Aggregation-Induced Polarization (AIP): Optical Rotation Amplification and Adjustment of Chiral Aggregates of Folding Oligomers and Polymers
- 1Department of Chemistry and Biochemistry, Texas Tech University, Lubbock, TX, United States
- 2Institute of Chemistry and BioMedical Sciences, School of Chemistry and Chemical Engineering, Nanjing University, Nanjing, China
- 3Continuous Flow Engineering Laboratory of National Petroleum and Chemical Industry, Changzhou University, Changzhou, China
The phenomenon of aggregation-induced polarization (AIP) was observed showing optical rotation amplification and adjustment. The relationship between optical rotations of chiral aggregates of multilayered chiral folding oligomers and polymers with water% in THF (fw) has been established accordingly. New multilayered chiral oligomers were synthesized under the asymmetric catalytic systems established by our laboratory recently. These products were well-characterized by UV-vis, NMR, and MALDI-TOF spectra. Absolute stereochemistry (enantio- and diastereochemistry) was assigned by comparison with similar asymmetric induction by the same catalyst in our previous reactions. The present AIP work can serve as a new tool to determine chiral aggregates, especially for those that cannot display emission. AIP would also complement AIE-based CPL since AIP serves as a new tool providing enhanced right- or left-hand polarized lights with individual wavelengths. It will find many applications in chemical and materials science in the future.
Introduction
The study of chirality and chiral targets has become increasingly important in science and technology because of the requirements of desired chemical, biomedical, and physical properties of advanced materials (Wang et al., 1983; Taniguchi et al., 2011; Krautwald et al., 2013; Bryliakov, 2020; Zhao et al., 2020; Zhang and Kürti, 2021). In the past several decades, a significant progress has been made in controlling optical properties related to radar, lasers, optical fibers, and wireless fiber telecommunications (Huang et al., 2018; Feng et al., 2019; Xu et al., 2019; Shi et al., 2020; Liu et al., 2021; Oki et al., 2021; Zheng et al., 2022). Among these properties are circular light polarization and optical rotation for evaluating the performance of chiral targets. It is well-known that the optical fields rotate at a constant rate in a plane during circular light polarization when the waves travel (Desmarais, 1997; Singh, 2015). When waves transmit through a solution containing chiral objects, two types of rotations occur, that is, the field is rotated toward either the right-hand or left-hand direction, called right- and left-circular polarization, respectively. Left- versus right-circularly polarized lights can be generated directly by chiral molecules, nanoaggregates, and soft matters. The optical and rotational abilities of these chiral objects are anticipated to play an important role for the design and synthesis of advanced materials and their applications. In fact, the research on circularly polarized luminescence (CPL) materials has attracted much attention due to their potential to serve as displaying and optical storage devices, probes, sensors, etc. (Shi et al., 2020; Liu et al., 2021; Zheng et al., 2022).
Meanwhile, aggregation-induced emission (AIE) has grabbed widespread attention since 2001 when Tang and coworkers coined this concept based on their discovery of organic molecules displaying strong fluorescence in aggregation states (Huang et al., 2018; Feng et al., 2019). The origin of AIE was made possible by restricting intramolecular motions (rotation and vibration) which limit or avoid aggregation-caused quenching (ACQ) of conventional luminophores. During our ongoing projects on multiple-layered 3D folding chirality, we found that chiral aggregates of folding monomers, oligomers, and polymers displayed strong AIE by gradually increasing water fractions (fw) in THF-H2O cosolvents (Wu et al., 2019; Liu et al., 2020; Wu et al., 2020; Wu et al., 2021a; Wu et al., 2021b; Tang et al., 2022b; Jin et al., 2022; Tang et al., 2022; Wang et al., 2022). Interestingly, we also found these polymers showed AIE curves bounding when fw was increased to 70%, which contrast with the observation of their monomer derivatives (Wu et al., 2021b; Tang et al., 2022; Wang et al., 2022). This big jump is attributed to the suppression of molecular motion in the polymer matrix, indicating that our chiral multilayer framework resulted in effective soft aggregates in THF-H2O co-solvents.
Since the AIE observation can conform to the formation of various chiral aggregates of multilayer chiral polymers, we envisioned that the coexistence of various chiral aggregates in THF-H2O solutions would lead to optical rotation enhancement or adjustment systematically. The literature search revealed nearly no documents on the X- and Y-coordinate relationship regarding aggregation-induced effects on optical rotation. In this communication, we would like to disclose our preliminary results on this study, temporally called aggregation-induced polarization (AIP).
Result and Discussion
The present AIP project was started by designing and synthesizing of new multilayer polymers and oligomers (Figures 1A,B). This work is based on the use of various new monomers which have showed success in previous asymmetric catalytic assembly of triple-columned and multiple-layered chiral folding polymers (Wu et al., 2021b; Tang et al., 2022; Wang et al., 2022) (Figures 1C,D). In the present work, new combinations of monomers were utilized by involving two pairs: 4,7-bis(8-(4,4,5,5-tetramethyl-1,3,2-dioxaborolan-2-yl)naphthalen-1-yl)benzo [c][1,2,5] thiadiazole (1) and 4,7-bis(8-bromonaphthalen-1-yl)benzo[c][1,2,5]thiadiazole (2), 4,7-bis(8-(4,4,5,5-tetramethyl-1,3,2-dioxaborolan-2-yl)naphthalen-1-yl)benzo [c][1,2,5] selenodiazole (3) and 4,7-bis(8-bromonaphthalen-1-yl)benzo[c][1,2,5]thiadiazole (1), respectively. As described in Figure 1, the resulting multilayer targets contain unique structural units of sandwich panels. The previous columns (piers) serve as decked layers holding [1,2,5]thiadiazole or [1,2,5]selenodiazole as unit cores. For potential applications of the new framework, electron transfer would occur concurrently through-space among three layers of sandwich panels and conjugation between sandwich units.
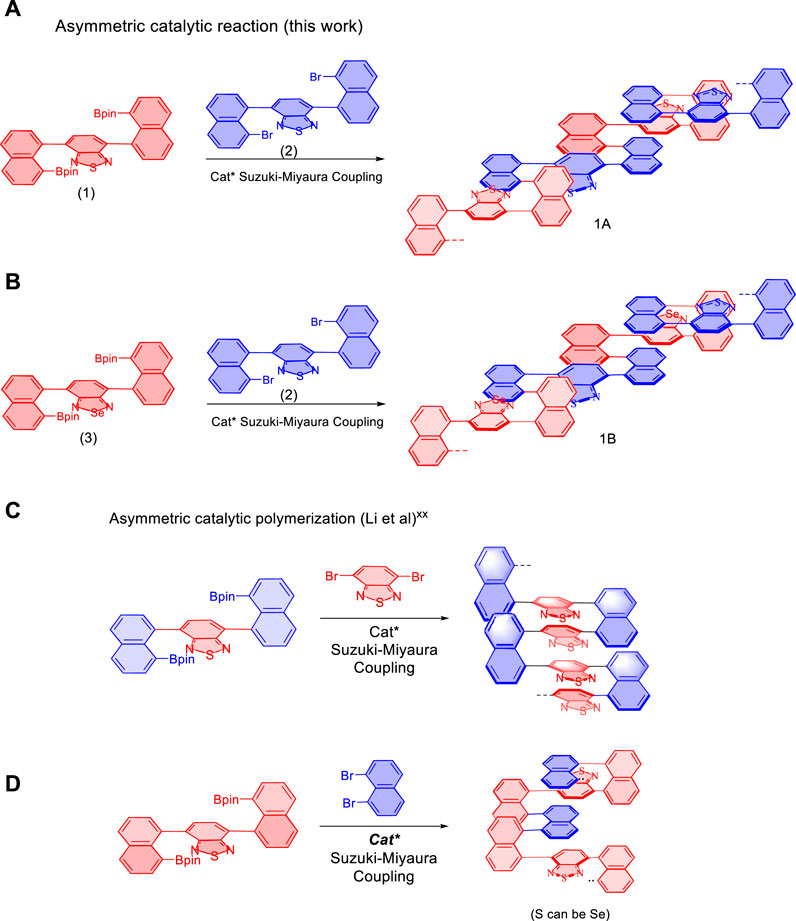
FIGURE 1. Synthesis of multilayer polymers and oligomers via an asymmetric approach. (A,B) Design and synthesis of new multilayer polymers and oligomers. (C,D) Reported asymmetric assembly of triple-columned and multiple-layered chiral folding polymers. Cat*: chiral catalysts.
In our previous work, both C and D series exhibited intense fluorescence activity and remarkable optical properties in aggregated states, more specifically, photoluminescence in solids and aggregation-induced emission (AIE) in solutions under specific wavelength irradiation (Wu et al., 2021b; Tang et al., 2022; Wang et al., 2022). In the meanwhile, C series presents were found to display not only AIE characteristics but also reversible redox properties in electrochemical performance.
Monomer 4,7-bis(4,4,5,5-tetramethyl-1,3,2-dioxaborolan-2-yl)benzo[c][1,2,5]thiadiazole (1) was synthesized by reacting bis(pinacolato)diboron with 4,7-bis(8-bromonaphthalen-1-yl)benzo[c][1,2,5]thiadiazole, which was preformed from the treatment of 4,7-bis(4,4,5,5-tetramethyl-1,3,2-dioxaborolan-2-yl)benzo[c][1,2,5]thiadiazole with 1,8-dibromonaphthalene under traditional Suzuki-Miyaura cross-coupling conditions followed by treating with bis(pinacolato)diboron (Miyaura and Suzuki, 1995; Kumar et al., 2020). Selenodiazoles (3) was derived from its thiadiazole counterpart through the reductive opening of thiadiazole ring by using an excess amount of sodium borohydride (4.0 equiv) in the presence of CoCl2·6H2O (10 mol%) (Scheme 1) (Neto et al., 2005; Idris et al., 2018). The resulting crude diamine product was directly subjected to the next step for the ring-closure reaction with selenium dioxide (2.0 equiv) in ethanol at 80°C (Scheme 1). The reasons for selecting thiadiazole and selenodiazole infrastructures for the present project are based on the fact that they are among the most frequently used scaffolds in polymers and materials science (Vyskocil et al., 2002; Jurok et al., 2010; Echeverri et al., 2020), and have been proven to be successful in asymmetric catalytic polymerization.
As reported previously, asymmetric polymerization was attempted under the standard catalytic system. [20-21] Pd(S-BINAP) Cl2 was utilized as the chiral catalyst in THF/H2O co-solvents in the presence of K2CO3 at 85°C for 6 days. Surprisingly, chiral polymers could not be well-formed under the standard condition. Instead, two sets of corresponding chiral oligomers were afforded in chemical yields of 57.7% for 1A and 47.9% for 1B, respectively. As shown in Figure 2, MALDI-TOF indicated that both 1A and 1B contain up to four layers in their layered frameworks. While 1B is attached with Br and 4,4,5,5-tetramethyl-1,3,2-dioxaborolan-2-yl moieties at each terminal of this oligomer, 1A does not contain these two moieties as shown by MALDI-TOF analysis (Figure 2).
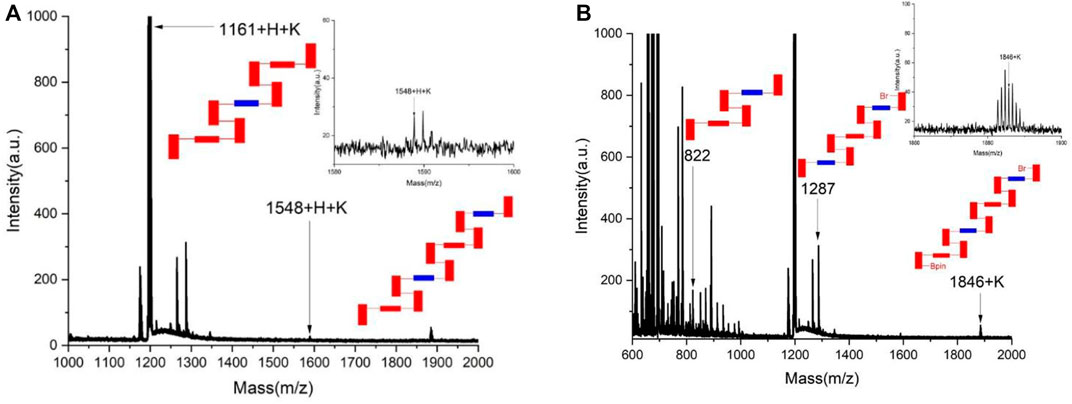
FIGURE 2. MALDI-TOF analysis of chiral oligomers 1A and 1B. (A) MALDI-TOF of the chiral oligomer 1A. (B) MALDI-TOF of the chiral oligomer 1B.
It is challenging to purify individual oligomers for X-ray structural analysis because various species coexist in the mixture products including relevant stereoisomers. The absolute configuration of major enantiomers was assigned by comparison with the previous stereoselective control by the same catalyst of Pd(S-BINAP)Cl2. This catalyst was anticipated to give the same asymmetric induction in the present catalytic processes (Wu et al., 2021a; Wu et al., 2021b; Tang et al., 2022; Wang et al., 2022). It should be noted that the asymmetric catalytic formation of 1A and 1B would provide a new atropisomeric framework for controlling orientational/rotational chirality if a chiral tetrahedron center replaces one of two Bpin or Br moieties in oligomer 1B.
The UV-Vis absorption of compounds 1A and 1B in THF was recorded (Figure 3). 1A and 2B exhibited similar maximum absorptions at 308 nm, and 1A showed a board absorption band between 355 and 450 nm. However, replacing sulfur from the heterocycle on the one side of the backbone with a heavier chalcogen atom Se led to a redshift on the broadband due to the lowered energy band (10 nm for 1B vs. 1A). Similar behavior can also be found in the emission spectrum, where 2B with the selenium atom shifted to the longer wavelength (520 nm, compared to 1A). Surprisingly, 1B with sulfur atoms on both sides of backbones exhibited six-fold stronger fluorescence intensity.
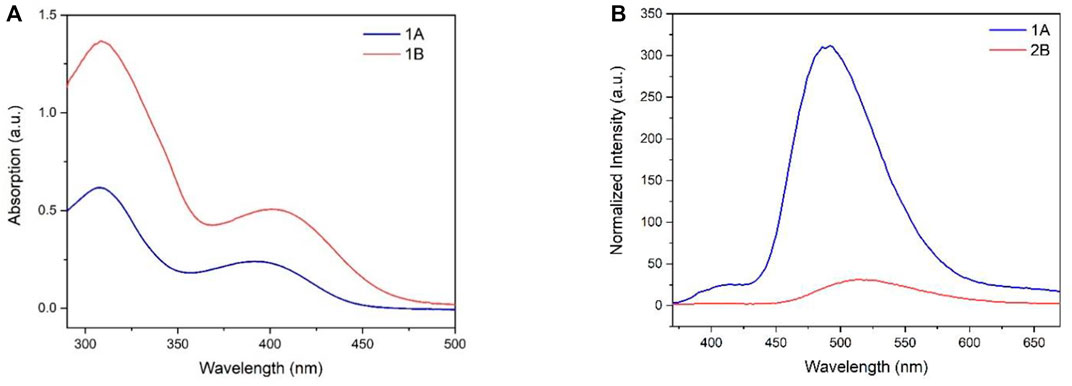
FIGURE 3. (A) UV/Vis absorption spectra of 1A and 1B in THF; c = 50 μg/ml; (B) Normalized fluorescent spectra of 1A and 1B (50 μg/ml) in THF, excitation wavelength: 350 nm.
After we determined their UV-vis, we attempted to determine aggregation-induced emission (AIE) for 1A and 1B, but we found none of them exhibited AIE. This result made it difficult to observe their aggregation in solution via emission spectroscopy directly. As shown in Figure 4 both 1A and 1B displayed positive optical rotation in THF, chloroform, and methanol, respectively. We, thus, decided to systematically investigate the relationship between their aggregation and optical rotation. This work would result in systematic polarization enhancement and adjustment simply by changing cosolvents and provide a new tool to study the chiral aggregation of chiral molecules that do not exhibit AIE.
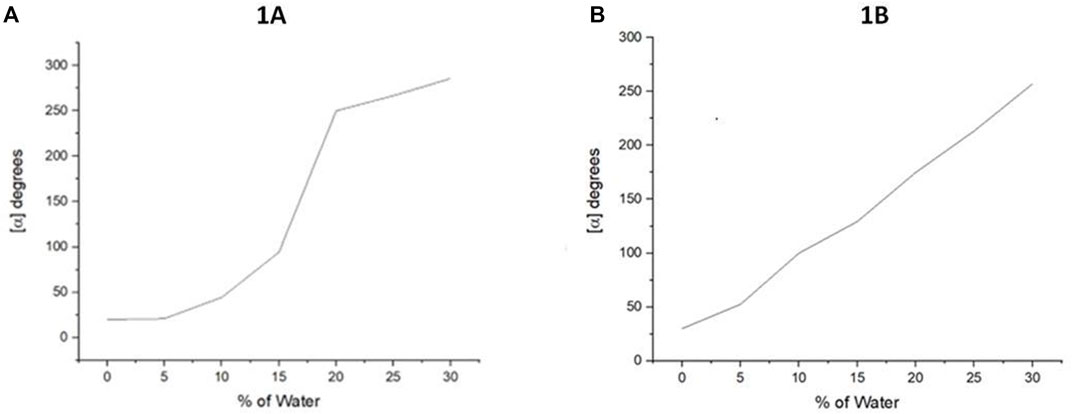
FIGURE 4. Aggregation-induced polarization (AIP) of new multilayer 3D chiral folding oligomers. (A) AIP of chiral oligomer 1A in THF; c = 1 mg/ml. (B) AIP of the chiral oligomer 1B in THF; c = 1 mg/ml.
The popularity of inexpensive polarimeters in most asymmetric synthesis labs makes conducting such an AIP study very convenient. We utilized a Rudolph polarimeter (Rudolph Research Analytical APIV/2W) to acquire optical rotation data of chiral polymers and oligomers at around room temperature with a sodium lamp as the light source. Measurements were performed in a vessel of 2 ml with concentrations (c = 1 mg/ml or 0.5 mg/ml) in THF and its cosolvents. When the fraction of water (fw) reached over 30% or 35%, the optical rotation data often showed a certain degree of instability for most solutions in this study, which would be attributed to the fact that the glass surface tension at the two ends of a vessel is enlarged.
In this measurement, the water fraction (fw) was set at the component of 5% (v/v) on the X-horizontal coordinate corresponding to specific rotation on the Y-vertical ordinate. As revealed in Figure 4 that under the standard aggregation cosolvents of THF and water, we observed a consistent relationship between the optical rotation of 1A and 1B with fw by gradually increasing water fractions in the cosolvents (Figure 4). Oligomer 1A showed a big jump when the water fraction was increased from 15 to 20%. However, its counterpart 1B showed a nearly straight line when fw was increased from 0 to 30%. This trend was not continued beyond 30% fw because of their solubility and optical rotation stability displayed in the instrument. The average data on three measurements for each sample were adopted in plotting the relationship curves in AIP Figures.
After we observed the aforementioned relationship, we checked previous multilayer chiral folding polymers (Figure 5) for the possible aggregation-induced polarization (AIP) (Wu et al., 2021b; Tang et al., 2022; Wang et al., 2022). Convincingly, we found these polymers also displayed noticeable AIP effects, albeit the resulting relationship curves appeared in different shapes (Figures 6–8). Polymer 1A exhibited a nearly straight line similar to oligomer 1B staying flat between 5 and 10%, but a big jump appeared when %water was increased from 10 to 15%. The optical rotation of polymer 2B stayed negative at −6.5° until the water fraction (fw) reached 25% showing positive optical rotation data as 12.2°. There was a big jump between 30 and 35% fw when optical rotation was enhanced from 5.7° to 67.7°, although optical rotation was slightly decreased in plot periods of 5–15%, and 25–30%, respectively.
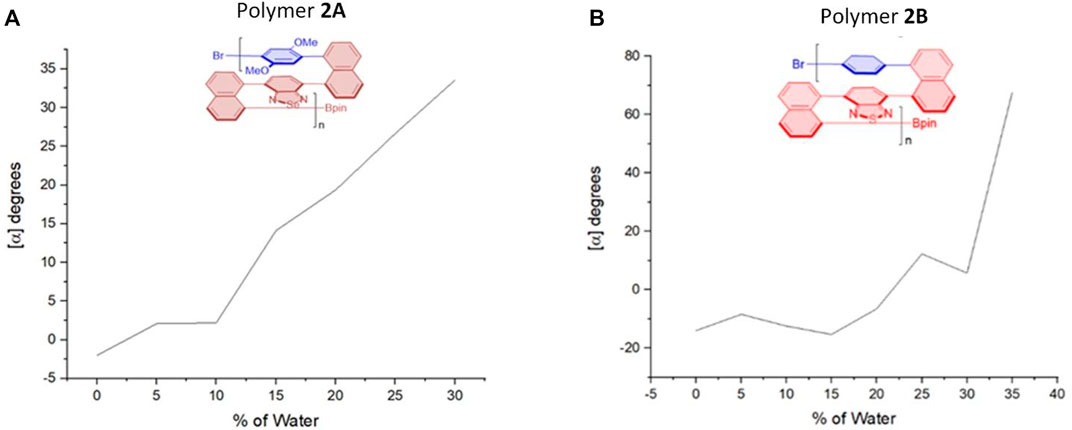
FIGURE 6. Aggregation-induced polarization (AIP) of reported multilayer 3D chiral folding polymers. (A) AIP of chiral folding polymer 2A in THF; c = 1 mg/ml. (B) AIP of chiral folding polymer 2B in THF; c = 0.5 mg/ml.
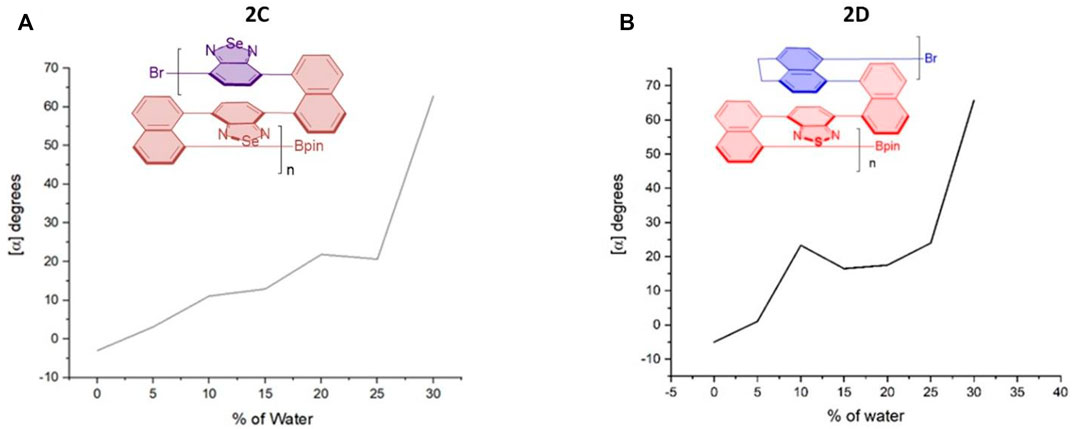
FIGURE 7. Aggregation-induced polarization (AIP) of reported multilayer 3D chiral folding polymers. (A) AIP of chiral folding polymer 2C in THF; c = 1 mg/ml. (B) AIP of chiral folding polymer 2D in THF; c = 1 mg/ml.
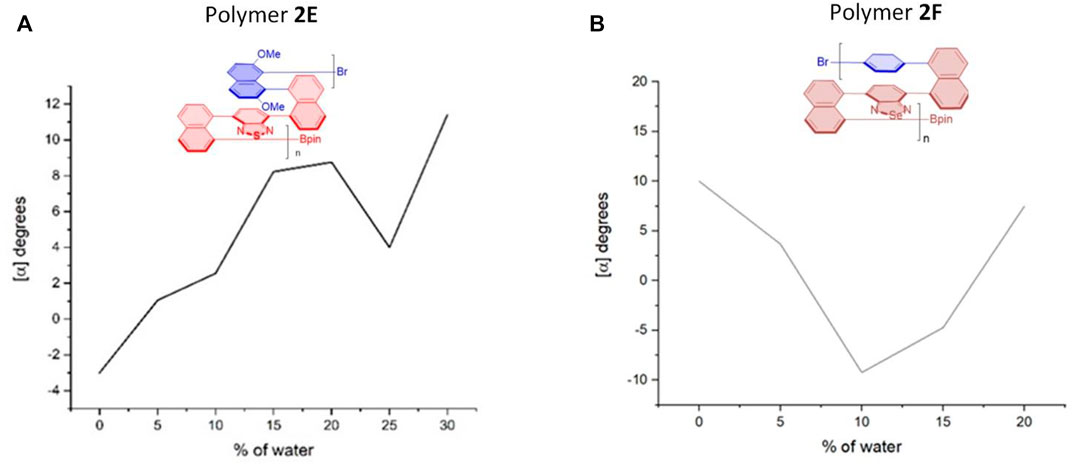
FIGURE 8. Aggregation-induced polarization (AIP) of reported multilayer 3D chiral folding polymers. (A) AIP of chiral folding polymer 2E in THF; c = 1 mg/ml. (B) AIP of chiral folding polymer 2F in THF; c = 1 mg/ml.
For the case of polymer 2C, optical rotation was started with negative data of --3.0o in THF in the absence of water (fw = 0%), but these data turned out to be positive when fw was increased to 5%. The positive data remained afterward until the water fraction reached 30%. Similar to the case of 2B, a big jump came out when fw was increased from 25 to 30%. Polymer 2D also showed an overall enhancement of optical rotation. Two big jumps of its optical rotation were observed when fw was increased from 5 to 20% and then from 25 to 30%, respectively, although the second jump is relatively higher than the first one, which is similar to 2B and 2C cases. Polymer 2D also displayed negative data in THF in the absence of water and showed positive when 5% water was added (Figure 7).
Interestingly, in the cases of polymers 2E and 2F (Figure 8), there are two “V” patterns in their AIP relationship curves. In the former, a smaller “V” appeared between water fractions of 20 and 30%, and a bigger “V” was observed during the whole fw range of 0–20%. While the optical rotation appeared from a negative number at the beginning (fw = 0%) for five cases (2A–2E), the opposite phenomenon was encountered for the case of 2F. Polymer 2F showed a positive optical rotation of 10.0o at the beginning, but quickly dropped to –9.2° when the water fraction was increased to 10%. The optical rotation was then turned in the opposite direction back to 7.5°.
For all of the cases that were examined in this AIP work, it is very difficult to provide a mechanistic explanation at this early stage. The complicated situation was caused by the fact that the multilayer chiral oligomers and polymers exist as complexes of different aggregation sizes. Thus far, there has been no direct correlation between optical rotation and structures of complex mixtures reported in the literature to the best of our knowledge lacking empirical data to study the AIP mechanism in detail. More research turned out to be necessary regarding molecular design and computational and physical organic chemistry in the future.
When the products of oligomers (1A & 1B) and polymers (2D & 2E) did not display aggregation-induced emission (AIE) (Wu et al., 2021b), four other polymers, 2A–2C and 2F, exhibited remarkable AIE in their solutions, as reported in this study. All of these polymers (2A–2F) displayed the optical property of photoluminescence in solids (Wu et al., 2021b; Tang et al., 2022; Wang et al., 2022). In addition, polymers 2A, 2B, 2C, and 2F were also found to exhibit reversible redox properties in electrochemical performance (Tang et al., 2022; Wang et al., 2022).
It should be pointed out that the right- or left-polarized lights generated via AIP is fundamentally different from that through CPL or AIE-based CPL regarding the light sources or polarization mechanisms. In addition, the AIP spectrum shows the relationship between water fraction (fw) and optical rotation ([α]). In contrast, the AIE spectrum represents the relationship between water fraction (fw) and PL intensity. We re-plotted the relationship of AIE polymer 2W and the PL intensity of its monomer with water fractions (fw) based on the maximum PL intensity of each curve (Figure 9), and a similar AIE effect can also be found in polymer 2A. As described in Figure 9, although that the relationships of these properties of 2E with water fraction (fw) are generally along the same direction, its AIP adopted water fractions (fw) until 35% since the glass surface tension at the two ends of the vessel is enlarged during its AIP measurements. Photographs of monomer & 2WE in THF/water system. Reprinted with permission from ref. 21. (Copyright 2021, Wiley-VCH Verlag GmbH & Co. KGaA).
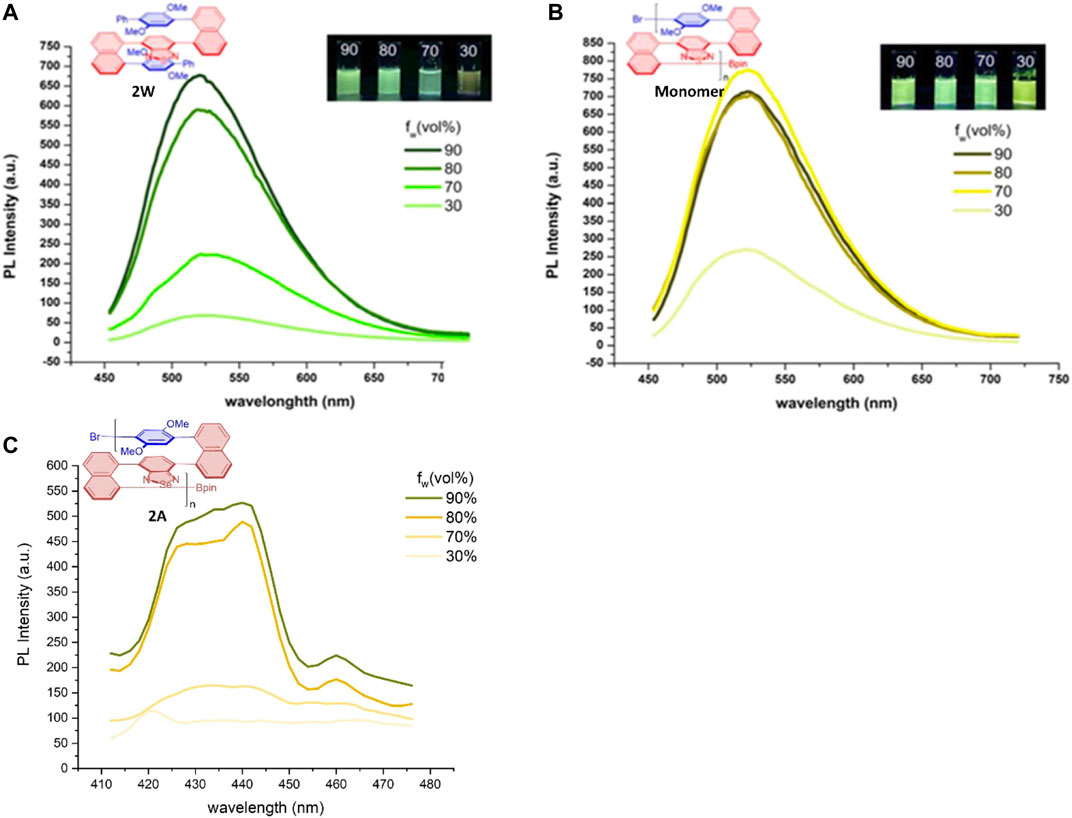
FIGURE 9. Aggregation-induced emission (AIE) of polymers and monomer. (A,B) PL spectra of 2W and its monomer in THF/water mixtures with different water fractions (fw); cmonomer = 0.1 mM, c2w = 50 ug/mL; λex(momomer) = 380 nm, λex (2W) = 385 nm; inset: fluorescence. * (C) PL spectra of 2A in THF/water mixtures with different water fractions (fw); c2A = 50 ug/mL; λex (2A) = 374 nm. * PL spectra of 2W and its monomer is reprinted from ref. 21. (Copyright 2021, Wiley-VCH Verlag GmbH & Co. KGaA).
It is noteworthy that AIE and CPL spectra belong to the molecular spectrum since their polarized left- or right-hand light beams are directly emitted from chiral molecules or chiral aggregates, which belong to emission spectroscopy analysis, and usually give a broad range of wavelengths. In contrast, AIP belongs to transmission spectroscopy analysis since its polarized left- or right-hand light beams are generated by transmitting through the solutions containing chiral compounds (about interaction with externally polarized lights). Its light beams are originally generated from metal or metal filament cycle lamps (external light sources, e.g., Na lamps) with individual wavelengths. A single wavelength of light beams (weak laser) is transmitted inside polarimeter equipped with a filter and detector, which would be treated as indirect light sources. Therefore, AIP would provide an alternative tool for generating left- or right-hand light beams in which the strength of the laser is controlled by atomic spectroscopy sources such as Na lump. We believe that the present AIP work is essential not only for material science in seeking challenging physical properties, but also for chemical reactions and syntheses by taking advantage of aggregate soft matters including chiral ones. Therefore, they would complement each other in research and applications in aggregation science.
Conclusion
In summary, we have established the relationship between the optical rotation of chiral aggregates of multilayered chiral folding oligomers and polymers with water% in THF. The typical aggregation cosolvent systems resulted in optical rotation amplification and adjustment, defined as aggregation-induced polarization (AIP). This phenomenon was discovered during the design and synthesis of novel multilayered chiral oligomers and then found to exist in THF-H2O systems of triple-column/multiple-layer chiral folding polymers. The multilayered chiral oligomers were efficiently synthesized under the asymmetric catalytic systems established by our laboratory recently. These products were well characterized by UV-vis, NMR, and MALDI-TOF spectra. Absolute stereochemistry (enantio- and diastereochemistry) was assigned by comparison with similar asymmetric induction by the same catalyst in our previous reactions. The AIP work can serve as a new tool to determine chiral aggregates, especially those that cannot display emissions. Therefore, AIP and AIE are anticipated to complement each other. In addition, AIP can also complement AIE-based CPL since AIP serves as a new tool providing enhanced right- or left-hand polarized lights with individual wavelengths. It is anticipated to find many applications in chemical and materials sciences in future.
Data Availability Statement
The original contributions presented in the study are included in the article/Supplementary Material, and further inquiries can be directed to the corresponding author.
Author Contributions
GL directed the research and wrote the manuscript. YT, SZ, TX, QY, J-YW, SJ, YW performed and JP, IG, and DC repeated all synthetic experiments and data analysis. GL directly participated in AIP measurements.
Funding
This work was supported by the Robert A. Welch Foundation (D-1361-20210327, United States) and the National Natural Science Foundation of China (22071102 and 91956110).
Conflict of Interest
The authors declare that the research was conducted in the absence of any commercial or financial relationships that could be construed as a potential conflict of interest.
Publisher’s Note
All claims expressed in this article are solely those of the authors and do not necessarily represent those of their affiliated organizations, or those of the publisher, the editors, and the reviewers. Any product that may be evaluated in this article, or claim that may be made by its manufacturer, is not guaranteed or endorsed by the publisher.
Supplementary Material
The Supplementary Material for this article can be found online at: https://www.frontiersin.org/articles/10.3389/fchem.2022.962638/full#supplementary-material
References
Bryliakov, K. P. (2020). Chemical Mechanisms of Prebiotic Chirality Amplification. Research 2020, 5689246. doi:10.34133/2020/5689246
Echeverri, M., Ruiz, C., Gámez-Valenzuela, S., Alonso-Navarro, M., Gutierrez-Puebla, E., Serrano, J. L., et al. (2020). Stimuli-responsive Benzothiadiazole Derivative as a Dopant for Rewritable Polymer Blends. ACS Appl. Mat. Interfaces 12, 10929–10937. doi:10.1021/acsami.9b21209
Feng, H.-T., Liu, C., Li, Q., Zhang, H., Lam, J. W. Y., and Tang, B. Z. (2019). Structure, Assembly, and Function of (Latent)-chiral AIEgens. ACS Mater. Lett. 1, 192–202. doi:10.1021/acsmaterialslett.9b00116
Huang, G., Wen, R., Wang, Z., Li, B. S., and Tang, B. Z. (2018). Novel Chiral Aggregation Induced Emission Molecules: Self-Assembly, Circularly Polarized Luminescence and Copper(ii) Ion Detection. Mat. Chem. Front. 2, 1884–1892. doi:10.1039/c8qm00294k
Idris, I., Tannoux, T., Derridj, F., Dorcet, V., Boixel, J., Guerchais, V., et al. (2018). Effective Modulation of the Photoluminescence Properties of 2,1,3-benzothiadiazoles and 2,1,3-benzoselenadiazoles by Pd-Catalyzed C-H Bond Arylations. J. Mat. Chem. C 6, 1731–1737. doi:10.1039/c7tc05395a
Jin, S., Wang, J.-Y., Tang, Y., Rouh, H., Zhang, S., Xu, T., et al. (2022). Central-to-folding Chirality Control: Asymmetric Synthesis of Multilayer 3D Targets with Electron-Deficient Bridges. Front. Chem. 10, 860398. doi:10.3389/fchem.2022.860398
Jurok, R., Cibulka, R., Dvořáková, H., Hampl, F., and Hodačová, J. (2010). Planar Chiral Flavinium Salts - Prospective Catalysts for Enantioselective Sulfoxidation Reactions. Eur. J. Org. Chem. 2010, 5217–5224. doi:10.1002/ejoc.201000592
Krautwald, S., Sarlah, D., Schafroth, M. A., and Carreira, E. M. (2013). Enantio- and Diastereodivergent Dual Catalysis: α-Allylation of Branched Aldehydes. Science 340, 1065–1068. doi:10.1126/science.1237068
Kumar, A., Bawa, S., Ganorkar, K., Ghosh, S. K., and Bandyopadhyay, A. (2020). Synthesis and Characterization of Acid-Responsive Luminescent Fe(II) Metallopolymers of Rigid and Flexible Backbone N-Donor Multidentate Conjugated Ligands. Inorg. Chem. 59, 1746–1757. doi:10.1021/acs.inorgchem.9b02985
Liu, T.-T., Yan, Z.-P., Hu, J.-J., Yuan, L., Luo, X.-F., Tu, Z.-L., et al. (2021). Chiral Thermally Activated Delayed Fluorescence Emitters-Based Efficient Circularly Polarized Organic Light-Emitting Diodes Featuring Low Efficiency Roll-Off. ACS Appl. Mat. Interfaces 13, 56413–56419. doi:10.1021/acsami.1c16223
Liu, Y., Wu, G., Yang, Z., Rouh, H., Katakam, N., Ahmed, S., et al. (2020). Multi-layer 3D Chirality: New Synthesis, AIE and Computational Studies. Sci. China Chem. 63, 692–698. doi:10.1007/s11426-019-9711-x
Miyaura, N., and Suzuki, A. (1995). Palladium-catalyzed Cross-Coupling Reactions of Organoboron Compounds. Chem. Rev. 95, 2457–2483. doi:10.1021/cr00039a007
Neto, B. A. D., Lopes, A. S., Wüst, M., Costa, V. E. U., Ebeling, G., and Dupont, J. (2005). Reductive Sulfur Extrusion Reaction of 2,1,3-benzothiadiazole Compounds: a New Methodology Using NaBH4/CoCl2·6H2O(cat) as the Reducing System. Tetrahedron Lett. 46, 6843–6846. doi:10.1016/j.tetlet.2005.08.017
Oki, O., Kulkarni, C., Yamagishi, H., Meskers, S. C. J., Lin, Z.-H., Huang, J.-S., et al. (2021). Robust Angular Anisotropy of Circularly Polarized Luminescence from a Single Twisted-Bipolar Polymeric Microsphere. J. Am. Chem. Soc. 143, 8772–8779. doi:10.1021/jacs.1c03185
Shi, Y., Sang, P., Yin, G., Gao, R., Liang, X., Brzozowski, R., et al. (2020). Aggregation‐Induced Emissive and Circularly Polarized Homogeneous Sulfono‐γ‐AApeptide Foldamers. Adv. Opt. Mat. 8, 1902122. doi:10.1002/adom.201902122
Tang, Y., Jin, S., Zhang, S., Wu, G.-Z., Wang, J.-Y., Xu, T., et al. (2022). Multilayer 3D Chiral Folding Polymers and Their Asymmetric Catalytic Assembly. Research 2022, 9847949. doi:10.34133/2022/9847949
Tang, Y., Wu, G., Jin, S., Liu, Y., Ma, L., Zhang, S., et al. (2022). From Center-To-Multilayer Chirality: Asymmetric Synthesis of Multilayer Targets with Electron-Rich Bridges. J. Org. Chem. 87, 5976–5986. doi:10.1021/acs.joc.2c00234
Taniguchi, K., Maeda, R., Ando, T., Okumura, T., Nakazawa, N., Hatori, R., et al. (2011). Chirality in Planar Cell Shape Contributes to Left-Right Asymmetric Epithelial Morphogenesis. Science 333, 339–341. doi:10.1126/science.1200940
Vyskocil, S., Meca, L., Tislerová, I., Císarová, I., Polásek, M., Harutyunyan, S. R., et al. (2002). 2,8'-disubstituted-1,1'-binaphthyls: a New Pattern in Chiral Ligands. Chemistry 8, 4633–4648. doi:10.1002/1521-3765(20021018)8:20<4633::AID-CHEM4633>3.0.CO;2-N
Wang, A. H.-J., Fujii, S., van Boom, J. H., and Rich, A. (1983). Right-handed and Left-Handed Double-Helical DNA: Structural Studies. Cold Spring Harb. Symposia Quantitative Biol. 47 (Pt 1), 33–44. doi:10.1101/sqb.1983.047.01.006
Wang, J. Y., Tang, Y., Wu, G. Z., Zhang, S., Rouh, H., Jin, S., et al. (2022). Asymmetric Catalytic Assembly of Triple‐Columned and Multilayered Chiral Folding Polymers Showing Aggregation‐Induced Emission (AIE). Chem. A Eur. J. 28, e202200183. doi:10.1002/chem.202200183
Wu, G., Liu, Y., Rouh, H., Ma, L., Tang, Y., Zhang, S., et al. (2021a). Asymmetric Catalytic Approach to Multilayer 3D Chirality. Chem. Eur. J. 27, 8013–8020. doi:10.1002/chem.202100700
Wu, G., Liu, Y., Yang, Z., Jiang, T., Katakam, N., Rouh, H., et al. (2020). Enantioselective Assembly of Multi-Layer 3D Chirality. Natl. Sci. Rev. 7, 588–599. doi:10.1093/nsr/nwz203
Wu, G., Liu, Y., Yang, Z., Katakam, N., Rouh, H., Ahmed, S., et al. (2019). Multilayer 3D Chirality and its Synthetic Assembly. Research 2019, 11. doi:10.34133/2019/6717104
Wu, G., Liu, Y., Yang, Z., Ma, L., Tang, Y., Zhao, X., et al. (2021b). Triple-columned and Multiple-Layered 3D Polymers: Design, Synthesis, Aggregation-Induced Emission (AIE), and Computational Study. Research 2021, 3565791. doi:10.34133/2021/3565791
Xu, H., Liu, L., Teng, F., and Lu, N. (2019). Emission Enhancement of Fluorescent Molecules by Antireflective Arrays. Research 2019, 3495841. doi:10.34133/2019/3495841
Zhang, J., and Kürti, L. (2021). Multi-layer 3D Chirality: its Enantioselective Synthesis and Aggregation-Induced Emission. Natl. Sci. Rev. 8, nwaa205. doi:10.1093/nsr/nwaa205
Zhao, T., Han, J., Duan, P., and Liu, M. (2020). New Perspectives to Trigger and Modulate Circularly Polarized Luminescence of Complex and Aggregated Systems: Energy Transfer, Photon Upconversion, Charge Transfer, and Organic Radical. Acc. Chem. Res. 53, 1279–1292. doi:10.1021/acs.accounts.0c00112
Keywords: chiral aggregates, multilayer folding chirality, multilayer folding oligomer, aggregation-induced polarization (AIP), multilayer folding polymer
Citation: Tang Y, Zhang S, Xu T, Yuan Q, Wang J-Y, Jin S, Wang Y, Pan J, Griffin I, Chen D and Li G (2022) Aggregation-Induced Polarization (AIP): Optical Rotation Amplification and Adjustment of Chiral Aggregates of Folding Oligomers and Polymers. Front. Chem. 10:962638. doi: 10.3389/fchem.2022.962638
Received: 06 June 2022; Accepted: 24 June 2022;
Published: 12 August 2022.
Edited by:
Zhendong Jin, The University of Iowa, United StatesReviewed by:
Shifa Zhu, South China University of Technology, ChinaWanbin Zhang, Shanghai Jiao Tong University, China
Copyright © 2022 Tang, Zhang, Xu, Yuan, Wang, Jin, Wang, Pan, Griffin, Chen and Li. This is an open-access article distributed under the terms of the Creative Commons Attribution License (CC BY). The use, distribution or reproduction in other forums is permitted, provided the original author(s) and the copyright owner(s) are credited and that the original publication in this journal is cited, in accordance with accepted academic practice. No use, distribution or reproduction is permitted which does not comply with these terms.
*Correspondence: Guigen Li, guigen.li@ttu.edu
‡Undergraduate participant
†These authors have contributed equally to this work