Landslide risk reduction through close partnership between research, industry, and public entities in Norway: Pilots and case studies
- 1Norwegian Geotechnical Institute, Oslo, Norway
- 2Department of Civil and Environmental Engineering, Norwegian University for Science and Technology, Trondheim, Norway
Cross-sectorial and cross-disciplinary collaboration, as well as public-private partnerships are necessary to handle the complexity of climate adaptation. The Research Council of Norway has established the Centres for Research-based Innovation (CRI) in which research- and education organizations, public entities and private enterprises join forces in 8-year long collaborations. CRI-Klima 2050 focuses on climate adaptation of buildings and infrastructure and runs several pilot projects to innovate new solutions for building resilience, stormwater- and landslide risk management. Several of the major infrastructure owners in Norway are partners in the centre. Norway is increasingly affected by precipitation triggered landslides. Klima 2050 pilot projects on landslide risk reduction include a web-based toolbox for prioritizing and choosing optimal mitigation measures, including Nature-Based Solutions, improved early warning systems and mitigation measures for slope instability, and improved local warning for hazardous weather systems, all developed in close collaboration between centre partners from different sectors and disciplines. The results of these projects can all be upscaled and are transferable to other infrastructure elements.
Introduction
Climate change enforces needs for adapting society both in terms of scientific and engineering knowledge, but also in terms of management and governance. The complexity of these challenges requires broad, multidisciplinary solutions, harnessing the capabilities of a diverse range of organisations and institutions. Klima 2050 is a research centre focusing on risk reduction through climate adaptation of buildings and infrastructure, where cooperation across sectors and disciplines, and the premise of active collaboration between research organisations, public entities, and commercial companies are fundamental premises to deliver on these ambitions.
Klima 2050 is funded by the Norwegian Research Council (RCN) under the instrument “Norwegian Centres for Research Based Innovation” (CRI). The CRI instrument carries funding for a period of 8 years, providing continuity and the opportunity to address deeper, more complex challenges. RCN has financed the CRI ‘Klima 2050′ specifically to focus on risk reduction through climate adaptation of buildings and infrastructure.
The purpose of this paper is to present the pilots and case studies implemented in Klima 2050 to promote research addressing landslide challenges. Landslide occurrences have a close link to precipitation, and thereby climate and climate change are important drivers for landslide hazards and risk. This is a topic of great interest in Norway and Europe, it is also broadly studied internationally. The largest EU funded landslide project to date was SafeLand, a research project funded through the 7th framework program between 2009 and 2012 (Nadim et al., 2014). Moore and McInnes (2016) presented a global perspective on the nature, scale and impact of landslides on the society in the context of climate change.
A number of studies assess the connection between precipitation and landslide activity (e.g., Dikau and Schrott, 1999; Jaedicke et al., 2014; Gariano and Guzetti, 2016; Tichavsky et al., 2019). Andersson-Sköld et al. (2013) point at the need for improved documentation and active communication between different stakeholders for more effective landslide risk management. Alcántara-Ayala et al. (2017) point at main future challenges for the integration of science into local, national, regional and international policy development for Landslide Disaster Risk Reduction within the Sendai Framework. Despite numerous studies on the connections between climate and landslide risk and the need for cross-disciplinary and cross-sectoral cooperation to turn science results into action, few national collaboration programmes between research, public entities and private businesses exist.
Changing climate is evidenced in Norway through meteorological data, for example temperature. The average air temperature in Norway has increased by more than 1°C since 1900, and precipitation has increased by 18% over the same period (Hanssen-Bauer et al., 2015) (Figures 1, 2). The trends are expected to continue through this century. It is estimated that the annual mean temperature will rise by between 2.8 and 5.0°C by the end of this century relative to the reference period 1971–2000, depending on the emission scenario (RCP4.5–RCP 8.5). The equivalent numbers for precipitation are 10%–18% for average values (Norwegian Climate Service Centre, 2021).
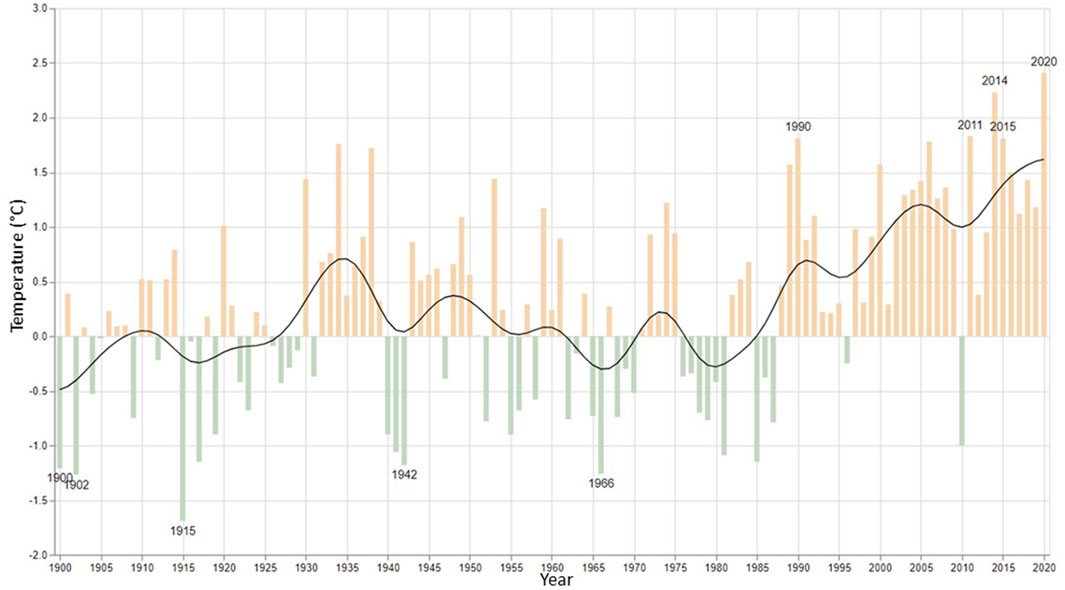
FIGURE 1. Temperature deviations from the 1961–1990 normal, from 1900 to 2020. Figures using the new normal, 1991–2020 are not available yet, but will show the same trend (Met.no).
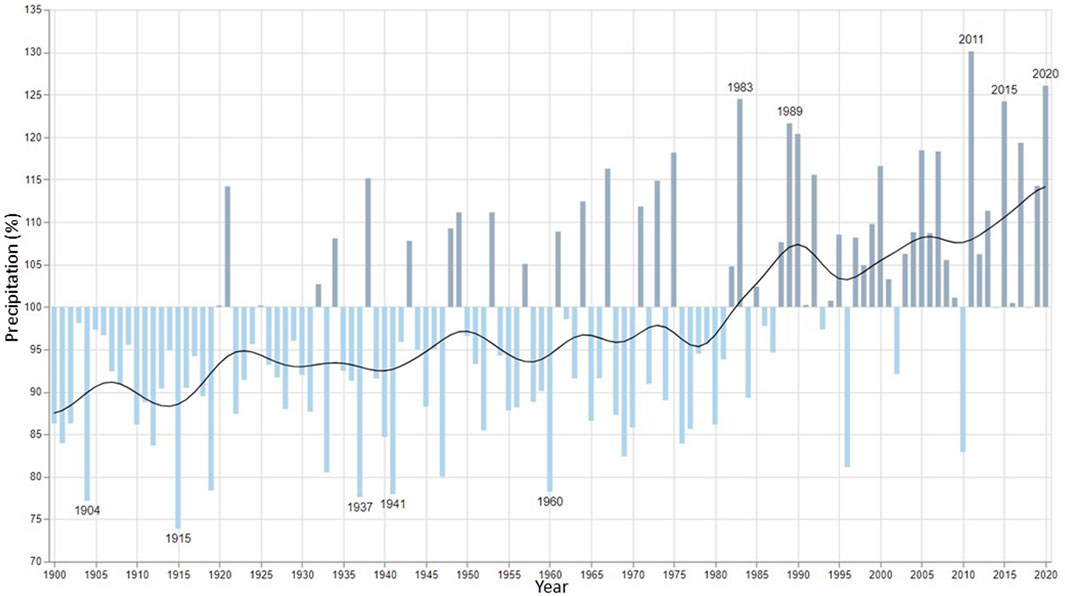
FIGURE 2. Precipitation in % of the 1961–1990 normal, from 1900 to 2020. Figures using the new normal, 1991–2020 are not available yet, but will show the same trend (Met.no).
Precipitation (rainfall and snow storage) are significant factors affecting landslide risk. Norway is already experiencing increases in both the frequency and intensity of extreme precipitation events (Hanssen-Bauer et al., 2015), leading to increased frequency of precipitation-triggered landslides and debris flows. In addition, the increase in precipitation leads to increased flooding and erosion in watercourses and may also trigger rockfall and rockslides. Considering the time lag in reversing the effects of climate change, and a life expectancy of 40 to more than 100 years for buildings and other infrastructure, adaptation to climate change within the built environment to accommodate the changing risk is inevitable (Lisø, 2006; Norwegian Government, 2010).
There are numerous challenges within climate change adaptation, and coping with these problems opens opportunities for research leading to improvements of methods and strategies as well as innovations in solutions and technology. As the challenges are multifaceted, it follows that the best solutions are likely resulting from multidisciplinary and multisector cooperation.
This paper presents the Klima 2050 research centre’s implementation of pilots and case studies to address various methods, tools and strategies for addressing climate induced landslide hazards. A key aspect of these is the interaction of public, private and research organisations as a specific strategy to develop and operate the pilots and case studies. In this paper, with focus on climate induced landslides, we present 1) the context for our activities (the Klima2050 centre and landslide management in Norway); 2) the methodology for establishing cases and pilots within the Klima2050 centre, and 3) the results of these activities focusing on one case (tool development) and three pilots (field sites for research and development). We use the presented pilots and case site to describe and discuss how cross-sectorial- and disciplinary cooperation in the CRIs are used to improve climate adaptation, as well as testing out practical application of adaptation measures and strategies.
Context
Klima 2050: A collaborative project to reduce meteorological risk and societal exposure
The CRI system is based on the premise of cooperation between research organisations and user organisations, where the user organisations consist of a mix of public entities and the private sector companies. Experience has proved that this is a successful arena for establishing and nourishing cooperation between these diverse types of organisations. (Damvad Analytics, 2018). The “User” partners have a strong role in the CRIs: the funding scheme mandates that these partners have the majority in the centre boards, and there are specific requirements regulating the proportions of user partners and research partners in the CRIs. In addition to performing excellent research, the CRIs are expected to have a strong applied focus and innovations in methods, products and services is the key aspect of the centres. The User partners are the intended recipients of the research products. In total 59 CRIs have been funded, within a wide range of disciplines (RCN, 2021).
The system builds on experiences from similar initiatives in various countries, although none are exactly similar in length, organization, themes and organization. The US Engineering Research Centres (https://www.nsf.gov/eng/eec/erc.jsp) started as early as 1985, and has funded 75 centres in different engineering-related fields. Most relevant are probably the centres in Sweden (VINN Excellence; Stern et al., 2013), Austria (COMET; Austrian Research Promotion Agency, 2016) and United Kingdom (Catapult; Hauser, 2014). These centres are comparable in having various mixes of private and public partners and funding schemes. The centres established under these schemes differ somewhat in their sectorial focus (public, business, etc.), their service focus and their organizational model (Stahlecker, 2015; Damvad Analytics, 2018).
Klima 2050 is funded under the CRI scheme from 2015 through 2022 and consists of 19 partners including research institutes and universities 5), public entities 6) and private sector companies 8). The funding of Klima 2050 comes from RCN (43%) and the partners (57%), with the latter as a combination of cash and in-kind contributions. The centre reports to the RCN annually and all CRIs must pass a mid-term evaluation to secure funding for the full 8 years period.
Klima 2050 is organized in a structure with 4 work packages, a management group, an international scientific advisory committee, and a board of directors (Figure 3). As shown in the figure, pilot projects form the main arena for innovation in Klima 2050 and is the underlying operational structure for applied research. The scientific advisory committee is specifically included in the centre to help ensure the relevance of the Klima2050 activities in an international perspective.
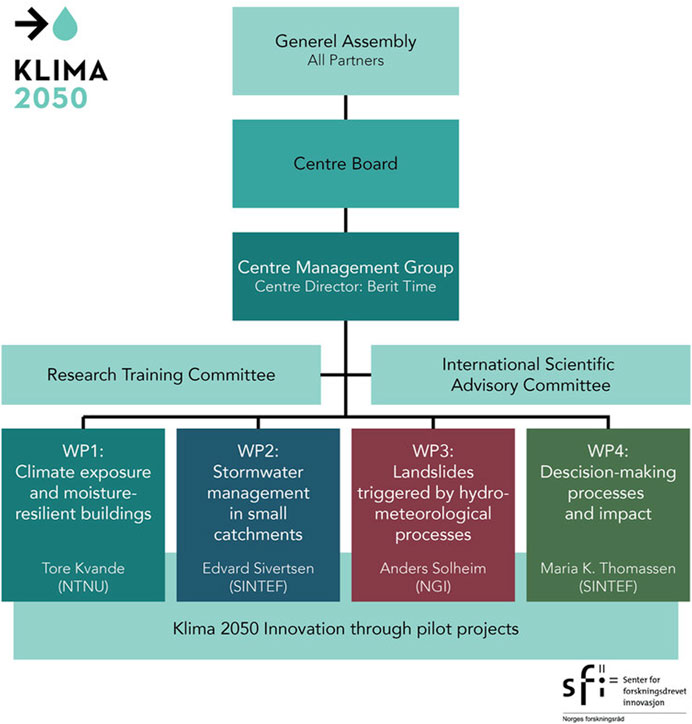
FIGURE 3. Organization of Klima 2050. Funding by the Norwegian Research Council under the CRI (SFI) instrument.
Landslide hazards in Norway
Most landslide events in Norway are triggered by precipitation, mostly as rain but sometimes also in combination with snowmelt (Krøgli et al., 2018; Bondevik and Sorteberg, 2020; Schilirio et al., 2021). There are many recent examples, but two cases are particularly illustrative: the Kvam and Jølster communities. The village of Kvam in Gudbrandsdalen, south-east Norway, was hit by extreme precipitation combined with snowmelt in the spring of both 2011 and 2013. Flooding combined with large volumes of debris from nearly 100 landslides into the river flowing through Kvam led to massive damage to housing areas and infrastructure, including closure of one of the main highways through Norway, European Road E6, as well as evacuation of hundreds of people. Estimated total damages cost is more than 200 mill. Euros (Mäki et al., 2015; Devoli et al., 2018; Schilirio et al., 2021). On 30 July 2019, local high intensity rain released more than 50 landslides in the Jølster community in western Norway (Rouault et al., 2020), causing one death, disruption of roads and other infrastructure and large economic losses. These landslides were largely unexpected because the intensity of the very localized event was not detected by the forecasting systems (Agersten et al., 2019; Villa, 2021).
The direct and indirect cost related to such incidents are large. During the last 10 years, landslides and snow avalanches comprised 6% of the events reported to the Norwegian Natural Perils Pool (a joint insurance scheme), with insured losses of roughly 180 mill Euros (Finance Norway, 2021). This figure represents only the material damage to buildings. Other forms of damage, for example to transport infrastructure, farmland, power- and communication lines, as well as environmental damage, come in addition, and add largely to the total cost. Indirect costs, for example due to delays or blockages of major transport routes can be significant if the downtime is long and increase the total cost further. In the largely rural and difficult terrain of Norway, alternative routes are often significantly slower or in some cases do not exist. Social consequences, such as fear among the population, are difficult to estimate but certainly come with a cost. Proper adaptation measures, despite often being expensive to establish, may have a high cost saving potential when viewed over multi-decadal life spans.
A recent report estimated the cost of mitigating landslide and flood hazards at approximately 8.5 billion Euros for all Norwegian buildings (except vacation homes) (NVE, 2021). The estimates were based on experiences from the last couple of decades and considered therefore only “traditional” mitigation measures. This has triggered a debate on several aspects of climate adaptation in Norway, including the need for new and innovative solutions, as well as the potential benefit of closer cooperation between research, public and private entities on climate adaptation. As important as closer collaboration between different entities, is the collaboration between different scientific disciplines. A holistic approach is needed to meet the challenges posed by climate change, and relevant scientific disciplines need to avoid “silo thinking” and develop better collaboration models and practices.
Norwegian policies and strategies for landslide risk management
The Norwegian Planning and Building Act (NPBA) requires risk assessment of natural hazards for all planning and construction activity in Norway. The purpose is to give the municipality the opportunity to direct new development to areas that are less prone to natural hazards or to implement risk mitigation measures in advance. Land use planning is central to preventing adverse consequences from floods and landslides. The level of risk acceptance for buildings is defined in the NPBA based on return periods, stating that for most family homes the annual probability of being affected by a landslide should not exceed 1/1,000. For secondary buildings (storage sheds, barns, garages, etc.) the corresponding return period is 100 years, whereas for larger buildings, apartment buildings, hotels, etc., housing more than 25 people, it is 5,000 years. Similar principles apply for roads, where the allowed return periods of landslides depend on the traffic density. For railways there currently are no defined minimum return periods for natural hazards, although general risk acceptance criteria exist as a general requirement for all hazard types.
Landslide problems are managed by different sectors in Norway. The National Public Roads Authority (NPRA) is responsible for landslide problems along roads; the state railway company, BaneNor is responsible for reducing landslide risk for railways, and the Norwegian Energy and Water Resources Directorate (NVE) manages landslide risk to houses and provides assistance to municipalities and the society in general with hazard mapping, mitigation measures, monitoring and early warning, as well as assistance in case of landslide events. All these entities are partners in CRI- Klima 2050.
The Norwegian Civil Protection Act assigns responsibility to the municipalities for the safety of their inhabitants, and as such the municipalities are crucial in managing landslide risk locally. Norwegian municipalities vary widely in size, and the smallest municipalities have populations of less than 1,000 inhabitants. This consequently results in a large span in competence and available resources to deal with landslide risk at the municipality level. There is a great need for guidelines and tools to support the municipalities in making good decisions, for example, in land use planning, where the planners must comply with the requirements in the Planning and Building Act. Equally important are economy and resource planning, where the municipalities must define and prioritize mitigation measures, often within limited economic means.
An important finding from Klima 2050 is that Norwegian municipalities have access to a wealth of guidelines and websites (74 and 10, respectively) for climate adaptation (Lappegard Hauge et al., 2016). We also experience that the municipalities react differently to the regional landslide hazard forecasts issued by NVE. Consistent guidance on active use of regional landslide forecasts to improve the municipal preparedness and thereby reducing the risk to the population is therefore needed. One of the products from Klima 2050 is a new set of such guidelines for use at the municipal level (Devoli et al., 2022).
The Norwegian legislation specifies requirements for safety against natural disasters for new buildings and imposes responsibility on the municipalities for the safety of the inhabitants. Implementation of physical mitigation measures is a common way to reduce the landslide risk. However, the legislation does not specify which measures to be applied in different situations, decisions which requires competence and experience which the problem owners often do not have. For example, selecting the most suitable measure requires considering site-specific geological and geotechnical conditions, local resources, socio-economic constraints, and environmental considerations.
For linear transport infrastructure, cost-effective mitigation strategy is often to establish early warning systems combined with restrictions for users or closure of the transportation line when the hazard is too high. One of several challenges is that traditional early warning systems depend on precise weather forecasts, particularly in forecasting torrential rain, which can be very local. Another main challenge is to establish plausible warning criteria, to minimise the issuing of false alarms and to avoid missed alarms. Physical models form the basis for establishing local early warning criteria. However, the use and calibration of such models has been very limited in Norway. There is a lack of data for calibration of models, i.e. data on landslide events, where the input parameters to the physical models are known.
Materials and methods
An essential activity in Klima2050 is the implementation of pilot projects and case studies to develop innovations and solutions to overcome identified challenges. Within the landslide work package (WP3), pilots have been established to address aspects of the challenges described in the Context section:
- A tool for better and more efficient process for choosing physical mitigation measures
- A new early warning model that uses more reliable warning of torrential rain
- A calibrated physical model for landslide release
- Verification of new types of mitigation measures
The method for planning and implementing a pilot is a well-defined process in Klima 2050. Several essential criteria are in place regarding type, ownership/responsibility, and purpose. The first is that the pilot must be a natural focal point for activity, for example a physical site, a building, a large infrastructure, or a digital infrastructure such as a database. Second, the pilot must be implemented as a partnership between the three categories of centre participants, with one or more participants from each: private company, public entity, and research organisation. Third, the pilot must have an “owner”, a lead partner with a specific interest in the research results arising from the pilot.
The activities implemented at the pilot shall focus on innovation, that is the development or testing of new methods, processes and tools, or adapting existing solutions to new application areas. Innovation is both a process and a product: the process is the value chain (Dwyer, 2020) from a fundamental research idea to a commercial service or product (Hansen and Birkinshaw, 2007; Roper et al., 2008), and the product is the strategic implementation of the value chain as improved products, services, methods, or knowledge and creating value for the user (Dogan, 2017). In Klima 2050, the pilots themselves are not the innovation, rather they are “sandboxes” for developing and testing innovative solutions and methods. These may be new innovations unique to Norway or represent the adaptation of existing international solutions and methods to Norwegian conditions.
To implement a pilot, an initiative is taken within one of the work packages to develop an initial concept for a pilot. Participation is negotiated with relevant partners, and together these partners develop a complete concept including site/location/infrastructure description, research and innovation objectives, implementation plan and financing plan. This concept is delivered as a proposal to the centre’s management team, which based on the merits of the proposal forwards this to the Centre Board for approval. Once the pilot is formally approved, planned budgets allocated to the relevant work package may be directed towards establishing the pilot’s activities.
Results
The discussion in this results section focus on four on-going pilot projects in WP3, “Landslides triggered by hydrometeorological processes” (Figure 3). These range from local to regional in scale and focus on different strategies to reduce the risk from landslides, from physical mitigation measures to improved early warning. We consider all to have considerable upscaling potential.
Landslide Risk Mitigation Toolbox: A tool for local and regional planners
Assessing landslide risk is a specialised task, often within the jurisdiction and responsibility of municipalities, however the specialised competency and experience needed is often unavailable within the municipalities’ staff. While assessing risk is a difficult task, an even larger challenge is to assess the most appropriate mitigation measures, particularly considering the transition to a more sustainable society and the implementation of methods supporting this. Nature Based Solutions (NBS) form a whole new suite of mitigation approaches, in which holistic and long-term thinking are key elements. Such new approaches are often not considered, or met with scepticism, due to lack of experience and competence (Solheim et al., 2021), a deficit which is also seen in the construction business, among those who build the physical mitigation measures.
These challenges are an important motivation for developing new guidelines, methods, and tools, such as the Landslide Risk Mitigation Toolbox (LaRiMiT) web portal (www.larimit.com) (Uzielli et al., 2017; Capobianco et al., 2022) to assist planners and others, from public entities at all levels to private businesses, in their work on risk reduction. The idea for LaRiMiT started in the EU FP7 project SafeLand (https://cordis.europa.eu/project/ id/226479/reporting) and has been under development in Klima 2050 since 2016.
The LaRiMiT pilot is organized by NGI, which is one of the research partners. Significant assistance during the development has been provided by several other Klima 2050 partners, in particular major government agencies, such as the Norwegian Railway Directorate, the Norwegian Energy and Water Resources Directorate and the Norwegian Public Roads Administration. Private partners have contributed with technology and experience represented in the technical solutions described in LaRiMiT. The tool was launched as a web service in 2018, and is now gaining national and international interest, both from academia and from practicing engineers both in public entities and in private businesses, such as consultants.
The LaRiMiT pilot is a web tool and corresponding database, providing a technical service for problem owners such as municipalities and infrastructure owners. Often these do not have the competence to tailor mitigation measures to the relevant hazard and addressing other factors such as cost and a host of local considerations. For example, selecting the most suitable measure requires considering site-specific geological and geotechnical conditions, local resources, socio-economic constraints, and environmental considerations. Through the LaRiMiT web-portal a user can define a problem site, and then identify, rank and select mitigation measures from an extensive database of landslide risk mitigation solutions, based on user-input attributes of site-specific slope movements and on expert scoring of the suitability of various mitigation solutions.
Inputs to LaRiMiT are provided by both users and experts (Figure 4), where user inputs define the problem, and the expert input defines the possible mitigation solutions and applicability of these. The algorithm integrating the inputs from these two groups and producing this assessment is the largest innovation in this pilot.
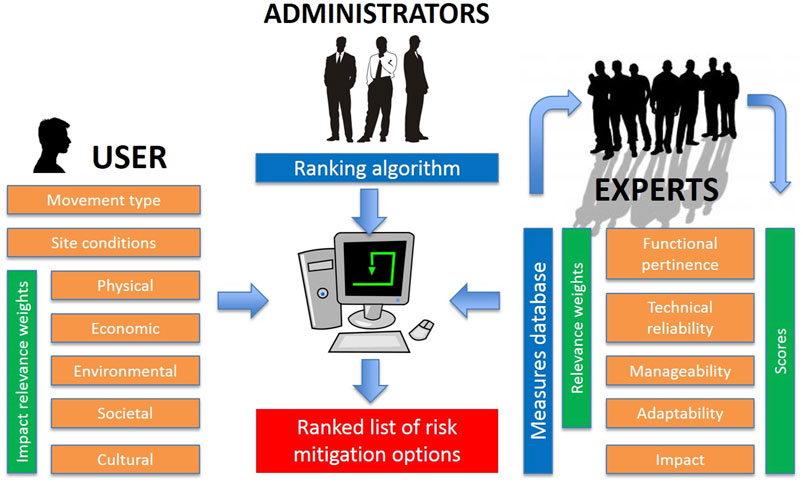
FIGURE 4. Schematic representation of the synergy between administrators, experts and users in the compilation and utilization of the LaRiMiT toolbox.
User inputs are on a case-by-case basis and include case-specific information defining the problem, for example: landslide type, site conditions, and the expected relevance of potential negative consequences and constraints (physical, ecological, economic, societal, environmental) brought by any mitigation measure or action for the specific case under investigation.
The experts’ input is a system input, applicable for all potential cases defined by users. The experts are from both academia and the private sector, and their input is used to define the relevance, applicability, and suitability of various mitigation measures. In this process we have been particularly focused on obtaining input from private consultancies. Expert inputs include sets of candidate mitigation measures and actions, and scores for each candidate. measure with respect to a set of ranking criteria.
The database initially included only conventional (grey) solutions relying on traditional engineering methods, but the database and toolbox have been recently expanded to include Nature Based Solutions (NBS), which are sustainable techniques for managing erosion and mitigating shallow landslides using vegetation and other natural materials, copying natural processes to the extent possible (European Commission, 2015). In addition to increased resilience regarding the landslide risk, other co-benefits, such as increased biodiversity, inhabitants’ well-being, aesthetics, local economy, etc. are highly valued effects of such solutions. NBS and conventional solutions can also be combined in hybrid solutions.
Following an evaluation of the website performed by Lappegard Hauge et al. (2019) improvements have been made in user friendliness, and thus effectiveness of the tool, which is open and available on-line. The website also comprises much information and examples of the various measures.
The Trollstigen pilot: Improved early warning of extreme precipitation
The Trollstigen Road in Western Norway is a scenic masterpiece of road engineering and is an extremely popular tourist destination. Unfortunately, the road is also susceptible to snow avalanches, shallow landslides, rock falls and debris flows. The investment in Trollstigen as a tourist destination will probably contribute to the traffic volume persisting or increasing, which means that the natural hazard risks will persist or increase in the future.
The road is seasonally closed during winter, alleviating the avalanche risk for persons, but during the summer months a combination of structural measures such as netting, rock bolting and water diversion, together with periodic road closures during episodes of excessive precipitation are necessary to maintain safety. Although road closures are an effective mitigation of risk to persons and vehicles, these periods need to be limited to when it is needed and warranted, as unnecessary road closures are harmful to tourism and inconvenient for residents.
The Trollstigen pilot was established to enable research and innovation activities to develop a framework for an early-warning system integrating regional and local weather data enabling optimal management of risk using road closure as a mitigation measure. By local weather data we mean measurements at or above the Isterdalen Valley, Trollstigen road and the plateau above (Figure 5).
The pilot is a cooperation between several Klima 2050 partners, including the National Public Road Authority (NPRA), The Meteorological Institute (MET), Norwegian Geotechnical Institute (NGI) and Multiconsult Ltd. Although the local county authorities own and operate the road, the NPRA is the owner of the Pilot and represents local county interests in the project. MET and NGI bring in meteorological and geotechnical research capacity, while the private company Multiconsult represents the practicing engineering community seeking tools and solutions to implement in projects.
The pilot infrastructure includes a permanent weather station installed on the plateau providing typical meteorological measurements (precipitation, wind speed, temperature, etc.), a mobile weather radar measuring weather systems just above the plateau. Eventually, local surface water run-off measurements will be deployed at key locations in the streams and rivers in the area. In addition, extensive background data are being collected including ground and aerial lidar data and detailed photographic imaging.
Multi-disciplinary collaboration is necessary to piece together meteorological data interpretation, now-casting of changing weather, impacts of short term and highly local precipitation on triggering of geohazards, and bring this knowledge together in practical tools that consulting engineers and contractors can implement as practical installations. This integration of meteorological data at this scale (single slope) is new in Norway, and the techniques developed will be an innovation.
The expected outcome of the research at the pilot is an improved systematic approach for evaluating local weather risks on susceptible stretches of infrastructure such as roads and railways. This approach may include recommendations for permanent monitoring systems. The benefit for society is clear: improving the ability to keep roads and railways open while maintaining an acceptable risk level for users of the infrastructure. The results from this pilot can be easily upscaled by implementing similar solutions at any other infrastructure facing weather related risks.
The Eidsvoll pilot: Early warning as an effective mitigation measure
A main Norwegian railroad passes by a national heritage site consisting of a 12th century church and cemetery in the municipality of Eidsvoll, Norway. The railroad passes by the eastern part of this area, at a much lower level in the terrain. The slope from the railway up to the heritage site is about 25–30 m hight, with an inclination of about 45° in the upper part (Heyerdahl et al., 2018) (Figure 6). Landslides are the primary threat at this location, as these may impact the railway and may also damage the church grounds. As this is a cultural heritage site, it is not acceptable to implement structural mitigation measures that change or otherwise impact the landscape (Figure 7).
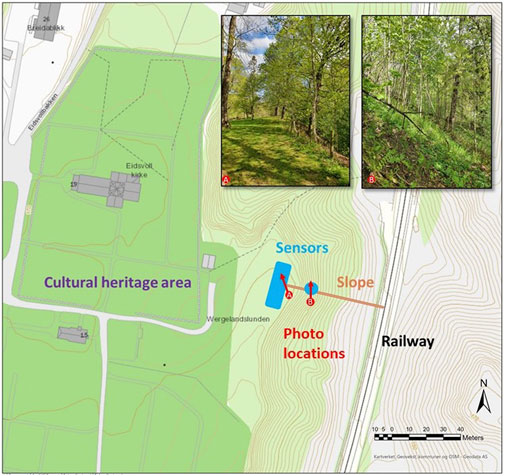
FIGURE 6. The pilot site at Eidsvoll, central south Norway. Slope, sensors, and railway location. Eidsvoll church (kirke) and the cemetery surrounding it are protected. Photos illustrate the vegetation conditions at the site.
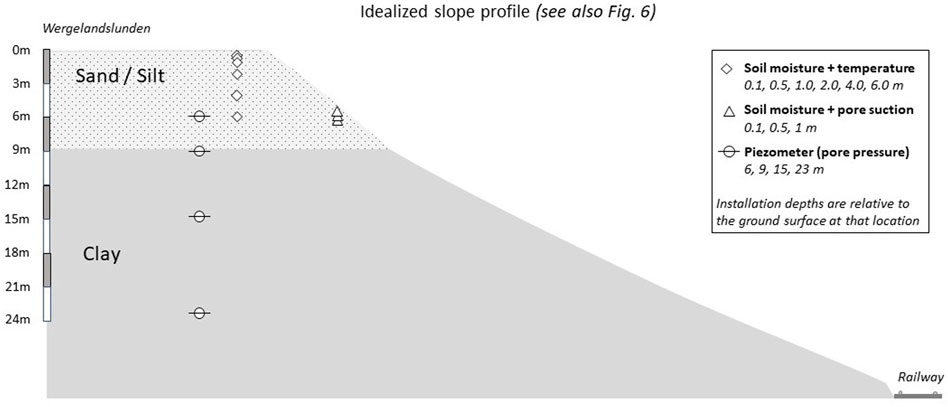
FIGURE 7. Schematic cross section of the studied slope (Figure 6) and depth location of the sensors.
This slope has been the focus of research activities for several years, including the installation of sensors on the upper part of the slope in 2016 to measure volumetric water content, pore water pressures, ground temperatures and tilt at various depths in the soil profile. The pore pressures are measured across the transition zone between silt and clay layers (Figure 7). Data from these sensors is measured at 1-h intervals and the data is available real-time on a cloud-based acquisition system. In 2021, additional sensors to measure soil-water content (SWC) and soil suction were installed at several depths. These data are also available online, but via a separate cloud-based data acquisition system. Samples have also been collected for laboratory testing to determine the geotechnical characteristics of the soil including water retention curves (Heyerdahl et al., 2018).
The slope has recently been established as a Klima 2050 pilot. The main aim for this pilot project is to transform the real-time monitoring of data for rainfall and soil water conditions into an early warning system (EWS) useful to issue warnings for the road and railway authority. The pilot is initiated by the Norwegian Railway Directorate with NGI as the principal research partner At present, only these two organizations are involved, but the involvement of private organisations for the future operations of the EWS is relevant.
Currently the work is focused on defining a reliable hydrological model able to back calculate the hydrogeological variables in the slope. Further analysis will be focused on the definition of triggering thresholds to be employed in the early warning system (Piciullo et al., 2018). A slope stability software and machine learning algorithms will be employed to determine the triggering values as a function of the main input (rainfall, snowmelt, temperature, vegetation). The approach being developed for this pilot will be available for adaptation and implementation as a slope scale Landslide Early Warning System (LEWS) to forecast the occurrence of landslides and prevent fatalities and damages, and it can easily be exported to other sites nationally and internationally.
The Bodø pilot: Innovative measures to increase slope stability
Owners of linear infrastructure (roads, railways) in Norway, often face problems in long unstable slopes along their assets, particularly during periods of heavy precipitation. As there are numerous such slopes along transport infrastructure elements in Norway (and elsewhere), new, efficient, and cost-effective measures to reduce the risk related to precipitation induced slope instability are needed.
The pilot project at the Bodø railway station is located at such a slope and is implemented as a close collaboration between a public entity (the Norwegian Railway Directorate), a research institute (NGI), and private businesses (Multiconsult Ltd., Leca Ltd., and Storm Aqua Ltd.), with the Norwegian Railway Directorate as the “pilot owner.” Over the last 25 years there has been three major slope failures at the site in connection with heavy rainfall, as well as numerous smaller incidents which also have caused problems for the infrastructure at the base of the slope, such as cables, drainage and of course for the rails.
The goal of the pilot is two-fold: i) to test the methods for early warning of slope instability and/or failure of railway embankments based on instrumentation and physical stability modeling, and ii) to test and compare mitigation measures along parts of the slope, primarily in the form of various drainage measures, including Nature Based Solutions (NBS). Relatively simple instrumentation is planned for installation (Figure 8) in the form of pore pressure and soil moisture measurements, combined with a local weather station (precipitation and temperature), as well as deformation measurements along the slope, which have had several instability events. This is also closely linked to the research being carried out at the Eidsvoll pilot site (above). The sensor installations were completed in July 2022.
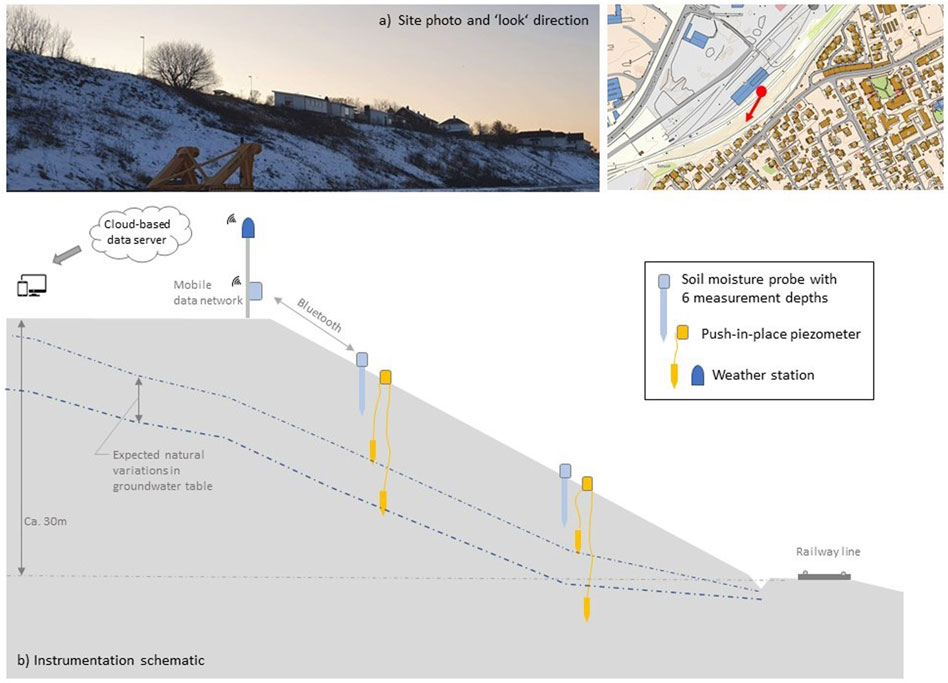
FIGURE 8. (A) Photo of the slope at Bodø station, and (B) schematic of the slope with installations.
The available slope is a long, linear structure that is relatively homogeneous both in terms of soil conditions, geometry, and exposure. Six geotechnical borings along three profiles were carried out to define ground conditions at the site, and the installed instrumentation consisting of pore pressure measurements, soil moisture and a simple visual indicator of surficial slope movements will provide the relevant parameters needed for assessing slope stability. A local weather station is installed to monitor precipitation specifically on the slope itself, providing better data than the regional measurements would provide.
The project has defined five test plots which will allow different mitigation measures to be implemented in each plot, and the effect of these measures to be directly comparable across the five locations (Figure 9). These plots are each approximately 40 m wide with a slope height of about 12 m. Implementation of measures in these plots is still in progress.
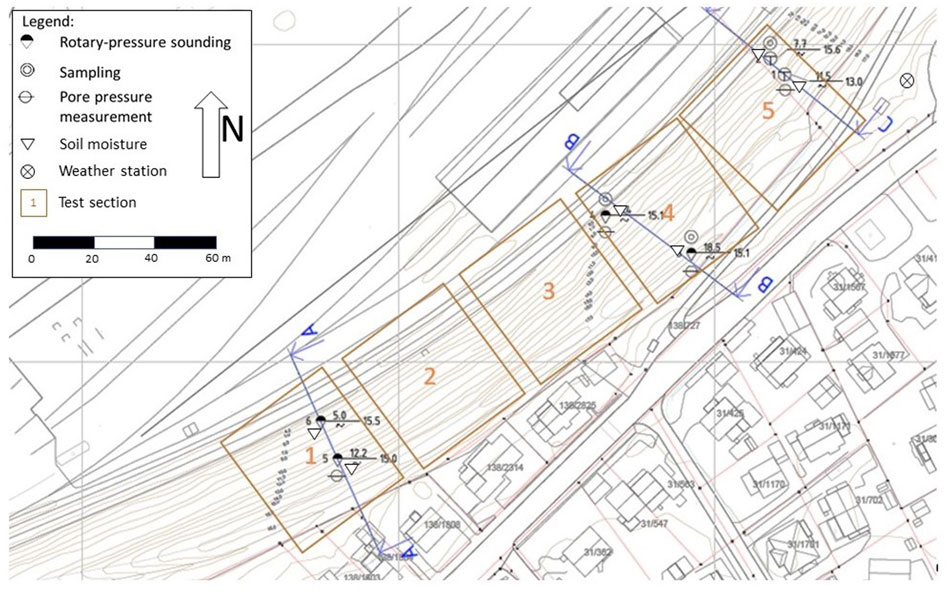
FIGURE 9. Outline of the geotechnical investigations and the five planned test fields in the slope at the Bodø Station.
The measures at the plots will, when complete, comprise traditional rock-filled drainage ditches, NBS (live fascines), two plots with innovative measures to be developed by two of the private industry partners, whereas one field is to be kept as per now for reference. The industry partners plan to test new field deployment designs/techniques using existing products, potentially creating a new market activity for these products. The NBS method is new in Norway, and requires adaptation using local plant species growing in Bodø. The cost and efficiency of implementation, as well as the maintenance requirements are also parameters of vital importance for the evaluation of the measures.
Discussion
An unfortunate potential pitfall in research, development and innovation is the development of information silos. An information silo is an insular knowledge system incapable of reciprocal operation with knowledge systems that are, or should be, related. As such, these systems have potential to share information with other systems but do not perform this in a satisfactorily way. Considerable localised knowledge is typically generated; but in a field characterised by information silos, information is not adequately shared but rather remains kept within each silo (Hadi et al., 2021).
However, broad collaboration between sectors and disciplines is crucial for research and innovation results that are practical and suitable for uptake and use by society. Such sharing and a holistic approach are crucial in the highly multi-disciplinary and multi-sectorial fields necessary for effective climate adaptation. The Sendai Framework for Disaster Risk Reduction (United Nations, 2015) clearly states that for all four priorities, cross-sectoral and cross-discipline collaboration are necessary, and that involvement of stakeholders at all levels is a necessity. This is further emphasized by the IPCC special report on Climate Change and land (IPCC, 2019), which also points at the importance of cross-sectorial and public-private cooperation in both climate change adaptation and mitigation.
Sectorial silo thinking was identified as one of the key barriers for implementing Nature Based Solutions (NBS) in climate adaptation work in urban areas by Kabisch et al. (2016). The same may be stated also for other new and innovative measures, where sectorial silos and scepticism to the “unknown” form main barriers. Wamsler et al. (2020) pointed out that sharing of experiences and knowledge between groups with different expertise and experiences is important for overcoming barriers. In their case this was linked to the context of NBS, but the conclusion is generally valid.
The CRI scheme is set up to mitigate these barriers, and to promote cross-sectoral collaboration. Promoting cross-sectorial cooperation through CRIs is an effective way to avoid discipline- and sectorial silos, in which ideas, data and information are not shared across scientific or sectoral boundaries. In Klima 2050, this is particularly relevant regarding climate adaptation, as several of the most important infrastructure owners are partners in the Centre. These comprise the Norwegian Public Road Administration, the National Railroad Administration, Statsbygg–the owner of all state-owned buildings in Norway, Avinor–which manages the civil airports in Norway, the Norwegian Energy and Water Resources Directorate as well as several building companies. All of these are involved in the active research of the centre, such as the pilot projects.
Klima 2050 performs much of its research in pilot projects, each of which can be considered research and demonstration projects. We have found this a fruitful way of developing and testing of new adaptation methods and strategies. The examples of pilot projects described above show how organizations within different fields and sectors (private–public–research) join forces to develop better methods and strategies. Although the pilots presented here focus on climate induced landslides, pilot projects also form important parts of the other work packages of Klima 2050. Examples include smart solutions for climate adaptation of buildings (WP1, Figure 3) made in close cooperation with the AEC industry partners of the CRI and innovative drainage solutions are made to mitigate against urban flooding (WP2, Figure 3), as well as networks for improved governance related to climate adaptation among municipalities in a county (WP4, Figure 3).
The value of joint pilot projects is also acknowledged by Frantzeskaki et al. (2019), who state that careful experimentation through demonstration projects can bring about powerful tools for codesign, and co-learning. Furthermore, demonstration projects provide opportunities for tracking the costs and benefits of actual “real” examples when they are of appropriate scale (Fink 2016). Such projects, in turn produce data and an evidence base for improved decision-making (Bulkeley et al., 2016; Voytenko et al., 2016).
Public–private partnerships (PPP) have become more common for large investments, for example in transport infrastructure in Norway. The Sendai Framework (United Nations, 2015) again points out that public and private investment in disaster risk prevention and reduction through structural and non-structural measures are essential to enhance the economic, social, health and cultural resilience of persons, communities, countries, and their assets, as well as the environment. These can be drivers of innovation, growth, and job creation. Such measures are cost-effective and instrumental to save lives, prevent and reduce losses and ensure effective recovery and rehabilitation. The RCN promotes these kinds of partnerships in the research and development activities in Norway through the strictly regulated partnerships in the CRIs. Results of the CRI activities will most likely lead to histories of successful collaboration between sectors, which, according to Chen et al. (2013) is likely to build social capital, and well-functioning partnerships.
Public - private partnerships have also been recognized as an important element by Seaberg et al., 2017) in their analyses of game theory applications in natural disaster management research. They found that game theory is used frequently to model both mitigation and disaster response. They also find that most research has been performed on disaster relief and suggest that future research should be more directed towards mitigation, preparedness, and recovery. This is well in line with Klima 2050 priorities, with its focus on preparedness and mitigation.
Concluding remarks
• The Centres for Research based Innovation (CRI) is a funding scheme which promotes cross-sectoral and cross-disciplinary collaboration. It also promotes public-private partnerships and is as such a well-suited instrument to overcome many of the barriers identified in the literature regarding climate adaptation strategies, as well as other themes, and as such provides excellent opportunities for innovation related to climate adaptation.
• CRI-Klima 2050 focuses on pilot projects, established as cooperative efforts between several of the centre partners. This is an efficient way of testing new methods and strategies and may also provide success histories which are important for export and upscaling of the innovations. While the pilots formally end with the completion of Klima 2050 in the spring 2023, it is the intention that these will continue as focus points for research, development, and innovation in continued cooperation between the Klima 2050 partners.
• Management of landslide risk is handled by several different entities in Norway, resulting in a wide range of regulations and guidelines. The municipalities have the final responsibility for the safety of their inhabitants but are often small and have limited resources, experience, and competence. Klima 2050 develops a common set of guidelines on how the municipalities should react when national landslide warnings are issued. The Centre has also released the web-based toolbox LaRiMiT to assist municipal planners and other stakeholders in choosing the optimal landslide mitigation measure, tailored to their specific problem and local physical and socio-economic conditions.
• Improved monitoring and early warning systems are being developed and tested in pilot projects at sites in different areas of Norway, representing different situations, topographic conditions, and climate. These systems aim at optimal traffic control (roads and railroads), particularly for areas where physical mitigation measures are not adequate or are impossible for various reasons. Information from sensors in the ground and remote sensing are combined in machine learning algorithms to issue precise warnings to be used by the relevant stakeholders.
• Results from the pilot projects are all relevant for upscaling and are transferable to other locations with similar challenges, in Norway as well as internationally. All data and results are also open and available for anyone, in accordance with Norwegian laws for publicly funded research.
Data availability statement
Publicly available datasets were analyzed in this study. This data can be found here: www.klima2050.no.
Author contributions
AS leads the Klima 2050 work package on landslides and is the lead author of the manuscript. All other co-authors have contributed equally to the manuscript.
Funding
CRI-Klima 2050 is funded by the Research Council of Norway, under grant no 237859. Part funding for the final preparation of the manuscript also came from a project funded by NordForsk; “NordicLink” (NordForsk project no. 98335), under which work at the pilot case site in Eidsvoll is also carried out.
Conflict of interest
The authors declare that the research was conducted in the absence of any commercial or financial relationships that could be construed as a potential conflict of interest.
Publisher’s note
All claims expressed in this article are solely those of the authors and do not necessarily represent those of their affiliated organizations, or those of the publisher, the editors and the reviewers. Any product that may be evaluated in this article, or claim that may be made by its manufacturer, is not guaranteed or endorsed by the publisher.
References
Agersten, S., Håvelsrud Andersen, A. S., Berger, A. C., Dyrrdal, A. V., Køltzow, M., Tunheim, K., et al. (2019). Intense byger med store konsekvenser I Sogn og Fjordane 30. METinfo no. 25/2019. Bergen, Norway: Norwegian Meteorological Institute.
Alcántara-Ayala, I., Murray, V., Daniels, P., and McBean, G. (2017). “International Council for Science (ICSU) – on the future challenges for the integration of science into international policy development for landslide disaster risk reduction,” in Advancing culture of living with landslides. 4th. Editor K. Sassa (Ljubljana, Slovenia: World Landslide Forum), 143–154. doi:10.1007/978-3-319-59469-9-10
Andersson-Sköld, Y., Bergman, R., Johansson, M., Persson, E., and Nyberg, L. (2013). Landslide risk management-A brief overview and example from Sweden of current situation and climate change. Int. J. Disaster Risk Reduct. 3, 44–61. doi:10.1016/j.ijdrr.2012.11.002
Austrian Research Promotion Agency (2016). Monitoring and evaluation concept for the COMET compe-tence centre programme. Vienna: FFG/BMWFW/BMVIT. AvaliableAt: https://www.ffg.at/sites/default/files/allgemeine_downloads/strukturprogramme/evaluier-ungskonzept_comet_2016_en.pdf.
Bondevik, S., and Sorteberg, A. (2020). Groundwater fluctuations during a debris flow event in western Norway - triggered by rain and snowmelt. Hydrol. Earth Syst. Sci. 25 (7), 4147–4158. doi:10.5194/hess-25-4147-2021
Bulkeley, H., Coenen, L., Frantzeskaki, N., Hartmann, C., Kronsell, A., Mai, L., et al. (2016). Urban living labs: Governing urban sustainability transitions. Curr. Opin. Environ. Sustain. 22, 13–17. doi:10.1016/j.cosust.2017.02.003
Capobianco, V., Uzielli, M., Kalsnes, B., Choi, J. C., Strout, J., von der Tann, L., et al. (2022). Recent innovations in the LaRiMiT risk mitigation tool: Implementing a novel methodology for expert scoring and extending the database to include nature-based solutions. Landslides 19, 1563–1583. doi:10.1007/s10346-022-01855-1
Chen, J., Chen, T. H. Y., Vertinsky, I., Yumagulova, L., and Park, C. (2013). Public-private partnerships for the development of disaster resilient communities. J. Contingencies Crisis Man. 21 (3), 130–143. doi:10.1111/1468-5973.12021
Damvad Analytics (2018). Evaluation of the scheme for research-based innovation (SFI). Report for the Research Council of Norway. Copenhagen, Denmark: Damvad Analytics, 96pp. Available at: https://www.forskningsradet.no/siteassets/publikasjoner/2018/evaluation_of_the_scheme_for-_research-based_innovation_sfi.pdf.
Devoli, G., Eidsvig, U., and Colleuille, H. (2022) Varsling av jordskredfare i Norge. Anbefalte oppgaver for lokale aktører innenfor jordskredvarslingen. Klima 2050 Report 32.
Devoli, G., Tiranti, D., Cremonini, R., Sund, M., and Boje, S. (2018). Comparison of landslide forecasting services in Piedmont (Italy) and Norway, illustrated by events in late spring 2013. Nat. Hazards Earth Syst. Sci. 18, 1351–1372. doi:10.5194/nhess-18-1351-2018
Dikau, R., and Schrott, L. (1999). The temporal stability and activity of landslides in Europe with respect to climatic change (TESLEC): Main objectives and results. Geomorphology 30, 1–12. doi:10.1016/S0169-555X(99)00040-9
Dogan, E. (2017). A strategic approach to innovation. Pressacademia 4, 290–300. doi:10.17261/pressacademia.2017.491
Dwyer, J. (2020). What is Innovation? Available At: https://digintent.com/what-is-innovation/ (accessed on April 4, 2022).
European Commission (2015). Towards an EU Research and Innovation policy agenda for nature-based solutions and re-naturing cities. Final Report of the Horizon 2020 Expert Group on “Nature-Based Solutions and Re-Naturing Cities”. Brussels: European Commission.
Finance Norway (2021). Klimarapport finans norge 2021. AvaliableAt: https://www.finansnorge.no/siteassets/skadeforsikring/klimarapporten/finans-norges-klimarapport-2021_enkeltsider.pdf.32pp
Frantzeskaki, N., McPhearson, T., Collier, M. J., Kendal, D., Bulkeley, H., Dumitru, A., et al. (2019). Nature-based solutions for urban climate change adaptation: Linking science, policy, and practice communities for evidence-based decision-making. BioScience 69 (6), 455–466. doi:10.1093/biosci/biz042
Gariano, S. L., and Guzzetti, F. (2016). Landslides in a changing climate. Earth-Science Rev. 162, 227–252. doi:10.1016/j.earscirev.2016.08.011
Hadi, A., Liu, Y., and Li, S. (2022). Transcending the silos through project management office: Knowledge transactions, brokerage roles, and enabling factors. Int. J. Proj. Manag. 40, 142–154. doi:10.1016/j.ijproman.2021.11.003
Hanssen-Bauer, I., Førland, E. J., Haddeland, I., Hisdal, H., Mayer, S., Nesje, A., et al. (2015). Climate in Norway 2100 - a knowledge base for climate adaptation. Oslo: Norwegian Climate Service Centre – NCCS Report no. 1/2017. AvaliableAt: https://klimaservicesenter.no/kss/rapporter/kin2100.
Hauser, H. (2014). Review of the Catapult network. Recommendations on the future shape, scope and ambition of the programme. London: Department for Business, Innovation and Skills. AvaliableAt: https://catapult.org.uk/wp-content/uploads/2016/04/Hauser-Review-of-the-Catapult-network-2014.pdf.
Heyerdahl, H., Hoydal, O. A., Kvistedal, Y., Gisnas, K. G., and Carotenuto, P. (2018). “Slope instrumentation and unsaturated stability evaluation for steep natural slope close to railway line,” in Unsat 2018: The 7th international conference on unsaturated soils (Hong Kong. International Society for Soil Mechanics and Geotechnical Engineering).
IPCC (2019). Climate change and land: An IPCC special report on climate change, desertification, land degradation, sustainable land management, food security, and greenhouse gas fluxes in terrestrial ecosystems. Report.
Jaedicke, C., Van Den Eeckhaut, M., Nadim, F., Hervás, J., Kalsnes, B., Vangelsten, B. V., et al. (2014). Identification of landslide hazard and risk 'hotspots' in Europe. Bull. Eng. Geol. Environ. 73, 325–339. doi:10.1007/s10064-013-0541-0
Kabisch, N., Frantzeskaki, N., Pauleit, S., Naumann, S., Davis, M., Artmann, M., et al. (2016). Nature-based solutions to climate change mitigation and adaptation in urban areas: Perspectives on indicators, knowledge gaps, barriers, and opportunities for action. E&S 21 (2), 39. doi:10.5751/es-08373-210239
Krøgli, I. K., Devoli, G., Colleuille, H., Boje, S., Sund, M., and Engen, I. K. (2018). The Norwegian forecasting and warning service for rainfall- and snowmelt-induced landslides. Nat. Hazards Earth Syst. Sci. 18, 1427–1450. doi:10.5194/nhess-18-1427-2018
Lappegard Hauge, Å., Almås, A. J., and Flyen, C. (2016). Veiledere for klimatilpasning av bygninger og infrastruktur – oversikt og tematisk analyse. Report 3-20+16.
Lappegard Hauge, Å., Moe, E., Venås, V., Flyen, C., and Kollberg Thomassen, M. (2019). Nye nettsider for klimaservice – etablering og brukerevaluering. Repport No 17.
Lisø, K. (2006). Building envelope performance assessments in harsh climates: Methods for geographically dependent design. Trondheim: Tapir Uttrykk, 185. Doctoral theses at NTNU.
Mäki, K., Forssen, K., and Vangelsten, B. V. (2015). “Factors contributing to CI vulnerability and resilience, INTACT deliverable D3.2,” in Project Co-funded by the European commission under the 7th frame-work programme; European commission (Tampere, Finland: CRC Press), 103p.
Moore, R., and McInnes, R. (2016). “Landslides and engineered slopes. Experience, theory and practice,” in Landslides and engineered slopes. Experience, theory and practice'. Proceedings of the 12th symposium on landslides, napoli, Italy, 12-19 june 2016). Editors S. Aversa, L. L. CasciniPicarelli, and C. Scavia (London: CRC Press). doi:10.1201/9781315375007
Nadim, F., Kalsnes, B. G., and Solheim, A. (2014). “Plenary: Progress of living with landslide risk in Europe,” in Landslide science for a safer society. Editors K. Sassa, P. Canuti, and Y. Yin (Cham: Springer), 1, 3–20. doi:10.1007/978-3-319-04999-1_1
Norwegian Climate Service Centre (2021). klimaservicesenter. AvaliableAt: https://klimaservicesenter.no/.
Norwegian Government (2010). Adapting to a changing climate - Norway’s vulnerability and the need to adapt to the impacts of climate change. Official Norwegian Reports NOU. AvaliableAt: https://www.regjeringen.no/contentassets/00f70698362f4f8 89cbe30c75bca4a48/pdfs/nou201020100010000en_pdfs.pdf.
Piciullo, L., Calvello, M., and Cepeda, J. M. (2018). Territorial early warning systems for rainfall-induced landslides. Earth-Science Rev. 179, 228–247. doi:10.1016/j.earscirev.2018.02.013
RCN (2021). Council of Norway. AvaliableAt: https://prosjektbanken.forskningsradet.no/explore/projects?Kilde=FORISS&distribution=Ar&chart=bar&calcType=funding&Sprak=no&sortBy=date&sortOrder=desc&resultCount=30&offset=0&ProgAkt.3=SFI-Sentre+for+forskn.drevet+innov&view=projects.
Roper, S., Du, J., and Love, J. H. (2008). Modelling the innovation value chain. Res. Policy 37, 961–977. doi:10.1016/j.respol.2008.04.005
Rouault, C., Lindsay, E., Nordal, S., and Lohne, J. (2020). Abstract title: Jølster landslide event July 2019: Predisposition, trigger and consequences. AvaliableAt: https://geologi.no/images/NGWM20/Abstractvolume_NGWM20.pdf.
Santiago Fink, H. S. (2016). Human-nature for climate action: Nature-based solutions for urban sustainability. Sustainability 8, 254. doi:10.3390/su8030254
Schilirò, L., Cepeda, J., Devoli, G., and Piciullo, L. (2021). Regional analyses of rainfall-induced landslide initiation in upper gudbrandsdalen (South-Eastern Norway) using TRIGRS model. Geosciences 11, 35. doi:10.3390/geosciences11010035
Seaberg, D., Devine, L., and Zhuang, J. (2017). A review of game theory applications in natural. Nat. Hazards 89, 1461–1483. doi:10.1007/s11069-017-3033-x
Solheim, A., Capobianco, V., Oen, A., Kalsnes, B., Wullf-Knutsen, T., Olsen, M., et al. (2021). Implementing nature-based solutions in rural landscapes: Barriers experienced in the PHUSICOS project. Sustainability 13, 1461. doi:10.3390/su13031461
Stahlecker, T. (2015). “COMET Case study,” in Public-private partnerships in research and innovation – case studies from Australia, Austria, Sweden and the United States. Working papers firms and region (Karlsruhe: Fraunhofer-ISI). AvaliableAt: http://www.isi.fraunhofer.de/isi-wAssets/docs/p/de/publikationen/forschungscampus/Forschung-scampus_case-studies_2015.pdf.
Stern, P. E., Carlberg, M., Fridholm, T., Rosemberg, C., and Terrell, M. (2013). Long term industrial im-pacts of the Swedish competence centres. VA: VINNOVA Analysis, 10.
Tichavský, R., Ballesteros-Cánovas, J. A., Šilhán, K., Tolasz, R., and Stoffel, M. (2019). Dry spells and extreme precipitation are the main trigger of landslides in Central Europe. Sci. Rep. 9, 14560. doi:10.1038/s41598-019-51148-2
Uzielli, M., Kalsnes, B., and Choi, J. C. (2017). “A web-based landslide risk mitigation portal,” in Proceedings 4th World Landslide Forum, June 2017 (Ljubljana, Slovenia: Advancing Culture of Living with Landslides). doi:10.1007/978-3-319-53487-9_50
Villa, I. T. (2021). High resolution forecasts of short duration extreme precipitation - forecasting quality and sensitivity to parameterization for two Norwegian cases. Norway: MSc. Thesis in meteorology, University of Bergen. Thesis. AvaliableAt: https://bora.uib.no/bora-xmlui/bitstream/handle/11250/2762654/Master_thesis_Villa.pdf?sequence=1.
Voytenko, Y., McCormick, K., Evans, J., and Schliwa, G. (2016). Urban Living labs for sustainability and low carbon cities in Europe: Towards a research agenda. J. Clean. Prod. 123, 45–54. doi:10.1016/j.jclepro.2015.08.053
Wamsler, C., Wickenberg, B., Hanson, H., Alkan Olsson, J. A., Stålhammar, S., Björn, H., et al. (2020). Environmental and climate policy integration: Targeted strategies for overcoming barriers to nature-based solutions and climate change adaptation. J. Clean. Prod. 247, 119154. doi:10.1016/j.jclepro.2019.119154
Keywords: landslides, Norway, pilot projects, cross-disciplinary, cross-sectorial, public-private partnership
Citation: Solheim A, Kalsnes B, Strout J, Piciullo L, Heyerdahl H, Eidsvig U and Lohne J (2022) Landslide risk reduction through close partnership between research, industry, and public entities in Norway: Pilots and case studies. Front. Earth Sci. 10:855506. doi: 10.3389/feart.2022.855506
Received: 15 January 2022; Accepted: 11 August 2022;
Published: 01 September 2022.
Edited by:
Irasema Alcántara-Ayala, Institute of Geography, National Autonomous University of Mexico, MexicoReviewed by:
Mateja Jemec Auflič, Geological Survey Ljubljana, SloveniaSusana Pereira, University of Lisbon, Portugal
Copyright © 2022 Solheim, Kalsnes, Strout, Piciullo, Heyerdahl, Eidsvig and Lohne. This is an open-access article distributed under the terms of the Creative Commons Attribution License (CC BY). The use, distribution or reproduction in other forums is permitted, provided the original author(s) and the copyright owner(s) are credited and that the original publication in this journal is cited, in accordance with accepted academic practice. No use, distribution or reproduction is permitted which does not comply with these terms.
*Correspondence: Anders Solheim, anders.solheim@ngi.no
†These authors have contributed equally to this work