Effects of Sacubitril-Valsartan on Clinical, Echocardiographic, and Polygraphic Parameters in Patients Affected by Heart Failure With Reduced Ejection Fraction and Sleep Apnea
- 1Department of Medical and Surgical Sciences, University Magna Græcia of Catanzaro, Catanzaro, Italy
- 2Department of Clinical and Experimental Medicine, University of Messina, Messina, Italy
- 3Department of Clinical and Molecular Medicine, Sapienza University of Rome, Rome, Italy
Background: Heart failure with reduced ejection fraction (HFrEF) is a clinical condition frequently diagnosed in clinical practice. In patients affected by HFrEF, sleep apnea (SA) can be detected among the most frequent comorbidities. Sacubitril–valsartan (sac/val) association has been proven to be effective in reducing disease progression and all-cause mortality in HFrEF patients. Sac/val treatment can potentially attenuate SA development via several pathophysiologic mechanisms, including improvement of global hemodynamics, reduction of extracellular fluid overload, and decrease of sympathetic neural activity.
Methods: We recruited 132 patients affected by HFrEF and SA, already under treatment with continuous positive airway pressure (CPAP), which was discontinued 24 h before the scheduled study timepoints. Physical examination, echocardiography, nocturnal cardio-respiratory monitoring, and laboratory tests were performed in each patient at baseline and after a 6-month treatment with sac/val.
Results: After 6 months, sac/val induced statistically significant changes in clinical, hemodynamic, biohumoral (NT-proBNP, serum electrolytes, creatinine, and uric acid), and echocardiographic parameters. In particular, cardiac index (CI), both atrial and ventricular volumes and global longitudinal strain (GLS) improved. Moreover, polysomnography, carried out during a temporary CPAP interruption, revealed a significant reduction in global apnea-hypopnea index (AHI) value (p < 0.0001), central AHI (p < 0.0001), obstructive AHI (p < 0.0001), oxygen desaturation index (ODI) (p < 0.0001), and percentage time of saturation below 90% (TC90) (p < 0.0001). The changes of CI, estimated glomerular filtration rate (eGFR), NT-proBNP, and tricuspid annular plane excursion (TAPSE) contributed to 23.6, 7.6, 7.3, and 4.8% of AHI variability, respectively, and the whole model accounted for a 43.3% of AHI variation.
Conclusions: Our results suggest that treatment with sac/val is able to significantly improve the cardiorespiratory performance of patients with HFrEF and SA, integrating the positive impact of CPAP. Thus, both CPAP and sac/val therapy may synergistically contribute to lower the risks of both cardiac and pulmonary complications in HFrEF patients with SA.
Introduction
Heart failure (HF) is a largely diffused clinical condition, being responsible for very high social and economic costs worldwide. In Western countries, about 1–2% of adult people are affected by HF, and the phenotype characterized by reduced ejection fraction (HFrEF) represents more than half of the cases (1). In spite of the relevant improvements recently achieved in pharmacological treatment of HF, prognosis remains quite poor, being characterized by high rates of hospitalization and death (2, 3). In more than 50% of patients with HF, central sleep apneas (CSA) and obstructive sleep apneas (OSA) can be detected among the most frequent comorbidities that significantly contribute to all-cause mortality (4–6). Many therapeutic strategies have been suggested to treat both CSA and OSA, but so far, no pharmacologic treatment is providing significant prognostic benefits for patients with HF (7–9). Although OSA can be regarded as an independent risk factor enhancing HF morbidity and mortality (10, 11), CSA seems to be an important indicator of HF severity, due to its association with a worse left ventricular function and an advanced New York Heart Association (NYHA) class (12). All sleep apnea (SA) phenotypes are characterized by an increased sympathetic activity, which represents a detrimental pathophysiologic condition for HF patients. In particular, an exaggerated function of the sympathetic neural pathway leads to an excessive activation of renin–angiotensin–aldosterone system (RAAS), responsible for high renal resorption of salt and water, associated with both increased heart rate (HR) and peripheral vasoconstriction (13, 14). Furthermore, hyperactivation of the adrenergic system directly causes vasoconstriction, as well as increases the overall arrhythmic risk. Therefore, SA is thought to be a potential target for therapeutic approaches which can improve the health status of HF patients (15). Whilst current evidence indicates that OSA treatment with continuous positive airway pressure (CPAP) decreases all-cause mortality in HF patients (16–18), the benefits of non-invasive ventilation (NIV) for CSA therapy are still debated (19–21), as suggested by the results of both SERVE-HF (22) and ADVENT-HF (23) randomized clinical trials.
Moreover, it is well-known that optimization of HFrEF therapy should represent the first line treatment of subjects affected by SA and HF (24, 25). In this scenario, sacubitril–valsartan (sac/val) association has been proven to be more effective than enalapril in reducing HF progression and all-cause mortality in HFrEF patients (26). This therapeutic combination inhibits neprilysin via LBQ657, the active metabolite of the prodrug sacubitril, and blocks the angiotensin II type-1 (AT1) receptor via valsartan. In a real-life setting, we have recently demonstrated that sac/val treatment is effective and safe, as shown by the long-lasting benefits including significant improvements in several clinical, hemodynamic, and echocardiographic parameters, observed in HFrEF outpatients monitored every 6 months up to 2-years (27). Indeed, sac/val is the gold-standard treatment of patients suffering from HFrEF, whose symptoms persist in spite of an optimized therapy including an angiotensin-converting enzyme inhibitor (ACE-I) or angiotensin receptor blockers (ARB), a beta-blocker, and a mineralocorticoid receptor antagonist (MRA) (26, 28, 29). Notably, sac/val treatment can potentially attenuate sleep apnea development via several mechanisms including improvement of global hemodynamics, decrease of extracellular fluid overload, and reduction of sympathetic neural activity (30).
To address this issue, we aimed to evaluate the effects of a 6-month therapy with sac/val on hemodynamic and metabolic parameters, as well as on apnea/hypopnea occurrence, and oxygen saturation in patients with HFrEF and SA, already under treatment with CPAP.
Methods
Study Design and Endpoints
The study population consisted of 132 consecutive outpatients enrolled from March 2018 to January 2020, referring to both the Chronic Heart Failure Unit of the Geriatrics Division, located at the “Mater Domini” University Hospital of Catanzaro, Italy, and the Internal Medicine Unit—Center for the Prevention, Diagnosis, and Management of Cardiovascular Disease, located at the University Hospital of Messina, Italy.
The study included outpatients complaining of HFrEF and eligible for treatment with sac/val, because of symptom persistence despite an optimized therapy. They were recruited according to the indications of the previous European Society of Cardiology (ESC) guidelines for the diagnosis and treatment of acute and chronic HF (31), which have been later updated, after the completion of our study. The eligibility criteria included: written informed consent; age ≥ 18 years; left ventricular ejection fraction (LVEF) ≤ 35%; NYHA class II–III; persistence of symptoms despite an optimized treatment with stable doses of ACE-Is or ARBs for at least 4 weeks; presence of SA under treatment with CPAP since at least 3 months. SA diagnosis was performed according to the current guidelines (32). No patient took drugs or other substances that could interfere with sleep. The exclusion criteria included: severe renal dysfunction (estimated glomerular filtrate-eGFR <30 mL/min/1.73 m2); severe hepatic impairment (Child-Pugh Class C); history of angioedema or side effects induced by ACE inhibitors or ARBs; pregnancy or breastfeeding, systolic blood pressure (SBP) < 100 mmHg; serum potassium levels > 5.4 mmol/L; current treatment with sac/val; chronic obstructive pulmonary disease (COPD) and relevant valvular heart diseases (VHD); resynchronization therapy within 3 months before the enrolment.
Complete physical examination, including assessments of body mass index (BMI), body surface area (BSA), waist circumference (WC), NYHA functional class, and quality of life were evaluated in each patient. Moreover, 12-lead electrocardiogram (ECG), echocardiography and laboratory tests aimed to evaluate metabolic disorders were also carried out. The evaluation of NYHA functional class was performed as suggested by the ESC guidelines for the diagnosis and treatment of acute and chronic HF (31). Minnesota Living with Heart Failure Questionnaire (MLHFQ) (33) and Epworth Sleepiness Scale (34) were used to evaluate quality of life. Moreover, arterial blood pressure (BP) was also checked. The local Ethics Committee approved the study protocol, and informed consent was obtained from all participants. All investigations were carried out accordingly to the principles of Helsinki Declaration.
In addition to previous treatments, eligible patients for sac/val discontinued ACE-I (at least 36 h before) or ARB, and received initial dosages of 24/26 mg or 49/51 mg bid according to clinical conditions. Moreover, sac/val dosage was increased every 2–4 weeks up to the maximum tolerated dose, as recommended. All clinical, laboratory and instrumental evaluations, such as echocardiography and nocturnal cardio-respiratory monitoring (CRM), were performed at baseline and after a 6-month treatment with sac/val. All patients were under treatment with CPAP, which provided a satisfactory SA correction. In order to evaluate the direct effects of sac/val on SA, at both baseline and 6-month timepoints CRM was performed during a temporary CPAP interruption, lasting 24 h.
Polygraphic Parameters
All patients underwent nocturnal CRM, as previously described (35). In particular, our CRM device (Somtè, Compumedics, Australia) included five cables used to record electrocardiogram through two bipolar leads, a nasal cannula assessing the flow-meter trace, a microphone which was necessary to record snoring, two piezoelectric belts utilized to detect thoraco-abdominal movements, a digital pulse oximeter measuring peripheral arterial oxyhemoglobin saturation (SpO2), and a gravity sensor able to localize patient's position. Heart rate (HR) values were also assessed. All recorded parameters were analyzed by the same operator, who was blinded to treatment protocol, and each event was judged to be either obstructive, central and/or mixed, apnoic and/or hypopnoic, according to the criteria of the American Academy of Sleep Medicine (32). The eventual presence of SA was checked, and patient population was stratified on the basis of baseline central/obstructive apnea-hypopnea index (AHI) values. Hypopneas were characterized according to a scoring system which established the threshold of 3% oxygen desaturation (32). On the basis of the presence or absence of respiratory effort, sleep apneas were classified as either obstructive or central, respectively (32). We diagnosed OSA when AHI was ≥5 events/h, and >50% of apneic events were obstructive, whilst we made a diagnosis of CSA when AHI was ≥5 events/h, and >50% of apneic events were central (32). Oxygen desaturation index (ODI) was defined as the number of desaturation episodes exceeding the percentage of 3% per hour of sleep. Moreover, we also recorded time below 90% (TC90), corresponding to the percentage time of saturation which resulted to be <90%.
Laboratory Parameters
All laboratory measurements were performed after at least 12 h of fasting. Blood levels of glucose, creatinine, uric acid (UA), total cholesterol, low-density lipoprotein (LDL) cholesterol, high-density lipoprotein (HDL) cholesterol, and triglycerides were measured. The estimation of glomerular filtration rate (eGFR) was based on the new CKD-EPI (Chronic Kidney Disease Epidemiology Collaboration) equation (36). The high sensitivity C-reactive protein (hs-CRP) and serum N-terminal pro-B-type natriuretic peptide (NT-proBNP) were also measured.
Echocardiographic Parameters
Standard left ventricular ultrasonography in both M-mode (motion mode) and B-mode (two-dimensional mode) was performed in all patients, according to the recommendations of the American Society of Echocardiography (37). Recordings were made using a VIVID E-95 ultrasound system (GE Technologies, Milwaukee, Wisconsin, USA) and a 2.5 MHz transducer. Echocardiographic parameters were detected by the same expert operator, in order to minimize measurement errors. However, the operator was not aware of patient's clinical data and the values represented the average of at least three measurements. Among the parameters of left ventricular global systolic function, LVEF, cardiac output (CO), and cardiac index (CI) were evaluated. Right ventricular systolic parameters were also measured, by estimating the systolic pulmonary arterial pressure (S-PAP). Diastolic dysfunction was detected by recording pulse-wave Doppler patterns at the mitral valve, in order to measure early (E) and late (A) diastolic filling velocities from the 4-chamber view. Assessment of the left ventricular global longitudinal strain (GLS) and multilayer parameters were obtained using a dedicated software (38).
Statistical Analysis
Continuous variables were expressed as mean ± standard deviation (SD) (normally distributed data) or as median with interquartile range (IQR) (non-normally distributed data), when appropriate. Categorical data were expressed as numbers and percentages. Normally distributed data were analyzed by t-test for paired data, whereas non-normally distributed data were analyzed by Wilcoxon's test for paired data. Subsequently, because of the absence of binary outcomes, a simple linear regression model was constructed with delta (Δ) as the dependent variable, i.e., changes in AHI between follow-up and baseline, and deltas of different variables that differed in a statistically significant manner between follow-up and baseline as independent variables. Therefore, changes in the variables that correlated significantly with changes in the dependent variable were entered into a multivariate linear regression model. Statistical analysis was carried out using SPSS V20.0 program for Windows (SPSS Inc., Chicago, Illinois, USA).
Results
The recruited subjects included 107 men (81.1%) and 25 women (18.9%), with an average age of 67 ± 9.8 years. Table 1 shows the baseline characteristics of the entire study population. In details, 59 (44.7%) patients received a treatment with sac/val 24/26 mg, 45 (34.1%) subjects assumed a dose of 49/51 mg, and 28 (21.2%) individuals received a 97/103 mg dose. Moreover, 66 (50.0%) patients had an implantable cardioverter defibrillator (ICD), and 42 (31.8%) subjects had a cardiac resynchronization therapy (CRT). After a 6-months follow-up, 39 (29.5%) patients were taking the lowest dose of sac/val (24/26), 60 (45.5%) patients the intermediate dose (49/51), and 33 (25%) the highest dose (97/103). Comparative evaluation between baseline values and 6-months follow-up data showed significant improvements in clinical and hemodynamic parameters, including reductions in HR (76.2 ± 2.8 vs. 71.7 ± 7.5 bpm; p < 0.0001), respiratory rate (RR) (17.7 ± 2.8 vs. 16.0 ± 1.8 breaths/min; p < 0.0001), SBP (122.1 ± 11.7 vs. 119.1 ± 11.7 mmHg; p < 0.0001), diastolic blood pressure (DBP) (72.4 ± 7.6 vs. 69.9 ± 6.7 mmHg; p < 0.0001). Furthermore, there was a significant change in MLHFQ score (89.5 ± 3.4 vs. 84.4 ± 4.5; p < 0.0001), indicating a relevant improvement in clinical symptoms (Table 2). After 6 months of treatment with sac/val, we also noticed significant improvements in patient functional status, as shown by the significant reduction of individual with NYHA class III from 68.9 to 22.0%; p < 0.0001.
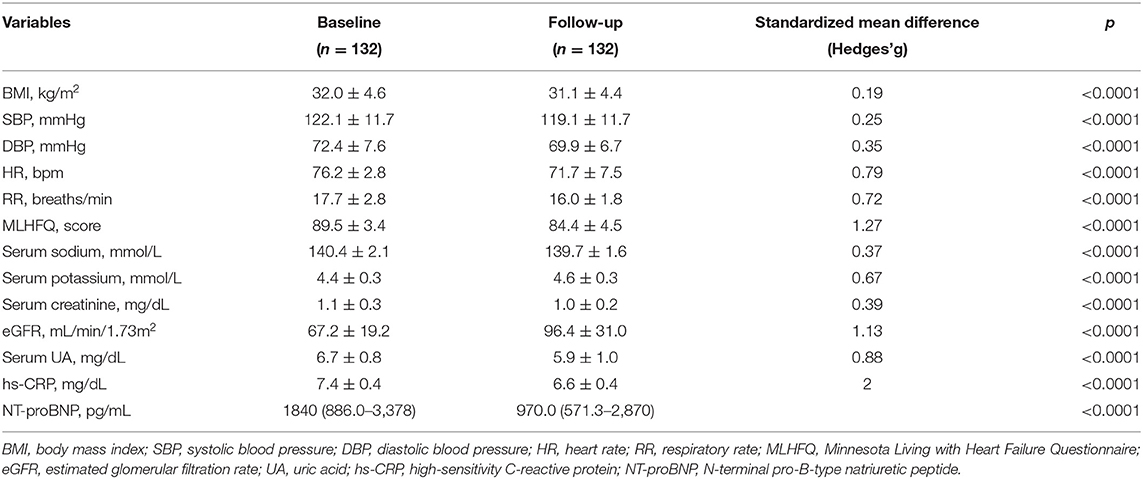
Table 2. Anthropometric, hemodynamic, and biohumoral characteristics at baseline and after 6 months of therapy with sac/val.
There were also statistically significant decreases of BMI (32.9 ± 4.6 vs. 31.1 ± 4.4 kg/m2; p < 0.0001), hs-CRP (7.4 ± 0.4 vs. 6.6 ± 0.4 mg/L; p < 0.0001), serum uric acid (6.7 ± 0.8 vs. 5.9 ± 1.0 mg/dL; p < 0.0001), NT-proBNP levels [1,840 (886.0–3,378] pg/mL vs. 970.0 (571.3–2,870) pg/mL; p < 0.0001). Furthermore, there was a significant reduction of serum creatinine levels (1.1 ± 0.3 vs. 1.0 ± 0.2 mg/dL; p < 0.0001), associated with a significant increase in eGFR (67.2 ± 19.2 vs. 96.4 ± 31.0 mL/min/1.73 m2; p < 0.0001). Additionally, a significant reduction in sodium (140.4 ± 2.1 vs. 139.7 ± 1.6 mmol/L; p < 0.0001) and potassium (4.4 ± 0.3 vs. 4.6 ± 0.3 mmol/L; p < 0.0001) serum levels were observed (Table 2).
Table 3 shows the echocardiographic characteristics of the study group. The echocardiographic analysis revealed an improvement of both left chambers diameters, as demonstrated by the reduction of LAVI, from 49.8 ± 13.7 to 46.1 ± 12.0 mL/m2 (p = 0.001). Moreover, LVEDV/BSA decreased from 89.6 ± 9.8 to 87.8 ± 8.4 mL/m2 (p < 0.0001), and LVESV/BSA from 61.0 ± 7.1 to 57.3 ± 5.9 mL/m2 (p < 0.0001), respectively. We also found significant reductions of the right chambers diameters, as shown by the changes in right ventricular outflow tract (RVOT), which decreased from 2.6 ± 0.4 to 2.1 ± 0.4 cm (p < 0.0001), and in the area of the right atrium (RAA) which decreased from 20.5 ± 2.8 to 19.3 ± 2.3 cm2 (p < 0.0001). A statistically significant reduction was found in left ventricular GLS, changing from −7.9 ± 1.7 to −9.0 ± 1.4% (p < 0.0001). Moreover, s-PAP changed from 44.5 ± 6.6 to 41.5 ± 6.6 mmHg (p < 0.0001) and E/e' decreased from 17.4 ± 3.5 to 15.9 ± 2.8 (p < 0.0001). In addition, we detected statistically significant improvement in LVEF, which increased from 31.9 ± 1.4 to 34.7 ± 1.6% (p < 0.0001), as well as in CI, which enhanced from 1,675.6 ± 199.9 to 1,856.6 ± 212.9 mL/min/m2 (p < 0.0001); inferior vena cava (IVC) diameter decreased from 20.2 ± 1.3 to 19.1 ± 3.3 mm (p < 0.0001). Furthermore, we observed a statistically significant increase in tricuspid annular plane excursion (TAPSE), which enhanced from 16.3 ± 1.1 to 17.1 ± 1.7 mm (p < 0.0001). The TAPSE/S-PAP ratio improved from 0.37 ± 0.06 to 0.42 ± 0.08 mm/mmHg (p < 0.0001).
At baseline, OSA and CSA were diagnosed in 55 (41.7%) and 77 (58.3%) patients, respectively, and all patients were under treatment with CPAP. Assessment of polygraphic parameters, evaluated during a temporary CPAP interruption, revealed a significant reduction in global AHI value (26.5 ± 10.4 vs. 21.7 ± 8.3 e/h; p < 0.0001), ODI (18.0 ± 3.7 vs. 13.5 ± 4.9 e/h; p < 0.0001), and TC90 (14.1 ± 4.5 vs. 6.8 ± 3.9%; p < 0.0001), respectively. Moreover, significant elevations were detected in mean SpO2, which increased from 91.3 ± 1.9 to 92.0 ± 2.0% (p < 0.0001) (Table 4). In addition, apnea severity improved, as indicated by the reduction of patients with severe apneas from 58 (43.9%) at baseline to 15 (11.4%) after 6 months of therapy with sac/val (p < 0.0001). Significant improvements of AHI, ODI, mean SpO2 and TC90 were also observed in both subgroups of patients with OSA (Figure 1) or CSA (Figure 2). The mean value of CPAP use time was 6.3 ± 0.17 h/night, remaining stable over time (6.3 ± 0.19 h/night, p = 0.999) thus reflecting the good patient compliance to device utilization.
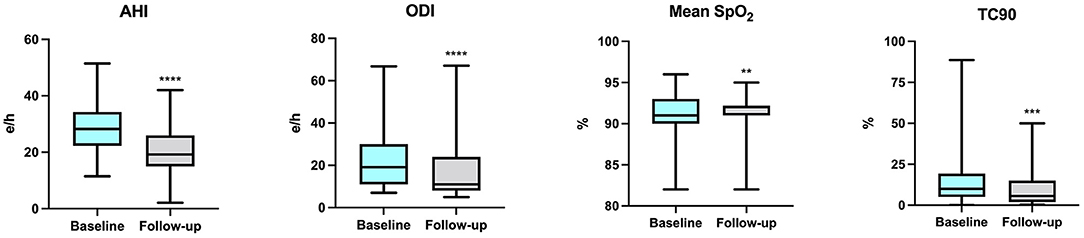
Figure 1. Changes of AHI, ODI, mean SpO2 and TC90 after 6 months of therapy with sac/val in the subgroup with OSA. AHI, apnea hypopnea index; ODI, oxygen desaturation index; SpO2, peripheral arterial oxyhemoglobin saturation; TC90, percentage time of saturation below 90%; OSA, obstructive sleep apnea. **p < 0.01; ***p < 0.001; ****p < 0.0001.
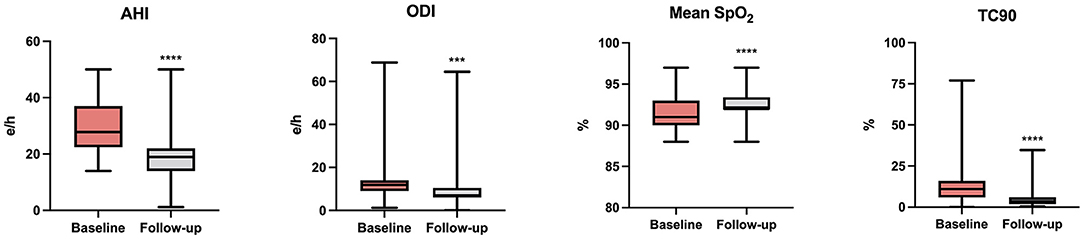
Figure 2. Changes of AHI, ODI, mean SpO2, and TC90 after 6 months of therapy with sac/val in the subgroup with CSA. AHI, apnea hypopnea index; ODI, oxygen desaturation index; SpO2, peripheral arterial oxyhemoglobin saturation; TC90, percentage time of saturation below 90%; CSA, central sleep apnea. ***p < 0.001; ****p < 0.0001.
Table 5 shows the differences occurring between the pharmacological treatments received at baseline and after 6 months of sac/val therapy, respectively. Notably, there was a significant reduction in diuretic drugs use.
Linear regression analysis using AHI variation (Δ AHI) as dependent variable revealed that AHI changes were significantly associated with variations of eGFR, CI, NT-proBNP, and TAPSE (Table 6). Moreover, variables significantly correlated to AHI changes were inserted in a multivariate linear regression model to determine the independent predictors of AHI changes. We found that the changes of CI, eGFR, NT-proBNP, and TAPSE contributed to 23.6, 7.6, 7.3, and 4.8% of AHI variability, respectively, and the whole model accounted for a 43.3% of AHI variation (Table 7).
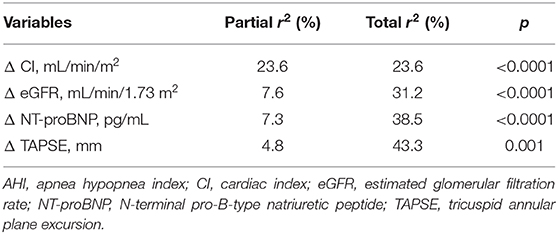
Table 7. Stepwise multiple regression analysis focused on AHI variation (Δ AHI) as dependent variable.
Therapy with sac/val was well-tolerated, and no serious adverse reactions occurred throughout this real-life observational study.
Discussion
The purpose of the present observational study was to evaluate the effects of sac/val on AHI, as well as on other clinical, functional and bio-humoral parameters patients affected by HFrEF and SA syndrome, under treatment with CPAP. In this regard, the ENTRESTO-SAS study has already shown that treatment with sac/val of patients with HFrEF and respiratory sleep disorders, induced significant AHI improvements after 3 months of therapy (39). Differently from the ENTRESTO-SAS study, our investigation was extended to 6 months, and was based not only on AHI evaluation, but also on polysomnography, as well as on the assessment of several other clinical, echocardiographic, and laboratory parameters. We observed significant improvements of AHI, ODI, TC90, mean SpO2, LVEF, CI, S-PAP, MLHFQ score, NT-proBNP, and eGFR in patients treated with sac/val for 6 months. Because all enrolled patients were on treatment with CPAP throughout the entire study period with a good adherence to non-invasive ventilation, the observed effects were likely to be attributable to the pharmacological action of sac/val. Indeed, this drug association combines angiotensin receptor antagonism and neprilysin inhibition. As a consequence of this dual mechanism of action, the resulting effective vasodilation can lead to a decrease of peripheral, and pulmonary congestion (40–43). In fact, vasodilation arises from suppression of angiotensin-dependent vasomotor tone, as well as from inhibition of degradation of vasodilatory natriuretic peptides (NP). This powerful vasodilation is further potentiated by natriuresis, ensuing from NP increased levels, and also from RAAS inhibition. The natriuretic action of sac/val also contributes to enhance eGFR and to lower serum UA levels, which are well-known cardiovascular risk factors (44, 45). Furthermore, the improved renal function decreases pulmonary congestion, thus explaining why eGFR change is a significant contributor to AHI variation in the multivariate linear regression model. Taken together, these therapeutic effects amplify a virtuous feed-forward loop, leading to LVEF and CI increases, as well as to partial reversal of cardiac remodeling (46, 47). Additionally, sac/val treatment leads to reduction in water content of lung interstitial spaces, thereby improving pulmonary congestion, ventilation dynamics, and gas exchange (48). Overall, these effects are also responsible for a decreased stimulation of lung stretch receptors, which positively impacts on respiratory mechanics (49). The increased cardiac output is paralleled by a decreased circulation time, associated with a reduced sensitiveness of the chemoreceptor reflex system to blood gas changes (50). Within the context of SA pathophysiology, the above pulmonary and cardiovascular improvements can contribute to dampen the hyperactivation of sympathetic neural pathways (51). Furthermore, sac/val treatment could reduce the cranial fluid influx occurring when patients like ours rest in supine position (52).
Similar to the characteristics of the PARADIGM-HF trial (26), also our patients experienced an overall very good response to sac/val. Moreover, our results are consistent with those of a very recent study carried out by Passino et al. (53), who showed that sac/val combination significantly improved the apnea burden due to CSA in patients with HFrEF; however, these authors did not detect significant changes in nighttime obstructive AHI referred to OSA patients. By contrast, we observed significant improvements in AHI which regarded both CSA and OSA in a quite larger number of enrolled patients. This discrepancy could be explained by the different baseline features of our patients, when compared with those enrolled by Passino et al., especially with regard to TC90 and AHI values. Additionally, despite the interruption of CPAP during the night of recording, some residual effects of mask treatment make explain the positive effects on OSA. Anyway, the two subgroups of patients (OSA and CSA), recruited in our study, do not appear to be characterized by relevant differences with regard to both body weight and BMI. In the AWAKE-HF study, the addition of sac/val therapy did not significantly improve sleep-disordered breathing or sleep duration in a cohort of patients with HFrEF, likely as a result of the short duration of the study (54). Overall, our patients responded quite well to sac/val in terms of SA improvement. However, 96 (72.7%) patients experienced the persistence of a residual AHI higher than 15, and 12 (9.1%) subjects were characterized by a residual AHI higher than 30. In particular, the persistence of about 20 events/h in both patient subgroups (OSA and CSA) suggests that the addition of further treatments should be tested, especially in regard to subjects with high loop gain OSA (e.g., acetazolamide and oxygen) (55). Acetazolamide, buspirone, and phrenic nerve stimulation could be useful for add-on treatment of CSA (56–60).
In conclusion, our results suggest that treatment with sac/val is able to significantly improve the cardiorespiratory performance of patients with HFrEF and SA. These findings are consistent with the recommendations of the current guidelines for the diagnosis and treatment of acute and chronic HF, that consider sac/val as a first-choice treatment in HFrEF (1). This real-life observation can be clinically relevant because cardiovascular diseases represent the main comorbidities of SA patients, negatively affecting both quality of life and survival. However, our study also presents some limitations. Firstly, similarly to all real-life experiences, the enrolled patients were not randomized, and, therefore, bias selection cannot be excluded. Secondly, we used CRM instead of polysomnography equipped with electroencephalography channels, which may provide a more detailed characterization of sleep patterns. A further limitation arises from the night-to-night AHI variability, which can at least in part confound the effects of sac/val (61). Moreover, although some patients can experience central apneas even at daytime, while awake and in the upright position (62, 63), we chose to focus our attention only on nighttime registration, with the aim of increasing patient adherence. A further limitation refers to a possible misclassification of hypopneas; indeed, some residual respiratory efforts can be present even in central hypopneas. Nevertheless, the present results indicate that in patients with HFrEF and SA pharmacological treatment with sac/val can represent a valuable therapeutic option, integrating the positive impact of CPAP. Thus, both CPAP and sac/val therapy may synergistically contribute to improve the global performance of these patients, as well as to significantly lower the risks of both cardiac and pulmonary complications. Of course, it cannot be ruled out that alternative pharmacologic strategies to sac/val could be useful in different patients, characterized by heart failure with either preserved ejection fraction (HFpEF) or midrange ejection fraction (HFmrEF).
Data Availability Statement
The raw data supporting the conclusions of this article will be made available by the authors, without undue reservation.
Ethics Statement
The studies involving human participants were reviewed and approved by Local Ethical Committee of Calabria Region. The patients/participants provided their written informed consent to participate in this study.
Author Contributions
All authors listed have made a substantial, direct, and intellectual contribution to the work and approved it for publication.
Conflict of Interest
The authors declare that the research was conducted in the absence of any commercial or financial relationships that could be construed as a potential conflict of interest.
Publisher's Note
All claims expressed in this article are solely those of the authors and do not necessarily represent those of their affiliated organizations, or those of the publisher, the editors and the reviewers. Any product that may be evaluated in this article, or claim that may be made by its manufacturer, is not guaranteed or endorsed by the publisher.
References
1. McDonagh TA, Metra M, Adamo M, Gardner RS, Baumbach A, Böhm M, et al. 2021 ESC Guidelines for the diagnosis and treatment of acute and chronic heart failure. Eur Heart J. (2021) 42:3599–726. doi: 10.1093/eurheartj/ehab368
2. Malik A, Gill GS, Lodhi FK, Tummala LS, Singh SN, Morgan CJ, et al. Prior heart failure hospitalization and outcomes in patients with heart failure with preserved and reduced ejection fraction. Am J Med. (2020) 133:84–94. doi: 10.1016/j.amjmed.2019.06.040
3. Wang H, Parker JD, Newton GE, Floras JS, Mak S, Chiu KL, et al. Influence of obstructive sleep apnea on mortality in patients with heart failure. J Am Coll Cardiol. (2007) 49:1625–31. doi: 10.1016/j.jacc.2006.12.046
4. Parati G, Lombardi C, Castagna F, Mattaliano P, Filardi PP, Agostoni P. Heart failure and sleep disorders. Nat Rev Cardiol. (2016) 13:389–403. doi: 10.1038/nrcardio.2016.71
5. Cowie MR, Gallagher AM. Sleep disordered breathing and heart failure: what does the future hold? JACC Heart Fail. (2017) 5:715–23. doi: 10.1016/j.jchf.2017.06.016
6. Luo Q, Zhang HL, Tao XC, Zhao ZH, Yang YJ, Liu ZH. Impact of untreated sleep apnea on prognosis of patients with congestive heart failure. Int J Cardiol. (2010) 144:420–2. doi: 10.1016/j.ijcard.2009.03.050
7. Emdin M, Passino C, Giannoni A. After the SERVE-HF trial, is there still a need for treatment of central apnea?. J Card Fail. (2015) 21:903–5. doi: 10.1016/j.cardfail.2015.09.005
8. Drager LF, McEvoy RD, Barbe F, Lorenzi-Filho G, Redline S, INCOSACT Initiative (International Collaboration of Sleep Apnea Cardiovascular Trialists). Sleep apnea and cardiovascular disease: lessons from recent trials and need for team science. Circulation. (2017) 136:1840–50. doi: 10.1161/CIRCULATIONAHA.117.029400
9. Selim BJ, Ramar K. Management of sleep apnea syndromes in heart failure. Sleep Med Clin. (2017) 12:107–21. doi: 10.1016/j.jsmc.2016.10.004
10. Gottlieb DJ, Yenokyan G, Newman AB, O'Connor GT, Punjabi NM, Quan SF, et al. Prospective study of obstructive sleep apnea and incident coronary heart disease and heart failure: the sleep heart health study. Circulation. (2010) 122:352–60. doi: 10.1161/CIRCULATIONAHA.109.901801
11. Khayat R, Jarjoura D, Porter K, Sow A, Wannemacher J, Dohar R, et al. Sleep disordered breathing and post-discharge mortality in patients with acute heart failure. Eur Heart J. (2015) 36:1463–9. doi: 10.1093/eurheartj/ehu522
12. Naughton MT. Heart failure and sleep-disordered breathing. The Chicken or the Egg?. Am J Respir Crit Care Med. (2016) 193:482–3. doi: 10.1164/rccm.201511-2176ED
13. Javaheri S, Barbe F, Campos-Rodriguez F, Dempsey JA, Khayat R, Javaheri S, et al. Sleep apnea: types, mechanisms, and clinical cardiovascular consequences. J Am Coll Cardiol. (2017) 69:841–58. doi: 10.1016/j.jacc.2016.11.069
14. Pearse SG, Cowie MR. Sleep-disordered breathing in heart failure. Eur J Heart Fail. (2016) 18:353–61. doi: 10.1002/ejhf.492
15. Piamjariyakul U, Shapiro AL, Wang K, Zulfikar R, Petitte T, Shafique S, et al. Impact of sleep apnea, daytime sleepiness, comorbidities, and depression on patients' heart failure health status. Clin Nurs Res. (2021) 30:1222–30. doi: 10.1177/10547738211015545
16. Javaheri S, Caref EB, Chen E, Tong KB, Abraham WT. Sleep apnea testing and outcomes in a large cohort of Medicare beneficiaries with newly diagnosed heart failure. Am J Respir Crit Care Med. (2011) 183:539–46. doi: 10.1164/rccm.201003-0406OC
17. Kasai T, Narui K, Dohi T, Yanagisawa N, Ishiwata S, Ohno M, et al. Prognosis of patients with heart failure and obstructive sleep apnea treated with continuous positive airway pressure. Chest. (2008) 133:690–6. doi: 10.1378/chest.07-1901
18. Damy T, Margarit L, Noroc A, Bodez D, Guendouz S, Boyer L, et al. Prognostic impact of sleep-disordered breathing and its treatment with nocturnal ventilation for chronic heart failure. Eur J Heart Fail. (2012) 14:1009–19. doi: 10.1093/eurjhf/hfs085
19. Holfinger S, Chan L, Donald R. All you need is sleep: the effects of sleep apnea and treatment benefits in the heart failure patient. Curr Heart Fail Rep. (2021) 18:144–52. doi: 10.1007/s11897-021-00506-1
20. Oates CP, Ananthram M, Gottlieb SS. Management of sleep disordered breathing in patients with heart failure. Curr Heart Fail Rep. (2018) 15:123–30. doi: 10.1007/s11897-018-0387-7
21. Naughton MT, Kee K. Sleep apnoea in heart failure: to treat or not to treat?. Respirology. (2017) 22:217–29. doi: 10.1111/resp.12964
22. Cowie MR, Woehrle H, Wegscheider K, Angermann C, d'Ortho MP, Erdmann E, et al. Adaptive servo-ventilation for central sleep apnea in systolic heart failure. N Engl J Med. (2015) 373:1095–105. doi: 10.1056/NEJMoa1506459
23. Lyons OD, Floras JS, Logan AG, Beanlands R, Cantolla JD, Fitzpatrick M, et al. Design of the effect of adaptive servo-ventilation on survival and cardiovascular hospital admissions in patients with heart failure and sleep apnoea: the ADVENT-HF trial. Eur J Heart Fail. (2017) 19:579–87. doi: 10.1002/ejhf.790
24. Javaheri S. Central sleep apnea in congestive heart failure: prevalence, mechanisms, impact, and therapeutic options. Semin Respir Crit Care Med. (2005) 26:44–55. doi: 10.1055/s-2005-864206
25. Maddox TM, Januzzi JL Jr, Allen LA, Breathett K, Butler J, Davis LL, et al. 2021 Update to the 2017 ACC expert consensus decision pathway for optimization of heart failure treatment: answers to 10 pivotal issues about heart failure with reduced ejection fraction: a report of the American College of Cardiology Solution Set Oversight Committee. J Am Coll Cardiol. (2021) 77:772–810. doi: 10.1016/j.jacc.2020.11.022
26. McMurray JJ, Packer M, Desai AS, Gong J, Lefkowitz MP, Rizkala AR, et al. Angiotensin-neprilysin inhibition versus enalapril in heart failure. N Engl J Med. (2014) 371:993–1004. doi: 10.1056/NEJMoa1409077
27. Armentaro G, D'Arrigo G, Magurno M, Toscani AF, Condoleo V, Miceli S, et al. Impact of sacubitril/valsartan on clinical and echocardiographic parameters in heart failure patients with reduced ejection fraction: data from a real life 2-year follow-up study. Front Pharmacol. (2021) 12:733475. doi: 10.3389/fphar.2021.733475
28. Mentz RJ, Xu H, O'Brien EC, Thomas L, Alexy T, Gupta B, et al. PROVIDE-HF primary results: patient-reported outcomes investigation following initiation of drug therapy with entresto (sacubitril/valsartan) in heart failure. Am Heart J. (2020) 230:35–43. doi: 10.1016/j.ahj.2020.09.012
29. Khariton Y, Fonarow GC, Arnold SV, Hellkamp A, Nassif ME, Sharma PP, et al. Association between sacubitril/valsartan initiation and health status outcomes in heart failure with reduced ejection fraction. JACC Heart Fail. (2019) 7:933–41. doi: 10.1016/j.jchf.2019.05.016
30. Jaffuel D, Molinari N, Berdague P, Pathak A, Galinier M, Dupuis M, et al. Impact of sacubitril-valsartan combination in patients with chronic heart failure and sleep apnoea syndrome: the ENTRESTO-SAS study design. ESC Heart Fail. (2018) 5:222–30. doi: 10.1002/ehf2.12270
31. Ponikowski P, Voors AA, Anker SD, Bueno H, Cleland JG, Coats AJ, et al. 2016 ESC Guidelines for the diagnosis treatment of acute chronic heart failure: the Task Force for the diagnosis treatment of acute chronic heart failure of the European Society of Cardiology (ESC). Developed with the special contribution of the Heart Failure Association (HFA) of the ESC. Eur J Heart Fail. (2016) 18:891–975. doi: 10.1002/ejhf.592
32. Berry RB, Budhiraja R, Gottlieb DJ, Gozal D, Iber C, Kapur VK, et al. Rules for scoring respiratory events in sleep: update of the 2007 AASM Manual for the Scoring of Sleep and Associated Events. Deliberations of the Sleep Apnea Definitions Task Force of the American Academy of Sleep Medicine. J Clin Sleep Med. (2012) 8:597–619. doi: 10.5664/jcsm.2172
33. Kularatna S, Senanayake S, Chen G, Parsonage W. Mapping the Minnesota living with heart failure questionnaire (MLHFQ) to EQ-5D-5L in patients with heart failure. Health Qual Life Outcomes. (2020) 18:115. doi: 10.1186/s12955-020-01368-2
34. Walker NA, Sunderram J, Zhang P, Lu SE, Scharf MT. Clinical utility of the Epworth sleepiness scale. Sleep Breath. (2020) 24:1759–65. doi: 10.1007/s11325-020-02015-2
35. Pelaia C, Armentaro G, Miceli S, Perticone M, Toscani AF, Condoleo V, et al. Association between sleep apnea and valvular heart diseases. Front Med (Lausanne). (2021) 8:667522. doi: 10.3389/fmed.2021.667522
36. Levey AS, Stevens LA, Schmid CH, Zhang YL, Castro AF 3rd, Feldman HI, et al. A new equation to estimate glomerular filtration rate. Ann Intern Med. (2009) 150:604–12. doi: 10.7326/0003-4819-150-9-200905050-00006
37. Lang RM, Badano LP, Mor-Avi V, Afilalo J, Armstrong A, Ernande L, et al. Recommendations for cardiac chamber quantification by echocardiography in adults: an update from the American Society of Echocardiography and the European Association of Cardiovascular Imaging. Eur Heart J Cardiovasc Imaging. (2015) 16:233–70. doi: 10.1093/ehjci/jev014
38. Kalam K, Otahal P, Marwick TH. Prognostic implications of global LV dysfunction: a systematic review and meta-analysis of global longitudinal strain and ejection fraction. Heart. (2014) 100:1673–80. doi: 10.1136/heartjnl-2014-305538
39. Jaffuel D, Nogue E, Berdague P, Galinier M, Fournier P, Dupuis M, et al. Sacubitril-valsartan initiation in chronic heart failure patients impacts sleep apnea: the ENTRESTO-SAS study. ESC Heart Fail. (2021) 8:2513–26. doi: 10.1002/ehf2.13455
40. Selvaraj S, Claggett B, Pozzi A, McMurray JJV, Jhund PS, Packer M, et al. Prognostic implications of congestion on physical examination among contemporary patients with heart failure and reduced ejection fraction: PARADIGM-HF. Circulation. (2019) 140:1369–79. doi: 10.1161/CIRCULATIONAHA.119.039920
41. Myhre PL, Vaduganathan M, Claggett B, Packer M, Desai AS, Rouleau JL, et al. B-type natriuretic peptide during treatment with sacubitril/valsartan: the PARADIGM-HF trial. J Am Coll Cardiol. (2019) 73:1264–72. doi: 10.1016/j.jacc.2019.01.018
42. Nougué H, Pezel T, Picard F, Sadoune M, Arrigo M, Beauvais F, et al. Effects of sacubitril/valsartan on neprilysin targets and the metabolism of natriuretic peptides in chronic heart failure: a mechanistic clinical study. Eur J Heart Fail. (2019) 21:598–605. doi: 10.1002/ejhf.1342
43. Sciacqua A, Succurro E, Armentaro G, Miceli S, Pastori D, Rengo G, et al. Pharmacological treatment of type 2 diabetes in elderly patients with heart failure: randomized trials and beyond. Heart Fail Rev. (2021). doi: 10.1007/s10741-021-10182-x. [Epub ahead of print].
44. Cassano V, Crescibene D, Hribal ML, Pelaia C, Armentaro G, Magurno M, et al. Uric Acid and vascular damage in essential hypertension: role of insulin resistance. Nutrients. (2020) 12:2509. doi: 10.3390/nu12092509
45. Pelaia C, Pastori D, Armentaro G, Miceli S, Cassano V, Barbara K, et al. Predictors of renal function worsening in patients with chronic obstructive pulmonary disease (COPD): a multicenter observational study. Nutrients. (2021) 13:2811. doi: 10.3390/nu13082811
46. Bayard G, Da Costa A, Pierrard R, Roméyer-Bouchard C, Guichard JB, Isaaz K. Impact of sacubitril/valsartan on echo parameters in heart failure patients with reduced ejection fraction a prospective evaluation. Int J Cardiol Heart Vasc. (2019) 25:100418. doi: 10.1016/j.ijcha.2019.100418
47. Romano G, Vitale G, Ajello L, Agnese V, Bellavia D, Caccamo G, et al. The effects of sacubitril/valsartan on clinical, biochemical and echocardiographic parameters in patients with heart failure with reduced ejection fraction: the “Hemodynamic Recovery”. J Clin Med. (2019) 8:2165. doi: 10.3390/jcm8122165
48. Giannoni A, Gentile F, Navari A, Borrelli C, Mirizzi G, Catapano G, et al. Contribution of the lung to the genesis of cheyne-stokes respiration in heart failure: plant gain beyond chemoreflex gain and circulation time. J Am Heart Assoc. (2019) 8:e012419. doi: 10.1161/JAHA.119.012419
49. Gunawardena S, Ravi K, Longhurst JC, Kaufman MP, Ma A, Bravo M, et al. Responses of C fiber afferents of the rabbit airways and lungs to changes in extra-vascular fluid volume. Respir Physiol Neurobiol. (2002) 132:239–1. doi: 10.1016/S1569-9048(02)00114-3
50. Mansukhani MP, Wang S, Somers VK. Chemoreflex physiology and implications for sleep apnoea: insights from studies in humans. Exp Physiol. (2015) 100:130–5. doi: 10.1113/expphysiol.2014.082826
51. Sascău R, Zota IM, Stătescu C, Boiăteanu D, Roca M, Maştaleru A, et al. Review of echocardiographic findings in patients with obstructive sleep apnea. Can Respir J. (2018) 2018:1206217. doi: 10.1155/2018/1206217
52. White LH, Bradley TD. Role of nocturnal rostral fluid shift in the pathogenesis of obstructive and central sleep apnoea. J Physiol. (2013) 591:1179–93. doi: 10.1113/jphysiol.2012.245159
53. Passino C, Sciarrone P, Vergaro G, Borrelli C, Spiesshoefer J, Gentile F, et al. Sacubitril-valsartan treatment is associated with decrease in central apneas in patients with heart failure with reduced ejection fraction. Int J Cardiol. (2021) 330:112–9. doi: 10.1016/j.ijcard.2021.02.012
54. Owens RL, Birkeland K, Heywood JT, Steinhubl SR, Dorn J, Grant D, et al. Sleep outcomes from AWAKE-HF: a randomized clinical trial of sacubitril/valsartan vs enalapril in patients with heart failure and reduced ejection fraction. J Card Fail. (2021) 27:1466–71. doi: 10.1016/j.cardfail.2021.07.021
55. White DP. Pharmacologic approaches to the treatment of obstructive sleep apnea. Sleep Med Clin. (2016) 11:203–12. doi: 10.1016/j.jsmc.2016.01.007
56. Javaheri S. Acetazolamide improves central sleep apnea in heart failure: a double-blind, prospective study. Am J Respir Crit Care Med. (2006) 173:234–7. doi: 10.1164/rccm.200507-1035OC
57. Ni YN, Yang H, Thomas RJ. The role of acetazolamide in sleep apnea at sea level: a systematic review and meta-analysis. J Clin Sleep Med. (2021) 17:1295–304. doi: 10.5664/jcsm.9116
58. Giannoni A, Borrelli C, Mirizzi G, Richerson GB, Emdin M, Passino C. Benefit of buspirone on chemoreflex and central apnoeas in heart failure: a randomized controlled crossover trial. Eur J Heart Fail. (2021) 23:312–20. doi: 10.1002/ejhf.1854
59. Costanzo MR, Ponikowski P, Javaheri S, Augostini R, Goldberg L, Holcomb R, et al. Transvenous neurostimulation for central sleep apnoea: a randomised controlled trial. Lancet. (2016) 388:974–82. doi: 10.1016/S0140-6736(16)30961-8
60. Fox H, Oldenburg O, Javaheri S, Ponikowski P, Augostini R, Goldberg LR, et al. Long-term efficacy and safety of phrenic nerve stimulation for the treatment of central sleep apnea. Sleep. (2019) 42:zsz158. doi: 10.1093/sleep/zsz158
61. Hayano J, Yuda E. Night-to-night variability of sleep apnea detected by cyclic variation of heart rate during long-term continuous ECG monitoring. Ann Noninvasive Electrocardiol. (2021) 27:e12901. doi: 10.1111/anec.12901
62. Emdin M, Mirizzi G, Giannoni A, Poletti R, Iudice G, Bramanti F, et al. Prognostic significance of central apneas throughout a 24-hour period in patients with heart failure. J Am Coll Cardiol. (2017) 70:1351–64. doi: 10.1016/j.jacc.2017.07.740
Keywords: heart failure, sleep apnea, sacubitril-valsartan, echocardiography, apnea-hypopnea index
Citation: Pelaia C, Armentaro G, Volpentesta M, Mancuso L, Miceli S, Caroleo B, Perticone M, Maio R, Arturi F, Imbalzano E, Andreozzi F, Perticone F, Sesti G and Sciacqua A (2022) Effects of Sacubitril-Valsartan on Clinical, Echocardiographic, and Polygraphic Parameters in Patients Affected by Heart Failure With Reduced Ejection Fraction and Sleep Apnea. Front. Cardiovasc. Med. 9:861663. doi: 10.3389/fcvm.2022.861663
Received: 24 January 2022; Accepted: 11 March 2022;
Published: 05 April 2022.
Edited by:
Atsushi Tanaka, Saga University, JapanReviewed by:
Eiichi Watanabe, Fujita Health University, JapanAlberto Giannoni, Sant'Anna School of Advanced Studies, Italy
Copyright © 2022 Pelaia, Armentaro, Volpentesta, Mancuso, Miceli, Caroleo, Perticone, Maio, Arturi, Imbalzano, Andreozzi, Perticone, Sesti and Sciacqua. This is an open-access article distributed under the terms of the Creative Commons Attribution License (CC BY). The use, distribution or reproduction in other forums is permitted, provided the original author(s) and the copyright owner(s) are credited and that the original publication in this journal is cited, in accordance with accepted academic practice. No use, distribution or reproduction is permitted which does not comply with these terms.
*Correspondence: Angela Sciacqua, sciacqua@unicz.it
†These authors have contributed equally to this work