Synthesis methods of 1,2,3-/1,2,4-triazoles: A review
- School of Pharmacy, Key Laboratory of Molecular Pharmacology and Drug Evaluation (Yantai University), Ministry of Education, Collaborative Innovation Center of Advanced Drug Delivery System and Biotech Drugs in Universities of Shandong, Yantai University, Yantai, China
Triazole, comprising three nitrogen atoms and two carbon atoms, is divided into two isomers 1,2,3-triazole and 1,2,4-triazole. Compounds containing a triazole are one of the significant heterocycles that exhibit broad biological activities, such as antimicrobial, analgesic, anti-inflammatory, anticonvulsant, antineoplastic, antimalarial, antiviral, antiproliferative, and anticancer activities. A great quantity of drugs with a triazole structure has been developed and proved, for example, ketoconazole and fluconazole. Given the importance of the triazole scaffold, its synthesis has attracted much attention. This review summarizes the synthetic methods of triazole compounds from various nitrogen sources in the past 20 years.
Introduction
Triazole is an important nitrogen heterocyclic compound, containing three nitrogen atoms and two carbon atoms. It had reported that compounds containing triazole have an important application value in various fields, such as (Brüggemann et al., 2022; Fallah et al., 2022; Sharma et al., 2022) agrochemistry (Georgiadis et al., 2018; Musarurwa and Tavengwa, 2021; Valencia-Quintana et al., 2022) and material chemistry (Scattergood et al., 2017; Rodrigues et al., 2020; Ahmed and Xiong, 2021). Its unique structure facilitates the formation of a variety of non-covalent bonds with enzymes and receptors inducing broad-spectrum biological activities, such as anticancer (Sharma et al., 2022), antituberculosis (Keri et al., 2015; Zhang et al., 2017), antibacterial (Gao et al., 2019; Zhang, 2019; Strzelecka and Swiatek, 2021), and anti-HIV (Patel et al., 2012).
There are two isomeric forms of triazole, namely, 1,2,3-triazole and 1,2,4-triazole (Figure 1A). Their derivatives have been widely applied in many medicinal scaffolds (Figure 1;2 and (c)). For example, Itraconazole (Navarro-Triviño, 2021; Neto et al., 2022), Fluconazole (Elzoheiry et al., 2022; Gao et al., 2022), and Voriconazole (Shettar et al., 2021; Lindsay et al., 2022) are commonly used antifungals in clinical application. Ribavirin (Burman et al., 2022; Tian et al., 2022) is a broad-spectrum antiviral drug used in the treatment of hepatitis. Rizatriptan (Moore, 2007; Chokshi et al., 2019) has been incorporated into drug candidates as antimigraine agents. Letrozole (Park et al., 2021; Slomovitz et al., 2022), anastrozole (Kucukguzel and Cikla-Suzgun, 2015), and vorozole (Kim et al., 2009; Takahashi et al., 2011) as anticancer drugs are very effective. In addition, CAI and cefatrizine (Lucht et al., 1996) are very successful as anticancer drugs. Rufinamide (Caraballo et al., 2020; Sharawat et al., 2021) was approved by FDA for the treatment of pediatric epilepsy. Tazobactam (Pfaller et al., 2021; Ramassamy et al., 2021) has been successfully used in antibiotic therapy. TSAO (Dheer et al., 2017) is used in inhibition of HIV reverse transcriptase.
Given the importance of the triazoles, their synthesis has attracted much attention. Under thermal conditions, 1,3-dipolar cycloaddition between azide and terminal alkyne (also known as Watson cycloaddition) is the most popular reaction, which is suitable for producing 1,2,3-triazole moiety (Di Antonio et al., 2012) (Figure 2). However, it was only used to prepare 1,2,3-trizole in early stage due to weak regioselectivity (1,4- or 1,5-disubstituted-1,2,3-triazole), bad chemical yield, and high temperature. This method was reported at the start of the 20th century, but its specific mechanism was described by Huisgen et al. (1967). The click chemistry method of the Cu-catalyzed azide–alkyne cycloaddition reaction (CuAAC) proposed by Sharples can synthesize 1,2,3-triazole in high yield (Kolb et al., 2001) (Figure 2). As a major example of click chemistry, the CuAAC reaction has a great impact on organic synthesis and widely used in industry and academia. In view of the important application of triazole scaffolds in pharmaceutical intermediates, it is very significant to find a more effective synthetic method. Others reviews published successively in recent years about 1,2,3- or 1,2,4-triazole compounds, which focused on summarizing the structure–activity relationships (SAR) and synthetic strategy (Matin et al., 2022; Moura and Tomé, 2022; Nemallapudi et al., 2022). In addition, there are also some reviews focused on the synthesis of mono-/bis-aminomercapto and fused 1,2,4-triazole derivatives (Gomha et al., 2015; Riyadh and Gomha, 2020; Riyadh et al., 2022). In this review, combined with the progress of triazole synthesis in past 20 years, several main synthetic methods from various nitrogen sources are summarized. The synthetic methods were focused on the key starting material used for the production of the 1,2,3-/1,2,4-triazole skeleton.
Synthesis of 1,2,3-triazoles
1,2,3-Triazole has strong stability for thermal and acid condition; otherwise, it is insensitive to redox, hydrolysis, and enzymatic hydrolase (Motornov et al., 2018). The main synthetic methods include sodium azido, trimethylsilyl azido, alkyl/aryl azido, hydrazone, sulfonamide, hydrazine, and diazo-compounds used as raw materials to provide nitrogen atoms for 1,2,3-triazole derivatives.
Sodium azide as nitrogen source
In 2013, a simple method to synthesize bis(1,2,3-triazole) and 5-alkynyl-1,2,3-triazole from alkyne and azido at various temperatures was reported (Li et al., 2013). Various azidoes and alkynes as substrates participated in reactions, which had been successfully used in synthesis of bioactive nucleoside analogues. In this method, based on the temperature effect, copper bromide is used as a catalyst in the absence of a ligand. Between 0oC and 70°C, the yield of bis(1,2,3-triazole) increased with the decrease of reaction temperature and reached 91% (Li et al., 2013) after stirring for 20 h at 0°C. The opposite phenomenon: the yield of 5-alkynyl-1,2,3-triazole was highest (68%) at 60°C. As the temperature increases, the yield increases, and 60°C is the optimum temperature (Scheme 1A).
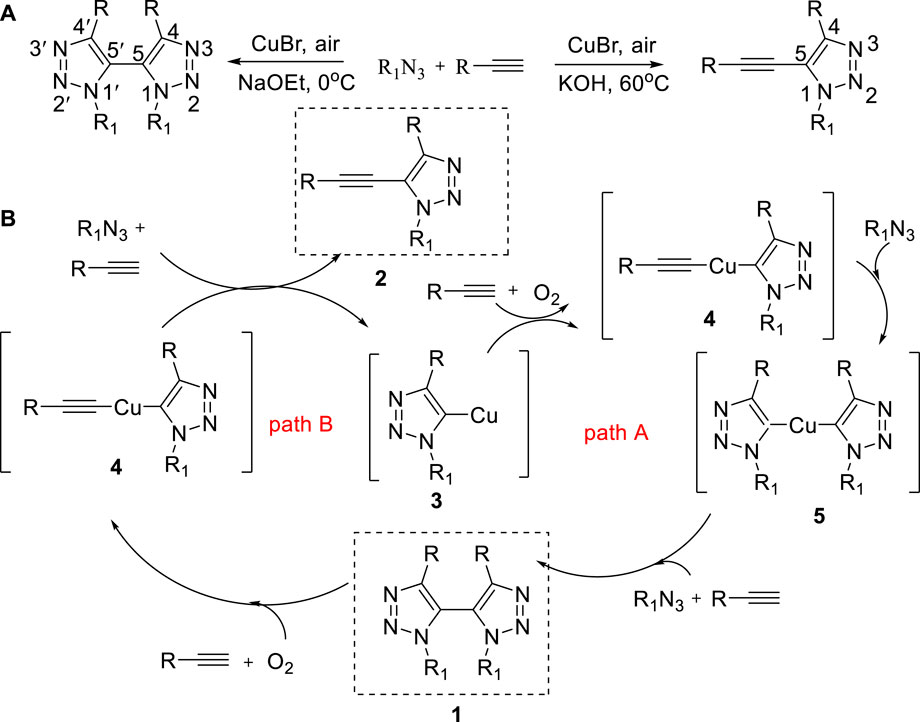
SCHEME 1. (A) Synthesis of bis(1,2,3-triazole) and 5-alkynyl-1,2,3-triazole by temperature regulation. (B) Possible mechanism of synthesis of bis(1,2,3-triazole) and 5-alkynyl-1,2,3-triazole.
A possible mechanism of the temperature-mediated reaction is described in Scheme 1B. The triazolyl–copper complex intermediate 3 was generated by the CuAAC reaction. The tandem aerobic oxidative coupling reaction might be the key of this reaction. In addition, temperature might affect the reactivity of the triazolyl–copper complex 4 and 5. At 0°C, the aerobic oxidative coupling reaction could react preferentially by Path A to construct bis(1,2,3-triazole) 1. Meanwhile, at 60°C, the aerobic oxidative coupling reaction could react preferentially by Path B to construct 5-alkynyl-1,2,3-triazole 2.
In 2008, Kalisiak reported an efficient three-component synthetic pathway for the production of 2-hydroxymethyl-2H-1,2,3-triazoles through Cu-catalyzed cycloaddition of terminal alkyne, NaN3, and formaldehyde in one pot (Jarosław Kalisiak and Fokin, 2008; Tian et al., 2022). Using copper as a catalyst, acetic acid was added in equal amounts and 1,4-dioxane was used as the solvent. At room temperature, yields ranged from 67% to 95% (Jarosław Kalisiak and Fokin, 2008; Tian et al., 2022). Due to the advantages of wide range of substrates and high compatibility of functional groups, the target products could be diversified (Scheme 2A).
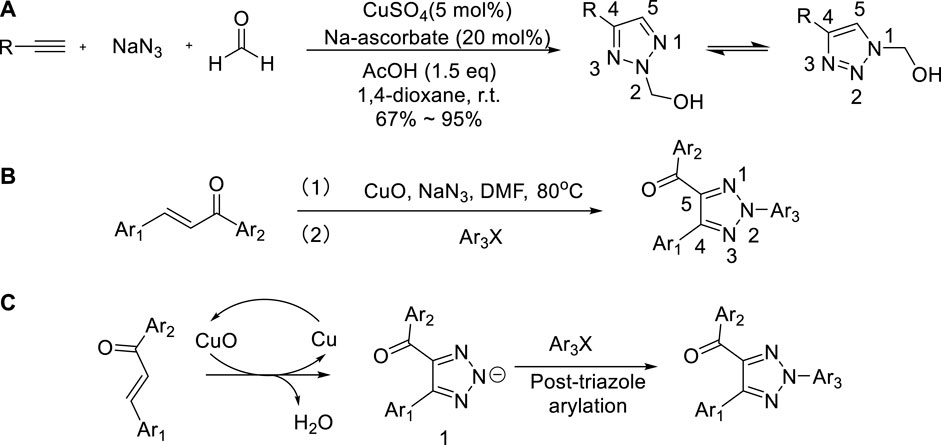
SCHEME 2. (A) Synthesis of 1,4- or 2,4-disubstituted-1,2,3-triazoles. (B) “One pot” three components synthesis of N2-substituted-1,2,3-triazole. (C) Possible mechanism of synthesis of the framework (b).
In 2012, the “one pot” three components preparation of N2-substituted-1,2,3-triazole with chalcone, sodium azide, and halogenated aromatics as raw materials through cuprous oxide was proposed by Chen et al. (Zhang et al., 2012). The method could prepare selectively N2-substituted-1,2,3-triazoles and yield achieved was 98% (Zhang et al., 2012). It was an efficient strategy for multiple component condensation sequences about the combination of azide–chalcone oxidative cycloaddition and post-triazole arylation presented in diversity-oriented Scheme 2B. The mechanism study showed that cuprous oxide was used as an oxidant and a primer to accelerate the reaction. Corresponding products were obtained via nitrogen anion intermediates arylated with halogenated aromatics (Scheme 2C).
Trimethylsilyl azide as nitrogen source
As an important nitride, trimethylsilyl azide is often used to synthesize various nitrogen-containing compounds. In 2003, Shin Kamijo used palladium/copper as a bimetallic catalyst to prepare 1,2,3-triazole based on the three-component cycloaddition reaction of alkynes, allyl methyl carbonate, and TMSN3 (Shin Kamijo et al., 2003). Using ethyl acetate as a solvent, 2-allyl-substituted-1,2,3-triazoles were obtained with moderate yield (56%–75%)(Shin Kamijo et al., 2003) at 100°C (Scheme 3A).
In 2016, another three-component reaction of alkynes, TMSN3, and dimethyl sulfoxide for synthesis of 1,2,3-triazole was developed (Phanindrudu et al., 2016). Kumar Tiwari et al. used nano-Cu0/Fe3O4 as an efficient catalyst for the regioselective synthesis of 1,2,3-triazoles by one-pot tandem; the yield was up to 96% (Phanindrudu et al., 2016). The reaction could be compatible with a variety of alkynes such as aromatic, aliphatic, heteraromatic, and polyaromatic compounds. The advantages of wide range of substrates and simple operation provided an attractive and convenient route for the synthesis of thiotriazole (Scheme 3B).
In 2019, Min Zhang et al. extended the substrate and employed silver sulfate as a catalyst, which showed the preparation of N1-vinyl-1,2,3-triazole by a three-component reaction of alkynes, TMSN3, and 1,3-diketone in high yield (82%)(Chen et al., 2019). N1-vinyl-1,2,3-triazoles were obtained using NaHCO3 as a base at 70°C. This method was simple to operate and had good compatibility with functional groups of substrates, providing an effective synthesis method for N1-vinyl-1,2,3-triazoles (Scheme 3C).
In 2019, Pengli Bao developed a similar reaction condition for selective production of 1,4- and 2,4-disubstitued-1,2,3-triazoles reaction of three-component of alkynes, TMSN3, and ethers by copper catalysis (Bao et al., 2019). The target products were obtained in high yield (86%) (Bao et al., 2019) at 80°C using tert-butanol peroxide as an oxidant and ether as a solvent and reactant. In addition, the synthesis of 1,4- and 2,4- disubstitued-1,2,3-triazole could be selectively regulated by changing the amount of cuprous chloride. This method featured high regioselectivity, good functional group tolerance, and a wide substrate scope (Scheme 3D).
The possible reaction mechanism of these reactions is shown in Scheme 3E. AgN3 was prepared based on anion interchange of TMSN3 with Ag2SO4 and AgOH in the wake of the liberation of (TMS)2SO4 and TMSOH in a basic solution. Then vinyl azide 2 was obtained because of AgN3 insertion into the C-C triple bond via protodemetalation of vinylsilver 1 with H2O. Then 2 underwent 1,3-dipolar cycloaddition with the tautomerization of 1,3-dicarbonyl compound in basic conditions, which generated the coupling adduct 3. Finally, the triazole products were passed through the dehydration-driven aromatization process.
In 2015, Ning Jiao described an effective Cu-catalyzed nitrogenation of alkynes and alkenes for the rapid synthesis of sulfur-containing triazoles (Shen et al., 2015). Available sulfoxides and azides were employed as the S- and N-source to readily prepare highly value N- and S-containing 1,2,3-trizoles. A direct and practical solution was provided to gain sulfur-containing triazoles in this method (Scheme 4).
Alkyl/Aryl azido as nitrogen source
In 2009, a simple method for the synthesis of 1,4-disubstituted-1,2,3-triazole from ethyl propionate was developed by Zhang et al. (2009). Sodium ascorbate and CuI were added to the suspension of 3-(4-azolophenyl) acrylic acid in CH3CN/H2O (9:1V/V) and stirred for 120 min under nitrogen atmosphere at air. 1-[4-(2-carboxyl vinyl) phenyl]-1,2,3-triazole-4-carboxylic acid ethyl ester could be prepared with high yield (90%)(Zhang et al., 2009) (Scheme 5A).
The substrates were large expanded based on aforementioned experiment. In 2015, the approach was reported by Zhenghu Xu which involved the production of multiple types of 1,4,5-trisubstituted-1,2,3-triazoles by Cu/Pd-catalyzed azide, alkyne, and aryl halide three-component reactions (Wei et al., 2015). 1,4,5-Trisubstituted-1,2,3-triazoles were assembled rapidly in one step with high yield (88%–98%)(Wei et al., 2015) and good regioselectivity using this route, which made up for the limitation of the traditional method CuAAC which was only suitable for the synthesis of triazoles from terminal alkynes (Scheme 5B).
In the following year, Zhenghu Xu further developed the method to construct N-alkyl-5-sulfur/selenium/amino-1,2,3-triazoles via using Cu-catalyzed alkyne, alkyl azide, and thiosulfonate or selenesulfonic ester (Wang et al., 2016). The reaction was reacted under mild conditions, with a broad scope of substrates, and various fatty mercaptols and aromatic thiophenols were well compatible with the conditions (Scheme 5C).
In 2019, Miha Virant et al. described an effective synthesis of 1,3,4-trisubstituted-1,2,3-triazole involving alkyne, azide, and aryl iodonium salt with the yield of 83% (Virant and Kosmrlj, 2019). The reaction had the advantages of simple operation and broad scope of substrates, which supplied a robust, selective, and effective method for the synthesis of various bioactive 1,3,4-triaryl-1,2,3-triazolium salts (Scheme 5D).
In 2018, a copper-catalyzed three-component reaction of alkynic acid, diesel ether, and azide to produce N-alkyl-5-seleno-1,2,3-triazoles was described by Wang et al. (2018). Using copper acetate as a catalyst, N-alkyl-5-seleno-1,2,3-triazolium compounds could be obtained efficiently at 120°C. The reaction has the characteristics of simple operation, high regioselectivity, and good compatibility under air. A variety of N-alkyl-5-seleno-1,2,3-triazole compounds have been synthesized to show effective anticancer activity in vitro (Scheme 6A).
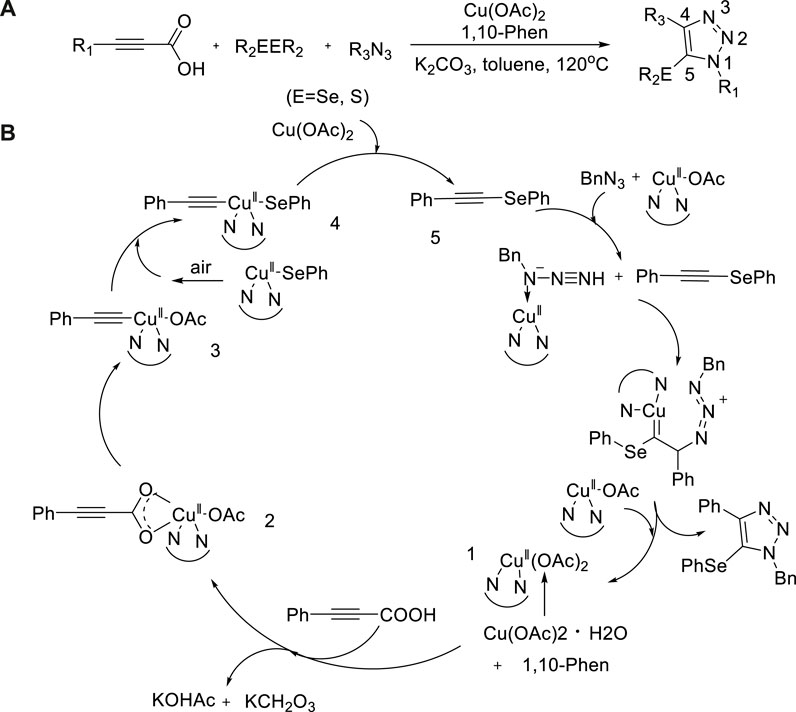
SCHEME 6. (A) Synthesis of N-alkyl-5-arylselanyl-1,2,3-triazoles. (B) Plausible mechanism of synthesize N-alkyl-5-seleno-1,2,3-triazoles.
A possible mechanism is described in Scheme 6B. First, 1,10-phenanthroline reacted with Cu(OAc)2·H2O to form the active Cu(II) intermediate 1 that then reacted with phenylacetic acid, further underwent decarboxylation, and CO2 was released to form 3. Subsequently, 4 reacting with diphenyl diselenide following reductive elimination to generate phenyl(phenylethynyl)selane 5, as well as copper(I) species. The complexation of copper intermediate with phenyl(phenylethynyl)-selane and -azide, which underwent oxidative cyclization, turned into products under air atmosphere to finish the reaction.
In 2015, Chen et al. reported the synthesized method of 4-NO2-1,5-trisubstituted-1,2,3-triazoles from nitroolefins and organic azides by copper-catalyzed [3+2] cycloaddition or oxidation reaction (Chen et al., 2015). At 110°C, 4-NO2-1,5-trisubstituted-1,2,3-triazoles were prepared at high yield (96%) (Chen et al., 2015). The reaction was simple to operate, and the substrate was suitable for a wide range, which provided an effective route for the synthesis of 1,2,3-triazoles (Scheme 7A).
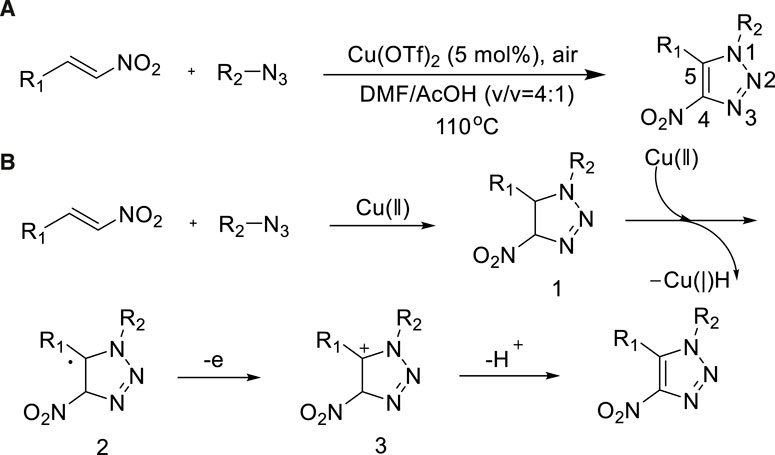
SCHEME 7. (A) Synthesis of 4-NO2-1,5-trisubstituted-1,2,3-triazoles. (B) Plausible mechanism for synthesis of 4-NO2-1,5-trisubstituted-1,2,3-triazoles.
A probable mechanism of copper-catalyzed [3+2] cycloaddition or oxidative reaction is proposed in Scheme 7B. The regioselective 1,3-dipolar cycloaddition took place between nitro-olefin and azide to form the triazole intermediate 1; transition metal complexes could promote this process. Then 2 is obtained through Cu(II) catalyst, which could be stabilized with the help of an Ar group. The cation intermediate 3 was given because of this the radical lost another electron; then, 4-NO2-1,5-trisubstituted-1,2,3-triazoles would be formed via cation intermediate 3 losing a proton. In this reaction, The Cu(II) as a catalyst play an important role, which could be regenerated in acidic condition from Cu(I) species with O2.
In 2018, Atul Kumar et al. showed that Cu-catalyzed decarboxylation of cinnamic acid with aryl azide to construct 1,5-disubstituted-triazoles (Kumar et al., 2018). Using Cu(OTf)2 as a catalyst and ascorbic acid/DMF as a solvent, 1,5-disubstituted-1,2,3-triazoles were obtained in good yield (80%) (Kumar et al., 2018) at 115°C. The adaptability of various aryl-substituted azides and cinnamic acid substrates was investigated, and it was found that various functional groups including halogen, nitro group, and cyanide group could be applied under the reaction conditions (Scheme 8A).
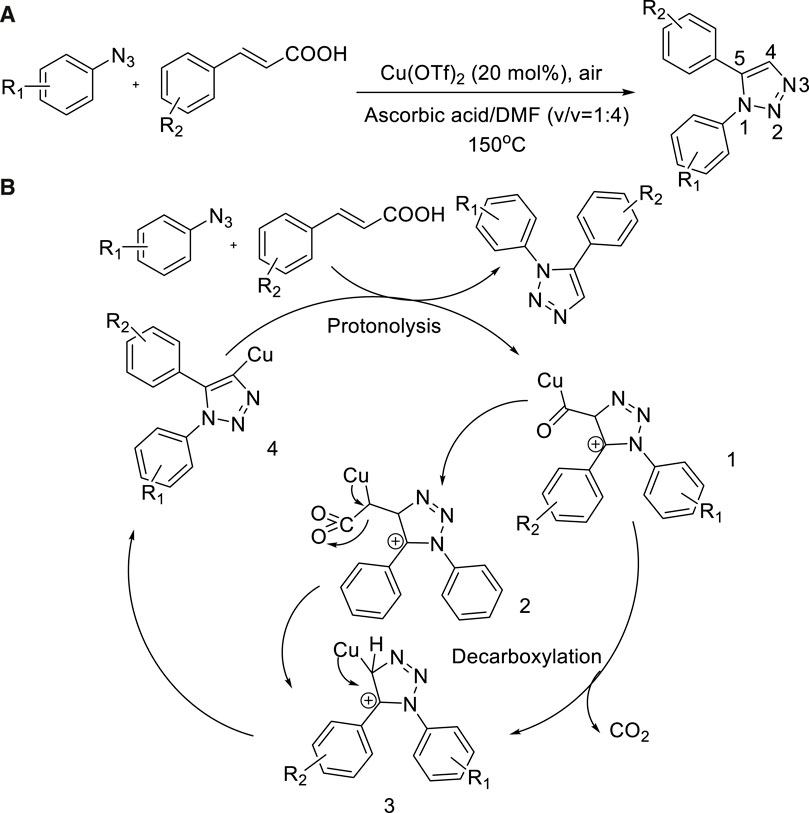
SCHEME 8. (A) Synthesis of 1,5-disubstituted-1,2,3-triazoles. (B) Plausible mechanism for Cu(II)-catalyzed 1,5-disubstituted-1,2,3-triazoles synthesis.
The proposed mechanistic pathway is shown in Scheme 8B: First, the regioselective 1,3-dipolar cycloaddition arose with azides and cinnamic acid to form the cation intermediate 1. Subsequently, 2 got passed decarboxylation to form copper triazoline 3 and then copper complex 1,4,5-trisubstituted-1,2,3-triazoles 4 were received because intermediate 3 lost a proton. The free 1,5-disubstituted-1,2,3-triazoles were provided due to 4 was easily undergone protonolysis. Also, it is important that this pathway allowed the Cu(II) species to rise again under the acidic media.
Hydrazone as nitrogen source
In recent years, a method of synthesis N-substituted-1,2,3-triazoles from hydrazones instead of azides had been developed. In addition, 30 years ago, Sakai developed a fascinating scheme for the production of 1,2,3-triazole via the condensation of an amine and α, α-dichlorotosyl hydrazone in mild reaction conditions. The approach does not need a metal catalyst and azide, but this pathway had not been used adopted widely for a long period (Sakai et al., 1986).
In 2010, Hanselmann further applied the cyclization reaction of α, α-dichlorotoluene sulfonyl–substituted hydrazone with primary amine mediated by DIPEA to synthesize N1-substituted-1,2,3-triazoles (Roger Hanselmann et al., 2010). N-substituted-1,2,3-triazoles with antibacterial activity can be synthesized in an ethanol solvent by this method. The key 1,2,3-triazole skeletons could be easy obtained under the presence of DIPEA on a large scale with the yield of 80% (Roger Hanselmann et al., 2010), which avoided potential safety concerns (Scheme 9A).
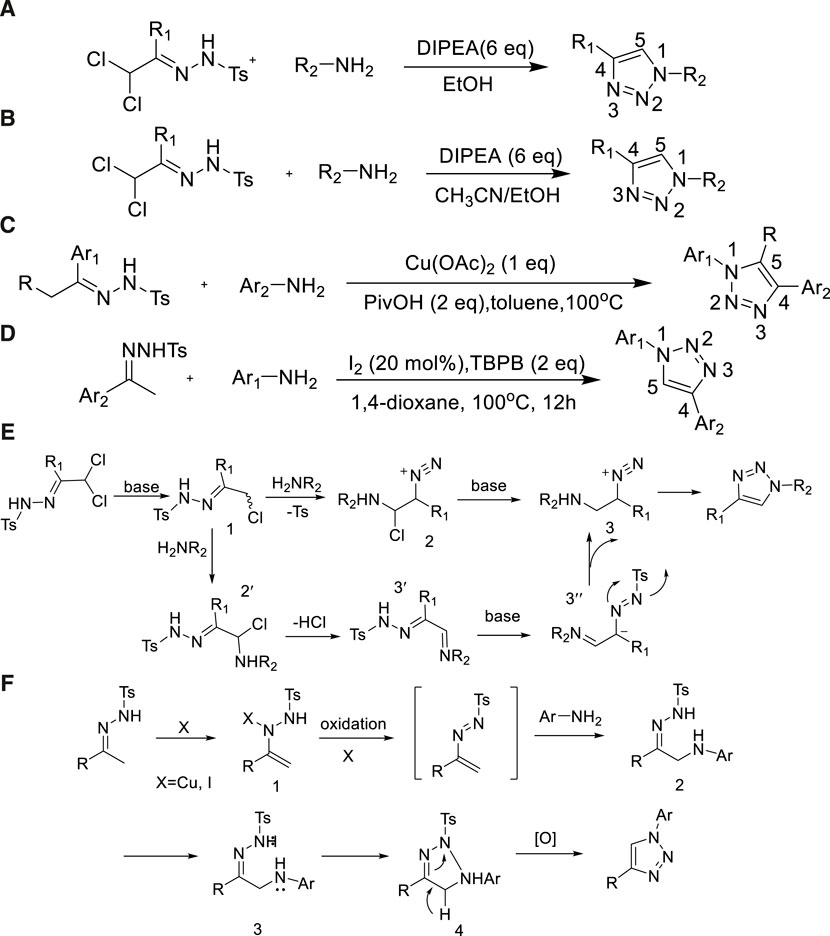
SCHEME 9. Synthesis of N-substituted-1,2,3-triazole framework and the mechanism of N-substituted-1,2,3-triazole framework.
Subsequently, Berkel et al. improved the reaction conditions by adopting a mixed solvent of acetonitrile and ethanol to broaden the range of reaction substrates (van Berkel et al., 2012) (Scheme 9B).
In 2013, Zhang showed the method to synthesis 1,4,5-trisubstituted-1,2,3-triazoles by copper-mediated reaction of N-tosylhydrazones and aniline with the yield of 82% (Chen et al., 2013). Significant features of this scheme involved the unique constituent of 1,4- or 1,4,5-substituted regioisomers, the advantages of wide scope with regard to low cost of reagents, and the convenient operating conditions for the N-tosylhydrazones and the aniline substrates (Scheme 9C).
In 2014, an efficient method for the synthesis of 1,4-diaryl-1,2,3-triazoles from N-tosylhydrazones with anilines under the mediation of I2/TBPB (terbutyl benzoate peroxide) system was reported by Cai et al. (2014). The method used I2 as a catalyst and TBPB as an oxidant without any metal catalyst. A variety of 1,4-diaryl-1,2,3-triazoles could be produced with moderate to good yields (89%) (Chen et al., 2013) (Scheme 9D).
The general mechanism of Scheme 9A,B was depicted as Scheme 9E. First, α, α-dichlorotosyl hydrazone was converted into vinyldiazine 1 that passed the process of elimination of HCl; intermediate 2 was formed via the amine addition and toluenesulfinate ion elimination. Finally, 1,2,3-triazoles were produced through deprotonation and cyclization.
The preliminary mechanism of Scheme 9C,D was depicted as Scheme 9F. The N-tosylhydrazone might isomerize to 1. Subsequently, 1-tosyl-2-vinyldiazene 2 was given via N-tosylhydrazone oxidation and then the intermediate 3 was produced by an aza-Michael addition with aniline. Finally, the 1,2,3-triazole derivatives were prepared through the Cu/I-catalyzed cyclization of 3 with the formation of a N-N bond and subsequent aromatization. A radical process occurred in the reaction.
In 2012, Guru developed a copper-catalyzed production of 2,4,5-triaryl-1,2,3-triazoles from bis(arylhydrazones) under a mild condition (Guru and Punniyamurthy, 2012). In this method, a variety of N2-aryl-1,2,3-triazoles were produced by continuous C-H functionalization and C-C, N-N, and C-N bond formation reactions with Cu(OAc)2 as a catalyst. This scheme took place under mild reaction conditions, could be utilized in large synthesis range, and possessed wide functional compatibility with easily available substrates (Scheme 10).
Hydrazine as nitrogen source
Hydrazine, as an important chemical raw material, is often used to construct various nitrogen-containing compounds.
In 2014, Zhang et al. reported the nonmetal-mediated synthesis of 1,4-disubstituted-1,2,3-triazole by the three-component reaction of aniline, aromatic ketone, and 4-methylbenzenesulfonohydrazide (Chen et al., 2014). In this method, various types of 1,4-disubstituted-1,2,3-triazoles were prepared which exhibited good to excellent yield (75%–92%)(Chen et al., 2014) by successive formation of C-N and N-N bonds. The reaction condition was mild and did not require metal reagent and azide providing nitrogen atom. (Scheme 11A).
In 2015, Wan Jiping expanded the scope of substrate, developed a method to construct 1,2,3-triazoles from enamine–ketone, primary amine and p-toluene sulfonyl hydrazine catalyzed by non-metallic iodine (Wan et al., 2015). The strategy was accomplished by continuous C-N bond and N-N bond formation and acyl migration, and multiple N1-substituted-1,2,3-triazoles were synthesized with good yields (78%)(Wan et al., 2015) (Scheme 11B).
A plausible pathway is proposed (Scheme 11C). Phenylglyoxal intermediate 1 was created by Kornblum oxidation with the aniline in the presence of iodine and DMSO. The 1 passed through condensation with the aniline to obtain the C-acyl imine intermediate 2, which passed by condensation with N-tosylhydrazine formed 3. Subsequently, the triazole products were obtained by intermediate 3 out of cyclization and aromatization under molecular iodine or O2.
Diazo compounds as nitrogen source
In 2015, a series of N1-substituted-1,2,3-triazoles were prepared through Cu-catalyzed [3+2] cycloaddition reaction of secondary amines and diazo compounds by Li et al. (Li et al., 2015). This reaction used oxygen as a green oxidant and had the advantages of easy availability of raw materials, mild conditions and good compatibility of functional groups. It provided a practical synthesis method for a series of N1-substituted-1,2,3-triazoles in 55%–84% yields (Li et al., 2015) (Scheme 12A).
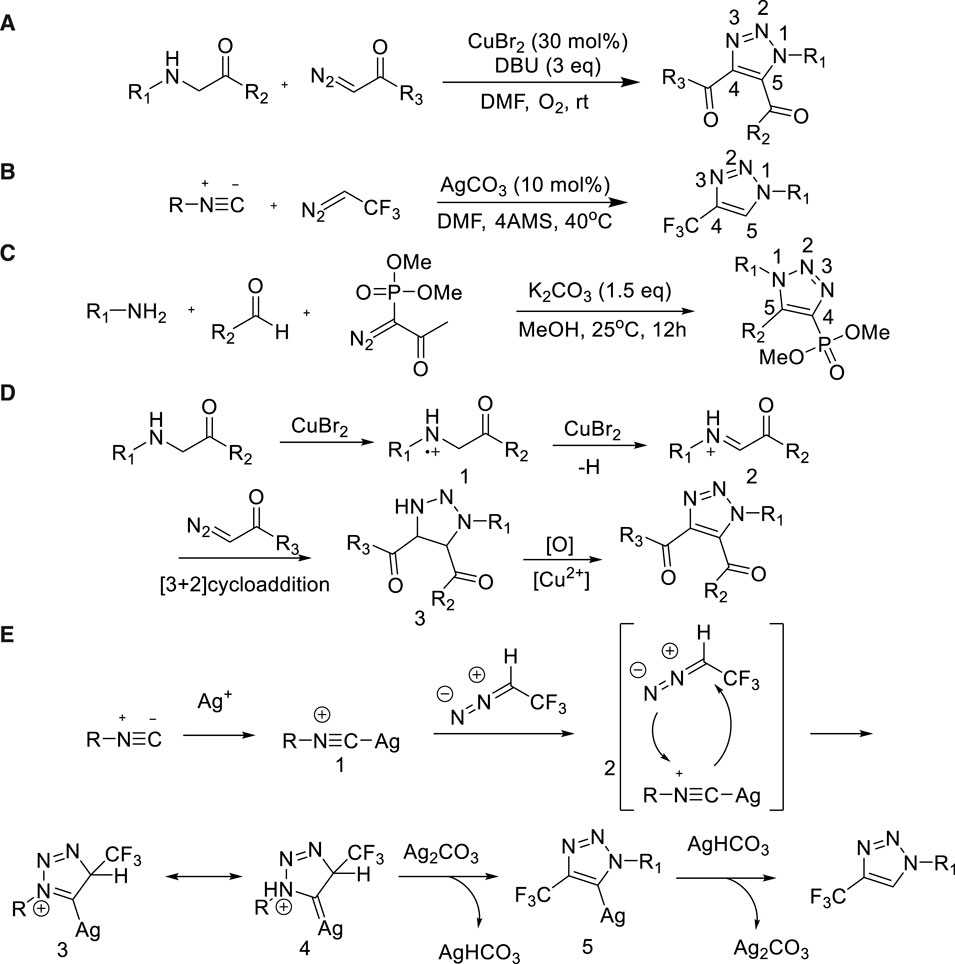
SCHEME 12. Method of synthesizing N-substituted-1,2,3-triazole by [3+2] cycloaddition reaction and the possible mechanism.
In 2015, Ma et al. reported a similar convenient method based on Ag-catalyzed cycloaddition reaction of isonitrile and diazide [3+2] to prepared a series of N1-substituted-1,2,3-triazoles (Wang et al., 2015) (Scheme 12B).
In 2016, Mohanan developed a metal-free, effective and easy way based on three-component reaction for the synthesis of 1,4,5-trisubstituted-1,2,3-triazoles (Ahamad et al., 2016). Using cheap and readily available aldehydes, amines and Bestmann Ohira reagents (BOR) as feedstock, the reaction provided an efficient synthesis strategy for 1,4,5-trisubstituted-1,2,3-triazoles with yield from 47% to 63% (Ahamad et al., 2016). Took into consideration the relevance of triazole scaffolds in the agrochemical and pharmaceutical, adopting this scheme to synthesize phosphonyl triazoles would be found potential applicability (Scheme 12C).
The mechanism of Scheme 12C was similar as Scheme 12(a) were described in Scheme 12D: The first step, amine was oxidized by CuBr2 passed electron transfer process to form amine radical cations 1. Then hydrogen radicals were transferred to form imine cations 2, which performed the [3+2] cycloaddition reaction with α-diazo compounds to obtain 3. Finally, three was oxidized by CuBr2 in the presence of oxygen to form N1-substituted-1,2,3-triazole.
The mechanism of Scheme 12B was described in the Scheme 12E: Firstly, isonitrile reacted with Ag + to produce silver isonitrile compound 1. And then, 1 with 2,2,2-trifluoromethyl diazomethane underwent the 1,3-dipolar cycloaddition reaction, following isomerization to obtain silver carbine compound 4 and then deprotonated. Finally, the silver was removed by hydrogenation reduction to form N1-substituted-1,2,3-triazole.
Synthesis of 1,2,4-triazoles
1,2,4-triazole is an important heterocyclic motif, which widely existed in the molecular structures with a sequence of bioactivities, including anticancer, antibacterial, anti-HIV and so on. The main synthesis methods include amidines, imidates, amidrazones, aryldiazoniums and hydrazones as raw material to provide nitrogen atoms to synthesize 1,2,4-triazole derivatives.
Amidines as nitrogen source
Amidine was widely used as an organic catalyst and ligand for the formation of nitrogen–carbon bonds due to the reactivity of nucleophilic nitrogen atoms. In 2011, a general method had been developed via one-pot, two-step process for the production of 1,3,5-trisubstituted-1,2,4-triazole with excellent yield (up to 90%) (Castanedo et al., 2011). The sequence started with the in situ formation of amide from carboxylic acid and amidine. Aniline further reacted with monosubstituted hydrazine and cyclized to trisubstituted triazole. This method had the advantages of high regioselectivity and good tolerance of functional groups (Scheme 13A).
In 2015, Huang described an efficient CuCl2 promoted synthesis of 2,4,6-trisubstituted and 1,3-disubstituted-1,2,4-triazoles (Huang et al., 2015). Triazole was prepared with high yield (85%) (Huang et al., 2015)under the presence of CuCl2 and DMF using K3PO4 as alkali, O2 as oxidant, amide and DMF as raw materials. In fact, nitrogen atoms and methyl groups from DMF were incorporated into the triazole. For amides replaced by various functional aromatic fragments (fluorine, bromine, methyl, methoxy, and trifluoromethyl), the reaction sequence appears to be highly effective (scheme 13B).
In 2019, Xia developed a facile copper-catalyzed one-pot method to prepare 3,5-disubstituted-1,2,4-triazole from amide and nitrile by cascade addition oxidation cyclization (Xia et al., 2019). In this reaction, O2 was used as an oxidant, and functionalization was catalyzed by the complex of McM-41 and cuprous bromide [phen-McM-41-CuBr] with high yield (91%) (Huang et al., 2015). The pathway was utilized to prepare a various of 1,2,4-triazole derivatives (Scheme 13C).
The possible reaction mechanism shown in Scheme 13D. As 2) showed Cu-catalyzed C (sp3)-H firing the amine to prepare imine-type intermediate 1 via a single-electron transfer (SET) procedure would be the first step of this reaction. In the following process, nucleophilic of 1 attack by the amidine to form intermediate 2. Taking the case of tertiary amine, the amine motif was delivered to get imine intermediate 3. Another molecular amidine 4 undertook the second attack of 3 with an intramolecular nucleophilic cyclization and followed oxidation to generate the final 1,3,5-triazine compounds.
Imidates as nitrogen source
Imidates are helpful precursors of triazoles, which have been extensive wielded in this field. In 2016, Guirado proposed a simplified method for the synthesis of 3-aryl-1,2,4-triazole chloroformamide, which was obtained via the reaction of benzamide with chloral hydrate in high yield (Guirado et al., 2016). When imidates reacted with the mixture of phosphorus pentachloride/phosphorus oxychloride, almost quantitatively converted to bis(1-tetrachloroethyl) benzimine chloride, which was then handled with hydrazine hydrate to produce 3-aryl-1,2,4-triazoles with high yield (86%) (Huang et al., 2015). The method had the advantages of good versatility, high yield, easy access to starting materials, mild and simple experimental steps (Scheme 14A).
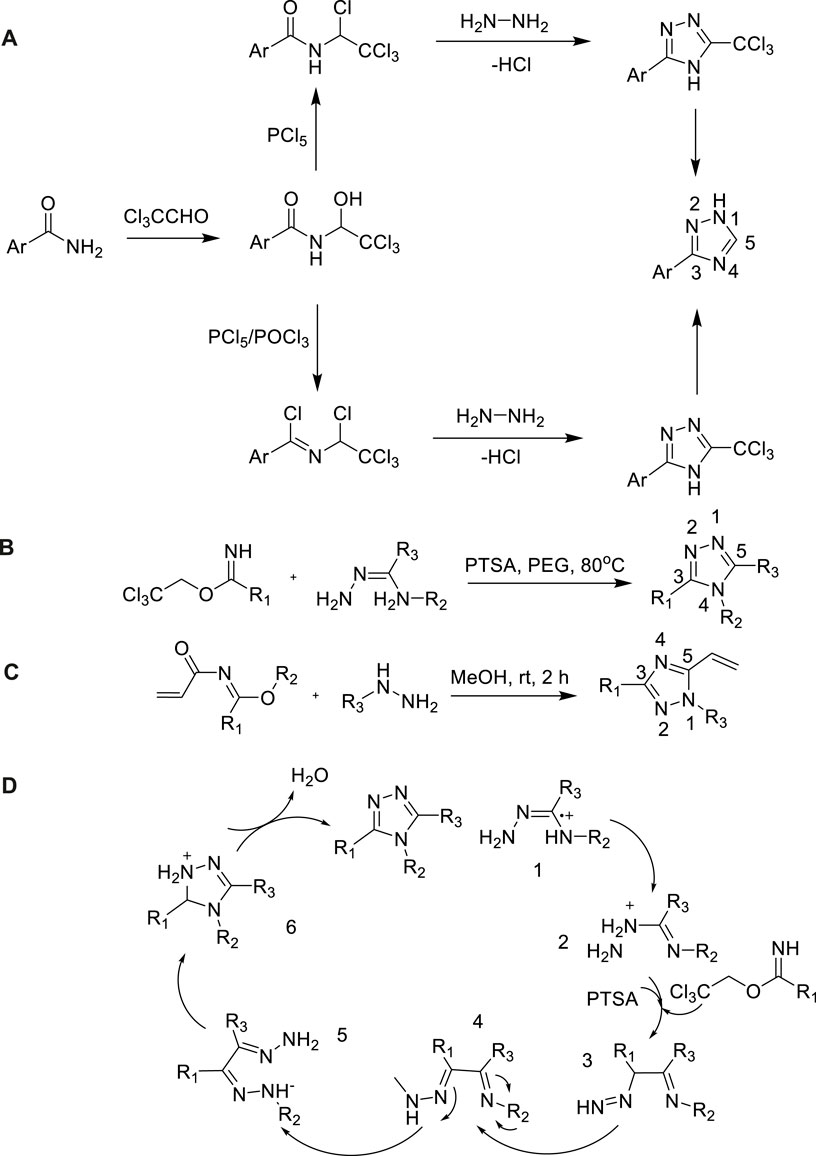
SCHEME 14. (A) Synthesis of 3-aryl-1,2,4-triazole. (B,C,D) Synthesis and mechanism of 3,4,5-trisubstituted-1,2,4-triazoles.
In 2014, Mangarao et al. had prepared a series of 3,4,5-trisubstituted-1,2,4-triazoles from 2,2,2-trichloroethyl imidate, which employed polyethylene glycol (PEG) as a solvent and used p-Toluenesulfonic acid (PTSA) as the catalyst during mild conditions (Mangarao et al., 2014). In conclusion, this was a facile, effective, and ecofriendly simple method for the synthesis of 1,2,4-triazole from 2,2,2-trichloroethyl imidate in PEG and employing PTSA as the catalyst leading to the corresponding 1,2,4-triazoles in mild conditions with excellent yields (92%) (Mangarao et al., 2014) (Scheme 14B).
In 2018, Azzouni et al. focued on the production of vinylimidates as precursors for the synthesis of functionalized 1,2,4-triazoles. (Azzouni et al., 2018). The method allowed a large array of substituents to be installed at (N1, C3, and C5) positions of 1,2,4-triazole with good yield (98%) (Azzouni et al., 2018). The experiment showed that the selectivity formation of 5-vinyl-1,2,4-triazoles could arrest take place potential five-member and seven-member by-products via theoretical calculations and NMR (Scheme 14C).
Take Scheme 14B as an example, the possible reaction mechanism described in Scheme 14D. PTSA catalyzed 2,2,2-trichloroethyl imidates undergone a series of oxidate and cyclization reaction to generate 1,2,4-triazole derivatives. PTSA played a significant role in this reaction. PTSA was not only a catalyst, but also a Lewis acid participated in reaction.
Amidrazones as nitrogen source
Amidine was an excellent raw material to provide nitrogen atom in the synthesis of triazole. In 2011, Siddaiah et al. reported that 1,2,4-triazole derivatives can be prepared at 80°C with moderate to high yields (55%–95%) in solvent-free conditions using HClO4-SiO2 as a catalyst (Siddaiah et al., 2011). The advantages were that the substituents on amide hydrazone and anhydride had good tolerance during optimized conditions, and the HClO4-SiO2 catalyst could be recycled continuously for at least three times (Scheme 15A).
In 2014, Vidavalur et al. developed a similar method via using ceric ammonium nitrate (CAN) oxidative cyclization synthesis of 3,4,5-trisubstituted-1,2,4-triazole in polyethylene glycol (PEG) with good to excellent yield from 61% to 97% (Vidavalur et al., 2014). In this reaction, CAN was not only an oxidant, but also a Lewis acid. After the activation of amino nitrogen atom, benzaldehyde was added to generate diazizone intermediate. Finally, oxidation cyclization reaction was carried out to synthesize 1-(sulfur)-phosphonated 5-amino-1,2,4-triazole framework. The reaction conditions were relatively easy to operate (Scheme 15B).
A possible mechanism was showed in Scheme 15C. Ceric ammonium nitrate reacted as both a Lewis acid and an oxidant. CAN play an irreplaceable role in promoting the occurrence of the reaction.
In 2013, the synthesis of 3-(β-d-Glucopyranosyl)-5–substituted 1,2,4-triazoles from c-glucose-toluene sulfonyl hydrazone was reported by Bokor et al. (Bokor et al., 2013). 3-(β-d-Glucopyranosyl)-5-substituted-1,2,4-triazoles were constructed by acylation of O-perbenzoylated N1-tosyl-C-β-d-glucopyranosyl formamidrazone and subsequent removed protecting groups. The acylation of C-glucosyl toluene sulfonyl hydrazone with aromatic acid chloride was accompanied by a complete N-decarboxylation reaction, yielding a high yield (62%–92%) (Azzouni et al., 2018) of 3-(B-d-glucosyl)-5-substituted-1,2,4-triazole. This series of compounds were evaluated as effective glycogen phosphorylase inhibitors, which extended the substrate of 1,2,4-triazole framework construction (Scheme 16A).
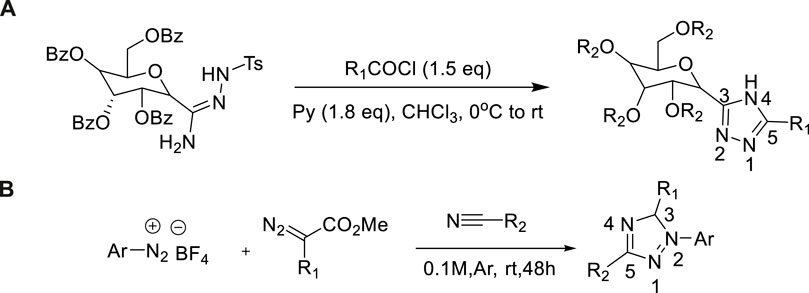
SCHEME 16. Synthesis of C-Glucopyranosyl-1,2,4-triazoles and 2-aryl-3,5-disubstituted-1,2,4-triazole derivatives.
In 2017, Benassi et al. composed a variety of 4,5-dihydro-1H-1,2,4-triazole from various amide hydrazones (Eliseeva et al., 2017). In the presence of pyridine in toluene, the group 1,2,4-triazole was firstly synthesized from amide hydrazone and dimethyl or diethyl ester to obtain the corresponding 2-aryl-3,5-disubstituted-1,2,4-triazole. Under microwave irradiation, aminolazone reacted with excess acetone to produce 5,5-dimethyl-4,5-dihydro-1,1,2,4-triazole compounds. Photophysical result indicated that most of the 1,2,4-triazole compounds with blue or yellow-green luminescence, and the fluorescence emission ability was medium, which had a good application value in the preparation of fluorescent probes (Motornov et al., 2018) (Scheme 16B).
Aryl diazonium salts as nitrogen source
Aryl diazonium salts as intermediates, which were utilized broadly as nitrogen source for the generation of abundant heterocycles compounds due to the advantages of shortcutting available and highly reactive. In 2020, Tian et al. developed the synthesis of 1,2,4-triazole by decarboxylation and cyclization of 2-aryl-2-isocyanate with aryl diazonium salts (Tian et al., 2020). In this metal-free reaction, the use of 1,4-diazocyclic [2.2.2]octane (DABCO) as a weak base was extremely critical (Scheme 17A).
A possible mechanism was described in Scheme 17B. Thus, bis(arylhydrazones) could be converted into the radical cation 1 via single-electron transfer (SET) to Cu(II)X2. Base on the elimination of HX, that intermediate 1 could be transformed to 2. 2 could lead to the formation of 3 pass by dimerization, which could isomerize to form the intermediate 4. The latter could afford a radical cation 5 by reductive elimination of elemental copper through SET process. The catalytic cycle might be completed via regenerate the active Cu(II) species from reduced Cu(0) species was reoxidized by air. This was conducive to promoting the process of response. Besides, DABCO is not only acting as a base but also playing a crucial role of an effective ligand for the Cu(II)-catalyzed oxidative cyclization.
In 2018, a highly effective method for the production of 1,5-disubsubstituted-1,2,4-triazole was reported by Liu et al. (Liu et al., 2018). According to the properties of catalytic system, triazole was synthesized by aryl diazonium salts and isocyanide [3+2] cycloaddition method. In fact, the utilization of Ag(I) as a catalyst produced selective 1,3-disubstituted-1,2,4-triazole in the yield of 88%, while the copper catalyst produced 1,5-disubstituted-1,2,4-triazole in the yield of 79% (Scheme 18A).
In 2019, Yu et al. firstly studied metal-free [3+2] cycloaddition/decarboxylation between aryl diazonium salts and azalactic acid to obtain 1,3,5-trisubstituted-1,2,4-triazole (Yu et al., 2019). The optimized reaction conditions were as follows: 20mol% N-Bu4NCl and Na2SO4 (as desiccant) in acetonitrile at room temperature. N-Bu4NCl could be used as a source of chlorine, which could stabilize the intermediate in the cycloaddition reaction of aryl diazo and enol type azalactone [3+2]. The intermediate was then subjected to ring-opening decarboxylation to form a 1,2,4-triazole target. It was a feasible synthesis of 1,2,4-triazole derivatives (Scheme 18B).
Hydrazones as nitrogen source
Hydrazones were popular precursors of nitrogen heterocycles in most methods for the production of fused 1,2,4-triazole. Characteristics of these compound involved highly reactive nitrogen pairs and SP-carbon atoms. Hydrazones could react with various amines to synthesize triazoles. In 2016, Chen et al. developed a synthetic method in which hydrazone could be easily converted to 1,2,4-triazole by combining amines with I2/TBHP in high yield (92%)(Chen et al., 2016). A wide range of functional groups were well tolerated in hydrazone and amine fragments for the synthesis of multiple compounds. The method has the characteristics of available raw materials, simple and easy operating conditions, a wide range of substrates (Scheme 19A).
A possible mechanism of the framework was presented in Scheme 19B. The Michael-type nucleophilic supplement of the terminal amino group of the benzamidrazone to form the malononitrile receptors 2. Then, the homologous spirocyclic 1,2,4-triazoles finished cyclization undergone an intramolecular nucleophilic supplement of the amino group to the imidic carbon atom to afford final 1,2,4-triazole derivatives.
In 2019, Guru et al. described a metal-free catalytic method for dehydrogenation cyclization based on B(C6F5)3 (Guru et al., 2019). B(C6F5)3 activated the hydrazine portion nucleophilic attack in progress, following in proper order amination, intramolecular cyclization and dehydrogenation steps to prepare the 3,4,5-tsubstituted-1,2,4-triazole with 85% yield (Guru et al., 2019). This reaction route was characterized by green economy, no oxidant, mild conditions and selectivity, which provided a potential platform for catalytic chemical conversion without the use of transition metal (Scheme 20A).
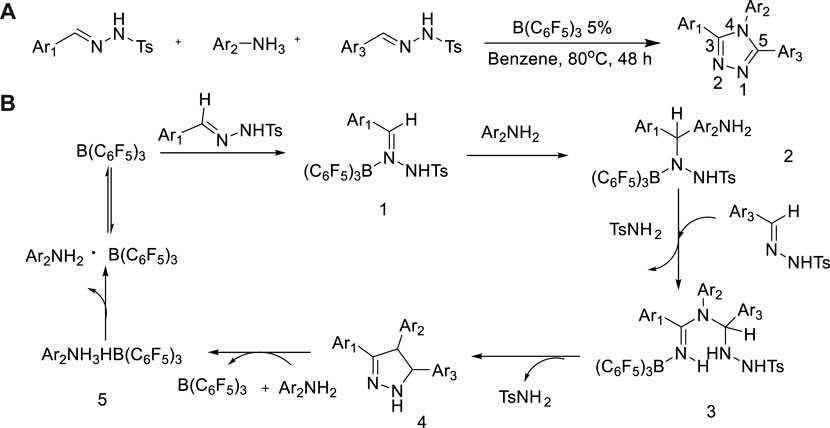
SCHEME 20. (A) Access to 3,4,5-trisubstituted-1,2,4-triazoles from N-tosylhydrazones and aromatic amines. (B) Plausible mechanism base on experimental.
A predicted mechanism was described in Scheme 20B (Kojima, 2016; Cai et al., 2014). On account of recommended mechanism of the B(C6F5)3-catalyzed receptors less dehydrogenative cyclization of N-tosyl-hydrazones with anilines, investigators had carried out DFT calculations to research the sophisticated reaction mechanism and to receive deep understanding of driving force for the production of the 1,2,4-triazoles. The experimental calculations results revealed that the rate-determining step involved intra-molecular hydrogen transfer between the N-centers after aniline gets bonded to the N-tosylhydrazone unit.
In 2011, Wang et al. developed the preparation of 1,3,5-trisubstituted-1,2,4-triazole from hydrazonoyl hydrochlorides and aldehyde (Tseng et al., 2011). In the presence of triethylamine, aromatic rings with various substituents were suitable for this method as a 1,3-dipolar nitroimide source. In this one-pot reaction, the aldehyde was firstly converted to an oxime intermediate by reacting with hydroxylamine. The resulting oxime and hydrazyl hydrochloric acid were reacted by 1,3-dipolar cycloaddition to obtain 3,5-dissubstituted 1,2,4-triazole with yields ranging from moderate to good (53%–91%) (Tseng et al., 2011). This method could be provided to a series of aldehydes including aliphatic, cyclic aliphatic, aromatic, and heterocyclic aldehydes (Scheme 21A).
The plausible mechanism was represented in Scheme 21B. Acetaldehyde was reacted with hydroxylamine hydrochloride in toluene under reflux to prepare oxime hydrochloride intermediate 1. Nitrilimine specie 2 was generated via deal with hydrazonoyl hydrochloride by a surfeit of triethylamine. The essential 1,3-dipolar cycloaddition dihydrotriazole three was constructed by disposing dipolarophile oxime with 1,3-dipole nitrilimine. When the subsequent dehydroxylation condensation was finished, the corresponding 1,3,5-trisubstituted-1,2,4-triazole product was produced.
In 2015, Zheng et al. reported an efficient and actual methodology for synthesizing 1,2,4-triazoles by oxidative cyclization of hydrazones using SeO2 (Zheng et al., 2015). A sequence of fused 1,2,4-triazoles were constructed by oxidative intramolecular cyclization between heterocyclic hydrazones and selenium dioxide. Simple applicability of this approach was corroborated base on these compounds of 1,2,4-triazolo [4,3-a] pyridines, 1,2,4-triazolo [4,3-a] pyrimidines, and 1,2,4-triazolo-[4,3-a] quinoxalines with moderate to good yield (79%–98%)(Zheng et al., 2015) (Scheme 22A).
The reaction mechanism may be through oxidative addition and reductive elimination processes (Scheme 22B). Hydrazones of aromatic and aliphatic aldehydes containing electron-withdrawing and electron-donating substituents were oxidized by SeO2, and then through a series of electron transfer, finally removed a molecule Se(OH)2 to give the corresponding 1,2,4-triazoles.
Conclusion
In this review, we summarized the synthetic methods of 1,2,3-/1,2,4-triazoles combined with the progress of triazole over the past 2 decades. Several main synthetic methods from various nitrogen sources were summarized. The synthetic methods were sorted according to the key starting material utilized for the production of the 1,2,3-/1,2,4-triazole skeleton. Sodium azido, trimethylsilyl azido, alkyl/aryl azido, hydrazone, sulfonamide, hydrazine, and diazo compounds are among the most correlative 1,2,3-triazole precursors. Amidines, imidates, amidrazones, aryldiazoniums, and hydrazones are among the most correlative 1,2,4-triazole precursors. In the synthesis of 1,2,3-triazole, sodium azide is a highly toxic compound, which is not suitable for large-scale application as a nitrogen source. Compared with sodium azide, azide trimethylsilane and alkyl/aryl azide are safer and more efficient. Hydrazone, aminohydrazine and diazo compounds are also the main materials of nitrogen sources, which were reported in a large number of literatures. The synthesis method of 1,2,4-triazole mainly uses amidine, amide, amidine hydrazone, aryl diazonium salt, and hydrazone as nitrogen sources. During the past few years, there have been excellent progress in copper-catalyzed cross-couplings in the synthesis methods of 1,2,3/1,2,4-triazole with inexpensive, low toxic, and good functional tolerance. Metal catalysis was the mainly choice at present; among them, copper-catalyzed was the most popular catalyst. With the promotion of the concept of “green chemistry”, metal-free catalytic synthesis such as electrocatalysis and photocatalysis are attracting researcher’ interest. It is believed that with the continuous efforts of researchers, more green economy and simple and mild synthesis methods will continue to develop.
Author contributions
The author JD contributed to the literature review and the writing of the review. The author ZL and ST put forward guiding opinions on the revision and writing.
Funding
This work was supported by the Taishan Scholar Project of Shandong Province, China and the Science and Technology Support Program for Youth Innovation in Universities of Shandong (2020KJM003).
Conflict of interest
The authors declare that the research was conducted in the absence of any commercial or financial relationships that could be construed as a potential conflict of interest.
Publisher’s note
All claims expressed in this article are solely those of the authors and do not necessarily represent those of their affiliated organizations, or those of the publisher, the editors and the reviewers. Any product that may be evaluated in this article, or claim that may be made by its manufacturer, is not guaranteed or endorsed by the publisher.
References
Ahamad, S., Kant, R., and Mohanan, K. (2016). Metal-free three-component domino approach to phosphonylated triazolines and triazoles. Org. Lett. 18, 280–283. doi:10.1021/acs.orglett.5b03437
Ahmed, F., and Xiong, H. (2021). Recent developments in 1, 2, 3-triazole-based chemosensors. Dyes Pigments 185, 108905. doi:10.1016/j.dyepig.2020.108905
Azzouni, S., Abdelli, A., Gaucher, A., Arfaoui, Y., Efrit, M. L., and Prim, D. (2018). From imidates to vinyl-1, 2, 4-triazoles: Synthesis, mechanistic aspects and first issues of their reactivity. Tetrahedron 74, 6972–6978. doi:10.1016/j.tet.2018.10.050
Bao, P., Yue, H., Meng, N., Zhao, X., Li, J., and Wei, W. (2019). Copper-catalyzed three-component reaction of alkynes, TMSN3, and ethers: Regiocontrollable synthesis of N(1)- and N(2)-oxyalkylated 1, 2, 3-triazoles. Org. Lett. 21, 7218–7222. doi:10.1021/acs.orglett.9b02295
Bokor, E., Docsa, T., Gergely, P., and Somsak, L. (2013). C-Glucopyranosyl-1, 2, 4-triazoles as new potent inhibitors of glycogen phosphorylase. ACS Med. Chem. Lett. 4, 612–615. doi:10.1021/ml4001529
Brüggemann, R. J., Verheggen, R., Boerrigter, E., Stanzani, M., Verweij, P. E., Blijlevens, N. M. A., et al. (2022). Management of drug–drug interactions of targeted therapies for haematological malignancies and triazole antifungal drugs. Lancet Haematol. 9, e58–e72. doi:10.1016/s2352-3026(21)00232-5
Burman, B., Drutman, S. B., Fury, M. G., Wong, R. J., Katabi, N., Ho, A. L., et al. (2022). Pharmacodynamic and therapeutic pilot studies of single-agent ribavirin in patients with human papillomavirus–related malignancies. Oral Oncol. 128, 105806. doi:10.1016/j.oraloncology.2022.105806
Cai, Z. J., Lu, X. M., Zi, Y., Yang, C., Shen, L. J., Li, J., et al. (2014). I2/TBPB mediated oxidative reaction of N-tosylhydrazones with anilines: Practical construction of 1, 4-disubstituted 1, 2, 3-triazoles under metal-free and azide-free conditions. Org. Lett. 16, 5108–5111. doi:10.1021/ol502431b
Caraballo, R. H., Pociecha, J., Reyes, G., Espeche, A., Galichio, S., Fasulo, L., et al. (2020). Rufinamide as add-on therapy in children with epileptic encephalopathies other than lennox–gastaut syndrome: A study of 34 patients. Epilepsy & Behav. 108, 107074. doi:10.1016/j.yebeh.2020.107074
Castanedo, G. M., Seng, P. S., Blaquiere, N., Trapp, S., and Staben, S. T. (2011). Rapid synthesis of 1, 3, 5-substituted 1, 2, 4-triazoles from carboxylic acids, amidines, and hydrazines. J. Org. Chem. 76, 1177–1179. doi:10.1021/jo1023393
Chen, J., Liang, T., Zhao, H., Lin, C., Chen, L., and Zhang, M. (2019). Silver-mediated three-component cycloaddition reaction for direct synthesis of 1-N-vinyl-substituted 1, 2, 3-triazoles. Org. Biomol. Chem. 17, 4843–4849. doi:10.1039/c9ob00686a
Chen, Y., Nie, G., Zhang, Q., Ma, S., Li, H., and Hu, Q. (2015). Copper-catalyzed [3 + 2] cycloaddition/oxidation reactions between nitro-olefins and organic azides: Highly regioselective synthesis of NO2-substituted 1, 2, 3-triazoles. Org. Lett. 17, 1118–1121. doi:10.1021/ol503687w
Chen, Z., Li, H., Dong, W., Miao, M., and Ren, H. (2016). I2-Catalyzed oxidative coupling reactions of hydrazones and amines and the application in the synthesis of 1, 3, 5-trisubstituted 1, 2, 4-triazoles. Org. Lett. 18, 1334–1337. doi:10.1021/acs.orglett.6b00277
Chen, Z., Yan, Q., Liu, Z., Xu, Y., and Zhang, Y. (2013). Copper-mediated synthesis of 1, 2, 3-triazoles from N-tosylhydrazones and anilines. Angew. Chem. Int. Ed. Engl. 52, 13566–13570. doi:10.1002/ange.201306416
Chen, Z., Yan, Q., Liu, Z., and Zhang, Y. (2014). Metal-free C-N- and N-N-bond formation: Synthesis of 1, 2, 3-triazoles from ketones, N-tosylhydrazines, and amines in one pot. Chem. Eur. J. 20, 17635–17639. doi:10.1002/chem.201405057
Chokshi, A., Vaishya, R., Inavolu, R., and Potta, T. (2019). Intranasal spray formulation containing rizatriptan benzoate for the treatment of migraine. Int. J. Pharm. 571, 118702. doi:10.1016/j.ijpharm.2019.118702
Dheer, D., Singh, V., and Shankar, R. (2017). Medicinal attributes of 1, 2, 3-triazoles: Current developments. Bioorg. Chem. 71, 30–54. doi:10.1016/j.bioorg.2017.01.010
Di Antonio, M., Biffi, G., Mariani, A., Raiber, E. A., Rodriguez, R., and Balasubramanian, S. (2012). Selective RNA versus DNA G-quadruplex targeting by in situ click chemistry. Angew. Chem. Int. Ed. Engl. 51, 11235–11240. doi:10.1002/ange.201206281
Eliseeva, A. I., Nesterenko, O. O., Slepukhin, P. A., Benassi, E., and BelskaYA, N. P. (2017). Synthesis and fluorescent behaviour of 2-aryl-4, 5-dihydro-1H-1, 2, 4-triazoles. J. Org. Chem. 82, 86–100. doi:10.1021/acs.joc.6b02144
Elzoheiry, M. A., Elmehankar, M. S., AboukamaR, W. A., el-Gamal, R., Sheta, H., Zenezan, D., et al. (2022). Fluconazole as schistosoma mansoni cytochrome P450 inhibitor: In vivo murine experimental study. Exp. Parasitol. 239, 108291. doi:10.1016/j.exppara.2022.108291
Fallah, Z., Tajbakhsh, M., Alikhani, M., Larijani, B., FarAMARZI, M. A., Hamedifar, H., et al. (2022). A review on synthesis, mechanism of action, and structure-activity relationships of 1, 2, 3-triazole-based α-glucosidase inhibitors as promising anti-diabetic agents. J. Mol. Struct. 1255, 132469. doi:10.1016/j.molstruc.2022.132469
Gao, d. x., song, s., kahn, j. s., cohen, s. r., fiumarA, K., Dumont, N., et al. (2022). Treatment of patients experiencing dupilumab facial redness with itraconazole and fluconazole: A single-institutional, retrospective medical record review. J. Am. Acad. Dermatology 86, 938–940. doi:10.1016/j.jaad.2021.03.070
Gao, F., Wang, T., Xiao, J., and Huang, G. (2019). Antibacterial activity study of 1, 2, 4-triazole derivatives. Eur. J. Med. Chem. 173, 274–281. doi:10.1016/j.ejmech.2019.04.043
Georgiadis, N., Tsarouhas, K., Tsitsimpikou, C., Vardavas, A., Rezaee, R., Germanakis, I., et al. (2018). Pesticides and cardiotoxicity. Where do we stand? Toxicol. Appl. Pharmacol. 353, 1–14. doi:10.1016/j.taap.2018.06.004
Gomha, S. M., Ahmed, S. A., and Abdelhamid, A. O. (2015). Synthesis and cytotoxicity evaluation of some novel thiazoles, thiadiazoles, and pyrido[2, 3-d] [1, 2, 4]triazolo[4, 3-a]pyrimidin-5(1H)-ones incorporating triazole moiety. Molecules 20, 1357–1376. doi:10.3390/molecules20011357
Guirado, A., López-Caracena, L., LóPEZ-SáNCHEZ, J. I., Sandoval, J., Vera, M., Bautista, D., et al. (2016). A new, high-yield synthesis of 3-aryl-1, 2, 4-triazoles. Tetrahedron 72, 8055–8060. doi:10.1016/j.tet.2016.10.045
Guru, M. M., De, S., Dutta, S., Koley, D., and Maji, B. (2019). B(C6F5)3-catalyzed dehydrogenative cyclization of N-tosylhydrazones and anilines via a Lewis adduct: A combined experimental and computational investigation. Chem. Sci. 10, 7964–7974. doi:10.1039/c9sc02492a
Guru, M. M., and Punniyamurthy, T. (2012). Copper(II)-catalyzed aerobic oxidative synthesis of substituted 1, 2, 3- and 1, 2, 4-triazoles from bisarylhydrazones via C-H functionalization/C-C/N-N/C-N bonds formation. J. Org. Chem. 77, 5063–5073. doi:10.1021/jo300592t
Huang, H., Guo, W., Wu, W., Li, C. J., and Jiang, H. (2015). Copper-catalyzed oxidative C(sp(3))-H functionalization for facile synthesis of 1, 2, 4-triazoles and 1, 3, 5-triazines from amidines. Org. Lett. 17, 2894–2897. doi:10.1021/acs.orglett.5b00995
Huisgen, R., Szeimies, G., and Möbius, L. (1967). 1.3‐Dipolare cycloadditionen, XXXII. Kinetik der Additionen organischer azide an CC‐mehrfachbindungen. Chem. Ber. 100, 2494–2507. doi:10.1002/cber.19671000806
Jarosław Kalisiak, K. B. S., and Fokin, V. V. (2008). Efficient synthesis of 2-substituted-1, 2, 3-triazoles. Org. Lett. 10, 3171–3174. doi:10.1021/ol8006748
Keri, R. S., Patil, S. A., Budagumpi, S., and Nagaraja, B. M. (2015). Triazole: A promising antitubercular agent. Chem. Biol. Drug Des. 86, 410–423. doi:10.1111/cbdd.12527
Kim, S. W., Biegon, A., Katsamanis, Z. E., Ehrlich, C. W., Hooker, J. M., Shea, C., et al. (2009). Reinvestigation of the synthesis and evaluation of [N-methyl-11C]vorozole, a radiotracer targeting cytochrome P450 aromatase. Nucl. Med. Biol. 36, 323–334. doi:10.1016/j.nucmedbio.2008.12.013
Kolb, H. C., Finn, M., and Sharpless, K. B. J. A. C. I. E. (2001). Click chemistry: Diverse chemical function from a few good reactions, 40, 2004.
Kucukguzel, S. G., and Cikla-Suzgun, P. (2015). Recent advances bioactive 1, 2, 4-triazole-3-thiones. Eur. J. Med. Chem. 97, 830–870. doi:10.1016/j.ejmech.2014.11.033
Kumar, N., Ansari, M. Y., Kant, R., and Kumar, A. (2018). Copper-catalyzed decarboxylative regioselective synthesis of 1, 5-disubstituted 1, 2, 3-triazoles. Chem. Commun. 54, 2627–2630. doi:10.1039/c7cc09934g
Li, L., Fan, X., Zhang, Y., Zhu, A., and Zhang, G. (2013). Controllable synthesis of bis(1, 2, 3-triazole)s and 5-alkynyl-triazoles via temperature effect on copper-catalyzed Huisgen cycloaddition. Tetrahedron 69, 9939–9946. doi:10.1016/j.tet.2013.09.093
Li, Y. J., Li, X., Zhang, S. X., Zhao, Y. L., and Liu, Q. (2015). Copper(II)-catalyzed oxidative [3+2] cycloaddition reactions of secondary amines with alpha-diazo compounds: A facile and efficient synthesis of 1, 2, 3-triazoles. Chem. Commun. 51, 11564–11567. doi:10.1039/c5cc02092a
Lindsay, J., Krantz, E. M., Morris, J., Sweet, A., Tverdek, F., Joshi, A., et al. (2022). Voriconazole in hematopoietic stem cell transplantation and cellular therapies: Real-world usage and therapeutic level attainment at a major transplantation center. Transplant. Cell Ther. 28 (8), 511.e1–511.e10. doi:10.1016/j.jtct.2022.05.030
Liu, J. Q., Shen, X., Wang, Y., WaNG, X. S., and Bi, X. (2018). [3 + 2] cycloaddition of isocyanides with aryl diazonium salts: Catalyst-dependent regioselective synthesis of 1, 3- and 1, 5-disubstituted 1, 2, 4-triazoles. Org. Lett. 20, 6930–6933. doi:10.1021/acs.orglett.8b03069
Lucht, F., Fauche, A., and Allaert, F. A. (1996). Céfatrizine (8 j) versus pénicilline V (10 j) dans l'angine à streptocoque A de l'enfant. Médecine Maladies Infect. 26, 557–561. doi:10.1016/s0399-077x(96)80072-2
Mangarao, N., Mahaboob Basha, G., Ramu, T., Srinuvasarao, R., Prasanthi, S., and Siddaiah, V. (2014). Brønsted acid-catalyzed simple and efficient synthesis of 1, 2, 4-triazoles and 1, 2, 4-oxadiazoles using 2, 2, 2-trichloroethyl imidates in PEG. Tetrahedron Lett. 55, 177–179. doi:10.1016/j.tetlet.2013.10.147
Matin, M. M., Matin, P., Rahman, M. R., Ben Hadda, T., Almalki, F. A., Mahmud, S., et al. (2022). Triazoles and their derivatives: Chemistry, synthesis, and therapeutic applications. Front. Mol. Biosci. 9, 864286. doi:10.3389/fmolb.2022.864286
Moore, J. (2007). “Rizatriptan,” in xPharm: The comprehensive pharmacology reference. Editors S. J. Enna, and D. B. Bylund (New York: Elsevier.
Motornov, V. A., Tabolin, A. A., NoviKOV, R. A., Shepel, N. E., Nenajdenko, V. G., and Ioffe, S. L. (2018). Synthesis of 2, 5-diaryl-4-halo-1, 2, 3-triazoles and comparative study of their fluorescent properties. Tetrahedron 74, 3897–3903. doi:10.1016/j.tet.2018.05.071
Moura, N. M. M., and Tomé, A. C. (2022). “5.01 - 1,2,3-Triazoles,” in Comprehensive heterocyclic chemistry IV. Editors D. S. Black, J. Cossy, and C. V. Stevens (Oxford: Elsevier).
Musarurwa, H., and Tavengwa, N. T. (2021). Sustainable extraction of pesticides in food and environmental samples using emerging green adsorbents. Sustain. Chem. Pharm. 24, 100545. doi:10.1016/j.scp.2021.100545
Navarro-Triviño, F. J. (2021). Leishmaniasis cutánea tratada con itraconazol oral. Piel 36, 563–565. doi:10.1016/j.piel.2020.10.006
Nemallapudi, B. R., Guda, D. R., Ummadi, N., Avula, B., Zyryanov, G. V., Reddy, C. S., et al. (2022). New methods for synthesis of 1,2,3-triazoles: A review. Polycycl. Aromat. Compd. 42 (6), 3874–3892. doi:10.1080/10406638.2020.1866038
Neto, F. D. P. R. A., De Oliveira, L. M. F., Silva, P. A. S., Duarte, A. M., de Freitas, J. J. A., Vechi, H. T., et al. (2022). Co-infecção leishmaniose visceral E histoplasmose em paciente hiv positivo. Braz. J. Infect. Dis. 26, 102120. doi:10.1016/j.bjid.2021.102120
Park, H. G., Kim, J. H., Dancer, A. N., Kothapalli, K. S., and Brenna, J. T. 2021. The aromatase inhibitor letrozole restores FADS2 function in ER+ MCF7 human breast cancer cells✰. Prostagl. Leukot. Essent. Fat. Acids, 171, 102312, doi:10.1016/j.plefa.2021.102312
Patel, N. B., Khan, I. H., Pannecouque, C., and De Clercq, E. (2012). Anti-HIV, antimycobacterial and antimicrobial studies of newly synthesized 1, 2, 4-triazole clubbed benzothiazoles. Med. Chem. Res. 22, 1320–1329. doi:10.1007/s00044-012-0129-4
Pfaller, M. A., Shortridge, D., Harris, K. A., Garrison, M. W., Deryke, C. A., Depestel, D. D., et al. (2021). Ceftolozane-tazobactam activity against clinical isolates of Pseudomonas aeruginosa from ICU patients with pneumonia: United States, 2015–2018. Int. J. Infect. Dis. 112, 321–326. doi:10.1016/j.ijid.2021.09.064
Phanindrudu, M., Tiwari, D. K., Aravilli, V. K., Bhardwaj, K. C., Sabapathi, G., Likhar, P. R., et al. (2016). Magnetically recoverable Cu0/Fe3O4-catalysed one-pot tandem synthesis of sulfur-containing triazoles from alkynes and azide: DMSO acts as an alkylating agent. Eur. J. Org. Chem., 4629–4634. doi:10.1002/ejoc.201600740
Ramassamy, M., Murris, M., Recoche, I., Mailhol, C., Didier, A., and Guilleminault, L. (2021). Piperacillin-tazobactam-induced fever in chronic respiratory diseases: Safe challenge test under premedication. Rev. Francaise D'allergologie. doi:10.1016/j.reval.2021.09.001
Riyadh, S. M., Abolibda, T. Z., Sayed, A. R., and Gomha, S. M. (2022). Synthetic utility of aminomercapto[1, 2, 4]triazoles in the preparation of fused triazoles. Curr. Org. Chem. 26, 693–714. doi:10.2174/1385272826666220417131159
Riyadh, S. M., and Gomha, S. M. (2020). Two decades of the synthesis of mono- and bis-aminomercapto[1, 2, 4]triazoles. RSC Adv. 10, 24994–25012. doi:10.1039/d0ra04208k
Rodrigues, L. D., Sunil, D., Chaithra, D., and Bhagavath, P. (2020). 1, 2, 3/1, 2, 4-Triazole containing liquid crystalline materials: An up-to-date review of their synthetic design and mesomorphic behavior. J. Mol. Liq. 297, 111909. doi:10.1016/j.molliq.2019.111909
Roger Hanselmann, G. E. J., Johnson, Graham, RongLIANG, L. O. U., Martynow, J. A. C. E. K. G., and Maxwellreeve, M. (2010). Synthesis of an antibacterial compound containing a 1,4-substituted 1H-1,2,3-Triazole: A scaleable alternative to the “click” reaction. Org. Proc. Res. Develop. 14, 152–158. doi:10.1021/op900252a
Sakai, K., Hida, N., and Kondo, K. J. B. O. T. C. S. O. J. (1986). Reactions of α-polyhalo ketone tosylhydrazones with sulfide ion and primary amines. Cyclization to 1, 2, 3-thiadiazoles and 1, 2, 3-triazoles. Bull. Chem. Soc. Jpn. 159, 179–183. 3-triazoles. doi:10.1246/bcsj.59.179
Scattergood, P. A., Sinopoli, A., and Elliott, P. I. P. (2017). Photophysics and photochemistry of 1, 2, 3-triazole-based complexes. Coord. Chem. Rev. 350, 136–154. doi:10.1016/j.ccr.2017.06.017
Sharawat, I. K., PanDA, P. K., Panda, P., and Dawman, L. (2021). Efficacy and safety of rufinamide as adjunctive therapy in patients with lennox gastaut syndrome: A systematic review and meta-analysis. Seizure 91, 296–307. doi:10.1016/j.seizure.2021.07.004
Sharma, A., Agrahari, A. K., Rajkhowa, S., and Tiwari, V. K. (2022). Emerging impact of triazoles as anti-tubercular agent. Eur. J. Med. Chem. 238, 114454. doi:10.1016/j.ejmech.2022.114454
Shen, T., Huang, X., Liang, Y. F., and Jiao, N. (2015). Cu-catalyzed transformation of alkynes and alkenes with azide and dimethyl sulfoxide reagents. Org. Lett. 17, 6186–6189. doi:10.1021/acs.orglett.5b03179
Shettar, A., Shankar, V. K., Ajjarapu, S., Kulkarni, V. I., Repka, M. A., and Murthy, S. N. (2021). Development and characterization of Novel topical oil/PEG creams of voriconazole for the treatment of fungal infections. J. Drug Deliv. Sci. Technol. 66, 102928. doi:10.1016/j.jddst.2021.102928
Shin Kamijo, T. J., Huo, Zhibao, Yamamoto, Yoshinori, and Yamamoto, Y. (2003). Synthesis of triazoles from nonactivated terminal alkynes via the three-component coupling reaction using a Pd(0)-Cu(I) bimetallic catalyst. J. Am. Chem. Soc. 125, 7786–7787. doi:10.1021/ja034191s
Siddaiah, V., Basha, G. M., Srinuvasarao, R., and Yadav, S. K. (2011). HClO4-SiO2: An efficient reusable catalyst for the synthesis of 3, 4, 5-trisubstituted 1, 2, 4-triazoles under solvent-free conditions. Catal. Lett. 141, 1511–1520. doi:10.1007/s10562-011-0665-4
Slomovitz, B. M., Filiaci, V. L., Walker, J. L., Taub, M. C., Finkelstein, K. A., Moroney, J. W., et al. (2022). A randomized phase II trial of everolimus and letrozole or hormonal therapy in women with advanced, persistent or recurrent endometrial carcinoma: A gog foundation study. Gynecol. Oncol. 164, 481–491. doi:10.1016/j.ygyno.2021.12.031
Strzelecka, M., and Swiatek, P. (2021). Triazoles as Important Antibacterial Agents. Pharmaceuticals (Basel) 114, 24. doi:10.3390/ph14030224
Takahashi, K., Yamagishi, G., Hiramatsu, T., HosOYA, A., Onoe, K., Doi, H., et al. (2011). Practical synthesis of precursor of [N-methyl-11C]vorozole, an efficient PET tracer targeting aromatase in the brain. Bioorg. Med. Chem. 19, 1464–1470. doi:10.1016/j.bmc.2010.12.057
Tian, Y. T., Zhang, F. G., Nie, J., Cheung, C. W., and Ma, J. A. (2020). Metal‐free decarboxylative annulation of 2‐Aryl‐2‐isocyano‐acetates with aryldiazonium salts: General access to 1, 3‐diaryl‐1, 2, 4‐triazoles. Adv. Synth. Catal. 363, 227–233. doi:10.1002/adsc.202001016
Tian, Y., Yang, W., Yang, R., Zhang, Q., Hao, L., Bian, E., et al. (2022). Ribavirin inhibits the growth and ascites formation of hepatocellular carcinoma through downregulation of type I CARM1 and type II PRMT5. Toxicol. Appl. Pharmacol. 435, 115829. doi:10.1016/j.taap.2021.115829
Tseng, W.-C., Wang, L.-Y., Wu, T.-S., and WonG, F. F. (2011). ‘One-flask’ synthesis to 3, 5-disubstituted 1, 2, 4-triazoles from aldehydes with hydrazonoyl hydrochlorides via 1, 3-dipolar cycloaddition. Tetrahedron 67, 5339–5345. doi:10.1016/j.tet.2011.05.003
Valencia-Quintana, R., Bahena-Ocampo, I. U., González-CastañEDA, G., Bonilla, E., Milić, M., Bonassi, S., et al. (2022). miRNAs: A potentially valuable tool in pesticide toxicology assessment-current experimental and epidemiological data review. Chemosphere 295, 133792. doi:10.1016/j.chemosphere.2022.133792
Van Berkel, S. S., Brauch, S., Gabriel, L., Henze, M., Stark, S., Vasilev, D., et al. (2012). Traceless tosylhydrazone-based triazole formation: A metal-free alternative to strain-promoted azide-alkyne cycloaddition. Angew. Chem. Int. Ed. 51, 5343–5346. doi:10.1002/anie.201108850
Vidavalur, S., Nakka, M., Tadikonda, R., Rayavarapu, S., and Sarakula, P. (2014). A simple and efficient synthesis of 3, 4, 5-trisubstituted/N-fused 1, 2, 4-triazoles via ceric ammonium nitrate catalyzed oxidative cyclization of amidrazones with aldehydes using polyethylene glycol as a recyclable reaction medium. Synthesis 47, 517–525. doi:10.1055/s-0034-1378909
Virant, M., and Kosmrlj, J. (2019). Arylation of click triazoles with diaryliodonium salts. J. Org. Chem. 84, 14030–14044. doi:10.1021/acs.joc.9b02197
Wan, J. P., Cao, S., and Liu, Y. (2015). A metal- and azide-free multicomponent assembly toward regioselective construction of 1, 5-disubstituted 1, 2, 3-triazoles. J. Org. Chem. 80, 9028–9033. doi:10.1021/acs.joc.5b01121
Wang, S. Y. A., Zeng, J.-L., Zheng, Y ., and Ma, J. A. (2015). Silver-catalyzed [3 + 2] cycloaddition of isocyanates to diazo: New regioselectivity yields 1, 4-disubstituted-1, 2, 3-triazoles. Org. Chem. 2, 1468. doi:10.1039/C5QO00219B
Wang, W., Lin, Y., Ma, Y., Tung, C. H., and Xu, Z. (2018). Copper(I)-Catalyzed three-component click/persulfuration cascade: Regioselective synthesis of triazole disulfides. Org. Lett. 20, 2956–2959. doi:10.1021/acs.orglett.8b01002
Wang, W., Wei, F., Ma, Y., Tung, C. H., and Xu, Z. (2016). Copper(I)-Catalyzed three-component click/alkynylation: One-pot synthesis of 5-alkynyl-1, 2, 3-triazoles. Org. Lett. 18, 4158–4161. doi:10.1021/acs.orglett.6b02199
Wei, F., Li, H., Song, C., Ma, Y., Zhou, L., Tung, C. H., et al. (2015). Cu/Pd-Catalyzed, three-component click reaction of azide, alkyne, and aryl halide: one-pot strategy toward trisubstituted triazoles. Org. Lett. 17, 2860–2863. doi:10.1021/acs.orglett.5b01342
Xia, J., Huang, X., and Cai, M. (2019). Heterogeneous copper(I)-Catalyzed cascade addition–oxidative cyclization of nitriles with 2-aminopyridines or amidines: Efficient and practical synthesis of 1, 2, 4-triazoles. Synthesis 51, 2014–2022. doi:10.1055/s-0037-1611712
Yu, X.-Y., Xiao, W.-J., and Chen, J.-R. (2019). Synthesis of trisubstituted 1, 2, 4-triazoles from azlactones and aryldiazonium salts by a cycloaddition/decarboxylation cascade. Eur. J. Org. Chem., 6994–6998. doi:10.1002/ejoc.201901467
Zhang, B. (2019). Comprehensive review on the anti-bacterial activity of 1, 2, 3-triazole hybrids. Eur. J. Med. Chem. 168, 357–372. doi:10.1016/j.ejmech.2019.02.055
Zhang, S., Xu, Z., Gao, C., ReN, Q. C., Chang, L., Lv, Z. S., et al. (2017). Triazole derivatives and their anti-tubercular activity. Eur. J. Med. Chem. 138, 501–513. doi:10.1016/j.ejmech.2017.06.051
Zhang, W., Su, C., Jiang, Y., and Kuang, C. (2009). Synthesis of phenylacetylene containing 1, 2, 3-triazole group. Res. Chem. Intermed. 35, 589–595. doi:10.1007/s11164-009-0065-1
Zhang, Y., Li, X., Li, J., Chen, J., Meng, X., Zhao, M., et al. (2012). CuO-promoted construction ofN-2-aryl- substituted-1,2,3-triazoles viaAzide-chalcone oxidative cycloaddition and post-triazole arylation. Org. Lett. 14, 26–29. doi:10.1021/ol202718d
Keywords: 1,2,3-triazole, 1,2,4-triazole, synthesis method, heterocycle, derivatives
Citation: Dai J, Tian S, Yang X and Liu Z (2022) Synthesis methods of 1,2,3-/1,2,4-triazoles: A review. Front. Chem. 10:891484. doi: 10.3389/fchem.2022.891484
Received: 07 March 2022; Accepted: 18 July 2022;
Published: 26 September 2022.
Edited by:
Xianqing Deng, Jinggangshan University, ChinaReviewed by:
Gopal Chandru Senadi, SRM Institute of Science and Technology, IndiaSimon E. Lopez, University of Florida, United States
Sobhi M. Gomha, Cairo University, Egypt
Copyright © 2022 Dai, Tian, Yang and Liu. This is an open-access article distributed under the terms of the Creative Commons Attribution License (CC BY). The use, distribution or reproduction in other forums is permitted, provided the original author(s) and the copyright owner(s) are credited and that the original publication in this journal is cited, in accordance with accepted academic practice. No use, distribution or reproduction is permitted which does not comply with these terms.
*Correspondence: Zongliang Liu, lzl_0_0@126.com