability (30). This treatment resulted in the release of inflammatory markers such as tumor necrosis factor alpha (TNF-α) and monocyte chemoattractant protein-1 (MCP-1), activation of nuclear factor kappa-light-chain-enhancer of activated B cells (NFκB) phosphorylation, apoptosis, and impaired insulin secretion (30). Moreover, asprosin promoted inflammation, islet β-cells dysfunction and apoptosis via activation of toll-like receptor 4 (TLR4) expression and JNK phosphorylation in a dose-dependent manner (30). Furthermore, asprosin showed activation of protein kinase C-δ (PKCδ)-associated endoplasmic reticulum (ER) stress/inflammation pathways in skeletal muscle causing insulin sensitivity impairment (31). Primarily, β‐cell dysfunction affects insulin production and leads to hyperglycemia (34). Asprosin stimulated β‐cell apoptosis via increasing the expression of caspase3 and inhibiting β‐cell autophagy via disrupting the AMP-activated protein kinase (AMPK) ‐ mechanistic target of rapamycin (mTOR) pathway (35).
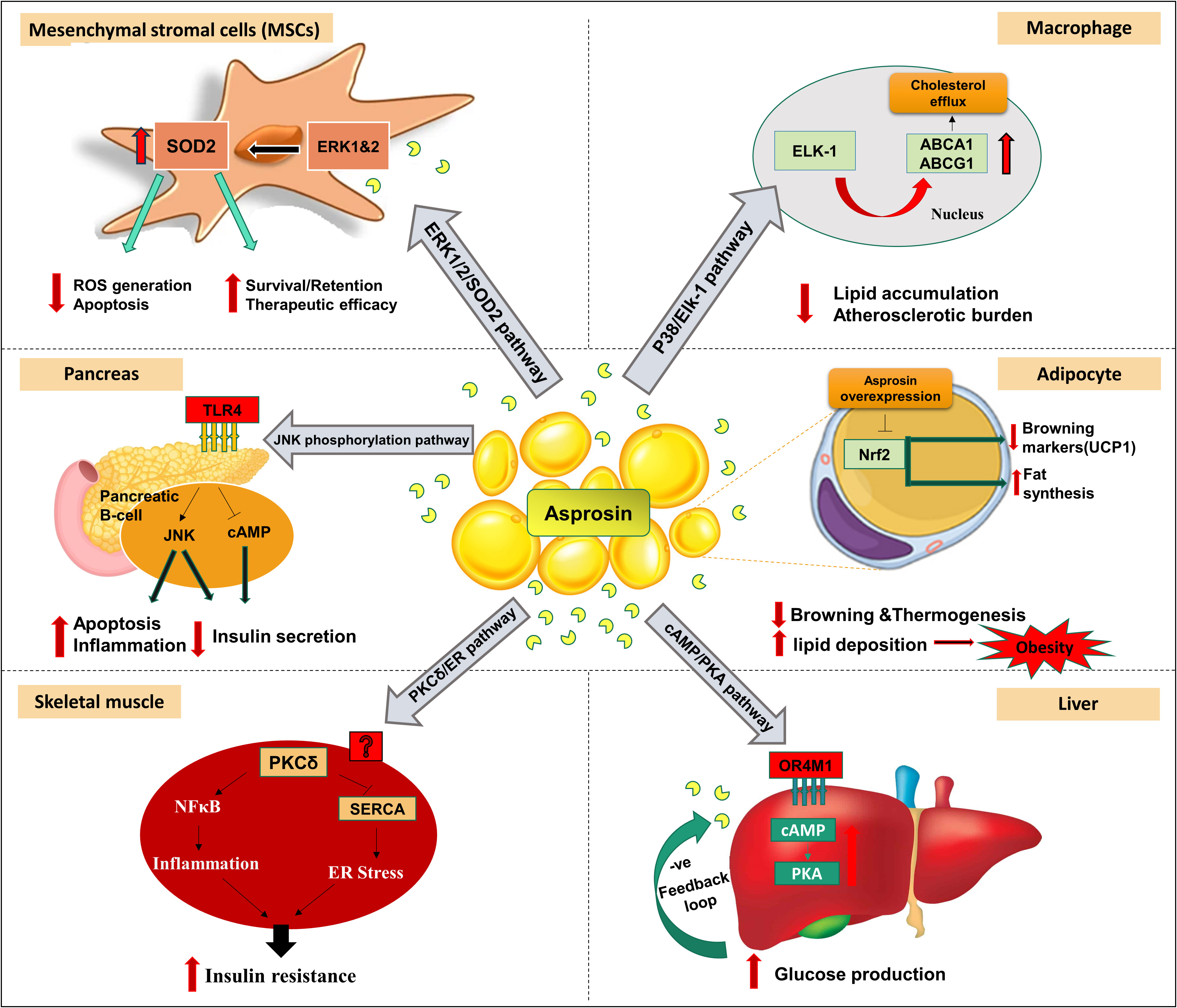
Figure 2 Signaling pathways and reported peripheral effects of asprosin in different organs, tissues, cells in vivo and in vitro models. In the liver, asprosin increased glucose production via a G-protein coupled receptor OR4M1 in the human (OLFR734 the mouse orthologue) through activation of cyclic adenosine monophosphate (cAMP)/protein kinase A (PKA) pathway. In pancreas, asprosin promotes inflammation, islet β-cells dysfunction and apoptosis via activation of toll-like receptor 4 (TLR4) expression and c-Jun N-terminal kinases (JNK) phosphorylation. In skeletal muscle caused insulin sensitivity impairment via activation of protein kinase C-delta (PKCδ)-associated endoplasmic reticulum (ER) stress/inflammation pathways. Overexpression of asprosin in adipocytes downregulated browning-specific markers (UCP1) and accelerated lipid deposition via inhibition of the nuclear factor erythroid 2–related factor 2 (Nrf2) pathway. Asprosin regulates the function and survival of mesenchymal stromal cells (MSC) against ROS generation and apoptosis via upregulation of superoxide dismutase 2 (SOD2) protein expression and the activation of extracellular signal-regulated kinase 1/2 (ERK1/2)-SOD2 pathway. Asprosin alleviated the macrophage’s inflammatory response and atherosclerotic burden via upregulating ATP-binding cassette transporters A1 (ABCA1) and ABCG1 by the stimulation of the P38/ETS-like transcription factor (Elk-1) pathway. Additional abbreviations within the figure: Sarco/endoplasmic reticulum Ca2+-ATPase (SERCA); Nuclear factor kappa-light-chain-enhancer of activated B cells (NFκB). (The illustration was created using the software: PowerPoint).
A recent study illustrated the role of asprosin in alleviating the macrophage’s inflammatory response and inducing atheroprotection effect (36). Asprosin upregulated ATP-binding cassette transporters A1 (ABCA1) and ABCG1 via the stimulation of the P38/Elk-1 pathway with subsequent intracellular cholesterol efflux (Figure 2) (36). This reduced lipid accumulation and atherosclerotic lesion progression. In addition, asprosin downregulated the expression of inflammatory cytokines Interleukin 1 beta (IL-1β) and IL-6 (36).
An in vivo study suggested that asprosin can regulate the function and survival of mesenchymal stromal cells (MSC) in improving cardiac function (myocardial infarction) (37). In the ischemic microenvironment, asprosin protected MSC against ROS generation and apoptosis (37). This cytoprotective effect was executed via upregulation of SOD2 protein expression and activating the ERK1/2-SOD2 pathway (Figure 2) (37). Similarly, a clinical study reported lower adverse cardiovascular events in dilated cardiac myopathy patients with higher asprosin levels compared to lower asprosin patients (38). Furthermore, the same investigation also revealed that, under hypoxic conditions, asprosin directly induced cardio-protective effects on H9c2 cardio myoblasts accompanied by recovery of the mitochondrial respiration process (38).
6 Asprosin role in obesity and obesity-related disorders
Obesity is considered the driving force of various adverse metabolic consequences such as IR which causes impaired glucose tolerance over time and consequently T2DM. Its prevalence increases due to the obesogenic environment upon insufficient physical activity and the disruption in both dietary intake and energy expenditure balance.
Plasma asprosin was reported to be pathologically increased in obese children and adults (18, 39–41) (Table 1). The available data regarding the role of plasma asprosin levels in childhood obesity is still limited (39, 41). Two studies documented significantly lower asprosin serum levels in obese boys relative to obese girls proposing the involvement of sex differences in asprosin effects in pediatric obesity (43, 44). Conversely, Silistre and coworkers observed significantly higher serum levels of asprosin in Turkish obese children with no significant differences regarding gender variability which agrees with data from Wang et al. on obese adults (40, 41).
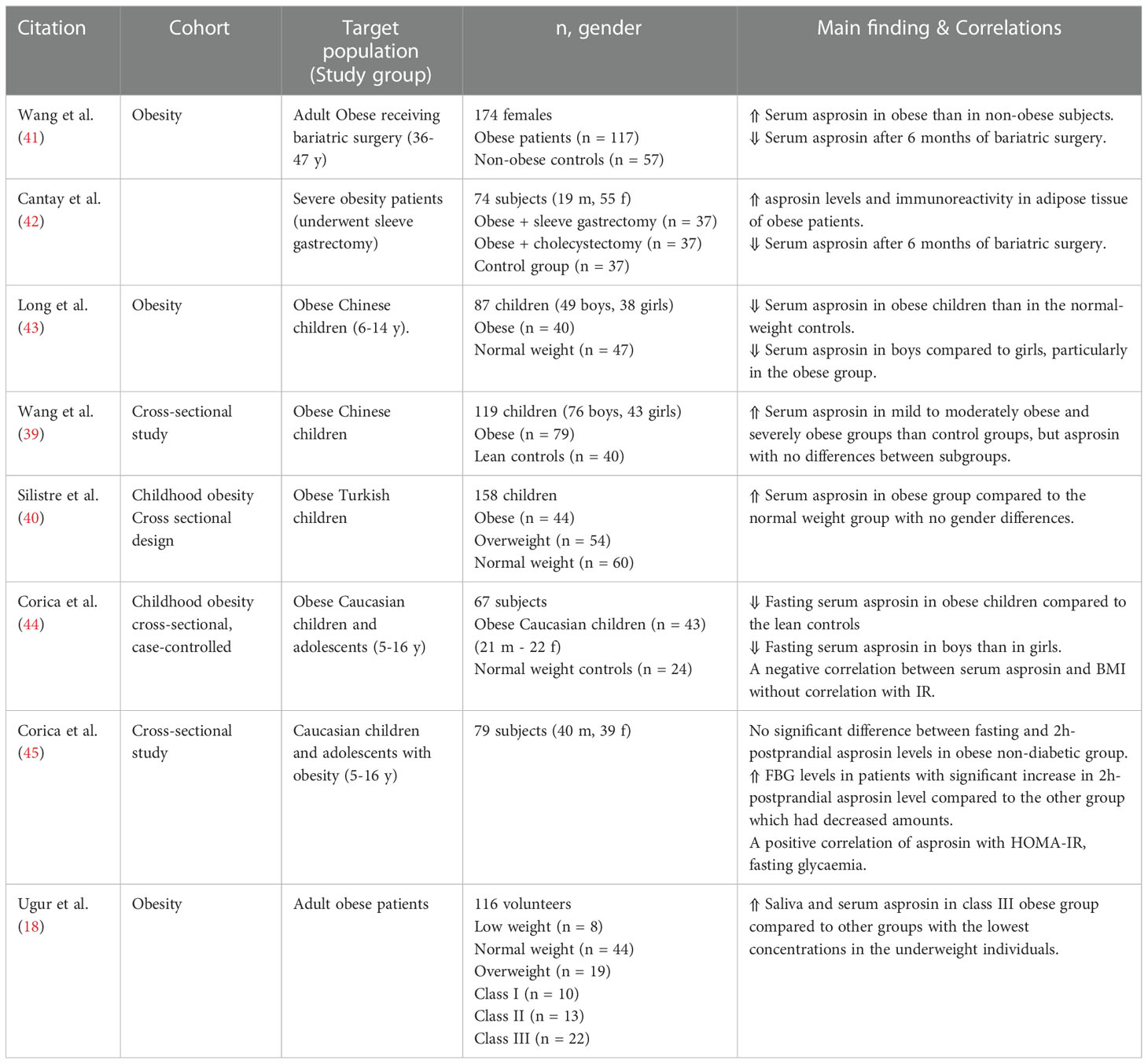
Table 1 Main findings of clinical trials investigating circulating asprosin levels and correlations in obesity.
A positive correlation between asprosin concentration and the homeostasis model of IR (HOMA-IR) has been observed (39, 41, 46, 47). Increased circulating asprosin levels were reported in IR obese children compared to the non-IR obese group (39), however, another study reported a negative correlation (43). Notably, salivary and blood asprosin concentrations were found elevated in class III obese subjects compared to the underweight group (18).
Prader–Willi syndrome (PWS) is a rare genetic disease that mainly affects the child’s metabolism and is characterized by uncontrolled feeding behavior with the development of severe obesity (48). A recent study found no differences in the asprosin fasting levels in children with PWS compared to overweight and standard weight groups (49). The same study also observed no significant differences between fasting and postprandial plasma asprosin levels in PWS children and the normal body mass index (BMI) controls (49). This finding agrees with a previous study which found increased fasting blood glucose (FBG) levels, but not the asprosin, in obese non-diabetic children who had a significant increase in 2h-postprandial asprosin level (45).
There is evidence suggesting an association between asprosin secreted levels and weight loss extent caused by bariatric surgery such as sleeve gastrectomy or cholecystectomy. Two studies reported significant decrease of serum asprosin levels after six months of weight loss surgical intervention (41, 42). Concentrations of fasting asprosin were significantly higher in obese individuals than in normal BMI subjects with no significant sex differences (41). Notably, obese group was found to express high asprosin levels and immunoreactivity in adipose tissue (42).
Regular exercise training remains the best and the safest non-pharmacological way for obesity management and avoidance. A preclinical study on experimental T1DM rats injected with 65 mg/kg intraperitoneal single dose of streptozotocin (STZ) found decreased levels of asprosin, PKA, and transforming growth factor-β (TGF-β), however, elevated AMPK and protein kinase B (AKT) levels in the liver under aerobic exercise training (50). Thus, aerobic exercise might modulate the hepatic asprosin-dependent downstream signaling pathways (cAMP/PKA) that lead to adjustment of the hepatic glucose release in T1DM (50). Additionally, the previously detected lowering impact of aerobic exercise on plasma asprosin and insulin levels was observed after morning and evening training in either standard weight or overweight and obese groups (51). Furthermore, a significant decrease in the blood asprosin and waist-hip ratio was observed in young women with abdominal obesity and metabolic diseases after two months of “Nordic” walking training program (52). Similar findings were noticed in obese sedentary men upon ending three months of different resistance training methods (traditional, circular, and interval) (53).
Asprosin hormone is closely related to non-alcoholic fatty liver disease (NAFLD), although the exact role remains unclear. NAFLD is the most common chronic liver complication among adult and pediatric obese subjects (Table 2). NAFLD Patients are usually susceptible to increased risk of IR and diabetes (59). The plasma asprosin levels were notably higher in obese Chinese children with NAFLD than the lean group with significant differences in liver enzymes alanine aminotransferase (ALT) and aspartate aminotransferase (AST) (55). Similarly, increased serum asprosin levels were detected in NAFLD adults (age > 18) with a positive correlation to HOMA-IR, FBG, triglycerides (TG), and serum albumin (ALB) (54).
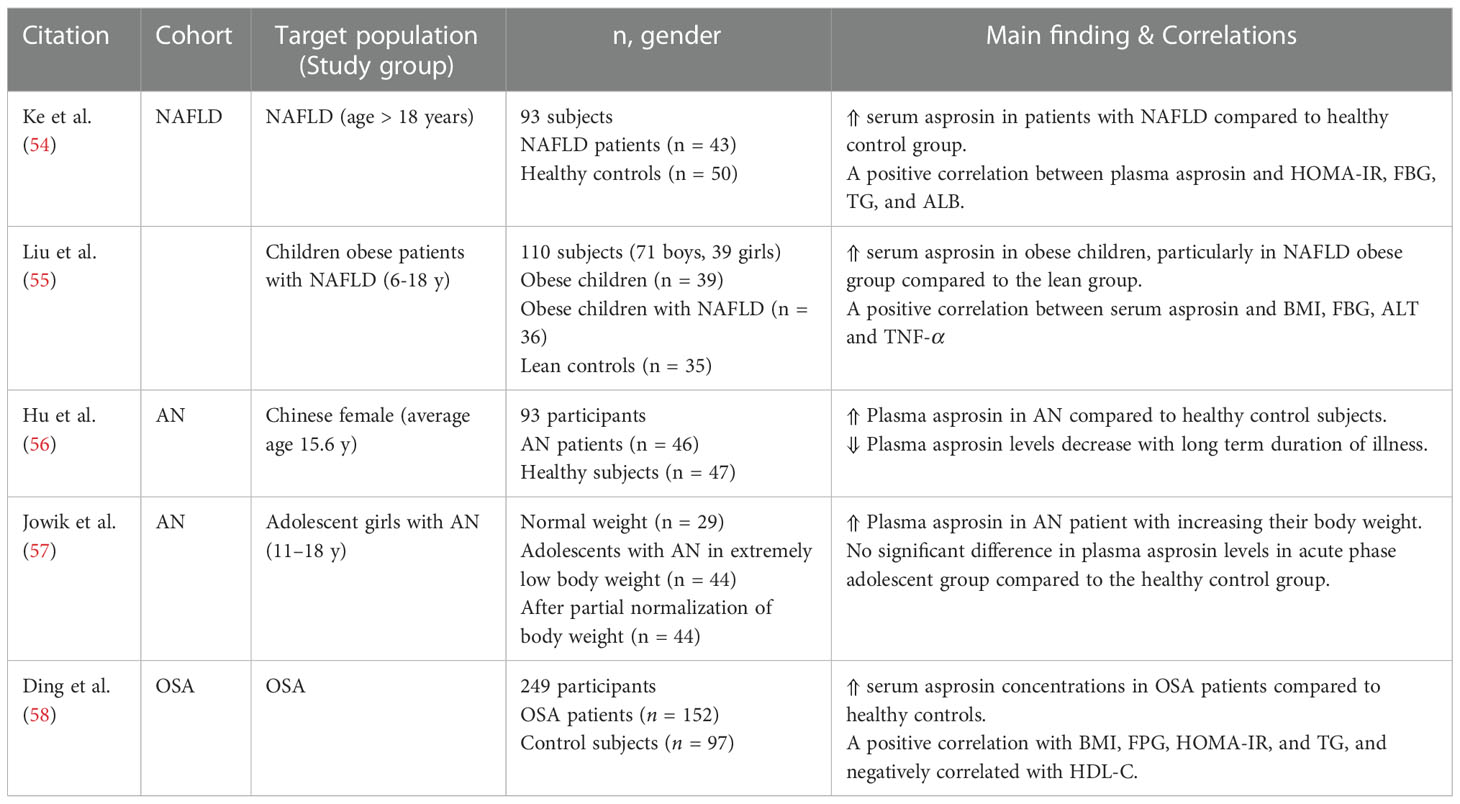
Table 2 Main findings of clinical trials investigating circulating asprosin levels and correlations in obesity related diseases.
Obesity is a shared risk factor between metabolic diseases and a common sleeping disorder known as obstructive sleep apnea (OSA) (60). The prevalence of OSA is estimated to be more than 50% among obese patients, particularly those who have abdominal and central fat deposition (61). One clinical study including 191 male population diagnosed with OSA found significantly increased serum asprosin concentrations compared with healthy control subjects (Table 2) (58).
On the other hand, plasma asprosin levels in patients with anorexia nervosa (AN) who suffer from self-induced extreme weight loss and malnutrition were increased compared to the corresponding healthy controls (Table 2) (56). These higher levels were decreased with long term deficient energy intake and depletion of body fat (56). Similarly, a recent study observed that plasma asprosin in AN acute phase were elevated with increasing body weight and positively correlated to the eating disorder symptoms (57). However, the same study reported no significant differences in the plasma asprosin between adolescents in the acute phase and the corresponding healthy controls (57).
7 Asprosin in type 2 diabetes mellitus
Elevated asprosin levels are associated with insulin resistance and T2DM (Table 3). Up to now, numerous clinical studies have ascertained increased plasma asprosin concentrations among pre-diabetic cases and T2DM patients (62–65). A hospital-based observational study comprised of 170 individuals showed a significant elevation in the serum asprosin in newly diagnosed T2DM adult patients compared to the normal glucose tolerance individuals (62). These findings were independently correlated to triglyceride levels, FBG levels, and adiposity-related parameters: BMI, waist circumference (WC), and waist-to-height ratio (WHR) in T2DM and persons without diabetes (62). Li et al. data were also in agreement with the previous study but with no association with plasma triglyceride levels (65).
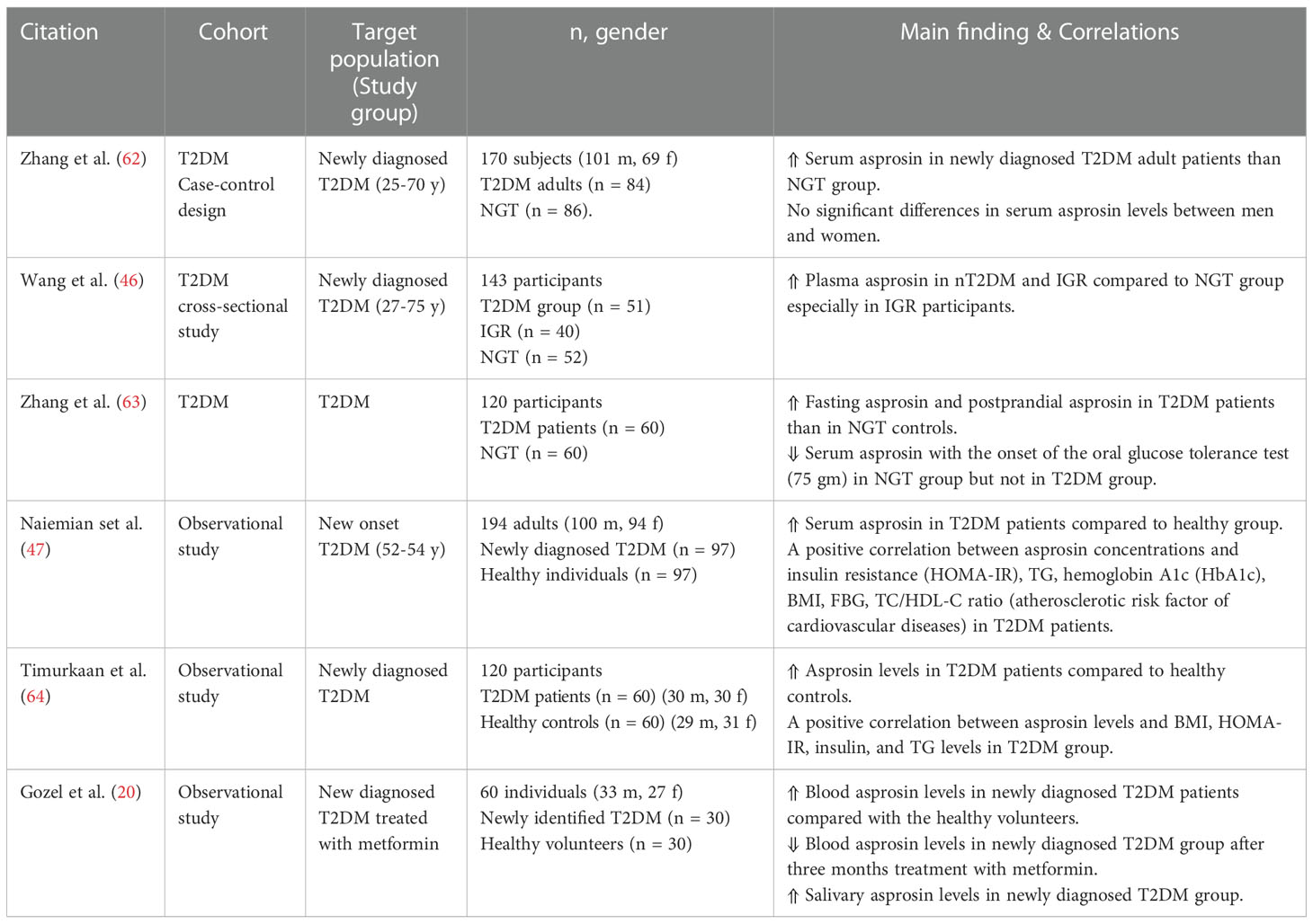
Table 3 Main findings of clinical trials investigating circulating asprosin levels and correlations in T2DM.
A cross-sectional study demonstrated significantly elevated plasma asprosin levels in newly diagnosed T2DM cases and impaired glucose regulation (IGR) compared to the healthy controls with no sex or age differences (46). Notably, plasma asprosin concentrations correlated positively with HOMA-IR, blood glucose, and WC (46). Another study also reported considerably higher serum asprosin levels in T2DM patients during fasting and postprandial events than in normal glucose tolerance (NGT) subjects (63). In addition, the postprandial asprosin concentrations seemed to be lower than the fasting levels in the NGT group (63). The same study also reported a negative correlation of serum asprosin levels with blood glucose after 75 g oral glucose administration in the NGT group but not in T2DM (63). These findings indicated an apparent response of asprosin to glucose variations in T2DM patients that may contribute to T2DM onset. Furthermore, Significant elevation of the asprosin concentration was found in old diagnosed T2DM Iraqi patients but not in the newly diagnosed cases (66). Besides, newly diagnosed T2DM patients treated for three months with metformin or 24 weeks with 10 mg/day sodium-glucose co-transporter-2 (SGLT2) inhibitor called dapagliflozin showed reduced levels of plasma asprosin (20).
8 Asprosin in diabetic complications
Literature data showed a potential link between high circulating asprosin and uncontrolled diabetes that usually displays microvascular diabetic complications such as nephropathy, retinopathy, and peripheral neuropathy (Table 4). As expected, circulating asprosin levels were significantly enhanced in T2DM patients with diabetic complications (67, 68, 70, 71). Diabetic nephropathy (DN) (also known as diabetic kidney disease), a common severe complication of both types of diabetes mellitus, is considered a major cause of end-stage renal failure that seriously affects diabetic patients’ quality of life (76). Various clinical studies reported a significant association between elevated serum asprosin levels and DN (67, 69, 70). Zhang et al. measured the circulating asprosin levels in three groups: NGT, T2DM group with early-stage DN, and T2DM without DN (67). Notably, plasma asprosin was increased in DN and non-DN patients compared to the NGT group with the highest amount in the diabetic patients suffering from DN (67). In addition, asprosin concentrations seem closely related to the clinical parameters of the DN early stages: estimated glomerular filtration rate (e-GFR) and the urine albumin-creatinine ratio (UACR) (67). A positive correlation was found between asprosin plasma levels and UACR suggesting asprosin as an early clinical indicator for DN progression (68). These data seem to be consistent with a recent study in which a significant higher asprosin levels were reported in T2DM patients with macroalbuminuria than both microalbuminuria group and patients who had no signs of albuminuria among both men and women (77).
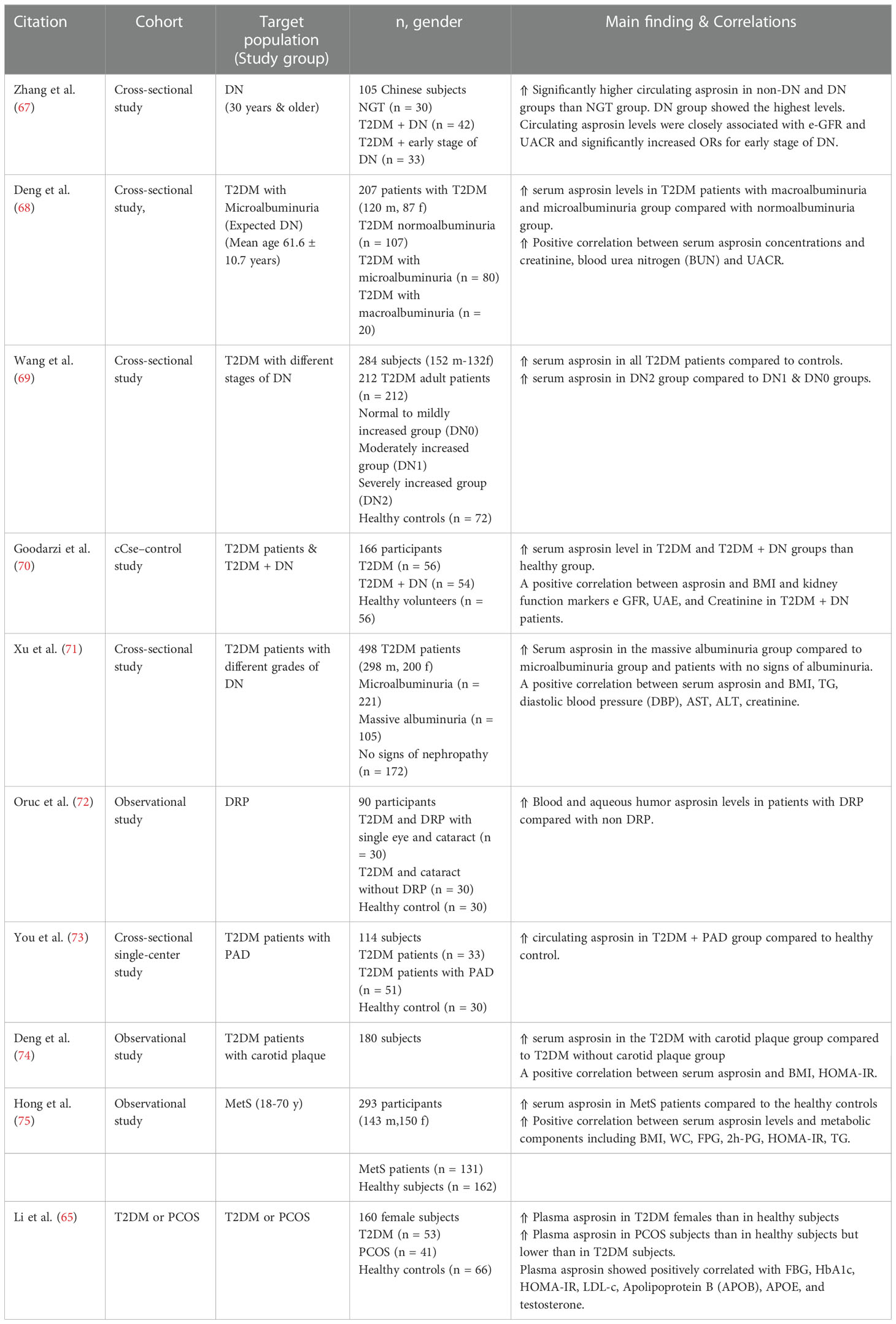
Table 4 Main findings of clinical trials investigating circulating asprosin levels and correlations in diabetic complications.
T2DM patients are usually subjected to high-risk of atherosclerotic plaques (74). Notably, a significant asprosin levels were detected in T2DM patients with carotid plaque compared to non-carotid plaque T2DM group (74). Furthermore, elevated plasma asprosin levels was reported in T2DM patients suffering from diabetic lower extremity peripheral artery disease (PAD) (73). Asprosin induced TGF-β signaling pathway activation that directly affects endothelial-to-mesenchymal transition (EndMT) (73).
Diabetic retinopathy (DRP), one major common cause of vision loss in diabetic people, was also found to be associated with circulating asprosin levels. DRP patients have been found to exhibit higher serum fasting asprosin values compared to non-diabetic retinopathy individuals or non-complicated cases of T2DM patients (72). Oruc et al. reported a significant increase in levels of serum asprosin and particular markers for oxidative stress: 4-hydroxynonenal (4-HNE) and 8-hydroxy-2”-deoxyguanosine (8-OHdG) in DRP patients (72). Interestingly, researchers have found a link between the asprosin production and central serous chorioretinopathy (CSCR), another retinopathic, yet non-diabetic, condition. It is an idiopathic retinal neurosensory disorder characterized by macular detachment with subsequent atrophy and unilateral vision loss (78). CSCR individuals exhibit a corrupted antioxidant defense system with a significant reduction of plasma antioxidative parameters (TAC and DHEA-S) (79). Celik and his team found significantly increased asprosin serum concentration in individuals with CSCR compared to the healthy subjects suggesting that this molecule might be implicated in the pathogenesis of CSCR (80).
In vivo study revealed decreased asprosin levels in a mouse model of neuropathic pain compared to healthy controls. In addition, treating these mice with recombinant asprosin yielded an anti-hypersensitivity effect suggesting an analgesic function of asprosin (81).
9 Asprosin in obstetrics and gynecology
During pregnancy, different adipokines are synthesized and secreted from the cord blood and placenta such as leptin, resistin and adiponectin having great effects on fetus development and metabolism (82, 83). In addition, maternal-related pathologies such as preeclampsia (PE) and gestational diabetes mellitus (GDM) are closely related to pre-gestational and gestational obesity (84, 85). Few published studies have addressed the role of asprosin during pregnancy and other gynecologic disorders (Table 5) (22). Asprosin concentration showed a possible impact on the incidence of diabetes developed during gestation.
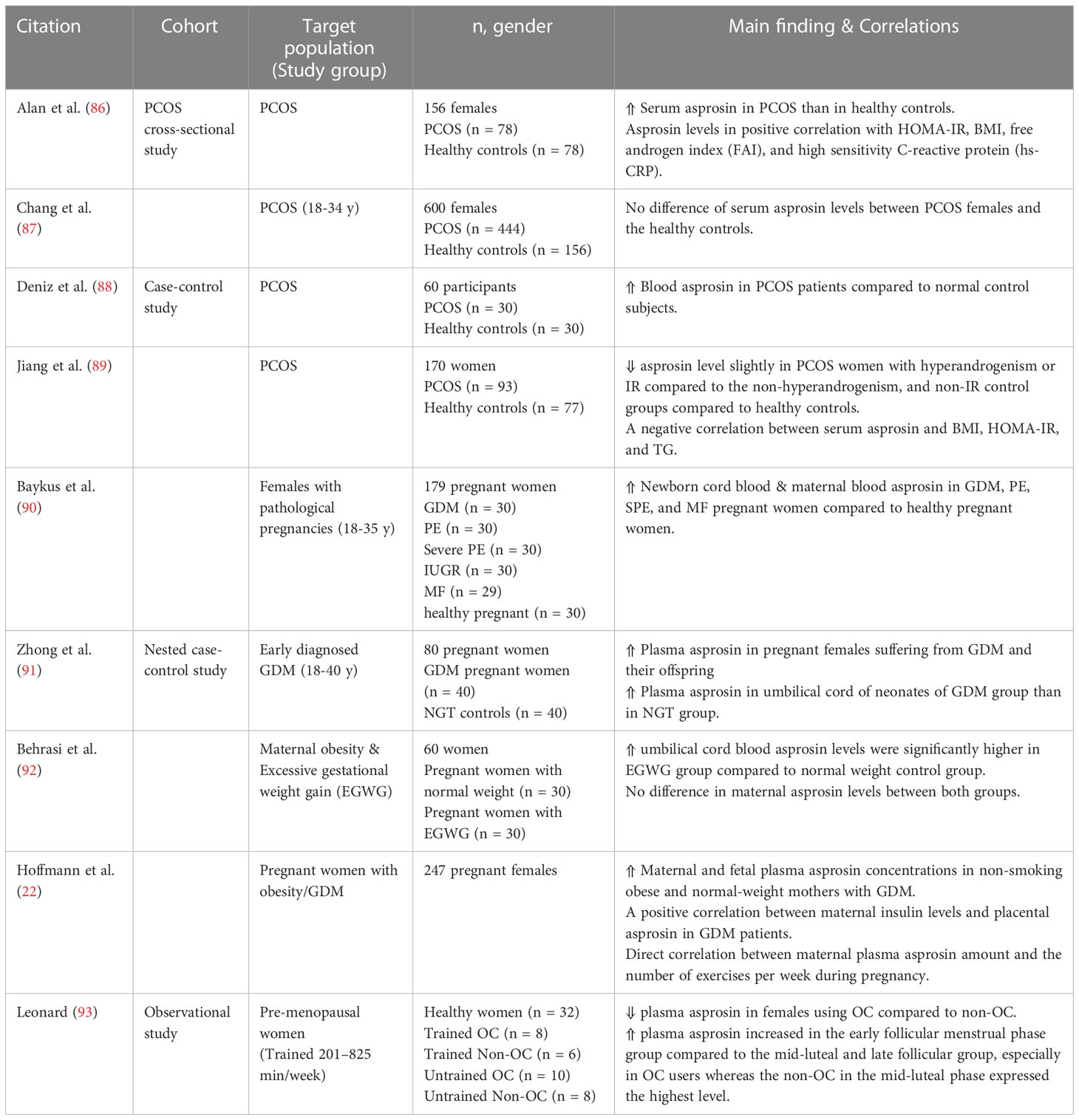
Table 5 Main findings of clinical trials investigating circulating asprosin levels and correlations in obstetrics and gynecology.
Baykus and coworkers investigated the asprosin levels in newborn umbilical cord blood and maternal blood in 149 female patients with different forms of pathological pregnancies (90). They found a statistically significant increase in newborn cord blood and maternal blood asprosin levels in abnormal pregnancies encompassing GDM, PE, severe PE, and macrosomic fetuses (MF) compared with healthy pregnant women. However, the intrauterine growth retardation cases showed reduced asprosin levels (90). These observations might suggest that increased asprosin concentrations in pathological pregnancies could be a predisposing factor for PE and severe PE in pregnant women as it contributes to glucose homeostasis imbalance. Following the previous findings, another study also found elevated asprosin plasma levels in pregnant females suffering from GDM and their offspring (91).
Hoffmann et al. found in a placenta cohort study on 247 pregnant females a strong correlation between different metabolic parameters (obesity and GDM) and asprosin plasma levels and distribution in placental cells and tissues (22). Notably, this study reported significant asprosin concentrations in maternal and fetus plasma in non-smoking obese and normal-weight mothers with GDM (22). Interestingly, there was a direct correlation between maternal plasma asprosin amount and the number of exercises per week during pregnancy (22). Asprosin levels were also found significantly higher in the umbilical cord blood of pregnant women with maternal obesity than in the normal weight control group with no difference in maternal asprosin concentrations (92).
Moreover, some studies have speculated that obesity, in particular the central obesity, may be the primary metabolic insult in a common reproductive endocrinopathy mainly affecting women in childbearing age known as polycystic ovarian syndrome (PCOS) (94). Up to 70% of PCOS women markedly express obesity and IR which is considered as vital risks for PCOS pathogenesis (95). Indeed, PCOS is a key risk factor for other metabolic comorbidities such as cardiovascular diseases, T2DM, GDM, endometrial cancer as well as pregnancy-related complications (96).
The potential impact of asprosin in the etiology of PCOS is still controversial. Some studies addressed elevated levels of circulating asprosin in women with PCOS compared to the control subjects (65, 86, 88). In contrast, a cohort study of 444 women suffering from PCOS (18 to 34 years old) reported non-significant difference in the circulating asprosin between PCOS patients and the corresponding controls (87). A recent study reported partly congruent findings in which slightly lower asprosin levels were found in PCOS women with hyperandrogenism or IR compared to the non-hyperandrogenism and non-IR control groups (89).
Fasting plasma asprosin levels in pre-menopausal women was investigated during different stages of the menstrual cycle (early follicular, late follicular, and mid-luteal) (93). The findings demonstrated a reduction of fasting plasma asprosin in females using oral contraceptives (OC) compared to non-users (93). In addition, plasma asprosin increased in the early follicular menstrual phase compared to the mid-luteal and late follicular, especially in OC users whereas the non-OC users in the mid-luteal phase expressed the highest level (93). Hence, the menstrual cycle phase and the use of OC appeared important to be considered throughout investigating the concentration of circulating asprosin in women (93).
10 Asprosin in cancer
Currently, the view of cancer as a genetic condition has gradually been replaced by considering it a metabolic case. Emerging evidence supports a general hypothesis that cancer is a disease of impaired cellular energy metabolism regardless of its cellular or tissue origin (97). Cancer cells exhibit distinctive characteristics through different reprogrammed metabolic activities involving rapid glucose uptake and lipid metabolism dysregulation (98). Typically, Warburg effect represents the central adaptation mechanism to support the growth and the survival of tumor cells. In this circumstance, even under aerobic glycolysis, the enhanced rate of glucose uptake accompanied with preferential lactate production allows uncontrolled proliferation and maintenance of the tumor microenvironment (99).
Research studies suggested that many adipokines may promotes tumorigenesis and cancer progression through the enhancement of cell migration and proliferation and other anti-apoptosis pathways (100). However, only a few data are available regarding levels of the asprosin peptide in cancer. Interestingly, Kerslake et al. identified high expression levels of asprosin and its conjugate olfactory receptor OR4M1 in normal human ovaries and malignant ovarian tissues revealing its implication in the tumor microenvironment (21). Recently, another in vitro study of the same group reported differential regulation of genes following 100 nM asprosin treatment of the ovarian cancer cell line SKOV-3 (101). Additionally, asprosin induced ERK1/2 phosphorylation and altered numerous signaling pathways associated with cell communication and proliferation (101). The functionally expressed genes such as Cadherin 11 (CDH11) and Fc fragment of immunoglobulin G receptor IIa (FCGR2A) were previously reported to be implicated in the regulating of metastatic progression and chemotherapeutic response in different cancers and may also have an influence on Warburg effect and tumor microenvironment in ovarian cancer (101–103).
Kocaman et al. observed enhanced expression and immunoreactivity of asprosin in a paraffin embedded specimens of rare surface tumor known as malignant mesothelioma (MM) compared to reactive mesothelial hyperplasia (RMH) specimens as a control group (104). The same author also suggested in a recent study the possibility of using asprosin in the differentiation between two types of skin cancer which originate from hair follicles called basal cell carcinoma (BBC) and hair follicle trichoblastoma (105). Asprosin immunoreactivity is also significantly higher in BCC samples with no expression illustrated in trichoblastoma samples (105).
As mentioned before, asprosin causes hyperinsulinemia and IR which in turn could affect oxidant-antioxidant balance and increase the expression of Insulin-like growth factor 1 (IGF-1) (12, 46) which promotes cancer development and metastasis (106).
As cancer progresses, the majority of cancer patients might develop cancer-associated malnutrition termed cachexia syndrome (CACS) with prevalence rates varying from 9% to 85% based on the type and stage of the tumor causing short-term survival and impaired quality of life (107). It is characterized by systemic inflammation, energy imbalance, and involuntary loss of lean body mass, with or without adipose tissue wasting (108). During anorexia, adipose tissue depletes in response to nutritional deficit, but a degeneration of adipose tissue and skeletal muscle occurs in CACS (109). Significantly lower asprosin levels were observed in cancer patients suffering from anorexia, but not CACS, than in non-anorexic subjects (110). More research is needed to clarify the possible role of asprosin in cancer pathogenesis and progression.
11 Conclusion
Asprosin is a novel glucose sensor secreted mainly by white adipose tissue during fasting and has multifaceted metabolic roles either peripherally or centrally. The 3’ truncated mutation in the FBN1 gene leads to deficient asprosin expression and reduces the maintenance of healthy fat tissue. FBN1 gene, in line with its expressed protein asprosin, could exert different effects on health and diseased states. In the hypothalamus, asprosin activates two nuclei ARH and PVN triggering appetite stimulation and sympathetic flow, respectively. Peripherally, asprosin directly affects insulin signaling causing increased insulin resistance, inflammation, and endoplasmic reticulum stress. Since its discovery, asprosin has provided new insights into clinical diagnostic practice, particularly in higher-prevalence chronic diseases such as obesity and T2DM. Pathologically elevated asprosin levels might be a potential biomarker for early alteration in glucose metabolism in obese and diabetic cases. However, there are inconsistencies between the asprosin values of both obese children and obese adults. Besides, higher levels of asprosin could be a risk factor for developing diabetic complications such as diabetic nephropathy and retinopathy. As mentioned before, asprosin was positively correlated with insulin resistance, obesity, cholesterol metabolism, renal function, and inflammatory markers. Taken together, these data suggest a potential role for this adipokine in the metabolic process. All these findings open the doors for asprosin to be a new potential therapeutic target in an array of metabolic-related pathologies. However, more comprehensive studies are needed to explore the exact molecular mechanisms underlying the diverse effects of asprosin in physiological and pathological events.
Author contributions
“Conceptualization, OG, DE, and MF; methodology, OG and AM, and MC; validation, FL, JP, and MG-G.; writing—original draft preparation, MF and YF; writing—review and editing, OG, MF, and YF; visualization, MF, MG-R, and AC-B; supervision, OG, YF, AS, and AE; funding acquisition, OG, JP, and FL. All authors contributed to the article and approved the submitted version.
Funding
OG and FL are Staff Personnel (I3SNS Stable Researcher) of Xunta de Galicia (Servizo Galego de Saude (SERGAS)) through a research-staff contract (ISCIII)/SERGAS). OG and MG-G are members of RICORS Program, RD21/0002/0025 via ISCIII and FEDER. FL is a member of Centro de Investigación Biomédica en Red de Enfermedades Cardiovasculares (CIBERCV). The work of OG and JP (PI20/00902), and FL (PI21/01145 and CB16/11/00226) is funded by ISCIII and FEDER. OG is a beneficiary of a project funded by the Research Executive Agency of the European Union in the framework of MSCA- RISE Action of the H2020 Program (project number 734899). OG is the beneficiary of a grant funded by Xunta de Galicia, Consellería de Educación, Universidade e Formación Profesional and Consellería de Economía, Emprego e Industria (GAIN) (GPC IN607B2022/03). MG-R is a recipient of a predoctoral contract funded by Xunta de Galicia (IN606A-2020/010). ISCIII and FEDER funded through a predoctoral research scholar to CR-F (Exp.18/00188). AC-B is a recipient of a predoctoral contract funded by Secretaría de Estado de Universidades, Investigación, Desarrollo e Innovación, Ministerio de Universidades (FPU2018- 04165). YF is a ‘Sara Borrell’ researcher funded by ISCIII and FEDER [CD21/00042]. The funders were not involved in study design, data collection and analysis, decision to publish, or manuscript preparation.
Conflict of interest
The authors declare that the research was conducted in the absence of any commercial or financial relationships that could be construed as a potential conflict of interest.
Publisher’s note
All claims expressed in this article are solely those of the authors and do not necessarily represent those of their affiliated organizations, or those of the publisher, the editors and the reviewers. Any product that may be evaluated in this article, or claim that may be made by its manufacturer, is not guaranteed or endorsed by the publisher.
Glossary
References
1. Kershaw EE, Flier JS. Adipose tissue as an endocrine organ. J Clin Endocrinol Metab (2004) p:2548–56. doi: 10.1210/jc.2004-0395
2. Tilg H, Moschen AR. Adipocytokines: Mediators linking adipose tissue, inflammation and immunity. Nat Rev Immunol (2006) 6:772–83. doi: 10.1038/nri1937
3. Cornier MA, Dabelea D, Hernandez TL, Lindstrom RC, Steig AJ, Stob NR, et al. The metabolic syndrome. Endocr Rev (2008) 29:777–822. doi: 10.1210/er.2008-0024
4. Cao H. Adipocytokines in obesity and metabolic disease. J Endocrinol (2014) 220:T47–T59. doi: 10.1530/JOE-13-0339
5. Guh DP, Zhang W, Bansback N, Amarsi Z, Birmingham CL, Anis AH. The incidence of co-morbidities related to obesity and overweight: A systematic review and meta-analysis. BMC Public Health (2009) 9:1–20. doi: 10.1186/1471-2458-9-88
6. Sun K, Kusminski CM, Scherer PE, Sun K, Kusminski CM, Scherer PE. Adipose tissue remodeling and obesity find the latest version: Review series adipose tissue remodeling and obesity. The Journal of Clinical Investigation (2011) 121:2094–101. doi: 10.1172/JCI45887.2094
7. Blüher M. Adipose tissue dysfunction in obesity. Exp Clin Endocrinol Diabetes (2009) 117:241–50. doi: 10.1055/s-0029-1192044
8. Czech MP. Insulin action and resistance in obesity and type 2 diabetes. Nat Med (2017) 23:804–14. doi: 10.1038/nm.4350
9. Bays H, Ballantyne C. Adiposopathy: why do adiposity and obesity cause metabolic disease? Future Lipidol (2006) 1:389–420. doi: 10.2217/17460875.1.4.389
10. González-rodríguez M, Ruiz-fernández C, Cordero-barreal A, Eldjoudi DA, Pino J, Farrag Y, et al. Adipokines as targets in musculoskeletal immune and inflammatory diseases. Drug Discovery Today (2022) (2022):103352. doi: 10.1016/j.drudis.2022.103352
11. Basu B, Jain M, Chopra AR. Caudamins, a new subclass of protein hormones. Trends Endocrinol Metab (2021) 32:1007–14. doi: 10.1016/j.tem.2021.09.005
12. Romere C, Duerrschmid C, Bournat J, Constable P, Jain M, Xia F, et al. Asprosin, a fasting-induced glucogenic protein hormone. Cell (2016) 165:566–79. doi: 10.1016/j.cell.2016.02.063
13. Duerrschmid C, He Y, Wang C, Li C, Bournat JC, Romere C, et al. Asprosin is a centrally acting orexigenic hormone. Nat Med (2017) 23:1444–53. doi: 10.1038/nm.4432
14. Mishra I, Duerrschmid C, Ku Z, He Y, Xie W, Silva ES, et al. Asprosin-neutralizing antibodies as a treatment for metabolic syndrome. Elife (2021) 10:1–21. doi: 10.7554/ELIFE.63784
15. Li E, Shan H, Chen L, Long A, Zhang Y, Liu Y, et al. OLFR734 mediates glucose metabolism as a receptor of asprosin. Cell Metab (2019) 30:319–328.e8. doi: 10.1016/j.cmet.2019.05.022
16. Miao Y, Qin H, Zhong Y, Huang K, Rao C. Novel adipokine asprosin modulates browning and adipogenesis in white adipose tissue. J Endocrinol (2021) 249:83–93. doi: 10.1530/JOE-20-0503
17. Kocaman N, Kuloğlu T. Expression of asprosin in rat hepatic, renal, heart, gastric, testicular and brain tissues and its changes in a streptozotocin-induced diabetes mellitus model. Tissue Cell (2020) 66. doi: 10.1016/j.tice.2020.101397
18. Ugur K, Aydin S. Saliva and blood asprosin hormone concentration associated with obesity. Int J Endocrinol (2019) 2019. doi: 10.1155/2019/2521096
19. Morcos YAT, Lütke S, Tenbieg A, Hanisch FG, Pryymachuk G, Piekarek N, et al. Sensitive asprosin detection in clinical samples reveals serum/saliva correlation and indicates cartilage as source for serum asprosin. Sci Rep (2022) 12:1340. doi: 10.1038/s41598-022-05060-x
20. Gozel N, Kilinc F. Investigation of plasma asprosin and saliva levels in newly diagnosed type 2 diabetes mellitus patients treated with metformin. Endokrynol Pol (2021) 72:37–43. doi: 10.5603/EP.a2020.0059
21. Kerslake R, Hall M, Vagnarelli P, Jeyaneethi J, Randeva HS, Pados G, et al. A pancancer overview of FBN1, asprosin and its cognate receptor OR4M1 with detailed expression profiling in ovarian cancer. Oncol Lett (2021) 22:1–14. doi: 10.3892/ol.2021.12911
22. Hoffmann T, Morcos YAT, Janoschek R, Turnwald EM, Gerken A, Müller A, et al. Correlation of metabolic characteristics with maternal, fetal and placental asprosin in human pregnancy. Endocr Connect (2022) 11:1–26. doi: 10.1530/EC-22-0069
23. Mishra I, Xie WR, Bournat JC, He Y, Wang C, Silva ES, et al. Protein tyrosine phosphatase receptor δ serves as the orexigenic asprosin receptor. Cell Metab (2022) 34:549–563.e8. doi: 10.1016/j.cmet.2022.02.012
24. Sohn JW. Network of hypothalamic neurons that control appetite. BMB Rep (2015) 48:229–33. doi: 10.5483/BMBRep.2015.48.4.272
25. Henry FE, Sugino K, Tozer A, Branco T, Sternson SM. Cell type-specific transcriptomics of hypothalamic energy-sensing neuron responses to weight-loss. Elife (2015) 4:1–30. doi: 10.7554/eLife.09800
26. Wang XL, Wang JX, Chen JL, Hao WY, Xu WZ, Xu ZQ, et al. Asprosin in the paraventricular nucleus induces sympathetic activation and pressor responses via cAMP-dependent ROS production. Int J Mol Sci (2022) 23:1–14. doi: 10.3390/ijms232012595
27. Ke X, Duan L, Gong F, Zhang Y, Deng K, Yao Y, et al. Serum levels of asprosin, a novel adipokine, are significantly lowered in patients with acromegaly. Int J Endocrinol (2020) 2020. doi: 10.1155/2020/8855996
28. Keskin T, Erden Y, Tekin S. Intracerebroventricular asprosin administration strongly stimulates hypothalamic-pituitary-testicular axis in rats. Mol Cell Endocrinol (2021) 538:111451. doi: 10.1016/j.mce.2021.111451
29. Donath MY, Shoelson SE. Type 2 diabetes as an inflammatory disease. Nat Rev Immunol (2011) 11:98–107. doi: 10.1038/nri2925
30. Lee T, Yun S, Jeong JH, Jung TW. Asprosin impairs insulin secretion in response to glucose and viability through TLR4/JNK-mediated inflammation. Mol Cell Endocrinol (2019) 486:96–104. doi: 10.1016/j.mce.2019.03.001
31. Jung TW, Kim HC, Kim HU, Park T, Park J, Kim U, et al. Asprosin attenuates insulin signaling pathway through PKCδ-activated ER stress and inflammation in skeletal muscle. J Cell Physiol (2019) 234:20888–99. doi: 10.1002/jcp.28694
32. Hashmi D, Shaikhali Y, Cassin-Scott R, Eng P, Distaso W, Tan T, et al. Predictors of adverse outcomes in COVID-19: A retrospective cohort study comparing the first two waves of COVID-19 hospital admissions in London, with a focus on diabetes. Endocr Abstr (2021) 77:2021. doi: 10.1530/endoabs.77.p186
33. Coll T, Eyre E, Rodríguez-Calvo R, Palomer X, Sánchez RM, Merlos M, et al. Oleate reverses palmitate-induced insulin resistance and inflammation in skeletal muscle cells. J Biol Chem (2008) 283:11107–16. doi: 10.1074/jbc.M708700200
34. Bugliani M, Mossuto S, Grano F, Suleiman M, Marselli L, Boggi U, et al. Modulation of autophagy influences the function and survival of human pancreatic beta cells under endoplasmic reticulum stress conditions and in type 2 diabetes. Front Endocrinol (Lausanne) (2019) 10:52. doi: 10.3389/fendo.2019.00052
35. Wang R, Hu W. Asprosin promotes β-cell apoptosis by inhibiting the autophagy of β-cell via AMPK-mTOR pathway. J Cell Physiol (2021) 236:215–21. doi: 10.1002/jcp.29835
36. Zou J, Xu C, Zhao ZW, Yin SH, Wang G. Asprosin inhibits macrophage lipid accumulation and reduces atherosclerotic burden by up-regulating ABCA1 and ABCG1 expression via the p38/Elk-1 pathway. J Transl Med (2022) 20:1–16. doi: 10.1186/s12967-022-03542-0
37. Zhang Z, Tan Y, Zhu L, Zhang B, Feng P, Gao E, et al. Asprosin improves the survival of mesenchymal stromal cells in myocardial infarction by inhibiting apoptosis via the activated ERK1/2-SOD2 pathway. Life Sci (2019) 231:116554. doi: 10.1016/j.lfs.2019.116554
38. Wen MS, Wang CY, Yeh JK, Chen CC, Tsai ML, Ho MY, et al. The role of asprosin in patients with dilated cardiomyopathy. BMC Cardiovasc Disord (2020) 20:1–8. doi: 10.1186/s12872-020-01680-1
39. Wang M, Yin C, Wang L, Liu Y, Li H, Li M, et al. Serum asprosin concentrations are increased and associated with insulin resistance in children with obesity. Ann Nutr Metab (2020) 75:205–12. doi: 10.1159/000503808
40. Sünnetçi Silistre E, Hatipoğl HU. Increased serum circulating asprosin levels in children with obesity. Pediatr Int (2020) 62:467–76. doi: 10.1111/ped.14176
41. Wang CY, Lin TA, Liu KH, Liao CH, Liu YY, Wu VCC, et al. Serum asprosin levels and bariatric surgery outcomes in obese adults. Int J Obes (2019) 43:1019–25. doi: 10.1038/s41366-018-0248-1
42. Cantay H, Binnetoglu K, Gul HF, Bingol SA. Investigation of serum and adipose tissue levels of asprosin in patients with severe obesity undergoing sleeve gastrectomy. Obesity (2022) 30:1639–46. doi: 10.1002/oby.23471
43. Long W, Xie X, Du C, Zhao Y, Zhang C, Zhan D, et al. Decreased circulating levels of asprosin in obese children. Horm Res Paediatr (2019) 91(4):271–277. doi: 10.1159/000500523
44. Corica D, Aversa T, Currò M, Tropeano A, Pepe G, Alibrandi A, et al. Asprosin serum levels and glucose homeostasis in children with obesity. Cytokine (2021) 142. doi: 10.1016/j.cyto.2021.155477
45. Corica D, Pepe G, Aversa T, Currò M, Curatola S, Li Pomi A, et al. Meal-related asprosin serum levels are affected by insulin resistance and impaired fasting glucose in children with obesity. Front Endocrinol (Lausanne) (2022) 12:805700. doi: 10.3389/fendo.2021.805700
46. Wang Y, Qu H, Xiong X, Qiu Y, Liao Y, Chen Y, et al. Plasma asprosin concentrations are increased in individuals with glucose dysregulation and correlated with insulin resistance and first-phase insulin secretion. Mediators Inflammation (2018) 2018. doi: 10.1155/2018/9471583
47. Naiemian S, Naeemipour M, Zarei M, Lari Najafi M, Gohari A, Behroozikhah MR, et al. Serum concentration of asprosin in new-onset type 2 diabetes. Diabetol Metab Syndr (2020) 12:1–8. doi: 10.1186/s13098-020-00564-w
48. Cassidy SB, Schwartz S, Miller JL, Driscoll DJ. Prader-willi syndrome. Genet Med (2012) 14:10–26. doi: 10.1038/gim.0b013e31822bead0
49. Alsaif M, Field CJ, Colin-Ramirez E, Prado CM, Haqq AM. Serum asprosin concentrations in children with prader–willi syndrome: Correlations with metabolic parameters. J Clin Med (2022) 11. doi: 10.3390/jcm11082268
50. Ko JR, Seo DY, Kim TN, Park SH, Kwak HB, Ko KS, et al. Aerobic exercise training decreases hepatic asprosin in diabetic rats. J Clin Med (2019) 8. doi: 10.3390/jcm8050666
51. Ceylan Hİ, Saygın Ö, Özel Türkcü Ü. Assessment of acute aerobic exercise in the morning versus evening on asprosin, spexin, lipocalin-2, and insulin level in overweight/obese versus normal weight adult men. Chronobiol Int (2020) 37:1252–68. doi: 10.1080/07420528.2020.1792482
52. Kantorowicz M, Szymura J, Szygula Z, Kusmierczyk J, Maciejczyk M, Wiecek M. Nordic Walking at maximal fat oxidation intensity decreases circulating asprosin and visceral obesity in women with metabolic disorders. Front Physiol (2021) 12:726783. doi: 10.3389/fphys.2021.726783
53. Jahangiri M, Shahrbanian S, Hackney AC. Changes in the level of asprosin as a novel adipocytokine after different types of resistance training. J Chem Heal Risks (2021) 11:179–88. doi: 10.22034/jchr.2021.1935792.1361
54. Ke F, Xue G, Jiang X, Li F, Lai X, Zhang M, et al. Combination of asprosin and adiponectin as a novel marker for diagnosing non-alcoholic fatty liver disease. Cytokine (2020) 134:155184. doi: 10.1016/j.cyto.2020.155184
55. Liu LJ, Kang YR, Xiao YF. Increased asprosin is associated with non-alcoholic fatty liver disease in children with obesity. World J Pediatr (2021) 17:394–9. doi: 10.1007/s12519-021-00444-x
56. Hu Y, Xu Y, Zheng Y, Kang Q, Lou Z, Liu Q, et al. Increased plasma asprosin levels in patients with drug-naive anorexia nervosa. Eat Weight Disord (2021) 26:313–21. doi: 10.1007/s40519-020-00845-3
57. Jowik K, Dmitrzak-Węglarz M, Pytlińska N, Jasińska-Mikołajczyk A, Słopień A, Tyszkiewicz-Nwafor M. Apelin-13 and asprosin in adolescents with anorexia nervosa and their association with psychometric and metabolic variables. Nutrients (2022) 14:4022. doi: 10.3390/nu14194022
58. Ding J, Wang S, Tan W, Song X, Zhang L. Association of serum asprosin concentrations with obstructive sleep apnea syndrome. Sleep Breath (2021) 25:1893–6. doi: 10.1007/s11325-021-02298-z
59. Gaggini M, Morelli M, Buzzigoli E, DeFronzo RA, Bugianesi E, Gastaldelli A. Non-alcoholic fatty liver disease (NAFLD) and its connection with insulin resistance, dyslipidemia, atherosclerosis and coronary heart disease. Nutrients (2013) 5:1544–60. doi: 10.3390/nu5051544
60. Vgontzas AN, Papanicolaou DA, Bixler EO, Hopper K, Lotsikas A, Lin HM, et al. Sleep apnea and daytime sleepiness and fatigue: Relation to visceral obesity, insulin resistance, and hypercytokinemia. J Clin Endocrinol Metab (2000) 85:1151–8. doi: 10.1210/jcem.85.3.6484
61. Resta O, Foschino-Barbaro MP, Legari G, Talamo S, Bonfitto P, Palumbo A, et al. Sleep-related breathing disorders, loud snoring and excessive daytime sleepiness in obese subjects. Int J Obes (2001) 25:669–75. doi: 10.1038/sj.ijo.0801603
62. Zhang L, Chen C, Zhou N, Fu Y, Cheng X. Circulating asprosin concentrations are increased in type 2 diabetes mellitus and independently associated with fasting glucose and triglyceride. Clin Chim Acta (2019) 489:183–8. doi: 10.1016/j.cca.2017.10.034
63. Zhang X, Jiang H, Ma X, Wu H. Increased serum level and impaired response to glucose fluctuation of asprosin is associated with type 2 diabetes mellitus. J Diabetes Investig (2020) 11:349–55. doi: 10.1111/jdi.13148
64. Timurkaan M, Timurkaan ES. Two important players for type 2 diabetes mellitus: Metrnl and asprosin. Clin Lab (2022) 68. doi: 10.7754/Clin.Lab.2021.211015
65. Li X, Liao M, Shen R, Zhang L, Hu H, Wu J, et al. Plasma asprosin levels are associated with glucose metabolism, lipid, and sex hormone profiles in females with metabolic-related diseases. Mediators Inflammation (2018) 2018. doi: 10.1155/2018/7375294
66. Ahmed Alobaidi MB, Hameed Al-Samarrai RR. Correlation between serum asprosin level and oxidative stress in Iraqi patients with type ii diabetes mellitus. Syst Rev Pharm (2020) 11:1729–33. doi: 10.31838/srp.2020.12.262
67. Zhang H, Hu W, Zhang G. Circulating asprosin levels are increased in patients with type 2 diabetes and associated with early-stage diabetic kidney disease. Int Urol Nephrol (2020) 52:1517–22. doi: 10.1007/s11255-020-02509-8
68. Deng X, Zhao L, Guo C, Yang L, Wang D, Li Y, et al. Higher serum asprosin level is associated with urinary albumin excretion and renal function in type 2 diabetes. Diabetes Metab Syndr Obes Targets Ther (2020) 13:4341–51. doi: 10.2147/DMSO.S283413
69. Wang R, Lin P, Sun H, Hu W. Increased serum asprosin is correlated with diabetic nephropathy. Diabetol Metab Syndr (2021) 13:1–6. doi: 10.1186/s13098-021-00668-x
70. Goodarzi G, Setayesh L, Fadaei R, Khamseh ME, Aliakbari F, Hosseini J, et al. Circulating levels of asprosin and its association with insulin resistance and renal function in patients with type 2 diabetes mellitus and diabetic nephropathy. Mol Biol Rep (2021) 48:5443–50. doi: 10.1007/s11033-021-06551-2
71. Xu L, Cui J, Li M, Wu Q, Liu M, Xu M, et al. Association between serum asprosin and diabetic nephropathy in patients with type 2 diabetes mellitus in the community: A cross-sectional study. Diabetes Metab Syndr Obes Targets Ther (2022) 15:1877–84. doi: 10.2147/DMSO.S361808
72. Oruc Y, Celik F, Ozgur G, Beyazyildiz E, Ugur K, Yardim M, et al. Altered blood and aqueous humor levels of asprosin, 4-hydroxynonenal, and 8-Hydroxy-Deoxyguanosine in patients with diabetes mellitus and cataract with and without diabetic retinopathy. Retina (2020) 40:2410–6. doi: 10.1097/IAE.0000000000002776
73. You M, Liu Y, Wang B, Li L, Zhang H, He H, et al. Asprosin induces vascular endothelial-to-mesenchymal transition in diabetic lower extremity peripheral artery disease. Cardiovasc Diabetol (2022) 21:1–15. doi: 10.1186/s12933-022-01457-0
74. Deng X, Zhao Z, Zhao L, Wang C, Li Y, Cai Z, et al. Association between circulating asprosin levels and carotid atherosclerotic plaque in patients with type 2 diabetes. Clin Biochem (2022) 109(110):44–50. doi: 10.1016/j.clinbiochem.2022.04.018
75. Hong T, Li JY, Wang Y, Qi XY, Liao ZZ, Bhadel P, et al. High serum asprosin levels are associated with presence of metabolic syndrome. Int J Endocrinol (2021) 2021:11–6. doi: 10.1155/2021/6622129
76. Tuttle KR, Bakris GL, Bilous RW, Chiang JL, De Boer IH, Goldstein-Fuchs J, et al. Diabetic kidney disease: A report from an ADA consensus conference. Diabetes Care (2014) 37:2864–83. doi: 10.2337/dc14-1296
77. Xu L, Lin X, Guan M, Liu Y. Correlation between different stages of diabetic nephropathy and neuropathy in patients with T2DM: A cross-sectional controlled study. Diabetes Ther (2018) 9:2335–46. doi: 10.1007/s13300-018-0519-9
78. van Rijssen TJ, van Dijk EHC, Yzer S, Ohno-Matsui K, Keunen JEE, Schlingemann RO, et al. Central serous chorioretinopathy: Towards an evidence-based treatment guideline. Prog Retin Eye Res (2019) 73. doi: 10.1016/j.preteyeres.2019.07.003
79. Türkcü FM, Yüksel H, Yüksel H, Şahin A, Cinar Y, Cingü AK, et al. Serum dehydroepiandrosterone sulphate, total antioxidant capacity, and total oxidant status in central serous chorioretinopathy. Graefe’s Arch Clin Exp Ophthalmol (2014) 252:17–21. doi: 10.1007/s00417-013-2396-2
80. Celik F, Coteli E, Gul FC, Ozsoy E, Kobat SG, Akkoc RF, et al. Laboratory evidence on a direct correlation between acute central serous chorioretinopathy and tenascin c, metalloprotein 1, BAX, BCL2, subfatin and asprosin. J Fr Ophtalmol (2022) 45:314–22. doi: 10.1016/j.jfo.2021.09.011
81. Ozcan S, Kelestemur MM, Hekim MG, Bulmus O, Bulut F, Bilgin B, et al. Asprosin, a novel therapeutic candidate for painful neuropathy: an experimental study in mice. Naunyn Schmiedebergs Arch Pharmacol (2022) 395:325–35. doi: 10.1007/s00210-021-02197-w
82. Hendler I, Blackwell SC, Mehta SH, Whitty JE, Russell E, Sorokin Y, et al. The levels of leptin, adiponectin, and resistin in normal weight, overweight, and obese pregnant women with and without preeclampsia. Am J Obstet Gynecol (2005) 193:979–83. doi: 10.1016/j.ajog.2005.06.041
83. Kleiblova P, Dostalova I, Bartlova M, Lacinova Z, Ticha I, Krejci V, et al. Expression of adipokines and estrogen receptors in adipose tissue and placenta of patients with gestational diabetes mellitus. Mol Cell Endocrinol (2010) 314:150–6. doi: 10.1016/j.mce.2009.08.002
84. Melchor I, Burgos J, Del Campo A, Aiartzaguena A, Gutiérrez J, Melchor JC. Effect of maternal obesity on pregnancy outcomes in women delivering singleton babies: A historical cohort study. J Perinat Med (2019) 47:625–30. doi: 10.1515/jpm-2019-0103
85. Catalano PM, McIntyre HD, Cruickshank JK, McCance DR, Dyer AR, Metzger BE, et al. The hyperglycemia and adverse pregnancy outcome study: Associations of GDM and obesity with pregnancy outcomes. Diabetes Care (2012) 35:780–6. doi: 10.2337/dc11-1790
86. Alan M, Gurlek B, Yilmaz A, Aksit M, Aslanipour B, Gulhan I, et al. Asprosin: a novel peptide hormone related to insulin resistance in women with polycystic ovary syndrome. Gynecol Endocrinol (2019) 35:220–3. doi: 10.1080/09513590.2018.1512967
87. Chang CL, Huang SY, Hsu YC, Chin TH, Soong YK. The serum level of irisin, but not asprosin, is abnormal in polycystic ovary syndrome patients. Sci Rep (2019) 9:1–11. doi: 10.1038/s41598-019-42061-9
88. Deniz R, Yavuzkir S, Ugur K, Ustebay DU, Baykus Y, Ustebay S, et al. Subfatin and asprosin, two new metabolic players of polycystic ovary syndrome. J Obstet Gynaecol (Lahore) (2021) 41:279–84. doi: 10.1080/01443615.2020.1758926
89. Jiang Y, Liu Y, Yu Z, Yang P, Zhao S. Serum asprosin level in different subtypes of polycystic ovary syndrome: A cross-sectional study. Rev Assoc Med Bras (2021) 67:590–6. doi: 10.1590/1806-9282.20201147
90. Baykus Y, Yavuzkir S, Ustebay S, Ugur K, Deniz R, Aydin S. Asprosin in umbilical cord of newborns and maternal blood of gestational diabetes, preeclampsia, severe preeclampsia, intrauterine growth retardation and macrosemic fetus. Peptides (2019) 120:170132. doi: 10.1016/j.peptides.2019.170132
91. Zhong L, Long Y, Wang S, Lian R, Deng L, Ye Z, et al. Continuous elevation of plasma asprosin in pregnant women complicated with gestational diabetes mellitus: A nested case-control study. Placenta (2020) 93:17–22. doi: 10.1016/j.placenta.2020.02.004
92. Behrasi F, Karajibani M, Khayat S, Fanaei H, Montazerifar F. Association of maternal and umbilical cord blood asprosin with excessive gestational weight gain. Nutr Bull (2022) 47:50–6. doi: 10.1111/nbu.12542
93. Leonard AN, Shill AL, Thackray AE, Stensel DJ, Bishop NC. Fasted plasma asprosin concentrations are associated with menstrual cycle phase, oral contraceptive use and training status in healthy women. Eur J Appl Physiol (2021) 121:793–801. doi: 10.1007/s00421-020-04570-8
94. Chen W, Pang Y. Metabolic syndrome and PCOS: Pathogenesis and the role of metabolites. Metabolites (2021) 11. doi: 10.3390/metabo11120869
95. DeUgarte CM, Bartolucci AA, Azziz R. Prevalence of insulin resistance in the polycystic ovary syndrome using the homeostasis model assessment. Fertil Steril (2005) 83:1454–60. doi: 10.1016/j.fertnstert.2004.11.070
96. Azziz R, Carmina E, Chen Z, Dunaif A, Laven JSE, Legro RS, et al. Polycystic ovary syndrome. Nat Rev Dis Prim (2016) 2. doi: 10.1038/nrdp.2016.57
97. Hainaut P, Plymoth A. Cancer as a metabolic disease. Curr Opin Oncol (2012) 24:56–7. doi: 10.1097/cco.0b013e32834e388a
98. Jones RG, Thompson CB. Tumor suppressors and cell metabolism: A recipe for cancer growth. Genes Dev (2009) 23:537–48. doi: 10.1101/gad.1756509
99. Manuscript A. Understanding the warburg effect: The metabolic requirements of cell proliferation. Encycl Neurosci (2008) 324:3828–8. doi: 10.1007/978-3-540-29678-2_5605
100. Booth A, Magnuson A, Fouts J, Foster M. Adipose tissue, obesity and adipokines: Role in cancer promotion. Horm Mol Biol Clin Investig (2015) 21:57–74. doi: 10.1515/hmbci-2014-0037
101. Kerslake R, Sisu C, Panfilov S, Hall M, Khan N, Jeyaneethi J, et al. Differential regulation of genes by the glucogenic hormone asprosin in ovarian cancer. J Clin Med (2022) 11:1–18. doi: 10.3390/jcm11195942
102. Qian J, Chen G, Zhang Y, Wu H, Zhao J, Wang L, et al. Lactic acid promotes metastatic niche formation in bone metastasis of colorectal cancer. Cell Commun Signal (2021) 19:1–15. doi: 10.1186/s12964-020-00667-x
103. Wang W, Somers EB, Ross EN, Kline JB, O’Shannessy DJ, Schweizer C, et al. FCGR2A and FCGR3A genotypes correlate with farletuzumab response in patients with first-relapsed ovarian cancer exhibiting low CA125. Cytogenet Genome Res (2017) 152:169–79. doi: 10.1159/000481213
104. Kocaman N, Artaş G. Can novel adipokines, asprosin and meteorin-like, be biomarkers for malignant mesothelioma? Biotech Histochem (2020) 95:171–5. doi: 10.1080/10520295.2019.1656344
105. Kocaman N, Yuksel EI, Demir B, Calik I, Cicek D. Two novel biomarker candidates for differentiating basal cell carcinoma from trichoblastoma; asprosin and meteorine like peptide. Tissue Cell (2022) 76. doi: 10.1016/j.tice.2022.101752
106. Ryu TY, Park J, Scherer PE. Hyperglycemia as a risk factor for cancer progression. Diabetes Metab J (2014) 38:330–6. doi: 10.4093/dmj.2014.38.5.330
107. Van Cutsem E, Arends J. The causes and consequences of cancer-associated malnutrition. Eur J Oncol Nurs (2005) 9:S51–S63. doi: 10.1016/j.ejon.2005.09.007
108. Fearon K, Strasser F, Anker SD, Bosaeus I, Bruera E, Fainsinger RL, et al. Definition and classification of cancer cachexia: An international consensus. Lancet Oncol (2011) 12:489–95. doi: 10.1016/S1470-2045(10)70218-7
109. Tisdale MJ. Cancer cachexia: Metabolic alterations and clinical manifestations. Nutrition (1997) 13:1–7. doi: 10.1016/S0899-9007(96)00313-9
Keywords: asprosin, adipokines, metabolic diseases, obesity, diabetes, PCOS
Citation: Farrag M, Ait Eldjoudi D, González-Rodríguez M, Cordero-Barreal A, Ruiz-Fernández C, Capuozzo M, González-Gay MA, Mera A, Lago F, Soffar A, Essawy A, Pino J, Farrag Y and Gualillo O (2023) Asprosin in health and disease, a new glucose sensor with central and peripheral metabolic effects. Front. Endocrinol. 13:1101091. doi: 10.3389/fendo.2022.1101091
Received: 17 November 2022; Accepted: 19 December 2022;
Published: 05 January 2023.
Edited by:
Valentina Perissi, Boston University, United StatesReviewed by:
Emmanouil Karteris, Brunel University London, United KingdomAndrea Carranza, Consejo Nacional de Investigaciones Científicas y Técnicas (CONICET), Argentina
Copyright © 2023 Farrag, Ait Eldjoudi, González-Rodríguez, Cordero-Barreal, Ruiz-Fernández, Capuozzo, González-Gay, Mera, Lago, Soffar, Essawy, Pino, Farrag and Gualillo. This is an open-access article distributed under the terms of the Creative Commons Attribution License (CC BY). The use, distribution or reproduction in other forums is permitted, provided the original author(s) and the copyright owner(s) are credited and that the original publication in this journal is cited, in accordance with accepted academic practice. No use, distribution or reproduction is permitted which does not comply with these terms.
*Correspondence: Oreste Gualillo, oreste.gualillo@sergas.es; Yousof Farrag, yousof.farrag.zakaria@sergas.es
†These authors have contributed equally to this work and share senior authorship