E-NTPDases: Possible Roles on Host-Parasite Interactions and Therapeutic Opportunities
- 1Laboratório de Bioquímica Celular, Instituto de Bioquímica Médica Leopoldo de Meis, Centro de Ciências da Saúde, Universidade Federal do Rio de Janeiro, Rio de Janeiro, Brazil
- 2Departamento de Bioquímica, Instituto de Química, Universidade Federal Rural do Rio de Janeiro, Seropédica, Brazil
- 3Instituto Nacional de Ciência e Tecnologia em Biologia Estrutural e Bioimagem, Rio de Janeiro, Brazil
Belonging to the GDA1/CD39 protein superfamily, nucleoside triphosphate diphosphohydrolases (NTPDases) catalyze the hydrolysis of ATP and ADP to the monophosphate form (AMP) and inorganic phosphate (Pi). Several NTPDase isoforms have been described in different cells, from pathogenic organisms to animals and plants. Biochemical characterization of nucleotidases/NTPDases has revealed the existence of isoforms with different specificities regarding divalent cations (such as calcium and magnesium) and substrates. In mammals, NTPDases have been implicated in the regulation of thrombosis and inflammation. In parasites, such as Trichomonas vaginalis, Trypanosoma spp., Leishmania spp., Schistosoma spp. and Toxoplasma gondii, NTPDases were found on the surface of the cell, and important processes like growth, infectivity, and virulence seem to depend on their activity. For instance, experimental evidence has indicated that parasite NTPDases can regulate the levels of ATP and Adenosine (Ado) of the host cell, leading to the modulation of the host immune response. In this work, we provide a comprehensive review showing the involvement of the nucleotidases/NTPDases in parasites infectivity and virulence, and how inhibition of NTPDases contributes to parasite clearance and the development of new antiparasitic drugs.
Introduction
There are four important subfamilies of ecto-nucleotidases with different substrate specificities (nucleotide hydrolysis): (i) the ecto-nucleoside triphosphate diphosphohydrolases (E-NTPDases), (ii) the ecto-5’nucleotidases (5’-NT), (iii) the ecto-nucleotidase pyrophosphatase/phosphodiesterases (NPPs), and (iv) alkaline phosphatases (Aps) (Barros et al., 2000; Meyer-Fernandes et al., 2000; de Souza Leite et al., 2007; Paletta-Silva and Meyer-Fernandes, 2012; Zimmermann et al., 2012).
The ecto-nucleoside triphosphate diphosphohydrolases are ecto-nucleotidases that hydrolyze different tri-and diphosphate nucleosides to their monophosphate counterpart (Zimmermann et al., 2012; Zimmermann, 2021a). The E-NTPDase activity is dependent on the presence of divalent cations, such as calcium and magnesium (Handa and Guidotti, 1996; Robson et al., 2006).
Eight members of the E-NTPDase family were identified in mammals. The isoforms 1 (known as CD39), 2, 3, and 8 are typical E-NTPDases, located on the cell surface. The isoforms 4 and 7 were found in the Golgi apparatus and intracellular membrane compartment, respectively. After heterologous expression, the isoforms 5 and 6 were found only in the supernatant, suggesting that they could be secreted forms (Robson et al., 2006; Zimmermann et al., 2012).
All these enzymes conserve five “apyrase conserved regions”, known as ACR1 to ACR5 (Robson et al., 2006; Zimmermann et al., 2012). They are usually located on the cell membrane with the catalytic site oriented to the extracellular milieu. Some of them are also bound to the organelle membrane, with the catalytic site oriented to the lumen of the organelle (Bernardes et al., 2000; Meyer-Fernandes, 2002; Sansom et al., 2008). The E-NTPDases are proteins characterized by having a high degree of glycosylation. Not only are there different numbers of N-glycosylation sites but also the placement of the sites relative to the apyrase conserved regions is different as well. For example, four out of seven glycosylation sites in NTPDase 3 are near the ACR regions, whereas NTPDase 4 and 5 have no glycosylation sites approximate to ACR regions (Zhong et al., 2017).
In parasites, nucleotidases/E-NTPDases seem to be involved in crucial processes, such as virulence, infectivity, purine salvage pathways, and parasite adhesion on the host cell (Sansom, 2012; Figueiredo et al., 2016; Paes-Vieira et al., 2018; Silva-Gomes et al., 2020). During parasite infection, the host cell releases ATP as a danger signal, leading to the augmentation of the extracellular ATP level (Trautmann, 2009). For instance, during Leishmania spp. infection, the parasite interacts with Toll-like receptors (TLR) present in the surface of the host cell, inducing the release of ATP to the extracellular milieu via pannexin-1 channels. The extracellular ATP can be now metabolized by parasite E-NTPDase and 5’-NT, increasing the extracellular Adenosine (Ado) level (Chaves et al., 2021). Such sequential hydrolysis of extracellular ATP to adenosine was demonstrated in different parasite species, indicating that not only E-NTPDases but also ecto-5`-nucleotidases could be present in protozoa parasites, including Leishmania spp., Trypanosoma spp., Trichomonas vaginalis, Tritrichomonas foetus, Toxoplasma gondii, and Schistosoma spp. (Berrêdo-Pinho et al., 2001; Meyer-Fernandes, 2002; Sansom, 2012; Pimentel et al., 2016; Paes-Vieira et al., 2018).
Many studies have suggested the involvement of parasite E-NTPDases in host immune defense suppression since their activity would lead to the reduction of ATP and ADP levels. According to these studies, parasite E-NTPDases (CD39 gene family) hydrolyze extracellular ATP to ADP and AMP, which is, in sequence, hydrolyzed by the parasite ecto-5’-nucleotidases (CD73 gene family). The hydrolysis of AMP by parasite ecto-5’-nucleotidases increases the levels of adenosine, leading to the reduction of the host inflammatory response (Fredholm et al., 2007; Sansom et al., 2008; Arora and Rai, 2019).
Parasite E-NTPDases and 5’NTs have received special attention because they are cell-surfaced enzymes whose activity can modulate the host purinergic signaling, a signal transduction pathway mediated by purine nucleotides and nucleosides, such as ATP and adenosine, which bind and activate specific surface proteins called purinergic receptors (Figueiredo et al., 2016; Zimmermann 2021b). In host cells, two families of purinergic receptors, P1 and P2, mediate purinergic signaling. The P1 receptors are metabotropic G protein-coupled receptors, encompass four subtypes, called A1, A2A, A2B, and A3; all of them use adenosine as ligand. The P2 receptors are responsive to tri- and diphosphonucleotides (ATP, ADP, UTP and UDP) and are divided into P2X and P2Y subtypes. The P2X receptor is also called ionotropic and functions as non-selective cation ion channel. Meanwhile, P2Y receptor is coupled to G protein. The family of P2Y receptors can modulate many cellular processes related to inflammatory and immune responses, such as cell differentiation, adhesion, migration, phagocytosis, and secretion in the host cell (such as macrophages, monocytes, neutrophils, and dendritic cells) (Coutinho-Silva and Savio, 2021; Klaver and Thurnher, 2021). The most well-studied of the P2X receptors is the P2X7 subtype. It has been shown that the interaction between ATP and the P2X7 receptor leads to an inflammatory response by the host immune system. Such inflammatory response triggered by extracellular ATP activates macrophage and dendritic cells, leading to an increase of IL-12 and TNF-α, reactive oxygen species (ROS), and nitric oxide (NO) production, culminating in intracellular pathogen elimination (Savio and Coutinho-Silva, 2019; Chaves et al., 2021; Coutinho-Silva and Savio, 2021).
Acting on P1 receptors of the host cell, extracellular adenosine, generated by parasite E-NTPDases and 5’-NTs, increases the intracellular level of cAMP in the host cell, leading to the modulation of the inflammatory response (Figueiredo et al., 2017). In summary, adenosine leads to the suppression of inflammatory cytokines by macrophages and dendritic cells and the production of antimicrobial substances by macrophages and neutrophils. In addition, adenosine increases the level of IL-10, an important regulatory cytokine (Wilson et al., 2009; Figueiredo et al., 2016; Haskó et al., 2018; Antonioli et al., 2019). Thus, the dual effect of both ATP and adenosine on the host immune response seems to depend on different factors such as ATP and adenosine concentration, time of exposure, and conditions of the host cell. Thus, the balance between these two molecules (ATP and adenosine) is crucial for the proper immune response (Zhao et al., 2017). Figure 1 summarizes the role/effect of parasite ectonucleotidases on the nucleotide/purinergic signaling of the host cell.
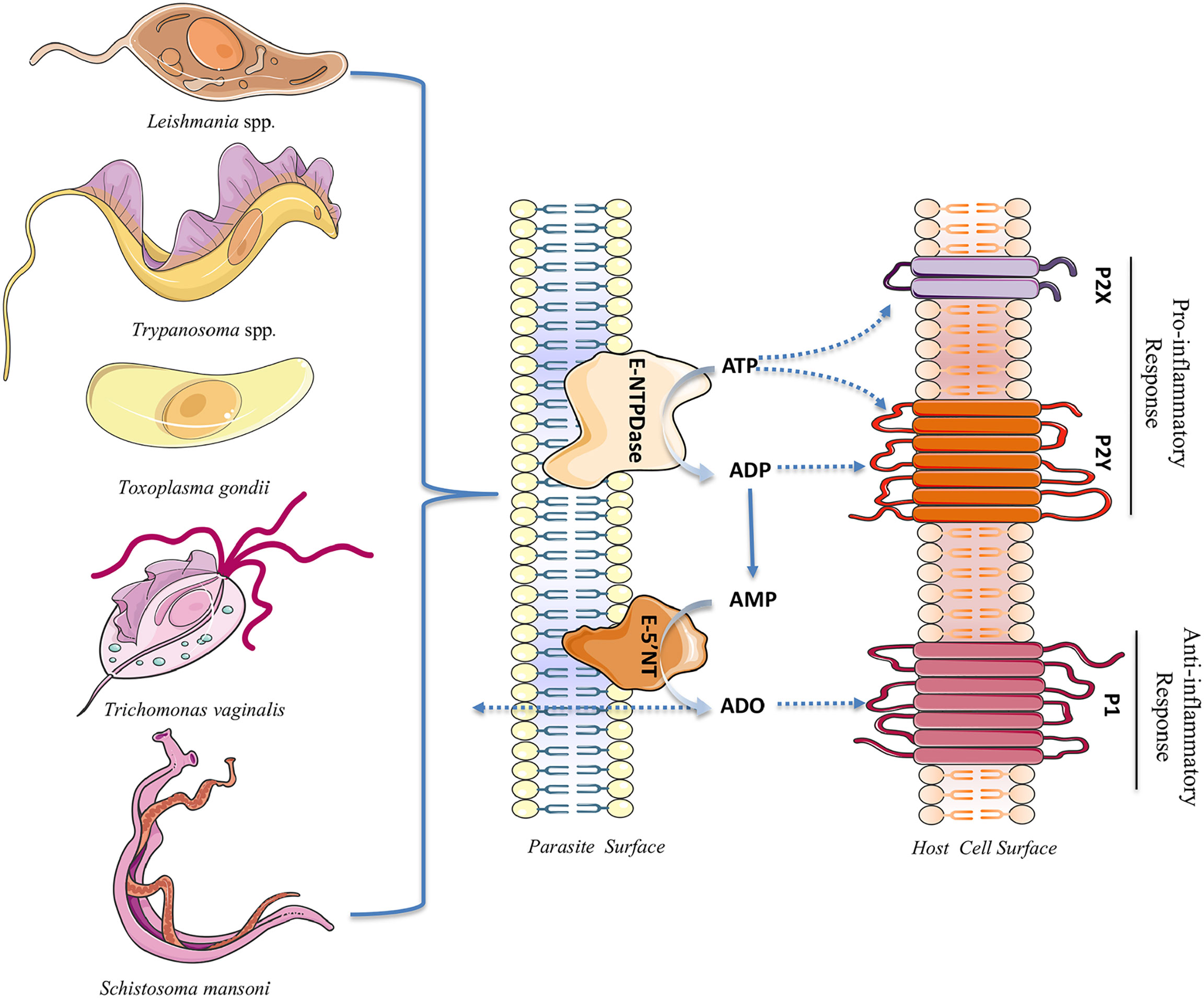
Figure 1 Extracellular nucleotidases in parasite cell surface and nucleotide/purinergic signaling in the host cell. During infection, the immune system interprets an increase in the levels of extracellular ATP as a risk situation and gives rise to an inflammatory response through the activation of P2X and P2Y receptors, culminating in parasitism control. In the parasite surface the enzyme NTPDase hydrolyzes extracellular ATP to ADP and ADP to AMP. The AMP generated can be subsequently hydrolyzed to adenosine (ADO) by Ecto-5’-nucleotidases (E-5’NT). Adenosine reduces the host inflammatory response via P1 receptors to favor the persistence of parasite in the infected cells. Changes in extracellular nucleotide and adenosine levels can modulate P2 and P1 receptors activity (the images used in the illustration are provided by https://smart.servier.com).
In this review article, we discuss the role of nucleotidases/E-NTPDases in parasite infectivity and virulence and show the potential of these enzymes as antiparasitic drug targets. In this sense, we also provide an overview of the latest studies concerning the development of nucleotidase/E-NTPDase inhibitors and their effect on parasite physiology.
Development of E-NTPDases Inhibitors
Ecto-nucleotidases have been implicated in different pathological conditions. The interplay of ecto-nucleotidases with the nucleotide and adenosine receptor systems has received special attention. P1 and P2 receptor activity can be controlled by changes in the extracellular levels of both adenosine and nucleotide (Kukulski et al., 2011). Thus, the search for strong and subtype-specific ecto-nucleotidase inhibitors is important not only for developing potential therapeutics but also for developing proper molecular tools for studying the role of ecto-nucleotidases in parasite-host interaction. However, the development of such inhibitors is an obstacle to overcome since they must be E-NTPDase-specific, not affecting other types of ecto-nucleotidases or nucleotide receptors (Zimmermann, 2021a; Zimmermann, 2021b).
While several non-selective chemical compounds have been used to inhibit E-NTPDases activity, strong and specific inhibitors are rare, most of them are nucleotide analogs (Zimmermann 2021a; Zimmermann, 2021b). The most well-known CD39 inhibitors include an impermeant inhibitor, DIDS (4,4′-diisothiocyanostilbene-2,2′-disulfonic acid) (Meyer-Fernandes et al., 1997; Bernardes et al., 2000), sodium azide (NaN3), suramin (Bisaggio et al., 2003; Fonseca et al., 2006), chelators (EDTA and EGTA) (Iqbal and Shah, 2018), ARL67156 (6-N, N-diethyl-D-b,g-dibromomethylene ATP) (Lévesque et al., 2007), 8-BuS-ATP derivatives (8-thiobutyladenosine 50-triphosphate) (Gendron et al., 2000; Lecka et al., 2013) and BG0136 (1-naphthol-3, 6-disulfonic acid) (Gendron et al., 2002). Such inhibitors can increase ATP and ADP levels, causing the augmentation of purinergic signaling. However, inhibitors like suramin bind to P2 receptors and antagonize their effects (Munkonda et al., 2007). Since these chemicals are not CD39-specific, their use has been shown to be limited (Battastini et al., 2021). Other classes have been reported as inhibitors of E-NTPDases, such as polyoxometalates (POMs) (Lee et al., 2015; Schachter et al., 2015; Jeffrey et al., 2020), thiadiazolopyrimidones (Afzal et al., 2020), Schiff bases of tryptamine (Kanwal et al., 2019), quinoline (Hayat et al., 2019; Murtaza et al., 2021) and anthraquinone derivatives (Al-Rashida and Iqbal, 2014; Baqi et al., 2020), sulfopolysaccharides (Lopez et al., 2021) and carboxamide derivatives (Afzal et al., 2021). Moreover, specific antibodies have also been used as inhibitors of NTPDases (Munkonda et al., 2009; Pelletier et al., 2017).
Since E-NTPDases have been implicated in different processes of parasite physiology, such as differentiation, nutrition, invasion, survival in the host cell, and modulation of the host immune response to establish infection, these enzymes have been seen as promising targets for drug development (Paes-Vieira et al., 2018). In this sense, many works have shown the effects of several compounds on parasite E-NTPDases (Knowles, 2011; Pimentel et al., 2016; de Carvalho et al., 2019; da Silva et al., 2021).
Leishmania spp.
The genus Leishmania encompasses at least 20 different species of protozoa parasites, which can cause a tropical disease called leishmaniasis. An estimated 0.7-1 million new cases of leishmaniasis per year are reported from nearly 100 endemic countries (Burza et al., 2018). Leishmaniasis is characterized by three different clinical manifestations, which are determined by the Leishmania species. Self-healing lesions in the infectious site characterize the cutaneous leishmaniasis (CL). In the mucocutaneous leishmaniasis (ML), besides cutaneous lesions, mucosal damage may occur, leading to disseminated or diffuse CL. In visceral leishmaniasis (VL), parasites migrate to the liver, spleen, and bone marrow, causing a more severe condition that can be fatal (Pace, 2014).
In Leishmania species (L. amazonensis, L. mexicana, L. tropica, L. braziliensis, L. donovani, L. infantum, L. major), two genes encoding putative NTPDases (LNTPDase 1 and LNTPDase 2) have been found in genome databases (Sansom, 2012; Paes-Vieira et al., 2018). In different Leishmania species, LNTPDase and ecto-5’-nucleotidase activities have been implicated with virulence and infectivity (Berrêdo-Pinho et al., 2001; Pinheiro et al., 2006; de Almeida Marques-da-Silva et al., 2008; Souza et al., 2011; Leite et al., 2012; Maia et al., 2013).
In L. amazonensis, LaNTPDase activity is differentially regulated among promastigote, amastigotes, and metacyclic promastigote forms. The authors showed that LaNTPDase activity is lower in promastigotes, and significantly higher in metacyclic promastigote forms (Paes-Vieira et al., 2021). Moreover, overexpression of ntpd1 and ntpd2 led to a reduced lesion in mice transfected with such overexpressing-L amazonensis, compared to control. It was suggested that L. amazonensis overexpressing ntpd1 and ntpd2 leads to the augmentation of extracellular adenosine levels, modulating the host immune response and promoting pathogen clearance (Paes-Vieira et al., 2021). A mutant of L. major lacking the Golgi NTPDase 1 exhibited a delayed capacity to induce lesions in susceptible mice by promastigote forms. On the other hand, parasites not expressing the secreted LmNTPDase 2 did not show alterations in L. major virulence (Sansom et al., 2014).
Interaction and infection of macrophage with L. amazonensis and L. infantum pretreated with anti-NTPDase antibodies were significantly reduced, suggesting that parasite E-NTPDases could be involved in host cell interaction and invasion (Pinheiro et al., 2006; Vasconcellos et al., 2014; Peres et al., 2018). In addition, the involvement of ecto-ATPases in the invasion process of macrophage by L. amazonensis was investigated in the works of Moreira et al. (2009) and Ennes-Vidal et al. (2011). The authors pretreated promastigote forms with an ecto-ATPase inhibitor known as CrATP (chromium (III) adenosine 5-triphosphate) and observed a decrement in the adhesion and endocytic indices. Polyclonal antibodies against a conserved B domain from the potato apyrase polypeptide (r-potDomain B) and synthetic peptides designed from the B domain (LbB1LJ and LbB2LJ) were able to reduce parasite NTPDase 1 activity in different species of Leishmania (Porcino et al., 2012; Detoni et al., 2013; Maia et al., 2013). These studies could contribute to the development of specific NTPDase inhibitors.
In L. amazonensis, extracellular adenosine (produced by sequential dephosphorylation of ATP by LaNTPDases and ecto-5′-nucleotidase) binds to P1 receptors (A2A and A2B) on macrophage membrane (Csóka et al., 2008; Figueiredo et al., 2012; Figueiredo et al., 2016). Because of the interaction between adenosine and P1 receptors, activated macrophages reduce IL-12 and TNF-α cytokines levels, leading to the decrement of nitric oxide (NO•) production and the establishment of parasites in the host cell. Thus, the increase of adenosine levels during Leishmania infection seems to contribute to the host-parasite interaction, increasing the parasitism and delaying the lesion remission (Olivier et al., 2005; Gomes et al., 2015; Vijayamahantesh et al., 2017). Interestingly, when the adenosine receptor is blocked or ablated, or even when adenosine is removed by enzymatic activity, during the infection, the host-parasite interaction is significantly reduced (Figueiredo et al., 2017).
The incubation of L. amazonensis (original strain) with DIDS (4,4’- diisothiocyanatostilbene 2,2’-disulfonic acid), a well-known LaNTPDase inhibitor, prevents the reduction of NO• by activated macrophages (J774), decreasing the parasite survival (Gomes et al., 2015). Moreover, the production of IL-12 and TNF-α by macrophages infected with DIDS-treated L. amazonensis was significantly higher than that observed for macrophages infected with untreated parasites. Such results suggest that the inhibition of the LaNTPDase activity by DIDS reduced L. amazonensis capacity to down-modulate the release of inflammatory cytokines, which are essential for NO• production by inducible Nitric Oxide Synthase (iNOS) (Gomes et al., 2015). The results obtained by Gomes et al. (2015) reinforce the role of LaNTPDase in modulating the host immune system, which is crucial for the success of L. amazonensis infection.
Gomes et al. (2015) showed that the blockage of receptors A2B in J774 macrophages led to the increase of NO• production and the reduction of parasite survival in stimulated macrophages. The authors also showed that the blockage of A2B receptors led to the increase of TNF-α and IL-12 production in macrophages infected with L. amazonensis, indicating the importance of adenosine production to the macrophages’ modulation. Interestingly, using the adenosine analog known as NECA (5’-(N-Ethylcarbox- amido) adenosine-NECA) during the infection of macrophages with the avirulent clone 1IIId, the authors observed an increase in the ability of the avirulent clone to survive within macrophages. Moreover, the treatment with NECA was able to reduce the levels of TNF-α, IL-12, and NO• in macrophages, showing that the interaction between adenosine and A2B receptors is essential for the host immune system modulation since the treatment with NECA was able to recover the capacity of the 1IIId clone to survive in stimulated macrophages. Thus, Gomes et al. (2015) described a mechanism that correlates LaNTPDase/nucleotidase activity and the capacity of the parasite to down-modulate the host immune system. Specifically, the augmentation of adenosine levels at the beginning of infection seems to compromise the macrophage activation, leading to the blockage of cytokines on the host cell (Gomes et al., 2015).
Working with L. amazonensis promastigotes resistant to vinblastine (a cell division blocker), Giarola et al. (2014) observed an increase in the ecto-ATPase activity and a more severe disease scenario. The authors also found a two-fold higher ecto-ATPase protein expression in vinblastine-resistant promastigotes than in control cells. The data obtained by Giarola et al. (2014) show that there seems to be a positive correlation between higher ecto-ATPase activity and greater infectivity/disease severity.
Trypanosoma spp.
Parasites from the genus Trypanosoma (Trypanosomatida, Kinetoplastea) are flagellated protozoa that cause a wide range of diseases in both humans and animals. Such parasites are transmitted between hosts by insect vectors (Hutchinson and Stevens, 2018). Among the numerous parasite species belonging to the genus Trypanosoma, some of them have medical (T. cruzi, which cause Chagas disease in the Americas and T. brucei rhodesiense and T. brucei gambiense that cause sleeping sickness in human African) and veterinarian (T. evansi, T. vivax, T. brucei, T. copemani, T. equiperdum) importance (Büscher et al., 2017).
Trypanosoma cruzi is the etiological agent of American trypanosomiasis or Chagas disease. The disease is endemic in the southern USA and 21 countries across Latin America, with ∼7 million people infected and 70 million at risk (Moretti et al., 2020). Trypanosoma evansi is the etiologic agent of surra, a disease that occurs in several animal species, such as equids, leading to significant losses in global production since it can be fatal when is late diagnosed (Desquesnes et al., 2013).
In T. cruzi, only one gene coding for TcNTPDase is present in its genome, named TcNTPDase 1 (Fietto et al., 2004; da Silva et al., 2021). The TcNTPDase 1 coding sequence has been cloned and expressed in the bacterial system. Biochemical characterization showed that TcNTPDase 1 is an Apyrase/CD39/E-NTPDase family member. Moreover, TcNTPDase1 activity on T. cruzi membrane has also been demonstrated (Bisaggio et al., 2003; Fietto et al., 2004; Meyer-Fernandes et al., 2004; Santos et al., 2009; Giarola et al., 2013; Mariotini-Moura et al., 2014; da Silva et al., 2021).
Recently, Silva-Gomes et al. (2020) engineered T. cruzi parasites overexpressing the TcNTPDase 1 gene and observed higher infectivity rates when compared to the control cell line. On the other hand, when TcNTPDase 1 gene was silenced, the infectivity rates decreased significantly. These data are in agreement with the idea that TcNTPDase 1 could be involved in T. cruzi infectivity (Silva-Gomes et al., 2020).
In vitro studies showed that T. cruzi adhesion and internalization into macrophages were significantly compromised when parasites were treated with DIDS and Suramin, a treatment that also inhibited the ecto-ATPase activity. On the other hand, the augmentation of ecto-ATPase activity was accompanied by the increase of parasite adhesion to macrophages (Bisaggio et al., 2003). Experiments using recombinant TcNTPDase 1 (acting as a competitor) or anti-TcNTPDase 1 polyclonal antibodies (acting as blockers) evidenced the involvement of TcNTPDase 1 in parasite-host interaction and cell adhesion (Mariotini-Moura et al., 2014).
Santos et al. (2009) also showed the importance of T. cruzi NTPDase 1 in infectivity and virulence. T. cruzi treated with E-NTPDase partial inhibitors (ARL67156, Gadolinium, and Suramin), and TcNTPDase 1 antibody markedly reduced trypomastigotes infectivity. The authors observed lower levels of parasitemia when TcNTPDase-inhibited trypomastigotes were used to infect mice, which increased the host survival (Santos et al., 2009).
Studies have shown that suramin is a potent inhibitor of T. cruzi E-NTPDase, and in vivo experiments suggest that this drug can negatively affect the purinergic signaling of host cells infected with T. cruzi (Bisaggio et al., 2003; Santos et al., 2009). Novaes et al. (2018) studied the effect of suramin in the chemotherapy of murine model of Chagas disease. T. cruzi-infected mice submitted to Suramin-based chemotherapy showed increased reactive tissue damage, inflammation, and parasitism. In addition, Suramin-based treatment aggravated myocarditis cases and increased the mortality rates (Novaes et al., 2018). This unexpected result could be explained by the fact that Santos et al. (2009) used Suramin only in the parasites, before infection, while Novaes et al. (2018) used Suramin in the host cells. In addition, it is worth mentioning that Suramin is a P2 antagonist in mammalian cells (Lambrecht et al., 2002). Thus, the apparent disagreement between the works of Santos et al. (2009) and Novaes et al. (2018) seems due to the absence of specificity of Suramin, acting on both P2 receptors and T. cruzi E-NTPDase. These results reinforce the importance of working with specific E-NTPDase inhibitors, whose absence has become an obstacle to be overcome (da Silva et al., 2021).
The enzyme adenosine deaminase (ADA) is responsible for regulating the extracellular adenosine concentration, converting adenosine to inosine (Franco et al., 1997). do Carmo et al. (2017) performed an experimental combined treatment of Chagas disease using both deoxyconformycin (a potent ADA inhibitor) and 3`-deoxyadenosine (adenosine analogue). According to the authors, the results observed in the experiments, which include reduced parasitemia and cardiac inflammatory infiltrates, are due to the modulation of seric NTPDase and ADA activities (do Carmo et al., 2017). Similar results were observed when mice were infected with T. evansi (Dalla Rosa et al., 2013; Dalla Rosa et al., 2015) and T. brucei (Rottenberg et al., 2005).
Fracasso et al. (2021) investigated the effects of benznidazole (BNZ, currently used in the treatment of Chagas Disease), and resveratrol (RSV, a natural polyphenol with antioxidant and neuroprotector activities) alone and in combination during acute T. cruzi infection in the mouse cerebral cortex. The treatment with RSV in the infected group reduced NTPDase and 5’-NT activity and diminished ATP, ADP, and AMP hydrolysis compared to the non-treated group. The combination of RSV + BNZ decreased AMP hydrolysis in infected animals compared to the non-treated group, exerting an anti-inflammatory effect. Treatment with BNZ and RSV alone or associated reduced adenosine (ADO) levels and converted inosine by E-ADA (adenosine deaminase extracellular). These results suggested a suitable immunosuppressive effect of RSV during acute T. cruzi infection. Thus, the authors concluded that resveratrol could act as a neuroprotective molecule, probably preventing inflammatory changes caused by infection by T. cruzi, even though the mice experienced high levels of parasitemia (Fracasso et al., 2021).
The pre-treatment with curcumin in rats infected with T. evansi decreased parasitemia and mortality (Wolkmer et al., 2013; Wolkmer et al., 2019), maintained the NTPDase activity reduced and enhanced ADA activity on the surface of lymphocytes. These results suggest that curcumin treatment can improve immunomodulatory response mediated by ecto-nucleotidases, favoring the response against the parasite (Wolkmer et al., 2019).
Taking together, these studies show that purinergic signaling carries out a pivotal function in the immune and inflammatory responses in the course of parasite infections.
Trichomonas vaginalis
The flagellated protozoan Trichomonas vaginalis infects the human urogenital tract causing trichomoniasis, a sexually transmitted disease. In women, such infection can lead to cervical cancers, infertility, pelvic inflammatory disease, and pregnancy complications (Mielczarek and Blaszkowska, 2016).
Five putative NTPDase genes (TvNTPDase1–5) were found in the T. vaginalis genome database (Frasson et al., 2016). Regarding substrate specificity, T. vaginalis NTPDase activity was able to hydrolyze both purine and pyrimidine nucleosides, 5-di- and 5-triphosphate (de Aguiar Matos et al., 2001; Frasson et al., 2012). In addition, TvNTPDases localization and activity have been shown on T. vaginalis surface (de Jesus et al., 2002; Tasca et al., 2004), as well as on T. foetus surface (Jesus et al., 2002). The presence of such enzymes on the cell surface is important for parasite viability when exposed to extracellular nucleotides. Cell surfaced-localized TvNTPDases play an important role concerning the maintenance of extracellular adenosine levels and the purine salvage pathway in the parasite (de Jesus et al., 2002; Jesus et al., 2002; Ferla and Tasca, 2021).
The effect of the compounds metronidazole (MTZ) and tinidazole (TNZ) on T. vaginalis NTPDase and 5’NT activities was investigated (Tasca et al., 2003). In this study, two strains were used, one long-term-grown isolate and one fresh clinical isolate. The hydrolysis of ATP and ADP was found to be 5- to 7-fold higher in the fresh clinical isolate than in the long-term-grown strain. In fresh clinical isolate, the compound TNZ inhibited the ATP hydrolysis by 33%. On the other hand, the same enzyme activity was induced by MTZ in long-term grown strain. When both isolates were treated with MTZ for 2 h, the ATP and ADP hydrolysis was significantly inhibited, while only fresh clinical isolate had the ATP and ADP hydrolysis inhibited by treatment with TNZ. The 5’NT activity was not changed by TNZ or MTZ treatment. Taken together, these data indicate that the parasite modulates the extracellular ATP and ADP levels when submitted to the treatment with TNZ and MTZ as a protection mechanism to survive in unfavorable conditions (Tasca et al., 2003; Ferla and Tasca, 2021).
The ecto-NTPDase activity of the T. vaginalis was inhibited by the plant alkaloids lycorine and candimine (Giordani et al., 2010; Petró-Silveira et al., 2020). Recent studies demonstrated that the effect of lycorine involves the inhibition of T. vaginalis NTPDase, generating extracellular ATP accumulation and stimulating ROS release by neutrophils. According to Petró-Silveira et al. (2020), lycorine could make T. vaginalis more susceptible to the host immune system, leading to parasite elimination. Moreover, during T. vaginalis infection, NO production by neutrophils, via A2A receptors activation, seems to be dependent on both the ecto-5′-nucleotidase activity and the adenosine levels (Frasson et al., 2012). In addition, the levels of ROS and IL-8 were decreased by Adenosine in neutrophils infected with T. vaginalis, probably due to the activation of A1 adenosine receptors (Frasson et al., 2017). Thus, TvNTPDAse and 5’NT activities carry out an important role in adenosine production, triggering an efficient purinergic-signaling cascade, which allows the parasite to escape the host immune response (Petró-Silveira et al., 2020). In addition, the treatment of trophozoites of T. vaginalis freshly isolated with hormones, especially steroids, also inhibited TvNTPDase activity, and this inhibition seems to be associated with the decreased gene expression of the TvNTPDases (Rückert et al., 2009; Rückert et al., 2010).
Toxoplasma gondii
The intracellular protozoan Toxoplasma gondii infects mammals and other animals, causing a disease known as toxoplasmosis, which can be severe to the fetus, when infection takes place during pregnancy, and to immunocompromised individuals (Montoya and Liesenfeld, 2004).
Both isoforms, TgNTPDase 1 and TgNTPDase 2 are expressed in virulent strains, while in avirulent strains only the TgNTPDase 2 isoform is expressed. These isoforms have different enzymatic specificity. TgNTPDase 1 hydrolyzes nucleoside triphosphate, and TgNTPDase 2 hydrolyzes diphosphate substrates. However, both forms of TgNTPDase are activated by dithiothreitol (Asai et al., 1995; Nakaar et al., 1998).
The T. gondii NTPDases have become a target in drug development. Parasites expressing an antisense TgNTPDase-RNA showed reduced TgNTPDase expression and activity. In addition, parasites expressing the antisense TgNTPDase-RNA showed a lower ability to proliferate, suggesting that TgNTPDases could be involved in T. gondii replication (Nakaar et al., 1999). This conclusion was supported by studies with monoclonal antibodies against NTPDase 2 of T. gondii that inhibited TgNTPDase activity and decreased T. gondii tachyzoites replication (Tan et al., 2010).
The treatment of mice infected with T. gondii with Diphenyl diselenide (PhSe)2 reduced histological inflammatory markers, ROS levels, and ADA activity in the spleen, besides reversing splenomegaly. In addition, TgNTPDase and 5’NT activity were increased in the spleen, stimulating the hydrolysis of ATP and the enhancement of adenosine production in the tissue. These results indicate that purinergic signaling could be involved in the pathogenesis of T. gondii infection (Doleski et al., 2017a; Doleski et al., 2017b).
Bottari et al. (2020) demonstrated that mice treated with resveratrol during chronic infection by T. gondii increased NTPDase and reduced ADA activities. The adenosine signalization by regulating A1 and A2A receptors expression promoted anti-inflammatory and neuroprotector mechanisms during T. gondii infection (Bottari et al., 2020).
Schistosoma spp.
The genus Schistosoma holds helminth parasites responsible for causing a human disease known as Schistosomiasis, which causes liver, intestinal, lung, and chronic pelvic inflammations (Colley and Secor, 2014).
In Schistosoma mansoni two NTPDase isoforms (SmATPDases 1 and 2) were detected and partially purified. It was shown that S. mansoni NTPDase activity can be found throughout the parasite life cycle, besides the involvement in the worm metabolism and the host-parasite interaction (Vasconcelos et al., 1993; Vasconcelos et al., 1996; DeMarco et al., 2003; Da'dara et al., 2014). SmATPDase 1 is located on the surface and SmATPDase 2 is in the tegument and secreted by eggs and adult worms (Faria-Pinto et al., 2004; Fietto et al., 2004; Levano-Garcia et al., 2007). Both isoforms showed immune cross-reactivity with antipotato apyrase antibodies (Faria-Pinto et al., 2008; Faria-Pinto et al., 2010). Macrophages incubated with antibodies against the ATPDase isoforms of S. mansoni showed reduced NTPDase 1 activity, and the parasite proliferation was inhibited (Marconato et al., 2017).
Schistosoma eggs found in the host tissues trigger an inflammatory response known as granulomatous, characterized by the presence of granulomas in the infected tissues (Farah et al., 2000). Gusmão et al. (2021) showed that immunization of C57BL/6 mice with potato apyrase was ineffective in triggering a protective immune response against Schistosoma infection. Differently, immunization using potato apyrase decreased the granulomatous response, accompanied by an enhancement of multinucleated giant cells. In this sense, immunotherapies developed from potato apyrase-immunization to avoid granuloma development seems to be a potential prophylaxis strategy (Gusmão et al., 2021).
Recently, studies have demonstrated that some flavonoids and chalcones (natural plant metabolites), such as licoflavone B and cardamonin display antiparasitic activities against adult worms of S. mansoni (de Carvalho et al., 2015; de Castro et al., 2015; Souza et al., 2017). The effects of licoflavone B and cardamonin on the S. mansoni NTPDase activity were investigated. In addition, a molecular docking approach was also used to study the mode of action of such compounds on S. mansoni ATPDase 1 (de Castro et al., 2015; de Carvalho et al., 2015). de Castro et al. (2015) showed that ATP-diphosphohydrolase activity was inhibited by about 82% by cardamonin (40 μM), with an IC50 of 23.54 μM, while ADPase activity was unchanged. In addition, interactions between cardamonin and SmATPase1 seem to be done especially through hydrogen and hydrophobic bonds, as revealed by molecular docking studies (de Castro et al., 2015). Regarding licoflavone B, a concentration of 40 μM was able to abolish 84% of the ATP-diphosphohydrolase activity, with an IC50 of 23.78 μM. Different from cardamonin that did not show SmADPase activity inhibition, licoflavone B in a concentration of 40 μM inhibited 56% of the SmADPase activity. The interaction between licoflavone B and SmATPase 1 seems to involve nine dock positions at the Nucleotide-Binding Site of the enzyme (de Carvalho et al., 2015; de Carvalho et al., 2019).
The schistosomicidal effect of two synthetic chalcones (E-4’-hydroxychalcone and E-2’-hydroxy-4-methoxychalcone) was studied by Pereira et al. (2018). The authors showed that both compounds can inhibit S. mansoni ATP-diphosphohydrolase activity, besides causing a decrease in parasite load in mice. Martins et al. (2000) evaluated the inhibitory effect of sesquiterpene lactones on S. mansoni ATP-diphosphohydrolase. The authors showed that the compounds thapsigargin and thapsigargicin inhibited 70% and 57% of the enzyme’s activity, respectively.
Concluding Remarks
Many studies have described the involvement of E-NTPDase activity in parasite nutrition, differentiation, invasion, survival in the host cell, modulation of the host immune response, and establishment of infection. Since E-NTPDases have been implicated in important events for different parasite species, such enzymes have been considered as ideal drug targets for many therapeutic applications, including parasitic diseases. In addition, ecto-nucleotidase activity associated with the purinergic signaling seems to be crucial to parasite-host interaction, besides contributing to adenine acquisition via the purine salvage pathway.
In this context, this review emphasizes the importance of ecto-nucleotidases in parasite physiology and pathogenesis. Moreover, we highlight the importance of such enzymes as drug target candidates to the development of new antiparasitic compounds, including those able to inhibit specifically the different ecto-nucleotidases isoforms.
Although many compounds have been assayed against ecto-nucleotidases from different parasites to inhibit the enzyme activity, the low specificity of these molecules represents an obstacle to be eliminated, still requiring huge efforts in the search for new selective and effective ecto-nucleotidase inhibitors.
Author Contributions
LP-V, AG-V, and JM-F designed the study and wrote the paper. LP-V, AG-V, and JM-F edited and reviewed the final version of the manuscript. All authors contributed equally to the article and approved the submitted version.
Funding
This work was supported by grants from the Brazilian funding agencies Conselho Nacional de Desenvolvimento Científico e Tecnológico (CNPq - Grant Number: 401134/2014–8), Coordenação de Aperfeiçoamento de Pessoal de Nível superior (CAPES - Grant Number: 0012017), Fundação Carlos Chagas Filho de Amparo à Pesquisa do Estado do Rio de Janeiro (FAPERJ - Grant Number: e-26/201.300/2014) to JM-F.
Conflict of Interest
The authors declare that the research was conducted in the absence of any commercial or financial relationships that could be construed as a potential conflict of interest.
Publisher’s Note
All claims expressed in this article are solely those of the authors and do not necessarily represent those of their affiliated organizations, or those of the publisher, the editors and the reviewers. Any product that may be evaluated in this article, or claim that may be made by its manufacturer, is not guaranteed or endorsed by the publisher.
References
Afzal, S., Al-Rashida, M., Hameed, A., Pelletier, J., Sévigny, J., Iqbal, J. (2021). Synthesis, In-Vitro Evaluation and Molecular Docking Studies of Oxoindolin Phenylhydrazine Carboxamides as Potent and Selective Inhibitors of Ectonucleoside Triphosphate Diphosphohydrolase (NTPDase). Bioorg. Chem. 112, 104957. doi: 10.1016/j.bioorg.2021.104957
Afzal, S., Zaib, S., Jafari, B., Langer, P., Lecka, J., Sévigny, J., et al. (2020). Highly Potent and Selective Ectonucleoside Triphosphate Diphosphohydrolase (ENTPDase1, 2, 3 and 8) Inhibitors Having 2-Substituted-7- Trifluoromethyl-Thiadiazolopyrimidone. Med. Chem. 16 (5), 689–702. doi: 10.2174/1573406415666190614095821
Al-Rashida, M., Iqbal, J. (2014). Therapeutic Potentials of Ecto-Nucleoside Triphosphate Diphosphohydrolase, Ecto-Nucleotide Pyrophosphatase/Phosphodiesterase, Ecto-5'-Nucleotidase, and Alkaline Phosphatase Inhibitors. Med. Res. Rev. 34 (4), 703–743. doi: 10.1002/med.21302
Antonioli, L., Fornai, M., Blandizzi, C., Pacher, P., Haskó, G. (2019). Adenosine Signaling and the Immune System: When a Lot Could be Too Much. Immunol. Lett. 205, 9–15. doi: 10.1016/j.imlet.2018.04.006
Arora, K., Rai, A. K. (2019). Dependence of Leishmania Parasite on Host Derived ATP: An Overview of Extracellular Nucleotide Metabolism in Parasite. J. Parasitol. Dis. 43 (1), 1–13. doi: 10.1007/s12639-018-1061-4
Asai, T., Miura, S., Sibley, L. D., Okabayashi, H., Takeuchi, T. (1995). Biochemical and Molecular Characterization of Nucleoside Triphosphate Hydrolase Isozymes From the Parasitic Protozoan Toxoplasma Gondii. J. Biol. Chem. 270 (19), 11391–11397. doi: 10.1074/jbc.270.19.11391
Baqi, Y., Rashed, M., Schäkel, L., Malik, E. M., Pelletier, J., Sévigny, J., et al. (2020). Development of Anthraquinone Derivatives as Ectonucleoside Triphosphate Diphosphohydrolase (NTPDase) Inhibitors With Selectivity for NTPDase2 and Ntpdase3. Front. Pharmacol. 11, 1282. doi: 10.3389/fphar.2020.01282
Barros, F. S., De Menezes, L. F., Pinheiro, A. A., Silva, E. F., Lopes, A. H., De Souza, W., et al. (2000). Ectonucleotide Diphosphohydrolase Activities in Entamoeba Histolytica. Arch. Biochem. Biophys. 375, 304–314. doi: 10.1006/abbi.1999.1592
Battastini, A. M. O., Figueiró, F., Leal, D. B. R., Doleski, P. H., Schetinger, M. R. C. (2021). CD39 and CD73 as Promising Therapeutic Targets: What Could Be the Limitations? Front. Pharmacol. 12, 633603. doi: 10.3389/fphar.2021.633603
Bernardes, C. F., Meyer-Fernandes, J. R., Saad-Nehme, J., Vannier-Santos, M. A., Peres- Sampaio, C. E., Vercesi, A. E. (2000). Effects of 4,4′ -Diisothyocyanatostilbene-2,2′ - Disulfonic Acid on Trypanosoma Cruzi Proliferation and Ca (2+) Homeostasis. Int. J. Biochem. Cell Biol. 32, 519–527. doi: 10.1016/S1357-2725(00)00002-9
Berrêdo-Pinho, M., Peres-Sampaio, C. E., Chrispim, P. P., Belmont-Firpo, R., Lemos, A. P., Martiny, A., et al. (2001). A Mg-Dependent Ecto-ATPase in Leishmania Amazonensis and its Possible Role in Adenosine Acquisition and Virulence. Arch. Biochem. Biophysics 391 (1), 16–24. doi: 10.1006/abbi.2001.2384
Bisaggio, D. F., Peres-Sampaio, C. E., Meyer-Fernandes, J. R., Souto-Padron, T. (2003). Ecto-ATPase Activity on the Surface of Trypanosoma Cruzi and its Possible Role in the Parasite-Host Cell Interaction. Parasitology Res. 91 (4), 273–282. doi: 10.1007/s00436-003-0965-8
Bottari, N. B., Reichert, K. P., Fracasso, M., Dutra, A., Assmann, C. E., Ulrich, H., et al. (2020). Neuroprotective Role of Resveratrol Mediated by Purinergic Signalling in Cerebral Cortex of Mice Infected by Toxoplasma Gondii. Parasitol. Res. 119 (9), 2897–2905. doi: 10.1007/s00436-020-06795-0
Burza, S., Croft, S. L., Boelaert, M. (2018). Leishmaniasis. Lancet (London England) 392 (10151), 951–970. doi: 10.1016/S0140-6736(18)31204-2
Büscher, P., Cecchi, G., Jamonneau, V., Priotto, G. (2017). Human African Trypanosomiasis. Lancet (London England) 390 (10110), 2397–2409. doi: 10.1016/S0140-6736(17)31510-6
Chaves, M. M., Savio, L., Coutinho-Silva, R. (2021). Purinergic Signaling: A New Front-Line Determinant of Resistance and Susceptibility in Leishmaniasis. Biomed. J. S2319-4170(21)00069-X. doi: 10.1016/j.bj.2021.06.003
Colley, D. G., Secor, W. E. (2014). Immunology of Human Schistosomiasis. Parasite Immunol. 36 (8), 347–357. doi: 10.1111/pim.12087
Coutinho-Silva, R., Savio, L. (2021). Purinergic Signalling in Host Innate Immune Defence Against Intracellular Pathogens. Biochem. Pharmacol. 187, 114405. doi: 10.1016/j.bcp.2021.114405
Csóka, B., Himer, L., Selmeczy, Z., Vizi, E. S., Pacher, P., Ledent, C., et al. (2008). Adenosine A2A Receptor Activation Inhibits T Helper 1 and T Helper 2 Cell Development and Effector Function. FASEB J. 22 (10), 3491–3499. doi: 10.1096/fj.08-107458
Da'dara, A. A., Bhardwaj, R., Skelly, P. J. (2014). Schistosome Apyrase SmATPDase1, But Not SmATPDase2, Hydrolyses Exogenous ATP and ADP. Purinergic Signal. 10 (4), 573–580. doi: 10.1007/s11302-014-9416-5
Dalla Rosa, L., da Silva, A. S., Gressler, L. T., Oliveira, C. B., Dambrós, M. G., Miletti, L. C., et al. (2013). Cordycepin (3'-Deoxyadenosine) Pentostatin (Deoxycoformycin) Combination Treatment of Mice Experimentally Infected With Trypanosoma Evansi. Parasitology 140 (5), 663–671. doi: 10.1017/S0031182012001990
Dalla Rosa, L., Da Silva, A. S., Oliveira, C. B., Gressler, L. T., Arnold, C. B., Baldissera, M. D., et al. (2015). Dose Finding of 3'deoxyadenosine and Deoxycoformycin for the Treatment of Trypanosoma Evansi Infection: An Effective and Nontoxic Dose. Microb. Pathog. 85, m21–m28. doi: 10.1016/j.micpath.2015.05.005
da Silva, W., da Rocha Torres, N., de Melo Agripino, J., da Silva, V., de Souza, A., Ribeiro, I. C., et al. (2021). ENTPDases From Pathogenic Trypanosomatids and Purinergic Signaling: Shedding Light Towards Biotechnological Applications. Curr. Topics Med. Chem. 21 (3), 213–226. doi: 10.2174/1568026620666201005125146
de Aguiar Matos, J. A., Borges, F. P., Tasca, T., Bogo, M. R., De Carli, G. A., da Graça Fauth, M., et al. (2001). Characterization of an ATP Diphosphohydrolase (Apyrase, EC 3.6.1.5) Activity in Trichomonas Vaginalis. Int. J. Parasitol. 31 (8), 770–775. doi: 10.1016/s0020-7519(01)00191-6
de Almeida Marques-da-Silva, E., de Oliveira, J. C., Figueiredo, A. B., de Souza Lima Júnior, D., Carneiro, C. M., Rangel Fietto, J. L., et al. (2008). Extracellular Nucleotide Metabolism in Leishmania: Influence of Adenosine in the Establishment of Infection. Microbes Infect. 10 (8), 850–857. doi: 10.1016/j.micinf.2008.04.016
de Carvalho, L., Alves, I. J., Jr, Junqueira, L. R., Silva, L. M., Riani, L. R., de Faria Pinto, P., et al. (2019). ATP-Diphosphohydrolases in Parasites: Localization, Functions and Recent Developments in Drug Discovery. Curr. Protein Pept. Sci. 20 (9), 873–884. doi: 10.2174/1389203720666190704152827
de Carvalho, L. S. A., Geraldo, R. B., De Moraes, J., Silva Pinto, P. L., De Faria Pinto, P., Pereira, O.d. S., et al. (2015). Schistosomicidal Activity and Docking of Schistosoma Mansoni ATPDase 1 With Licoflavone B Isolated From Glycyrrhiza Inflata (Fabaceae). Exp. Parasitol. 159, 207–214. doi: 10.1016/j.exppara.2015.09.015
de Castro, C. C., Costa, P. S., Laktin, G. T., de Carvalho, P. H., Geraldo, R. B., de Moraes, J., et al. (2015). Cardamonin, a Schistosomicidal Chalcone From Piper Aduncum L. (Piperaceae) That Inhibits Schistosoma Mansoni ATP Diphosphohydrolase. Phytomedicine 22 (10), 921–928. doi: 10.1016/j.phymed.2015.06.009
de Jesus, J. B., de Sá Pinheiro, A. A., Lopes, A. H., Meyer-Fernandes, J. R. (2002). An Ectonucleotide ATP-Diphosphohydrolase Activity in Trichomonas Vaginalis Stimulated by Galactose and its Possible Role in Virulence. Z Naturforsch. C. 57, 890–896. doi: 10.1515/znc-2002-9-1022
DeMarco, R., Kowaltowski, A. T., Mortara, R. A., Verjovski-Almeida, S. (2003). Molecular Characterization and Immunolocalization of Schistosoma Mansoni ATP-Diphosphohydrolase. Biochem. Biophys. Res. Commun. 307 (4), 831–838. doi: 10.1016/S0006-291X(03)01268-3
de Souza Leite, M., Thomaz, R., Fonseca, F. V., Panizzutti, R., Vercesi, A. E., Meyer-Fernandes, J. R. (2007). Trypanosoma Brucei Brucei: Biochemical Characterization of Ecto-Nucleoside Triphosphate Diphosphohydrolase Activities. Exp. Parasitol. 115 (4), 315–323. doi: 10.1016/j.exppara.2006.09.002
Desquesnes, M., Dargantes, A., Lai, D. H., Lun, Z. R., Holzmuller, P., Jittapalapong, S. (2013). Trypanosoma Evansi and Surra: A Review and Perspectives on Transmission, Epidemiology and Control, Impact, and Zoonotic Aspects. BioMed. Res. Int. 2013, 321237. doi: 10.1155/2013/321237
Detoni, M. L., Fessel, M. R., Maia, A. C., Porcino, G. N., Quellis, L. R., Faria-Pinto, P., et al. (2013). An Antigenic Domain of the Leishmania Amazonensis Nucleoside Triphosphate Diphosphohydrolase (NTPDase 1) Is Associated With Disease Progression in Susceptible Infected Mice. Parasitol. Res. 112 (8), 2773–2782. doi: 10.1007/s00436-013-3445-9
do Carmo, G. M., Doleski, P. H., de Sá, M. F., Grando, T. H., Azevedo, M. I., Manzoni, A. G., et al. (2017). Treatment With 3'-Deoxyadenosine and Deoxycoformycin in Mice Infected by Trypanosoma Cruzi and Its Side Effect on Purinergic Enzymes. Microb. Pathog. 113, 51–56. doi: 10.1016/j.micpath.2017.10.030
Doleski, P. H., Leal, D., Machado, V. S., Bottari, N. B., Manzoni, A. G., Casali, E. A., et al. (2017b). Diphenyl Diselenide Modulates Nucleotidases, Reducing Inflammatory Responses in the Liver of Toxoplasma Gondii-Infected Mice. Purinergic Signal. 13 (4), 489–496. doi: 10.1007/s11302-017-9575-2
Doleski, P. H., Ten Caten, M. V., Passos, D. F., Castilhos, L. G., Leal, D., Machado, V. S., et al. (2017a). Toxoplasmosis Treatment With Diphenyl Diselenide in Infected Mice Modulates the Activity of Purinergic Enzymes and Reduces Inflammation in Spleen. Exp. Parasitol. 181, 7–13. doi: 10.1016/j.exppara.2017.07.001
Ennes-Vidal, V., Castro, R. O., Britto, C., Barrabin, H., D’Avila-Levy, C. M., Moreira, O. C. (2011). CrATP Interferes in the Promastigote-Macrophage Interaction in Leishmania Amazonensis Infection. Parasitology 138 (8), 960–968. doi: 10.1017/S0031182011000710
Farah, I. O., Nyindo, M., King, C. L., Hau, J. (2000). Hepatic Granulomatous Response to Schistosoma Mansoni Eggs in BALB/c Mice and Olive Baboons (Papio Cynocephalus Anubis). J. Comp. Pathol. 123 (1), 7–14. doi: 10.1053/jcpa.1999.0378
Faria-Pinto, P., Meirelles, M. N., Lenzi, H. L., Mota, E. M., Penido, M. L., Coelho, P. M., et al. (2004). ATP Diphosphohydrolase From Schistosoma Mansoni Egg: Characterization and Immunocytochemical Localization of a New Antigen. Parasitology 129, 51–57. doi: 10.1017/s0031182004005244
Faria-Pinto, P., Montesano, M. A., Jacinto, A. A., Santos, R. S., Bordin, F. H., Ferreira, A. P., et al. (2010). Antibody Reactivity Against Potato Apyrase, a Protein That Shares Epitopes With Schistosoma Mansoni ATP Diphosphohydrolase Isoforms, in Acute and Chronically Infected Mice, After Chemotherapy and Reinfection. Memorias do Inst Oswaldo Cruz 105 (4), 374–379. doi: 10.1590/s0074-02762010000400005
Faria-Pinto, P., Rezende-Soares, F. A., Molica, A. M., Montesano, M. A., Marques, M. J., Rocha, M. O., et al. (2008). Mapping of the Conserved Antigenic Domains Shared Between Potato Apyrase and Parasite ATP Diphosphohydrolases: Potential Application in Human Parasitic Diseases. Parasitology 135 (8), 943–953. doi: 10.1017/S0031182008004538
Ferla, M., Tasca, T. (2021). The Role of Purinergic Signaling in Trichomonas Vaginalisinfection. Curr. Topics Medicinal Chem. 21 (3), 181–192. doi: 10.2174/1568026620999200904122212
Fietto, J. L., DeMarco, R., Nascimento, I. P., Castro, I. M., Carvalho, T. M., de Souza, W., et al. (2004). Characterization and Immunolocalization of an NTP Diphosphohydrolase of Trypanosoma Cruzi. Biochem. Biophys. Res. Commun. 316, 454–460. doi: 10.1016/j.bbrc.2004.02.07
Figueiredo, A. B., Serafim, T. D., Marques-da-Silva, E. A., Meyer-Fernandes, J. R., Afonso, L. C. C. (2012). Leishmania Amazonensis Impairs DC Function by Inhibiting CD40 Expression via A2B Adenosine Receptor Activation. Eur. J. Immunol. 42, 1203–1215. doi: 10.1002/eji.201141926
Figueiredo, A. B., Souza-Testasicca, M. C., Afonso, L. C. C. (2016). Purinergic Signaling and Infection by Leishmania: A New Approach to Evasion of the Immune Response. Biomed. J. 39 (4), 244–250. doi: 10.1016/j.bj.2016.08.004
Figueiredo, A. B., Souza-Testasicca, M. C., Mineo, T. W. P., Afonso, L. C. C. (2017). Leishmania Amazonensis-Induced cAMP Triggered by Adenosine A2B Receptor Is Important to Inhibit Dendritic Cell Activation and Evade Immune Response in Infected Mice. Front. Immunol. 8, 849. doi: 10.3389/fimmu.2017.00849
Fonseca, F. V., Fonseca de Souza, A. L., Mariano, A. C., Entringer, P. F., Gondim, K. C., Meyer-Fernandes, J. R. (2006). Trypanosoma Rangeli: Characterization of a Mg-Dependent Ecto ATP-Diphosphohydrolase Activity. Exp. Parasitology 112 (2), 76–84. doi: 10.1016/j.exppara.2005.09.005
Fracasso, M., Reichert, K., Bottari, N. B., da Silva, A. D., Schetinger, M., Monteiro, S. G., et al. (2021). Involvement of Ectonucleotidases and Purinergic Receptor Expression During Acute Chagas Disease in the Cortex of Mice Treated With Resveratrol and Benznidazole. Purinergic Signal. 17 (3), 493–502. doi: 10.1007/s11302-021-09803-9
Franco, R., Casadó, V., Ciruela, F., Saura, C., Mallol, J., Canela, E. I., et al. (1997). Cell Surface Adenosine Deaminase: Much More Than an Ectoenzyme. Prog. Neurobiol. 52 (4), 283–294. doi: 10.1016/s0301-0082(97)00013-0
Frasson, A. P., De Carli, G. A., Bonan, C. D., Tasca, T. (2012). Involvement of Purinergic Signaling on Nitric Oxide Production by Neutrophils Stimulated With Trichomonas Vaginalis. Purinergic Signal. 8 (1), 1–9. doi: 10.1007/s11302-011-9254-7
Frasson, A. P., Dos Santos, O., Meirelles, L. C., Macedo, A. J., Tasca, T. (2016). Five Putative Nucleoside Triphosphate Diphosphohydrolase Genes Are Expressed in Trichomonas Vaginalis. FEMS Microbiol. Lett. 363 (2), fnv221. doi: 10.1093/femsle/fnv221
Frasson, A. P., Menezes, C. B., Goelzer, G. K., Gnoatto, S. C. B., Garcia, S. C., Tasca, T. (2017). Adenosine Reduces Reactive Oxygen Species and Interleukin-8 Production by Trichomonas Vaginalis-Stimulated Neutrophils. Purinergic Signal. 13 (4), 569–577. doi: 10.1007/s11302-017-9584-1
Fredholm, B. B., Chern, Y., Franco, R., Sitkovsky, M. (2007). Aspects of the General Biology of Adenosine A2A Signaling, Prog. Neurobiol. 83, 263–276. doi: 10.1016/j.pneurobio.2007.07.005
Gendron, F. P., Benrezzak, O., Krugh, B. W., Kong, Q., Weisman, G. A., Beaudoin, A. R. (2002). Purine Signaling and Potential New Therapeutic Approach: Possible Outcomes of NTPDase Inhibition. Curr. Drug Targets 3 (3), 229–245. doi: 10.2174/1389450023347713
Gendron, F. P., Halbfinger, E., Fischer, B., Duval, M., D'Orléans-Juste, P., Beaudoin, A. R. (2000). Novel Inhibitors of Nucleoside Triphosphate Diphosphohydrolases: Chemical Synthesis and Biochemical and Pharmacological Characterizations. J. Med Chem. 43 (11), 2239–2247. doi: 10.1021/jm000020b
Giarola, N. L., de Almeida-Amaral, E. E., Collopy-Júnior, I., Fonseca-de-Souza, A. L., Majerowicz, D., Paes, L. S., et al. (2013). Trypanosoma Cruzi: Effects of Heat Shock on Ecto-ATPase Activity. Exp. Parasitol. 133 (4), 434–441. doi: 10.1016/j.exppara.2012.12.014
Giarola, N. L., Silveira, T. S., Inacio, J. D., Vieira, L. P., Almeida-Amaral, E. E., Meyer-Fernandes, J. R. (2014). Leishmania Amazonensis: Increase in Ecto-ATPase Activity and Parasite Burden of Vinblastine-Resistant Protozoa. Exp. Parasitol. 146, 25–33. doi: 10.1016/j.exppara.2014.08.013
Giordani, R. B., Weizenmann, M., Rosemberg, D. B., De Carli, G. A., Bogo, M. R., Zuanazzi, J. A., et al. (2010). Trichomonas Vaginalis Nucleoside Triphosphate Diphosphohydrolase and Ecto-5'-Nucleotidase Activities are Inhibited by Lycorine and Candimine. Parasitol. Int. 59 (2), 226–231. doi: 10.1016/j.parint.2010.02.004
Gomes, R. S., de Carvalho, L. C., de Souza Vasconcellos, R., Fietto, J. L., Afonso, L. C. (2015). E-NTPDase (Ecto-Nucleoside Triphosphate Diphosphohydrolase) of Leishmania Amazonensis Inhibits Macrophage Activation. Microbes Infect. 17 (4), 295–303. doi: 10.1016/j.micinf.2014.12.009
Gusmão, M., Júnior, S. M., Marconato, D. G., Emídio, N. B., Farani, P., Gollner, Â.M., et al. (2021). Potato Apyrase Reduces Granulomatous Area and Increases Presence of Multinucleated Giant Cells in Murine Schistosomiasis. Parasitology Int. 83, 102317. doi: 10.1016/j.parint.2021.102317
Handa, M., Guidotti, G. (1996). Purification and Cloning of a Soluble ATP-Diphosphohydrolase (Apyrase) From Potato Tubers (Solanum Tuberosum). Biochem. Biophys. Res. Commun. 218 (3), 916–923. doi: 10.1006/bbrc.1996.0162
Haskó, G., Antonioli, L., Cronstein, B. N. (2018). Adenosine Metabolism, Immunity and Joint Health. Biochem. Pharmacol. 151, 307–313. doi: 10.1016/j.bcp.2018.02.002
Hayat, K., Afzal, S., Saeed, A., Murtaza, A., Ur Rahman, S., Khan, K. M., et al. (2019). Investigation of New Quinoline Derivatives as Promising Inhibitors of NTPDases: Synthesis, SAR Analysis and Molecular Docking Studies. Bioorg. Chem. 87, 218–226. doi: 10.1016/j.bioorg.2019.03.019
Hutchinson, R., Stevens, J. R. (2018). Barcoding in Trypanosomes. Parasitology 145 (5), 563–573. doi: 10.1017/S0031182017002049
Iqbal, J., Shah, S. (2018). Molecular Dynamic Simulations Reveal Structural Insights Into Substrate and Inhibitor Binding Modes and Functionality of Ecto-Nucleoside Triphosphate Diphosphohydrolases. Sci. Rep. 8 (1), 2581. doi: 10.1038/s41598-018-20971-4
Jeffrey, J. L., Lawson, K. V., Powers, J. P. (2020). Targeting Metabolism of Extracellular Nucleotides via Inhibition of Ectonucleotidases CD73 and CD39. J. Med. Chem. 63 (22), 13444–13465. doi: 10.1021/acs.jmedchem.0c01044
Jesus, J. B., Lopes, A. H., Meyer-Fernandes, J. R. (2002). Characterization of an Ecto-ATPase of Tritrichomonas Foetus. Vet. Parasitol. 103, 29–42. doi: 10.1016/s0304-4017(01)00576-3
Kanwal, Mohammed Khan, K., Salar, U., Afzal, S., Wadood, A., Taha, M., et al. (2019). Schiff Bases of Tryptamine as Potent Inhibitors of Nucleoside Triphosphate Diphosphohydrolases (NTPDases): Structure-Activity Relationship. Bioorg. Chem. 82, 253–266. doi: 10.1016/j.bioorg.2018.10.046
Klaver, D., Thurnher, M. (2021). Control of Macrophage Inflammation by P2Y Purinergic Receptors. Cells 10 (5), 1098. doi: 10.3390/cells10051098
Knowles, A. F. (2011). The GDA1_CD39 Superfamily: NTPDases With Diverse Functions. Purinergic Signal. 7 (1), 21–45. doi: 10.1007/s11302-010-9214-7
Kukulski, F., Lévesque, S. A., Sévigny, J. (2011). Impact of Ectoenzymes on P2 and P1 Receptor Signaling. Adv. Pharmacol. 61, 263–299. doi: 10.1016/B978-0-12-385526-8.00009-6
Lambrecht, G., Braun, K., Damer, M., Ganso, M., Hildebrandt, C., Ullmann, H., et al. (2002). Structure-Activity Relationships of Suramin and Pyridoxal-5'-Phosphate Derivatives as P2 Receptor Antagonists. Curr. Pharm. Design 8 (26), 2371–2399. doi: 10.2174/1381612023392973
Lecka, J., Gillerman, I., Fausther, M., Salem, M., Munkonda, M. N., Brosseau, J. P., et al. (2013). 8-BuS-ATP Derivatives as Specific NTPDase1 Inhibitors. Br. J. Pharmacol. 169 (1), 179–196. doi: 10.1111/bph.12135
Lee, S. Y., Fiene, A., Li, W., Hanck, T., Brylev, K. A., Fedorov, V. E., et al. (2015). Polyoxometalates–Potent and Selective Ecto-Nucleotidase Inhibitors. Biochem. Pharmacol. 93 (2), 171–181. doi: 10.1016/j.bcp.2014.11.002
Leite, P. M., Gomes, R. S., Figueiredo, A. B., Serafim, T. D., Tafuri, W. L., de Souza, C. C., et al. (2012). Ecto-Nucleotidase Activities of Promastigotes From Leishmania (Viannia) Braziliensis Relates to Parasite Infectivity and Disease Clinical Outcome. PLoS Neglected Trop. Dis. 6 (10), e1850. doi: 10.1371/journal.pntd.0001850
Levano-Garcia, J., Mortara, R. A., Verjovski-Almeida, S., DeMarco, R. (2007). Characterization of Schistosoma Mansoni ATPDase2 Gene, a Novel Apyrase Family Member. Biochem. Biophys. Res. Commun. 352 (2), 384–389. doi: 10.1016/j.bbrc.2006.11.023
Lévesque, S. A., Lavoie, E. G., Lecka, J., Bigonnesse, F., Sévigny, J. (2007). Specificity of the Ecto-ATPase Inhibitor ARL 67156 on Human and Mouse Ectonucleotidases. Br. J. Pharmacol. 152 (1), 141–150. doi: 10.1038/sj.bjp.070736
Lopez, V., Schäkel, L., Schuh, H., Schmidt, M. S., Mirza, S., Renn, C., et al. (2021). Sulfated Polysaccharides From Macroalgae Are Potent Dual Inhibitors of Human ATP-Hydrolyzing Ectonucleotidases NPP1 and CD39. Marine Drugs 19 (2), 51. doi: 10.3390/md19020051
Maia, A. C., Porcino, G. N., Detoni, M., Emídio, N. B., Marconato, D. G., Faria-Pinto, P., et al. (2013). An Antigenic Domain Within a Catalytically Active Leishmania Infantum Nucleoside Triphosphate Diphosphohydrolase (NTPDase 1) is a Target of Inhibitory Antibodies. Parasitol. Int. 62 (1), 44–52. doi: 10.1016/j.parint.2012.09.004
Marconato, D. G., Gusmão, M., Melo, J., Castro, J., Macedo, G. C., Vasconcelos, E. G., et al. (2017). Antischistosome Antibodies Change NTPDase 1 Activity From Macrophages. Parasite Immunol. 39 (11), e12487. doi: 10.1111/pim.12487
Mariotini-Moura, C., Silva e Bastos, M., de Castro, F. F., Trindade, M. L., Vasconcellos, R., Neves-do-Valle, M. A., et al. (2014). Trypanosoma Cruzi Nucleoside Triphosphate Diphosphohydrolase 1 (TcNTPDase-1) Biochemical Characterization, Immunolocalization and Possible Role in Host Cell Adhesion. Acta Tropica 130, 140–147. doi: 10.1016/j.actatropica.2013.11.008
Martins, S. M., Torres, C. R., Ferreira, S. T. (2000). Inhibition of the Ecto-ATPdiphosphohydrolase of Schistosoma Mansoni by Thapsigargin. Biosci Rep. 20 (5), 369–381. doi: 10.1023/a:1010330017583
Meyer-Fernandes, J. R. (2002). Ecto-ATPases in Protozoa Parasites: Looking for a Function. Parasitol. Int. 51 (3), 299–303. doi: 10.1016/s1383-5769(02)00017-x
Meyer-Fernandes, J. R., Dutra, P. M., Rodrigues, C. O., Saad-Nehme, J., Lopes, A. H. (1997). Mg-Dependent Ecto-ATPase Activity in Leishmania Tropica. Arch. Biochem. Biophys. 341 (1), 40–46. doi: 10.1006/abbi.1997.9933
Meyer-Fernandes, J. R., Lanz-Mendoza, H., Gondim, K. C., Willott, E., Wells, M. A. (2000). Ectonucleotide Diphosphohydrolase Activities in Hemocytes of Larval Manduca Sexta. Arch. Biochem. Biophys. 382 (1), 152–159. doi: 10.1006/abbi.2000.1980
Meyer-Fernandes, J. R., Saad-Nehme, J., Peres-Sampaio, C. E., Belmont-Firpo, R., Bisaggio, D. F., Do Couto, L. C., et al. (2004). A Mg-Dependent Ecto-ATPase Is Increased in the Infective Stages of Trypanosoma Cruzi. Parasitol. Res. 93 (1), 567–576. doi: 10.1007/s00436-003-1066-4
Mielczarek, E., Blaszkowska, J. (2016). Trichomonas Vaginalis: Pathogenicity and Potential Role in Human Reproductive Failure. Infection 44 (4), 447–458. doi: 10.1007/s15010-015-0860-0
Montoya, J. G., Liesenfeld, O. (2004). Toxoplasmosis. Lancet (London England) 363 (9425), 1965–1976. doi: 10.1016/S0140-6736(04)16412-X
Moreira, O. C., Rios, P. F., Esteves, F. F., Meyer-Fernandes, J. R., Barrabin, H. (2009). CrATP as a New Inhibitor of Ectoatpases of Trypanosomatids. Parasitology 136, 35–44. doi: 10.1017/S0031182008005118
Moretti, N. S., Mortara, R. A., Schenkman, S. (2020). Trypanosoma Cruzi. Trends Parasitol. 36 (4), 404–405. doi: 10.1016/j.pt.2019.10.002
Munkonda, M. N., Kauffenstein, G., Kukulski, F., Lévesque, S. A., Legendre, C., Pelletier, J., et al. (2007). Inhibition of Human and Mouse Plasma Membrane Bound NTPDases by P2 Receptor Antagonists. Biochem. Pharmacol. 74 (10), 1524–1534. doi: 10.1016/j.bcp.2007.07.033
Munkonda, M. N., Pelletier, J., Ivanenkov, V. V., Fausther, M., Tremblay, A., Künzli, B., et al. (2009). Characterization of a Monoclonal Antibody as the First Specific Inhibitor of Human NTP Diphosphohydrolase-3: Partial Characterization of the Inhibitory Epitope and Potential Applications. FEBS J. 276 (2), 479–496. doi: 10.1111/j.1742-4658.2008.06797.x
Murtaza, A., Afzal, S., Zaman, G., Saeed, A., Pelletier, J., Sévigny, J., et al. (2021). Divergent Synthesis and Elaboration of Structure Activity Relationship for Quinoline Derivatives as Highly Selective NTPDase Inhibitor. Bioorg Chem. 115, 105240. doi: 10.1016/j.bioorg.2021.105240
Nakaar, V., Beckers, C. J., Polotsky, V., Joiner, K. A. (1998). Basis for Substrate Specificity of the Toxoplasma Gondii Nucleoside Triphosphate Hydrolase. Mol. Biochem. Parasitol. 97 (1-2), 209–220. doi: 10.1016/S0166-6851(98)00153-4
Nakaar, V., Samuel, B. U., Ngo, E. O., Joiner, K. A. (1999). Targeted Reduction of Nucleoside Triphosphate Hydrolase by Antisense RNA Inhibits Toxoplasma Gondii Proliferation. J. Biol. Chem. 274 (8), 5083–5087. doi: 10.1074/jbc.274.8.5083
Novaes, R. D., Santos, E. C., Cupertino, M. C., Bastos, D., Mendonça, A., Marques-da-Silva, E. A., et al. (2018). Purinergic Antagonist Suramin Aggravates Myocarditis and Increases Mortality by Enhancing Parasitism, Inflammation, and Reactive Tissue Damage in Trypanosoma Cruzi-Infected Mice. Oxid. Med. Cell. Longev. 2018, 7385639. doi: 10.1155/2018/7385639
Olivier, M., Gregory, D. J., Forget, G. (2005). Subversion Mechanisms by Which Leishmania Parasites can Escape the Host Immune Response: A Signaling Point of View. Clin. Microbiol. Rev. 18, 293–305. doi: 10.1128/CMR.18.2.293-305.2005
Paes-Vieira, L., Gomes-Vieira, A., Meyer-Fernandes, J. R. (2018). NTPDase Activities: Possible Roles on Leishmania Spp Infectivity and Virulence. Cell Biol. Int. 42, 670–682. doi: 10.1002/cbin.10944
Paes-Vieira, L., Rocco-Machado, N., Freitas-Mesquita, A. L., Dos Santos Emiliano, Y. S., Gomes-Vieira, A. L., de Almeida-Amaral, E. E., et al. (2021). Differential Regulation of E-NTPdases During Leishmania Amazonensis Lifecycle and Effect of Their Overexpression on Parasite Infectivity and Virulence. Parasitol. Int. 85, 102423. doi: 10.1016/j.parint.2021.102423
Paletta-Silva, R., Meyer-Fernandes, J. R. (2012). Adenosine and Immune Imbalance in Visceral Leishmaniasis: The Possible Role of Ectonucleotidases. J. Trop. Med. 2012:650874. doi: 10.1155/2012/650874
Pelletier, J., Agonsanou, H., Delvalle, N., Fausther, M., Salem, M., Gulbransen, B., et al. (2017). Generation and Characterization of Polyclonal and Monoclonal Antibodies to Human NTPDase2 Including a Blocking Antibody. Purinergic Signal. 13 (3), 293–304. doi: 10.1007/s11302-017-9561-8
Pereira, V., Junior, I., da Silveira, L. S., Geraldo, R. B., de F Pinto, P., Teixeira, F. S., et al. (2018). In Vitro and In Vivo Antischistosomal Activities of Chalcones. Chem. Biodivers 15 (12), e1800398. doi: 10.1002/cbdv.201800398
Peres, N. T. A., Cunha, L. C. S., Barbosa, M. L. A., Santos, M. B., Oliveira, F. A., de Jesus, A. M. R., et al. (2018). Infection of Human Macrophages by Leishmania Infantum Is Influenced by Ecto-Nucleotidases. Front. Immunol. 8, 1954. doi: 10.3389/fimmu.2017.01954
Petró-Silveira, B., Rigo, G. V., da Silva Trentin, D., Macedo, A. J., Sauer, E., de Oliveira Alves, E., et al. (2020). Trichomonas Vaginalis NTPDase Inhibited by Lycorine Modulates the Parasite-Neutrophil Interaction. Parasitol. Res. 119 (8), 2587–2595. doi: 10.1007/s00436-020-06739-8
Pimentel, F. G., Bastos, M. S., Santos, R. F., Mariotini-Moura, C., Bressan, G. C., Silva-Junior, A., et al. (2016). The Leishmania-Macrophage Interactions: Role of E-NTPDases and Purinergic Signaling. Macrophage 3, 1143–1155. doi: 10.14800/Macrophage.1143
Pinheiro, C. M., Martins-Duarte, E. S., Ferraro, R. B., Fonseca de Souza, A. L., Gomes, M. T., Lopes, A. H., et al. (2006). Leishmania Amazonensis: Biological and Biochemical Characterization of Ecto-Nucleoside Triphosphate Diphosphohydrolase Activities. Exp. Parasitol. 114 (1), 16–25. doi: 10.1016/j.exppara.2006.02.007
Porcino, G. N., Carvalho-Campos, C., Maia, A. C., Detoni, M. L., Faria-Pinto, P., Coimbra, E. S., et al. (2012). Leishmania (Viannia) Braziliensis Nucleoside Triphosphate Diphosphohydrolase (NTPDase 1): Localization and In Vitro Inhibition of Promastigotes Growth by Polyclonal Antibodies. Exp. Parasitol. 132 (2), 293–299. doi: 10.1016/j.exppara.2012.08.009
Robson, S. C., Sévigny, J., Zimmermann, H. (2006). The E-NTPDase Family of Ectonucleotidases: Structure Function Relationships and Pathophysiological Significance. Purinergic Signal. 2 (2), 409–430. doi: 10.1007/s11302-006-9003-5
Rottenberg, M. E., Masocha, W., Ferella, M., Petitto-Assis, F., Goto, H., Kristensson, K., et al. (2005). Treatment of African Trypanosomiasis With Cordycepin and Adenosine Deaminase Inhibitors in a Mouse Model. J. Infect. Dis. 192 (9), 1658–1665. doi: 10.1086/496896
Rückert, C., Stuepp, C., Gottardi, B., Rosa, J., Cisilotto, J., Borges, F. P., et al. (2009). Steroid Hormones Alter AMP Hydrolysis in Intact Trophozoites of Trichomonas Vaginalis. Parasitol. Res. 105 (6), 1701–1706. doi: 10.1007/s00436-009-1618-3
Rückert, C., Stuepp, C., Gottardi, B., Rosa, J., Cisilotto, J., Borges, F. P., et al. (2010). Trichomonas Vaginalis: Dehydroepiandrosterone Sulfate and 17beta-Estradiol Alter NTPDase Activity and Gene Expression. Exp. Parasitol. 125 (3), 187–195. doi: 10.1016/j.exppara.2010.01.029
Sansom, F. M. (2012). The Role of the NTPDase Enzyme Family in Parasites: What do We Know, and Where to From Here? Parasitology 139, 963–980. doi: 10.1017/S003118201200025X
Sansom, F. M., Ralton, J. E., Sernee, M. F., Cohen, A. M., Hooker, D. J., Hartland, E. L., et al. (2014). Golgi-Located NTPDase1 of Leishmania Major is Required for Lipophosphoglycan Elongation and Normal Lesion Development Whereas Secreted NTPDase2 Is Dispensable for Virulence. PLoS Negl. Trop. Dis. 8, e3402. doi: 10.1371/journal.pntd.0003402
Sansom, F. M., Robson, S. C., Hartland, E. L. (2008). Possible Effects of Microbial Ecto-Nucleoside Triphosphate Diphosphohydrolases on Host-Pathogen Interactions. Microbiol. Mol. Biol. Rev. 72 (4), 765–781. doi: 10.1128/MMBR.00013-08
Santos, R. F., Pôssa, M. A., Bastos, M. S., Guedes, P. M., Almeida, M. R., Demarco, R., et al. (2009). Influence of Ecto-Nucleoside Triphosphate Diphosphohydrolase Activity on Trypanosoma Cruzi Infectivity and Virulence. PLoS Negl. Trop. Dis. 3 (3), e387. doi: 10.1371/journal.pntd.0000387
Savio, L., Coutinho-Silva, R. (2019). Immunomodulatory Effects of P2X7 Receptor in Intracellular Parasite Infections. Curr. Opin. Pharmacol. 47, 53–58. doi: 10.1016/j.coph.2019.02.005
Schachter, J., Delgado, K. V., Barreto-de-Souza, V., Bou-Habib, D. C., Persechini, P. M., Meyer-Fernandes, J. R. (2015). Inhibition of Ecto-ATPase Activities Impair HIV-1 Infection of Macrophages. Immunobiology 220 (5), 589–596. doi: 10.1016/j.imbio.2014.12.004
Silva-Gomes, N. L., Rampazzo, R. C. P., Moreira, C. M. N., Porcino, G. N., Bezerra dos Santos, C. M., Krieger, M. A., et al. (2020). Knocking Down TcNTPDase-1 Gene Reduces In Vitro Infectivity of Trypanosoma Cruzi. Front. Microbiol. 11, 434. doi: 10.3389/fmicb.2020.00434
Souza, R. L., Gonçalves, U. O., Badoco, F. R., de Souza Galvão, L., Santos, R., de Carvalho, P., et al. (2017). Licochalcone A Induces Morphological and Biochemical Alterations in Schistosoma Mansoni Adult Worms. Biomed. Pharmacother. 96, 64–71. doi: 10.1016/j.biopha.2017.09.128
Souza, V. L., Veras, P. S., Welby-Borges, M., Silva, T. M., Leite, B. R., Ferraro, R. B., et al. (2011). Immune and Inflammatory Responses to Leishmania Amazonensis Isolated From Different Clinical Forms of Human Leishmaniasis in CBA Mice. Memorias do Inst Oswaldo Cruz 106 (1), 23–31. doi: 10.1590/s0074-02762011000100004
Tan, F., Hu, X., Pan, C. W., Ding, J. Q., Chen, X. G. (2010). Monoclonal Antibodies Against Nucleoside Triphosphate Hydrolase-II can Reduce the Replication of Toxoplasma Gondii. Parasitol. Int. 59 (2), 141–146. doi: 10.1016/j.parint.2009.12.007
Tasca, T., Bonan, C. D., De Carli, G. A., Sarkis, J. J. (2004). Trichomonas Vaginalis: Cytochemical Localization of a NTPDase1 and an Ecto-5'-Nucleotidase and Effects of Adenine Nucleotides on Cellular Viability. Parasitol. Res. 93 (4), 300–303. doi: 10.1007/s00436-004-1126-4
Tasca, T., Borges, F. P., Bonan, C. D., De Carli, G. A., Battastini, A. M., Sarkis, J. J. (2003). Effects of Metronidazole and Tinidazole on NTPDase1 and Ecto-5'-Nucleotidase From Intact Cells of Trichomonas Vaginalis. FEMS Microbiol. Lett. 226 (2), 379–384. doi: 10.1016/S0378-1097(03)00637-2
Trautmann, A. (2009). Extracellular ATP in the Immune System: More Than Just a "Danger Signal. Sci. Signaling 2 (56), pe6. doi: 10.1126/scisignal.256pe6
Vasconcellos, R., Mariotini-Moura, C., Gomes, R. S., Serafim, T. D., Firmino, R., Silva E Bastos, M., et al. (2014). Leishmania Infantum Ecto-Nucleoside Triphosphate Diphosphohydrolase-2 is an Apyrase Involved in Macrophage Infection and Expressed in Infected Dogs. PLoS Negl. Trop. Dis. 8 (11), e3309. doi: 10.1371/journal.pntd.0003309
Vasconcelos, E. G., Ferreira, S. T., Carvalho, T. M., Souza, W., Kettlun, A. M., Mancilla, M., et al. (1996). Partial Purification and Immunohistochemical Localization of ATP Diphosphohydrolase From Schistosoma Mansoni. Immunological Cross-Reactivities With Potato Apyrase and Toxoplasma Gondii Nucleoside Triphosphate Hydrolase. J. Biol. Chem. 271 (36), 22139–22145. doi: 10.1074/jbc.271.36.22139
Vasconcelos, E. G., Nascimento, P. S., Meirelles, M. N., Verjovski-Almeida, S., Ferreira, S. T. (1993). Characterization and Localization of an ATP-Diphosphohydrolase on the External Surface of the Tegument of Schistosoma Mansoni. Mol. Biochem. Parasitol. 58 (2), 205–214. doi: 10.1016/0166-6851(93)90042-v
Vijayamahantesh, Amit, A., Dikhit, M. R., Mishra, A., Singh, A. K., Das, V. N., et al. (2017). Adenosine Generated by Ectonucleotidases Modulates the Host Immune System During Visceral Leishmaniasis. Cytokine 91, 170–179. doi: 10.1016/j.cyto.2017.01.001
Wilson, J. M., Ross, W. G., Agbai, O. N., Frazier, R., Figler, R. A., Rieger, J., et al. (2009). The A2B Adenosine Receptor Impairs the Maturation and Immunogenicity of Dendritic Cells. J. Immunol. (Baltimore Md.: 1950) 182 (8), 4616–4623. doi: 10.4049/jimmunol.0801279
Wolkmer, P., Pereira, A. B., da Silva, C. B., Paim, F. C., Palma, H. E., Bueno, A., et al. (2019). Curcumin Pre-Treatments Modulate the Activities of Adenine Nucleotide and Nucleoside Degradation Enzymes in Lymphocyte of Rats Infected With Trypanosoma Evansi. Parasitol. Int. 73, 101948. doi: 10.1016/j.parint.2019.101948
Wolkmer, P., Silva, C. B., Paim, F. C., Duarte, M. M., Castro, V., Palma, H. E., et al. (2013). Pre-Treatment With Curcumin Modulates Acetylcholinesterase Activity and Proinflammatory Cytokines in Rats Infected With Trypanosoma Evansi. Parasitol. Int. 62 (2), 144–149. doi: 10.1016/j.parint.2012.11.004
Zhao, H., Bo, C., Kang, Y., Li, H. (2017). What Else can CD39 Tell Us? Front. Immunol. 8, 72. doi: 10.3389/fimmu.2017.0072
Zhong, A. H., Gordon Jiang, Z., Cummings, R. D., Robson, S. C. (2017). Various N-Glycoforms Differentially Upregulate E-NTPDase Activity of the NTPDase3/CD39L3 Ecto-Enzymatic Domain. Purinergic Signal. 13 (4), 601–609. doi: 10.1007/s11302-017-9587-y
Zimmermann, H. (2021a). Ectonucleoside Triphosphate Diphosphohydrolases and Ecto-5’-Nucleotidase in Purinergic Signaling How the Field Developed and Where We are Now. Purinergic Signal. 17, 117–125. doi: 10.1007/s11302-020-09755-6
Zimmermann, H. (2021b). History of Ectonucleotidases and Their Role in Purinergic Signaling. Biochem. Pharmacol. 187, 114322. doi: 10.1016/j.bcp.2020.114322
Keywords: Ecto-nucleotidases, E-NTPDases, purinergic signaling, parasite infection, antiparasitic drugs
Citation: Paes-Vieira L, Gomes-Vieira AL and Meyer-Fernandes JR (2021) E-NTPDases: Possible Roles on Host-Parasite Interactions and Therapeutic Opportunities. Front. Cell. Infect. Microbiol. 11:769922. doi: 10.3389/fcimb.2021.769922
Received: 02 September 2021; Accepted: 25 October 2021;
Published: 09 November 2021.
Edited by:
Martin M. Edreira, Universidad de Buenos Aires, ArgentinaCopyright © 2021 Paes-Vieira, Gomes-Vieira and Meyer-Fernandes. This is an open-access article distributed under the terms of the Creative Commons Attribution License (CC BY). The use, distribution or reproduction in other forums is permitted, provided the original author(s) and the copyright owner(s) are credited and that the original publication in this journal is cited, in accordance with accepted academic practice. No use, distribution or reproduction is permitted which does not comply with these terms.
*Correspondence: José Roberto Meyer-Fernandes, meyer@bioqmed.ufrj.br; Lisvane Paes-Vieira, lisvane@bioqmed.ufrj.br