Trypanosoma cruzi Induces Regulatory B Cell Alterations in Patients With Chronic Chagas Disease
- 1Laboratorio de Inmunología de las Infecciones por Tripanosomátidos (LIIT), Instituto de Investigaciones en Ingeniería Genética y Biología Molecular “Dr. Héctor N. Torres” (INGEBI), Consejo de Investigaciones Científicas y Técnicas (CONICET), Buenos Aires, Argentina
- 2Laboratorio de Biología Molecular de la Enfermedad de Chagas (LabMECh), Instituto de Investigaciones en Ingeniería Genética y Biología Molecular “Dr. Héctor N. Torres” (INGEBI), Consejo de Investigaciones Científicas y Técnicas (CONICET), Buenos Aires, Argentina
- 3Instituto Nacional de Parasitología “Dr. Mario Fatala Chabén”, Buenos Aires, Argentina
- 4Hospital General de Agudos “Dr. Ignacio Pirovano”, Buenos Aires, Argentina
The clinical evolution of patients with chronic Chagas disease (CCD) is mainly associated with an excessive inflammation and a defective immunomodulatory profile caused by the interaction between T. cruzi and the host. Regulatory B (Breg) cells exert immune suppression mostly through IL-10 production (B10 cells), but also through IL-10-independent mechanisms. Previously, we demonstrated that CCD patients with cardiomyopathy show changes in the ex vivo Breg cell phenotypic distribution although maintain IL-10 production capacity. Here, we sought to identify potential alterations on Breg cells upon in vitro stimulation. Isolated B cells from CCD patients with or without cardiomyopathy and non-infected (NI) donors were stimulated with T. cruzi lysate or CpG + CD40L, and characterized by flow cytometry based on the expression of CD24, CD27, CD38, and the regulatory molecules IL-10 and PD-L1. IL-10 and IL-17 secretion in the supernatant of B cells was evaluated by ELISA. Data showed that T. cruzi stimulation diminished the expression of CD24 and CD38 on CD27− B cells while reducing the percentage of CD24high inside CD27+ B cells. Furthermore, T. cruzi induced a regulatory B cell phenotype by increasing B10 cells and IL-10 secretion in all the groups. The innate-like B10 cells expansion observed in patients with cardiomyopathy would be associated with CD27− B10 cell subsets, while no predominant phenotype was found in the other groups. Patients with cardiomyopathy also displayed higher IL-17 secretion levels in T. cruzi–activated B cells. CpG + CD40L stimulation revealed that B cells from CCD patients and NI donors had the same ability to differentiate into B10 cells and secrete IL-10 in vitro. Additionally, CCD patients showed an increased frequency of CD24−CD27− B cells and a reduction in the percentage of CD24highCD27+ Breg cells, which appeared to be inversely correlated with the presence of T. cruzi DNA in blood. Finally, CCD patients exhibited a higher frequency of PD-L1+ B cells in T. cruzi–stimulated samples, suggesting that IL-10-independent mechanisms could also be tangled in the control of inflammation. Altogether, our results provide evidence about the potential role of Breg cells in the immune response developed against T. cruzi and its contribution to chronic Chagas cardiomyopathy.
Introduction
Chagas disease, which etiological agent is the parasite Trypanosoma cruzi, remains affecting about 6 to 7 million people worldwide, mostly in Latin America, but also in the United States of America, Canada, and many European and some African, Eastern Mediterranean, and Western Pacific countries (WHO, 2021). After infection with the parasite, the innate and adaptive immunity elicited by the host leads to rapid control of the acute parasitemia (Cardoso et al., 2016). However, if the infected individuals have no access to the current available antiparasitic drug, Benznidazol, the infection cannot be completely resolved, and the disease progresses to the chronic phase. The clinical features of the chronic Chagas disease (CCD) vary widely. About 70% of patients experience no detectable signs or symptoms and can stay symptom-free for years or even for the entirety of their lifetime, while up to 30% of patients develop cardiac alterations and up to 10% suffer from digestive lesions (particularly enlargement of the esophagus or colon) or both manifestations (cardiac and digestive) (Pérez-Molina and Molina, 2018). The immune response, encompassing all its stimulatory and regulatory mediators, is one of the main factors that tilt the balance between the two clinical forms of CCD (Acevedo et al., 2018; Chevillard et al., 2018).
Immature transitional CD19+CD24highCD38high and CD19+CD24highCD27+ B cells have immunosuppressive capacity and are considered the most representative subsets of the so-called regulatory B (Breg) cells in human peripheral blood (de Masson et al., 2014; Oleinika et al., 2018). CD19+CD24highCD38high B cells inhibit the differentiation of CD4+ T cells into the pro-inflammatory Th1 and Th17 and promote Treg cells (Blair et al., 2010; Flores-Borja et al., 2013). Deficiencies in the number and function of these cells have been first reported in patients with systemic lupus erythematosus (Blair et al., 2010), spreading then to other autoimmune diseases (multiple sclerosis, rheumatoid arthritis, Sjögren’s syndrome), allergic rhinitis, and chronic infections (Abebe et al., 2021). Furthermore, CD19+CD24highCD38high Breg represents a high percentage of the bulk of B lymphocyte in cord and peripheral blood of healthy neonate and was found increased in late-onset sepsis, linking these cells with pregnancy success (Li et al., 2018). On the other hand, CD19+CD24highCD27+ B cell subset harnesses its immunomodulatory activity mostly by regulating the production of pro-inflammatory cytokines, tumor necrosis factor (TNF)-α in monocytes and interferon (IFN)-γ in CD4+ T cells (Iwata et al., 2011; Hasan et al., 2019; Jansen et al., 2021). Alterations in the frequency of this memory-like Breg have been detected in similar scenarios than the CD19+CD24highCD38high Breg, including cancer (Abebe et al., 2021; Jansen et al., 2021).
However, and depending on the different disease models, stimulation conditions as well as the panel of cell surface markers used in the flow cytometry analysis, other B cells are also classified as Breg. Thus, CD19+CD5+CD1d+ (Zhang et al., 2012; Zhang et al., 2014), CD19+Tim-1+ (Aravena et al., 2017; Gu et al., 2017b), and CD19+CD25+CD71+CD73− cells (van de Veen et al., 2013) participate by ameliorating the inflammatory response elicited in different immune-related pathologies (Abebe et al., 2021). Although still under investigation, the immunomodulatory mechanisms exerted by these heterogeneous mix of B cells encompass mainly the secretion of the anti-inflammatory cytokine interleukin (IL)-10, but also IL-35 and transforming growth factor (TGF)-β production, or the expression of the surface molecules, like CD80, CD86, Fas ligand (FasL), programmed death ligand-1 (PD-L1), CD40L, or CD1d, via direct cell–cell interactions (Harris et al., 2000; Rosser and Mauri, 2015; Abebe et al., 2021).
Data on the role of Breg cells in chronic T. cruzi infection in humans are scarce. In a first study, Fares et al. (2013) evaluated the expression of CD5 and CD1d markers and IL-10 production within CD19+ B cells in an ex vivo context. The authors observed that IL-10 production in CD19+CD5+CD1d+ B cells is higher in patients with CCD when compared with non-infected individuals. But remarkably, they also found that the percentage of IL-10 within CD19+CD5−CD1d− subsets is increased in patients with the cardiac form of CCD, facing the conundrum about the other phenotype of B cells with regulatory functions (Fares et al., 2013). Recently, we demonstrated, in an ex vivo study and focusing on CD24, CD27, and CD38 cell surface markers, that the phenotypic profile of B cells is altered in patients with CCD, depending on their clinical status (Girard et al., 2021). Hence, total B cells and transitional CD24highCD38high B cell subpopulation are expanded in CCD patients with cardiac involvement compared to non-infected donors, while the CD24highCD27+ B cell subset remains unchanged. In addition, and although no differences were observed in the frequency of total IL-10-producing B cells (B10) between patients with and without cardiac alterations, the former showed an increased proportion of CD24intCD38int naïve B10 cells and a trend to a lower frequency of CD24highCD38low memory B10 cells. Since naïve and memory B10 cells have different functions in immune-related diseases, we speculate that naïve B10 cells would be involved in the maintenance of the autoimmune disorders raised in cardiac patients. In the same line of finding, we observed that the phenotypic distribution within B10 cells in terms of naïve, transitional, and memory subsets in CCD patients without cardiac involvement displays the same pattern detected in non-infected individuals.
In the present study, we have expanded on those previous results by broadly delving the phenotypic traits of B and B10 cells from CCD patients with or without cardiac alterations and non-infected donors, in response to T. cruzi stimulation. For this purpose, isolated B cells were examined by flow cytometry using the combination of CD19, CD24, CD27, and CD38 cell surface markers, while the secretion of IL-10 and IL-17 was analyzed in the culture supernatant by ELISA. It was also our interest to phenotypically characterize Breg cells under the non-specific stimulation with cytosine-phosphate-guanosine (CpG) oligodeoxynucleotide plus CD40L. Finally, the expression of PD-L1 was explored on B cells as another mediator, in addition to IL-10, for Breg cells function in the context of human chronic Chagas disease.
Materials and Methods
Study Population and Blood Sample Collection
Donors were enrolled either at Instituto Nacional de Parasitología “Dr. Mario Fatala Chabén” or at Hospital General de Agudos “Dr. Ignacio Pirovano,” Buenos Aires, Argentina. T. cruzi infection was diagnosed according to national and international guidelines by indirect immunofluorescence assay (IFI), indirect hemagglutination assay (IHA), and enzyme-linked immunosorbent assay (ELISA) (WHO, 2002). Subjects positive in at least two of these tests were considered to be infected. Patients in the chronic phase of the infection underwent a complete clinical and cardiological examination and were grouped according to a modified version of the Kuschnir classification (Ribeiro et al., 2012) as follows: Group 0, patients without demonstrable cardiac pathology (G0, n=13); and Group 1, patients with cardiac involvement (G1, n=13). Subjects in Group 0 (Kuschnir 0 or K0) had a normal ECG and normal chest radiography, whereas individuals within Group 1 had cardiac alterations such as right and/or left branch blockage and different degrees of conductive functional alterations (Kuschnir 1 or K1, n=12) or heart failure symptoms (Kuschnir 3 or K3, n=1). Due to sample availability reasons, most of the donors included in the G1 group belonged to Kuschnir stage 1. A random group of subjects with negative serological tests for T. cruzi infection were included as the non-infected control group (NI, n=13). The exclusion criteria included record of history of treatment with Benznidazole or Nifurtimox and presence of systemic arterial hypertension, diabetes mellitus, thyroid dysfunction, renal insufficiency, chronic obstructive pulmonary disease, hydroelectrolytic disorders, alcoholism, history suggesting coronary artery obstruction and/or rheumatic disease, as well as the impossibility of undergoing the examinations. The clinical features of the study population are summarized in Table 1. Samples from patients and non-infected donors consisted of 50–60 ml of peripheral venous blood, collected in tubes with EDTA as anticoagulant (Vacutainer, BD Biosciences, NJ, USA). All participants were permanent residents of the greater Buenos Aires area, a location free of Triatoma infestans, for at least the last 10 years.
Blood samples were drawn after the purposes of the investigation were explained, and written informed consent was signed from all participants.
PBMC and Plasma Isolation
An aliquot of 2 ml of whole blood from each participant was separated for parasite load detection, while a similar volume was centrifuged for 15 min at 800 g to separate plasma, which was stored at −20°C for T. cruzi specific antibody analysis. The remaining whole blood underwent Ficoll-Hypaque density gradient centrifugation (GE Healthcare Bio-Sciences AB, Uppsala, Sweden), according to manufacturer-provided instructions to obtain PBMC; this procedure was carried out within 4 h of blood collection. Isolated PBMCs were resuspended in heat-inactivated fetal bovine serum (FBS, Natocor, Córdoba, Argentina) containing 10% dimethylsulfoxide (DMSO) and cryopreserved in multiple aliquots in liquid nitrogen until used. After thawing, obtained PBMCs with viability greater than 85% assessed by light microscopy and trypan blue staining were used in the experiments.
Characterization of Blood Samples
The titer of T. cruzi–specific IgG antibodies in the plasma of patients was determined as previously described (Longhi et al., 2014; Girard et al., 2018). For parasite load detection, total DNA was extracted from whole blood aliquots using the High Pure PCR Template Preparation kit (Roche Diagnostics Corp., IN, USA) following manufacturer-provided instructions, and a multiplex real-time PCR was performed as previously described (Duffy et al., 2013; Ramírez et al., 2015).
Trypanosoma cruzi Antigen Preparation
Whole trypomastigote lysate was obtained from T. cruzi CL Brener (DTU Tc VI) infected VERO cells (multiplicity of infection, MOI 10:1) supernatants as previously described (Acevedo et al., 2017). Briefly, VERO cells were infected in MEM culture medium supplemented with 10% inactivated FBS, 100 U/ml penicillin, 100 µg/ml streptomycin, and 2 mM L-glutamine (all from Gibco, Thermo Fisher Scientific, Waltham, USA). After 1 h of incubation, extracellular parasites were removed by aspirating cell culture medium followed by the addition of fresh MEM with 3% FBS. Trypomastigotes were harvested from day 6 to 7 post-infection in the supernatant of VERO cells by centrifugation at 2,000 g for 10 min and after cellular debris was removed. Trypomastigotes were washed once with phosphate-buffered saline (PBS) and stored as dry pellet at −80˚C until lysate preparation. Successive parasite collection rounds were performed until obtaining a total of 2.109 total trypomastigotes. To prepare whole antigenic lysate, parasites were resuspended in lysis buffer (1 mM EDTA, 0.1% Tritón X-100, 0.1% NP40, 100 mM NaCl, 1 mM PMSF in distilled water) and submitted to five cycles of freezing-thawing in liquid nitrogen, which were followed by sonication. After lysis procedure, the suspension was filter sterilized through a 0.2-mm-pore-size membrane, assayed for protein concentration, aliquoted, and stored at −80°C until use.
B Cell Isolation and Culture
B cells were isolated from thawed PBMC by negative selection using magnetic-bead purification MACS kit (EasySep Human Pan B cell isolation kit; Miltenyi Biotec, Bergisch Gladbach, Germany) and the EasySep Purple Magnet (STEMCELL Technologies, Vancouver, Canada), following the manufacturer-provided protocol. The frequency of CD3−CD19+ cells prior and after magnetic separation was evaluated by FACS staining on a BD FACSCanto II cytometer (BD Biosciences, Franklin Lakes, NJ, USA). The purity of isolated B cells was 91.65 ± 5.58% (mean ± standard deviation). All cultures were performed in RPMI-1640 medium supplemented with 100 U/ml penicillin, 100 µg/ml streptomycin, 2 mM L-glutamine, and 10% FBS. B cells, seeded in 96-well U-bottom plates at a density of 400.000 cells/well, were incubated for 48 h at 37°C in a humidified 5% CO2 incubator, with T. cruzi trypomastigote lysate (10 µg/ml), CpG oligodeoxynucleotide (2.5 μg/ml; CpG-ODN2006-5’-tcgtcgttttgtcgttttgtcgtt-3’; InvivoGen, San Diego, CA, USA) + recombinant human CD40L (1 μg/ml; Biolegend, San Diego, USA), a combination of T. cruzi lysate + CpG + CD40L or culture medium alone as non-stimulated control. For intracellular IL-10 detection, 2 µl/ml of cell stimulation cocktail (eBiosciences, Thermo Fisher Scientific, Waltham, MA, USA) and 1 µl/ml of Brefeldin A (equivalent to 5 µg/ml; Biolegend), formally called PIB, were added for the last 5 h of incubation. Of note, cell stimulation cocktail contains Phorbol-12-myristate-13-acetate (PMA; 0.05 µg/ml) and ionomycin (Iono; 1 µg/ml).
Flow Cytometry Staining
B cells were transferred to a 96-well V-bottom plate and washed once with PBS by centrifugation at 700 g for 3 min at room temperature (RT). Supernatants were discarded, and cells were incubated for 30 min with a staining solution containing BV421 anti-CD3 (clone UCHT1), PECy5 anti-CD19 (clone HIB19), APC anti-CD27 (clone M-T271), PECy7 anti-CD24 (clone ML5), APCCy7 anti-CD38 (clone HB-7), and FITC anti-PD-L1 (clone MIH1) fluorochrome-conjugated antibodies (Abs) or isotype control Abs specific for human. After surface markers staining, cells were washed with PBS, fixed with Fixation Buffer (Biolegend) for 20 min at RT in the dark, and washed with PBS by centrifugation. For intracellular IL-10 detection, cells were permeabilized with Perm-Wash Buffer (Biolegend), stained with PE anti-IL-10 antibody (clone JES3-9D7) and fixed again for 20 min with Fixation Buffer. Zombie-Aqua Fixable viability dye (Biolegend) was used to exclude non-viable cell events. Fluorescence-minus-one (FMO) controls were used to determine the cut point for the IL-10 staining, and isotype-matched Ab control staining was considered for cell surface markers gate setting. All Abs were purchased from Biolegend and BD Biosciences and were used at optimal concentrations determined by previous titration experiments. A minimum of 20.000 events within the B cell population were acquired in a FACSCanto II flow cytometer using FACS Diva Software (BD Biosciences). Data analysis was performed with FlowJo Software V10 (FlowJo LLC, Ashland, OR, USA). The general gating strategy is illustrated in Supplementary Figure 1.
Cytokine Secretion
IL-10 and IL-17 secretion was measured in culture supernatants of B cells by ELISA (ELISA Max Standard Set Human IL-10, Biolegend and Human IL-17A ELISA Ready-SET-Go!, eBiosciences, San Diego, CA, USA), according to the manufacturer’s instructions. The supernatants were collected after 43 h of culture and before PIB addition and stored at −80°C until the ELISA assay was performed.
Statistical Analysis
A linear mixed-effects regression model (LMER) was applied to analyze differences in the frequency of cell populations, the mean fluorescence intensity (MFI) of markers, and the cytokine concentration among different stimulation conditions and groups. Group and culture conditions were considered fixed factors, sample was included as random factor, while the frequency of cells and the MFI or cytokine concentration were set as dependent variables. For post-hoc comparisons, Tukey HSD (honest significant difference) contrasts were used. The frequency of cells, MFI, and cytokine concentration data were tested for normality and homoscedasticity using Shapiro-Wilk and Bartlett tests, respectively. LMER models were fitted in R version 4.0.2 (R Core Team, 2013) with lmer function from lme4 package (Bates et al., 2010) and glht function from multcomp package (Hothorn et al., 2014). p-value less than 0.05 was considered statistically significant.
Results
The Phenotype of Breg Cells Changes Upon In Vitro Stimulation With T. cruzi in Patients With Chronic Chagas Disease
Aiming to characterize Breg cell compartment in patients with CCD with different clinical forms and NI donors following T. cruzi stimulation, B cells isolated from PBMC were incubated with T. cruzi lysate or medium only (control) during 48 h with the addition of PIB in the last 5 h of culture, and then applied flow cytometry to evaluate the expression of CD24, CD27, and CD38 cell surface markers. By using the combination of CD24 and CD38, we assessed the well-established subpopulations of B cells, identified as CD24highCD38high (immature transitional), CD24intCD38int (primarily mature naïve), CD24highCD38low (primarily memory), and CD24lowCD38high (plasmablasts) (Figure 1A). Results showed that T. cruzi lysate stimulation induced a decrease in the frequency of immature transitional B cells in patients without cardiac involvement (p=0.002), although no statistically significant differences were found among the groups (Figure 1B). The rest of the subpopulations stayed unchanged under these conditions and was comparable among the groups (Figure 1B).
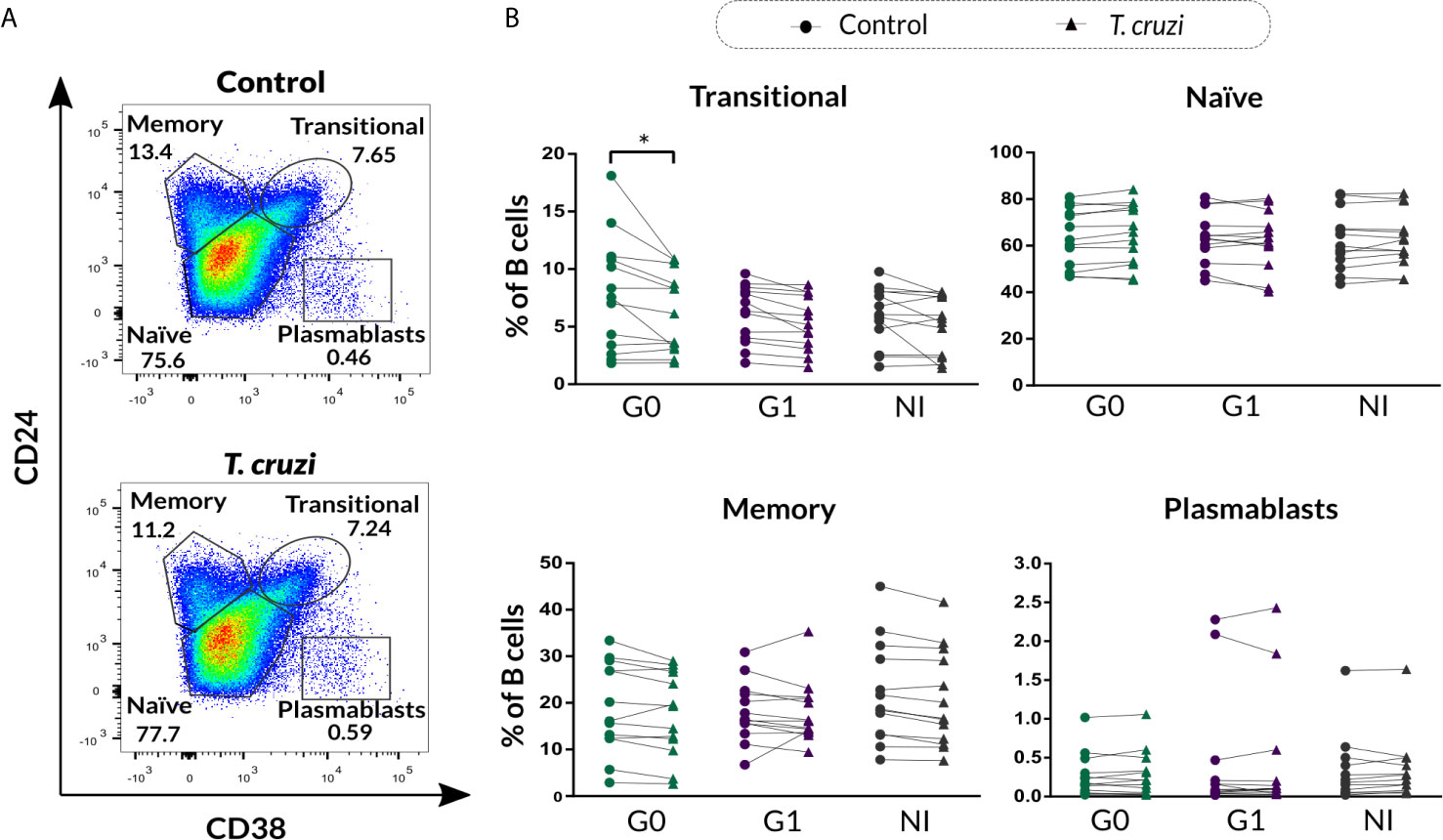
Figure 1 Phenotypic distribution based on CD24 and CD38 expression of isolated B cells from patients with CCD and NI donors upon T. cruzi stimulation. (A) Gating strategy used to identify B cell subsets according to CD24 and CD38 expression. Dot plots from one NI donor are illustrated. (B) Frequency of transitional, naïve, memory, and plasmablasts subsets in Control or T. cruzi stimulated B cells from CCD patients (G0, G1) and NI donors. Each symbol represents data from one individual. Statistically significant differences are indicated with an asterisk (*) p < 0.05.
We further evaluated the percentage of B cell subsets according to CD24-CD27 (Figure 2A) and CD27-CD38 (Figure 2B) markers expression upon T. cruzi stimulation. Considering the former combined expression, patients with CCD with and without cardiac involvement showed a minor frequency of CD24+CD27− B cells in T. cruzi–stimulated cultures, compared to NI donors (G0: p=0.025, G1: p<0.001; Figure 2C). The frequency of CD24−CD27− subset increased in response to T. cruzi antigens in subjects from the three groups (p<0.001), whereas only patients without cardiac involvement showed an expansion of CD24−CD27+ B cell subset (p=0.0037, Figure 2C). Interestingly, the percentage of CD24highCD27+ B cells was lower in G0 and G1 groups (G0 vs. NI: p=0.02, G1 vs. NI: p=0.014; Figure 2C), and the magnitude of this subset was found diminished in those patients with detectable T. cruzi DNA in peripheral blood (PCR+), independently of their clinical form (p=0.007). Conversely, CD24−CD27− B cell frequency was higher in G0 and G1 groups (G0 vs. NI: p=0.032, G1 vs. NI p=0.02; Figure 2C). Of note, CD24−CD27− and CD24highCD27+ B cell alterations in CCD patients were observed independently of stimulation condition (Figure 2C). Concerning the percentage of CD24+CD27+ B cell subset, no statistically significant differences were detected neither upon T. cruzi stimulation nor comparing among the groups (Figure 2C). Then, we examined the frequencies of B cell subsets based on CD27-CD38 expression. A reduced frequency of the double negative population (CD27−CD38−) was identified in T. cruzi–stimulated B cells from patients with CCD and NI donors (G0: p=0.049, G1: p=0.011, NI: p=0.0044), with no differences among the groups (Figure 2D). Besides, the percentages of CD27+CD38−, CD27+CD38+, and CD27−CD38+ B cell subsets did not change after in vitro stimulation with T. cruzi, and no statistically significant differences were detected by comparing the groups (Figure 2D).
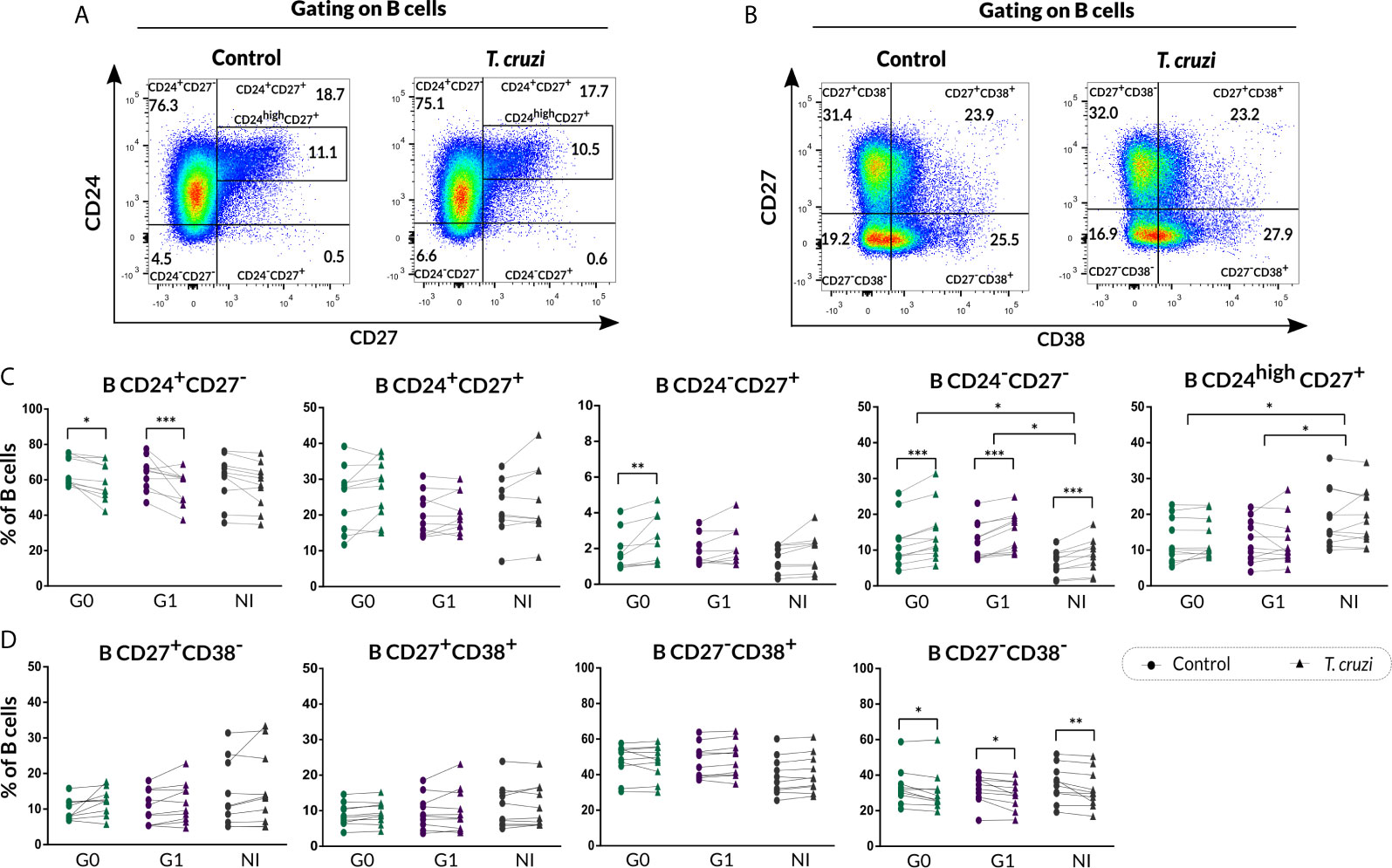
Figure 2 Phenotypic distribution based on CD24-CD27 and CD27-CD38 markers expression of isolated B cells from CCD patients and NI donors upon T. cruzi stimulation. (A, B) Dot plots showing the gating strategy used to identify B cell subsets defined by CD24-CD27 and CD27-CD38 expression, respectively. (C, D) Frequencies of B cell subpopulations in CCD patients (G0, G1) and NI donors in Control or T. cruzi–stimulated samples, according to CD24-CD27 and CD27-CD38 markers expression, respectively. Each symbol represents data from one individual. Statistically significant differences are indicated with asterisks (*) p < 0.05, (**) p < 0.01, (***) p < 0.001.
The alterations of Breg cells unveiled upon unspecific stimulation in patients with some autoimmune diseases and cardiomyopathies (Bankó et al., 2017; Jiao et al., 2018) led us to examine the phenotypic distribution of B cells after stimulation with the oligonucleotide CpG plus recombinant CD40L (CpG + CD40L) in our cohort of CCD patients and NI donors (Supplementary Figures 2A, B, 3A–D). The change induced by CpG + CD40L stimulus was almost equivalent all across the B cell subpopulations in the three groups of subjects; an increase of plasmablasts and a reduction of CD24highCD27+ subpopulation were detected in NI donors (Supplementary Figures 2B, 3C). Furthermore, the most substantial differences were found in the augmentation of CD24+CD27+ in G0 and NI, together with a drop in the frequency of and CD27−CD38+ B cells in CCD patients with cardiomyopathy (Supplementary Figures 3C, D).
Trypanosoma cruzi Induces an Innate-Like Regulatory B10-Cell Phenotype in CCD Patients With Different Clinical Forms and NI Donors
We next characterized the B10 cell population from isolated B cell stimulated with T. cruzi or with the B10 cell differentiation-inducing molecules CpG + CD40L, in CCD patients and NI donors. As was illustrated in Figure 3A, FMO controls were used to determine IL-10+ B cells in each sample. This analysis revealed that T. cruzi stimulation increased total B10-cell frequency in both groups of CCD patients and NI donors (p<0.001; Figure 3B), while no statistical differences were found among the groups. Interestingly, the mean fluorescence intensity (MFI) for IL-10 in B10 cells significantly raised in patients without cardiac involvement (p<0.001; Figure 3C). In parallel, CpG + CD40L stimulation induced a great expansion not only in the frequency of B10 cells but also in MFI compared with culture medium only (p<0.0001; Figures 3D, E), suggesting that B cells from patients with CCD and NI donors have the same ability to differentiate into B10 cells in vitro (Figures 3D, E).
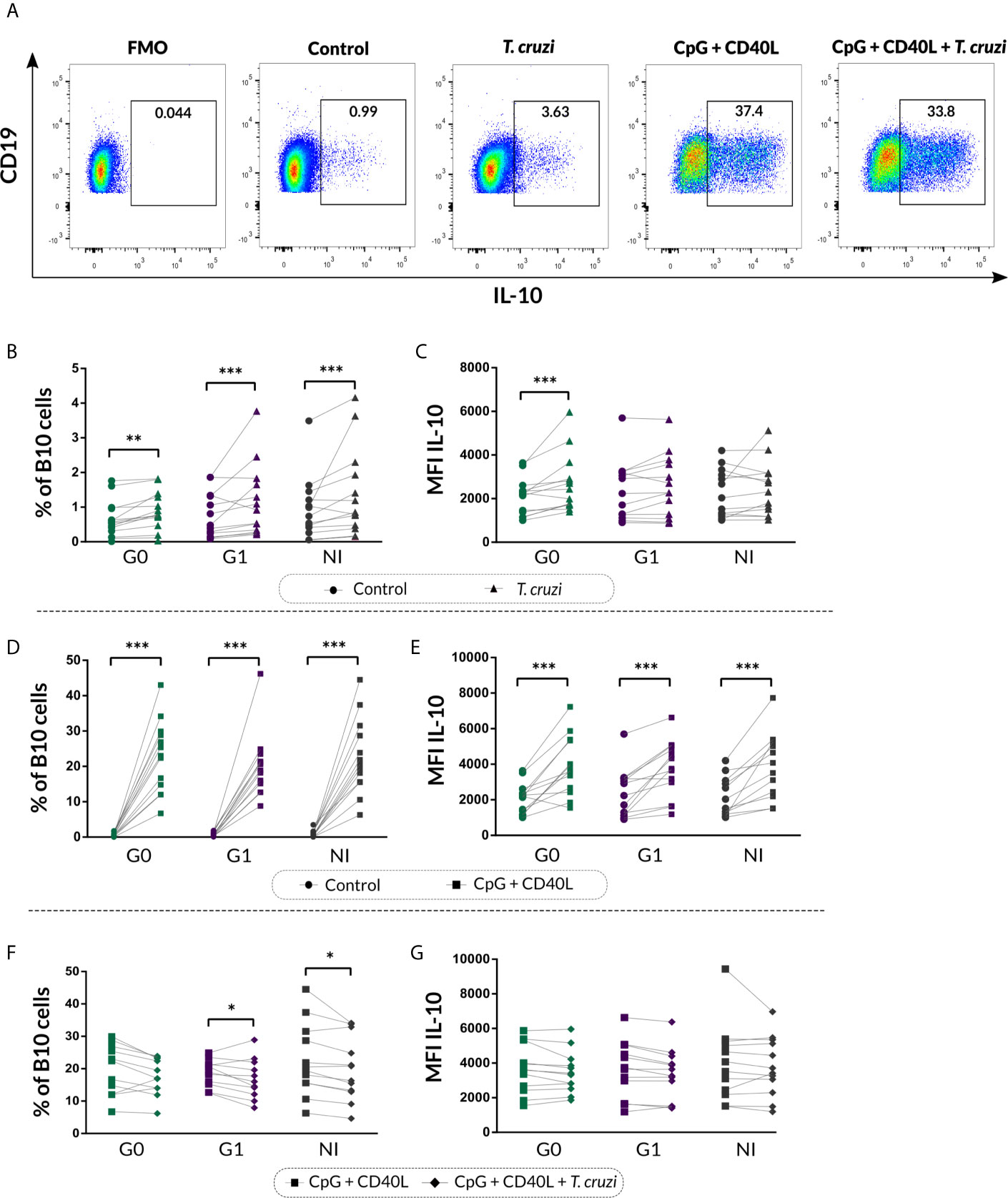
Figure 3 Frequency of B10 cells and MFI of IL-10 on isolated B cells from patients with CCD and NI donors upon stimulation with T. cruzi or CpG + CD40L. (A) Dot plots from one NI donor showing the gating strategy used to determine the frequency of total B10 cells on B cell cultures following different stimulus. Fluorescence-minus-one (FMO) controls for each donor were used to determine the cutoff for IL-10 staining on B cells. (B, C) Frequency of B10 cells and MFI for IL-10 on Control B cells or T. cruzi–stimulated samples. (D, E) Frequency of B10 cells and MFI for IL-10 on Control B cells or CpG + CD40L stimulated samples. (F, G) Frequency of B10 cells and MFI for IL-10 on B cells stimulated with CpG + CD40L or CpG + CD40L + T. cruzi. Each symbol represents data from one individual. Statistically significant differences are indicated with asterisks (*) p < 0.05, (**) p < 0.01, (***) p < 0.001.
We therefore asked whether T. cruzi could alter the B cell activation exerted by the non-specific stimulus. To address this question, B10 cell frequency and MFI for IL-10 were evaluated in B cells incubated with CpG + CD40L + T. cruzi and compared with CpG + CD40L–stimulated samples. Results showed that the percentage of B10 cell diminished in CpG + CD40L + T. cruzi–stimulated B cells in patients with cardiac involvement and NI donors (G1: p=0.024, NI: p=0.017; Figure 3F), although no differences were detected in patients belonging to G0. IL-10 MFI levels were comparable between both conditions and also among the groups (Figure 3G).
In order to investigate the phenotypic distribution of B10 cells based on CD24 and CD38 markers, the gating strategy shown in Figure 4A was applied to analyze the IL-10 secreting B cells within transitional, naïve, memory, and plasmablast subsets in control and T. cruzi–stimulated cultures. A significant drop in the percentage of transitional B10 cells (IL-10+CD24highCD38high) was detected only in subjects of the G0 group (p=0.010), and no differences were detected among the groups (Figure 4B). With regard to naïve, memory, and plasmablasts B10 subsets frequency, no statistically significant differences were found in response to T. cruzi stimulation (Figure 4B).
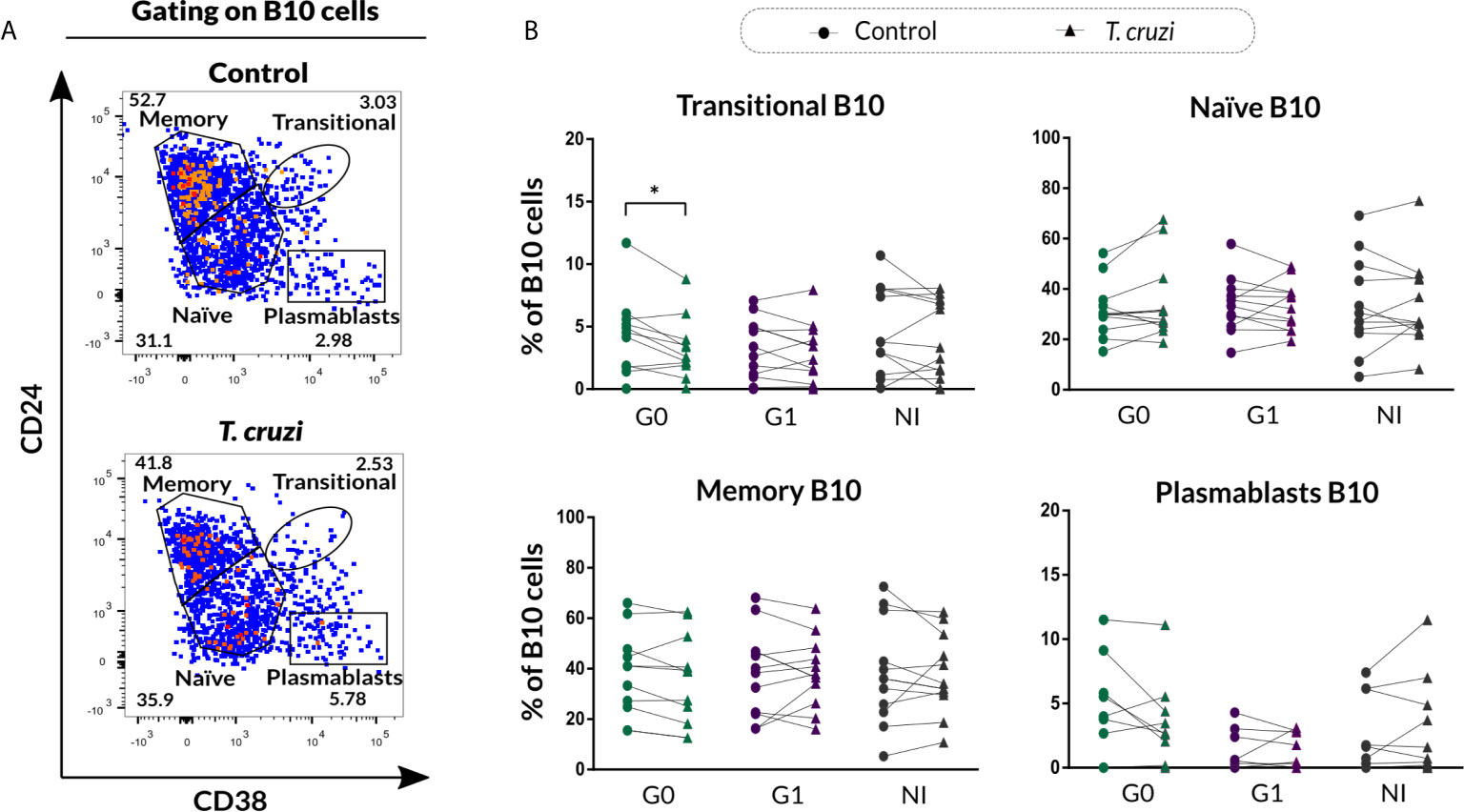
Figure 4 Phenotypic distribution of B10 cells based on CD24 and CD38 expression from patients with CCD and NI donors upon T. cruzi stimulation. (A) Dot plots showing the gating strategy used to identify B10 cell subsets according to CD24 and CD38 expression for one NI donor. Large dots were used to facilitate visibility of events. (B) Frequency of transitional, naïve, memory, and plasmablasts B10 cell subsets in Control or T. cruzi stimulated B cells from CCD patients (G0, G1) and NI donors. Each symbol represents data from one individual. Statistically significant differences are indicated with an asterisk (*) p < 0.05.
The phenotypic traits of B10 cells were also evaluated according to CD24-CD27 (Figure 5A) and CD27-CD38 (Figure 5B) markers expression in T. cruzi–stimulated B cells. The analysis showed a higher frequency of CD24−CD27− B10 cells in patients with cardiac involvement (p=0.013), while no additional differences were identified in the other phenotypic CD24-CD27 subpopulations (Figure 5C). Furthermore, patients of the G1 group displayed a diminished percentage of CD27+CD38+ B10 subset (p=0.027) and an augmented percentage of CD27−CD38+ B10 subset (p=0.023) in T. cruzi–stimulated samples (Figure 5D). Additionally, CD38 negative B10 cell subsets were not altered between conditions and neither among the groups (Figure 5D).
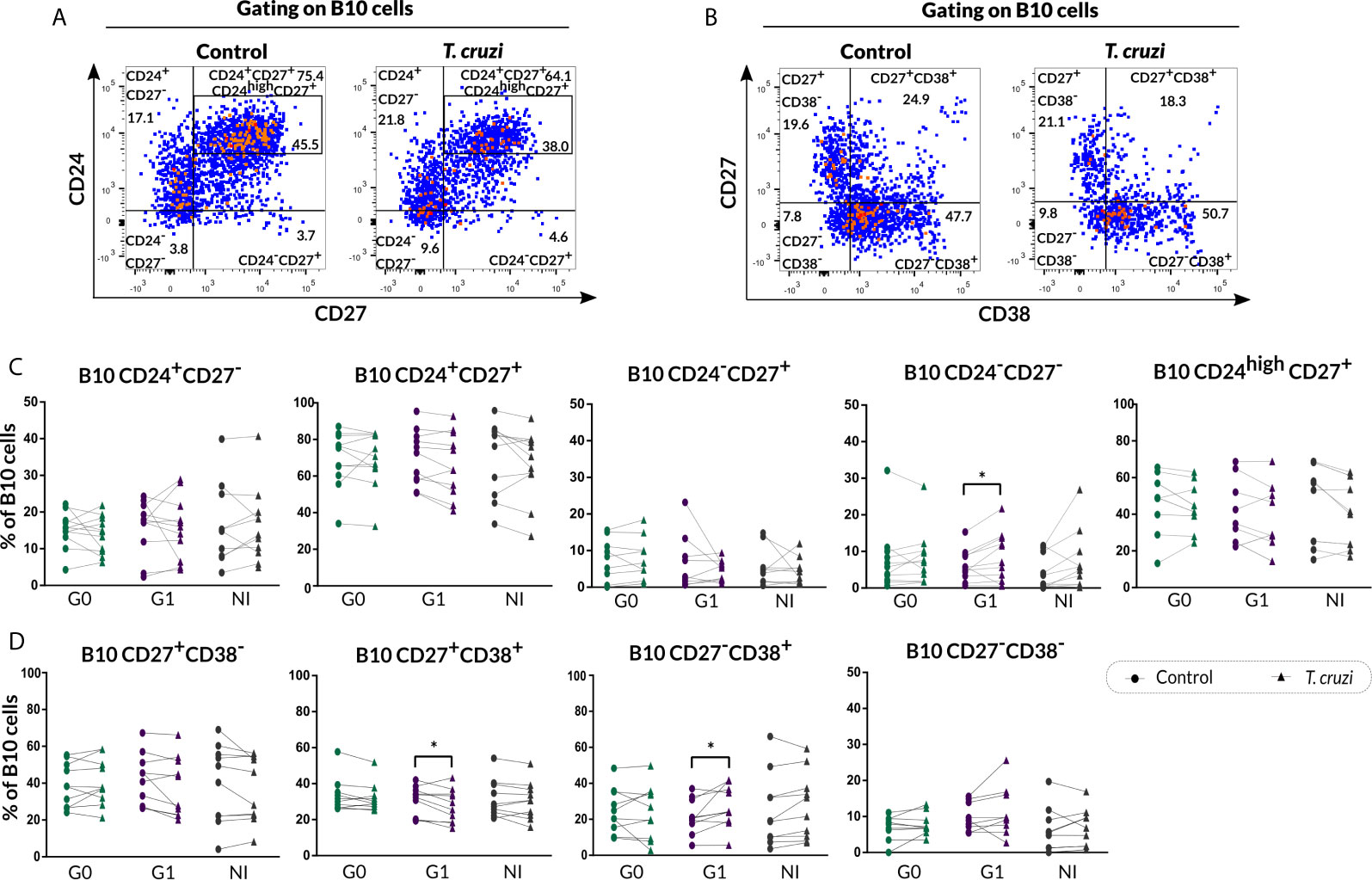
Figure 5 Phenotypic distribution of B10 cells in terms of CD24-CD27 and CD27-CD38 markers expression from CCD patients and NI donors upon T. cruzi stimulation. (A, B) Dot plots corresponding to one NI donor showing the gating strategy used to identify B cell subsets defined by CD24-CD27 and CD27-CD38 expression, respectively. (C, D) Frequencies of B cell subpopulations from CCD patients (G0, G1) and NI donors in Control or T. cruzi–stimulated samples, according to CD24-CD27 and CD27-CD38 markers expression, respectively. Each symbol represents data from one individual. Statistically significant differences are indicated with asterisks (*) p < 0.05.
Similar to the analysis carried out in B cells, we sought to study B10 cell subsets upon CpG + CD40L stimulation (Supplementary Figures 4A, B, 5A–D). Our results showed comparable changes in the frequencies of the distinct phenotypes except for the CD27−CD38+ subpopulation, which was augmented in CCD patients with no significant differences in NI group donors (Supplementary Figure 5D).
The Secretion of IL-17 Increases in B Cells From Patients With the Cardiac Form of CCD
IL-10 and IL-17 secretion in the supernatants of stimulated B cell cultures was quantified by ELISA. Results showed that T. cruzi increased IL-10 secretion in CCD patients and NI donors (p<0.001; Figure 6A), and no differences were found among the groups. Moreover, patients with the cardiac form exhibited higher IL-17 secretion in the supernatant of T. cruzi–stimulated B cells in contrast to controls (p<0.001), while patients without cardiac involvement and NI donors did not show statistically significant differences (Figure 6B). When comparing among the groups, statistically significant differences were found only between patients with cardiomyopathy and NI individuals (p=0.031; Figure 6B).
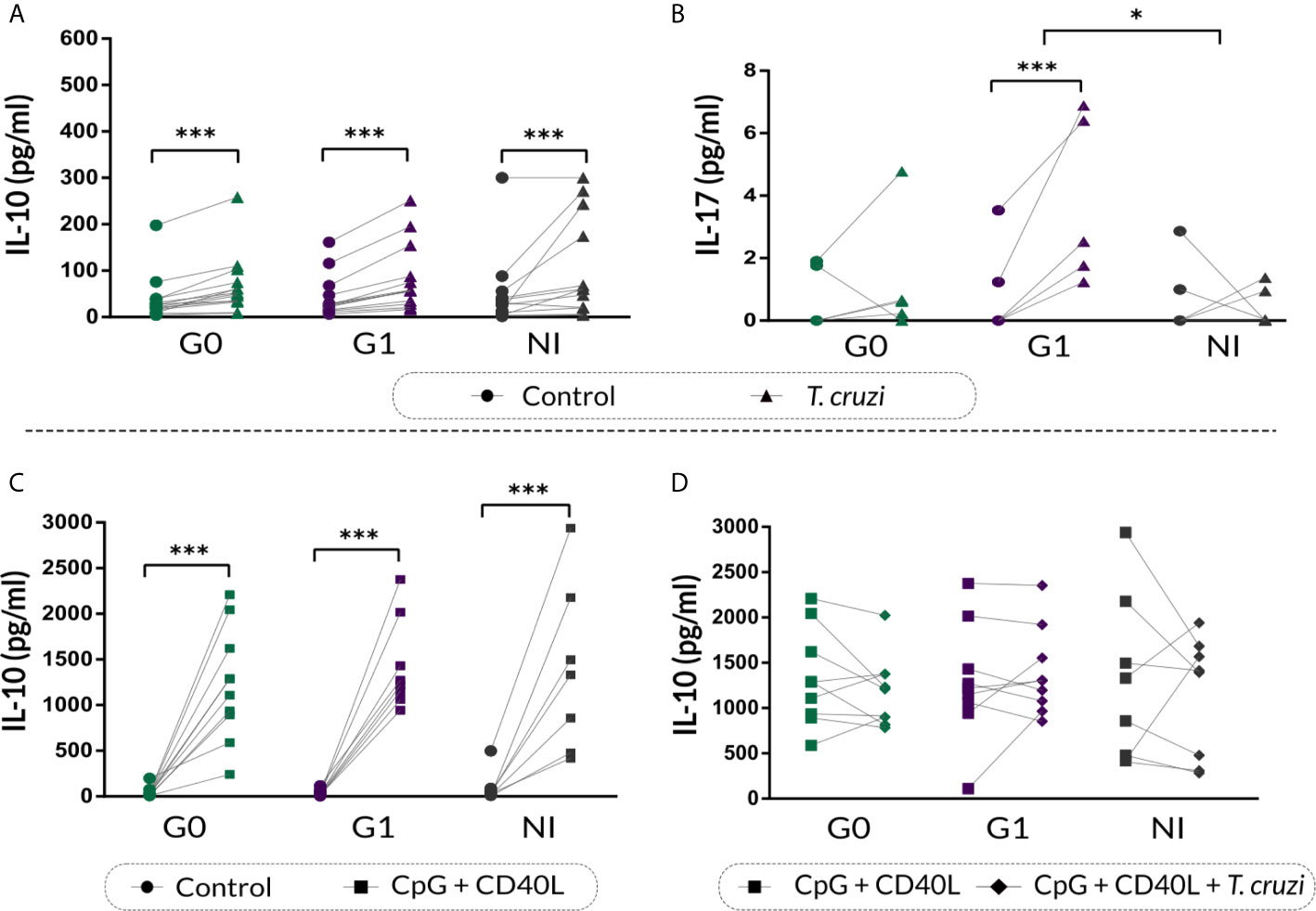
Figure 6 IL-10 and IL-17 cytokine secretion on supernatant of B cells from patients with CCD and NI donors following T. cruzi or CpG + CD40L stimulation. (A, B) IL-10 and IL-17 secretion in supernatants of Control or T. cruzi–stimulated B cells from CCD patients (G0, G1) and NI donors. (C) IL-10 secretion in supernatants of Control or CpG + CD40L stimulated B cells from CCD patients and NI donors. (D) IL-10 secretion in supernatants of B cells following stimulation with CpG + CD40L or CpG + CD40L + T. cruzi in CCD patients and NI donors. Each symbol represents data from one individual. Statistically significant differences are indicated with asterisks (*) p < 0.05, (***) p < 0.001.
We further assessed IL-10 secretion in the supernatant of B cells incubated with CpG + CD40L, and similar increased levels were detected in all the groups (p<0.001; Figure 6C). However, this augmentation was slightly higher in patients with cardiac involvement (mean percentage of increase, G0: 2930%, G1: 4340%, NI: 1533%). On the other hand, none of the groups showed changes in IL-10 secretion upon CpG + CD40L + T. cruzi stimulation compared with CpG + CD40L (Figure 6D).
B Cells From Patients With CCD Exhibit Higher Levels of the Regulatory Molecule PD-L1 Upon T. cruzi Stimulation
Given that B cells can modulate the immune response through IL-10-independent mechanisms, such as PD-L1 regulatory molecule expression (Khan et al., 2015), we decided to measure PD-L1 expression on B cells from CCD patients and NI donors following the same stimulation conditions mentioned above. In Figure 7A, fluorescence histograms from one representative NI donor show the analysis strategy used to evaluate PD-L1 expression on B cells in control cultures or upon T. cruzi, CpG + CD40L, and CpG + CD40L + T. cruzi stimulation. Results evidenced an increase in the percentage of PD-L1+ B cells in CCD patients with different clinical forms upon T. cruzi stimulation (G0: p=0.032, G1: p=0.0029; Figure 7B), but no differences were detected in MFI for PD-L1 on B cells (Figure 7C).
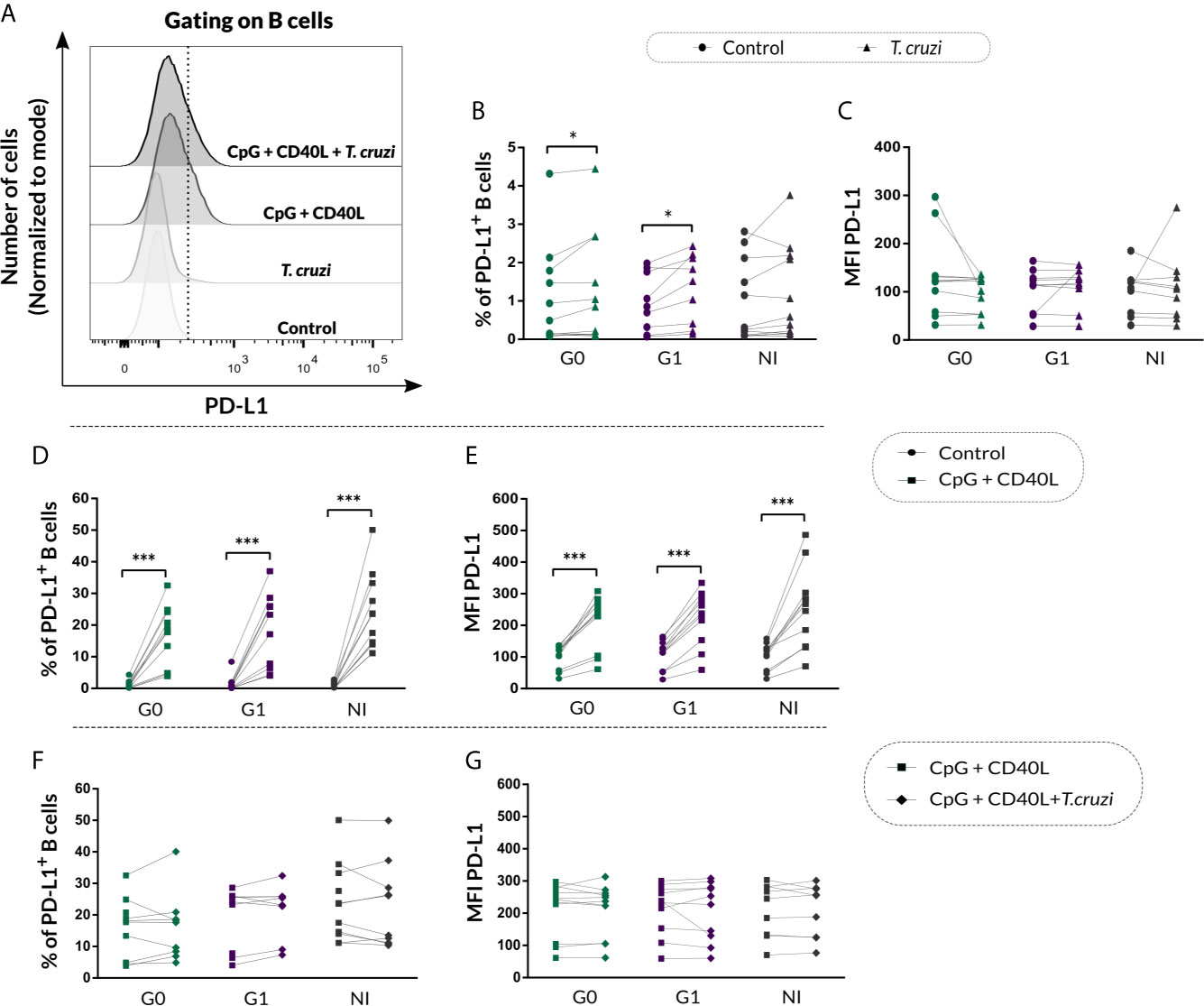
Figure 7 PD-L1 expression on isolated B cells from patients with CCD and NI donors following T. cruzi or CpG + CD40L stimulation. (A) Fluorescence histograms showing PD-L1 expression in T. cruzi, CpG + CD40L, or CpG + CD40L + T. cruzi–stimulated or Control B cells. Data from one NI donor are illustrated. Dotted line shows the cut point to identify PD-L1+ B cells (CD19+PD-L1+). (B, C) Frequency of PD-L1+ B cells and MFI for PD-L1 on Control or T. cruzi–stimulated B cells from CCD patients (G0, G1) and NI donors. (D, E) Frequency of PD-L1+ B cells and MFI for PD-L1 on Control or CpG + CD40L–stimulated B cells from CCD patients (G0, G1) and NI donors. (F, G) Frequency of PD-L1+ B cells and MFI for PD-L1 on CpG + CD40L or CpG + CD40L + T. cruzi–stimulated B cells from CCD patients (G0, G1) and NI donors. Each symbol represents data from one individual. Statistically significant differences are indicated with asterisks (*) p < 0.05, (***) p < 0.001.
In addition, both, the frequency of PD-L1+ B cells (p<0.001; Figure 7D) and MFI for this marker (p<0.0001; Figure 7E) increased in response to the non-specific stimulation with CpG + CD40L in CCD patients and NI donors, and this augmentation remained unchanged in the presence of T. cruzi lysate for all the groups (Figures 7F, G).
Discussion
The balance between effector and regulatory functions of the different immune cells is pivotal for maintaining a homeostatic state both in health and in an ongoing infection. Alterations in the phenotype or function of Breg cells contribute to the pathogenesis of immune-mediated diseases. In this work, we evaluated potential phenotypic alterations of Breg cells from patients with CCD with or without cardiomyopathy, as well as from non-infected donors in response to T. cruzi stimulation.
The results of our approach showed that T. cruzi stimulation alters the expression of surface markers associated with regulatory phenotypes such as CD24, CD27, and CD38 on isolated B cells from patients with CCD and non-infected donors. Hence, T. cruzi reduced the expression of CD24 in CD27− B cells in CCD patients and NI donors, and also in CD27+ B cell population but only in patients without cardiac manifestations. CD24 is a highly glycosylated protein located in the plasma membrane of B cells, usually as glycosyl-phosphatidylinositol (GPI)-anchored protein or forming part of lipid complexes (lipid rafts) (Mensah et al., 2018). In mature human B cells, the expression of CD24 fluctuates throughout their life cycle until it is lost when they differentiate into plasma cells. Sanz et al. (2008) identified a high expression of CD24 in CD27+ B cells, while CD27− presented a low expression of this molecule. In addition, it was shown that CD24 expression level is related to the energy metabolism of B cells throughout their differentiation, and in turn, this role differs among different subpopulations of B cells. In vitro assays revealed that CD24− B cells proliferate more actively, while CD24+ B cells are more likely to remain in an anergic state or die by apoptosis upon stimulation (Mensah et al., 2018). In face of our results, we hypothesize that parasite antigens would be contributing to the predominance of naive and memory CD24− B cells, more prone to proliferate when stimulated and less able to persist in a state of anergy or undergo apoptosis when faced with signals from the environment. The meaning of this mechanism should be explored in order to understand whether it has a protective or detrimental role in B cell response to T. cruzi infection.
Interestingly, an increased frequency of CD24-CD27 double negative B cell subset and a decreased proportion of CD24highCD27+ B cells were detected in patients with CCD compared with non-infected subjects regardless of the culture condition (control or T. cruzi stimulated). A recent study evidenced that CD24highCD38high and CD24highCD27+ populations, contrary to the CD24−CD27− B cell subset, can suppress proliferation and secretion of IFN-γ and IL-17 in CD4+ T cells. Furthermore, this ability depends on the production of IL-10 and the regulatory molecule PD-L1 (Hasan et al., 2019). In line with our findings, the minor percentage detected of CD24highCD27+ subset seems to be a trait that was not related with T. cruzi stimulation on B cells directly; however, it was associated with CCD. Conversely, the increase in the CD24−CD27− subset was directly associated with T. cruzi antigen stimulation, since it was found in cells from CCD patients, who already had a higher frequency, and also in B cells from non-infected donors. We could speculate that in chronic T. cruzi infection, CD24−CD27− B cell subset would be favored in detriment of populations with high expression of both markers, thus contributing to the Breg population being defective in CCD. We also found a negative association between the frequency of the CD24highCD27+ subset and the level of parasitemia in CCD patients, which suggest that these cells could be related to the control of parasite clearance in the chronic phase of Chagas disease.
CD24highCD27+ Breg cell population is characterized by a high expression of the suppressive cytokine IL-10, but also by other regulatory molecules such as PD-L1, TGF-β1, Granzyme B, as well as the CD39 ectonucleotidase (Hasan et al., 2019), which is also expressed in Treg cells (Gu et al., 2017a), in suppressor cells of myeloid origin (Li et al., 2017), as well as in other Breg cell populations (Kaku et al., 2014; Figueiró et al., 2016). Several studies have reported that adenosine converted from ATP by the combined activity of CD39 and CD73 ectoenzymes is a newly recognized “immune checkpoint mediator” that suppresses the immune response in various pathologies (Antonioli et al., 2013). In the context of Chagas disease, Santos et al. (2009) demonstrated that one of the mechanisms by which T. cruzi can evade the immune response is through the expression of ectonucleotidases of the CD39 family, since inhibitors of this enzyme favor infectivity and virulence of the parasite in in vitro and in vivo conditions. Even more, a transient increase in CD39/CD73 attenuated the inflammatory macrophage response against T. cruzi, and CD73 inhibition was beneficial in establishing the inflammatory profile of macrophages in the myocardium of mice with acute T. cruzi infection (Ponce et al., 2016). This finding emphasizes the role of these molecules in modulating the local immune response in the myocardium. Likewise, a recent study showed that Treg cells from patients with the indeterminate form of CCD express higher levels of CD39, indicating that this molecule would be involved in the control of inflammation in these patients (Damasio et al., 2019). In the same way that T. cruzi expresses molecules of the CD39 family that modulate the immune response, it could also induce an increase in the expression of these ectonucleotidases on B cells or decrease the subsets that typically express high levels of these enzymes, such as the CD24highCD27+ B cell population, thus favoring persistence and contributing to disease progression.
By analyzing IL-10-producing B cells, we found that T. cruzi increased the frequency of total B10 cells and IL-10 secretion in the culture supernatant from CCD patients and NI subjects. These findings suggest that T. cruzi induces a B10 cell regulatory phenotype that does not depend on antigen experience, but constitutes an “innate-like” B cell response to relatively short stimulations (48 h) with parasite antigens. In the same line of thought and given that the experiments were carried out using isolated B cells, IL-10 production would be induced by signaling through a direct engagement of Toll-like receptors (TLR) and/or B cell receptor (BCR) with parasite molecules or through the secretion of autocrine cytokines. It is known that not only TLR agonists such as CpG (TLR9) and R848 (TLR7 and TLR8) are capable of inducing IL-10 production in B cells, but also IFN-α modulates IL-10 production mediated by activation of TLR7 and TLR8. Particularly, Liu et al. (2014) reported that IFN-α regulates the production of IL-10 in B cells through signaling via ERK and STAT3. Upon T. cruzi infection, the parasite is internalized by macrophages and dendritic cells by different mechanisms, and once inside the phagolysosome, its DNA and RNA contact the TLR7 and TLR9 receptors. This interaction promotes the activation of NF-kβ through the Myd88 signaling pathway (Bartholomeu et al., 2008). As with these other APCs, the activation of TLR7 and TLR9 could occur in B cells by the different antigens contained in T. cruzi lysate, promoting the production of IL-10. It is also important to consider that in B cells there is a constant mechanism of cross-talk between TLR7 and TLR9 activation and direct signaling through BCR stimulation. In fact, this joint signaling is essential in the development of self-reactivity mechanisms, promoting or decreasing the activation of potentially harmful B cells (Suthers and Sarantopoulos, 2017). When excessive cellular or tissue damage occurs, the activation of TLR7 or TLR9 by endogenous nucleic acids can be exacerbated, leading to autoimmunity phenomena (Suthers and Sarantopoulos, 2017). In CCD, TLR-BCR signaling mediated by certain T. cruzi antigens as well as endogenous antigen products of the immune response itself could also be contributing to the development of autoreactive mechanisms in patients, as well as to activation of potentially harmful or dysfunctional B10 cells.
By reducing the innate and adaptive immune responses, IL-10-mediated suppression can contribute to favorable conditions for the persistence of pathogens and lead to chronic infection. On the other hand, it prevents the immune-mediated tissue lesions as a result of an exacerbated inflammatory state (Mege et al., 2006; Couper et al., 2008). In both the acute and chronic phases of the disease, T. cruzi induces an increase of IL-10 as part of its escape mechanism, which makes it difficult to interpret whether the secretion of this cytokine is a cause or a consequence of parasitemia in patients (Flávia Nardy et al., 2015; Cardoso et al., 2016). A recent study showed a positive relationship between the IL-10 levels in sera of CCD patients with detectable parasitemia (PCR+) (Salvador et al., 2020). However, in our cohort of patients, we did not find an association between parasitemia and the frequency of B10 cells or IL-10 secretion levels in B cell culture supernatants, suggesting that other cell types are the source of IL-10 in chronic T. cruzi infection. In fact, as it was mentioned before, a negative correlation between parasite detection in blood and the percentage of CD24highCD27+ B cell exists in CCD patients, and this subset is identified as the central IL-10 secreting B cells in humans (Jansen et al., 2021).
The alterations detected in the phenotypic distribution of B10 cells upon stimulation with T. cruzi disclose that their increase in patients with Chagas disease cardiomyopathy would be mainly associated with those populations lacking CD27, such as CD24−CD27− and CD27−CD38+. Interestingly, the former was also found augmented in our ex vivo analysis (Girard et al., 2021), underpinning this subpopulation as a possible trait in patients with the cardiac form of CCD, and if so, it requires further investigation. Under the same experimental stimulation with CpG + CD40L, Jiao et al. (2018) observed that patients with Idiopathic Dilated Cardiomyopathy (IDC), a heart disease sharing some features with the cardiac form of CCD, presented a decrease in total B10 cells compared to healthy individuals, particularly within CD24highCD27+ subset. What is even more interesting is that the frequency of B10 cells correlated with parameters of cardiac function in patients, suggesting the contribution of these cells in the severity of heart damage and the progression of IDC (Jiao et al., 2018). Our data proved to be similar between patients with CCD and non-infected donors, illustrating that the Breg response mounted against the stimulus, different from the parasite itself, could be equally effective.
An additional finding of our study was the higher IL-17 secretion by B cells upon T. cruzi stimulation in patients with cardiac involvement. Studies in vitro with human tonsillar cells pinpoint B cells as an important source of IL-17 (B17 cells) in response to T. cruzi infection, although the specific phenotype of these cells has not yet been identified (Bermejo et al., 2013). Likewise, studies evaluating IL-17 in patients infected with T. cruzi suggest a protective immunomodulatory function of this cytokine to prevent cardiac damage in CCD (Amezcua Vesely et al., 2020). Patients with cardiac manifestations have lower levels of IL-17 in total lymphocytes and a lower frequency of Th17 cells than patients without clinical symptoms. In turn, IL-17 levels positively correlate with better cardiac function (Magalhães et al., 2013; Sousa et al., 2017). Our results also align with these findings and point to a protective function of B17 cells in patients undergoing heart disease.
Finally, we investigated whether Breg cells could modulate inflammatory responses by mechanisms other than IL-10 production, for example by expression of PD-L1, which engages the inhibitory receptor programmed-cell death 1 (PD1) on T cells. In fact, the only finding regarding the inhibitory molecules PD1/PD-L1 in chronic infection with T. cruzi comes from the murine model in which the blockade of this interaction increased leukocyte infiltration in cardiac tissue and diminished blood parasite load (Fonseca et al., 2018). In accordance, we observed that stimulation with T. cruzi induced an increase in PD-L1+ B cells in patients with CCD, highlighting that this molecule could protect the heart from an excessive immune response.
The study has some potential limitations. First, the number of subjects included in each group was small. Second, the inability to recruit patients with different cardiac disorders, means stratified as K2 and K3 according to Kuschnir classification, restrained to analyze how the phenotypic traits of B and B10 cells vary with the progression of CCD. Third, the low quantity of B cells isolated per individual limited our ability to measure other anti-inflammatory cytokines such IL-35 and TGF-β, as well as cell surface proteins like TIM-1, and CD73, which would help to unravel unknown aspects of the regulatory mechanisms raised by B cells in patients with chronic Chagas disease. Here, we have detailed the phenotypic characteristics of B and B10 cells based on the expression of CD24, CD27, and CD38 that provides evidence about the potential role of Breg cells in the immune response developed against T. cruzi, illustrated in Figure 8. Taken together, our findings mainly point out that the frequency of the most representative Breg cells in humans, CD19+CD24highCD38high and CD19+CD24highCD27+ B cells, are altered in patients with CCD; while both phenotypes dropped in subjects without symptoms, only the latter decreased in patients with cardiac manifestations. Further research is required to uncover the functional effects exerted by these cells to regulate other immune cells within the complex response against T. cruzi.
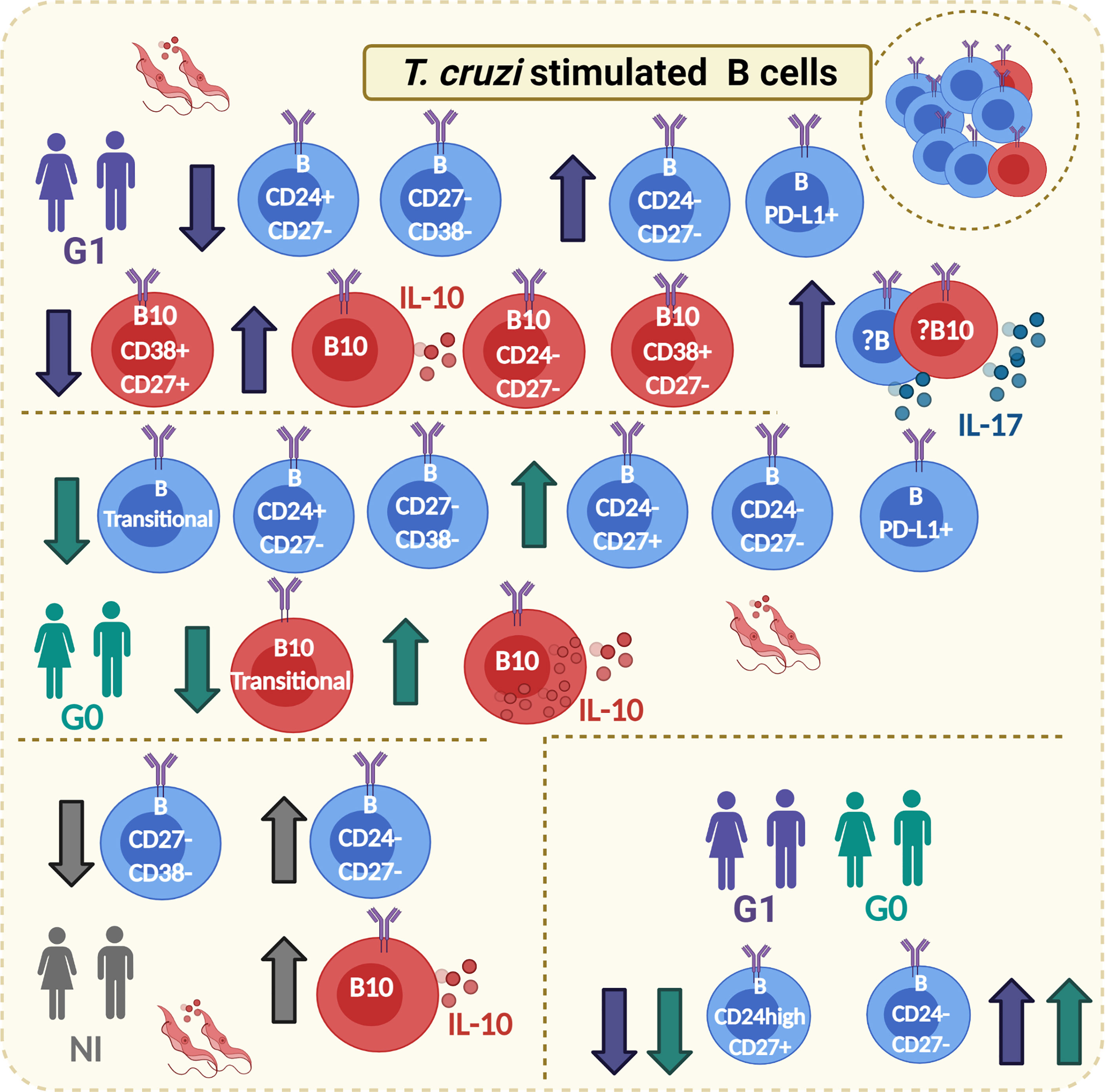
Figure 8 Graphical abstract. Main phenotypic traits identified on B and B10 cells from CCD patients with or without cardiac manifestations and NI donors in response to T. cruzi stimulation. Boxes with T. cruzi parasite images in red show changes observed on T. cruzi–stimulated B cells, whereas the lower box on the right corresponds to changes on subpopulations of B cells that were independent of the stimulation condition. The direction of change is indicated with arrows (increase/decrease). The color of the arrows indicates in which groups of subjects was detected the mentioned alteration (green arrows: G0; purple arrows: G1; gray arrows: NI). Full circles in the cell cytoplasm indicate increased MFI for IL-10, while full circles outside the cell cytoplasm indicate increased secretion of the corresponding cytokine (IL-10 or IL-17). Created with Biorender.com.
Data Availability Statement
The raw data supporting the conclusions of this article will be made available by the authors, without undue reservation.
Ethics Statement
All experimental procedures have been carried out following the standard laboratory biosecurity guidance established by the Instituto de Investigaciones en Ingeniería Genética y Biología Molecular (INGEBI-CONICET).
Subjects were recruited at Instituto Nacional de Parasitología “Dr. Mario Fatala Chabén” under the guidance of Marisa Fernandez, MS, and Yolanda Hernández-Vásquez, MS (Study Protocol N° 3-2018 and 19-2019), and at Hospital General de Agudos “Dr. Ignacio Pirovano,” under the supervision of Raúl Chadi, MS (Study Protocol N° 56-2015). The sampling process followed the tenets of the Declaration of Helsinki and of both institutions’ medical Ethics Committees. Written informed consent was provided by all of the participants.
Author Contributions
MG designed and performed the experiments, analyzed the data, and wrote the manuscript. MO performed PCR for T. cruzi detection. AM-C carried out the parasite culture to produce parasite lysate. MF, YH-V, and RC recruited and classified donors and collected blood samples. KG supervised and designed the research and wrote the manuscript. All authors contributed to the article and approved the submitted version.
Funding
This research was supported by grants from Consejo Nacional de Investigaciones Científicas y Técnicas (CONICET; PIP Number 112-2015010-0547) and Agencia Nacional de Promoción Científica y Tecnológica, Argentina (ANPCyT; PICT Number 2014-1026). The funders had no role in study design, data collection and interpretation, or the decision to submit the work for publication.
Conflict of Interest
The authors declare that the research was conducted in the absence of any commercial or financial relationships that could be construed as a potential conflict of interest.
Publisher’s Note
All claims expressed in this article are solely those of the authors and do not necessarily represent those of their affiliated organizations, or those of the publisher, the editors and the reviewers. Any product that may be evaluated in this article, or claim that may be made by its manufacturer, is not guaranteed or endorsed by the publisher.
Acknowledgments
The authors express our immense gratitude to sample donors involved in this study for their generous participation. We are indebted to Violeta Chiauzzi from Instituto de Biología y Medicina Experimental (IBYME-CONICET) for technical assistance in blood sample collection and to Dr. Eloisa Arana from INIGEM (UBA-CONICET) for guiding us in the B cell experimental setup. We are also grateful to Dr. María Victoria Delpino for providing IL-17 ELISA kit.
Supplementary Material
The Supplementary Material for this article can be found online at: https://www.frontiersin.org/articles/10.3389/fcimb.2021.723549/full#supplementary-material
References
Abebe, E. C., Dejenie, T. A., Ayele, T. M., Baye, N. D., Teshome, A. A., Muche, Z. T. (2021). The Role of Regulatory B Cells in Health and Diseases: A Systemic Review. J. Inflammation Res. 14, 75. doi: 10.2147/JIR.S286426
Acevedo, G. R., Girard, M. C., Gómez, K. A. (2018). The Unsolved Jigsaw Puzzle of the Immune Response in Chagas Disease. Front. Immunol. 9:1929. doi: 10.3389/fimmu.2018.01929
Acevedo, G. R., Longhi, S. A., Bunying, A., Sabri, N., Atienza, A., Zago, M. P., et al. (2017). Methodological Approach to the Ex Vivo Expansion and Detection of T. Cruzi-Specific T Cells From Chronic Chagas Disease Patients. PloS One 12 (5), e0178380. doi: 10.1371/journal.pone.0178380
Amezcua Vesely, M. C., Rodríguez, C., Gruppi, A., Acosta Rodríguez, E. V. (2020). Interleukin-17 Mediated Immunity During Infections With Trypanosoma Cruzi and Other Protozoans. Biochim. Biophys. Acta (BBA) - Mol. Basis Dis. 1866 (5), 165706. doi: 10.1016/j.bbadis.2020.165706
Antonioli, L., Pacher, P., Vizi, E. S., Haskó, G. (2013). CD39 and CD73 in Immunity and Inflammation. Trends Mol. Med. 19 (6), 355–367. doi: 10.1016/j.molmed.2013.03.005
Aravena, O., Ferrier, A., Menon, M., Mauri, C., Aguillón, J. C., Soto, L., et al. (2017). TIM-1 Defines a Human Regulatory B Cell Population That is Altered in Frequency and Function in Systemic Sclerosis Patients. Arthritis Res. Ther. 19 (1), 8. doi: 10.1186/s13075-016-1213-9
Bankó, Z., Pozsgay, J., Szili, D., Tóth, M., Gáti, T., Nagy, G., et al. (2017). Induction and Differentiation of IL-10–Producing Regulatory B Cells From Healthy Blood Donors and Rheumatoid Arthritis Patients. J. Immunol. 198 (4), 1512–1520. doi: 10.4049/jimmunol.1600218
Bartholomeu, D. C., Ropert, C., Melo, M. B., Parroche, P., Junqueira, C. F., Teixeira, S. M. R., et al. (2008). Recruitment and Endo-Lysosomal Activation of TLR9 in Dendritic Cells Infected With Trypanosoma Cruzi. J. Immunol. 181 (2), 1333–1344. doi: 10.4049/jimmunol.181.2.1333
Bates, D. M.. (2010). lme4: Mixed-Effects Modeling With R. New York: Springer. Available at: http://lme4.r-forge.r-project.org/book/front.pdf.
Bermejo, D. A., Jackson, S. W., Gorosito-Serran, M., Acosta-Rodriguez, E. V., Amezcua-Vesely, M. C., Sather, B. D., et al. (2013). Trypanosoma Cruzi Trans-Sialidase Initiates a Program Independent of the Transcription Factors Rorγt and Ahr That Leads to IL-17 Production by Activated B Cells. Nat. Immunol. 14 (5), 514–522. doi: 10.1038/ni.2569
Blair, P. A., Noreña, L. Y., Flores-Borja, F., Rawlings, D. J., Isenberg, D. A., Ehrenstein, M. R., et al. (2010). CD19+CD24hiCD38hi B Cells Exhibit Regulatory Capacity in Healthy Individuals But Are Functionally Impaired in Systemic Lupus Erythematosus Patients. Immunity 32 (1), 129–140. doi: 10.1016/j.immuni.2009.11.009
Cardoso, M. S., Reis-Cunha, J. L., Bartholomeu, D. C. (2016). Evasion of the Immune Response by Trypanosoma Cruzi During Acute Infection. Front. Immunol. 6 (659), 24–38. doi: 10.3389/fimmu.2015.00659
Chevillard, C., Nunes, J. P. S., Frade, A. F., Almeida, R. R., Pandey, R. P., Nascimento, M. S., et al. (2018). Disease Tolerance and Pathogen Resistance Genes May Underlie Trypanosoma Cruzi Persistence and Differential Progression to Chagas Disease Cardiomyopathy. Front. Immunol. 9:2791 (2791). doi: 10.3389/fimmu.2018.02791
Couper, K. N., Blount, D. G., Riley, E. M. (2008). IL-10: The Master Regulator of Immunity to Infection. J. Immunol. 180 (9), 5771–5777. doi: 10.4049/jimmunol.180.9.5771
Damasio, M. P. S., Rocha, M. O. C., Sousa, G. R., Ferreira, K. S., Fares-Gusmão, R. C. G., Medeiros, N. I., et al. (2019). PD1 and PDL1 Molecules Control Suppressor Activity of Regulatory T Cells in Chronic Chagas Cardiomyopathy Patients. Hum. Immunol. 80 (7), 517–522. doi: 10.1016/j.humimm.2019.03.009
de Masson, A., Le Buanec, H., Bouaziz, J.-D. (2014). “Purification and Immunophenotypic Characterization of Human B Cells With Regulatory Functions”, in Regulatory B Cells. (New York: Springer Protocols, Humana Press), 45–52.
Duffy, T., Cura, C. I., Ramirez, J. C., Abate, T., Cayo, N. M., Parrado, R., et al. (2013). Analytical Performance of a Multiplex Real-Time PCR Assay Using TaqMan Probes for Quantification of Trypanosoma Cruzi Satellite DNA in Blood Samples. PloS Neglected Trop. Dis. 7 (1), e2000. doi: 10.1371/journal.pntd.0002000
Fares, R. C. G., Correa-Oliveira, R., de Araújo, F. F., Keesen, T. S. L., Chaves, A. T., Fiuza, J. A., et al. (2013). Identification of Phenotypic Markers of B Cells From Patients With Chagas Disease. Parasite Immunol. 35 (7-8), 214–223. doi: 10.1111/pim.12038
Figueiró, F., Muller, L., Funk, S., Jackson, E. K., Battastini, A. M. O., Whiteside, T. L. (2016). Phenotypic and Functional Characteristics of CD39high Human Regulatory B Cells (Breg). OncoImmunology 5 (2), e1082703. doi: 10.1080/2162402X.2015.1082703
Flores-Borja, F., Bosma, A., Ng, D., Reddy, V., Ehrenstein, M. R., Isenberg, D. A., et al. (2013). CD19+CD24hiCD38hi B Cells Maintain Regulatory T Cells While Limiting TH1 and TH17 Differentiation. Sci. Trans. Med. 5 (173), 173ra123–173ra123. doi: 10.1126/scitranslmed.3005407
Fonseca, R., Salgado, R. M., Borges da Silva, H., Nascimento, R., D’Império-Lima, M. R., Alvarez, J. M. (2018). Programmed Cell Death Protein 1–PDL1 Interaction Prevents Heart Damage in Chronic Trypanosoma Cruzi Infection. Front. Immunol. 9:997. doi: 10.3389/fimmu.2018.00997
Girard, M. C., Acevedo, G. R., López, L., Ossowski, M. S., Piñeyro, M. D., Grosso, J. P., et al. (2018). Evaluation of the Immune Response Against Trypanosoma Cruzi Cytosolic Tryparedoxin Peroxidase in Human Natural Infection. Immunology 155 (3), 367–378. doi: 10.1111/imm.12979
Girard, M. C., Acevedo, G. R., Ossowski, M. S., Fernández, M., Hernández, Y., Chadi, R., et al. (2021). Ex Vivo Characterization of Breg Cells in Patients With Chronic Chagas Disease. Sci. Rep. 11 (1), 1–15. doi: 10.1038/s41598-021-84765-x
Gu, X.-L., He, H., Lin, L., Luo, G.-X., Wen, Y.-F., Xiang, D.-C., et al. (2017b). Tim-1+ B Cells Suppress T Cell Interferon-Gamma Production and Promote Foxp3 Expression, But Have Impaired Regulatory Function in Coronary Artery Disease. APMIS 125 (10), 872–879. doi: 10.1111/apm.12729
Gu, J., Ni, X., Pan, X., Lu, H., Lu, Y., Zhao, J., et al. (2017a). Human CD39hi Regulatory T Cells Present Stronger Stability and Function Under Inflammatory Conditions. Cell. Mol. Immunol. 14 (6), 521–528. doi: 10.1038/cmi.2016.30
Harris, D. P., Haynes, L., Sayles, P. C., Duso, D. K., Eaton, S. M., Lepak, N. M., et al. (2000). Reciprocal Regulation of Polarized Cytokine Production by Effector B and T Cells. Nat. Immunol. 1 (6), 475–482. doi: 10.1038/82717
Hasan, M. M., Thompson-Snipes, L., Klintmalm, G., Demetris, A. J., O’Leary, J., Oh, S., et al. (2019). CD24hiCD38hi and CD24hiCD27+ Human Regulatory B Cells Display Common and Distinct Functional Characteristics. J. Immunol. 203 (8), 2110–2120. doi: 10.4049/jimmunol.1900488
Hothorn, T., Bretz, F, Westfall, P., Heiberger, R. M., Schuetzenmeister, A., Scheibe, S., et al. (2014). Multcomp: Simultaneous Inference in General Parametric Models. 1.3-2.
Iwata, Y., Matsushita, T., Horikawa, M., DiLillo, D. J., Yanaba, K., Venturi, G. M., et al. (2011). Characterization of a Rare IL-10–Competent B-Cell Subset in Humans That Parallels Mouse Regulatory B10 Cells. Blood 117 (2), 530–541. doi: 10.1182/blood-2010-07-294249
Jansen, K., Cevhertas, L., Ma, S., Satitsuksanoa, P., Akdis, M., van de Veen, W. (2021). Regulatory B Cells, A to Z. Allergy. doi: 10.1111/all.14763
Jiao, J., Lu, Y. Z., Xia, N., Wang, Y. Q., Tang, T. T., Nie, S. F., et al. (2018). Defective Circulating Regulatory B Cells in Patients With Dilated Cardiomyopathy. Cell. Physiol. Biochem. 46 (1), 23–35. doi: 10.1159/000488405
Kaku, H., Cheng, K. F., Al-Abed, Y., Rothstein, T. L. (2014). A Novel Mechanism of B Cell–Mediated Immune Suppression Through CD73 Expression and Adenosine Production. J. Immunol. 193 (12), 5904–5913. doi: 10.4049/jimmunol.1400336
Khan, A. R., Hams, E., Floudas, A., Sparwasser, T., Weaver, C. T., Fallon, P. G. (2015). PD-L1hi B Cells are Critical Regulators of Humoral Immunity. Nat. Commun. 6 (1), 5997. doi: 10.1038/ncomms6997
Li, S., Ma, F., Hao, H., Wang, D., Gao, Y., Zhou, J., et al. (2018). Marked Elevation of Circulating CD19+CD38hiCD24hi Transitional B Cells Give Protection Against Neonatal Sepsis. Pediatr. Neonatol. 59 (3), 296–304. doi: 10.1016/j.pedneo.2017.10.005
Liu, B.-S., Cao, Y., Huizinga, T. W., Hafler, D. A., Toes, R. E. M. (2014). TLR-Mediated STAT3 and ERK Activation Controls IL-10 Secretion by Human B Cells. Eur. J. Immunol. 44 (7), 2121–2129. doi: 10.1002/eji.201344341
Li, J., Wang, L., Chen, X., Li, L., Li, Y., Ping, Y., et al. (2017). CD39/CD73 Upregulation on Myeloid-Derived Suppressor Cells via TGF-β-mTOR-HIF-1 Signaling in Patients With non-Small Cell Lung Cancer. OncoImmunology 6 (6), e1320011. doi: 10.1080/2162402X.2017.1320011
Longhi, S. A., Atienza, A., Perez Prados, G., Buying, A., Balouz, V., Buscaglia, C. A., et al. (2014). Cytokine Production But Lack of Proliferation in Peripheral Blood Mononuclear Cells From Chronic Chagas’ Disease Cardiomyopathy Patients in Response to T. Cruzi Ribosomal P Proteins. PloS Neglected Trop. Dis. 8 (6), e2906. doi: 10.1371/journal.pntd.0002906
Magalhães, L. M. D., Villani, F. N. A., Nunes, M., Gollob, K. J., Rocha, M. O. C., Dutra, W. O. (2013). High Interleukin 17 Expression Is Correlated With Better Cardiac Function in Human Chagas Disease. J. Infect. Dis. 207 (4), 661–665. doi: 10.1093/infdis/jis724
Mege, J.-L., Meghari, S., Honstettre, A., Capo, C., Raoult, D. (2006). The Two Faces of Interleukin 10 in Human Infectious Diseases. Lancet Infect. Dis. 6 (9), 557–569. doi: 10.1016/S1473-3099(06)70577-1
Mensah, F. F. K., Armstrong, C. W., Reddy, V., Bansal, A. S., Berkovitz, S., Leandro, M. J., et al. (2018). CD24 Expression and B Cell Maturation Shows a Novel Link With Energy Metabolism: Potential Implications for Patients With Myalgic Encephalomyelitis/Chronic Fatigue Syndrome. Front. Immunol. 9:2421. doi: 10.3389/fimmu.2018.02421
Nardy, F. A., Freire-de-Lima, C. G., Morrot, A. (2015). Immune Evasion Strategies of Trypanosoma Cruzi. J. Immunol. Res. 2015, 7. doi: 10.1155/2015/178947
Oleinika, K., Rosser, E. C., Matei, D. E., Nistala, K., Bosma, A., Drozdov, I., et al. (2018). CD1d-Dependent Immune Suppression Mediated by Regulatory B Cells Through Modulations of iNKT Cells. Nat. Commun. 9 (1), 684. doi: 10.1038/s41467-018-02911-y
Pérez-Molina, J. A., Molina, I. (2018). Chagas Disease. Lancet 391 (10115), 82–94. doi: 10.1016/S0140-6736(17)31612-4
Ponce, N. E., Sanmarco, L. M., Eberhardt, N., García, M. C., Rivarola, H. W., Cano, R. C., et al. (2016). CD73 Inhibition Shifts Cardiac Macrophage Polarization Toward a Microbicidal Phenotype and Ameliorates the Outcome of Experimental Chagas Cardiomyopathy. J. Immunol. 197 (3), 814–823. doi: 10.4049/jimmunol.1600371
R Core Team. (2013). R: A Language and Environment for Statistical Computing [Computer software manual]. Vienna, Austria. Available at: http://www.R-project.org/ (Version 3.0.0).
Ramírez, J. C., Cura, C. I., da Cruz Moreira, O., Lages-Silva, E., Juiz, N., Velázquez, E., et al. (2015). Analytical Validation of Quantitative Real-Time PCR Methods for Quantification of Trypanosoma Cruzi DNA in Blood Samples From Chagas Disease Patients. J. Mol. Diagnostics 17 (5), 605–615. doi: 10.1016/j.jmoldx.2015.04.010
Ribeiro, A. L., Nunes, M. P., Teixeira, M. M., Rocha, M. O. C. (2012). Diagnosis and Management of Chagas Disease and Cardiomyopathy. Nat. Rev. Cardiol. 9 (10), 576–589. doi: 10.1038/nrcardio.2012.109
Rosser, E. C., Mauri, C. (2015). Regulatory B Cells: Origin, Phenotype, and Function. Immunity 42 (4), 607–612. doi: 10.1016/j.immuni.2015.04.005
Salvador, F., Sánchez-Montalvá, A., Martínez-Gallo, M., Sulleiro, E., Franco-Jarava, C., Sao Avilés, A., et al. (2020). Serum IL-10 Levels and Its Relationship With Parasitemia in Chronic Chagas Disease Patients. Am. J. Trop. Med. Hygiene 102 (1), 159–163. doi: 10.4269/ajtmh.19-0550
Santos, R. F., Pôssa, M. A. S., Bastos, M. S., Guedes, P. M. M., Almeida, M. R., DeMarco, R., et al. (2009). Influence of Ecto-Nucleoside Triphosphate Diphosphohydrolase Activity on Trypanosoma Cruzi Infectivity and Virulence. PloS Neglected Trop. Dis. 3 (3), e387. doi: 10.1371/journal.pntd.0000387
Sanz, I., Wei, C., Lee, F. E.-H., Anolik, J. (2008). Phenotypic and Functional Heterogeneity of Human Memory B Cells. Semin. Immunol. 20 (1), 67–82. doi: 10.1016/j.smim.2007.12.006
Sousa, G. R., Gomes, J. A. S., Damasio, M. P. S., Nunes, M. C. P., Costa, H. S., Medeiros, N. I., et al. (2017). The Role of Interleukin 17-Mediated Immune Response in Chagas Disease: High Level Is Correlated With Better Left Ventricular Function. PLOS ONE 12 (3), e0172833. doi: 10.1371/journal.pone.0172833
Suthers, A. N., Sarantopoulos, S. (2017). TLR7/TLR9- and B Cell Receptor-Signaling Crosstalk: Promotion of Potentially Dangerous B Cells. Front. Immunol. 8 (775), 21–28. doi: 10.3389/fimmu.2017.00775
van de Veen, W., Stanic, B., Yaman, G., Wawrzyniak, M., Söllner, S., Akdis, D. G., et al. (2013). IgG4 Production Is Confined to Human IL-10–Producing Regulatory B Cells That Suppress Antigen-Specific Immune Responses. J. Allergy Clin. Immunol. 131 (4), 1204–1212. doi: 10.1016/j.jaci.2013.01.014
World Health Organization (2002) Control of Chagas Disease: Second Report of the WHO Expert Committee. Available at: https://apps.who.int/iris/handle/10665/42443.
World Health Organization (2021) Chagas Disease (American Trypanosomiasis) [Online]. Available at: https://www.who.int/es/news-room/fact-sheets/detail/chagas-disease-(american-trypanosomiasis) (Accessed May 21, 2021).
Zhang, M., Zeng, G., Yang, Q., Zhang, J., Zhu, X., Chen, Q., et al. (2014). Anti-Tuberculosis Treatment Enhances the Production of IL-22 Through Reducing the Frequencies of Regulatory B Cell. Tuberculosis 94 (3), 238–244. doi: 10.1016/j.tube.2013.12.003
Keywords: regulatory B cells, IL-10-producing B cells, chronic Chagas disease, human Trypanosoma cruzi infection, chronic Chagas cardiomyopathy, PD-L1
Citation: Girard MC, Ossowski MS, Muñoz-Calderón A, Fernández M, Hernández-Vásquez Y, Chadi R and Gómez KA (2021) Trypanosoma cruzi Induces Regulatory B Cell Alterations in Patients With Chronic Chagas Disease. Front. Cell. Infect. Microbiol. 11:723549. doi: 10.3389/fcimb.2021.723549
Received: 10 June 2021; Accepted: 21 July 2021;
Published: 12 August 2021.
Edited by:
Giovane R. Sousa, Harvard Medical School, United StatesReviewed by:
Claudia M. Calvet, University of California, San Diego, United StatesMarcos Damasio, Massachusetts General Hospital and Harvard Medical School, United States
Copyright © 2021 Girard, Ossowski, Muñoz-Calderón, Fernández, Hernández-Vásquez, Chadi and Gómez. This is an open-access article distributed under the terms of the Creative Commons Attribution License (CC BY). The use, distribution or reproduction in other forums is permitted, provided the original author(s) and the copyright owner(s) are credited and that the original publication in this journal is cited, in accordance with accepted academic practice. No use, distribution or reproduction is permitted which does not comply with these terms.
*Correspondence: Karina A. Gómez, gomez@dna.uba.ar