Dietary therapy in abdominal aortic aneurysm — Insights from clinical and experimental studies
- 1Department of Surgery, School of Medicine, University of Virginia, Charlottesville, VA, United States
- 2School of Medicine and Health Sciences, George Washington University, Washington, DC, United States
Abdominal aortic aneurysm (AAA) is a prevalent vascular disease with high mortality rates upon rupture. Despite its prevalence in elderly populations, there remain limited treatment options; invasive surgical repair, while risky, is the only therapeutic intervention with proven clinical benefits. Dietary factors have long been suggested to be closely associated with AAA risks, and dietary therapies recently emerged as promising avenues to achieve non-invasive management of a wide spectrum of diseases. However, the role of dietary therapies in AAA remains elusive. In this article, we will summarize the recent clinical and pre-clinical efforts in understanding the therapeutic and mechanistic implications of various dietary patterns and therapeutic approaches in AAA.
Introduction
Abdominal aortic aneurysm (AAA) is a localized, progressive weakening and dilation of the aortic wall, primarily in the infrarenal segment. It is a common vascular disease in elderly populations (i.e., >65-year old), and up to 8% of males and 6% of females are estimated to develop AAA over a lifetime (1). While most cases are asymptomatic, AAA features a highly unpredictable disease course, which could culminate in the highly deadly rupture of the aneurysmal aorta (2). Ruptured AAAs have up to an 85% mortality rate, and thus far no single parameter or tool can robustly predict the risk of rupture (3). Owing to the expanded AAA screening in at-risk populations, an increasing number of diagnosed yet asymptomatic patients have been identified, albeit the majority of which are small-diameter AAAs. Unfortunately, these patients do not meet the criteria for open or endovascular repairs, which remain the standard of care (4). In these scenarios, a “watchful waiting” strategy is often employed to monitor the AAA growth rate overtime to triage patients that are considered stable; but due to the lack of effective pharmacotherapies to block AAA expansion, these patients are left unprotected from the risk of unpredictable and lethal rupture (5). The absence of therapeutic modalities alternative and complementary to surgical repairs has greatly hindered the success brought about by the AAA surveillance initiative, causing unnecessary anxiety to the patients as well as burdens to the healthcare system (6).
Strategies to counter AAA's modifiable risk factors have long been recommended as secondary preventative measures to reduce overall cardiovascular mortality risks (5). Aside from the non-modifiable risk factors such as advanced age, male gender, Caucasian race, and family history, modifiable risk factors offer potential avenues for risk reduction strategies (7–9). Tobacco smoking is arguable the strongest modifiable risk factor associated with AAA expansion and rupture, and smoking cessation has been widely and strongly recommended for all patients with AAA (10, 11). Other modifiable factors, including hypertension and atherosclerosis, have also been subjected to extensive studies. However, at present, none of the anti-hypertensive (e.g., beta blockers) or atherosclerosis medications (e.g., statins) have demonstrated clear therapeutic efficacies against AAA expansion and rupture (12).
The lack of definitive clinical benefits in the foregoing risk reduction strategies necessitates further efforts toward the first non-surgical treatment of AAA. Over the past two decades, numerous FDA-approved drugs have been repurposed for treating AAAs, yet none have yielded any clinical success (12). The most prominent example is doxycycline, a previously approved tetracycline antibiotic for anti-bacterial infections that was hypothesized to possess potent anti-AAA efficacy due to its additional benefits in inhibiting proteolytic enzyme activities and inflammasome activation. Unfortunately, after almost twenty years of active investigations, the two randomized clinical trials — the N-TA3CT trial in the US and the PHAST trial in Netherland — led to the conclusion that doxycycline had no benefits against, if not further exacerbating the expansion of small-diameter AAAs (13, 14). Other promising candidate drugs, such as metformin and sirolimus, are still far from the clinical utility at the current stage (15, 16). As such, other alternative strategies are urgently needed to address the unmet clinical need, that is a safe and effective non-invasive management of AAA.
In light of the aforementioned challenges and obstacles, recent years have witnessed a surging interest in pursuing lifestyle changes for AAA management. While smoking cessation and physical exercise both have shown promising benefits in reducing AAA risks, there is a scarcity of studies concerning the role of healthy dietary patterns, at both clinical and preclinical levels (10, 17, 18). Especially with the ever-increasing benefits of various dietary regimens against cardiovascular diseases as recently reported (19, 20), understanding the therapeutic and mechanistic implications of certain dietary patterns would hold significant value in informing the future guideline of AAA management. Herein, we will summarize the recent progress in dietary therapies for AAA based on epidemiological and experimental evidence, (see Figure 1). Additionally, we will discuss the perspectives of emerging dietary regimens and potential molecular basis.
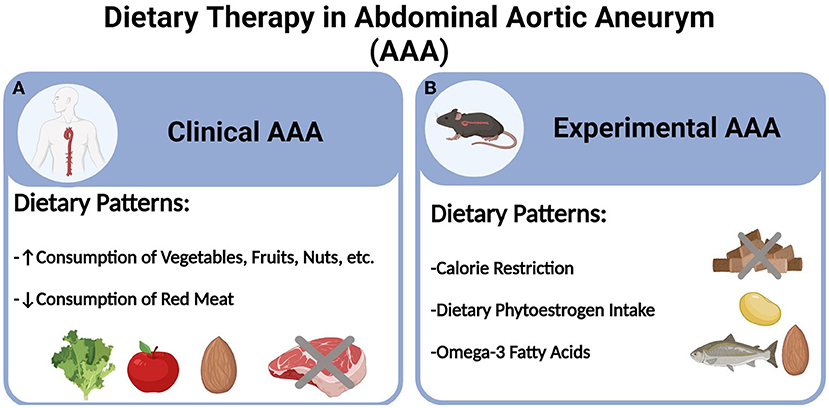
Figure 1. Dietary therapy in abdominal aortic aneurysm (AAA). (A) Clinical AAA and (B) Experimental AAA.
Clinical evidence supporting dietary regimens in AAA
In contrast to surgical repairs and drug treatments, dietary therapies are uniquely advantageous in multiple aspects, ranging from accessibility to the lack of invasiveness. For the management of cardiovascular diseases and metabolic syndromes, healthy dietary patterns have long been recommended to patients with proven benefits (19–22). However, in the case of AAA, the number of clinical studies examining the role of dietary patterns is sparse, with the epidemiological data almost exclusively derived from the following cohort studies: the Life Line Screening study, Atherosclerosis Risk in Communities (ARIC) study, the Malmö Diet and Cancer Study (MDCS), the Cohort of Swedish Men and the Swedish Mammography Cohort, and the Health In Men Study (HIMS), and most recently, the Brazilian Cardioprotective Nutritional Program Trial (23).
The first epidemiological evidence concerning dietary elements came from the Life Line Screening study in the US. In this retrospective cohort of 3.1 million patients, Kent et al. reported that consumption of nuts, vegetables, and fruits at a frequency of >three times per week were associated with reduced risk of AAA, as was the case for exercise (≥1 time per week) and smoking cessation (7). However, in this questionnaire study, fruits and vegetables were combined, thus their respective link to AAA risk was not discerned.
Built upon the seminal work from Kent et al., Stackelberg et al. continued to investigate dietary factors, particularly fruit and vegetable consumption, using the prospective Cohort of Swedish Men and Mammography Cohort (24). Surprisingly, while consumption of fruit was found to be negatively associated with the risk of AAA (particularly the ruptured ones), no link was established for vegetable consumption. In a follow-up study, the same team reported their finding that moderate alcohol consumption was inversely associated with AAA (25).
The role of dietary fiber and vegetable intake in AAA was not established until the reports from Harring et al. in 2018 and Bergwall et al. in 2019. In the former study, the ARIC study cohort was investigated regarding the association between AAA risk and their adherence to the Dietary Approaches To Stop Hypertension (DASH) dietary patterns (26). In addition to fruits, nuts, and legumes, high consumption of vegetables, whole grains, and low-fat dairy were revealed to be associated with decreased AAA burden, respectively. The latter study, conducted in the MDCS cohort, showed that linear protective associations between high intakes of fruits, berries, vegetables with AAA. Surprisingly, potato consumption was positively associated with AAA risk (27). Of particular note in both studies is the lack of statistically significant correlation between AAA risk and salt consumption, contradicting a prior population screening study in 11,742 elderly Australian males (28). Considering the uncertain clinical benefits of anti-hypertensive medications and the increasing popularity of the DASH dietary style, further experimental and clinical investigations are warranted to clarify the interventional value of such dietary patterns with restricted salt intake.
Similar to the DASH diet studied in the ARIC cohort, other similar dietary patterns have also been studied, including the anti-inflammatory diet and Mediterranean diet (29, 30). Similarly, diets high in antioxidant contents have also been recently reported to be associated with reduced AAA risks (23, 31). All these dietary patterns feature similar compositions, such as high consumption of fruit, vegetables, nuts, legumes, wholegrains, fish, and low consumption of red and processed meat. From a utility perspective, studying dietary patterns and eating styles may hold more translational value than focusing on individual food item consumption, as the former can encompass a variety of healthy dietary elements as aforementioned.
Preclinical studies concerning dietary patterns in AAA
Dietary therapies in experimental models
Notwithstanding the growing interest and epidemiological evidence, experimental studies concerning dietary therapies are very limited. Based on our literature search, three types of dietary regimens have been identified with preclinical therapeutic efficacies in murine models of AAA, i.e., calorie restriction, dietary phytoestrogen intake, and consumption of long-chain Omega-3 polyunsaturated fatty acids (PUFAs).
Calorie restriction
Calorie restriction is a dietary regimen that consists of decreased calorie intake without causing malnutrition and is often prescribed to achieve weight loss. Experimental data derived from rodent and non-human primate models support a pleiotropic role of calorie-restricted diets in mediating a myriad of health benefits, most notably in prolonging lifespan (32, 33). Data from the calorie restriction and cardiometabolic risk (CALERIE) study revealed a clear benefit of calorie restriction in reducing cardiometabolic risk factors (34). To date, only two experimental reports concerning the impact of such dietary patterns are available. Liu et al. observed profound mitigation of AAA formation using a calorie-restricted diet in angiotensin II (AngII)-infused, Apoe-/- mice (35). Additionally, they further found that sirtuin 1 (SIRT1), but not other metabolic/energy sensors such as SIRT3, mechanistic target of rapamycin (mTOR), and AMP-activated protein kinase α (AMPKα), was the main mediator of the aortic benefits exerted by calorie restriction. Such AAA-protective effect was later recapitulated using the same murine model and dietary regimen, and an alternative mediator, p53, was discovered to contribute to the aforementioned phenotype, possibly through maintaining mitochondrial bioenergetics (36).
Phytoestrogen diet
Phytoestrogens are a group of plant-derived chemicals with structural and functional similarities to 17-β-estradiol, an estrogen with a presumed protective role against AAA. The primary sources of phytoestrogen intake are through consumption of soy and many other legumes, which have been previously linked to reduced AAA risk. Using a murine model with topical elastase application, Lu et al. showed that a phytoestrogen-rich diet could effectively ameliorate AAA development in male but not in female mice (37). In line with this observation, supplementation with the phytoestrogen Daidzein attenuated AngII-induced AAA in murine models (38). However, a follow-up study by Fashandi et al. using a modified AngII model failed to demonstrate any impact of a high-phytoestrogen Western diet on AAA rupture rates or survival (39). The inconsistency amongst these studies could be potentially attributed to the different experimental models, dietary regimens, and the phytoestrogen level/compositions in different chows, as demonstrated in the work from Lu et al. Considering the striking gender dimorphism in AAA, future experimental efforts are poised to address the question concerning the link among dietary phytoestrogen, endogenous estrogen, and AAA risk (40).
Omega-3 PUFA-rich diet
Increased consumption of the PUFAs (e.g., Omega-3) — often deemed as the healthy fats — is a key component of the aforementioned diets (e.g., Mediterranean diet) featuring high consumption of fish and nuts. Indeed, numerous Omega-3 PUFAs have been studied in murine models, in which dietary supplementation could reduce AAA development (41–43). While small-scale clinical studies suggest a potential correlation between Omega-3 PUFAs and reduced AAA risk as well as early benefits in improving pre-AAA pathologies (i.e., aortic stiff), further studies are warranted to fully elucidate the role of such dietary component(s) in the clinical management of AAA (44, 45).
AAA-promoting diets in experimental models
In stark contrast to the paucity of experimental studies concerning dietary therapies, a plethora of literature are available, detailing the pathophysiological/phenotypic impacts upon experimental AAA pathogenesis. Most notable examples include dietary patterns that recapitulate AAA's established risk factors, such as high-fat diets (atherosclerosis/hypercholesterolemia) (46, 47), high-salt consumption (hypertension), excessive supplementation with homocysteine, or methionine (red meat consumption and hyperhomocysteinemia), etc (48–50).
Perspectives
Emerging dietary regimens to be explored in preclinical AAA models
Protein restriction
Similar to calorie restriction, protein restriction has also been shown to help prolong lifespan in fruit flies and mice (51, 52). In epidemiological studies, reduced intake of proteins, especially red meat consumption, has been related to reduced risk of all-cause mortality in populations aged 50–65 years. However, in elderly populations aged above 65 years that are also at risk of AAA, low protein intake rather increased, whereas high protein consumption reduced all-cause mortality (53). These observations highlight the duality behind dietary restrictions on general nutrient intake: on the one hand, such strategies have yielded pleiotropic benefits; but on the flip side, concerns of malnutrition and frailty have been persistently plaguing their widespread adoption and long-term adherence, particularly for the AAA-prone aged populations that are more susceptible to side effects like sarcopenia. Interestingly, recent studies suggest that short-term dietary restrictions, such as pre-operative protein restriction under inpatient settings, may present an alternative, viable path as dietary preconditioning to safely capitalize on the benefits of such dietary therapies. Indeed, mounting preclinical evidence has pointed to the role of pre-operative protein restriction in reducing surgical stress as well as vascular (re)stenosis. Inspired by the safety data from an exploratory trial in elective carotid endarterectomy patients (54), another trial is currently underway to chart the baseline information from healthy subjects, with the ultimate goal of comparing with patients undergoing AAA surgical repair in a future study (NCT03995979). However, sufficient protein intake has also been suggested to be critical in post-operative recovery, and hence cautions should be noted concerning the frailties caused by protein restriction (55, 56).
Amino acid restriction
Unlike calorie or protein restriction, limiting the intake of amino acid(s) has been shown to produce similar health benefits in preclinical studies without significant risks of malnutrition. Clinical studies further established the safety profile as well as the early efficacy of a methionine-restricted diet in healthy and cancer patients, with additional randomized clinical trials currently ongoing (57, 58). While restrictions of single (e.g., methionine) or multiple (e.g., branch-chained amino acids) amino acids have been studied in experimental models and even human subjects of other cardiometabolic diseases, their implications in AAA have not been reported (59–63). Considering the clinical association between certain amino acids/metabolites (e.g., homocysteine, methionine) and AAA risk, it is of significant translational value to pursue the preclinical impacts of such dietary restriction patterns in future studies.
Intermittent fasting
Intermittent fasting is a dietary pattern that features time-restricted eating with or without reducing calorie intake. Due to its reduced risk of malnutrition and ease of adherence, intermittent fasting has garnered significant popularity during the past decade as an alternative regimen. Clinical studies have suggested beneficial associations of intermittent fasting with cardiometabolic risks, albeit long-term outcomes are still pending (64, 65). In light of the aortic protection exerted by calorie restriction in murine AAA models, it is reasonable to postulate whether a similar effect could be observed with intermittent fasting. However, it is worth noting that current intermittent fasting protocols are highly variable, thus making comparisons amongst different studies difficult. A recent murine study further demonstrated that the circadian schedules of the time-restricted eating could critically determine the outcomes of different fasting protocols, which informs the challenges and critical parameters to be considered when designing future preclinical studies in AAA (66).
Emerging diet-mediated mechanisms potentially implicated in AAA
There is no pathway or molecular basis that could completely account for the health benefits of a given diet. Past studies have unveiled various mechanisms implicated in the aforementioned dietary regimens, such as overall energy expenditure reduction, antioxidant supplementation, increased production of hepatokines (e.g., FGF21) (67–71), etc. Due to the scarcity of relevant literature on AAA, we will focus on two emerging dietary mechanisms that are recently implicated in AAA pathophysiologies while more in-depth investigations are still needed.
Metabolism/nutrient sensors
Studies from other disciplines have uncovered a series of sensor proteins and pathways for cellular metabolism, energy expenditure, and certain nutrient cues. Amongst the sensors known thus far, the NAD+-dependent protein deacetylase sirtuin family member SIRT1 is the most notable example. Not only has SIRT1 been established to negatively modulate AAA pathogenesis (35, 72), experimental evidence further revealed its critical role in mediating the benefits of calorie restriction in mitigating AAA risk. Similarly, deletion of SIRT3, another sirtuin member, exacerbated, whereas its overexpression mitigated aneurysmal formation and progression (73). Another kinase commonly associated with dietary restriction is AMPKα, the activation of which has been the presumed mode of action behind established therapeutic regimens such as calorie restriction and metformin. Indeed, although no definitive link has been made between AMPKα and any diet-mediated AAA mitigation, data in murine models did suggest a protective role of AMPKα against AAA formation (74, 75); and while metformin, a calorie restriction mimetic therapy recapitulated the therapeutic benefits, AMPK blockade effectively abolished the protection against AAA (15, 74, 76).
mTOR and general control non-derepressible 2 (GCN2) constitute the only two identified sensors for amino acids (77). While mTOR is activated by recognizing the presence and intracellular level of amino acids, GCN2, on the other hand, senses amino acid starvation (78, 79). Both mTOR (inactivation) and GCN2 (activation) have been implicated in the cardiometabolic benefits of dietary restrictions (60, 80, 81). While chronic activation of mTOR was recently reported to drive aortic degeneration and hence aneurysmal formation, the specific role of GCN2 remains unknown in AAA (82). Further studies concerning GCN2 and amino acid restriction are poised to determine the clinical utility of such innovative dietary therapies.
Gut microbiome
You are what you eat. This is particularly true for the gut microbiome, as all the aforementioned diets have been shown to modulate gut microbiota (83–88). Although largely neglected as “bystanders” for a long time, the gut microbiome has been increasingly recognized to contribute to a wide range of biological and pathological processes (89). Only very recently were alterations of gut microbiota reported in experimental and clinical subjects with AAA, suggesting a microbial dysbiosis that is yet to be elucidated in aneurysm (90, 91). Although no studies thus far have investigated the exact role of diet-induced microbiota in AAA, it is highly plausible that certain dietary regimens could impact AAA pathogenesis via cultivating distinct microbiome compositions and hence driving a shift toward beneficial microbiota-derived metabolic compositions. In fact, some microbiota-derived metabolites that are profoundly inhibited upon dietary restrictions (57, 92, 93), such as trimethylamine N-oxide (TMAO), were recently revealed to contribute to AAA development (94).
Closing remarks
The past decade has witnessed tremendous progress in dietary therapies for the management of cardiometabolic diseases and cancer, yet their therapeutic and mechanistic implications in AAA are still elusive. The current study provides the first comprehensive review of preclinical and clinical evidence supporting the potential adoption of certain dietary patterns in preventing and managing AAA. Also discussed are selected emerging dietary regimens as well as diet-mediated mechanisms, in which further investigations are still pending. We envision that future research efforts will be increasingly dedicated to a better understanding of as well as translational development of the first non-surgical management of AAA in the form of dietary therapies.
Author contributions
LY and BW conceived the manuscript. AG, AR, NH, ZI, JA, QL, PP, MZ, XH, MM, and EK conducted the literature search and contributed to the drafting and editing of the manuscript. ZI, PP, and MM created the graphical abstract using BioRender.com. All authors contributed to the article and approved the submitted version.
Funding
This work was supported by the National Institute of Health (NIH) grant R01HL162895 (to BW).
Conflict of interest
Author BW is the corresponding author, the coordinator for this research topic, and has recused from the peer-review and editorial processes.
The remaining authors declare that the research was conducted in the absence of any commercial or financial relationships that could be construed as a potential conflict of interest.
Publisher's note
All claims expressed in this article are solely those of the authors and do not necessarily represent those of their affiliated organizations, or those of the publisher, the editors and the reviewers. Any product that may be evaluated in this article, or claim that may be made by its manufacturer, is not guaranteed or endorsed by the publisher.
References
1. Tang W, Yao L, Roetker NS, Alonso A, Lutsey PL, Steenson CC, et al. lifetime risk and risk factors for abdominal aortic aneurysm in a 24-year prospective study: the aric study (atherosclerosis risk in communities). Arterioscler Thromb Vasc Biol. (2016) 36:2468–77. doi: 10.1161/ATVBAHA.116.308147
2. Karthikesalingam A, Holt PJ, Vidal-Diez A, Ozdemir BA, Poloniecki JD, Hinchliffe RJ, et al. Mortality from ruptured abdominal aortic aneurysms: clinical lessons from a comparison of outcomes in England and the USA. Lancet. (2014) 383:963–9. doi: 10.1016/S0140-6736(14)60109-4
3. Force USPST, Owens DK, Davidson KW, Krist AH, Barry MJ, Cabana M, et al. Screening for abdominal aortic aneurysm: us preventive services task force recommendation statement. JAMA. (2019) 322:2211–8. doi: 10.1001/jama.2019.18928
4. Wanhainen A, Verzini F, Van Herzeele I, Allaire E, Bown M, Cohnert T, et al. Editor's choice - European Society for Vascular Surgery (ESVS) 2019 clinical practice guidelines on the management of abdominal aorto-iliac artery aneurysms. Eur J Vasc Endovasc Surg. (2019) 57:8–93. doi: 10.1016/j.ejvs.2018.09.020
5. Chaikof EL, Dalman RL, Eskandari MK, Jackson BM, Lee WA, Mansour MA, et al. The Society for Vascular Surgery practice guidelines on the care of patients with an abdominal aortic aneurysm. J Vasc Surg. (2018) 67:2–77 e2. doi: 10.1016/j.jvs.2017.10.044
6. Arnaoutakis DJ, Upchurch GRJr. Abdominal aortic aneurysm screening is safe yet lacks effectiveness. Circulation. (2019) 139:1381–3. doi: 10.1161/CIRCULATIONAHA.118.038809
7. Kent KC, Zwolak RM, Egorova NN, Riles TS, Manganaro A, Moskowitz AJ, et al. Analysis of risk factors for abdominal aortic aneurysm in a cohort of more than 3 million individuals. J Vasc Surg. (2010) 52:539–48. doi: 10.1016/j.jvs.2010.05.090
8. Lindholt JS, Juul S, Fasting H, Henneberg EW. Screening for abdominal aortic aneurysms: single centre randomized controlled trial. BMJ. (2005) 330:750. doi: 10.1136/bmj.38369.620162.82
9. van Vlijmen-van Keulen CJ, Pals G, Rauwerda JA. Familial abdominal aortic aneurysm: a systematic review of a genetic background. Eur J Vasc Endovasc Surg. (2002) 24:105–16. doi: 10.1053/ejvs.2002.1692
10. Aune D, Schlesinger S, Norat T, Riboli E. Tobacco smoking and the risk of abdominal aortic aneurysm: a systematic review and meta-analysis of prospective studies. Sci Rep. (2018) 8:14786. doi: 10.1038/s41598-018-32100-2
11. Wilmink TB, Quick CR, Day NE. The association between cigarette smoking and abdominal aortic aneurysms. J Vasc Surg. (1999) 30:1099–105. doi: 10.1016/S0741-5214(99)70049-2
12. Lindeman JH, Matsumura JS. Pharmacologic management of aneurysms. Circ Res. (2019) 124:631–46. doi: 10.1161/CIRCRESAHA.118.312439
13. Baxter BT, Matsumura J, Curci JA, McBride R, Larson L, Blackwelder W, et al. Effect of doxycycline on aneurysm growth among patients with small infrarenal abdominal aortic aneurysms: a randomized clinical trial. JAMA. (2020) 323:2029–38. doi: 10.1001/jama.2020.5230
14. Meijer CA, Stijnen T, Wasser MN, Hamming JF, van Bockel JH, Lindeman JH, et al. Doxycycline for stabilization of abdominal aortic aneurysms: a randomized trial. Ann Intern Med. (2013) 159:815–23. doi: 10.7326/0003-4819-159-12-201312170-00007
15. Yuan Z, Heng Z, Lu Y, Wei J, Cai Z. The protective effect of metformin on abdominal aortic aneurysm: a systematic review and meta-analysis. Front Endocrinol. (2021) 12:721213. doi: 10.3389/fendo.2021.721213
16. Rouer M, Xu BH, Xuan HJ, Tanaka H, Fujimura N, Glover KJ, et al. Rapamycin limits the growth of established experimental abdominal aortic aneurysms. Eur J Vasc Endovasc Surg. (2014) 47:493–500. doi: 10.1016/j.ejvs.2014.02.006
17. Aune D, Sen A, Kobeissi E, Hamer M, Norat T, Riboli E. Physical activity and the risk of abdominal aortic aneurysm: a systematic review and meta-analysis of prospective studies. Sci Rep. (2020) 10:22287. doi: 10.1038/s41598-020-76306-9
18. Weston M, Batterham AM, Tew GA, Kothmann E, Kerr K, Nawaz S, et al. Patients awaiting surgical repair for large abdominal aortic aneurysms can exercise at moderate to hard intensities with a low risk of adverse events. Front Physiol. (2016) 7:684. doi: 10.3389/fphys.2016.00684
19. Brandhorst S, Longo VD. Dietary restrictions and nutrition in the prevention and treatment of cardiovascular disease. Circ Res. (2019) 124:952–65. doi: 10.1161/CIRCRESAHA.118.313352
20. Fontana L, Meyer TE, Klein S, Holloszy JO. Long-term calorie restriction is highly effective in reducing the risk for atherosclerosis in humans. Proc Natl Acad Sci U S A. (2004) 101:6659–63. doi: 10.1073/pnas.0308291101
21. Oh M, Kim S, An KY, Min J, Yang HI, Lee J, et al. Effects of alternate day calorie restriction and exercise on cardio-metabolic risk factors in overweight and obese adults: an exploratory randomized controlled study. BMC Public Health. (2018) 18:1124. doi: 10.1186/s12889-018-6009-1
22. Huffman KM, Parker DC, Bhapkar M, Racette SB, Martin CK, Redman LM, et al. Calorie restriction improves lipid-related emerging cardiometabolic risk factors in healthy adults without obesity: distinct influences of BMI and sex from CALERIE a multicentre, phase 2, randomised controlled trial. EClin Med. (2022) 43:101261. doi: 10.1016/j.eclinm.2021.101261
23. da Silva A, Caldas APS, Pinto SL, Hermsdorff HHM, Marcadenti A, Bersch-Ferreira AC, et al. Dietary total antioxidant capacity is inversely associated with cardiovascular events and cardiometabolic risk factors: a cross-sectional study. Nutrition. (2021) 89:111140. doi: 10.1016/j.nut.2021.111140
24. Stackelberg O, Bjorck M, Larsson SC, Orsini N, Wolk A. Fruit and vegetable consumption with risk of abdominal aortic aneurysm. Circulation. (2013) 128:795–802. doi: 10.1161/CIRCULATIONAHA.112.000728
25. Stackelberg O, Bjorck M, Larsson SC, Orsini N, Wolk A. Alcohol consumption, specific alcoholic beverages, and abdominal aortic aneurysm. Circulation. (2014) 130:646–52. doi: 10.1161/CIRCULATIONAHA.113.008279
26. Haring B, Selvin E, He X, Coresh J, Steffen LM, Folsom AR, et al. Adherence to the dietary approaches to stop hypertension dietary pattern and risk of abdominal aortic aneurysm: results from the ARIC study. J Am Heart Assoc. (2018) 7:e009340. doi: 10.1161/JAHA.118.009340
27. Bergwall S, Acosta S, Sonestedt E. Intake of fibre and plant foods and the risk of abdominal aortic aneurysm in a large prospective cohort study in Sweden. Eur J Nutr. (2020) 59:2047–56. doi: 10.1007/s00394-019-02054-w
28. Golledge J, Hankey GJ, Yeap BB, Almeida OP, Flicker L, Norman PE. Reported high salt intake is associated with increased prevalence of abdominal aortic aneurysm and larger aortic diameter in older men. PLoS ONE. (2014) 9:e102578. doi: 10.1371/journal.pone.0102578
29. Kaluza J, Stackelberg O, Harris HR, Bjorck M, Wolk A. Anti-inflammatory diet and risk of abdominal aortic aneurysm in two Swedish cohorts. Heart. (2019) 105:1876–83. doi: 10.1136/heartjnl-2019-315031
30. Kaluza J, Stackelberg O, Harris HR, Akesson A, Bjorck M, Wolk A. Mediterranean diet is associated with reduced risk of abdominal aortic aneurysm in smokers: results of two prospective cohort studies. Eur J Vasc Endovasc Surg. (2021) 62:284–93. doi: 10.1016/j.ejvs.2021.04.017
31. Nordkvist S, Sonestedt E, Acosta S. Adherence to diet recommendations and risk of abdominal aortic aneurysm in the Malmo Diet and Cancer Study. Sci Rep. (2018) 8:2017. doi: 10.1038/s41598-018-20415-z
32. Weindruch R, Walford RL, Fligiel S, Guthrie D. The retardation of aging in mice by dietary restriction: longevity, cancer, immunity and lifetime energy intake. J Nutr. (1986) 116:641–54. doi: 10.1093/jn/116.4.641
33. Mattison JA, Colman RJ, Beasley TM, Allison DB, Kemnitz JW, Roth GS, et al. Caloric restriction improves health and survival of rhesus monkeys. Nat Commun. (2017) 8:14063. doi: 10.1038/ncomms14063
34. Kraus WE, Bhapkar M, Huffman KM, Pieper CF, Krupa Das S, Redman LM, et al. 2 years of calorie restriction and cardiometabolic risk (CALERIE): exploratory outcomes of a multicentre, phase 2, randomized controlled trial. Lancet Diabetes Endocrinol. (2019) 7:673–83. doi: 10.1016/S2213-8587(19)30151-2
35. Liu Y, Wang TT, Zhang R, Fu WY, Wang X, Wang F, et al. Calorie restriction protects against experimental abdominal aortic aneurysms in mice. J Exp Med. (2016) 213:2473–88. doi: 10.1084/jem.20151794
36. Gao P, Zhang H, Zhang Q, Fang X, Wu H, Wang M, et al. Caloric restriction exacerbates angiotensin II-induced abdominal aortic aneurysm in the absence of p53. Hypertension. (2019) 73:547–60. doi: 10.1161/HYPERTENSIONAHA.118.12086
37. Lu G, Su G, Zhao Y, Johnston WF, Sherman NE, Rissman EF, et al. Dietary phytoestrogens inhibit experimental aneurysm formation in male mice. J Surg Res. (2014) 188:326–38. doi: 10.1016/j.jss.2013.11.1108
38. Liu YF, Bai YQ Qi M. Daidzein attenuates abdominal aortic aneurysm through NF-kappaB, p38MAPK and TGF-beta1 pathways. Mol Med Rep. (2016) 14:955–62. doi: 10.3892/mmr.2016.5304
39. Fashandi AZ, Spinosa M, Salmon M, Su G, Montgomery W, Mast A, et al. Female mice exhibit abdominal aortic aneurysm protection in an established rupture model. J Surg Res. (2020) 247:387–96. doi: 10.1016/j.jss.2019.10.004
40. Lo RC, Bensley RP, Hamdan AD, Wyers M, Adams JE, Schermerhorn ML, et al. Gender differences in abdominal aortic aneurysm presentation, repair, and mortality in the Vascular Study Group of New England. J Vasc Surg. (2013) 57:1261–8. doi: 10.1016/j.jvs.2012.11.039
41. Wang JH, Eguchi K, Matsumoto S, Fujiu K, Komuro I, Nagai R, et al. The omega-3 polyunsaturated fatty acid, eicosapentaenoic acid, attenuates abdominal aortic aneurysm development via suppression of tissue remodeling. PLoS ONE. (2014) 9:e96286. doi: 10.1371/journal.pone.0096286
42. Yoshihara T, Shimada K, Fukao K, Sai E, Sato-Okabayashi Y, Matsumori R, et al. Omega 3 polyunsaturated fatty acids suppress the development of aortic aneurysms through the inhibition of macrophage-mediated inflammation. Circ J. (2015) 79:1470–8. doi: 10.1253/circj.CJ-14-0471
43. Kavazos K, Nataatmadja M, Wales KM, Hartland E, Williams C, Russell FD. Dietary supplementation with omega-3 polyunsaturated fatty acids modulate matrix metalloproteinase immunoreactivity in a mouse model of pre-abdominal aortic aneurysm. Heart Lung Circ. (2015) 24:377–85. doi: 10.1016/j.hlc.2014.11.005
44. Meital LT, Schulze K, Magee R, O'Donnell J, Jha P, Meital CY, et al. Long chain omega-3 polyunsaturated fatty acids improve vascular stiffness in abdominal aortic aneurysm: a randomized controlled trial. Nutrients. (2020) 13. doi: 10.3390/nu13010138
45. Aikawa T, Miyazaki T, Shimada K, Sugita Y, Shimizu M, Ouchi S, et al. Low serum levels of EPA are associated with the size and growth rate of abdominal aortic aneurysm. J Atheroscler Thromb. (2017) 24:912–20. doi: 10.5551/jat.38315
46. Saburi M, Yamada H, Wada N, Motoyama S, Sugimoto T, Kubota H, et al. Maternal high-fat diet promotes abdominal aortic aneurysm expansion in adult offspring by epigenetic regulation of irf8-mediated osteoclast-like macrophage differentiation. Cells. (2021) 10:2224. doi: 10.3390/cells10092224
47. Lu H, Howatt DA, Balakrishnan A, Graham MJ, Mullick AE, Daugherty A. Hypercholesterolemia induced by a PCSK9 gain-of-function mutation augments angiotensin II-induced abdominal aortic aneurysms in C57BL/6 mice-brief report. Arterioscler Thromb Vasc Biol. (2016) 36:1753–7. doi: 10.1161/ATVBAHA.116.307613
48. Nishida N, Aoki H, Ohno-Urabe S, Nishihara M, Furusho A, Hirakata S, et al. High salt intake worsens aortic dissection in mice: involvement of IL (Interleukin)-17A-dependent ECM (extracellular matrix) metabolism. Arterioscler Thromb Vasc Biol. (2020) 40:189–205. doi: 10.1161/ATVBAHA.119.313336
49. Liu Z, Luo H, Zhang L, Huang Y, Liu B, Ma K, et al. Hyperhomocysteinemia exaggerates adventitial inflammation and angiotensin II-induced abdominal aortic aneurysm in mice. Circ Res. (2012) 111:1261–73. doi: 10.1161/CIRCRESAHA.112.270520
50. Fan Y, Li N, Liu C, Dong H, Hu X. Excessive methionine supplementation exacerbates the development of abdominal aortic aneurysm in rats. J Vasc Res. (2019) 56:230–40. doi: 10.1159/000501313
51. Richardson NE, Konon EN, Schuster HS, Mitchell AT, Boyle C, Rodgers AC, et al. Lifelong restriction of dietary branched-chain amino acids has sex-specific benefits for frailty and lifespan in mice. Nat Aging. (2021) 1:73–86. doi: 10.1038/s43587-020-00006-2
52. Babygirija R, Lamming DW. The regulation of healthspan and lifespan by dietary amino acids. Transl Med Aging. (2021) 5:17–30. doi: 10.1016/j.tma.2021.05.001
53. Kitada M, Ogura Y, Monno I, Koya D. The impact of dietary protein intake on longevity and metabolic health. EBioMed. (2019) 43:632–40. doi: 10.1016/j.ebiom.2019.04.005
54. Kip P, Trocha KM, Tao M, O'Leary J J, Ruske J, Giulietti JM, et al. Insights from a short-term protein-calorie restriction exploratory trial in elective carotid endarterectomy patients. Vasc Endovascular Surg. (2019) 53:470–6. doi: 10.1177/1538574419856453
55. Yeung SE, Hilkewich L, Gillis C, Heine JA, Fenton TR. Protein intakes are associated with reduced length of stay: a comparison between Enhanced Recovery After Surgery (ERAS) and conventional care after elective colorectal surgery. Am J Clin Nutr. (2017) 106:44–51.
56. Weimann A, Braga M, Carli F, Higashiguchi T, Hubner M, Klek S, et al. ESPEN guideline: clinical nutrition in surgery. Clin Nutr. (2017) 36:623–50. doi: 10.1016/j.clnu.2017.02.013
57. Gao X, Sanderson SM Dai Z, Reid MA, Cooper DE, Lu M, et al. Dietary methionine influences therapy in mouse cancer models and alters human metabolism. Nature. (2019) 572:397–401. doi: 10.1038/s41586-019-1437-3
58. Hoffman RM. Clinical studies of methionine-restricted diets for cancer patients. Methods Mol Biol. (2019) 1866:95–105. doi: 10.1007/978-1-4939-8796-2_9
59. Han L, Wu G, Feng C, Yang Y, Li B, Ge Y, et al. Dietary methionine restriction improves the impairment of cardiac function in middle-aged obese mice. Food Funct. (2020) 11:1764–78. doi: 10.1039/C9FO02819F
60. Longchamp A, Mirabella T, Arduini A, MacArthur MR, Das A, Trevino-Villarreal JH, et al. Amino acid restriction triggers angiogenesis via GCN2/ATF4 regulation of VEGF and H2S production. Cell. 2018;173:117–29 e14. doi: 10.1016/j.cell.2018.03.001
61. Ables GP, Ouattara A, Hampton TG, Cooke D, Perodin F, Augie I, et al. Dietary methionine restriction in mice elicits an adaptive cardiovascular response to hyperhomocysteinemia. Sci Rep. (2015) 5:8886. doi: 10.1038/srep08886
62. Ramzan I, Taylor M, Phillips B, Wilkinson D, Smith K, Hession K, et al. A novel dietary intervention reduces circulatory branched-chain amino acids by 50%: a pilot study of relevance for obesity and diabetes. Nutrients. (2020) 13:95. doi: 10.3390/nu13010095
63. McGarrah RW, Zhang GF, Christopher BA, Deleye Y, Walejko JM, Page S, et al. Dietary branched-chain amino acid restriction alters fuel selection and reduces triglyceride stores in hearts of Zucker fatty rats. Am J Physiol Endocrinol Metab. (2020) 318:E216–E23. doi: 10.1152/ajpendo.00334.2019
64. Patikorn C, Roubal K, Veettil SK, Chandran V, Pham T, Lee YY, et al. Intermittent fasting and obesity-related health outcomes: an umbrella review of meta-analyses of randomized clinical trials. JAMA Netw Open. (2021) 4:e2139558. doi: 10.1001/jamanetworkopen.2021.39558
65. Maroofi M, Nasrollahzadeh J. Effect of intermittent vs. continuous calorie restriction on body weight and cardiometabolic risk markers in subjects with overweight or obesity and mild-to-moderate hypertriglyceridemia: a randomized trial. Lipids Health Dis. (2020) 19:216. doi: 10.1186/s12944-020-01399-0
66. Acosta-Rodriguez V, Rijo-Ferreira F, Izumo M, Xu P, Wight-Carter M, Green CB, et al. Circadian alignment of early onset caloric restriction promotes longevity in male C57BL/6J mice. Science. (2022) 376:1192–202. doi: 10.1126/science.abk0297
67. Capo X, Martorell M, Ferrer MD, Sureda A, Pons V, Domingo JC, et al. Calorie restriction improves physical performance and modulates the antioxidant and inflammatory responses to acute exercise. Nutrients. (2020) 12:930. doi: 10.3390/nu12040930
68. Most J, Redman LM. Impact of calorie restriction on energy metabolism in humans. Exp Gerontol. (2020) 133:110875. doi: 10.1016/j.exger.2020.110875
69. Hill CM, Berthoud HR, Munzberg H, Morrison CD. Homeostatic sensing of dietary protein restriction: a case for FGF21. Front Neuroendocrinol. (2018) 51:125–31. doi: 10.1016/j.yfrne.2018.06.002
70. Laeger T, Henagan TM, Albarado DC, Redman LM, Bray GA, Noland RC, et al. FGF21 is an endocrine signal of protein restriction. J Clin Invest. (2014) 124:3913–22. doi: 10.1172/JCI74915
71. Ungvari Z, Parrado-Fernandez C, Csiszar A, de Cabo R. Mechanisms underlying caloric restriction and lifespan regulation: implications for vascular aging. Circ Res. (2008) 102:519–28. doi: 10.1161/CIRCRESAHA.107.168369
72. Budbazar E, Rodriguez F, Sanchez JM, Seta F. The role of Sirtuin-1 in the vasculature: focus on aortic aneurysm. Front Physiol. (2020) 11:1047. doi: 10.3389/fphys.2020.01047
73. Qiu L, Yi S, Yu T, Hao Y. Sirt3 protects against thoracic aortic dissection formation by reducing reactive oxygen species, vascular inflammation, and apoptosis of smooth muscle cells. Front Cardiovasc Med. (2021) 8:675647. doi: 10.3389/fcvm.2021.675647
74. He J, Li N, Fan Y, Zhao X, Liu C, Hu X. metformin inhibits abdominal aortic aneurysm formation through the activation of the AMPK/mTOR signaling pathway. J Vasc Res. (2021) 58:148–58. doi: 10.1159/000513465
75. Yang L, Shen L, Gao P, Li G, He Y, Wang M, et al. Effect of AMPK signal pathway on pathogenesis of abdominal aortic aneurysms. Oncotarget. (2017) 8:92827–40. doi: 10.18632/oncotarget.21608
76. Fujimura N, Xiong J, Kettler EB, Xuan H, Glover KJ, Mell MW, et al. Metformin treatment status and abdominal aortic aneurysm disease progression. J Vasc Surg. (2016) 64:46–54 e8. doi: 10.1016/j.jvs.2016.02.020
77. Eleftheriadis T, Pissas G, Antoniadi G, Liakopoulos V, Tsogka K, Sounidaki M, et al. Differential effects of the two amino acid sensing systems, the GCN2 kinase and the mTOR complex 1, on primary human alloreactive CD4(+) T-cells. Int J Mol Med. (2016) 37:1412–20. doi: 10.3892/ijmm.2016.2547
78. Goberdhan DC, Wilson C, Harris AL. Amino acid sensing by mTORC1: intracellular transporters mark the spot. Cell Metab. (2016) 23:580–9. doi: 10.1016/j.cmet.2016.03.013
79. Battu S, Minhas G, Mishra A, Khan N. amino acid sensing via general control nonderepressible-2 kinase and immunological programming. Front Immunol. (2017) 8:1719. doi: 10.3389/fimmu.2017.01719
80. Sciarretta S, Forte M, Frati G, Sadoshima J. New insights into the role of mTOR signaling in the cardiovascular system. Circ Res. (2018) 122:489–505. doi: 10.1161/CIRCRESAHA.117.311147
81. Qin P, Arabacilar P, Bernard RE, Bao W, Olzinski AR, Guo Y, et al. Activation of the amino acid response pathway blunts the effects of cardiac stress. J Am Heart Assoc. (2017) 6:e004453. doi: 10.1161/JAHA.116.004453
82. Li G, Wang M, Caulk AW, Cilfone NA, Gujja S, Qin L, et al. Chronic mTOR activation induces a degradative smooth muscle cell phenotype. J Clin Invest. (2020) 130:1233–51. doi: 10.1172/JCI131048
83. von Schwartzenberg RJ, Bisanz JE, Lyalina S, Spanogiannopoulos P, Ang QY, Cai J, et al. Caloric restriction disrupts the microbiota and colonization resistance. Nature. (2021) 595:272–7. doi: 10.1038/s41586-021-03663-4
84. Karakan T. Intermittent fasting and gut microbiota. Turk J Gastroenterol. (2019) 30:1008. doi: 10.5152/tjg.2019.101219
85. Fan P, Liu P, Song P, Chen X, Ma X. Moderate dietary protein restriction alters the composition of gut microbiota and improves ileal barrier function in adult pig model. Sci Rep. (2017) 7:43412. doi: 10.1038/srep43412
86. Seyed Hameed AS, Rawat PS, Meng X, Liu W. Biotransformation of dietary phytoestrogens by gut microbes: a review on bidirectional interaction between phytoestrogen metabolism and gut microbiota. Biotechnol Adv. (2020) 43:107576. doi: 10.1016/j.biotechadv.2020.107576
87. Wallis KF, Melnyk SB, Miousse IR. Sex-specific effects of dietary methionine restriction on the intestinal microbiome. Nutrients. (2020) 12:781. doi: 10.3390/nu12030781
88. De Filippis F, Pellegrini N, Vannini L, Jeffery IB, La Storia A, Laghi L, et al. High-level adherence to a Mediterranean diet beneficially impacts the gut microbiota and associated metabolome. Gut. (2016) 65:1812–21. doi: 10.1136/gutjnl-2015-309957
89. Keshavarzian A, Engen P, Bonvegna S, Cilia R. The gut microbiome in Parkinson's disease: a culprit or a bystander? Prog Brain Res. (2020) 252:357–450. doi: 10.1016/bs.pbr.2020.01.004
90. Xie J, Lu W, Zhong L, Hu Y, Li Q, Ding R, et al. Alterations in gut microbiota of abdominal aortic aneurysm mice. BMC Cardiovasc Disord. (2020) 20:32. doi: 10.1186/s12872-020-01334-2
91. Zhang K, Yang S, Huang Y, Qin X, Qu K, Chen Y, et al. Alterations in gut microbiota and physiological factors associated with abdominal aortic aneurysm. Med Device Techno. (2022) 14:100122. doi: 10.1016/j.medntd.2022.100122
92. Videja M, Sevostjanovs E, Upmale-Engela S, Liepinsh E, Konrade I, Dambrova M. Fasting-mimicking diet reduces trimethylamine N-oxide levels and improves serum biochemical parameters in healthy volunteers. Nutrients. (2022) 14:1093. doi: 10.3390/nu14051093
93. Erickson ML, Malin SK, Wang Z, Brown JM, Hazen SL, Kirwan JP. Effects of lifestyle intervention on plasma trimethylamine N-oxide in obese adults. Nutrients. (2019) 11:179. doi: 10.3390/nu11010179
Keywords: diet, abdominal aortic aneurysm, dietary therapy, dietary restriction (DR), nutrient-sensing pathway, gut microbiome
Citation: Yin L, Gregg AC, Riccio AM, Hoyt N, Islam ZH, Ahn J, Le Q, Patel P, Zhang M, He X, McKinney M, Kent E and Wang B (2022) Dietary therapy in abdominal aortic aneurysm — Insights from clinical and experimental studies. Front. Cardiovasc. Med. 9:949262. doi: 10.3389/fcvm.2022.949262
Received: 20 May 2022; Accepted: 29 August 2022;
Published: 21 September 2022.
Edited by:
Zhenjie Liu, The Second Affiliated Hospital of Zhejiang University School of Medicine, ChinaReviewed by:
Lei Ji, Zhejiang University, ChinaCopyright © 2022 Yin, Gregg, Riccio, Hoyt, Islam, Ahn, Le, Patel, Zhang, He, McKinney, Kent and Wang. This is an open-access article distributed under the terms of the Creative Commons Attribution License (CC BY). The use, distribution or reproduction in other forums is permitted, provided the original author(s) and the copyright owner(s) are credited and that the original publication in this journal is cited, in accordance with accepted academic practice. No use, distribution or reproduction is permitted which does not comply with these terms.
*Correspondence: Bowen Wang, bw2pw@virginia.edu