Metagenomic Next-Generation Sequencing Versus Traditional Laboratory Methods for the Diagnosis and Treatment of Infection in Liver Transplantation
- 1Liver Surgery Intensive Care Unit, Department of Intensive Care Medicine, Zhongshan Hospital, Fudan University, Shanghai, China
- 2Department of Liver Surgery and Transplantation, Liver Cancer Institute, Zhongshan Hospital, Fudan University; Key Laboratory of Carcinogenesis and Cancer Invasion (Fudan University), Ministry of Education, Shanghai, China
- 3Shanghai Key Laboratory of Organ Transplantation, Zhongshan Hospital, Fudan University, Shanghai, China
- 4Department of Infectious Diseases, Zhongshan Hospital, Fudan University, Shanghai, China
- 5Cancer Research Institute, Central South University; Key Laboratory of Carcinogenesis and Cancer Invasion, Ministry of Education, Changsha, China
- 6Institute of Biomedical Sciences, Fudan University, Shanghai, China
Background: Metagenomic next-generation sequencing (mNGS) has emerged as an effective method for the noninvasive and precise detection of infectious pathogens. However, data are lacking on whether mNGS analyses could be used for the diagnosis and treatment of infection during the perioperative period in patients undergoing liver transplantation (LT).
Methods: From February 2018 to October 2018, we conducted an exploratory study using mNGS and traditional laboratory methods (TMs), including culture, serologic assays, and nucleic acid testing, for pathogen detection in 42 pairs of cadaveric liver donors and their corresponding recipients. Method performance in determining the presence of perioperative infection and guiding subsequent clinical decisions was compared between mNGS and TMs.
Results: The percentage of liver donors with mNGS-positive pathogen results (64.3%, 27/42) was significantly higher than that using TMs (28.6%, 12/42; P<0.05). The percentage of co-infection detected by mNGS in liver donors was 23.8% (10/42) significantly higher than 0.0% (0/42) by TMs (P<0.01). Forty-three pathogens were detected using mNGS, while only 12 pathogens were identified using TMs. The results of the mNGS analyses were consistent with results of the TM analyses in 91.7% (11/12) of donor samples at the species level, while mNGS could be used to detect pathogens in 66.7% (20/30) of donors deemed pathogen-negative using TMs. Identical pathogens were detected in 6 cases of donors and recipients by mNGS, among which 4 cases were finally confirmed as donor-derived infections (DDIs). For TMs, identical pathogens were detected in only 2 cases. Furthermore, 8 recipients developed early symptoms of infection (<7 days) after LT; we adjusted the type of antibiotics and/or discontinued immunosuppressants according to the mNGS results. Of the 8 patients with infections, 7 recipients recovered, and 1 patient died of severe sepsis.
Conclusions: Our preliminary results show that mNGS analyses can provide rapid and precise pathogen detection compared with TMs in a variety of clinical samples from patients undergoing LT. Combined with symptoms of clinical infection, mNGS showed superior advantages over TMs for the early identification and assistance in clinical decision-making for DDIs. mNGS results were critical for the management of perioperative infection in patients undergoing LT.
Introduction
Liver transplantation (LT) is the most effective treatment for end-stage liver cirrhosis and liver cancer (Dogan and Kutluturk, 2020). The civilian organ donation program has been the sole source of organs for transplant in China since January 2015, and the number of voluntary donations has increased every year (Huang et al., 2015). Infection-related complications have become the leading cause of morbidity and mortality for patients undergoing LT due to the use of immunosuppressive agents (Nam et al., 2018). Perioperative infections are particularly serious and can lead to liver graft failure and even death (Heldman et al., 2019). Such infections in liver recipients can arise from reactivation of latent pathogens, donor-derived infections (DDIs), or primary infections (Pettengill et al., 2019). The early and precise detection of infectious pathogens can be used to optimize the administration of antibiotics and immunosuppressants to improve clinical outcomes for patients undergoing LT (Huang et al., 2020). Therefore, development of a more rapid, sensitive, and specific method for the identification of potential pathogens for these patients is urgently needed.
Traditional laboratory methods (TMs) for the screening of potential pathogens usually include cell culture, serologic assays, and nucleic acid testing. However, testing all potential pathogens in liver donors and corresponding recipients using TMs is extremely time-consuming. Metagenomic next-generation sequencing (mNGS) is a promising approach to determine the presence and abundance of transplant-related infections and identify co-infection in an unbiased manner (Simner et al., 2018). The use of mNGS can overcome the limitations of current diagnostic tests, allowing for hypothesis-free, culture-independent, pathogen detection directly from clinical specimens regardless of the type of microbe; mNGS can even be used for novel organism discovery (Simner et al., 2018). To date, there are few reports on the use of mNGS to identify potential pathogens in liver donors and their corresponding recipients.
In this study, the diagnostic performance of mNGS was evaluated and compared with the use of TMs in patients undergoing LT. Furthermore, the feasibility of using mNGS for the diagnosis and treatment of perioperative infections in LT recipients was evaluated. We found that the use of mNGS provided rapid and precise detection of pathogens compared with TMs in a variety of clinical samples from patients undergoing LT. Combined with symptoms of clinical infection, the use of mNGS could offer an advantage over the use of TMs for the diagnosis of DDIs and the precise treatment of these perioperative infections.
Materials and Methods
Ethics Statement
An application for ethical review was approved by the Ethical Review Committee of Zhongshan Hospital affiliated with Fudan University.
Patients, Perioperative Management, and Sample Collection
This study was a single-center, prospective cohort study from February 1, 2018 to October 30, 2018. A total of 42 cadaveric liver donors and their corresponding recipients were enrolled. All donors’ clinical data were obtained prior to procurement. All recipients received orthotropic LT and induction of immunosuppression intraoperatively with basiliximab and methylprednisolone. The regimen for antibiotic prophylaxis for LT consisted of cefepime and micafungin for 7 days postoperatively. Immunosuppressant therapy after LT consisted of a triple-drug regimen of cyclosporine or tacrolimus, mycophenolate mofetil, and methylprednisolone; the doses of these drugs were decreased over 7 days. Recipient outcomes were examined for the entire length of the hospital stay.
The donor’s samples, including blood, preservation fluid, liver and perihepatic tissue (diaphragm or omentum), were obtained preoperatively. Microbiological monitoring of the liver recipients involved the routine sampling of blood and abdominal drainage fluid on postoperative days (POD) 1, 4, and 7. When the recipient was diagnosed with a postoperative infection, additional samples from the sputum, bronchoalveolar lavage fluid, and urine were collected for pathogen analysis according to the clinical situation. All samples were subjected to TMs as well as mNGS testing in a pairwise manner. TMs for pathogen detection included culture of bacterial and fungal; PCR-based assay of Epstein-Barr virus (EBV), Cytomegalovirus (CMV), Hepatitis B virus (HBV), Hepatitis C virus (HCV); serological assay including 1,3-Beta-D-glucan, Galactomannan antigen, Interferon-gamma release assays for Tuberculosis, Cryptococcus antigen, HBV, HCV and Human immunodeficiency virus (HIV) serological test, EBV early antigen and viral capsid antigen, CMV immunoglobulin G/M (IgG/M), Toxoplasma gondii IgG/M, Rapid plasma reagin (RPR) test for Syphilis and stool microscopy for parasitic ova. The diagnostic assessment performances of the TMs and mNGS were compared.
Sample Processing
All samples were promptly stored in sterile containers and placed at 4°C prior to analysis. For blood samples, 3–4 mL of blood was centrifuged at 4,000 rpm for 10 min at 4°C within 8 h of collection, and plasma samples were transferred to new sterile tubes. An aliquot of 3–5 mL preservation fluid or drainage fluid was collected, according to standard sterile procedures (Cornaglia et al., 2012). Tissue homogenates, including those of liver and perihepatic tissues, were processed similarly to preservation fluid (Cornaglia et al., 2012); 1.5-mL microcentrifuge tubes containing 0.5 mL sample and 1 g of 0.5-mm glass beads were attached to a horizontal platform on a vortex mixer and agitated vigorously at 2,800-3,200 rpm for 30 min.
mNGS
DNA was extracted from 300 µL samples using the TIANamp Micro DNA Kit (DP316, TIANGEN BIOTECH, Beijing, China), following the manufacturer’s instructions. DNA libraries were constructed through DNA fragmentation, end-repair, adapter-ligation, and PCR amplification (Long et al., 2016). The reagents were taken out from the kit, and the enzymatic reagents were briefly centrifuged and placed on ice for use; The other reagents were melted on ice, mixed with oscillation, and briefly centrifuged for use. Magnetic beads should be balanced at room temperature for 30min before use, and thoroughly mixed before adding. Anhydrous ethanol and molecular water are used to prepare 75% ethanol. internal standard (200×) was diluted 200 times in nuclease-free water. Then, the terminal repair reaction mixture was prepared for end-repair. The extracted nucleic acid was added, and then the terminal repair reaction mixture 7.0μL was added. The mixture was placed on PCR and incubated. At the end of the reaction, the PCR tube was removed for instantaneous centrifugation. 30.0μL connecting reaction mixture was added to the PCR tube, and the mixture was fully mixed and centrifuged immediately. The mixture was placed on the PCR instrument and incubated for 23 minutes, ligase was used for adapter-ligation. After PCR amplification, Agilent 2100 instrument (Agilent Technologies, Santa Clara, CA) was used for quality control of the DNA libraries and the Qubit 2.0 fluorometer (Invitrogen, Foster City, CA, USA). A qualified double-stranded DNA library was transformed into a single-stranded circular DNA library by DNA denaturation and circularization. DNA nanoballs (DNBs) were generated from single-stranded circular DNA using rolling circle amplification (da Silva et al., 2016). The DNBs were qualified by fluorometry (Fang et al., 2018). Qualified DNBs were loaded in the flow cell and sequenced on the BGISEQ-50 platform (Jeon et al., 2014). High-quality sequencing data were generated by removing low-quality and short reads (length <35 bp), followed by computational subtraction of human host sequences mapped to the human reference genome (hg19) using Burrows-Wheeler alignment (Li and Durbin, 2010). After removal of low-complexity reads, the remaining sequencing data were classified by simultaneous alignment to sequences in the bacterial, viral, fungal, and parasite microbial genome databases.
The reference database RefSeq, downloaded from the National Center Biotechnology Information website (https://ftp.ncbi.nlm.nih.gov/genomes/), contains 4,945 whole-genome sequences of viral taxa, 6,350 bacterial genomes or scaffolds, 1,064 fungal sequences related to human infections, and 234 parasite sequences associated with human diseases.
Analyses of mNGS Results
The criteria for a positive mNGS result have been described previously (Miao et al., 2018). Briefly, bacteria, viruses, and parasites (species level) were identified with a coverage rate 10-fold greater than that of any other bacteria, virus, or parasite. Fungi (species level) were identified with a coverage rate 5-fold higher than that of any other fungi because of their low biomass after DNA extraction. Mycobacterium tuberculosis was considered positive when at least one read was mapped (genus or species level). Nontuberculous mycobacteria were considered positive when the mapped read number at either the species or genus level was in the top 10 of the list of bacteria.
The number of unique reads of standardized species (SDSMRN) was defined as the number of reads that were strictly aligned to the genome of a species after normalizing the total number of sequencing reads to 20 million (Li et al., 2020). Probable DDI was defined as the transmission of the identical pathogen detected from donor to recipient by mNGS and/or TMs (Kaul et al., 2021) and the recipient developed early infection symptoms (<7 days) as fever and/or purulent drainage observed with increased markers of laboratory infection after LT (Bandali et al., 2020).
Statistical Analysis
Sensitivity, specificity, positive predictive value (PPV), negative predictive value (NPV), and accuracy (ACC) were calculated, and the performance of mNGS and TMs for diagnostic assessments was compared using the χ2 test. A two-tailed P value of 0.05 was considered statistically significant. Data were analyzed using SPSS, version 24.0 (SPSS, Chicago, IL, USA).
Results
Recipient Characteristics
Demographic features of the recipients in the study are provided in Table 1. All 42 recipients underwent orthotopic cadaveric LT. The median patient age was 49 years (range, 21-72 years). Most recipients were male (36/42, 85.7%) and had been diagnosed with primary malignant liver cancer (29/42, 69.1%), followed by decompensated liver cirrhosis (9/42, 21.5%). Ascites was present in 81.0% of recipients (34/42), and antibiotics were used in 33.3% of recipients (14/42) one month before LT for either the treatment of infection or prevention of spontaneous peritonitis due to cirrhosis with ascites or upper gastrointestinal bleeding. Of the 42 patients, 8 recipients were diagnosed with postoperative infection, with pneumonia (6/8, 75.0%) being the most common infection.
The Spectrum of Pathogens in Liver Donors Detected by mNGS and TMs
The percentage of liver donors with mNGS-positive pathogen results (64.3%, 27/42) was significantly higher than when using TMs (28.6%, 12/42; P<0.05), and the percentage of co-infection of several common pathogens, detected by mNGS in liver donors was 23.8% (10/42) compared with 0.0% as detected by TMs (0/42; P<0.001; Figure 1).
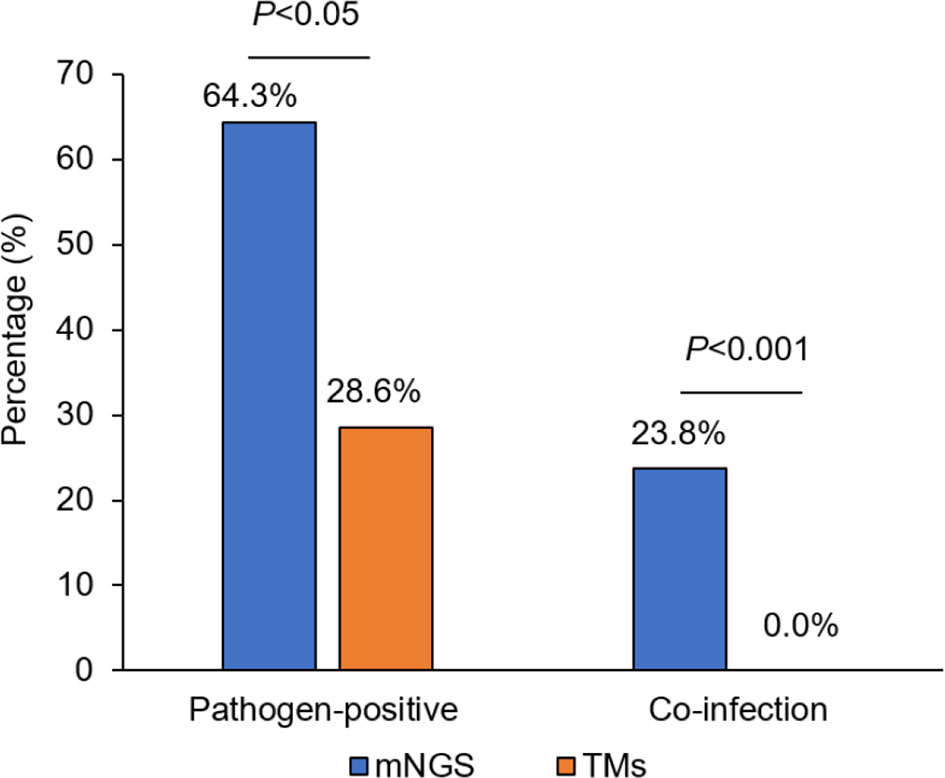
Figure 1 The comparison of pathogen-positive percentage and co-infection rate detected separately by mNGS and TMs in liver donors. mNGS for metagenomic next-generation sequencing and TMs for traditional laboratory methods.
mNGS detected 43 pathogens (bacteria: 55.8%, 24/43; viruses: 25.6%, 11/43; fungi: 14.0%, 6/43; parasites: 4.7%, 2/43), whereas only 12 pathogens were identified using TMs (bacteria: 75.0%, 9/12; viruses: 25.0%, 3/12; Figures 2A, B). No fungi or parasites were identified using TMs. Among 30 donors who tested negative for pathogens using TMs, mNGS identified new pathogens in 20 cases (20/30, 66.7%), including fastidious bacteria, fungi [e.g., Pneumocystis jirovecii and Candida albicans (CA)], virus [e.g., Torque Teno Virus (TTV), Human parvovirus B19, CMV and EBV] and parasite [e.g., Echinococcus multilocularis and Clonorchis sinensis]. This result reflected the low sensitivity of TMs in screening donor-derived pathogens (Figure 2C).
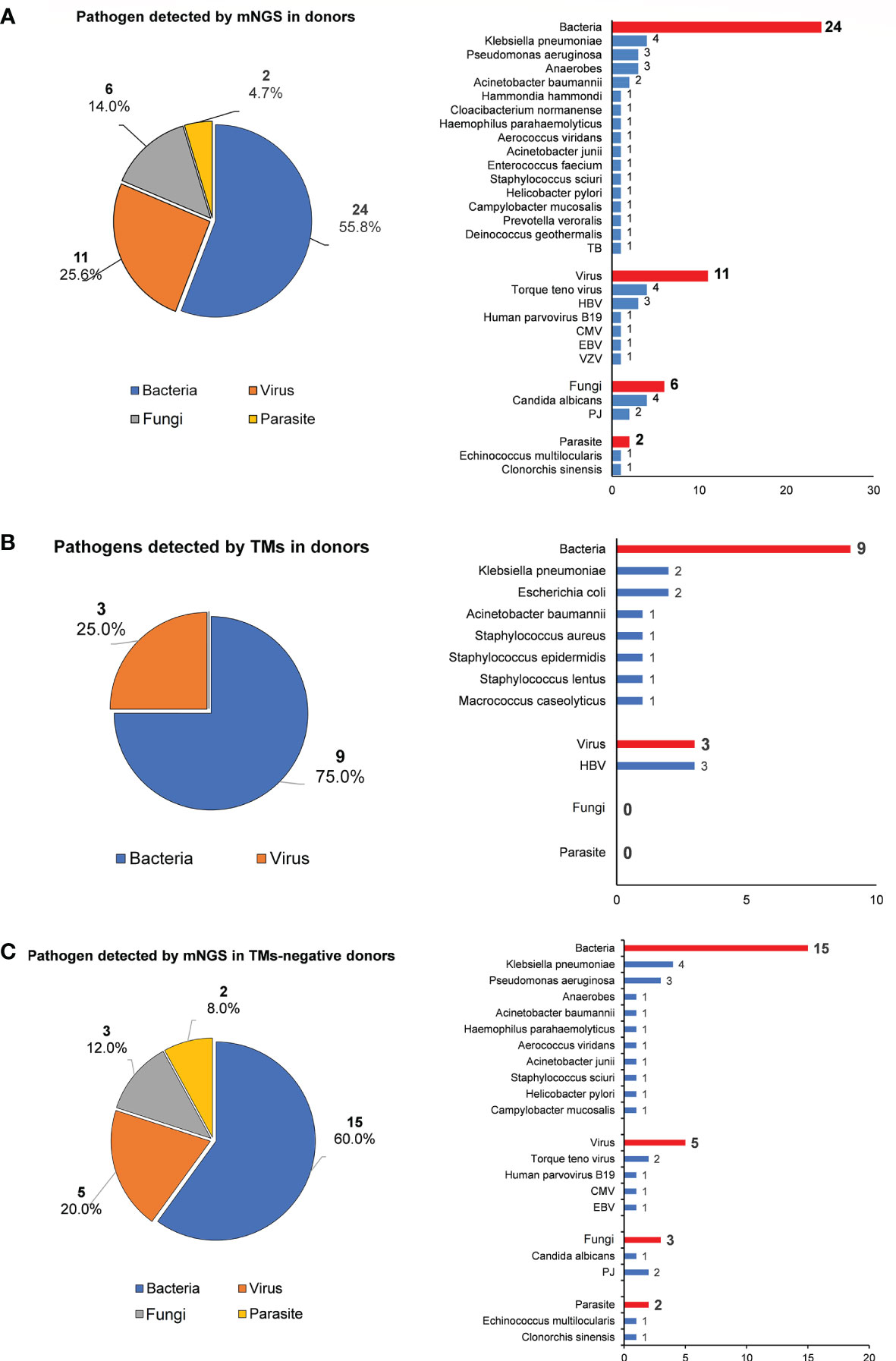
Figure 2 The pathogen spectrum in liver donor detected by mNGS and TMs. (A) Pie chart demonstrating the distribution of different types of pathogens detected by NGS in liver donors, and a total of 43 species of pathogens were detected in donor samples with their corresponding frequencies plotted in histograms. (B) Pie chare demonstrating the distribution of different types of pathogens detected by TMs in donors, and a total of 12 species of pathogens were detected in donor samples with their corresponding frequencies plotted in histograms. (C) Pie chart shows the distribution of different types of pathogens detected in TMs-negative donor samples by mNGS, and species of pathogens were detected with their corresponding frequencies plotted in histograms.
Among 12 donors who tested positive for pathogens using TMs, the results of the mNGS analysis were consistent in 11 out of 12 (91.7%) donor samples at the species level. However, at least one unique pathogen read was detected by mNGS when co-infection was observed. For the detected pathogen spectrum, common bacteria such as Klebsiella pneumoniae (KP), Acinetobacter baumannii (AB), Escherichia coli, and Staphylococcus could be detected by both TMs and mNGS, whereas fungi and unexpected viruses were only able to be identified by mNGS. Inconsistent results were only found for Donor 1 (D1) between mNGS and TMs: Staphylococcus lentus was identified by culture in both preservation fluid and perihepatic tissues, but was not detected by mNGS in any D1-related samples (Table 2).
The Performance of TMs and mNGS for the Detection of Donor-Recipient Transmitted Pathogens
The performance of TMs and mNGS for the detection of donor-transmitted pathogens was further evaluated. The transmission of pathogens was detected in 6 cases (6/42) from donor to recipient by mNGS, among which 4 cases were confirmed as DDIs with a 100% sensitivity and a 94.7% specificity. By TMs, the transmission was only detected in 2 cases (2/42) confirmed as DDI with sensitivity of 50.0% and a specificity of 100%. The PPV and NPV of mNGS were 66.7% and 100% for DDI diagnoses, respectively, compared with 100.0% and 95.0% for TMs, respectively. The ACC of both mNGS and TMs was 95.2% (Table 3). The new emerging pathogens transmitted via liver graft were identified by mNGS in six cases (Table 4). We found that KP (3/6, 50.0%), AB (2/6, 33.3%), Varicella zoster virus (VZV) (1/6, 16.7%), Candida glabrata (CG) (1/6, 16.7%), and Clonorchis sinensis (CS) (1/6, 16.7%) were detected by mNGS in both donor and corresponding recipient. There were 2 cases (2/6, 33.3%) with co-infection detected by mNGS in samples from D24 and D41, which could not be identified by TMs, especially the fungi and viruses.
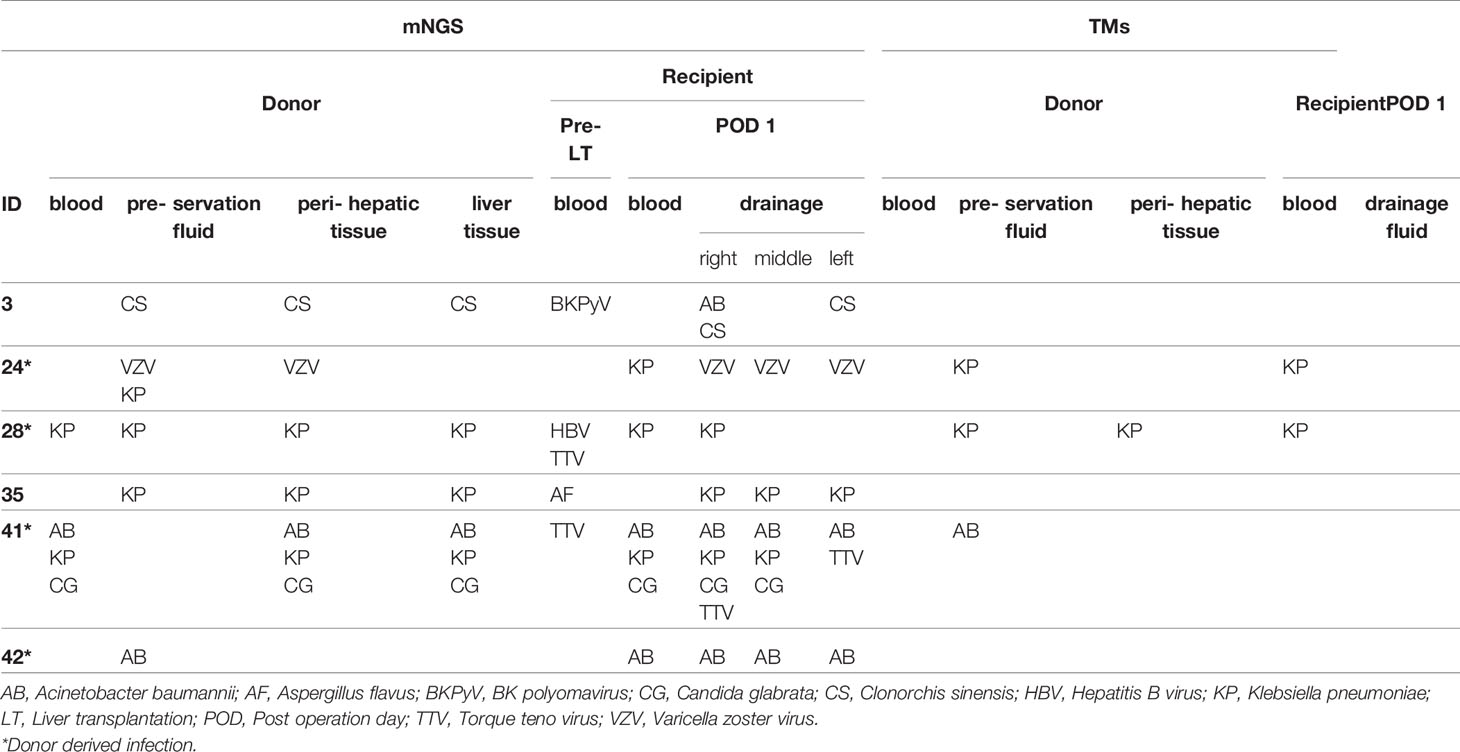
Table 4 Transmission of pathogens detected by mNGS and TMs from liver donor to corresponding recipient.
Guided Treatment for Perioperative Infection by mNGS in Patients When TMs Results Appear Invalid
The postoperative treatment regimen of antibiotics and immunosuppressants are first routinely adjusted according to the pathogens detected in donor and corresponding recipient by TMs. Under this situation, a total of 8/42 (19.0%) recipients developed early infection symptoms (<7 days) (Table 5). The mean time was 2.0 days to symptom onset (range, 1–6 days) after LT, and clinical manifestations included fever, purulent drainage fluid, increased procalcitonin (PCT) or C-reactive protein (CRP) levels, and positive imaging results. Sequentially, we modified the therapeutic regimen according to the results of mNGS in all eight infected recipients, and finally seven recipients recovered and one (R24) died of severe sepsis.
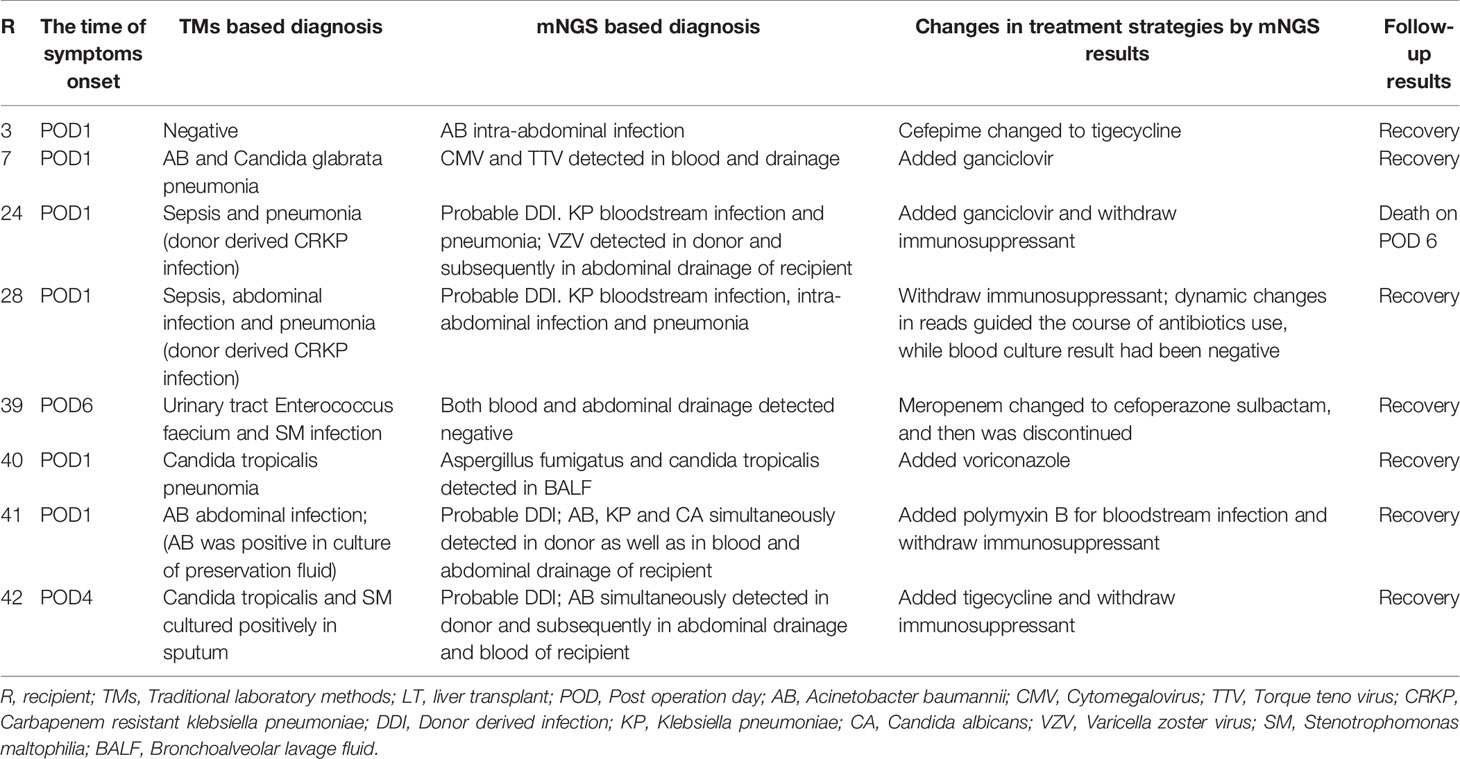
Table 5 The precise treatment of perioperative infection guided by mNGS infailure cases of TMs in LT.
Types of antibiotics were adjusted and/or immunosuppressants were withdrawed for R3, R7, R24, R28, R40, R41, and R42 (7/8, 87.5%), according to the additional pathogens identified by mNGS; the mNGS-negative results in R39 led to discontinue unnecessary broad-spectrum antibiotics (such as meropenem, which was replaced by cefoperazone sulbactam and then discontinued) (Table 5). The dynamic changes in SDSMRN by continuous mNGS surveillance in R28 guided the complete course of antibiotics for 30 days, as result of blood culture had been negative from POD 11 (Table 6). One recipient (R24) died after treatment adjustment and had received LT for drug-related acute liver failure (Model for End-stage Liver Disease score 41). In this case, VZV was only detected by mNGS in the donor liver and subsequently found in the abdominal drainage fluid of the recipient, suggesting a latent VZV infection in the donor. Despite the prompt use of antiviral therapy guided by mNGS, the recipient still succumbed to severe sepsis, which resulted in fatal liver failure on POD 6.
Discussion
Infection-related complications have become the leading cause of morbidity and mortality for patients in the first months after LT (Nam et al., 2018). The early and precise detection of infectious pathogens can optimize the use of targeted antibiotics and immunosuppressants to improve clinical outcomes for patients after LT. It is great challenge to test all potential pathogens existing in liver donors and their corresponding recipients using TMs. mNGS can overcome the limitations of the current diagnostic testing (Zhou et al., 2019). In this study, we prospectively evaluated the clinical value of mNGS for the identification of pathogens in different types of samples during LT. We found that mNGS could provide rapid and precise detection of pathogens and was an ideal tool for the diagnosis of DDIs. Furthermore, mNGS was able to guide the precise treatment of perioperative infections in patients undergoing LT.
Our data revealed that positive results using mNGS analyses were consistent with those from TMs 91.7% of the time. These results suggest that mNGS can effectively detect the same pathogens as TMs and identify more latent pathogens carried by donors. As mNGS analyses often detected more than one pathogen in a single test, clinicians need to have a comprehensive understanding of results indicating the presence of co-infection (Tarabichi et al., 2018). Thus, we used our own criteria (Miao et al., 2018) to uncover co-infections and/or distinguish the causative pathogens. We found that CA (4/43, 9.3%) was the most common fungi within the co-infection detected by mNGS. Donor-derived fungal infections have been associated with life-threatening complications in transplant recipients (Mishkin, 2021), so mNGS analyses would allow the precise and timely detection of fungi to enable prompt treatment. It is worth noting that the average turnaround time for culture results is more than 72 hours for commonly encountered bacteria and up to weeks for more insidious pathogens such as Aspergillus fumigates (Simner et al., 2018). Thus, the turnaround time of mNGS (average of 48 hours) (Pendleton et al., 2017; Afshinnekoo et al., 2017) will hasten clinical decision-making, which is critical for immunocompromised recipients after LT (Simner et al., 2018).
Metagenomic sequencing combined with phylogenetic analysis could effectively identify the frequent transmission of JC polyomavirus from kidney transplant donor to recipient (Schreiber et al., 2019). Our study demonstrated the transmission of identical pathogens from donors to corresponding recipients in 6 of 42 cases (14.3%) by mNGS, which can promptly assist in the diagnosis of DDIs with clinical infection symptoms. As compared with TMs, mNGS was more sensitive (100% vs. 50%) with a similar specificity (94.7% vs. 100.0%) in terms of diagnoses of DDIs, respectively. As immunocompromised recipients are generally critical ill, the timely identification of the pathogens causing DDI is crucial for a precise diagnosis, which is necessary for proper treatment (Nam et al., 2018). More importantly, the mNGS-negative results of donor and corresponding recipient can assist to exclude DDI in clinical work.
With antimicrobial treatment guided by the TMs results, 8 of 42 (19.0%) recipients developed an early infection (<7 days) after LT. Targeted antibiotics were adjusted and/or immunosuppressants were discontinued according to the additional pathogens identified by mNGS. Finally, 7 recipients recovered. These examples demonstrate that mNGS can effectively guide the treatment of perioperative infection after LT, especially when routine TMs results appear inefficacy. The decrease or disappear of pathogen unique reads monitored by mNGS are correlated with improvement of clinical infection symptoms and indirectly guide the course of antibiotics (Zhang et al., 2019), especially in DDI cases. Blood culture of one recipient (R28) with DDI had been negative since POD 11, the course of antibiotics was guided by dynamic changes of unique pathogen reads detected by mNGS with clinical index of PCT for 30 days. Therefore, mNGS results might be a reliable indicator to help understand how the pathogens progress and guide the adjustment of antibiotics in DDI cases (Ai et al., 2018).
TTV load is modulated by the immune, viral, and inflammatory status, and often considered as potential marker associated with immunity status as well as infectious diseases in LT (Mrzljak and Vilibic-Cavlek, 2020). As reported, TTV viremia was significantly higher during CMV infections (Ruiz et al., 2019). In our cohort, CMV and TTV were detected in blood and drainage in one recipient (R7) with early infection (<7 days) (Table 5), but we did not find TTV in the other infection recipients. The reason might be TTV loads progressively increased and peaks around 3 months post-transplant, positively correlating with the intensity of immunosuppression (Schreiber et al., 2019; Mrzljak and Vilibic-Cavlek, 2020), and then virus specific PCR monitoring will have higher sensitivity for detection of TTV loads after LT.
There was a discordant result between TMs and mNGS in one donor (D1). S. lentus was positively identified by TMs in preservation fluid and perihepatic tissue, but was not detected by mNGS. A possible reason may be that pathogen reads make up a minute fraction of the sequencing results and are of low sequencing depth, which means mNGS results could be further improved by increasing sequencing depth. One recipient (R24) died of fulminant liver failure and severe sepsis even with treatment guided by mNGS results. It is worth noting that this outcome might be associated with the poor condition of the recipient prior to LT and acute liver graft dysfunction caused by the recurrence of VZV infection after LT. Disseminated visceral VZV infection has been described as a rare but severe disease with a high mortality rate, especially in immunocompromised hosts (Kikuchi et al., 2019). More early diagnosis and timely intervention for those patients, such as the discontinuation of immunosuppressants, might be crucial to improve their clinical outcome (Mehta et al., 2021).
There were some limitations of our study. First, the results of the mNGS were not reconfirmed by PCR-based assays, and a phylogenetic analysis of the pathogens from both the donor and corresponding recipient will be very helpful to confirm diagnosis of DDI. Second, there lacks a unified standardized protocol for mNGS currently in clinical diagnosis. Due to potential breadth of detection and nucleic acid contamination in the process, interpretation of mNGS results directly from clinical specimens can be difficult and requires careful consideration. Additionally, unbiased mNGS was not routinely performed alongside RNA sequencing.
untargeted mNGS was not routinely performed alongside RNA sequencing.
Our study showed that, as compared with TMs, mNGS could yield higher sensitivity for the early identification of fastidious pathogens in patients undergoing LT, especially for DDI diagnoses. Importantly, mNGS does not replace current TMs. Alternatively, it may be considered for immunocompromised patients where achieving a timely diagnosis and treatment is imperative for improved outcomes. The large-scale multicenter randomized controlled studies are needed to further confirm the value of mNGS in routine clinical care of patients undergoing LT.
Data Availability Statement
The data presented in the study are deposited in the CNGB Sequence Archive (CNSA) of China National GeneBank DataBase (CNGBdb), accession number CNP0003068. (http://db.cngb.org/cnsa/project/CNP0003068_275abe06/reviewlink/).
Ethics Statement
The studies involving human participants were reviewed and approved by the Ethical Review Committee of Zhongshan Hospital affiliated with Fudan University. The patients/participants provided their written informed consent to participate in this study.
Author Contributions
Research design was conceived by X-RY, JZ and J-FH. J-FH, QM and J-WC performed the research and analyzed the results. First draft manuscript was prepared by J-FH and QM. AH, D-ZG, TW, L-XY, D-MZ, YC, X-WH and JF contributed to manuscript revisions. All authors contributed to the article and approved the submitted version.
Funding
This study was jointly supported by the National Key R&D Program of China (2019YFC1315800, 2019YFC1315802), the State Key Program of National Natural Science of China (81830102), the National Natural Science Foundation of China (82150004, 81772578, 81772551, 81872355 and 82072715), the Shanghai Municipal Health Commission Collaborative Innovation Cluster Project (2019CXJQ02), Shanghai “Rising Stars of Medical Talent” Youth Development Program (Outstanding Youth Medical Talents), the Projects from the Shanghai Science and Technology Commission (19441905000 and 21140900300), Shanghai Municipal Key Clinical Specialty.
Conflict of Interest
The authors declare that the research was conducted in the absence of any commercial or financial relationships that could be construed as a potential conflict of interest.
The reviewer CZ declared a shared parent affiliation with the authors J-FH, QM, J-WC, AH, D-ZG, TW, L-XY, D-MZ, X-WH, JF, JZ, X-RY to the handling editor at the time of review.
Publisher’s Note
All claims expressed in this article are solely those of the authors and do not necessarily represent those of their affiliated organizations, or those of the publisher, the editors and the reviewers. Any product that may be evaluated in this article, or claim that may be made by its manufacturer, is not guaranteed or endorsed by the publisher.
Acknowledgments
The authors thank all the patients included in our study.
Abbreviations
AB, acinetobacter baumannii; ACC, accuracy; CA, candida albicans; CMV, Cytomegalovirus; CRP, C-reactive protein; CS, clonorchis sinensis; D, donor; DDI, donor-derived infection; DNBs, DNA nanoballs; EBV, Epstein-Barr virus; HBV, Hepatitis B virus; HCV, Hepatitis C virus; HIV, Human immunodeficiency virus; KP, klebsiella pneumoniae; LT, liver transplantation; mNGS, metagenomic next-generation sequencing; NPV, negative predictive value; PCT, procalcitonin; POD, postoperative days; PPV, positive predictive value; R, recipient; RPR, Rapid plasma reagin; SDSMRN, the number of unique reads of standardized species; TMs, traditional laboratory methods; TTV, Torque Teno Virus; VZV, varicella zoster virus.
References
Afshinnekoo, E., Chou, C., Alexander, N., Ahsanuddin, S., Schuetz, A. N., Mason, C. E. (2017). Precision Metagenomics: Rapid Metagenomic Analyses for Infectious Disease Diagnostics and Public Health Surveillance. J. Biomol. Tech. 28, 40–45. doi: 10.7171/jbt.17-2801-007
Ai, J. W., Zhang, H. C., Cui, P., Xu, B., Gao, Y., Cheng, Q., et al. (2018). Dynamic and Direct Pathogen Load Surveillance to Monitor Disease Progression and Therapeutic Efficacy in Central Nervous System Infection Using a Novel Semi-Quantitive Sequencing Platform. J. Infect. 76, 307–310. doi: 10.1016/j.jinf.2017.11.002
Bandali, A., Bias, T. E., Lee, D. H., Malat, G. (2020). Duration of Perioperative Antimicrobial Prophylaxis in Orthotopic Liver Transplantation Patients. Prog. Transplant. 30, 265–270. doi: 10.1177/1526924820933824
Cornaglia, G., Courcol, R., Hermann, J. L. (2012). European Manual of Clinical Microbiology (Société franaise de microbiologie).
da Silva, F. R., Cibulski, S. P., Daudt, C., Weber, M. N., Guimaraes, L. L., Streck, A. F., et al. (2016). Novel Bovine Papillomavirus Type Discovered by Rolling-Circle Amplification Coupled With Next-Generation Sequencing. PLoS One 11, e0162345. doi: 10.1371/journal.pone.0162345
Dogan, S. M., Kutluturk, K. (2020). Living Donor Versus Deceased Donor Liver Transplantation for HCC. J. Gastrointest Cancer 51, 1104–1106. doi: 10.1007/s12029-020-00481-1
Fang, C., Zhong, H., Lin, Y., Chen, B., Han, M., Ren, H., et al. (2018). Assessment of the cPAS-Based BGISEQ-500 Platform for Metagenomic Sequencing. Gigascience 7, 1–8. doi: 10.1093/gigascience/gix133
Heldman, M. R., Ngo, S., Dorschner, P. B., Helfrich, M., Ison, M. G. (2019). Pre- and Post-Transplant Bacterial Infections in Liver Transplant Recipients. Transpl Infect. Dis. 21, e13152. doi: 10.1111/tid.13152
Huang, J., Jiang, E., Yang, D., Wei, J., Zhao, M., Feng, J., et al. (2020). Metagenomic Next-Generation Sequencing Versus Traditional Pathogen Detection in the Diagnosis of Peripheral Pulmonary Infectious Lesions. Infect. Drug Resist. 13, 567–576. doi: 10.2147/IDR.S235182
Huang, J., Millis, J. M., Mao, Y., Millis, M. A., Sang, X., Zhong, S. (2015). Voluntary Organ Donation System Adapted to Chinese Cultural Values and Social Reality. Liver Transpl. 21, 419–422. doi: 10.1002/lt.24069
Jeon, Y. J., Zhou, Y., Li, Y., Guo, Q., Chen, J., Quan, S., et al. (2014). The Feasibility Study of non-Invasive Fetal Trisomy 18 and 21 Detection With Semiconductor Sequencing Platform. PLoS One 9, e110240. doi: 10.1371/journal.pone.0110240
Kaul, D. R., Vece, G., Blumberg, E., La Hoz, R. M., Ison, M. G., Green, M., et al. (2021). Ten Years of Donor-Derived Disease: A Report of the Disease Transmission Advisory Committee. Am. J. Transplant. 21, 689–702. doi: 10.1111/ajt.16178
Kikuchi, T., Arai, M., Koda, Y., Kato, J., Shimizu, T., Katano, H., et al. (2019). Late-Onset Visceral Varicella-Zoster Virus Infection Presented as Acute Liver Failure After Allogeneic Hematopoietic Stem Cell Transplantation. Transpl Infect. Dis. 21, e13121. doi: 10.1111/tid.13121
Li, H., Durbin, R. (2010). Fast and Accurate Long-Read Alignment With Burrows-Wheeler Transform. Bioinformatics 26, 589–595. doi: 10.1093/bioinformatics/btp698
Li, Y., Sun, B., Tang, X., Liu, Y. L., He, H. Y., Li, X. Y., et al. (2020). Application of Metagenomic Next-Generation Sequencing for Bronchoalveolar Lavage Diagnostics in Critically Ill Patients. Eur. J. Clin. Microbiol. Infect. Dis. 39, 369–374. doi: 10.1007/s10096-019-03734-5
Long, Y., Zhang, Y., Gong, Y., Sun, R., Su, L., Lin, X., et al. (2016). Diagnosis of Sepsis With Cell-Free DNA by Next-Generation Sequencing Technology in ICU Patients. Arch. Med. Res. 47, 365–371. doi: 10.1016/j.arcmed.2016.08.004
Mehta, V., Ramachandran, K., Agarwal, R., Alam, S., Pamecha, V., Gupta, E. (2021). Varicella Infection in an Immunized Pediatric Living Donor Liver-Transplant Recipient. J. Glob Infect. Dis. 13, 142–144. doi: 10.4103/jgid.jgid_233_20
Miao, Q., Ma, Y., Wang, Q., Pan, J., Zhang, Y., Jin, W., et al. (2018). Microbiological Diagnostic Performance of Metagenomic Next-Generation Sequencing When Applied to Clinical Practice. Clin. Infect. Dis. 67, S231–S240. doi: 10.1093/cid/ciy693
Mishkin, A. (2021). Emerging Fungal Pathogens in Solid Organ Transplantation. Curr. Opin. Organ Transplant. 26, 440–444. doi: 10.1097/MOT.0000000000000889
Mrzljak, A., Vilibic-Cavlek, T. (2020). Torque Teno Virus in Liver Diseases and After Liver Transplantation. World J. Transplant. 10, 291–296. doi: 10.5500/wjt.v10.i11.291
Nam, H., Nilles, K. M., Levitsky, J., Ison, M. G. (2018). Donor-Derived Viral Infections in Liver Transplantation. Transplantation 102, 1824–1836. doi: 10.1097/TP.0000000000002326
Pendleton, K. M., Erb-Downward, J. R., Bao, Y., Branton, W. R., Falkowski, N. R., Newton, D. W., et al. (2017). Rapid Pathogen Identification in Bacterial Pneumonia Using Real-Time Metagenomics. Am. J. Respir. Crit. Care Med. 196, 1610–1612. doi: 10.1164/rccm.201703-0537LE
Pettengill, M. A., Babu, T. M., Prasad, P., Chuang, S., Drage, M. G., Menegus, M., et al. (2019). Probable Donor-Derived Human Adenovirus Type 34 Infection in 2 Kidney Transplant Recipients From the Same Donor. Open Forum Infect. Dis. 6, ofy354. doi: 10.1093/ofid/ofy354
Ruiz, P., Martinez-Picola, M., Santana, M., Munoz, J., Perez-Del-Pulgar, S., Koutsoudakis, G., et al. (2019). Torque Teno Virus Is Associated With the State of Immune Suppression Early After Liver Transplantation. Liver Transpl 25, 302–310. doi: 10.1002/lt.25374
Schreiber, P. W., Kufner, V., Hubel, K., Schmutz, S., Zagordi, O., Kaur, A., et al. (2019). Metagenomic Virome Sequencing in Living Donor and Recipient Kidney Transplant Pairs Revealed JC Polyomavirus Transmission. Clin. Infect. Dis. 69, 987–994. doi: 10.1093/cid/ciy1018
Simner, P. J., Miller, S., Carroll, K. C. (2018). Understanding the Promises and Hurdles of Metagenomic Next-Generation Sequencing as a Diagnostic Tool for Infectious Diseases. Clin. Infect. Dis. 66, 778–788. doi: 10.1093/cid/cix881
Tarabichi, M., Shohat, N., Goswami, K., Alvand, A., Silibovsky, R., Belden, K., et al. (2018). Diagnosis of Periprosthetic Joint Infection: The Potential of Next-Generation Sequencing. J. Bone Joint Surg. Am. 100, 147–154. doi: 10.2106/JBJS.17.00434
Zhang, X. X., Guo, L. Y., Liu, L. L., Shen, A., Feng, W. Y., Huang, W. H., et al. (2019). The Diagnostic Value of Metagenomic Next-Generation Sequencing for Identifying Streptococcus Pneumoniae in Paediatric Bacterial Meningitis. BMC Infect. Dis. 19, 495. doi: 10.1186/s12879-019-4132-y
Zhou, X., Wu, H., Ruan, Q., Jiang, N., Chen, X., Shen, Y., et al. (2019). Clinical Evaluation of Diagnosis Efficacy of Active Mycobacterium Tuberculosis Complex Infection via Metagenomic Next-Generation Sequencing of Direct Clinical Samples. Front. Cell Infect. Microbiol. 9, 351. doi: 10.3389/fcimb.2019.00351
Keywords: liver transplantation, metagenomic next-generation sequencing, donor-derived infection, perioperative infection, immunocompromised patient
Citation: Huang J-F, Miao Q, Cheng J-W, Huang A, Guo D-Z, Wang T, Yang L-X, Zhu D-M, Cao Y, Huang X-W, Fan J, Zhou J and Yang X-R (2022) Metagenomic Next-Generation Sequencing Versus Traditional Laboratory Methods for the Diagnosis and Treatment of Infection in Liver Transplantation. Front. Cell. Infect. Microbiol. 12:886359. doi: 10.3389/fcimb.2022.886359
Received: 28 February 2022; Accepted: 17 May 2022;
Published: 16 June 2022.
Edited by:
Jinmin Ma, Beijing Genomics Institute (BGI), ChinaReviewed by:
Chiyu Zhang, Fudan University, ChinaJianwei Chen, Beijing Genomics Institute (BGI), China
Akira Shishido, University of Maryland Medical Center, United States
Copyright © 2022 Huang, Miao, Cheng, Huang, Guo, Wang, Yang, Zhu, Cao, Huang, Fan, Zhou and Yang. This is an open-access article distributed under the terms of the Creative Commons Attribution License (CC BY). The use, distribution or reproduction in other forums is permitted, provided the original author(s) and the copyright owner(s) are credited and that the original publication in this journal is cited, in accordance with accepted academic practice. No use, distribution or reproduction is permitted which does not comply with these terms.
*Correspondence: Xin-Rong Yang, yang.xinrong@zs-hospital.sh.cn; Jian Zhou, zhou.jian@zs-hospital.sh.cn
†These authors have contributed equally to this work